- University of Bordeaux, EPOC, UMR 5805, Pessac, France
This study evaluated the vulnerability of early life stages of native oysters (Crassostrea gigas) from the Arcachon Bay (SW, France) to pollutants at risk in the lagoon in particular copper and S-metolachlor. Developmental abnormalities in wild and cultivated oyster D-larvae were investigated during 2 breeding-seasons (2013 and 2014) at different sampling sites and dates. In addition, copper, and metolachlor concentrations were determined both in seawater and in mature oysters. Bioaccumulation of Cu was observed at higher levels in wild than in farmed specimens. Metolachlor was accumulated at much lower levels. After 24 h exposure, significant increases of the percentage of abnormal D-larvae were observed when exposed at 1 μg L−1 of copper or 10 ng L−1 of metolachlor in comparison with the controls whatever the date, the site, and rearing conditions of the genitors. The current study demonstrates that environmental concentrations of copper and metolachlor can induce a significant increase of developmental abnormalities in farmed and wild populations of oysters. However, no significant differences of sensitivity were observed according to temporal, spatial parameters, and lifestyle of genitors. In addition, oyster larvae obtained from the hatchery displayed the same sensitivity to pollutants as larvae from the field, demonstrating their suitability for toxicity assays and water quality monitoring.
Introduction
Bivalve mollusks such as oysters, mussels, and clams contribute significantly to world aquaculture and the Pacific oyster Crassostrea gigas (Thunberg) is ranked number one in terms of world aquaculture production with 555,913 tons estimated in 2013 (FAO, 2010). The Arcachon Bay is an area of high ecological productivity, linked to various socio-economic activities (oyster-farming, fishing, boating leisure, etc.). Regarding oyster farming, the total production of marketable oysters reached 7,000 tons (6.9% of the French production) in 2012 (CNC, 2012). In this lagoon, oysters are grown either by off-bottom farming i.e., breeding in bags on trestles held above the seafloor (cultivated oysters) or by traditional on-bottom production from wild oyster reefs (wild oysters). To date no study has been conducted to compare the sensitivity of wild and farmed populations while they both contribute to larval recruitment in the bay. Since 1998, several years have been particularly unfavorable for oyster larval recruitment and several episodes of high mortality of oyster spat have been recorded in the Arcachon Bay. In many countries worldwide, the occurrence of significant mass mortalities in both adult and juvenile C. gigas populations has been reported, generally during the summer months (Mori, 1979; Perdue et al., 1981; Watermann et al., 2008; Cotter et al., 2010; Pernet et al., 2011). Although the mass mortality phenomenon encountered in recent years is often associated with infectious agents, it is also often related to environmental changes and highlights the delicate balance that governs an ecosystem. Coastal ecosystems such as Arcachon Bay, are under constant increase of anthropic pressure. The quality of coastal waters has been particularly deteriorated in recent years as the population and human activities have increased (Newton et al., 2003). These areas have become over the years the recipient of many contaminants generated by human activities and are exposed to a wide variety of natural and anthropogenic stressors (Adams, 2005). Exposure to pollutants could at least partly account for the reduction in early life stages and adult oyster survival. Among the large variety of pollutants that can contribute to aquatic pollution, pesticides have been widely recorded in waters and sediments from various estuaries, coastal areas, and lakes and other surface waters that support aquatic life (Gilliom, 2007). The pesticide contamination in the Arcachon bay is widely dominated by S-metolachlor at around 10 ng L−1 and its metabolites (Auby et al., 2007; Budzinski et al., 2011). The Arcachon bay is indeed the final recipient of several rivers draining a watershed of 4,138 km2 mainly dominated by agriculture and urban areas. Moreover, copper (Cu) is among the most toxic metal for bivalve larvae (His et al., 1999). Throughout the world, Cu is used in antifouling paint (Turner, 2010). The Cu mean concentration in seawater of the French Atlantic coast was 0.7 μg L−1 at the early 2000 (Geffard et al., 2002). The most recent data in the Arcachon bay indicate concentration above 1 μg L−1 (Mai et al., 2012; Gamain et al., 2016). Copper bioaccumulation has been monitored in oysters from the Arcachon bay since 1983. Results show an increase from around 100 mg Kg−1 dry weight in 1983 to around 400 mg Kg−1 dry weight in 2015 (ROCCH, 2015). We have previously shown that D-larvae, obtained from hatchery oysters, exposed to environmental doses of copper, or metolachlor have abnormal development (Mai et al., 2012, 2013; Gamain et al., 2016). Our objectives here were 2-fold (1) determine if the temporal and spatial parameters or lifestyle of the broodstock oyster of the Arcachon Bay affect the sensitivity of the D-larvae to pollutants and (2) assess if offsprings from adult oysters from the Arcachon Bay which have been living all their life in a Cu and S-metolachlor contaminated area, were more tolerant or not than those from hatchery.
Materials and Methods
Chemicals and Seawater
Reference toxicants (CuSO4, 5 H2O, and S-metolachlor) and formalin were purchased from Sigma–Aldrich Chemical (St. Quentin Fallavier, France). For experiments with oysters from hatchery, seawater was collected just outside the Arcachon Bay (SW France) near the Banc d'Arguin. For experiments with oysters from the Arcachon bay, water was collected at Grand Banc in 2013 and Arguin in 2014 (Figure 1). Salinity was measured at 33 u.s.i. in Grand Banc and Arguin using a salinity probe (Wissenschaftlich Techische Werkstätten Multi 340i, sonde TetraCon 325). Immediately after sampling, seawater was filtered using membrane filter of 0.45 μm and then 0.2 μm (Xilab) to eliminate debris and microorganisms. Filtered seawater (FSW) was stocked at 4°C in the dark and was used within 3 days. A few hours before experiment, FSW was filtered again at 0.2 μm. This reference water was chemically analyzed for pollutant concentration determination.
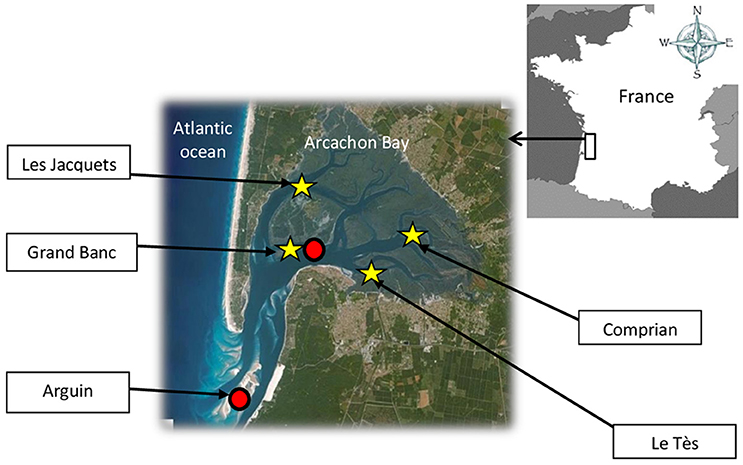
Figure 1. Sampling site for mature oysters (stars) and water (ring) in the Arcachon Bay (SW France).
Animals
Adult wild and cultivated oysters (C. gigas, Thunberg, 1793) were collected during the natural reproduction period from four different sites in the Arcachon Bay: Le Tès and Grand Banc in summer 2013 (July 10, July 22, August 6), Comprian and Les Jacquets in summer 2014 (June 23, July 8, July 15, July 29, August 11; Figure 1 and Table 1). Individuals of three-year-old were sampled for cultivated oysters. For wild oysters, individuals of equivalent size to cultivated oysters were collected (90.8 mm ± 14.1 of length; 48.4 mm ± 5.7 of width). Animals were brought back to the laboratory in tanks filled with seawater from the same site and were kept overnight in this water aerated by air bubbling at a regulated temperature of 12°C. Oysters from hatchery came from a commercial hatchery specialized in the year-round production of mature oysters (Guernsey Sea Farms, UK). Oysters were kept at around 10°C for transportation and then acclimatized in FSW before the beginning of experiments. All oysters were used immediately.
Preparation of Metal and Pesticide Solutions
The range of experimental concentrations was chosen on the basis of preliminary studies (Mai et al., 2012, 2014). The metal and pesticide solutions were made up from analytical grade copper sulfate (CuSO4, 5H2O) and S-metolachlor. Working solutions were obtained diluting the stock solutions (100 mg L−1 for copper and 250 mg.L−1 for metolachlor prepared in pure Milli-Q-water) in FSW (collected at one site for each sampling date). Environmental concentrations in the Arcachon bay were selected for test concentrations of copper (1 μg L−1) and S-metolachlor (10 ng L−1). Three concentrations of copper (1, 10, and 50 μg L−1) and S-metolachlor (10, 100, and 1,000 ng L−1) were selected for calculation of EC50.
Chemical Analysis
Reference Sea Water and Contamination Solutions
The working solutions were chemically analyzed for pollutant concentration confirmation. For chemical analysis of metals, three replicats of about 10 mL of each sample (stock solutions and FSW) was acidified with 5% final of nitric acid (Nitric acid 65%, Fluka). Samples were then analyzed by Inductively-Coupled Plasma Optic Emission Spectrometry (ICP-OES 720, Agilent Technologies, Arcachon, France). The validity of the method was periodically checked with Tort-2 and Dolt-4 certified materials (lobster hepatopancreas and dogfish liver from NRCC-CNRC, Ottawa, Canada) and values obtained were within the certified ranges. Quantification limit was 0.3 μg L−1.
Metolachlor level was determined using a HPLC/MS/MS system from Agilent Technologies company (HPLC 1290 system coupled to 6460 mass spectrometer; mobile phase: water (5 mM ammonium acetate + 0.1% acetic acid)/methanol (100/0%–0/100% within 17 min at 0.5 mL/min); column: Kinetex C18 100 mm (length), 2.1 mm (internal diameter), 1.7 mm (porosity); ionization mode: ESI +). One microliter of each individual solution was mixed with 50 μL of mixture of internal standards (metolachlor d6) in methanol (nominal concentrations 10 ng L−1) and were directly analyzed. Procedural blanks (three blanks between each sample) were performed to ensure the absence of laboratory-contamination. Moreover, it ensured optimal cleaning of the chromatographic system between each sample and allowed to achieve detection limits as low as possible while avoiding cross-contamination. Metolachlor was quantified using a quantification transition. Its presence is attested through a transition confirmation but also thanks to the relationship between abundance and the quantification of transition. Recovery and reproducibility were determined using spiked water samples (at nominal concentration of 10 ng L−1) processed at the same time as the samples to be characterized. Detection and quantification limits were, respectively of 0.6 and 1.9 ng L−1.
Oysters
For the determination of pollutant body burden, the whole body of 10 oysters was dissected carefully, drained on absorbent paper, and then ground at 8,000 rounds min−1 and 9,500 rounds min−1 (Ultra Turrax T2S, Janke and Kunkel, IKA Labortechnik). The homogenate obtained was distributed in four polypropylene tubes: two were used for the analysis of organic contaminants and two other for the analysis of metal contaminants. These tubes were then frozen at −20°C before analysis.
For metal concentration analysis, ground oyster samples were thawed and incubated 48 h at 45°C to remove water. In this way, the results are expressed relative to the dry weight. The lyophilized samples of oysters tissues were first digested in 3 mL of pure HNO3 in a pressurized medium at 100°C for 3 h (hot block CAL 3300, Environmental Express, USA). Samples were then diluted up to 18 mL with ultra-pure water (Milli-Q, Bedford, MA, USA). Cu content was then measured by Inductively-Coupled Plasma Optic Emission Spectrometry (ICP-OES 720, Agilent Technologies) on three replicats per sample. Metal concentration in dry tissues was expressed in mg kg−1 dry weight (d.w.). For Cu, the validity of the method was periodically checked with Tort-2 and Dolt-4 certified materials (lobster hepatopancreas and dogfish liver from NRCC-CNRC, Ottawa, Canada) and values obtained were within the certified ranges. The limits of detection for Cu was 0.5 mg.kg−1.
Before pesticide analysis, samples were thawed and weighed to obtain fresh weight. Samples from the same site and same lifestyle (wildversus cultivated) but from the different sampling date were pooled in 2013 and 2014. The samples are then refrozen and then lyophilized and weighed to obtain the dry weight. The following different compounds were analyzed in oyster extracts: 1-(2,4-Dichlorophenyl) urea, 1-(3,4-Dichlorophenyl) urea, 1-(3,4-Dichlorophenyl)-3-methyl urea, acetochlor, acetochlor ESA, acetochlor OA, alachlor, amethryn, atrazine, atrazine 2 hydroxy, azoxystrobine, bentazone, carbendazime, carbetamide, carbofuran, carbosulfan, chlorotoluron, chlorsulfuron, cyanazine, cyromazine, deséthylatrazine, atrazine desisopropyl, diflufenican, dimetachlor, diuron, DMSA, DMST, flazasulfuron, fluazifop-p-butyl, flusilazole, hexazinone, hydroxy simazine, imidacloprid, irgarol, isoproturon, linuron, metazachlor, methiocarb, metolachlor, metolachlor ESA, metolachlor OA, metoxuron, metsulfuron-methyl, nicosulfuron, promethryn, propachlor propazine, propiconazole, prosulfuron, pymethrozine, quizalofop-ethyl, quizalofop-efuryl, simazine, terbutryn, terbutylazine, terbutylazine desethyl, thiamethoxan. These compounds are representative of those commonly found in the waters of the Bay of Arcachon. They were analyzed by LC/MS /MS using an MRM method in peak to peak, associated with a solid phase extraction protocol SPE (Solid Phase Extraction). 0.5 g of the studied matrix was spiked with an internal standard (alachlor d13, atrazine 2 hydroxy d5, atrazine d5, bentazone d7, carbendazime d3, carbetamide d5, carbofuran d3, chlorotoluron d6, DEA-d7, DIA d5, diuron d6, hexazinone d6, imidacloprid d4, irgarol d9, isoproturon D6, linuron d6, metolachlore d6, metoxuron d6, metoxuron d6, metsulfuron-methyl d3, nicosulfuron d6, promethryn d14, Propazine D14, Propazine D14, quizalofop-ethyl d3, quizalofop-ethyl d3, simazine d10, terbutryn D5, terbutylazine d5, terbutylazine desethyl d9, thiamethoxan d3) according to the presumed contamination of the matrix.
A triplicate of the first sample of the series was made in order to overcome the variability of the manipulator. To test the efficiency of the extraction, supplemented sample was used. In all these samples, a solvent mixture (methanol) and water acidified at pH 2 is added in the proportion of 12 mL. A blank protocol containing only solvent (methanol and water at pH 2) allowed us to characterize the contamination that could be recovered in the machine. All samples were prepared by microwave extraction using the mixture methanol/water 50/50 v/v. Once completed, a SPE was performed. The samples were rinsed with methanol. The remaining solvent was removed using the RapidVap Labconco at a temperature of 60°C and a pressure of 700 mbar for 60 min. For the campaign of summer 2014, solvent evaporation was performed in nitrogen flow. Once evaporated, extracts were finally purified by solid phase extraction (SPE). SPE cartridges used for the extraction (Oasis® HLB 3 cc, 60 mg) were successively conditioned with 3 mL of methanol and 3 mL of acidified water at pH 2 (HCl). The passage of liquid through the cartridges was facilitated by applying a vacuum. The sample was then percolated and the analytes were then retained on the receptor phase. The analytes and internal standards were then eluted from the adsorbent with 3 mL of methanol. The eluates recovered in vials were evaporated under nitrogen flux to be transferred into injection vials restrictor 300 μL. The detection and quantification limits were calculated with reports on signal noise above 3 or 9, respectively (Supplementary Materials 1 and 2).
Embryotoxicity Assay
Gametes were obtained by stripping the gonad of mature oysters. Gonad was isolated from the rest of the body, put into a beaker with 0.2 μm FSW and then pressed gently to bring out the mature gametes which were controlled under microscope to select the best cells qualities: highly concentrated and motile spermatozoa and even, pear-shaped oocytes. Eggs and sperm from two individuals were selected to give a single pairing. Sperms and eggs were sieved separately through a 50 and 100 μm meshes (Sefar Nitex), respectively to eliminate debris and feces. Eggs were counted under microscope (LEICA DME) at a magnification of 100. Eggs were fertilized with sperm at ratio of 1:10 (egg/sperm cells) homogenizing with an agitator to prevent polyspermy. Fertilization success was checked under microscope, and embryos were then counted and transferred to 24-well microplates (Greiner Bio-One, Cellstar) for embryotoxicity assays. The embryotoxicity assay protocol has been described in details by His et al. (1997) and Quiniou et al. (2007) and the standardized AFNOR procedure (AFNOR XP-T90-3821) published in 2009. Fertilized eggs (around 500) were exposed in wells containing 2 ml of toxicant solution. The microplates were then incubated at 24°C for 24 h in the dark. After incubation, 25 μl of 37% buffered formalin were added per well and the percentage of abnormal oyster larvae was then recorded. Hundred individuals per well were directly observed under inverted microscope (Nikon eclipse) to determine the number of abnormal D-shaped larvae (including embryos in early or late arrested development or D-larvae showing shell/hinge abnormalities, mantle abnormalities (hypertrophies) or shell, and/or hinge + mantle abnormalities) according to the criteria described in His et al. (1999) and Quiniou et al. (2007). An important prerequisite to validate the test was the presence, in control condition (24°C in the absence of contamination) of <20% of abnormal larvae. In this study, various numbers of couples were used according to the study condition (Table 1) and four replicates were performed for each couple.
Statistical Analysis
All data is expressed as means ± standard deviation (SD). Data were first transformed using the following formula: p = arc sin where p is the percentage of abnormalities, specified in p-values from 0 to 1 (Legendre and Legendre, 1998). Homogeneity of variance (Levene's test) was verified and then statistical comparisons were performed by the Kruskal-Wallis tests. Paired comparisons were then performed using Kruskal post hoc-test. Significance difference between conditions was accepted when p < 0.05. To test the independent effects of the factors “date,” “location,” and “lifestyle” on the variability of the percentage of larval abnormalities, analysis of covariance was used (ANCOVA). For correlation matrix, the XLStat logiciel was used. Correlation was confirmed for a p < 0.001 or <0.05. The relative dispersion measure was calculated using the coefficient of variation (Aerts et al., 2015):
Where σ is average of the values and μ the standard deviation. Non-linear regressions (Hill equation) were used to calculate the EC50-values. These regressions were obtained using the GraphPad Prism software version 5.04 for Windows (GraphPad Software, San Diego, CA, USA). The EC50-values were calculated using the measured contaminant concentrations.
Results
Chemical Analysis of Tested Pollutant Solutions
Table 2 shows Cu and metolachlor concentrations determined in the control condition and in the contamination solutions in 2013 and 2014. Analyses revealed the presence of copper in reference seawaters at concentrations ≤3.5 μg L−1 in 2013 and ≤2 μg L−1 in 2014. For seawater spiked with Cu at 1 μg L−1, the measured Cu concentrations varied from 3.8 to 4.2 μg L−1 and from 2.1 to 3.8 μg L−1 in 2013 and 2014 respectively. Analyses also revealed the presence of metolachlor in reference seawaters at very low concentrations in 2014 (4.3 ± 1.3 ng L−1). In 2013, higher metolachlor concentrations were measured up to (17.8 ± 8.5 ng L−1). Moreover, higher variability was observed between sampling dates and sites in 2013 compare to 2014. For instance, the highest metolachlor concentration was found for the date 7/10/13 with 30 and 27 ng L−1 for Le Tès and Grand Banc respectively (data not shown). For seawater spiked with 10 ng L−1 metolachlor, the measured concentrations varied between 14 and 44 ng L−1 depending on the background levels of the reference FSW.
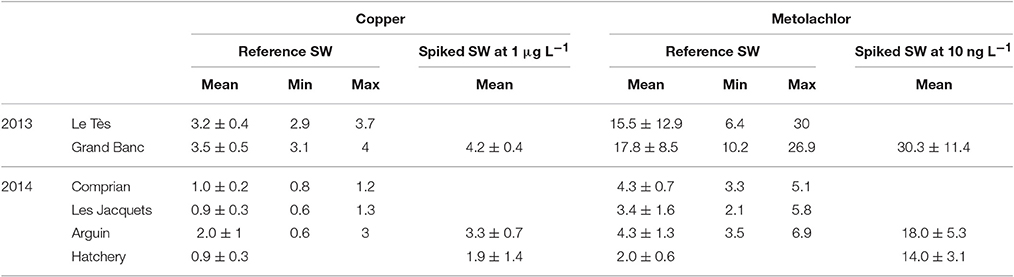
Table 2. Copper (μg L−1) and metolachlor (ng L−1) concentrations determined in the reference FSW and in spiked FSW during summer 2013 and 2014.
Analysis of reference seawater from Arguin used for oyster hatchery experiments revealed the presence of copper at concentrations of 0.9 ± 0.3 μg L−1 (Table 2). For the 1 μg L−1 spiked seawater, the measured concentration reached 1.9 ± 1.4 μg L−1 which is higher than expected since it also included the Cu initially present in the reference seawater. Background metolachlor levels in this reference seawater used for the oyster hatchery experiments was low, in average 2 ± 0.6 ng L−1. For the 10 ng L−1 spiked water, measured metolachlor concentration was 14 ± 3.1 ng L−1 in agreement with the expected value.
Bioaccumulation
Copper Bioaccumulation
Copper concentrations in oyster tissues were lower in 2013 compared to 2014 keeping in mind that the sampling sites were different between the 2 years (Figure 2).
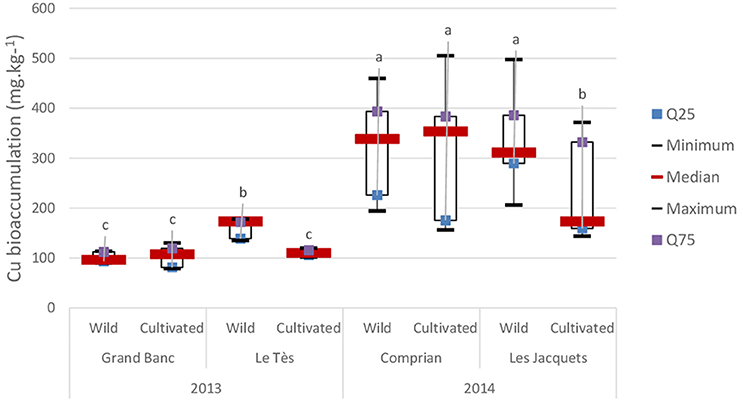
Figure 2. Copper bioaccumulation (mg Kg−1) in adult oysters from different locations in the Arcachon Bay. Box plots represent the minimum, maximum, median, 1st quartile (Q25), and 3rd quartile (Q75) concentrations for 30 individuals per condition in 2013 and 50 individuals in 2014. Data are expressed in mg Kg−1 dry weight ± SD.
In 2013, wild oysters collected at “Le Tès” have bioaccumulated significantly more copper that oysters collected at “Grand Banc.” Moreover, cultivated oysters from “Le Tès” have bioaccumulated significantly less Cu with an average of 110.8 ± 5.9 mg kg−1 than wild oysters with an average of 161.9 ± 19 mg kg−1 (Figure 2).
In 2014, higher Cu bioaccumulation was observed in wild oysters collected at “Les Jacquets” (median of 311 mg kg−1) compared to cultivated ones (median of 173 mg kg−1; Figure 2), with one exception for cultivated oysters sampled at Comprian 07/15/14 that reach the highest Cu concentration with 473 mg kg−1 (data not shown). The most Cu contaminated oysters were found at the two last sampling dates in 2014 (356 ± 28 mg kg−1 in July 29 2014 and 397 ± 48 mg.kg−1 in August 11 2014) regardless of the site or the lifestyle (data not shown). This maximum Cu concentration was obtained just after the spawning peak 07/20/2014.
Pesticide Bioaccumulation
This study monitored more than 50 pesticides residues in oyster tissues. But few pesticide compounds and low concentrations were detected during these two campaigns. Irgarol, metolachlor, and its metabolite metolachlor OA were the main chemicals detected in oysters (Figure 3). There is no significant concentration difference for each detected compound whatever the site or the sampling date considered. While in 2013 only three pesticides were detected: irgarol, metolachlor and metolachlor OA, in 2014, seven pesticides were detected with additional compounds: alachlore, azoxystrobine, dimetachlor, and terbuthylazine. Strikingly, metolachlor OA was only found in cultivated oysters with a concentration between 7.3 and 16.9 ng g−1 but not in wild oysters whatever the date and the sampling site.
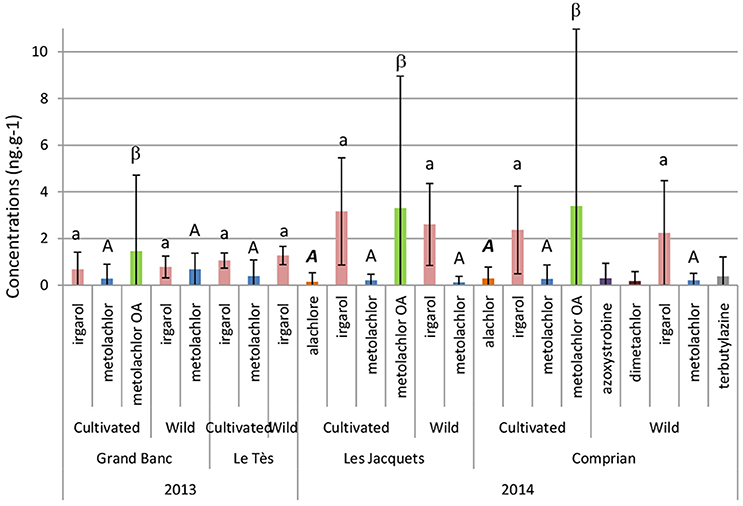
Figure 3. Pesticide bioaccumulation (ng g−1) in summer 2013 and 2014 in adult oysters. Data represent the average concentrations in ng g−1 dry weight ± SD of the 3 sampling dates in 2013 and the 5 sampling dates in 2014. Each sample represents a pool of 10 oysters. Statistical analysis was performed on each individual pesticide.
Larval Abnormalities
In the experiments with copper, the percentage of larval abnormalities in controls range from 4 to 29% with an average of 14.5 ± 5.1% for oysters from the Arcachon bay (Figure 4). The highest background level of abnormalities was observed for cultivated oysters at Grand Banc with an average of 18.2 ± 2.1%. For oysters from hatchery values range from 8 to 23% with an average of 14 ± 4.6%. After a 24 h exposure to 1 μg L−1 of copper, a significant increase (p < 0.05) of the percentage of abnormal D-larvae was observed in all studied conditions in comparison with the control (Figure 4) excepted for the cultivated oysters in Grand Banc in 2013. The median percentage of larval abnormalities over the 2 years sampling period was 15% for control larvae and 35% for larvae exposed to 1 μg L−1 of Cu (data not shown). The highest abnormal larval rate was observed for cultivated oysters from Le Tès (50.2 ± 15.9%). The analysis of dose-response data for copper exposure (Table 3) showed that the 24 h EC50-values were 9.8 μg L−1 for oyster D larvae from hatchery, 6.5 to 10.1 μg L−1 in 2013 and 7.4 to 14.8 μg L−1 in 2014 for oyster D-larvae from the Arcachon Bay depending on their lifestyle.
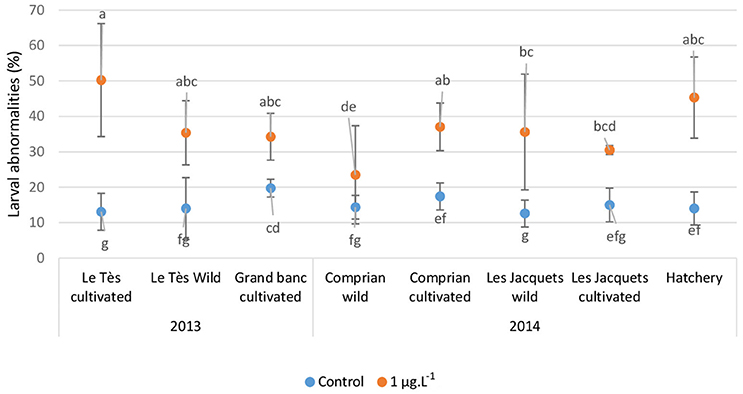
Figure 4. Percentages (Mean ± SD) of abnormal oyster D-larvae following embryo exposure to 1 μg L−1 of copper. Embryos were obtained from either wild or cultivated oysters from the Arcachon bay or oysters from a hatchery. Different letters indicated significant differences between concentrations, dates, or sampling sites (p < 0.05).
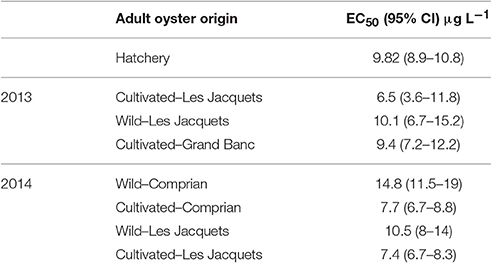
Table 3. EC50-24H values with 95% confidence intervals for oyster D-larvae exposed to copper during summer 2013 and 2014.
In the experiments with metolachlor, the percentage of larval abnormalities in controls range from 2 to 21% with an average of 13.8 ± 4.6% for oysters from the Arcachon bay. For oysters from hatchery, values range from 10 to 22% with an average of 12.1 ± 2.8% (Figure 5). After a 24 h exposure to 10 ng L−1 metolachlor, a significant increase (p < 0.05) of the percentage of abnormal D-larvae was observed in all conditions in comparison with the control (Figure 5). A median percentage of larval abnormalities over the 2 years sampling period was 15% for control larvae and 38% for metolachlor-exposed larvae (data not shown). It should be noted that it was not possible to estimate a reliable EC50−value for metolachlor exposure because the highest tested metolachlor concentration (1000 ng L−1) did not exceed the 50% effect.
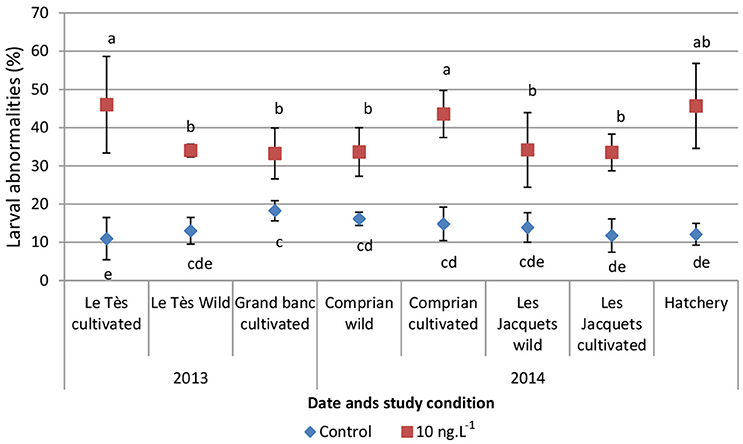
Figure 5. Percentages (Mean ± SD) of abnormal oyster D-larvae following embryo exposure to 10 ng L−1 of metolachlor. Embryos were obtained either from wild or cultivated oysters from the Arcachon bay or oysters from a hatchery. Different letters indicated significant differences between concentrations, dates, or sampling sites (p < 0.05).
Relationship between Developmental Abnormalities and Lifestyle and Location or Date of Sampling
Developmental abnormality frequency was not correlated to the lifestyle of brood stocks except for wild vs. cultivated oysters from Le Tès exposed to 10 ng L−1 of metolachlor (p < 0.0001). No more correlation was observed to the location or date of sampling (Table 4).
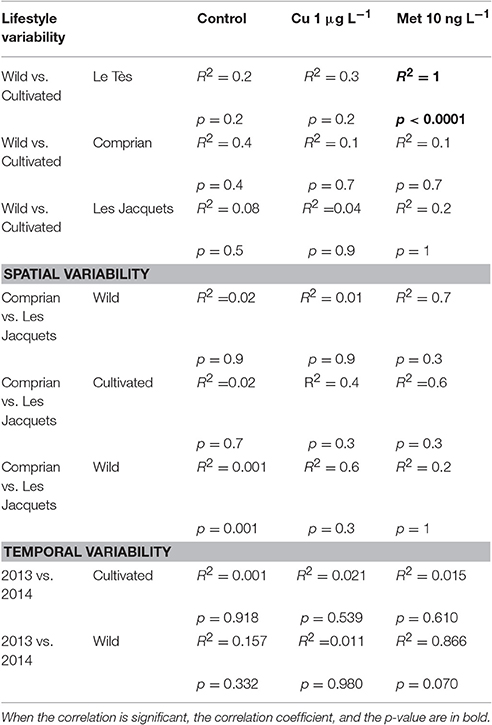
Table 4. Results of the correlation matrix between developmental defects and temporal, spatial and lifestyle ok brood stock in control conditions or in the presence of 1 μg L−1 of copper or 10 ng L−1 of metolachlor.
Discussion
Water Reference Chemistry and Background Embryotoxicity
Anthropogenic activities, such as the growing use of copper as an antifouling (Claisse and Alzieu, 1993), have resulted in copper becoming increasingly prevalent in coastal ecosystems. The most recent data in the Arcachon bay indicate a mean copper concentration of 0.8 and 1.1 μg L−1 for water collected in April 2011 and October 2013, respectively (Mai et al., 2012; Gamain et al., 2016). In the present study, copper analysis revealed higher concentrations in the seawater sampled in summer 2013 with an average concentration of 3.5 μg L−1 compare to summer 2014 where an average concentration of 1.2 μg L−1. However, in 2014 higher levels were recorded in mid-July to mid-August at the entrance of the bay (Arguin), exceeding the usual value by 3- to 4-fold (data not shown). It could likely be related to the increased Cu inputs in summer time due to the increased boating activity at Arguin and to the enclosed geometry of this site (surrounded by numerous sandbanks). Actually, copper mean concentration in sea water collected in Arguin outside of summer time was 0.9 ± 0.3 μg L−1. Interestingly, the larval abnormalities obtained for all control conditions did not show significant differences (14 ± 4.8 and 13 ± 5.2% for oysters from the Arcachon bay or oyster from hatchery respectively), although the reference seawaters sampled at different dates and locations were differently contaminated with copper and oysters were from different origins.
Metolachlor is one of the most intensively used herbicides in agriculture in the watershed of the Arcachon bay and the most abundant in the water column (Auby et al., 2007; Budzinski et al., 2011). Chemical analyses showed that metolachlor concentration in seawater during summer season was higher in 2013 (16.5 ± 9.8 ng L−1) than in 2014 (4 ± 1.2 ng L−1). These values albeit slightly higher are in accordance with published concentrations ranged from a minimum of 2 ng L−1 to a maximum of 10 ng L−1 for the Arcachon Bay during spring and summer (Budzinski et al., 2011; Mai et al., 2012, 2014; Gamain et al., 2016). Higher concentrations were particularly noticed for the water sample collected the 10th of July 2013 at Le Tès and Grand Banc with 30 and 27 ng L−1 of metolachlor respectively. Metolachlor concentration outside the summer time (hatchery's oysters experiment) was in the same range than in 2014 with a concentration of about 2 ± 1.2 ng L−1. As for Cu experiences, no significant difference was observed in the percentage of larval abnormalities in control condition between oysters from the Arcachon bay and oysters from the hatchery.
Since adult oysters from the Arcachon Bay are chronically exposed to Cu, S-metolachlor, and other pollutants, we can hypothesize they have developed some specific mechanisms to withstand the toxic effects of those contaminants. A previous field study has shown the enhanced metal tolerance of Crassostrea sikamea offspring from heavily contaminated sites (Weng and Wang, 2014). Genetic and epigenetic studies are in progress to determine if there was any genetic component to the higher pollutant tolerance of C. gigas from the Arcachon Bay.
Influence of Lifestyle and Sampling Date on Pollutant Bioaccumulation
In addition to the water column chemistry, health of aquatic environments can be monitored using bivalves organisms, particularly mussels, and oysters (Lauenstein, 1995). Because of their biological and ecological characteristics (e.g., capacity of bioaccumulation, worldwide distribution, abundance, and sessile way of life), bivalves are among the best bioindicator species for pollution biomonitoring (Bustamante et al., 2012; Luo et al., 2014). Oysters are filter-feeders which can bioaccumulate and therefore strongly concentrate a large variety of pollutants. Copper concentrations in oyster tissues were significantly lower in 2013 compared to 2014 (119.1 ± 29 and 298.4 ± 103.9 mg kg−1, respectively) keeping in mind that the sampling sites were different between the 2 years. Cu concentrations in 2014 were higher than the Arcachon Bay median value which was estimated at 206 mg kg−1 for the period 2003–2007 (ROCCH, 2015). In recent years, concentrations of around 400 mg.kg−1 dry weight (Ifremer, 2014) were reached in the Arcachon Bay. Our data are in accordance with the data from ROCCH (2015) and confirmed the temporal increase of copper bioaccumulated in oysters from the Arcachon bay. For C. gigas, dissolved copper in ambient water, as opposed to copper in phytoplankton food and sediment, has been shown to be the most important source of copper in tissues (Ettajani and Amiard, 1995). However, in the present study, the correlation between the copper concentration in oyster tissues and in the water column is weak. Actually, water contamination is highly variable and the point-sampling approach used in this study likely miss most of episodic pollution events in contrast to oysters which integrate pollution over time. Wild oysters have accumulated more copper than cultivated oysters. Wild oysters in contrast to cultivated ones are present in the same environment and probably accumulate pollutants over their all life. Furthermore, unlike wild oysters living in the water-sediment interface, farmed oysters are cultured in bags laid across trestles, which may cause differences in accumulated metals due to difference in metal bioavailability and oyster feeding behavior.
In 2013, wild oysters collected at “Le Tès” have bioaccumulated more copper that oysters collected at “Grand Banc.” Le Tès is located inside the lagoon and retention time of water and pollutants is more elevated at this site (Plus et al., 2009). In 2014, a higher Cu accumulation was observed for the last two sampling dates (July 29 and August 11) for wild and cultivated oysters (data not shown). This can be likely explained by the decrease of oysters' body weight after spawning. Indeed, the main spawning episode in the Bay was recorded the 20th of July (Ifremer, 2014). A total of 7 of the 53 pesticides and their metabolites analyzed were detected in the tissues showing that these molecules can be directly bioaccumulated by oysters. Bivalves accumulate pesticides primarily by filtering water, initially storing such compounds in the gills (Gunkel and Streit, 1980). Pesticide column accumulation depends both on their concentration in the water and on their octanol–water partition coefficient (Muñoz and Rosés, 2000). Since 2010, the REPAR network has monitored a wide range of pesticide molecules in the Arcachon Bay but also in the tributaries (Budzinski et al., 2011). Total average concentration of the 57 measured pesticides was 45 ± 31 ng L−1 in Arguin and 95 ± 7 8 ng L−1 in Grand Banc in 2010 (Budzinski et al., 2011). Antifouling biocides such as irgarol, diuron, and its metabolite DCPMU (1-(3,4-dichlorophenyl)-3-methylurea), as well as DMSA (Dimercaptosuccinic acid) and DMST (N,N-Dimethyl-N'-p-tolylsulphamide) were detected in the Arcachon Bay mostly at low concentrations <10 ng.L−1 (Budzinski et al., 2011; Fauvelle et al., 2012). The most abundant pesticides were metolachlor and alachlor with a dominance of their metabolites (Auby et al., 2007; Budzinski et al., 2011). Relatively low pesticide concentrations were detected in oyster tissues in 2013 and 2014 (<10 ng g−1 dw). Higher concentrations of irgarol (2.6 ± 1.9 and 0.9 ± 0.5 ng g−1, respectively) and metolachlor OA (3.3 ± 6.1 and 1.5 ± 3.2 ng g−1, respectively) and a broader variety of compounds (alachlore, azoxystrobine, dimetachlor, and terbutylazine) were detected in 2014 than in 2013. Interestingly, the metolachlor OA was never detected in wild oysters but it was measured in cultivated oysters. One explanation could be the absence of S-metolachlor accumulated in sediments of the Arcachon bay (Auby et al., 2007) and the main contamination of oysters by the water column.
Very few studies have investigated currently used pesticides which are far less hydrophobic than legacy pesticides. In most of recent studies, no pesticide is found in the flesh of oysters on the field but some exposure experiments have already demonstrate differential accumulation of various pesticides in oyster (Buisson et al., 2008). For instance, none of the acetanilide herbicides were detected in oysters despite their high concentration in the water (up to 60 ng L−1) of Chesapeake Bay in Maryland (Lehotay et al., 1998). In contrast, diuron was very rapidly bioaccumulated in oyster soft tissues after a short exposure to high concentrations (Luna-acosta et al., 2012). Our results showed that oysters sampled in the summer 2014 have bioaccumulated more organic and inorganic pollutants than in the summer 2013. However, the amount of copper and pesticides accumulated in soft tissues of oyster genitors from the Arcachon Bay does not seem to alter embryo development of offspring at least till D-larvae stage. In fact, although oysters were more contaminated in 2014, embryotoxic effects were obtained at lower concentrations for both contaminants with an increase by a factor 2–5 between control condition and environmental concentrations. There is no significant difference of embryotoxic effects between 2013 and 2014 whatever the site or the contaminant. Analysis of covariance (ANCOVA) showed that only 20.8 and 8.6%, respectively of the variability of the percentage of larval abnormalities in the absence of added contamination is explained by sampling condition (date and location) and lifestyle in 2013 and 2014, respectively. Following Cu exposure 26.5 and 9.4% of larval abnormality variability is explained by sampling condition (date and location) and lifestyle in 2013 and 2014, respectively and following metolachlore exposure, 25.2 and 55.5% is related in 2013 and 2014, respectively. So the rest of the variability could be related to inter-individual variability, toxic effects of other pollutants, etc. To explain these results, genetic studies are underway on oysters from the 2014 sampling.
Effects of Spatial, Temporal Parameters, and Lifestyle of Oyster Genitors on Offspring Development
Besides, water contaminants can be filtered by adult oysters without being bioaccumulated. These pollutants could also interfere with the physiology of genitors and notably their capacity to produce normal D-larvae. In control condition, no significant difference was observed between the different origins of oyster studied. However, in summer 2013, adult oysters were collected in a seawater four times more contaminated with metolachlor (16.5 ± 9.8 ng L−1) than during summer 2014 (4.0 ± 1.2 ng L−1). It can be assumed that oysters collected in 2013 could have developed molecular or physiological adaptations which would allow the progeny to be more resistant to contaminant exposure. This hypothesis could explain the similar percentage of larval abnormalities in 2013 and 2014 while concentration of metolachlor was significantly different with a shift from 30.3 ± 11.4 to 18 ± 5.3 ng L−1, respectively.
For exposure at lower pollutant concentrations, the response in term of developmental defect is enhanced with oyster from hatchery compared to native oysters. Indeed, the mean larval abnormalities for hatchery oysters is almost always the highest although not statistically significant due to large variations between oyster couples. Hatchery oysters could seem more sensitive than oysters from Arcachon but when considering the whole range of copper concentrations, the Cu-EC50 values were in the same range for larvae from hatchery and from the Arcachon bay (9.82 and 6.5–10.1 μg L−1 in 2013 or 7.4–14.8 μg L−1, respectively). Alltogether, oyster genitors from the hatchery are a good alternative to cultivated oysters from the Arcachon Bay to perform the embryo-larval assay. Moreover, results of EC50 suggest that cultivated oysters are slightly more sensitive to copper contamination than wild oyster in 2013 and 2014 (no statistical comparisons was possible as the number of couples was insufficient). In 2014, when the embryos were exposed to Cu, the 24 h EC50-values from wild oyster genitors were up to 1.9-fold higher than those from cultivated ones. This suggested that environmental exposure history of adult oysters can influence the ability of their offspring to manage with metal exposure.
Correlation matrices were used to study the relationships between lifestyles and sampled locations. Overall, there was no correlation between background level of developmental defects in the progeny and location and date of sampling or lifestyle of oysters. However, following metolachlor exposure, the percentage of larval abnormalities between cultivated and wild oysters from Le Tès was positively correlated (p < 0.0001).
Conclusion
This study demonstrated that low environmental concentrations of copper, and metolachlor can induce developmental abnormalities in the progeny of farmed and wild populations of oysters from the Arcachon bay. Bioaccumulation of Cu, and to a lesser extent metolachlor, was observed in oysters from the Arcachon Bay and Cu concentration was higher in wild oysters than in cultivated ones. The sensitivity of D-larvae to pollutants was not significantly correlated to the spatial and temporal parameters or lifestyle of genitors. However, there is a trend showing a better tolerance to copper of oyster D-larvae produced using wild oysters from contaminated sites. Indeed, copper contamination is constantly increasing in oysters from the Arcachon bay and this could be a threat for oyster farming in the lagoon. Oyster larvae from hatchery respond to pollutant exposure in a same manner and with the same sensitivity than larvae obtained from the Arcachon Bay. Oyster genitors from hatchery are thus a good alternative to oysters from the field to perform the embryo-larval assay.
Author Contributions
JC has co-coordinated the study and corrected the paper. PeG has carried out the experiment on oyster larvae and has written the paper. PaG has performed oyster and water sampling and has coorected the paper. HB has performed organic chemical analyses. PYG has performed metal analyses. BM has co-coordinated the study and corrected the paper.
Conflict of Interest Statement
The authors declare that the research was conducted in the absence of any commercial or financial relationships that could be construed as a potential conflict of interest.
Acknowledgments
The authors thank the Aquitaine Region (OSQUAR Project), CPER A2E, Intermunicipal Union of Arcachon Bay (SIBA), and Water Agency Adour Garonne (AEAG) for their financial supports. We gratefully acknowledge the staff of the SEPANSO for their help to get the reference seawater. This work was part of the LABEX COTE cluster of excellence “Continental To coastal Ecosystems: evolution, adaptability and gouvernance.” The authors would like to thank James Emery for providing English proofreading services and Danielle Maurer and Isabelle Auby for their scientific and technical advices.
Supplementary Material
The Supplementary Material for this article can be found online at: http://journal.frontiersin.org/article/10.3389/fmars.2017.00058/full#supplementary-material
Footnotes
1. ^AFNOR XP T 90-382, 209. Water quality - Bioindicator of potential toxicity in aqueous media - Determination of the potential toxicity of aqueous samples on bivalves embryo-larval development.
References
Adams, S. M. (2005). Assessing cause and effect of multiple stressors on marine systems. Mar. Pollut. Bull. 51, 649–657. doi: 10.1016/j.marpolbul.2004.11.040
Aerts, S., Haesbroeck, G., and Ruwet, C. (2015). Multivariate coefficients of variation: comparison and influence functions. J. Multivar. Anal. 142, 183–198. doi: 10.1016/j.jmva.2015.08.006
Auby, I., Bocquene, G., Quiniou, F., and Dreno, J. P. (2007). Etat de la Contamination du Bassin d'Arcachon par les Insecticides et les Herbicides sur la Période 2005-2006. Impact Environnemental 24:108 (Ifremer). Available online at: http://archimer.ifremer.fr/doc/00000/2398/
Buisson, S., Bouchart, V., Guerlet, E., Malas, J., and Costil, K. (2008). Level of contamination and impact of pesticides in cupped oyster, Crassostrea gigas, reared in a shellfish production area in Normandy (France). J. Environ. Sci. Heal. Part B 43, 655–664. doi: 10.1080/03601230802352732
Bustamante, P., Luna-Acosta, A., Clemens, S., Cassi, R., Thomas-Guyon, H., and Warnau, M. (2012). Bioaccumulation and metabolisation of 14C-pyrene by the Pacific oyster Crassostrea gigas exposed via seawater. Chemosphere 87, 938–944. doi: 10.1016/j.chemosphere.2012.01.049
Claisse, D., and Alzieu, C. (1993). Copper contamination as a result of antifouling paint regulations? Mar. Pollut. Bull. 26, 395–397. doi: 10.1016/0025-326X(93)90188-P
CNC (2012). La production Française. Available online at: http://www.cnc-france.com/La-Production-francaise.aspx
Cotter, E., Malham, S. K., O'Keeffe, S., Lynch, S. A., Latchford, J. W., King, J. W., et al. (2010). Summer mortality of the Pacific oyster, Crassostrea gigas, in the Irish Sea: the influence of growth, biochemistry and gametogenesis. Aquaculture 303, 8–21. doi: 10.1016/j.aquaculture.2010.02.030
Ettajani, H., and Amiard, J. C. (1995). Biodisponibilité de quelques métaux fixés sur les matières en suspension (MES) en milieu estuarien. Hydroécologie Appliquée 7, 75–89. doi: 10.1051/hydro:1995005
FAO (2010). La Situation Mondiale des Pêches et De L'aquaculture - 2010. Département des pêches l'aquaculture la FAO. Organ. des nations Unies pour l'alimentation l'agriculture, Rome.
Fauvelle, V., Mazzella, N., Delmas, F., Madarassou, K., Eon, M., and Budzinski, H. (2012). Use of mixed-mode ion exchange sorbent for the passive sampling of organic acids by polar organic chemical integrative sampler (POCIS). Environ. Sci. Technol. 46, 13344–13353. doi: 10.1021/es3035279
Gamain, P., Gonzalez, P., Cachot, J., Pardon, P., Tapie, N., Gourves, P. Y., et al. (2016). Combined effects of pollutants and salinity on embryo-larval development of the Pacific oyster, Crassostrea gigas. Mar. Environ. Res. 113, 31–38. doi: 10.1016/j.marenvres.2015.11.002
Geffard, O., Budzinski, H., and His, E. (2002). The effects of elutriates from PAH and heavy metal polluted sediments on Crassostrea gigas (Thunberg) embryogenesis, larval growth and bio-accumulation by the larvae of pollutants from sedimentary origin. Ecotoxicology 11, 403–416. doi: 10.1023/A:1021024415695
Gilliom, R. J. (2007). Pesticides in U.S. streams and groundwater. Environ. Sci. Technol. 41, 3408–3414. doi: 10.1021/es072531u
Gunkel, G., and Streit, B. (1980). Mechanisms of bioaccumulation of herbicide (atrazine, s-triazine) in a freshwater mollusc (Ancylus fluviatilis mull.) and a fish (Coregonus fera jurine). Water Res. 14, 1573–1584. doi: 10.1016/0043-1354(80)90061-5
His, E., Heyvang, I., Geffard, O., and De Montaudouin, X. (1999). A comparison between oyster (Crassostrea gigas) and sea urchin (Paracentrotus lividus) larval bioassays for toxicological studies. Water Res. 33, 1706–1718. doi: 10.1016/S0043-1354(98)00381-9
His, E., Seaman, N. M. L., and Beiras, R. (1997). A simplification the bivalve embryogenesis and larval development bioassay method for water quality assessment. Water Res. 31, 351–355. doi: 10.1016/S0043-1354(96)00244-8
Newton, A., Icely, J. D., Falcao, M., Nobre, A., Nunes, J. P., Ferreira, J. G., et al. (2003). Evaluation of eutrophication in the Ria Formosa coastal lagoon, Portugal. Cont. Shelf Res. 23, 1945–1961. doi: 10.1016/j.csr.2003.06.008
Ifremer (2014). Qualité du Milieu Marin Littoral - Bulletin de la surveillance 2014. Laboratoire Environnement Ressources d'Arcachon. Océanographie et Dynamique des Ecosystèmes Unité Littoral Laboratoire Environnement Ressources Arcachon.
Lauenstein, G. G. (1995). Comparison of organic contaminants found in mussels and oysters from a current mussel watch project with those from archived mollusc samples of the 1970s. Mar. Pollut. Bull. 30, 826–833. doi: 10.1016/0025-326x(95)00089-6
Legendre, P., and Legendre, L. F. J. (1998). “Developments in environmental Modelling,” in Numerical Ecology, eds P. Legendre and L. Legendre (Amsterdam: Elsevier Science BV), 1–853.
Lehotay, S. J., Harman-fetcho, J. A., and McConnell, L. L. (1998). Agricultural pesticide residues in oysters and water from two Chesapeake Bay Tributaries 37, 32–44. doi: 10.1016/S0025-326X(98)00129-5
Luna-acosta, A., Renault, T., Thomas-guyon, H., Faury, N., Saulnier, D., and Budzinski, H. (2012). Chemosphere detection of early effects of a single herbicide (diuron) and a mix of herbicides and pharmaceuticals (diuron, isoproturon, ibuprofen) on immunological parameters of Pacific oyster (Crassostrea gigas) spat. Chemosphere 87, 1335–1340. doi: 10.1016/j.chemosphere.2012.02.022
Luo, L., Ke, C., Guo, X., Shi, B., and Huang, M. (2014). Metal accumulation and differentially expressed proteins in gill of oyster (Crassostrea hongkongensis) exposed to long-term heavy metal-contaminated estuary. Fish Shellfish Immunol. 38, 318–329. doi: 10.1016/j.fsi.2014.03.029
Mai, H., Cachot, J., Brune, J., Geffard, O., Belles, A., Budzinski, H., et al. (2012). Embryotoxic and genotoxic effects of heavy metals and pesticides on early life stages of Pacific oyster (Crassostrea gigas). Mar. Pollut. Bull. 64, 2663–2670. doi: 10.1016/j.marpolbul.2012.10.009
Mai, H., Gonzalez, P., Pardon, P., Tapie, N., Budzinski, H., Cachot, J., et al. (2014). Comparative responses of sperm cells and embryos of Pacific oyster (Crassostrea gigas) to exposure to metolachlor and its degradation products. Aquat. Toxicol. 147, 48–56. doi: 10.1016/j.aquatox.2013.11.024
Mai, H., Morin, B., Pardon, P., Gonzalez, P., Budzinski, H., and Cachot, J. (2013). Environmental concentrations of irgarol, diuron and S-metolachlor induce deleterious effects on gametes and embryos of the Paci fi c oyster, Crassostrea gigas. Mar. Environ. Res. 89, 1–8. doi: 10.1016/j.marenvres.2013.04.003
Mori, K. (1979). Effects of artificial eutrophication on the metabolism of the Japanese oyster Crassostrea gigas. Mar. Biol. 53, 361–369. doi: 10.1007/bf00391619
Muñoz, I., and Rosés, N. (2000). Comparison of extraction methods for the determination of atrazine accumulation in freshwater molluscs (Physa acuta Drap. and Ancylus fluviatilis Müll, Gastropoda). Water Res. 34, 2846–2848. doi: 10.1016/S0043-1354(99)00394-2
Perdue, J. A., Beattie, J. H., and Chew, K. K. (1981). Some relationships between gametogenic cycle and summer mortality phenomenon in the Pacific Oyster (Crassostrea gigas) in Washington State. J. Shellfish Res. 1, 9–16.
Pernet, F., Barret, J., Le Gall, P., Franck, L., Fiandrino, A., Huvet, A., et al. (2011). Mortalités Massives de l'Huître Creuse : Causes et Perspectives. Rapport final du programme de recherche sur les mortalités d' huîtres creuses Crassostrea gigas dans l' étang de Thau. Available online at: archimer.ifremer.fr/doc/00043/15404/12764.pdf
Plus, M., Dumas, F., Stanisière, J.-Y., and Maurer, D. (2009). Hydrodynamic characterization of the Arcachon Bay, using model-derived descriptors. Cont. Shelf Res. 29, 1008–1013. doi: 10.1016/j.csr.2008.12.016
Quiniou, F., Damiens, G., Gnassia-Barelli, M., Geffard, A., Mouneyrac, C., Budzinski, H., et al. (2007). Marine water quality assessment using transplanted oyster larvae. Env. Int. 33, 27–33. doi: 10.1016/j.envint.2006.06.020
ROCCH (2015). Les Contaminants Chimiques dans les Huîtres et les Moules du Littoral Français. Available online at: http://envlit.ifremer.fr/var/envlit/storage/documents/parammaps/contaminants-chimiques/
Turner, A. (2010). Marine pollution from antifouling paint particles. Mar. Pollut. Bull. 60, 159–171. doi: 10.1016/j.marpolbul.2009.12.004
Watermann, B. T., Herlyn, M., Daehne, B., Bergmann, S., Meemken, M., and Kolodzey, H. (2008). Pathology and mass mortality of Pacific oysters, Crassostrea gigas (Thunberg), in 2005 at the East Frisian coast, Germany. J. Fish Dis. 31, 621–630. doi: 10.1111/j.1365-2761.2008.00953.x
Keywords: metolachlor, copper, pacific oyster, developmental effects, bioaccumulation
Citation: Gamain P, Cachot J, Gonzalez P, Budzinski H, Gourves P-Y and Morin B (2017) Do Temporal and Spatial Parameters or Lifestyle of the Pacific Oyster Crasssostrea gigas Affect Pollutant Bioaccumulation, Offspring Development, and Tolerance to Pollutants? Front. Mar. Sci. 4:58. doi: 10.3389/fmars.2017.00058
Received: 28 October 2016; Accepted: 16 February 2017;
Published: 16 March 2017.
Edited by:
Hans Uwe Dahms, Kaohsiung Medical University, TaiwanReviewed by:
Andrés Hugo Arias, CONICET, ArgentinaIsabella Buttino, Italian Institute for Environmental Protection and Research, Italy
Copyright © 2017 Gamain, Cachot, Gonzalez, Budzinski, Gourves and Morin. This is an open-access article distributed under the terms of the Creative Commons Attribution License (CC BY). The use, distribution or reproduction in other forums is permitted, provided the original author(s) or licensor are credited and that the original publication in this journal is cited, in accordance with accepted academic practice. No use, distribution or reproduction is permitted which does not comply with these terms.
*Correspondence: Jérôme Cachot, amVyb21lLmNhY2hvdEB1LWJvcmRlYXV4LmZy