- 1Grupo Inmunovirología, Facultad de Medicina, Universidad de Antioquia, Medellín, Colombia
- 2Grupo Infettare, Facultad de Medicina, Universidad Cooperativa de Colombia, Medellín, Colombia
Introduction: The HIV-exposed seronegative (HESN) status is for individuals who remain seronegative despite repeated exposure to HIV. One of the main cohorts within this group is men who have sex with men (MSM). Studies of this cohort have revealed different immunological and genetic mechanisms that can explain the phenomenon of natural HIV resistance. NK cells’ higher effector capacity is related to natural resistance to HIV. Besides, a new population of NK cells with adaptive features was described recently. These cells are increased in some HESN cohorts and appear to be involved in better control of viral replication in primarily HIV-infected subjects. The present study evaluated the role of NK cells in the natural resistance to HIV-1 infection in MSM.
Methodology: Phenotypic and functional features were evaluated in NK cells from two groups of MSM, at different risks of HIV infection, according to the number of sexual partners. The production of IFN-γ and β-chemokines was included in the analysis, as well as the cytotoxic capacity and adaptive NK cell frequency. Genetic features, such as HLA and KIR allele frequencies, were also explored.
Results: High-risk MSM exhibit an increased frequency of fully mature and CD57+/NKG2Chigh NK cells. These individuals also show higher cytotoxic capacity and IFN-γ production in response to K562 stimuli. NK cells with a CD107a+/IFN-γ+ functional profile were found more frequently and displayed higher IFN-γ production capacity among high-risk MSM than among low-risk MSM. The protective allele HLA-B∗18 was only present in the high-risk MSM group as well as HLA-B∗ 39. The protective phenotype KIR3DL1/S1-HLA-B∗Bw4, in a homozygous state, was particularly abundant in the high-risk population. Notably, some of these functional features were related to higher frequencies of mature and CD57+/NKG2Chigh NK cells, which, in turn, were associated with a higher number of sexual partners.
Conclusion: The changes observed in the NK cell compartment can be driven by the magnitude of sexual exposure and immunological challenges of high-risk individuals, which could influence their resistance/susceptibility to HIV infection.
Introduction
Human inmunodeficienty virus (HIV) researchers worldwide have focused on HIV-exposed seronegative (HESN) individuals, given valuable information about natural resistance to HIV that can be obtained from studies involving this population (1). These studies have revealed different immunogenetic mechanisms that can explain the phenomenon of natural HIV resistance. The most widely described of these is the CCR5Δ32 mutation, which confers resistance to infection with R5 strains of HIV (2, 3). This knowledge has led to the development of antiretroviral drugs that act to block this co-receptor, emphasizing the importance of research on mechanisms of natural resistance to HIV in order to formulate new therapeutic strategies and vaccines.
Men who have sex with men (MSM) represent an interesting cohort for studying natural resistance mechanisms, based on their social and biological characteristics, that make them a group at high risk for HIV infection. This cohort represents nearly 69% of HIV-positive men around the world (4). Natural resistance mechanisms described in other HESN cohorts, such as serodiscordant couples and commercial sex workers, have also been found in MSM. However, many other mechanisms remain to be studied, including increased effector capacity of NK cells, which represents an important natural resistance mechanism (5, 6).
NK cells may contribute to HIV infection control in several ways. These are essential to the induction of adaptive immune responses and can eliminate infected cells through cytotoxic mechanisms (7) and the production of β-chemokines, which prevent the infection of new cells by blocking viral co-receptors (5, 8–11). In 2003, Scott-Algara et al. reported, for the first time, increased effector capacity of NK cells in intravenous drug users (IDUs) who remained uninfected after several years of practices associated with a high risk of exposure to HIV. NK cells from HESN IDUs showed a higher cytotoxic capacity than NK cells from healthy controls and other IDUs who seroconverted during the study, showing the importance of NK cell effector capacity for natural resistance to HIV (5).
In 2006, NK cells with memory characteristics were described in murine models (12). Later, in 2015, Reeves et al. reported these cells could eliminate dendritic cells pulsed with vaccine proteins from simian immunodeficiency virus in vaccinated rhesus macaques in a specific way (13). A population of CD57+/NKG2C+ NK cells has been found at higher frequencies in a cohort of HESN individuals than in healthy donors and HIV-infected individuals (14). Studies carried out in primarily HIV-infected individuals showed that a higher frequency of NKG2C+ NK cells correlates with a lower viral set point establishment and better immunological parameters (i.e., lower plasma levels of IL-6, and lower PD-1 expression on mDCs). This suggests that CD57+/NKG2C+ NK cells can contribute to HIV replication control (15), implying a resistant phenotype.
Materials and Methods
Study Population
A cross-sectional study involving a cohort of 42 MSM recruited from Medellín, Colombia is presented. The MSM were divided into two groups according to the frequency of sexual partners in the 3 months before enrolling in the study: (i) MSM at high risk of HIV infection: those with more than 15 different sexual partners in the last 3 months with unprotected sexual intercourse (high-risk MSM) and (ii) MSM at lower risk of HIV infection: those with four or fewer than four different sexual partners in the last 3 months with unprotected sexual intercourse (low-risk MSM); all individuals included reported having receptive anal sexual encounters. MSM younger than 18 years of age, positive for HIV 1/2 rapid test (SD BIOLINE, Abbott), positive for HIV-1 proviral DNA by PCR, or homozygous for CCR5 Δ32 mutation were excluded.
Signed informed consent and a questionnaire on risky behaviors were obtained from each individual after the study protocol was explained to them. Later, 50 mL of peripheral blood was taken with a disposable syringe. This study was performed following the Helsinki Declaration (1975, revised in 2000) and approved by the bioethics board of the Universidad de Antioquia.
Frequency and Phenotype of NK Cells
Aliquots of whole blood were stained for 25 min in the dark using the following monoclonal antibodies: CD45 (HI30, APC-eFluor780), CD56 (CMSSB, PE-Cy5), CD3 (UCHT1, Alexa Fluor 700), and CD57 (TB01, FITC), obtained from Thermo Scientific (Wilmington, DE, United States); NKG2C (134591, PE; R&D Systems, MN, United States); and CD16 (3G8, V450; BD Biosciences, San Jose, CA, United States). After staining, the samples were treated with red blood cell lysing solution (BD Biosciences) following the manufacturer’s instructions. Finally, cells were washed twice with phosphate-buffered saline (PBS) (Lonza, Rockland, ME, United States) and suspended in 2% paraformaldehyde. Cells were acquired using LS Fortessa (BD Biosciences, San Jose, CA, United States) and data were analyzed using FlowJo version 10.5.3 (FlowJo, LLC, Ashland, Oregon, United States).
Natural Cytotoxicity Assays
Peripheral blood mononuclear cells (PBMCs) were isolated through a density gradient with Ficoll-Histopaque (Sigma-Aldrich, St. Louis, MO, United States) by centrifugation at 400 g for 30 min. They were then washed three times with PBS to eliminate platelets and debris. Next, the cells were counted and frozen until used.
PBMCs were thawed and left in culture with RPMI (Sigma-Aldrich, St. Louis, MO, United States) supplemented with 10% fetal bovine serum (FBS) (Gibco, Grand Island, NY, United States) for 24 h before each experiment. K562 cells were used as targets because there was no expression of classical HLA-I molecules. Before culturing, 1 × 106 K562 cells were stained with 0.1 mM eFluor670 (Thermo Scientific, Wilmington, DE, United States) in PBS for 10 min at 37°C to identify them as target cells in the flow cytometry analysis. Then, PBMCs were co-cultured with K562 cells in round-bottomed tubes at a 10:1 ratio in 300 μL of RPMI with 10% FBS for 4 h at 37°C and 5% CO2.
After incubation, the cells were stained with propidium iodide (PI) and DIOC-6 (both from Thermo Scientific, Wilmington, DE, United States) for 15 min in the dark. PI and DIOC-6 were used to evaluate the integrity of the cell and mitochondrial membranes, respectively. K562 cells were cultured in the absence of PBMCs as a spontaneous death control. This control was carried out for every assay. Spontaneous death control had to be lower than 15% for the experiment to be valid. The cytotoxicity percentage was adjusted based on spontaneous death control.
NK Cell Activation Assays
Target K562 cells were cultured in RPMI supplemented with 10% FBS at a density of 1 × 106/mL until the co-culture. Fresh PBMCs (2 × 106 cells/mL) were stimulated with 25 ng/mL IL-15 (Thermo Scientific, Wilmington, DE, United States) in six-well plates with 2 mL of RPMI supplemented with 10% FBS overnight to prime NK cells for IFN-γ production. Then, PBMCs were cultured in round-bottomed tubes at a 10:1 ratio with K562 (1 × 106 PBMC:1 × 105 K562) in 300 μL of RPMI with 10% FBS, 6 μg/mL brefeldin A (Thermo Scientific, Wilmington, DE, United States), 2 mM monensin (Thermo Scientific, Wilmington, DE, United States), and 1 μL of anti-CD107a (BD Biosciences, San Jose, CA, United States) for 5 h at 37°C and 5% CO2. A replica of every tube with no brefeldin A and monensin was included for Cytometric Bead Array (CBA) analysis.
Before staining, the cells were incubated with 100 μL of IgG (20 μg/mL) to block Fc receptors. Then, the cells were stained with monoclonal antibodies (Mabs) against CD45, CD56, and CD16 for 20 min in the dark. The cells were treated with Foxp3/Transcription Factor Staining Buffer Set (Thermo Scientific, Wilmington, DE, United States), following the manufacturer’s instructions to permeabilize cells. Subsequently, cells were stained with anti-CD3, -IFN-γ (4S.B3, BV711; BioLegend, San Diego, CA, United States), -Granzyme B (BG11, FITC; BD Biosciences), and -MIP-1β (PE; BD Biosciences) for 25 min in the dark. Finally, cells were suspended in 2% paraformaldehyde and acquired using LS Fortessa. Data were analyzed using FlowJo version 10.5.3.
Quantification of Effector Molecules by CBA
Supernatants of NK cell activation assays were collected and stored at −80°C until used. Supernatants were thawed at 4°C, just before the CBA assay. CBA flex set for MIP-1α, RANTES, TNF-α, and IFN-γ (BD Biosciences) was used and performed following the manufacturer’s instructions. Bead complexes were acquired using LS Fortessa. Data were analyzed using FlowJo version 10.5.3.
Quantification of mRNA by Real-Time RT-PCR
Total RNA was purified from PBMCs using the Direct-zol RNA Miniprep kit (Zymo Research, Orange, CA, United States), following the manufacturer’s instructions. The RNA was reverse-transcribed to cDNA using a high-capacity cDNA reverse transcription kit (Thermo Scientific, Wilmington, DE, United States). PCR reactions were performed using the Maxima SYBR Green qPCR master mix kit (Fermentas, France). Real-time RT-PCR was performed in a QuantStudio 5 Real-Time PCR System (Thermo Scientific, Wilmington, DE, United States). The data are expressed as relative units, normalized against the constitutive gene PGK (phosphoglycerate kinase), using formula 1.8−[ΔCt], where 1.8 corresponds to the mean PCR efficiency of 80%. The primer sequences and PCR conditions are described in Supplementary Table S1.
HLA and KIR Genotyping
HLA and KIR genotyping were performed in collaboration with the Hospital San Vicente Fundación, Colombia. HLA genotyping was performed using the DNA hybridization assay (LIFECODES HLA-SSO Typing) with SSO probes attached to multicolored beads; the bead complexes were detected with a Luminex 100 flow cytometer. KIR alleles were genotyped using RSSOKIR (One-Lambda, Canoga Park, CA, United States), a multiplex polymerase chain reaction sequence-specific primer reaction.
Determination of Anti-HCMV IgG Titers
Titers of IgG antibodies specific for HCMV were determined using a Human Anti-Cytomegalovirus IgG ELISA Kit (CMV) (Abcam, Cambridge, MA, United States) in serum samples of all participants, following the manufacturer’s instructions.
Statistical Analysis
To compare data from high-risk MSM vs. low-risk MSM, Mann–Whitney U or Student’s t-test was performed, depending on the bivariate normality assumption according to the Shapiro–Wilk normality test. Correlation analyses were based on the calculation of Spearman’s correlation coefficient. A p-value < 0.05 was considered statistically significant. The statistical tests were performed using GraphPad Prism Software version 7.02.
Results
MSM Socio-Demographic Data
Forty-two MSM who met the inclusion criteria were enrolled in this study. Their socio-demographic data are summarized in Table 1. The median number of lifetime sexual partners among the high-risk MSM was 1200, compared with 29 for the low-risk MSM group (p = 0.0001). Therefore, the high-risk group had higher sexual exposure, not only in the 3 months prior to the study, per the inclusion criteria, but also during their entire active sexual lives. In the high-risk MSM group, there were more frequent findings of a history of HIV-positive sexual partners, sexually transmitted infections (STI), and heterozygosity for CCR5 Δ32 mutation compared with the low-risk MSM group. The rate of condom use was near 50% in both groups. The ages upon starting to have sexual intercourse were similar between the two groups; however, the high-risk MSM were older and had a longer duration of high-risk sexual activity than the low-risk MSM.
The most common STIs were gonorrhea and syphilis in the entire study population (Figure 1A). When STI frequency was evaluated, based on the causative agent, the results showed that STIs with viral origins, such as herpes and condyloma, were more frequent in low-risk MSM (Figure 1B).
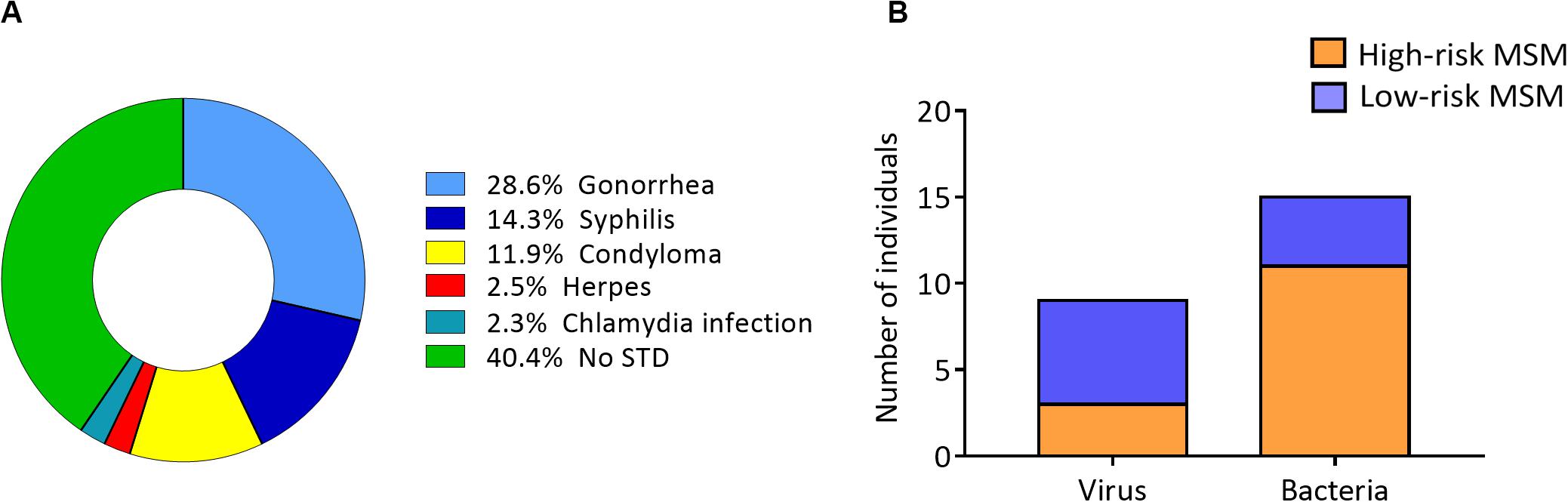
Figure 1. Lower frequency of viral STIs in high-risk MSM. (A) STI distribution among all study participants (STI history was self-reported by each individual in the questionnaire). (B) STI distribution according to the causative agent (viral or bacterial) in the two groups.
High-Risk MSM Exhibits a Mature Phenotype in the NK Cell Compartment Compared With Low-Risk MSM
The frequency and phenotype of NK cells in the peripheral blood of MSM were analyzed by flow cytometry. The two main NK cell subpopulations, CD56bright and CD56dim, as well as terminally differentiated NK cells, identified by the expression of the maturation marker CD57, were analyzed. CD57+/NKG2Chigh NK cells, which are terminally differentiated NK cells with high expression of activating receptor NKG2C, were also included in the analysis (Figure 2A).
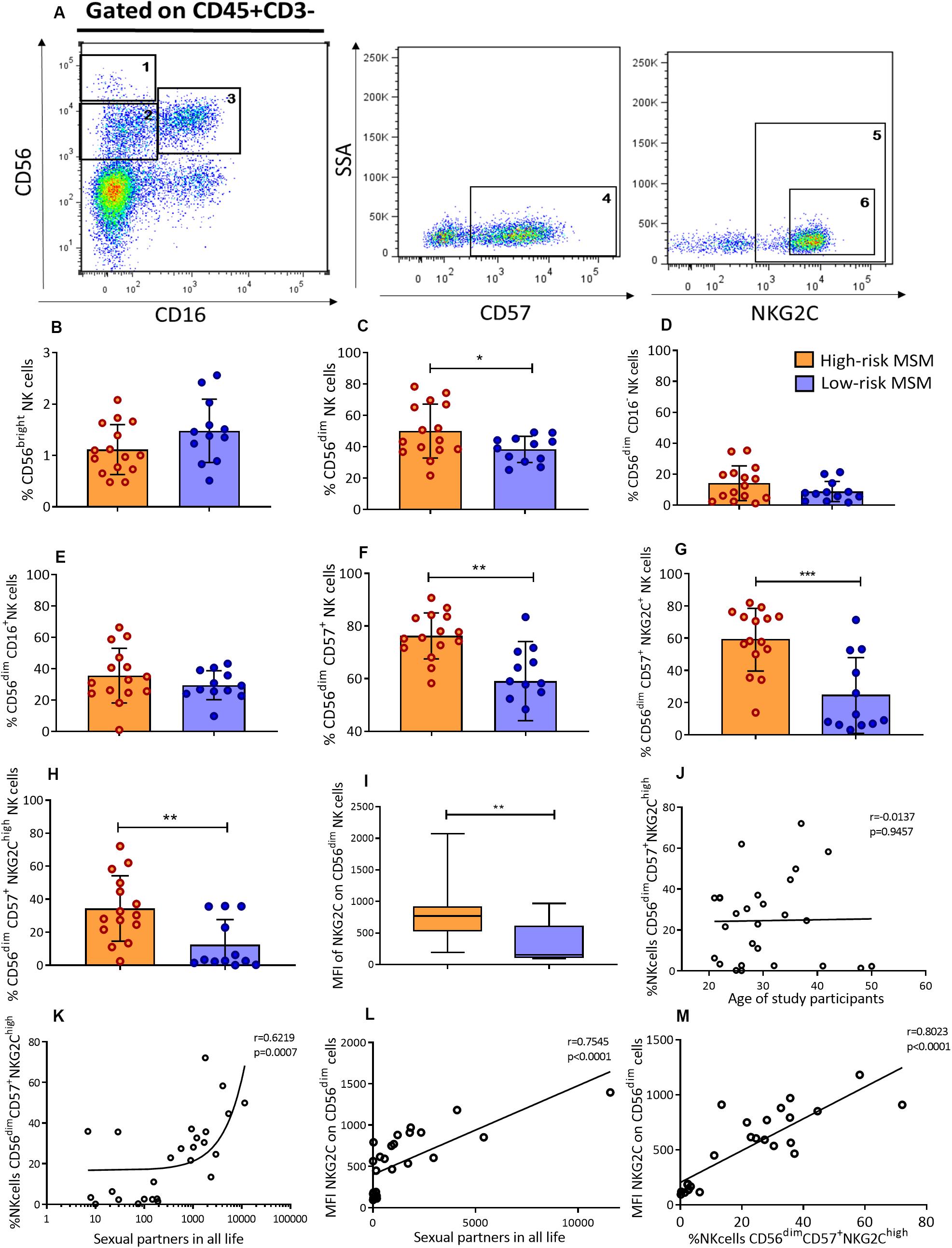
Figure 2. High-risk MSM have a higher frequency of CD56dim cells and adaptive CD57+/NKG2Chigh NK cells. (A) Representative gating strategy identifying NK cell subpopulations. NK cells were selected as CD45+CD3– cells from peripheral blood; subsequently, according to CD56 and CD16 expression, three NK cell subpopulations were defined: (1) CD56bright, (2) CD56dimCD16–, and (3) CD56dimCD16+. In the CD56dim subpopulation (2 + 3), the cells expressing CD57 marker were defined as terminally differentiated NK cells: (4) CD56dimCD57+. In the last subpopulation, two gating were performed: (5) CD56dimCD57+NKG2C+ and (6) CD56dimCD57+NKG2Chigh. (B–G) Frequencies of NK cell subpopulations in peripheral blood. (H) Frequency of adaptive CD57+/NKG2Chigh NK cells. (I) The MFI of NKG2C expression on CD56dim NK cells. (J,K) Correlations of CD57+/NKG2Chigh NK cell frequency with age and the number of sexual partners throughout the life span, respectively. (L) Correlation of the MFI of NKG2C expression on CD56dim NK cells and the number of lifetime sexual partners. (M) Correlation of the MFI of NKG2C expression on CD56dim NK cells and the frequency of CD57+/NKG2Chigh NK cells. Statistical evaluations were performed using Mann–Whitney U, unpaired t-test or Spearman’s correlation test. *p < 0.05, **p < 0.01, and ***p < 0.001.
Although there were no significant differences in the percentage of CD56bright NK cells (1.1 ± 0.5 vs. 1.5 ± 0.6, p = 0.09) (Figure 2B) between the two groups, the rate of total CD56dim NK cells was higher in high-risk than low-risk MSM (50 ± 17.1 vs. 38.4 ± 8.3, p = 0.041) (Figure 2C). Although no significant differences were observed in the frequency of CD56dim subpopulations, CD56dimCD16– and CD56dimCD16+ (Figures 2D,E), the frequency of terminally differentiated NK cells (CD56dim/CD57+) was also higher in high-risk MSM (76.2 ± 8.7 vs. 59.0 ± 15.0, p = 0.001) as well as CD57+/NKG2C+ NK cells (Figures 2F,G).
NK cell subpopulations may correspond to different maturation stages. CD56bright is the most immature stage and CD56dim is the most mature stage; the latter of these is the cellular compartment, where most of the terminally differentiated NK cells are located. The CD57 maturation marker is positively regulated during the maturation process, and it allows the identification of those maturation stages. Higher percentages of DC56dim and CD57+ NK cells indicate a mature phenotype in high-risk compared with low risk-MSM, this population also have a higher density of CD57 expression were NK cell subpopulation were compared between both groups (Supplementary Figure S1).
CD57+/NKG2Chigh NK Cells Are More Frequent in High-Risk MSM Than in Low-Risk MSM
The cumulative evidence indicates that there are NK cell subpopulations with certain adaptive characteristics. CD57+/NKG2Chigh NK cells are terminally differentiated NK cells that, under certain stimuli, undergo some phenotypic and functional changes that are frequently associated with HCMV infection. These changes include the acquisition of adaptive features and the upregulation of NKG2C expression, which represents a key activation receptor of CD57+/NKG2Chigh NK cells.
The frequency of CD57+/NKG2Chigh NK cells, as well as the MFI of NKG2C, was evaluated in both groups. The results showed that high-risk MSM had not only a higher frequency of these cells than low-risk MSM (34.3 ± 19.7 vs. 12.4 ± 15.2, p = 0.006) (Figure 2H), but also a higher density of NKG2C expression on the CD56dim NK cell compartment (837.4 ± 455.5 vs. 334.2 ± 312.6, p = 0.0026) (Figure 2I).
Higher levels of anti-HCMV IgG are related to higher numbers of CD57+/NKG2Chigh NK cells, as shown previously. For that reason, anti-HCMV IgG levels were evaluated to explain the higher frequency of CD57+/NKG2Chigh NK cells found in high-risk MSM. High-risk MSM exhibited titers of anti-HCMV IgG ranging from 176.2 to 1845 UI/mL, while those in low-risk MSM ranged from 79 to 814.5 UI/mL, with no significative difference between them (p = 0.217).
The frequency of CD57+/NKG2Chigh NK cells is related to the aging process along with the exposure to HCMV. As shown in the socio-demographic data, the high-risk MSM were older than the low-risk ones; therefore, the correlation between the frequency of CD57+/NKG2Chigh NK cells and age was evaluated in both MSM groups. There was no correlation between these variables (r = 0.0137, p = 0.9457) (Figure 2J). However, there was a strong positive correlation between the frequency of CD57+/NKG2Chigh NK cells and the number of lifetime sexual partners (r = 0.6219, p = 0.0007) (Figure 2K), but not with sexual partners in the previous 3 months (Supplementary Figure S2). These data suggest that the magnitude of sexual exposure, measured as the number of sexual partners, could stay contributing to CD57+/NKG2Chigh NK cell expansion rather than aging or HCMV infection.
A strong correlation was also found between the density of NKG2C expression on CD56dim NK cells (r = 0.7545, p < 0.0001) and the number of lifetime sexual partners (Figure 2L), as well as with the frequency of CD57+/NKG2Chigh NK cells (r = 0.8023, p < 0.0001) (Figure 2M). This confirmed the strong nexus between sexual exposure and the frequency of CD57+/NKG2Chigh NK cells found in the peripheral blood of MSM individuals.
Cytotoxic Activity of NK Cells Is Higher in High-Risk MSM Than in Low-Risk MSM
A higher cytotoxic capacity of NK cells has been reported in several cohorts for the study of HESN individuals related to protection against HIV infection. In this study, the cytotoxic capacity in both groups of MSM was evaluated by co-cultures of PBMCs with the K562 cell line. A representative scheme of the cytotoxicity assessment is shown in Figure 3A.
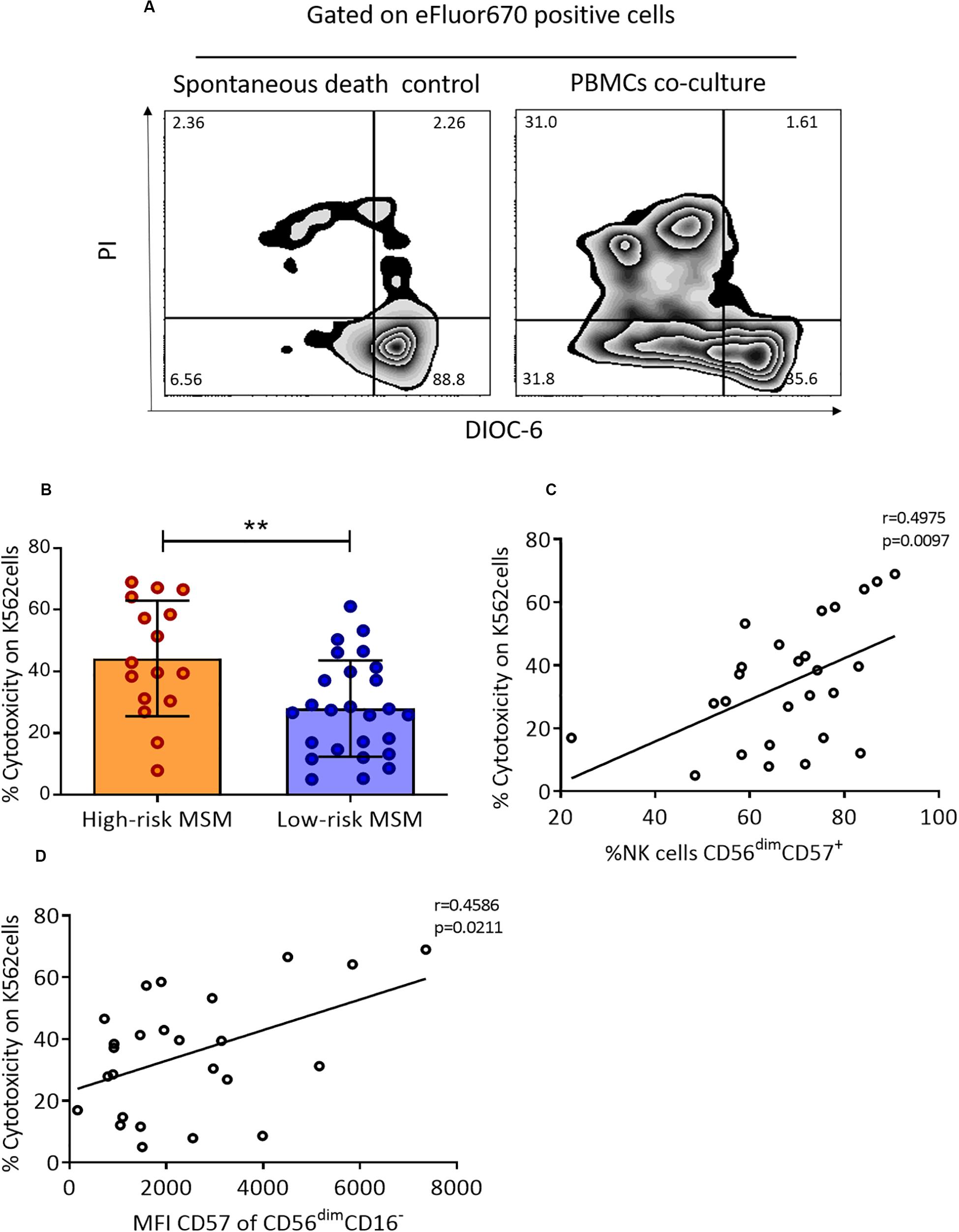
Figure 3. PBMCs from high-risk MSM exhibit higher cytotoxic activity against K562 cells. (A) Representative gate of target cells (K562) selected by eFluor670 expression after 4 h of culture. On the left, the apoptosis control with target cells alone (in the absence of effector cells); on the right, target cells after 4 h of co-culture with PBMCs. (B) The cytotoxic capacity over K562 cells. The cytotoxicity rate is expressed as the cell death percentage, corrected using an apoptosis control (live target cells are DIOC-6+/PI–). The results are shown as mean ± SD, n: 16;26. Statistical evaluations were performed with unpaired t-test. (C) Correlation of cytotoxicity with terminally differentiated NK cells. Spearman’s correlation test. (D) Correlation of cytotoxicity with the MFI of CD57 in the CD56dimCD16– subpopulation. Spearman’s correlation test. **p < 0.01.
High-risk MSM showed a higher cytotoxic capacity than low-risk MSM against the tumoral cell line K562 (44.2 ± 18.7 vs. 27.9 ± 15.5, p = 0.002) (Figure 3B). The cytotoxic capacity of NK cells and CD8+ T cells was previously shown to be related to the expression of CD57; for this reason, the correlation between the frequency of cells expressing this marker and the cytotoxic capacity was evaluated. There was a positive correlation between the cytotoxic capacity and the frequency of total CD56dimCD57+ NK cells (r = 0.497, p = 0.009) (Figure 3C). Finally, a correlation between the MFI of CD57 expression and cytotoxicity was found in CD56dimCD16– NK cells (r = 0.458, p = 0.021) (Figure 3D), but not in any other NK cell subpopulation.
High-Risk MSM Exhibit a Higher Frequency of IFN-γ-Positive NK Cells and Higher Levels of MIP-1α Production After K562 Co-culture
Granzyme B, CD107a, MIP-1β, and IFN-γ were evaluated as activation markers in NK cells after co-culture with K562 cells, by flow cytometry. A representative scheme of the activation assessment is shown in Figure 4A. High-risk MSM had a higher frequency (42.5 ± 6.8 vs. 32.6 ± 14.6, p = 0.039) of cells positive for IFN-γ in response to K562 cells (Figure 4B). No statistically significant differences were observed in the production of the other molecules (Figure 4B).
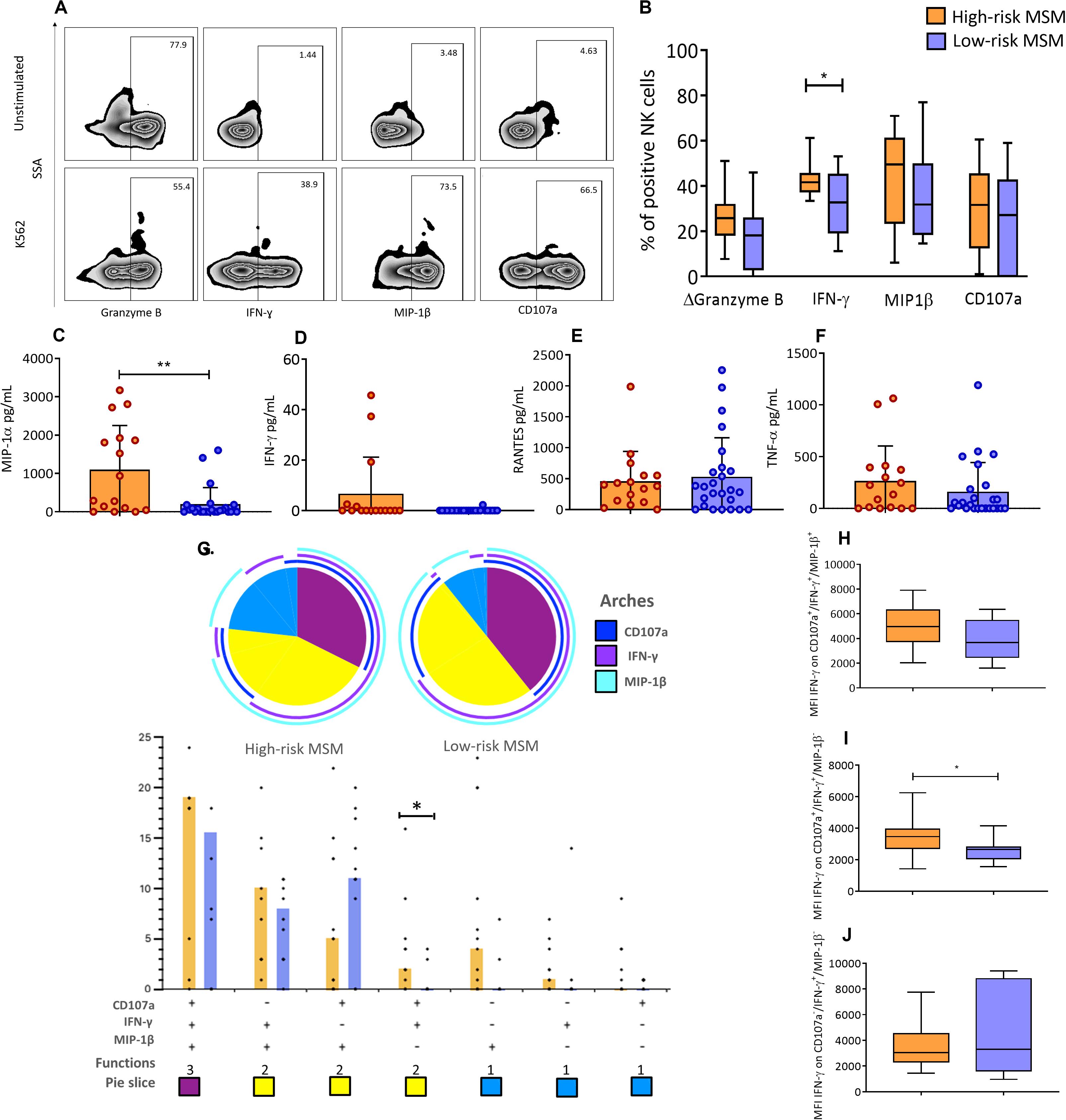
Figure 4. NK cells in high-risk MSM show different functional profiles compared to low-risk MSM. (A) Expression of activation markers in total NK cells before the stimulus and after 4 h of K562 co-culture. (B) Frequency of NK cells positive for effector molecules after 4 h of co-culture. Granzyme B is shown as ΔGranzyme B (% Granzyme B-positive cells in unstimulated condition – % Granzyme B-positive cells after K562 stimuli); the subtraction represents the percentage of NK cells that degranulate Granzyme B in response to the stimulus. The line inside the box indicates the mean, and whiskers indicate min. to max. value. (C–F) The concentration of effector molecules in the supernatant after 4 h of PBMC and K562 co-culture. (G) Functional profile analysis of NK cells after co-culture with the K562 cell line. The results are presented as mean. n: 15;10. Statistical evaluations were performed using unpaired t-test. *p < 0.05. In the permutation analysis carried out in the SPICE platform only data higher than 0.1% were included. (H–J) The MFI of IFN-γ in CD107a+/IFN-γ+/MIP-1β+, CD107a+/IFN-γ+/MIP-1β–, and CD107a–/IFN-γ+/MIP-1β– NK cells, respectively. The line inside the box indicates the mean, and whiskers indicate min. to max. value. n: 15;10. Statistical evaluations were performed using unpaired t-test or Mann–Whitney U test. *p < 0.05 and **p < 0.01.
The expression of MIP-1α, TNF-α, RANTES, and IFN-γ was also evaluated by CBA in the supernatants of the K562-PBMC co-culture. High-risk MSM showed higher MIP-1α production than low-risk MSM (1102 ± 1149 vs. 199 ± 432, p = 0.002) (Figure 4C). IFN-γ production was detected in 18.7% of high-risk MSM supernatants but was not detected in any low-risk MSM supernatants (Figure 4D). There were no differences in the production of TNF-α and RANTES between the groups (Figures 4E,F).
High-Risk MSM Showed a Distinct Functional Profile of NK Cells Compared With Low-Risk MSM
The functional profile of NK cells was evaluated in response to K562 cell stimulus. The measured molecules included CD107a, as a degranulation marker, MIP-1β, and IFN-γ.
The functional profile analytical results showed that monofunctional responses were more frequent among high-risk MSM. Analyses of NK cells with two functions revealed that there is a different NK cell population between the two groups, which was characterized by a CD107a+/IFN-γ+/MIP-1β– profile. These cells were more frequent in high-risk MSM than in low-risk MSM (p = 0.043) (Figure 4G). The frequencies of NK cells positive for the three markers were similar between the two groups.
Next, the MFI of IFN-γ in NK cells with different functional profiles was determined. The MFI of IFN-γ in NK cells with a CD107a+/IFN-γ+/MIP-1β– profile was higher in high-risk MSM than in low-risk MSM (3499 ± 1363 vs. 2546 ± 737, p = 0.035) (Figure 4I). Differences in the MFI of IFN-γ were not observed in NK cells with other functional profiles between the groups (Figures 4H,J). These results showed that high-risk MSM exhibit not only a higher frequency of NK cells but also major IFN-γ production by these cells with this functional profile, which could explain their better functional activity.
Basal mRNA of IFN-γ Is Higher in High-Risk MSM Than in Low-Risk MSM
The relative transcriptional expression of molecules associated with the effector function of NK cells was evaluated. Cytotoxic molecules (granzyme B and perforin), β-chemokines (MIP-1α, MIP-1β, and RANTES), IFN-γ, and TNF-α were evaluated.
The mRNA levels of IFN-γ in PBMCs were higher in high-risk MSM that in low-risk MSM at the basal state (0.07 ± 0.06 and 0.04 ± 0.03, p = 0.040) (Figure 5A). The IFN-γ transcript levels were also correlated with the frequency of CD57+/NKG2Chigh NK cells (r = 0.405, p = 0.039) (Figure 5B). No differences between groups were found in the relative expression of other molecules (Figures 5C–H).
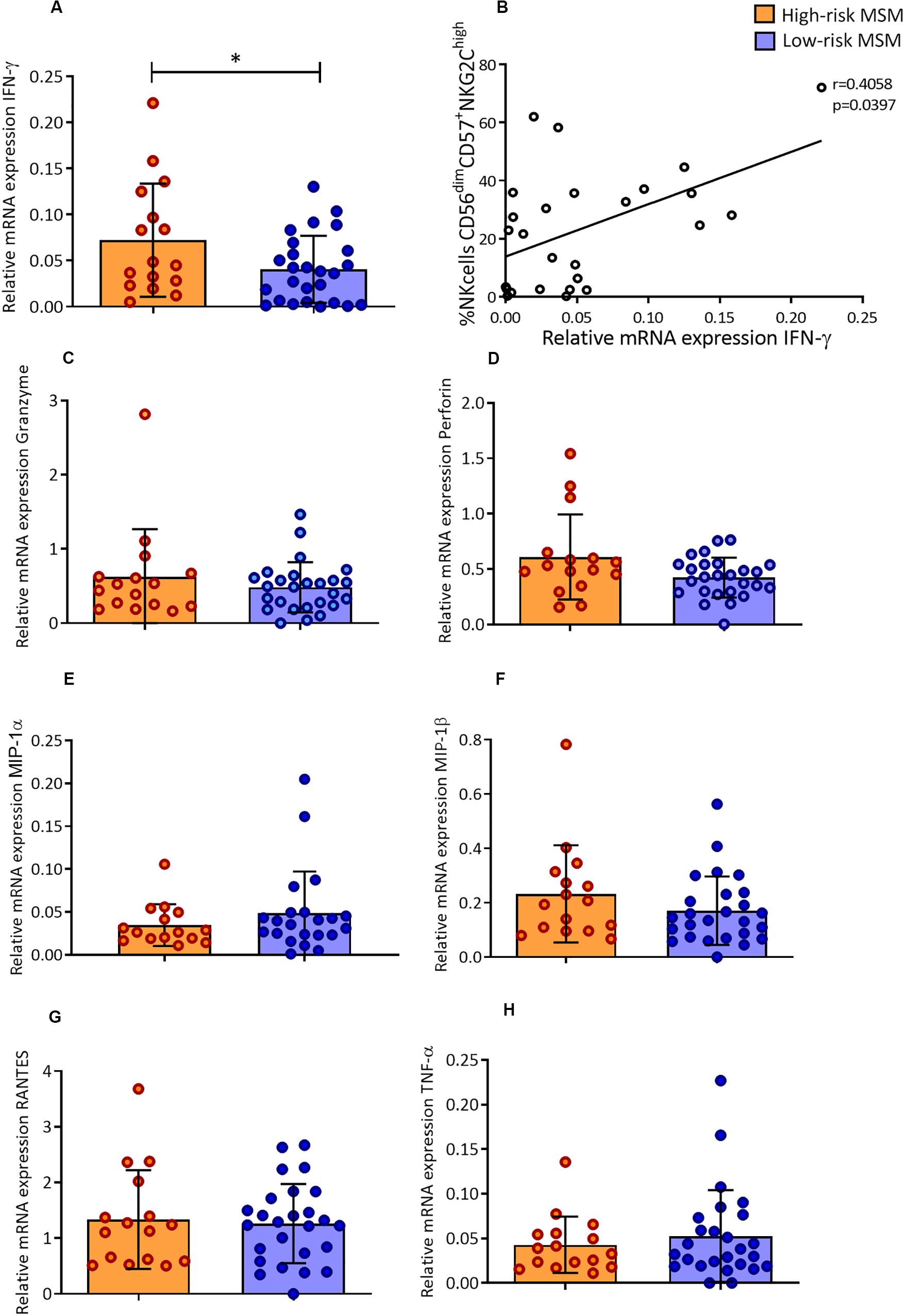
Figure 5. High-risk MSM have higher levels of IFN-γ mRNA in a basal state, which are correlated with the frequency of memory-like NK cells. (A) Relative mRNA expression of IFN-γ. n: 16;26. Statistical evaluations were performed using unpaired t-test. Results are shown as mean ± SD, *p < 0.05. (B) Correlation of relative mRNA expression of IFN-γ with the frequency of CD57+/NKG2Chigh NK cells. Spearman’s correlation test. (C–H) Relative mRNA expression of the other molecules evaluated; the results are shown as mean ± SD. n: 16;26. Statistical evaluations were performed using unpaired t-test.
KIR/HLA Protective Combination Frequency
Finally, considering that combinations of certain HLA-KIR genotypes have been associated with resistance to HIV infection, the frequency of HLA-B and KIR alleles was determined in the MSM groups. The HLA-B allele HLA-B∗18 was present at a rate of 23% in the high-risk MSM but was absent in the low-risk group, as well as HLA-B∗39 (Supplementary Tables S2, S3).
The frequency of the protective combination KIR3DL1/S1-HLA-B∗Bw4 in the homozygous state was determined in the study population. The protective phenotype KIR3DL1/S1-HLA-B∗Bw4/Bw4 was present at a rate of 30.7% in the high-risk MSM, while this phenotype was present in only 8% of the low-risk MSM. Three of the four high-risk individuals carrying the protective phenotype presented at least one HLA Bw4 80I allele (Table 2).
Discussion
This study provides information on combinatorial mechanisms and key characteristics of the NK cells, which could correlate with natural protection against HIV infection (or even other viruses) during sexual exposure of high-risk MSM. To the best of our knowledge, this the first report of increased NK cell activity in the MSM population.
The frequency of sexual partners in our high-risk MSM group exceeds the numbers reported in previous studies of high-risk MSM, ranging from three to six different sexual partners in the past 3 months (16, 17). That frequency is considered a high-risk practice, indicating that our high-risk population has even higher, exposure than other MSM cohorts. The median number of lifetime sexual partners indicates consistent high-risk practices throughout the sexually active part of the lifespan. This point, along with the high prevalence of STIs and the fact that nearly 60% of our high-risk group reported having at least one HIV-positive sexual partner, reflects the magnitude of sexual exposure and the immunological experience of this population. One dilemma in high-risk sexual cohort studies is the selection of control subjects. Many studies include low-risk (non-MSM) individuals as controls; however, it is important to mention that including this kind of control does not allow evaluation of factors such as repeated anal intercourse, allo-exposure (due to sperm contact), and STIs, which most likely affect baseline immunological parameters (1). In this study, the low-risk MSM individuals were from the same MSM community (considered globally as a high-risk population), with less infectious pressure, and insufficient to be considered HIV-resistant, based on the previously mentioned factors.
Demographic data revealed that the frequency of CCR5 Δ32 mutation in a heterozygous state in our high-risk population (18.7%) was similar to the frequency found in other MSM cohorts from the United States (12.9%) and Italy (20%) (18, 19), but higher than frequencies reported for the general population (2%) (20). This is related to a certain degree of protection in HESN cohorts (18, 19), but not in the general population (21). These findings suggest that the high frequency of CCR5 Δ32 mutation in a heterozygous state can contribute to the fact that high-risk MSM remains seronegative despite long periods of high-risk sexual behaviors (22).
Evaluation of the NK cell phenotype revealed higher frequencies of mature, terminally differentiated and adaptive CD57+/NKG2Chigh NK cells in high-risk MSM. This population also shows a higher expression of the maturation marker CD57 in all NK cell subpopulations (23). Moreover, it has been reported that NK cell maturation is an age-dependent process, where young people have high frequencies of CD56bright cells compared with older people, who show a higher frequency of CD56dim cells expressing maturation markers, such as CD57 (24, 25). However, it is not clear whether this phenomenon is explained by an intrinsic aging process or by cumulative lifetime exposure. Goodier et al. reported that in a Gambian population with a high frequency of HCMV infection, children reached the percentage of terminally differentiated NK cells (CD56dim/CD57+/NKG2C+) of an adult (nearly 70%) by the age of six, while Europeans barely reached these numbers in adulthood (nearly 50%) (26). A similar phenomenon has been reported in transplant recipients, where CD57+NKG2C+ NK cells are detected within 3 months in patients with reactivated HCMV infection after transplantation, while these cells can take more than 1 year to emerge in patients without infection reactivation (27). The expansion of CD57+NKG2C+ NK cells has also been reported in individuals infected with hantavirus, chikungunya, hepatitis B and C viruses (28–30), suggesting that exposure to infections, rather than aging, is a determinant for NK cell maturation rates.
Some factors explaining differences in the frequencies of mature and CD57+/NKG2Chigh NK cells between high-risk and low-risk MSM were evaluated. For instance, age and IgG titers against HCMV were reported to be positively correlated with the frequency of CD57+/NKG2Chigh NK cells in HESN individuals (14); however, we did not found significative differences in the HCMV IgG titers between both groups. High-risk MSM were older than low-risk MSM, although there was no correlation between CD56dimCD57+ or CD56dimCD57+NKG2Chigh frequencies and age. Interestingly, we found a strong correlation between the number of sexual partners in the lifetime and the CD56dimCD57+NKG2Chigh NK cells frequency and the NKG2C MFI (an important activating receptor of CD57+/NKG2Chigh NK cells). These results suggest that the magnitude of exposure, measured as the number of sexual partners, may be implicated in the expansion of CD57+/NKG2Chigh NK cells observed in high-risk MSM.
A mature phenotype was previously related to better cytotoxic capacity (23, 31). In our study, high-risk MSM exhibited higher cytotoxicity against the tumoral cell line K562 than low-risk MSM. In fact, the frequency of mature NK cells was positively correlated with the cytotoxic capacity, in terms of both the frequency and the density of CD57 expression.
As functional characteristics, after the K562 target cell stimulation, high-risk MSM exhibited a higher frequency of IFN-γ+ NK cells and higher production of MIP-1α. High rates of IFN-γ+ NK cells and β-chemokine production are associated with protection against HIV in other HESN cohorts, including serodiscordant couples, UDIs, and babies born to HIV-positive mothers (5, 8, 9); likewise, they are related to delayed progression to AIDS in long-term non-progressors (32).
Functional analysis revealed the presence of CD107a+/IFN-γ+/MIP-1β– NK cells. This population was more common in high-risk MSM, and it exhibited a higher IFN-γ production capacity than it did in the same population in low-risk MSM. NK cells with a CD107a+/IFN-γ+ functional profile have been associated with better control of HIV infection (33, 34). In 2013, Jiang et al. reported that long-term non-progressors had higher frequencies of these cells compared with typical progressors and healthy controls (32). The higher frequency of NK cells with this functional profile has also been associated with lower viral load and a lower CD4+ T-cell slope (34). Our findings suggest that the high number of CD107a+/IFN-γ+ NK cells found in high-risk MSM could play a role in natural resistance against HIV.
Higher mRNA levels of IFN-γ were found in PBMCs from high-risk MSM, and positively correlated with the number of CD57+/NKG2Chigh NK cells. Along with phenotypic changes, a big proportion of CD57+/NKG2Chigh NK cells suffer a series of epigenetic changes, including demethylation in the IFNG gene, resulting in an enhanced capacity of IFN-γ production (35), which was consistently observed in our functional analysis. There are several IFN-γ-induced proteins with antiviral activity, which can inhibit viral protein synthesis, edit viral sequences, degrade RNA, and impair the transport of viral nucleocapsid to the nucleus; this can, in turn, explain why higher IFN-γ levels are beneficial and related to protection in HESN individuals (36, 37). This is the first report describing high cytotoxic capacity as well as higher IFN-γ and MIP-1α production by NK cells in high-risk MSM, showing that these mechanisms are conserved among different cohorts of HESN individuals. However, a mature phenotype and higher frequency of CD57+/NKG2Chigh NK cells are poorly reported features in HESN individuals. In fact, higher frequencies of fully mature and CD57+/NKG2C+ NK cells have only been reported in a cohort of serodiscordant couples from Brazil, with NK cells showing increased CD107a and IFN-γ expression (14). Thus, higher frequencies of CD57+/NKG2Chigh NK cells in our MSM group as well, suggest that these cells could be involved in natural resistance to HIV infection.
It has been extensively reported that NK cells need priming to acquire functional competence, including cytokines, such as type I IFN, IL-12, IL-15, and IL-18 to acquire adequate cytotoxicity (38–40). Experimental models of persistent viral infections have shown that lower, but persistent, levels of cytokines induce NK cell activation for long periods (41, 42). The high-risk MSM have increased sexual exposure to different pathogens or antigens; this drives the environment required for the priming process, which explains the enhanced cytotoxicity and IFN-γ production that is characteristic of an experienced NK cell population.
Although proinflammatory stimulus may explain some long-term adaptations in conventional NK cells, the response of CD57+/NKG2Chigh NK cells to a proinflammatory stimulus is low or absent due to decreased expression of cytokine receptors (43). However, some infections such as hantavirus, chikungunya, and HIV are effective at activating or expanding the compartment of CD57+/NKG2Chigh NK cells generated after HCMV infection (28, 29, 44). Although the mechanism underlying this phenomenon is unclear, some of these responses have been attributed to elevated HLA-E expression (28). The upregulation of HLA-E, the ligand of NKG2C, is a common feature found in viral infections, such as dengue, hantavirus, and HIV (28, 45, 46). The engagement of NKG2C, the signature activating receptor of CD57+/NKG2Chigh NK cells, leads to polyfunctional responses characterized by degranulation of cytolytic molecules as well as TNF-α and IFN-γ release (29). In this way, cross-linking of NKG2C alone is sufficient to drive IFN-γ production in CD57+/NKG2Chigh NK cells (47), contradicting previously described requirements for NK cell activation (48).
When the frequency of STIs was evaluated according to the nature of the causative agent, high-risk MSM showed lower frequencies of viral STIs, but not bacterial ones. The reduced frequency of viral STIs in high-risk MSM could, potentially, be explained by this phenomenon, where CD57+/NKG2Chigh NK cells, activated through NKG2C by HLA-E, induce a strong antiviral response mediated by CD57+/NKG2Chigh NK cells that can protect these individuals against a variety of viral infections, including HIV.
In both cases, higher frequencies of experienced conventional NK cells and CD57+/NKG2Chigh adaptive NK cells indicate that there is a well-trained and powerful army of effector cells that can mediate a rapid and strong response against a variety of pathogens. As observed in our cohort, where fully mature and CD57+/NKG2Chigh adaptive NK cells might play an important role in natural resistance to HIV infection. Via either adaptive responses, generated after HIV contact or heterologous responses, generated by high exposure to other pathogens, NK cells might mediate an adequate control, avoiding the establishment of HIV infection.
In addition to the particular phenotypic and functional features, some genetic characteristics differed between groups. Protective alleles HLA-B∗18 and HLA-B∗39 were enriched in the high-risk MSM group. In babies born to seropositive mothers, the frequencies of the HLA-B∗18 allele were associated with a significantly lower risk of early HIV-1 transmission via breastfeeding (49); likewise, HLA-B∗18 has also been shown to be related to HIV protection in serodiscordant couples (50). The HLA-B∗39 allele has been associated with HIV protection in some studies (51, 52), although less often.
Besides, the protective phenotype of KIR3DL1/S1, in combination with HLA-Bw4 in the homozygous state, was found in 30.7% of high-risk MSM compared with a rate of 8% in low-risk MSM. HLA alleles containing the Bw4 epitope act as KIR ligands, while alleles with the Bw6 epitope do not. NK cells recognize self-HLA through KIR receptors, generating an inhibitory signal preventing auto aggression. Downregulation of HLA molecules at the cell membrane, a common phenomenon in viral infections, allows NK cell activation by “missing-self recognition” (53). KIR3DL1/S1 + NK cells educated in the context of Bw4 alleles exhibit a stronger capacity to kill HIV-1-infected cells and, as a consequence, patients with KIR3DL1/S1 + NK cells exhibit lower viral load outcomes during the infection (54–57). Boudreau et al. reported that KIR3DL1 and Bw4-80I partnerships endow NK cells with the greatest reactivity against HLA-negative targets, whereas NK cells exhibiting KIR3DL1 in combination with HLA-Bw4, not 80I, demonstrate intermediate responsiveness and Bw4-/KIR3DL1 + NK cells are poorly responsive (55). This evidence suggests that the expression of HLA-Bw4 alleles, in combination with KIR3DL1/S1, can be associated with resistance to both progression to AIDS and HIV infection in different HESN cohorts (33, 58, 59).
The data presented in this article suggest that NK cells of high-risk MSM present functional characteristics that could be related to the fact that MSM remain seronegative despite years of high-risk practices. The higher frequency of CD57+/NKG2Chigh NK cells exhibited by this population was correlated with these characteristics, indicating this subset’s important role during HIV exposure. Interestingly, similar anti-HCMV titers found between the two groups indicate that inducing CD57+/NKG2Chigh NK cells can be modulated by several factors that increase their frequency and impact their functional capacity, opening up an interesting field of research in HIV treatment based on boosting NK cells.
Data Availability Statement
All datasets generated for this study are included in the article/Supplementary Material.
Ethics Statement
The studies involving human participants were reviewed and approved by the Ethics committee Universidad de Antioquia. The patients/participants provided their written informed consent to participate in this study.
Author Contributions
LF-Á, JH, and WZ designed the research, analyzed the data, and wrote the manuscript. LF-Á, YB, KR, and AO-G performed the research. PV contributed to analytical tools. WZ supervised the project. All authors contributed to the article and approved the submitted version.
Funding
This work was supported by the Universidad de Antioquia, COLCIENCIAS (code 111577757209) and Universidad Cooperativa de Colombia (code INV2193).
Conflict of Interest
The authors declare that the research was conducted in the absence of any commercial or financial relationships that could be construed as a potential conflict of interest.
Acknowledgments
The authors thank the volunteers who kindly participated in this study. They also thank Corporación Stonewall, Consejo Consultivo LGBTI de Medellín, Fundación Antioqueña de Infectología [FAI], Red de Apoyo Social de Antioquia [RASA], and Andrés Felipe Cardona for their support.
Supplementary Material
The Supplementary Material for this article can be found online at: https://www.frontiersin.org/articles/10.3389/fimmu.2020.537044/full#supplementary-material
References
1. Horton RE, McLaren PJ, Fowke K, Kimani J, Ball TB. Cohorts for the study of HIV−1–exposed but uninfected individuals: benefits and limitations. J Infect Dis. (2010) 202:S377–81.
2. Liu R, Paxton WA, Choe S, Ceradini D, Martin SR, Horuk R, et al. Homozygous defect in HIV-1 coreceptor accounts for resistance of some multiply-exposed individuals to HIV-1 infection. Cell. (1996) 86:367–77. doi: 10.1016/s0092-8674(00)80110-5
3. Dean M, Carrington M, Winkler C, Huttley GA, Smith MW, Allikmets R, et al. Genetic restriction of HIV-1 infection and progression to AIDS by a deletion allele of the CKR5 structural gene. Hemophilia growth and development study, multicenter aids cohort study, multicenter hemophilia cohort study, San Francisco City Cohort, ALIVE Study. Science. (1996) 273:1856–62. doi: 10.1126/science.273.5283.1856
4. CDC Monitoring Selected National HIV Prevention and Care Objectives by Using HIV Surveillance Data—United States and 6 U.S. Dependent Areas—2011. HIV Surveillance Supplemental Report. (Vol. 13). Atlanta, GA: Centers for Disease Control and Prevention. (2013).
5. Scott-Algara D, Truong LX, Versmisse P, David A, Luong TT, Nguyen NV, et al. Cutting edge: increased NK cell activity in HIV-1-exposed but uninfected Vietnamese intravascular drug users. J Immunol. (2003) 171:5663–7. doi: 10.4049/jimmunol.171.11.5663
6. Tomescu C, Seaton KE, Smith P, Taylor M, Tomaras GD, Metzger DS, et al. Innate activation of MDC and NK cells in high-risk HIV-1-exposed seronegative IV-drug users who share needles when compared with low-risk nonsharing IV-drug user controls. J Acquir Immune Defic Syndr. (2015) 68:264–73. doi: 10.1097/qai.0000000000000470
7. Florez-Alvarez L, Hernandez JC, Zapata W. NK cells in HIV-1 infection: from basic science to vaccine strategies. Front Immunol. (2018) 9:2290. doi: 10.3389/fimmu.2018.02290
8. Lohman-Payne B, Slyker JA, Moore S, Maleche-Obimbo E, Wamalwa DC, Richardson BA, et al. Breast milk cellular HIV-specific interferon γ responses are associated with protection from peripartum HIV transmission. AIDS. (2012) 26:2007–16. doi: 10.1097/qad.0b013e328359b7e0
9. Montoya CJ, Velilla PA, Chougnet C, Landay AL, Rugeles MT. Increased IFN-gamma production by NK and CD3+/CD56+ cells in sexually HIV-1-exposed but uninfected individuals. Clin Immunol. (2006) 120:138–46. doi: 10.1016/j.clim.2006.02.008
10. Quillay H, Costa HE, Durie M, Marlin R, Cannou C, Madec Y, et al. NK cells control HIV−1 infection of macrophages through soluble factors and cellular contacts in the human decidua. Retrovirology. (2016) 13:39.
11. Vega JA, Villegas-Ospina S, Aguilar-Jiménez W, Rugeles MT, Bedoya G, Zapata W, et al. Haplotypes in CCR5-CCR2, CCL3 and CCL5 are associated with natural resistance to HIV-1 infection in a Colombian cohort. Biomédica. (2017) 37:267–73.
12. O’Leary JG, Goodarzi M, Drayton DL, von Andrian UH. T cell– and B cell–independent adaptive immunity mediated by natural killer cells. Nat Immunol. (2006) 7:507–16. doi: 10.1038/ni1332
13. Reeves RK, Li H, Jost S, Blass E, Li H, Schafer JL, et al. Antigen-specific NK cell memory in rhesus macaques. Nat Immunol. (2015) 16:927–32. doi: 10.1038/ni.3227
14. Lima JF, Oliveira LMS, Pereira NZ, Mitsunari GE, Duarte AJS, Sato MN. Distinct natural killer cells in HIV-exposed seronegative subjects with effector cytotoxic CD56dim and CD56bright cells and memory-Like CD57+NKG2C+CD56dim Cells. JAIDS J Acquir Immune Defic Syndr. (2014) 67:463–71. doi: 10.1097/qai.0000000000000350
15. Gondois-Rey F, Chéret A, Granjeaud S, Mallet F, Bidaut G, Lécuroux C, et al. NKG2C+memory-like NK cells contribute to the control of HIV viremia during primary infection: optiprim-ANRS 147. Clin Transl Immunol. (2017) 6:e150. doi: 10.1038/cti.2017.22
16. Pines HA, Karris MY, Little SJ. Sexual partner concurrency among partners reported by MSM with recent HIV infection. AIDS Behav. (2017) 21:3026–34. doi: 10.1007/s10461-017-1855-x
17. Tieu HV, Nandi V, Frye V, Stewart K, Oquendo H, Bush B, et al. Concurrent partnerships and HIV risk among men who have sex with men in New York City. Sex Transm Dis. (2014) 41:200–8. doi: 10.1097/olq.0000000000000090
18. Marmor M, Sheppard HW, Donnell D, Bozeman S, Celum C, Buchbinder S, et al. Homozygous and heterozygous CCR5-Delta32 genotypes are associated with resistance to HIV infection. J Acquir Immune Defic Syndr. (2001) 27:472–81. doi: 10.1097/00126334-200108150-00009
19. Trecarichi EM, Tumbarello M, de Gaetano Donati K, Tamburrini E, Cauda R, Brahe C, et al. Partial protective effect of CCR5-Delta 32 heterozygosity in a cohort of heterosexual Italian HIV-1 exposed uninfected individuals. AIDS Res Ther. (2006) 3:22.
20. Gupta A, Padh H. The global distribution of CCR5 delta 32 polymorphism: role in HIV-1 protection. BMC Infect Dis. (2012) 12:O16. doi: 10.1186/1471-2334-12-S1-O16
21. Liu S, Kong C, Wu J, Ying H, Zhu H. Effect of CCR5-Delta32 heterozygosity on HIV-1 susceptibility: a meta-analysis. PLoS One. (2012) 7:e35020. doi: 10.1371/journal.pone.0035020
22. de Silva E, Stumpf MP. HIV and the CCR5-Delta32 resistance allele. FEMS Microbiol Lett. (2004) 241:1–12. doi: 10.1016/j.femsle.2004.09.040
23. Nielsen CM, White MJ, Goodier MR, Riley EM. Functional significance of CD57 expression on human NK cells and relevance to disease. Front Immunol. (2013) 4:422. doi: 10.3389/fimmu.2013.00422
24. Björkström NK, Riese P, Heuts F, Andersson S, Fauriat C, Ivarsson MA, et al. Expression patterns of NKG2A, KIR, and CD57 define a process of CD56dim NK-cell differentiation uncoupled from NK-cell education. Blood. (2010) 116:3853–64. doi: 10.1182/blood-2010-04-281675
25. Le Garff-Tavernier M, Beziat V, Decocq J, Siguret V, Gandjbakhch F, Pautas E, et al. Human NK cells display major phenotypic and functional changes over the life span. Aging Cell. (2010) 9:527–35. doi: 10.1111/j.1474-9726.2010.00584.x
26. Goodier MR, White MJ, Darboe A, Nielsen CM, Goncalves A, Bottomley C, et al. Rapid NK cell differentiation in a population with near-universal human cytomegalovirus infection is attenuated by NKG2C deletions. Blood. (2014) 124:2213–22. doi: 10.1182/blood-2014-05-576124
27. Foley B, Cooley S, Verneris MR, Pitt M, Curtsinger J, Luo X, et al. Cytomegalovirus reactivation after allogeneic transplantation promotes a lasting increase in educated NKG2C+ natural killer cells with potent function. Blood. (2012) 119:2665–74. doi: 10.1182/blood-2011-10-386995
28. Bjorkstrom NK, Lindgren T, Stoltz M, Fauriat C, Braun M, Evander M, et al. Rapid expansion and long-term persistence of elevated NK cell numbers in humans infected with hantavirus. J Exp Med. (2011) 208:13–21. doi: 10.1084/jem.20100762
29. Beziat V, Dalgard O, Asselah T, Halfon P, Bedossa P, Boudifa A, et al. CMV drives clonal expansion of NKG2C+ NK cells expressing self-specific KIRs in chronic hepatitis patients. Eur J Immunol. (2012) 42:447–57. doi: 10.1002/eji.201141826
30. Petitdemange C, Becquart P, Wauquier N, Beziat V, Debre P, Leroy EM, et al. Unconventional repertoire profile is imprinted during acute chikungunya infection for natural killer cells polarization toward cytotoxicity. PLoS Pathog. (2011) 7:e1002268. doi: 10.1371/journal.ppat.1002268
31. Lopez-Vergès S, Milush JM, Pandey S, York VA, Arakawa-Hoyt J, Pircher H, et al. CD57 defines a functionally distinct population of mature NK cells in the human CD56dimCD16+ NK-cell subset. Blood. (2010) 116:3865–74. doi: 10.1182/blood-2010-04-282301
32. Jiang Y, Zhou F, Tian Y, Zhang Z, Kuang R, Liu J, et al. Higher NK cell IFN-gamma production is associated with delayed HIV disease progression in LTNPs. J Clin Immunol. (2013) 33:1376–85. doi: 10.1007/s10875-013-9930-1
33. Jiang Y, Chen O, Cui C, Zhao B, Han X, Zhang Z, et al. KIR3DS1/L1 and HLA-Bw4-80I are associated with HIV disease progression among HIV typical progressors and long-term nonprogressors. BMC Infect Dis. (2013) 13:405. doi: 10.1186/1471-2334-13-405
34. Chung AW, Navis M, Isitman G, Wren L, Silvers J, Amin J, et al. Activation of NK cells by ADCC antibodies and HIV disease progression. J Acquir Immune Defic Syndr. (2011) 58:127–31. doi: 10.1097/qai.0b013e31822c62b9
35. Luetke-Eversloh M, Hammer Q, Durek P, Nordström K, Gasparoni G, Pink M, et al. Human cytomegalovirus drives epigenetic imprinting of the IFNG locus in NKG2Chi natural killer cells. PLoS pathogens. (2014) 10:e1004441. doi: 10.1371/journal.ppat.1004441
36. Schroder K, Hertzog PJ, Ravasi T, Hume DA. Interferon-gamma: an overview of signals, mechanisms and functions. J Leukoc Biol. (2004) 75:163–89. doi: 10.1189/jlb.0603252
37. Samuel CE. Antiviral actions of interferons. Clin Microbiol Rev. (2001) 14:778–809. doi: 10.1128/cmr.14.4.778-809.2001
38. Chaix J, Tessmer MS, Hoebe K, Fuseri N, Ryffel B, Dalod M, et al. Cutting edge: priming of NK cells by IL-18. J Immunol. (2008) 181:1627–31. doi: 10.4049/jimmunol.181.3.1627
39. Fehniger TA, Cai SF, Cao X, Bredemeyer AJ, Presti RM, French AR, et al. Acquisition of murine NK cell cytotoxicity requires the translation of a pre-existing pool of granzyme B and perforin mRNAs. Immunity. (2007) 26:798–811. doi: 10.1016/j.immuni.2007.04.010
40. Lucas M, Schachterle W, Oberle K, Aichele P, Diefenbach A. Dendritic cells prime natural killer cells by trans-presenting interleukin 15. Immunity. (2007) 26:503–17. doi: 10.1016/j.immuni.2007.03.006
41. Bukowski JF, Biron CA, Welsh RM. Elevated natural killer cell-mediated cytotoxicity, plasma interferon, and tumor cell rejection in mice persistently infected with lymphocytic choriomeningitis virus. J Immunol. (1983) 131:991–6.
42. Barton ES, White DW, Cathelyn JS, Brett-McClellan KA, Engle M, Diamond MS, et al. Herpesvirus latency confers symbiotic protection from bacterial infection. Nature. (2007) 447:326–9. doi: 10.1038/nature05762
43. Min-Oo G, Lanier LL. Cytomegalovirus generates long-lived antigen-specific NK cells with diminished bystander activation to heterologous infection. J Exp Med. (2014) 211:2669–80. doi: 10.1084/jem.20141172
44. Gumá M, Cabrera C, Erkizia I, Bofill M, Clotet B, Ruiz L, et al. Human cytomegalovirus infection is associated with increased proportions of NK cells that express the CD94/NKG2C receptor in aviremic HIV−1–positive patients. J Infect Dis. (2006) 194:38–41. doi: 10.1086/504719
45. Drews E, Adam A, Htoo P, Townsley E, Mathew A. Upregulation of HLA-E by dengue and not Zika viruses. Clin Transl Immunol. (2018) 7:e1039. doi: 10.1002/cti2.1039
46. Martini F, Agrati C, D’Offizi G, Poccia F. HLA-E up-regulation induced by HIV infection may directly contribute to CD94-mediated impairment of NK cells. Int J Immunopathol Pharmacol. (2005) 18:269–76. doi: 10.1177/039463200501800209
47. Liu LL, Landskron J, Ask EH, Enqvist M, Sohlberg E, Traherne JA, et al. Critical role of CD2 Co-stimulation in adaptive natural killer cell responses revealed in NKG2C-deficient humans. Cell Rep. (2016) 15:1088–99. doi: 10.1016/j.celrep.2016.04.005
48. Bryceson YT, Ljunggren HG, Long EO. Minimal requirement for induction of natural cytotoxicity and intersection of activation signals by inhibitory receptors. Blood. (2009) 114:2657–66. doi: 10.1182/blood-2009-01-201632
49. Farquhar C, Rowland-Jones S, Mbori-Ngacha D, Redman M, Lohman B, Slyker J, et al. Human leukocyte antigen (HLA) B∗18 and protection against mother-to-child HIV type 1 transmission. AIDS Res Hum Retroviruses. (2004) 20:692–7. doi: 10.1089/0889222041524616
50. Chaudhari DV, Chavan VR, Ahir SP, Kerkar SC, Mehta PR, Mania-Pramanik J. Human leukocyte antigen B distribution in HIV discordant cohort from India. Immunol Lett. (2013) 156:1–6. doi: 10.1016/j.imlet.2013.09.002
51. Valenzuela-Ponce H, Alva-Hernandez S, Garrido-Rodriguez D, Soto-Nava M, Garcia-Tellez T, Escamilla-Gomez T, et al. Novel HLA class I associations with HIV-1 control in a unique genetically admixed population. Sci Rep. (2018) 8:6111.
52. Payne R, Muenchhoff M, Mann J, Roberts HE, Matthews P, Adland E, et al. Impact of HLA-driven HIV adaptation on virulence in populations of high HIV seroprevalence. Proc Natl Acad Sci USA. (2014) 111:E5393–400.
53. Raulet DH. Missing self recognition and self tolerance of natural killer (NK) cells. Semin Immunol. (2006) 18:145–50. doi: 10.1016/j.smim.2006.03.003
54. Alter G, Rihn S, Walter K, Nolting A, Martin M, Rosenberg ES, et al. HLA class I subtype-dependent expansion of KIR3DS1+ and KIR3DL1+ NK cells during acute human immunodeficiency virus type 1 infection. J Virol. (2009) 83:6798–805. doi: 10.1128/jvi.00256-09
55. Boudreau JE, Mulrooney TJ, Le Luduec JB, Barker E, Hsu KC. KIR3DL1 and HLA-B density and binding calibrate NK education and response to HIV. J Immunol. (2016) 196:3398–410. doi: 10.4049/jimmunol.1502469
56. Boulet S, Song R, Kamya P, Bruneau J, Shoukry NH, Tsoukas CM, et al. HIV protective KIR3DL1 and HLA-B genotypes influence NK cell function following stimulation with HLA-devoid cells. J Immunol. (2010) 184:2057–64. doi: 10.4049/jimmunol.0902621
57. Song R, Lisovsky I, Lebouche B, Routy JP, Bruneau J, Bernard NF. HIV protective KIR3DL1/S1-HLA-B genotypes influence NK cell-mediated inhibition of HIV replication in autologous CD4 targets. PLoS Pathog. (2014) 10:e1003867. doi: 10.1371/journal.ppat.1003867
58. Habegger de Sorrentino A, Sinchi JL, Marinic K, Lopez R, Iliovich E. KIR-HLA-A and B alleles of the Bw4 epitope against HIV infection in discordant heterosexual couples in Chaco Argentina. Immunology. (2013) 140:273–9. doi: 10.1111/imm.12137
Keywords: CD57+/NKG2Chigh NK cell, HIV, natural resistance, men who have sex with men, Highly exposed seronegative, cytotoxicity
Citation: Flórez-Álvarez L, Blanquiceth Y, Ramírez K, Ossa-Giraldo AC, Velilla PA, Hernandez JC and Zapata W (2020) NK Cell Activity and CD57+/NKG2Chigh Phenotype Are Increased in Men Who Have Sex With Men at High Risk for HIV. Front. Immunol. 11:537044. doi: 10.3389/fimmu.2020.537044
Received: 21 February 2020; Accepted: 14 August 2020;
Published: 11 September 2020.
Edited by:
Christian Körner, Heinrich-Pette-Institut, Leibniz-Institut für Experimentelle Virologie, GermanyReviewed by:
R. Keith Reeves, Harvard Medical School, United StatesQuirin Hammer, Karolinska Institutet (KI), Sweden
Costin Tomescu, Wistar Institute, United States
Copyright © 2020 Flórez-Álvarez, Blanquiceth, Ramírez, Ossa-Giraldo, Velilla, Hernandez and Zapata. This is an open-access article distributed under the terms of the Creative Commons Attribution License (CC BY). The use, distribution or reproduction in other forums is permitted, provided the original author(s) and the copyright owner(s) are credited and that the original publication in this journal is cited, in accordance with accepted academic practice. No use, distribution or reproduction is permitted which does not comply with these terms.
*Correspondence: Wildeman Zapata, wildeman.zapatab@campusucc.edu.co; wzbuiles@gmail.com