- 1Department of Environmental Sciences, College of Agriculture and Environmental Sciences, University of South Africa, Roodepoort, South Africa
- 2UNESCO-UNISA Africa Chair in Nanosciences/Nanotechnology, College of Graduate Studies, University of South Africa, Pretoria, South Africa
- 3Institute for Nanotechnology and Water Sustainability, College of Science, Engineering and Technology, University of South Africa, Johannesburg, South Africa
- 4Department of Chemistry, Faculty of Science, University of Kinshasa, Kinshasa, Democratic Republic of Congo
Introduction: The Nubui River is a primary source of water for drinking purposes and other domestic activities in the rural communities dotted along its riparian zone, with agriculture being the major activity occurring in this important ecotone. The river has become a potential sink for agrochemical residue, including heavy metals, and has apparent aesthetic water quality issues, with associated health consequences. This study, therefore, assessed the health risks of heavy metals within the rural populations in the catchment areas, who have limited sources of improved water supply.
Methods: The concentration of iron (Fe), lead (Pb), cadmium (Cd), mercury (Hg), and zinc (Zn) was assessed on cumulatively 275 water samples, using a Perkin Elmer PINAAcle 900 T atomic absorption spectrophotometer for 11 months. A cross-sectional survey was conducted amongst 338 community members, following field observations on utilisation types, aesthetic appeal, and perceived quality of water from the Nubui River. To determine the potential human and ecological risks of heavy metals, the hazard quotient, chronic daily intake, contamination factor, and health pollution indices of heavy metals were computed. STATA version 16 was used to analyse the survey results.
Results: Descriptive statistics of average concentrations of heavy metals in surface water at all sampling stations showed the pattern Hg < Pb < Cd < Zn < Fe, with relatively low concentrations, between 0.001 and 0.004 mg/L for Hg, 0.0011 and 0.0019 mg/L for Pb, 0.0461 and 0.0739 mg/L for Zn, and 0.2409 and 0.377 mg/L for Fe. The findings, however, showed relatively high cadmium levels between 0.0215 mg/L and 0.0383 mg/L in two of five sampling stations in comparison with the World Health Organisation (WHO) drinking water guideline values in some months. Hazard quotient values indicate that the population is safe from the non-carcinogenic health risks of exposure to heavy metals through oral routes. The contamination factor and heavy metal pollution indices for cadmium exceed recommended guideline values of 1 and 100, respectively. Meanwhile, 73.1% of community members evidently preferred the Nubui River for various domestic activities with 86.1% of them utilising it for drinking purposes. This occurrence results in exposure to associated health risks.
1 Introduction
Heavy metals (HMs) are elements with high atomic weights and have a density of five times that of water (Paithankar et al., 2021). HM in the aquatic environment has recently received international attention due to its environmental toxicity, abundance, persistence, and toxicological effects on human health (Cui et al., 2021; Mahabeer and Tekere, 2021; Hao et al., 2022). The source of HM accumulation in surface water is from both natural origin (such as rock weathering and volcanic eruptions) and anthropogenic activities, including industrial processes, agricultural practices, fuel burning, domestic waste, and discharge run-offs (Hoang et al., 2021; Islam et al., 2022). These activities increase the concentration of environmental HMs (Paithankar et al., 2021) characterised by large quantities being discharged into rivers and lakes globally every year because of the rapid global population growth and intensity of these industrial activities (Agarwal et al., 2015; Cui et al., 2021).
Nonetheless, environmental laws governing the release of untreated effluents containing HMs into freshwater in developing nations are not being implemented because there is a lack of both equipment and technical capabilities to detect and monitor water quality, thereby exposing populations to the vagaries of HM pollution (Imran et al., 2019). This obvious challenge has therefore attracted international attention due to the toxicities, persistence, and capacity to infiltrate the food chain (Sharma et al., 2024).
The excessive levels of HMs in rivers have negative consequences for riverine ecosystems and human health, causing widespread concerns (Hao et al., 2022). Although it has been asserted that HMs serve an important role in the human body, excessive exposure can cause health problems (Atangana and Oberholster, 2021). Even though there is a theory that heaviness and toxicity are interrelated, some HMs can induce toxicity even at low levels of exposure (Cui et al., 2021). Furthermore, heavy metals such as cadmium, Mercury, Lead and Chromium have deleterious effects, even in very small quantities and causes acute and chronic toxicities in the human body (Verma and Dwivedi, 2013 Niede and Benbi, 2022). It is for these reasons that the World Health Organization has included HMs such as barium, chromium, cadmium, lead, cobalt, and selenium as chemicals that constitute a substantial public health concern and are described as highly toxic HMs that are not required by organisms even at low doses (Saroop and Tamchos, 2021).
Evidently, a primary concern of long-term exposure to HMs is the accumulation in target tissues within the human body, including the brain, liver, bones, and kidneys, posing major health risks, depending on the element and its chemical form (Mohammadi et al., 2019). To this end, international bodies including the Agency for Toxic Substances and Disease Registry (ATSDR) in Atlanta, Georgia, have classified HMs as a public health concern, with lead being ranked first, followed by mercury (Obiri-Yeboah et al., 2021). Lead is an extremely toxic HM that alters numerous plant and human physiological procedures, animals, and the environment even at very low concentrations (Antoniadis et al., 2020; Aidoo et al., 2021). Mercury is also very toxic and remarkably bioaccumulative, causing neurological disorders, oedema, encephalopathy, and birth defects once transformed into methyl mercury in the human body (Zhao et al., 2022).
In Ghana, agriculture and mining are the main sources of HM pollution in surface water (Fianko et al., 2011). The record of high usage of agrochemicals by farmers in recent years and the unregulated small-scale mining have resulted in the contamination of surface water (Mattah et al., 2015). The residual elements inclusive of HMs have reached an alarming level in open water bodies, leading to a reduction in water quality (Naccarato et al., 2020). For instance, pesticide and herbicide applications, as well as excessive fertilisation, have been discovered to be significant sources of potentially dangerous traces of elements such as lead, mercury, chromium, and arsenic (Atafar et al., 2010). The potential health and environmental implications of HMs on human health require a periodic health risk assessment to understand the emerging carcinogenic and non-carcinogenic risks involved in being exposed to HM, regardless of concentration.
Apart from agriculture and mining activities, previous studies on the ecological risk assessment of HMs in sediments (Leal-Acosta et al., 2022) showed that the HMs in sediments can significantly contribute to the HM concentrations in water due to their release from the sediments to the water column. It therefore reiterates the fact that health risk assessment of HMs in water is essential, particularly in rural populations, because of limited sources of alternate supplies and potential over-dependence on unimproved sources of water. Health risk assessment of drinking water is also important because ingestion and absorption are established to be the most critical exposure pathway (Imran et al., 2019).
Health risk assessments are done by incorporating water quality parameters into mathematical models and computer technology making it easier, faster, and more reliable to employ. Over the years, health risk assessment studies have focused on locations that are highly industrialised, urbanised, ‘overpopulated’, or areas with apparent problems (Qadeer et al., 2020; Luo et al., 2022). This current study, however, focused on rural populations with limited alternate options for water supply. An apparent low concentration of HM is concluded as unproblematic, and typically, tested parameters are compared to the WHO guideline limits or environmental protection standards as the method for assessing the safety of water (Liang et al., 2011; Attiogbe et al., 2020). However, comparing average concentrations with the WHO guideline limits may be insufficiently valid for providing comprehensive hazard levels and exposure. Issues on HMs have grown in importance, necessitating more studies to further assess associated health risks (Imran et al., 2019). In view of this, the current study specifically evaluated the cumulative health risk within rural populations with limited sources of improved water supply. In this study, detectable levels of five HMs were assessed in the water catchments of the Nubui River. Furthermore, the ecological risk and human health risks of HMs were also computed.
2 Materials and methods
2.1 Description of the study area
This study took place in communities living along the Nubui River in Fodome, a traditional area under the Agumatsa sub-district, located in the Hohoe Municipality of the Volta region (Ghana Statistical Service, 2021). This community is located on longitude 60 52′ 0″ North and latitude 00 17′ 0″ and shares a border with Togo on the east. It further shares a border on the southeast with the Afadzato district, southwest with Kpando municipal, and North of Jasikan district. The Fodome traditional area has a total of 14 communities, namely, Agbetsido, Dzorkpe, Fodome Agbesia, Fodome Agorxoe, Fodome Ahor, Fodome Amele, Fodome Ando NO.1, Fodome Ando NO.2, Fodome Dzogbega, Fodome Helu, Fodome Hloma, Fodme Woe, Kodzeto, and Tomegbe. The people of Fodome are mainly Ewes who are multi-religious (Islam, Christianity, and Traditional religion). The population is estimated to be 9,190, with a total of 5,572 men and 5,423 women, with the majority of the inhabitants being farmers (Ghana Statistical Service, 2021). Predominantly, the sources of water for the inhabitants are mechanised boreholes, packaged water, and a stream (Nubui) that passes through some communities in the area. This study was conducted within five sub-communities (Helu, Woe, Hloma, Ahor, and Amele) in Fodome. Communities living less than 100 m from the Nubui River study were selected for sampling as shown in Figure 1. For the purposes of this study, the selection of communities was based on their proximity to the water abstraction points, dependence on the water source, and anthropogenic (agricultural) activities close to the water source.
2.2 Rainfall pattern and vegetation
The annual rainfall total in the communities ranges between 1,100 and 1,500 mm, averaging 1,300 mm. The rainfall pattern is bimodal with two apparent rainy seasons (a major season from April through to July) and (a minor season from September through November). However, these rainfall patterns are becoming more and more unpredictable because of climate change issues (Das et al., 2022). The month with the highest number of rainy days is August (28.00 days), and the month with the lowest number of rainy days is January (5 days).
2.3 Water sample collection
The sampling locations were geo-referenced and plotted on the study site map using a portable Garmin eTrex GPS (Figure 1). Water sampling, transportation, and analysis were done in accordance with the American Public Health Association (APHA) (2005) standard methods. At each sampling point, water samples were collected into 500-mL glass bottles. The bottles had been prewashed with detergents, soaked, and washed in 10% nitric acid for 24 h. It was then rinsed thoroughly with double-distilled water and oven-dried at 90°C–95°C (APHA, 2005 Boadi et al., 2020). Sample bottles were carefully labelled with sample locations and dates.
At each sampling location, sample bottles were pre-rinsed with surface water samples before collection. Surface water samples were collected at the pelagic zone, from each drinking source point in each community, kept on ice and immediately transported to the laboratory, and stored at 4°C till analysis. All water samples collected for dissolved metal analysis were filtered through a 0.45-μm filter on site, whereas those for total recoverable metal analysis were not filtered. The samples were then acidified with 5 mL of nitric acid (HNO3) to a pH below 2.0.
2.4 Laboratory analysis for concentrations of HMs in water
An HM analysis was performed using powder pillows and a flame atomic absorption spectrophotometer as prescribed by the ASTM standard protocols. Prior to the analysis, the water sample was digested by measuring 50 mL aliquot of water sample into a beaker and 2 mL of concentrated HNO3 and 5 mL of concentrated HCl were added. The mixture was then heated on a hot plate at 90°C–95°C (to almost evaporate) until the volume was reduced to 15–20 mL. The beaker was removed from the hot plate and allowed to cool. The walls of the beaker were rinsed with distilled water and transferred into a 50-mL volumetric flask and the final volume adjusted to the 50-mL mark. The concentration of HMs was measured using a Perkin Elmer PINAAcle 900 T atomic absorption spectrophotometer for total recoverable metals.
To ensure the accuracy of the analysis, quality assurance (QA) and quality control (QC) were strictly implemented using the USEPA guidelines in the Laboratory. All the reagents used were of analytical grade, and deionised water was used in all analytical procedures. Plastic and glass wares were soaked in 10% HNO3 for 24 h, rinsed with distilled water, and oven-dried overnight before use. Each sample was analysed in triplicates, and two standards were tested after every 10 samples to check for interference and cross-contamination. Minimum detection limits (MDLs) of HMs were established, aiding in analysis and reporting with 99% confidence intervals, meaning the smallest amount of a substance is reliably detected. The standard solution for each HM parameter was prepared and used to calibrate the system before analysing each water sample. A blank was run for each digestion procedure to correct the measurement.
2.5 Health risk assessment
The hazard quotient (HQ) and chronic daily intake (CDI) were applied to evaluate the human health risks due to exposure to HMs, based on the risk-based concentration, shown in Supplementary Tables S1, S2. Degree of contamination, contamination factor, and heavy metal pollution indices were also applied to assess the ecological risk of HMs in the Nubui River. Details on parameters and procedures are detailed in Supplementary material.
2.6 Field observation, cross-sectional survey on water preference, and utilisation
Field observations were carried out using a checklist to assess the utilisation types, alternate sources of water, and types of agrochemicals used within riparian zones. A cross-sectional survey was also conducted amongst the population on utilisation preference of the Nubui River and other alternate sources of water. This was done using a pretested and validated questionnaire amongst an estimated sample size of 338 participants.
2.7 Statistical analysis
The IBM SPSS software (version 22) was used in the statistical analysis for this study. The correlations between the synergistic contamination levels and HM concentrations were analysed at a confidence level of p < 0.05. HM concentrations were analysed using a two-way analysis of Variance (ANOVA) to assess significant differences amongst the sampling points and seasons. Health risk calculations were performed using Microsoft Excel. Further representation of the metal concentration and hazard indices were tabulated and plotted using Microsoft Excel and Word.
3 Results
3.1 Mean heavy metal content of water samples and spatiotemporal variation
3.1.1 Heavy metal concentrations
Figure 2 shows the dissolved HM content of water samples collected from the Nubui River. Water samples collected from Ahor, Amle, Helu, Woe, and Hloma communities recorded a similar HM concentration pattern of Hg < Pb < Cd < Zn < Fe, with iron having the highest concentration. Throughout the sampling seasons, mercury concentrations remained low and within the WHO drinking water quality guidelines of 0.006 mg/L in all the communities. Lead recorded mean values ranging from 0.001 to 0.022 mg/L, whereas for cadmium, mean values ranged between 0.02 and 0.035 mg/L, and for zinc, the mean values ranged from 0.042 to 0.067 mg/L.
3.1.2 Spatiotemporal variation
As shown in Supplementary Table S8, spatiotemporal variation indicates that lead concentrations were evidently high between the months of May, July, and September, with the Hloma community recording 0.22 mg/L in May and the Woe community measuring 0.18 mg/L also in May. In July, the recorded lead concentration was 0.01 mg/L and increased in the Amele community to 0.02 mg/L in July and August.
In the Ahor community, cadmium concentration was high in March, April, July, and October with concentration values of 0.77, 0.15, 0.003, and 0.01 mg/L, respectively (Supplementary Tables S8–S11). This was marked by peak rainfall in April–July and pockets of rain in the dry season. However, in the Amele community, cadmium concentration was high in March and April, recording 0.24 mg/L and 0.08 mg/L, respectively. Cadmium concentration remained consistently high in March (0.093 mg/L), April (0.117), through September (0.004) to December (0.008 mg/L), in the Helu community.
In contrast, iron concentration was high between March in the Hloma community (0.70 mg/L) and the Amele community (0.75 mg/L), decreased in May and June, and peaked between July (0.3 mg/L) and September (1.09 in Hloma), and between November (0.5 mg/L in Woe) and January (0.53 mg/L in Helu), throughout the sampling season (Table 1). In Supplementary Table S11, zinc concentration remained low throughout the sampling season, recording values between 0.11 mg/L in the Ahor community and 0.25 mg/L in the Amele community, hence displaying distinct differences in peak rainy seasons and dry seasons. Similarly in September, 0.316 mg/L was recorded in Ahor and increased to 0.43 mg/L in Amele. It is evident that between August and September, significantly higher concentrations were recorded than between October and February, which recorded low concentrations between 0.00 mg/L and 0.01 mg/L, respectively.
As shown in Supplementary Tables S3–S7, a statistical analysis of HMs throughout the sampling seasons recorded a weak positive correlation (r = 0.0797 and p = 0.038) in concentrations within months 3 and 2. In addition, a very weak negative correlation (r = −0.0797 and p = 0.039) was also recorded between months 4 and 3. The correlation between lead concentrations in months 7 and 3 also shows a very weak negative correlation (−0.07968 and a p = 0.038), whilst a very weak negative correlation was recorded in months 8 and 3 with a correlation coefficient of −0.0795 and p = 0.039 (Table 1). Meanwhile, cadmium concentration showed a significant negative correlation for all pairs of measurements (p < 0.05), with varying strengths of correlation from weak to moderate and coefficients ranging from −0.023 to −0.123. The strongest negative correlation (r = −0.123, p < 0.001) was observed between months 1 and 9. Iron recorded a strong negative correlation (r = −0.29372, p = 0.211) between months 2 and 3 and a weak negative correlation (r = −0.00028, p = 1.000) between months 4 and 3 (Table 2). There was a moderate positive correlation between periods 5 and 4 (r = 0.0772, p = 1.000) and a strong positive correlation (r = 0.396, p = 0.011) between months 6 and 5. Finally, zinc concentration showed weak negative to positive correlations with each other, but no significant correlations with other months during the sampling period.
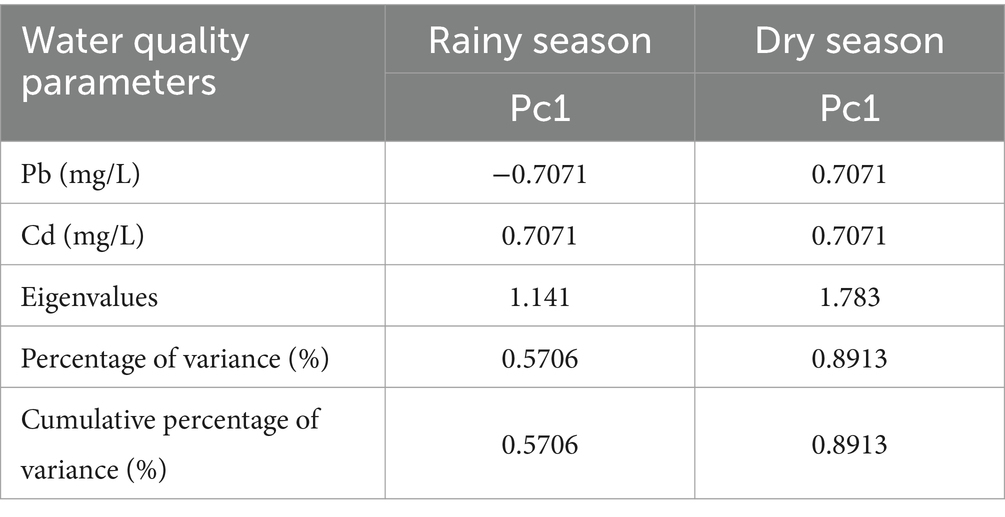
Table 2. Surface river water quality variables (heavy metals) on rotated Pcs for datasets during the dry and wet seasons.
3.1.3 Principal composites analysis
Principal component analysis (PCA) is a widely used statistical technique that summarises many variables to a few important scores (components) to identify possible sources of pollutants and extract the eigenvalues and eigenvectors from the correlation matrix. PCA of 0.5/6 and above is significant be it positive or negative, indicating a strong correlation.
The percentage of variance shows the proportion of total variance that each principal component indicates in the rainy season. Pc1 explains 0.5706 of the total variance, and in the dry season, Pc1 explains approximately 0.8913 of the total variance. This means Pc1 is quite important in understanding the overall variability in the water quality parameters. The cumulative percentage of variance shows the accumulated contribution of each principal component. In this case, Pc1 alone captures all the variance in both seasons as the cumulative percentage matches the percentage of variance (Table 2) for Pc1. Based on these results, Pc1 is the most significant component in both seasons when it comes to explaining the water quality parameters. The positive values for Pb (0.7071) and Cd (0.7071) in Pc1 indicate a positive correlation between these pollutants and the overall water quality parameter. This means that when Pb and Cd levels increase, it suggests a potential decrease in water quality in the rainy months or season.
3.2 Health risk assessment: chronic daily intake and hazard quotient
Table 3 displays the health and ecological risks of the HM content (lead, cadmium, iron, mercury, and zinc) of the Nubui River. The health risk of lead, cadmium, iron, mercury, and zinc in Nubui was assessed by applying the models CDI and HQ. These models assess the oral exposure pathway of HM in water in each sampling site. The result indicates that CDI for both adults and children were below the threshold of 1 with iron recording the highest value. Similarly, HQ values of lead, cadmium, iron, mercury, and zinc were also below the threshold of 1 throughout all study sites with Cd having the highest value. Therefore, CDI and HQ values indicate that both children and adults are not exposed to non-carcinogenic health risks because of the utilisation of the Nubui River water for drinking purposes.
3.2.1 Contamination factor
Figure 3 shows the contamination factor (Cf) of HMs in the Nubui River sampled in the Helu, Hloma, Woe, Ahor, and Amle communities. The results revealed that the mean concentration levels of individual HMs were in the order Hg < Pb < Cd < Zn < Fe with the mean CF values ranging between 0.00 and 8.38 in Helu, 0.00 and 7.5 in Hloma, 0.8 and 7.1 in Woe, 0.00 and 8.6 in Ahor, and 1.09 and 12.7 in Amle. Lead recorded moderate contamination factors of 1.9 and 2.4 in the Woe and Hloma communities, respectively. In addition, cadmium recorded a very high contamination factor in all sites, with values between 7.17 and 12.7. Meanwhile, iron, zinc, and mercury recorded a contamination factor of less than 1.
3.2.2 Heavy metal pollution indices
Heavy metal pollution indices of Nubui River (HPI > 100) indicate that the level of HM pollution in a water body exceeds its maximum acceptable level. As shown in Table 4, the HPI overall values of metals throughout the study sites appeared to exceed 100 with the Helu, Hloma, Woe, Ahor, and Amle communities having HPI values of 434.69, 434.711, 391.66, 441.797, and 657.021, respectively. The result also revealed that the overall values were impacted by the HPI values of cadmium recorded in Helu (429.4), Hloma (397.5), Woe (363.8), Ahor (439.6), and Amle (647.9) indicating cadmium pollution in the river (Supplementary Figure S1). Meanwhile, HPI levels of mercury, iron, and lead were less than 100 throughout the sampling sites, with zinc recording zero, an indication that these HMs were not individually polluting the Nubui River. However, this was different when a cumulative test of all target metals was considered. The cumulative test of all target metals that HPI overall values exceeded the threshold of 100.
3.3 Field observation and cross-sectional survey on water preference and utilisation
3.3.1 Sociodemographic characteristics
Table 5 shows the demographic characteristics of participants in the study. A total sample of 338 participants were included in this survey. The mean age and standard deviation of the participants was 41.7 ± (15.1) years old. The highest number of participants (31.9%) in this survey had an age of 50+ years old, followed by those between 30 and 39 years old (27.5%). Participants of ages between 40 and 49 years old had the lowest participation percentage (17.25). For gender, it was noted that women had the highest participation percentage of 62.6% when compared to men. Moreover, almost all participants had formal education (91.45) and most of them had an educational level of junior high school (44.7%) and only 9.4% had tertiary education. Most participants interviewed during this study were employed apart from 14.2% who were unemployed. Amongst those employed, the majority were self-employed (41.15) and farmers or farm workers (37%).
3.3.2 Water preference and utilisation
From Table 6, most of the participants preferred Nubui water, and 247 (73.1%) and 85 (25.1%) preferred water from the borehole, whereas 1.5% preferred rainwater and 0.3% preferred pure water (Supplementary Figures S2, S3). This preference could be due to accessibility and cost issues. When it came to utilisation, most of the participants used water from the Nubui River for drinking purposes (86.1%), laundry (93.2%), and cooking (87.3%).
4 Discussion
4.1 Health risk of HMs in water
HMs are given a lot of attention because of their non-biodegradable, persistent, biological toxic properties, and associated health risks (Cui et al., 2021). Even if the concentration is extremely low, HMs will have a significant long-term impact on human health (Hao et al., 2022) and the quality of the water environment (Wang et al., 2021). According to Mohammadi et al. (2019), human life depends on the availability of clean drinking water, and safe drinking water should not pose a significant risk to human health.
Given the findings from the field observations, HM exposure through a dermal and oral route is possible. As reiterated by Hong et al. (2020), the main exposure pathways from surface water to humans are through water, food, and dermal contact whilst swimming. Throughout the sampling seasons, the average concentrations of mercury, cadmium, iron, zinc, and lead detected in surface water in all sampling points (Figure 1) are presented in Figure 2. HMs recorded relatively low concentrations in comparison with WHO guideline values apart from cadmium, which recorded high concentrations of 0.0243, 0.022, and 0.022 mg/L, in the Woe, Hloma, Ahor, and Amele communities, respectively. Reconnaissance surveys and field observations confirmed the use of phosphate fertilisers and possible misapplication on farms within 50 m of the riparian zone, contributing to the high levels of cadmium residue in the surface water source. Typically, the main sources of cadmium in water include agrochemical products such as pesticides and herbicides. These chemicals are released into the environment through the manufacture and use of phosphate fertilisers, as these contain significant quantities of cadmium, lead, and zinc (Kladsomboon et al., 2020). Meanwhile, to overcome low yields and increase productivity, farmers depend on agrochemicals such as fertilisers and pesticides. The desire for increased production has led to an all-time high usage of agrochemicals in recent years (Mattah et al., 2015). This emphasises the issue of misapplication of agrochemicals amongst farmers, which calls for the intensification of education on agrochemical handling and usage.
HQ values of lead, cadmium, iron, mercury, and zinc were less than 1, in the Helu, Hloma, Woe, Ahor, and Amele communities, an indication that there is no serious non-carcinogenic health risk amongst adults and children who utilise the Nubui River for drinking purposes. However, field observations indicate possible risk through dermal and oral exposures in children as they were observed swimming during daily errands of fetching water or doing laundry. In the Hloma community, lead exceeded drinking water guideline values at 0.0243 mg/L, but permissible levels of average HMs were detected in the Woe and Ahor communities. Regardless, industrial activities are notably non-existent in the study communities and therefore health effects of HMs such as injury to the central nervous system, as observed by Fernandes Azevedo et al. (2012), are least expected to be health issues to plague an already vulnerable community via this drinking water.
Finally, it is also worth noting that children are the most vulnerable to HMs if the CDI or HQ levels are high because they were observed to be most likely to have multiple exposures through incidental ingestion during swimming. This only means children have weaker immune function and are more sensitive to HM pollution (Nguyen et al., 2020). In Ghana, though HM contamination in water is potentially problematic in agricultural communities, the Water Resources Commission (WRC) reports that 60% of Ghana’s water bodies are polluted with many in critical conditions, including the Ankobra River and River Offin (Nunoo et al., 2022) and Oda and Pra River (Nti et al., 2023). Others such as the Lake Amponsah record alarming levels of mercury due to small-scale mining activities around the lake, with an unusually high concentration of Arsenic from geogenic sources in Bibiani (Attiogbe et al., 2020). Increased levels of HMs in the sediments of the River Subri, Birim River, Nyam River, and Bonsa River dissolve into water columns due to mining activities, whilst they are also important sources of freshwater for many communities (Hadzi et al., 2024). Consequently, this situation has raised human rights concerns, as the Commission of Human Rights and Administrative Justice (CHRAJ) reports that many water resources in mining communities have been destroyed, polluted, or dried up, limiting access to safe drinking water, amongst already vulnerable rural populations (Owusu-Prempeh et al., 2022). Fortunately, the riparian region of the Nubui River is devoid of such industrial activities and hence attributed to the low HM concentrations recorded so far; otherwise, the consequence would have been dire because the Nubui River is the main source of water for the rural communities.
The detected average HM concentration exhibited varying levels of spatiotemporal characteristics; in the peak rainy seasons, between May and September, the concentration was observed to increase following heavy rains and reduced when the ‘dry’ season started. This phenomenon is observed in previous studies where the concentration of metals in wet seasons was high and attributed to the dissolution of rocks and increased runoff (Abdelhafiz et al., 2021), exposing surface water to non-point sources of pollution. Zhang et al. (2021) also reported that HMs recorded the highest concentrations during summer along with intense local agriculture. Lead concentration was high during the peak wet seasons between May and August in all sampling sites. Again, the cadmium level was evidently high in March and April, a dry season characterised by pockets of rainfall events. This coincided with the results of the study in Hao et al. (2022) whereby Cd and Zn concentration was minimum in autumn and high in summer.
However, wet and dry seasons in the study area were not clearly defined during the sampling period, as the wet seasons were interspersed with pockets of dry spells, which influenced the concentrations of metals at each sampling time. So basically, rainfall events influenced the pattern of results during the different sampling times. Climate change is a major contributor to having undefined seasons; long-term climate changes, resulting in changing rainfall patterns, characterise shifts in stresses that affect water resource availability and quality (Nijhawan and Howard, 2022) and pose serious threats to drinking water quality and health.
4.2 Ecological risk
Contamination factor of the individual HMs over the 11-month sampling period as reported indicates contamination risk from cadmium levels recorded in all sampling sites between 7.17 and 12.7 (Figure 3), with lead recording moderate contamination factors of 1.9 and 2.4 in the Woe and Hloma communities, respectively. The contamination factor of cadmium was high because average concentrations were considerably high in surface water compared to the expected low WHO permissible standards as also reported by Hoang et al. (2021) in the Houjing River in China. The implication is that water from the Nubui River showed signs of contamination, unsafe and potentially posed human health risks through the main exposure pathways, based on CF values.
HPI values for HMs in each sample site show the HPI levels of mercury, lead, iron, and zinc do not exceed the critical metal pollution index of 100 except for cadmium. The HPI of cadmium levels recorded in water on all sites are consistently high and contributed to the overall pollution indices of the Nubui River, thereby posing major risks to the environment and potentially to human health. It is observed that the results of the HPI values and contamination factor aligned, where cadmium was the highest contributor to the overall pollution indices. This is also observed in Anyanwu et al. (2020), where it was explained that the alignment of both heavy metal pollution indices and contamination factor reflected the possible effects of geogenic influence exacerbated by season and human activities in the river. This reportedly was confirmed by Nguyen et al. (2020) whereby HPI values of HMs increase in drier seasons compared to rainy seasons.
Similar to the Nubui River, the Nile River in Egypt is utilised for drinking purposes and yet recorded HPI values exceeding critical metal pollution of 100 as reported by Abdel-Satar et al. (2017), thus making the Nile water critically contaminated with HMs. The heavy metal pollution index and alignment of contamination index suggested that geogenic influence has been exacerbated by seasonal variations and human activity in the river. The elevated levels of cadmium in the Nubui River,contributed a lot to the overall metal indices, making it questionable for drinking purposes.
To highlight the seriousness of metal pollution, Setia et al. (2020) reported on the high HPI levels in the Sutlej River in the South-western parts of Punjab, India. Meanwhile, this surface water source is used for drinking purposes. Consequently, survey data reported higher cancer incidences within the south-west Punjab regions than in central and north-east Punjab and this was linked to the drinking of critically polluted water with HMs (Setia et al., 2020).
4.3 Water preference and utilisation
Notwithstanding the aesthetic quality of the Nubui River and the health risks potentially posed by HMs, the majority of rural community members preferred the Nubui River compared to other sources such as boreholes, rainwater, and pure water. Aesthetic issues, as indicated by previous studies, include offensive taste, odour, colour corrosion, and staining problems, which are contributed by the high iron concentrations (Ochoo et al., 2017; Dave and Yang, 2022). Nubui River was utilised for various domestic purposes, such as washing, cooking, laundry, and other recreational purposes like swimming, whereas 86% of the community members preferred it for drinking purposes, regardless of other improved sources available. For typical rural communities with limited sources of water, it is expected to observe over-dependence on these limited supplies, even from unimproved sources (Eticha et al., 2022). Hence, upon comparing the dependence on various sources of available water, the majority preferred water from the Nubui River, followed by a borehole and finally pure water. This corroborated with the report by the Joint Monitoring Programme in 2019, which conclusively showed that people who live in rural areas are the most vulnerable population, with eight of 10 people lacking access to clean water; several previous studies also mentioned socioeconomic status, having an explicit relationship with unequal access to improved drinking water (Cassivi et al., 2019). Therefore, depending on the utilisation type, continuous monitoring of health risks for various exposure routes are important parameter in every health risk study.
Several reports have shown that surface sources are used for drinking in most low- and middle-income countries (Debnath et al., 2022; Chathuranika et al., 2023), which is consistent with this study. It must however be noted that the quality of surface water is impacted significantly by anthropogenic activities and compromises the quality of the water by having nutrients, HMs, and microbes, compared to the WHO standards (Rad Fard et al., 2019). Water for drinking most often should not contain contaminants, which would pose a health risk to the consumer (Haldar et al., 2022). The safety of the Nubui River for drinking is questionable, and there is a need for treatment with an overarching aim of protecting consumer health and making it aesthetically quality. However, the treatment of raw water requires complex processes depending on various factors, such as the source of the water. Raw water may contain pollutants that need to be removed before it is safe for drinking (Njewa and Shikuku, 2023).
A good number of rural communities prefer the Nubui River, regardless of quality. It is important to investigate and understand the reasons, although a previous study highlights socioeconomic reasons as playing a major role. Moreover, few studies have, however, reported a relationship between rural people and their environment, including water resources, and this relationship is deeply rooted in sociocultural values and norms (Wasonga et al., 2016). Therefore, it would be important to investigate the sociocultural influence of water use in rural communities.
5 Conclusion and recommendations
This study shows that average HM levels were low in the Nubui River throughout the sampling period, and exhibited minimal spatial variations. The apparent low concentration of most HM is an indication of the little environmental changes in the community, but the agricultural riparian activities threaten a long-term risk, for vulnerable communities with limited alternate sources of drinking water. Ecological risk of cadmium was significant with a high contamination factor greater than 1 and HPI values greater than 100 and contributed by high average concentrations, exposing community members to associated health risks.
The Nubui River is a major source of drinking water for rural communities. Therefore, it is important to protect this basic resource by enforcing a riparian buffer zone policy through local stakeholder engagement and community-based interventions. Meanwhile, rural populations should be properly educated on sustainable resource use through sensitisation projects, community-based interventions, and training programmes. Specifically, farmers need to be properly educated by agricultural extension officers to avoid the misapplication of agrochemicals, which results in water contamination through surface runoff. Additionally, observing the mode of applications and disregard for the use of personal protective equipment by farmers necessitate future research on occupational toxic exposures from agrochemicals in rural communities. Research into self-reported toxicity symptoms and agrochemical waste disposal is recommended. This would be a relevant baseline study and useful to prevent serious health risks to farmers and their households.
With an understanding from previous literature, which indicates that the relationship between people and their environmental resources is deeply rooted in culture, exploring the sociocultural influences of resource use in remote rural communities would be relevant. This is because other studies have reported on sociocultural issues and barriers influencing rural people’s acceptability of scientific interventions.
6 Limitations
From field observations, it is evident that the health risks through dermal exposure would be significant in children. However, that was not the initial focus of the study. Due to ethical issues, field observations and pictorial evidence cannot be fully reported in this paper, even though that information would have been relevant. Additionally, some sensitive pictures could not be taken during data collection for the same ethical reasons.
Data availability statement
The original contributions presented in the study are included in the article/Supplementary material, further inquiries can be directed to the corresponding authors.
Ethics statement
Written informed consent was obtained from the individual(s) for the publication of any potentially identifiable images or data included in this article.
Author contributions
FN: Writing – review & editing, Writing – original draft, Visualization, Validation, Software, Methodology, Investigation, Formal analysis, Data curation. SA: Writing – review & editing, Validation, Supervision, Resources, Project administration, Funding acquisition, Conceptualization. XF: Writing – review & editing. EA: Writing – review & editing, Formal analysis. AI: Writing – review & editing, Data curation. LS: Resources, Writing – review & editing. MM: Writing – review & editing, Resources. IK: Writing – review & editing, Supervision, Software, Resources, Project administration, Funding acquisition, Formal analysis, Conceptualization.
Funding
The author(s) declare that financial support was received for the research, authorship, and/or publication of this article. This work was supported by UNISA Postgraduate Bursaries and the South African Coal, Oil, and Gas Corporation-National Research Foundation (SASOL-NRF) University Collaborative Research Grants (Grant No. 138626), Reference (SASOL800405).
Conflict of interest
The authors declare that the research was conducted in the absence of any commercial or financial relationships that could be construed as a potential conflict of interest.
Publisher’s note
All claims expressed in this article are solely those of the authors and do not necessarily represent those of their affiliated organizations, or those of the publisher, the editors and the reviewers. Any product that may be evaluated in this article, or claim that may be made by its manufacturer, is not guaranteed or endorsed by the publisher.
Supplementary material
The Supplementary material for this article can be found online at: https://www.frontiersin.org/articles/10.3389/frwa.2024.1397853/full#supplementary-material
References
Abdelhafiz, M. A., Elnazer, A. A., Mostafa, A., Salman, A. G. A., and Xinbin, A. S. (2021). Chemical and bacterial quality monitoring of the Nile River water and associated health risks in Qena–Sohag sector, Egypt. Environ. Geochem. Health 43, 4089–4104. doi: 10.1007/s10653-021-00893-3
Abdel-Satar, A. M., Ali, M. H., and Goher, M. E. (2017). Indices of water quality and metal pollution of Nile River, Egypt. Egypt. J. Aquat. Res. 43, 21–29. doi: 10.1016/j.ejar.2016.12.006
Agarwal, A., Prajapati, R., Singh, O. P., Raza, S. K., and Thakur, L. K. (2015). Pesticide residue in water—a challenging task in India. Environ. Monit. Assess. 187:54. doi: 10.1007/s10661-015-4287-y
Aidoo, E. N., Appiah, S. K., Awashie, G. E., Boateng, A., and Darko, G. (2021). Geographically weighted principal component analysis for characterising the spatial heterogeneity and connectivity of soil heavy metals in Kumasi, Ghana. Heliyon 7:e08039. doi: 10.1016/j.heliyon.2021.e08039
American Public Health Association (APHA). (2005). Standard methods for the examination of Water and Wastewater. 19th ed. Washington, DC: American Public Health Association (APHA).
Antoniadis, V., Shaheen, S. M., Levizou, E., Shahid, M., Niazi, N. K., Vithanage, M., et al. (2020). A critical prospective analysis of the potential toxicity of trace element regulation limits in soils worldwide: are they protective concerning health risk assessment?—a review. Environ. Int. 127, 819–847. doi: 10.1016/j.envint.2019.03.039
Anyanwu, E. D., Adetunji, O. G., and Nwachukwu, E. D. (2020). Application of pollution indices and health risk assessment of heavy metals in the waters of a South-Eastern Nigeria river. Pollution 6, 909–922. doi: 10.22059/poll.2020.303140.820
Atafar, Z., Mesdaghinia, A., Nouri, J., Homaee, M., Yunesian, M., Ahmadimoghaddam, M., et al. (2010). Effect of fertilizer application on soil heavy metal concentration. Environ. Monit. Assess. 160, 83–89. doi: 10.1007/s10661-008-0659-x
Atangana, E., and Oberholster, P. J. (2021). Using heavy metal pollution indices to assess water quality of surface and groundwater on catchment levels in South Africa. J. African Earth Sci. 182:104254. doi: 10.1016/j.jafrearsci.2021.104254
Attiogbe, F. K., Mohammed, A. R., and Kingslove, Q. (2020). Assessing the potential health impact of selected heavy metals that pollute lake Amponsah in Bibiani, Western north region, Ghana. Scientific African 9:e00531. doi: 10.1016/j.sciaf.2020.e00531
Boadi, N. O., Saah, S. A., Baa-Poku, F., Mensah, E. A., and Addo, M. (2020). Safety of borehole water as an alternative drinking water source. Scientific African, 10:e00657. doi: 10.1016/j.sciaf.2020.e00657
Cassivi, A., Guilherme, S., Bain, R., Tilley, E., Waygood, E. O. D., and Dorea, C. (2019). Drinking water accessibility and quantity in low and middle-income countries: a systematic review. Int. J. Hyg. Environ. Health 222, 1011–1020. doi: 10.1016/j.ijheh.2019.06.011
Chathuranika, I. M., Sachinthanie, E., Zam, P., Gunathilake, M. B., Denkar, D., Muttil, N., et al. (2023). Assessing the water quality and status of water resources in urban and rural areas of Bhutan. J Hazard Mater Adv 12:100377. doi: 10.1016/j.hazadv.2023.100377
Cui, L., Wang, X., Li, J., Gao, X., Zhang, J., and Liu, Z. (2021). Ecological and health risk assessments and water quality criteria of heavy metals in the Haihe River. Environ. Pollut. 290:117971. doi: 10.1016/j.envpol.2021.117971
Das, S., Kamruzzaman, M., Reza, A., and Islam, T. (2022). Assessment of characteristic changes of regional estimation of extreme rainfall under climate change: a case study in a tropical monsoon region with the climate projections from CMIP6 model. J. Hydrol. 610:128002. doi: 10.1016/j.jhydrol.2022.128002
Dave, D. M., and Yang, M. (2022). Lead in drinking water and birth outcomes: a tale of two water treatment plants. J. Health Econ. 84:102644. doi: 10.1016/j.jhealeco.2022.102644
Debnath, P., Mamun, M. M. A., Karmakar, S., Uddin, M. S., and Nath, T. K. (2022). Drinking water quality of Chattogram city in Bangladesh: an analytical and residents’ perception study. Heliyon 8:e12247. doi: 10.1016/j.heliyon.2022.e12247
Eticha, M., Geremew, A., Dirirsa, G., Bayu, K., Girma, H., and Mengistu, D. A. (2022). Household water treatment practice and associated factors among households dependent on unimproved water sources in Ameya district, Oromia, Ethiopia. J Water Sanit Hyg Dev 12, 432–442. doi: 10.2166/washdev.2022.034
Fernandes Azevedo, B., Barros Furieri, L., Peçanha, F. M., Wiggers, G. A., Frizera Vassallo, P., Ronacher Simões, M., et al. (2012). Toxic effects of mercury on the cardiovascular and central nervous systems. Bio Med Res Int 2012:9048. doi: 10.1155/2012/949048
Fianko, J. R., Donkor, A., Lowor, S. T., and Yeboah, P. O. (2011). Agrochemicals and the Ghanaian environment, a review. J. Environ. Prot. 2, 221–230. doi: 10.4236/jep.2011.23026
Ghana Statistical Service. (2021). 2021 population and housing census: national analytical report. Ghana Statistics Service. Available at: https://census2021.statsghana.gov.gh/
Hadzi, G. Y., Essumang, D. K., and Ayoko, G. A. (2024). Assessment of contamination and potential ecological risks of heavy metals in riverine sediments from gold mining and pristine areas in Ghana. J Trace Elem Minerals 7:100109. doi: 10.1016/j.jtemin.2023.100109
Haldar, K., Kujawa-Roeleveld, K., Hofstra, N., Datta, D. K., and Rijnaarts, H. (2022). Microbial contamination in surface water and potential health risks for peri-urban farmers of the Bengal delta. Int. J. Hyg. Environ. Health 244:114002. doi: 10.1016/j.ijheh.2022.114002
Hao, M., Zuo, Q., Li, J., Shi, S., Li, B., and Zhao, X. (2022). A comprehensive exploration on distribution, risk assessment, and source quantification of heavy metals in the multi-media environment from Shaying River basin, China. Ecotoxicol. Environ. Saf. 231:113190. doi: 10.1016/j.ecoenv.2022.113190
Hoang, H. G., Chiang, C. F., Lin, C., Wu, C. Y., Lee, C. W., Cheruiyot, N. K., et al. (2021). Human health risk simulation and assessment of heavy metal contamination in a river affected by industrial activities. Environ. Pollut. 285:117414. doi: 10.1016/j.envpol.2021.117414
Hong, Z., Zhao, Q., Chang, J., Peng, L., Wang, S., Hong, Y., et al. (2020). Evaluation of water quality and heavy metals in wetlands along the yellow river in Henan province. Sustainability 12, 1–19. doi: 10.3390/su12041300
Imran, U., Ullah, A., Shaikh, K., Mehmood, R., and Saeed, M. (2019). Health risk assessment of the exposure of heavy metal contamination in surface water of lower Sindh, Pakistan. SN Appl Sci 1, 1–10. doi: 10.1007/s42452-019-0594-1
Islam, M. S., Ismail, Z., Jamal, M. H., Ibrahim, Z., Jumain, M., Islam, A. R. M. T., et al. (2022). Heavy metals from different land use soil in the capital of ancient Pundranagar, Bangladesh: a preliminary study for ecological risk assessment. Chem. Ecol. 38, 720–743. doi: 10.1080/02757540.2022.2100360
Kladsomboon, S., Jaiyen, C., Choprathumma, C., Tusai, T., and Apilux, A. (2020). Heavy metals contamination in soil, surface water, crops, and resident blood in Uthai District, Phra Nakhon Si Ayutthaya, Thailand. Environ. Geochem. Health 42, 545–561. doi: 10.1007/s10653-019-00388-2
Leal-Acosta, M. L., de Jesús Bastidas-Bastidas, P., Cruz-Acevedo, E., Aguilar-Jiménez, E. E., Perea-Domínguez, X. P., Martínez-Álvarezd, I. G., et al. (2022). Pesticides in water and sediments of Chacahua-Pastoria lagoon system, Oaxaca, Mexico. Mar. Pollut. Bull. 174:113177. doi: 10.1016/j.marpolbul.2021.113177
Liang, N., Yang, L., Dai, J., and Pang, X. (2011). Heavy metal pollution in surface water of Linglong gold mining area, China. Procedia Environ. Sci. 10, 914–917. doi: 10.1016/j.proenv.2011.09.146
Luo, H., Wang, Q., Guan, Q., Ma, Y., Ni, F., Yang, E., et al. (2022). Heavy metal pollution levels, source apportionment and risk assessment in dust storms in key cities in Northwest China. J. Hazard. Mater. 422:126878. doi: 10.1016/j.jhazmat.2021.126878
Mahabeer, P., and Tekere, M. (2021). Anthropogenic pollution influences on the physical and chemical quality of water and sediments of the Umdloti river system, Kwazulu-Natal. Phys. Chem. Earth 123:103030. doi: 10.1016/j.pce.2021.103030
Mattah, M. M., Mattah, P. A. D., and Futagbi, G. (2015). Pesticide application among farmers in the catchment of Ashaiman irrigation scheme of Ghana: health implications. J. Environ. Public Health 2015:547272, 1–7. doi: 10.1155/2015/547272
Mohammadi, A. A., Zarei, A., Majidi, S., Ghaderpoury, A., Hashempour, Y., Saghi, M. H., et al. (2019). Carcinogenic and non-carcinogenic health risk assessment of heavy metals in drinking water of Khorramabad, Iran. Methods X 6, 1642–1651. doi: 10.1016/j.mex.2019.07.017
Naccarato, A., Tassone, A., Cavaliere, F., Elliani, R., Pirrone, N., Sprovieri, F., et al. (2020). Agrochemical treatments as a source of heavy metals and rare earth elements in agricultural soils and bioaccumulation in ground beetles. Sci. Total Environ. 749:141438. doi: 10.1016/j.scitotenv.2020.141438
Nguyen, B. T., Do, D. D., Nguyen, T. X., Nguyen, V. N., Phuc Nguyen, D. T., Nguyen, M. H., et al. (2020). Seasonal, spatial variation, and pollution sources of heavy metals in the sediment of the Saigon River, Vietnam. Environ. Pollut. 256:113412. doi: 10.1016/j.envpol.2019.113412
Niede, R., and Benbi, D. K. (2022). Integrated review of the nexus between toxic elements in the environment and human health. AIMS Public Health. 9, 758–789. doi: 10.3934/publichealth.2022052
Nijhawan, A., and Howard, G. (2022). Associations between climate variables and water quality in low-and middle-income countries: a scoping review. Water Res. 210:117996. doi: 10.1016/j.watres.2021.117996
Njewa, J. B., and Shikuku, V. O. (2023). Recent advances and issues in the application of activated carbon for water treatment in Africa: a systematic review (2007–2022). Appl Surf Sci Adv 18:100501. doi: 10.1016/j.apsadv.2023.100501
Nti, E. K., Kranjac-Berisavljevic, G., Doke, D. A., Wongnaa, C. A., Attafuah, E. E., and Gyan, M. A. (2023). The impact of artisanal gold mining on the sustainability of Ghana’s river basins: the case of the Pra basin. Environ Sustain Indicat 19:100264. doi: 10.1016/j.indic.2023.100264
Nunoo, S., Manu, J., Owusu-Akyaw, F. K. B., and Nyame, F. K. (2022). Impact of artisanal small-scale (gold and diamond) mining activities on the Offin, Oda and Pra rivers in southern Ghana, West Africa: a scientific response to public concern. Heliyon 8:e12323. doi: 10.1016/j.heliyon.2022.e12323
Obiri-Yeboah, A., Nyantakyi, E. K., Mohammed, A. R., Yeboah, S. I. I. K., Domfeh, M. K., and Abokyi, E. (2021). Assessing potential health effect of lead and mercury and the impact of illegal mining activities in the Bonsa river, Tarkwa Nsuaem, Ghana. Scientific African 13:e00876. doi: 10.1016/j.sciaf.2021.e00876
Ochoo, B., Valcour, J., and Sarkar, A. (2017). Association between perceptions of public drinking water quality and actual drinking water quality: a community-based exploratory study in Newfoundland (Canada). Environ. Res. 159, 435–443. doi: 10.1016/j.envres.2017.08.019
Owusu-Prempeh, N., Awuah, K. O., Abebrese, I. K., and Amaning, E. N. (2022). Analysis of the status and ecological risks of heavy metals contamination in artisanal and small-scale gold mine-spoils at the Atewa Forest landscape, Ghana. Scientific African 16:e01235. doi: 10.1016/j.sciaf.2022.e01235
Paithankar, J. G., Saini, S., Dwivedi, S., Sharma, A., and Chowdhuri, D. K. (2021). Heavy metal associated health hazards: an interplay of oxidative stress and signal transduction. Chemosphere 262:128350. doi: 10.1016/j.chemosphere.2020.128350
Qadeer, A., Saqib, Z. A., Ajmal, Z., Xing, C., Khan Khalil, S., Usman, M., et al. (2020). Concentrations, pollution indices and health risk assessment of heavy metals in road dust from two urbanized cities of Pakistan: comparing two sampling methods for heavy metals concentration. Sustain. Cities Soc. 53:101959. doi: 10.1016/j.scs.2019.101959
Rad Fard, M., Seif, M., Ghazizadeh Hashemi, A. H., Zarei, A., Saghi, M. H., Shalyari, N., et al. (2019). Protocol for the estimation of drinking water quality index (DWQI) in water resources: artificial neural network (ANFIS) and arc-Gis. Methods X 6, 1021–1029. doi: 10.1016/j.mex.2019.04.027
Saroop, S., and Tamchos, S. (2021). “4-Monitoring and impact assessment approaches for heavy metals,” in Heavy Metals in the Environment. Eds. V. Kumar, A. Sharma, and A Cerdà. (Amsterdam, Netherlands, NL: Elsevier), 57–86. doi: 10.1016/B978-0-12-821656-9.00004-3
Setia, R., Dhaliwal, S. S., Kumar, V., Singh, R., Kukal, S. S., and Pateriya, B. (2020). Impact assessment of metal contamination in surface water of Sutlej River (India) on human health risks. Environ. Pollut. 265:114907. doi: 10.1016/j.envpol.2020.114907
Sharma, A. K., Sharma, M., Sharma, S., Malik, D. S., Sharma, M., and Sharma, A. K. (2024). A systematic review on assessment of heavy metals toxicity in freshwater fish species: current scenario and remedial approaches. J. Geochem. Explor. 262:107472. doi: 10.1016/j.gexplo.2024.107472
Verma, R., and Dwivedi, P. (2013). Heavy metal water pollution-a case study. Recent Res. Sci. Technol. 5, 98–99.
Wang, Z., Sun, Y., Yao, W., Ba, Q., and Wang, H. (2021). Effects of cadmium exposure on the immune system and immunoregulation. Frontiers in immunology, 12:695484. doi: 10.3389/fimmu.2021.695484
Wasonga, J., Okowa, M., and Kioli, F. (2016). Sociocultural determinants to adoption of safe water, sanitation, and hygiene practices in Nyakach, Kisumu County, Kenya: a descriptive qualitative study. J. Anthropol. 2016, 1–5. doi: 10.1155/2016/7434328
Zhang, Q., Qian, H., Xu, P., Li, W., Feng, W., and Liu, R. (2021). Effect of hydrogeological conditions on groundwater nitrate pollution and human health risk assessment of nitrate in Jiaokou Irrigation District. Journal of Cleaner Production, 298:126783. doi: 10.1016/j.jclepro.2021.126783
Keywords: water quality, heavy metals, health risk, Nubui River, Ghana
Citation: Norvivor FA, Azizi S, Fuku X, Atibu EK, Idris AO, Sibali L, Maaza M and Kamika I (2024) Ecological and human health risk of heavy metals in Nubui River: a case of rural remote communities. Front. Water. 6:1397853. doi: 10.3389/frwa.2024.1397853
Edited by:
Nitish Sharma, The State University of New Jersey, United StatesReviewed by:
Ayomi Jayarathne, The University of Queensland, AustraliaRanju Kumari Rathour, Himachal Pradesh University, India
Copyright © 2024 Norvivor, Azizi, Fuku, Atibu, Idris, Sibali, Maaza and Kamika. This is an open-access article distributed under the terms of the Creative Commons Attribution License (CC BY). The use, distribution or reproduction in other forums is permitted, provided the original author(s) and the copyright owner(s) are credited and that the original publication in this journal is cited, in accordance with accepted academic practice. No use, distribution or reproduction is permitted which does not comply with these terms.
*Correspondence: Shohreh Azizi, azizis@unisa.ac.za; Ilunga Kamika, kamiki@unisa.ac.za