Measurement of Aquaculture Chemotherapeutants in Flocculent Matter Collected at a Hard-Bottom Dominated Finfish Site on the South Coast of Newfoundland (Canada) After 2 Years of Fallow
- 1Aquaculture, Biotechnology and Aquatic Animal Health Section, Department of Fisheries and Oceans, Northwest Atlantic Fisheries Center, St. John's, NL, Canada
- 2Department of Chemistry, Memorial University of Newfoundland, St. John's, NL, Canada
- 3Department of Biology, Memorial University of Newfoundland, St. John's, NL, Canada
- 4Atlantic Fisheries Fund, Department of Fisheries and Oceans, Northwest Atlantic Fisheries Center, St. John's, NL, Canada
The expansion of aquaculture is resulting in sites being installed over a diversity of substrate types, leading to different potential chemotherapeutant degradation scenarios. There is little to no information on the biodegradation and/or persistence of chemotherapeutants at hard-bottom dominated aquaculture sites having little natural sediment. In this study, we measured organic matter (OM) content and concentrations of chemicals linked to finfish aquaculture activities (trace elements, antibiotics and parasiticides) in flocculent matter samples collected close to cages at a site fallowed for 2 years and at an active site, the latter being indicative of flocculent chemical signature during production. Our results show persistence of flocculent matter after 2 years of fallow. The chemical signature of the samples confirms that Cu, Zn, Ca, and P, present around fish cages, are direct markers of aquaculture wastes. Persistence of two pyrethroids, one avermectin, and one antibiotic in grab samples show a potentially lasting association between these chemicals and the OM from fish feed and wastes, even after 2 years. Overall, the concentrations measured do not indicate a direct lethal toxicity on marine organisms (as per studies described in literature) except for Zn. Nevertheless, the long-term persistence of a flocculent mixture rich in chemicals and the lack of information on sublethal and synergistic effects on hard-bottom communities calls for caution and additional studies.
Introduction
Chemicals released from finfish aquaculture sites include cage antifoulants and disinfectants, as well as anasthetics and compounds used to treat diseases (chemotherapeutants) such as antibiotics and parasiticides. These compounds can accumulate in sediments, affect the diversity and fitness of the local biota (i.e., non-target organisms), and promote antibiotic resistance in microbes (Burridge et al., 2010; White et al., 2016). Effects of aquaculture-derived chemotherapeutants on non-target organisms depend on their dissipation and degradation following release (Benskin et al., 2016). In sediments, chemotherapeutants may undergo chemically or biologically-mediated transformation, but co-exposure to pesticides, antibiotics and/or trace metals used in aquaculture may hinder their bacterial transformation leading to increased persistence in sediments (Benskin et al., 2016). For parasiticides such as pyrethroids (and likely other compounds as well), degradation rates depend on several factors, including temperature, light, pH, organic matter (OM) content, and oxygen availability (Farghaly et al., 2013), which affect the half-life of these substances (Benskin et al., 2016). Research on the accumulation and degradation of chemotherapeutants in sediments around aquaculture sites is needed to evaluate dissipation rates (Benskin et al., 2016) and assess the potential for adverse effects to benthic invertebrates (Van Geest et al., 2014). In particular, the expansion of aquaculture in countries like Canada, Norway, and New Zealand is resulting in sites being installed over a diversity of substrate types such as hard-bottom leading to different potential chemotherapeutant degradation scenarios.
Along the South Coast of Newfoundland (NL), Canada, most salmonid farms are located in deep bays or fjords, where predominantly hard bottom substrates (bedrock, rock or cobble) contain localized depositional areas in protected seafloor depressions (e.g., Anderson et al., 2005; Hamoutene et al., 2014). Depth and bathymetry determine the location of patches of fine-grained natural deposits (Anderson et al., 2005). Deposition and resuspension are episodic and mostly driven by wind or sporadic winter storms, current speed being generally low above the seafloor (Schwinghamer et al., 1994; Salcedo-Castro and Ratsimandresy, 2013; Hamoutene et al., 2016). Flocculent matter, which is a viscous composite of decomposing fish feed, fish feces, microbes and deposited OM (Salvo et al., 2015a), can be seen in benthic video surveys at hard bottom sites. Flocculent matter often co-occurs with indicators of organic enrichment defined as bacterial mats and opportunistic polychaetes, and its accumulation on hard substrates produces a dramatically different substrate for benthic fauna and flora (Hamoutene et al., 2014, 2016). There is no information on the potential biodegradation and/or persistence of chemotherapeutants in deposits of flocculent matter containing little to no natural sediment.
This study aims to measure concentrations of chemicals linked to aquaculture activities (such as trace metals, antibiotics and paraciticides) in flocculent matter samples collected close to cages at a finfish site fallowed for 2 years. Some metals are valuable tracers of aquaculture wastes because they are chemically stable and can be quantified at low cost. In addition, background concentrations of trace metals or other elements of interest can generally be measured in surrounding sites (Yeats et al., 2005). We chose to measure the following elements, some of which can be related to aquaculture wastes in flocculent samples: P, Ca, Cr, Fe, Co, Ni, Cu, Zn, Se, Cd, and Pb. Aquaculture wastes can be highly enriched in Cu from cage antifouling agents and in Zn, a component of salmon diets (Richardson et al., 1985; Yeats et al., 2005; Skretting Informa, 2014). Fish manure may contain relatively high concentrations of Mn, Cd, Cr, Pb, Fe, and Zn (Naylor et al., 1999) and those metals might be enriched in aquaculture wastes. Among the potential chemotherapeutants used in salmonid aquaculture, two broad spectrum antibiotics (amoxicillin and florfenicol) and three parasiticides (two pyrethroids: cypermethrin and deltamethrin; and one avermectin: emamectin benzoate [EB]) were also measured as part of this study. Amoxicillin is a β-lactam antibiotic that is effective against gram positive and gram negative bacteria and used in the aquaculture industry as a treatment for furunculosis (Burridge et al., 2010). Florfenicol is employed to treat furunculosis and classical vibriosis in salmon (Lunestad and Samuelsen, 2008). Pyrethroids are used to treat sea lice infestations and administered as bath treatments under different formulations: AlphaMax® (2.0 μg·L−1 deltamethrin; Scottish Environmental Protection Agency (SEPA), 2008) and Excis® (5.0 μg·L−1 cypermethrin; Scottish Environmental Protection Agency (SEPA), 1998). While Excis® has not been approved for use in Canada, it was applied in the mid-1990s under a research permit. AlphaMax® was registered for sea lice control in southwest New Brunswick in 2009 and 2010, and is used in other countries (Health Canada-Pest Management Regulatory Agency (HC-PMRA), 2010; Benskin et al., 2016). EB, also used against sea lice, is administered through feed and acts on muscle and nerve tissues in invertebrates (Roy et al., 2000). As resistance toward EB has increased, this parasiticide has been been used less frequently, and has been replaced with alternatives such as diflubenzuron, teflubenzuron, deltamethrin, and cypermethrin where authorized (Langford et al., 2014).
In terms of persistence in the environment, β-lactams (amoxicillin) and florfenicol are generally not considered to be problematic (e.g., Miranda and Rojas, 2007; Burridge et al., 2010). However, avermectins and pyrethroids have low water solubility, may bind to particulate matter, and tend to accumulate in marine sediments (Scottish Environmental Protection Agency (SEPA), 1999; Burridge et al., 2010). Cu and Zn were also found to be persistent at aquaculture sites (Yeats et al., 2005). In NL sites, flocculent matter, bacterial mats and opportunistic annelids remained present after 15 months of fallowing, likely due to low temperatures and low water currents (Hamoutene et al., 2016; Salvo et al., 2017a,b). We hypothesize that some chemotherapeutic compounds may persist in the flocculent matter remaining at the fallow site due to the elevated OM content, paucity of natural sediment and expected paucity of associated infauna, together with cold temperatures and low current speeds. Results are discussed in relation to the OM content and concentrations of samples collected at an active site, the latter being indicative of the chemical signature (i.e., overall range of all measured chemicals present in the sample) of flocculent matter during production.
Material and Methods
Study Sites
Sites are located on the South Coast of NL; exact locations are confidential as per industry agreements. The fallowed site is dominated by hard bottom substrates (bedrock and boulders) and has been stocked with Atlantic salmon Salmo salar (Linnaeus, 1758) intermittently since 2005 with a first production cycle of 485,000 fish harvested in 2008. After 2009, this site held fish for a year and was restocked in 2010 with 882,000 fish. Important fish mortality occurred in 2012 due to a decrease in dissolved oxygen in the cages, which resulted in remaining fish being either harvested or moved to another site. In 2013, salmon were reintroduced for the most recent production cycle with 57,000 fish harvested a year later in 2014. At the time of sampling in 2016, the site was in fallow for two years. Samples were collected on September 7, 2016 along two different transects (directions) at the following distances to cage: Transect 1 (south - 3S) sampled at cage edge (n = 2), and single samples at 20, 40, 80, 120, and 160 m from cage edge; Transect 2 (west - 8W) consisting of one sample at cage edge and one sample at 40 m. Along transects, depths were fairly similar across stations, varying between 67 and 84 m.
In addition to the fallow site, 3 samples were collected at an active site as an indication of “production” values. Sampling occurred on October 25, 2016 while the site was in production for 5 months with 620,000 fish. Similarly to the previous site, this one was in production before, with a harvest date of June 2015 (649,000 fish) prior to a restocking in May 2016. Three samples were collected: two at cage edge (depths of 91 and 65 m) and one at 20 m (depth of 95 m) from net pens. Logistical limitations in obtaining grab samples at depths greater than 100 m restricted our ability to perform additional sampling further from cages at the active site. The substrate type as determined in one of the production site stations consisted of boulders with hard packed sand.
Grab Sampling/Waste Collection
To identify stations where grab sampling was achievable, we used underwater video monitoring following a procedure designed for NL regulatory purposes (Department of Fisheries and Oceans (DFO), 2012; Mabrouk et al., 2014). Flocculent matter appeared as a non-consolidated layer, into which the cage supporting the video camera would sink on contact. Flocculent matter was collected using an Eckman grab (6 × 6 × 10 inches). Grab content was transferred into 500 mL HDPE bottles, 20 mL scintillation vials and 50 mL plastic centrifuge tubes for chemotherapeutant, OM and elemental analysis, respectively. Samples were kept at 4°C on board and immediately frozen upon returning to the laboratory (−20°) until processing.
Wastes (i.e., degraded feed pellets and fish faces) were also collected in our tank room facility. A nitex bag was secured to the outflow drain of a 2,700 L fiberglass tank holding 20 adult Atlantic salmon (age ~6 years) for 3 days; fish were fed similar food as at NL aquaculture sites. Contents were poured into a 10 L bucket and allowed to settle; after siphoning out water, material was collected and stored at 4°C until processing.
Organic Matter Analysis
Flocculent matter samples were freeze-dried and OM was quantified by loss-on-ignition (4 h, 450°C) using duplicates from each station.
Waste samples from tanks (n = 5 subsamples) were dried for a minimum of 48 h at 80°C before assessing OM content by loss-on-ignition (4 h, 450°C).
Chemotherapeutant Analyses
Standards of analytical standard grade amoxicillin trihydrate, deltamethrin, florfenicol, cypermethrin and emamectin benzoate (Sigma-Aldrich, St. Louis, Mo, USA) were used with no additional purification. Organic solvents [dichloromethane, acetonitrile (ACN) and methanol] and water (H2O) were purchased in Optima UPLC Grade from Fisher Scientific. Stock multi-component solutions were prepared in a solution of ACN: H2O (80:20, v/v). We prepared working solutions by diluting stock solutions with distilled water.
Approximately 2 grams of sample were weighed and left over 24 h to dry at 80°C. The percent moisture was calculated with 0.11% relative standard deviation. An Acquity UPLC with a BEH C18 column (2.1 × 50 mm, 1.7 μm particle size) was coupled to a XEVO TQ-S mass spectrometer equipped with an electrospray ionization source (Waters, Milford). Gradient combination of ACN and water with 0.1% HCOOH was used as mobile phase at a flow rate of 0.4 mL·min−1. The mass spectrometer was operated in full scan with a scan range of 100-900 m/z and in multiple reaction monitoring mode for each compound using appropriate parameters. Calibration curves for compound determination were obtained by linear regression from analysis of multi-component solutions made in various concentration ranges for each compound: 10.00–1,000.00 mg·L−1 for cypermethrin, deltamethrin and florfenicol, 1–100.00 mg·L−1 for emamectin benzoate and 5.00–500.00 mg·L−1 for amoxicillin, with R2-values > 0.97. All analyses were done in triplicate and expressed per dry weight of sample. The detection limits for the chemotherapeutants are indicated between brackets in ng.g−1 dry weight: amoxicillin trihydrate (0.00584), deltamethrin (0.23647), florfenicol (0.11713), cypermethrin (0.02839), and emamectin benzoate (0.00068).
Elemental Analyses
All samples were freeze-dried before elemental analyses were performed at the CREAIT ICP-MS lab (Memorial University, Canada) between February and March 2017. Sample aliquots (0.05–0.1 g) were weighed into Savillex® PFA screw cap vials before closed-vessel digestion was carried out (1 mL distilled 16M HNO3 + 1 mL 30% H2O2) at approximately 70°C during 12 h in successive steps. Once completed, samples were dried and then suspended with 5 mL of distilled 6M HCl (to ensure the extraction of trace metals from any remaining OM). After 1 h in an ultrasonic bath, samples were kept at 100°C overnight before assessing weight when cooled. Solutions were then centrifuged for ~30 min at 7,000 rpm and the supernatant was dried. Samples were thereafter converted to nitrates by adding 0.5 mL of distilled 16M HNO3 and fluxing capped on the hotplate for a few hours, after which they were dried again. In preparation for analysis, samples were brought back into solution with 12 mL of distilled 0.2 M HNO3 and further diluted to a total of ~3000-fold.
Elements were measured using a Perkin Elmer Elan DCR II Quadrupole Inductively Coupled Plasma Mass Spectrometer, using multi-element external calibration solutions and Sc, Re, Rh, and Th as internal standards to monitor for instrumental drift, similarly to the method of Friel et al. (1990). During the course of this study, QA-QC was assured by processing and analyzing a procedural blank, a mussel reference material (NIST 2977), three duplicate samples, and one replicate. We measured the following compounds: P, Ca, Cr, Fe, Co, Ni, Cu, Zn, Se, Cd, Pb.
Statistical Analyses
OM content of wastes sampled from tanks was not used for statistical analyses, but rather to provide an estimate of fish waste organic content. Similarly, we performed no direct comparisons between chemical data collected at the fallowed site and at the production site, but considered the latter to be indicative of the chemical signature of an active site.
For correlation and multivariate analyses, pyrethroids were pooled together per sample (i.e., cypermethrin and deltamethrin). The validity of the approach is supported by the general chemistry of cypermethrin and deltamethrin. They are structurally similar, differing only in the halogen substituents (cypermethrin: C22H19Cl2NO3 and deltamethrin: C22H19Br2NO3), share common metabolic/degradation pathways leading to similar intermediate and final products, and have similar half-lives (Krieger, 2010). Therefore, the summed measurements indicated the total environmental load of pyrethroids, which should be reflected in the samples. Correlations were completed between all pairs of parameters using Spearman rank order correlations in SIGMAPLOT 13.0 software. In addition, multivariate analysis was performed in PRIMER 7.0 and PERMANOVA+ add-on (Anderson et al., 2008; Clarke et al., 2014; Clarke and Gorley, 2015). Concentrations of trace elements, pyrethroids and antibiotics were combined in a single data set and data were log(x+1) transformed. A principal components analysis (PCA) was run on normalized data. For PERMANOVA testing, we used Euclidian distances. PERMANOVA was run on the complete dataset testing OM as covariate, and distance from cage as a fixed factor using 99,999 permutations and type 3 sums of squares (unbalanced design); Monte Carlo testing was used to correct P when permutations were limited.
Results
Range of Chemical Analyses and Correlations
Ranges of values of all chemical analyses completed at the fallowed and production sites are summarized in Table 1 and correlations between parameters in Table 2.
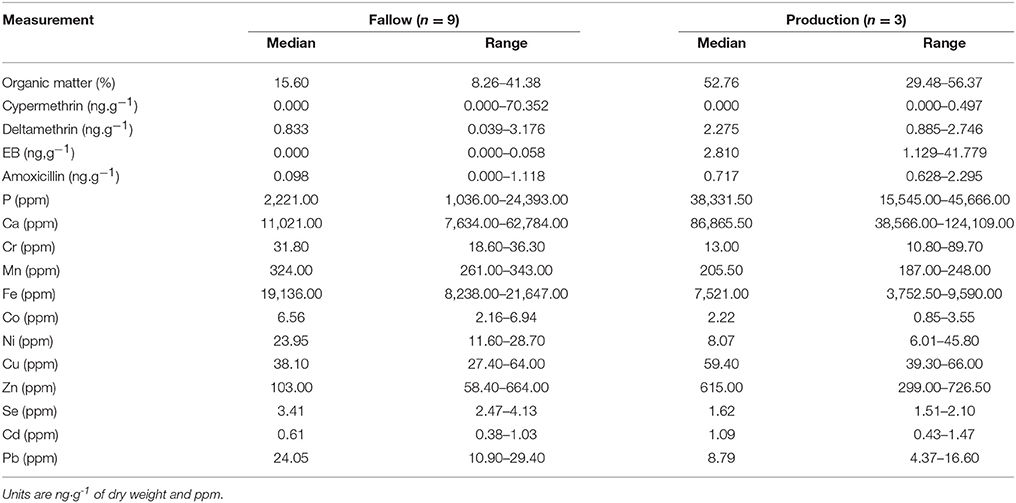
Table 1. Concentration of OM, elements, and chemotherapeutants (median and range) contained in 9 samples of flocculent matter collected at a fallowed site and 3 at a production site (5 months).
The average OM content and standard deviation in the samples from tanks housing salmon equalled 47.7 ± 3.1%. OM content of the flocculent samples collected at the fallowed site varied between 8.3 and 41.4%, and between 29.5 and 56.4% at the production site. Florfenicol was not detected in any of the flocculent samples. A high variability was detected in chemotherapeutant concentrations with compounds such as cypermethrin, EB, and amoxicillin below detection limits in some samples and reaching a maximum of 70.352 ng·g−1 for cypermethrin.
Correlations indicated that OM decreased significantly with distance to cage (Table 2). Similarly, Zn, Cd, and pyrethroid concentrations were significantly negatively correlated with distance to cage; P, Ca, and Cu showed the same tendency but with P values exceeding 0.100. Interestingly, P, Ca, Cd, Zn, and Cu showed a tendency to be positively correlated with OM contents but P values were > 0.1. On the other hand, Cu, Zn, and Cd were correlated to each other as well as to pyrethroids, Ca, and P. Similarly, Cr, Mn, Fe, Co, Ni, and Se were positively correlated with each other. Among chemotherapeutants, only amoxicillin appeared to be significantly positively correlated to EB.
Multivariate Analyses
In the PCA analysis, PC1 and PC2 explained 56.5 and 25.5% of the variation, respectively, for a total of 86.0%. No single variable drove the distribution of stations. Along PC1, the variability observed was explained (i.e., eigenvalues >0.24; listed below) in decreasing order by Co (−0.336), Fe (−0.335), amoxicillin (0.332), Pb (−0.314), Ni (−0.310), Ca (0.299), Zn (0.286), Cr (−0.282), P (0.276) and Cu (0.247) and along PC2 by pyrethroids (0.437), Cd (0.415) and to a lesser extent by Cu (0.284), P (0.283), Zn (0.269), and Cr (0.26). Among the three stations located closest to cage edge (Figure 1, in brown), station 8W differed from the two 3S stations due to a higher % OM (i.e., deposition) at the former and a higher concentration of pyrethroids at the latter stations. PERMANOVA analyses showed that OM is a significant (P > 0.01) co-variable, confirming the trends detected with Spearman correlations: stations further away from cages tended to be closer in composition and linked to a lower deposition (OM). On the other hand, there was no significant effect of distance to cage on the variability observed between stations (though probability is close to 0.05 with P = 0.0625) (Table 3).
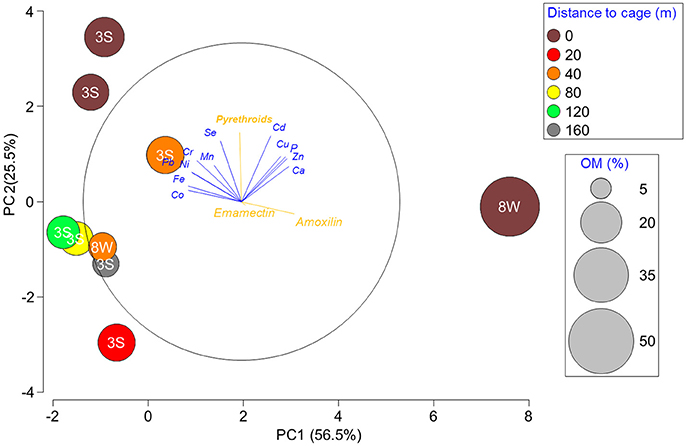
Figure 1. PCA representation of stations at the fallow site according to element and chemotherapeutant concentrations, after log+1 transformation and normalization of data. Circle sizes are relative to % OM and colors indicate distance to cage.
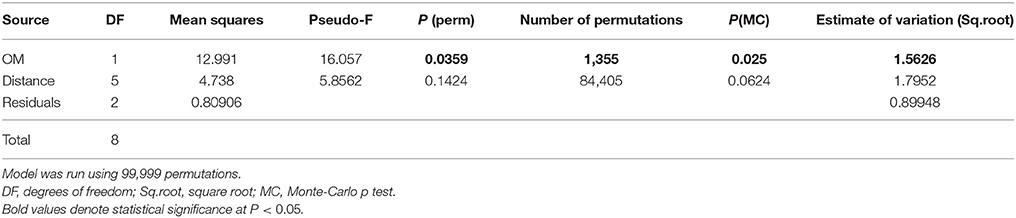
Table 3. PERMANOVA on data from all chemical analyses combined after log x + 1 transformation, and matrix of similarity based on Euclidian distance.
Discussion
Organic Matter Content and Origin
OM contents ranged from 8.3 to 41.4% at the fallowed site and 29.5–56.4% at the production site. Anderson et al. (2005) reported lower values of sedimentary OM (2–23%) at NL aquaculture sites. NL finfish farm sites exhibited a high variability in substrate composition, with little or no natural sediments: out of a series of grab attempts at 23 aquaculture sites on the south coast of NL, only 44.5% resulted in samples that could be used to perform sulfide and redox measurements (i.e., with a substrate thickness > 5 cm; Hamoutene et al., 2014). Sites sampled by Anderson et al. (2005) contained natural sediments from which sulfide and redox measurements were feasible; in contrast, substrates at our study sites were dominated by bedrock and cobble, suggesting that fine sediments were rare and flocculent matter consisted mostly of aquaculture wastes (feed pellets, fish faces). OM content at our sites was similar to that of the degraded pellets and faces collected from a salmon tank (47.7%) and to the organic carbon composition of fresh fish feed pellets (50%; Corner et al., 2006), further supporting the aquaculture origin of samples. The latter was also substantiated by the negative correlation of OM content with distance to cage. Likewise, PERMANOVA analyses result show that OM content was a significant factor explaining variability in the concentration of chemicals indicative of aquaculture deposition. Other sources (e.g., primary producers, outputs from sewage or fish processing plants) may contribute to the OM content of samples collected near fish farms (Yeats et al., 2005). In the context of our study, these alternate anthropogenic contributions are likely low given the absence of other industries or communities in this remote area. Nevertheless, natural sedimentation can also contribute to the OM content measured at the seafloor: pristine sites around the NL coast recorded from 2 to 35% OM (median: 8%) (Anderson et al., 2005). Despite a 2-year fallow period, OM-rich flocculent matter was present at the former production site, suggesting persistence of aquaculture deposits. The fallowed site studied here has experienced several cycles of production and falls into the Keeley model (Keeley et al., 2015) showing that ecosystem recovery is more difficult at sites having experienced multiple production cycles. In addition, the current speeds measured near the substrate in NL are often below 10 cm·s−1 (the critical resuspension velocity defined by Cromey et al., 2002), and only exceed this threshold < 3% of the time; therefore, the resuspension of wastes is rare (Hamoutene et al., 2016). Site depth and water stratification (Wildish et al., 2004) can also be affect the dispersal of OM at aquaculture sites. Both the production and fallowed sites are located in areas with comparable mean current speeds at 10 m depth (4 and 5 cm.s−1, respectively) and at 5 m above the seafloor (3 cm.s−1 at both sites) (Hamoutene et al., 2016; see sites 3 and 4, Table 1). Similarly, the bottom temperatures averaged 6.0 and 6.5°C at the production and fallowed sites respectively. However, some differences exist in temperature ranges (measured during 50 days in the fall) with 2–9.2°C for the production site and 2.4–15.2°C at the fallowed site (Hamoutene et al., 2016). Low temperatures (Anderson et al., 2005) may slow recovery processes, and accumulated OM could sustain opportunistic taxa over long periods and limit the recruitment of epibenthic organisms (Brooks et al., 2004; Salvo et al., 2017a). However, some authors question the temperature effect on recovery in cold environments, arguing that native microbial populations are adapted to local conditions (Arndt et al., 2013). Notably, low oxygen conditions were recorded at the study site where growers experienced fish mortality likely due to hypoxia in the water column. Lack of oxygen can clearly influence rates of degradation and explain the persistence of OM rich deposits.
Persistence of Chemicals Measured and Association With Aquaculture
Metals and Other Elements
Elements such as Cu, Cd, Zn, or Pb in sediments surrounding aquaculture sites are often linked to OM and used as aquaculture markers (Chou et al., 2002; Mendiguchía et al., 2006). In our study, Zn and Cd were the only metals whose concentrations were negatively correlated to distance to cage, and tended toward a correlation with OM (P = 0.0769 and 0.124 for Zn and Cd, respectively). Milligan and Law (2005) also reported correlations between Zn concentration and OM deposition at aquaculture sites. In addition, Cu, Zn, and Cd concentrations were highly correlated to P and Ca, which are enriched in manure. Concentrations of P and Ca at the production site have a tendency to be higher (no statistical comparisons were completed) than in the fallowed site, indicating recent fish faces deposition. Certain elements (Cu, Zn, P) can be considered to be direct tracers of aquaculture waste (feed, faces, and anti-fouling agents) while others (U, Cd, Mo,) are indicative of sedimentary organic enrichment and anoxia (Sutherland and Yeats, 2011). Interestingly, there was a lack of significant effect of distance to cage on the chemical signature of flocculent matter in the PERMANOVA. This could reflect the higher number of stations close to cages (0 and 20 m), which hinders our ability to identify gradients of deposition (only observable in distance-correlations of some single elements) for all chemicals combined. In addition, indicators of organic enrichment can be patchily distributed, and the effect of distance to cage on waste distribution is complex, non-linear and dependent on current direction (e.g., Hamoutene et al., 2015). The higher OM content at the near-cage 8W station coincided with greater amounts of the aquaculture feed markers Cd, Cu, Zn, P, Ca, and contrasted with high amounts of pyrethroids on the 3S transect stations. These differences could be due to current directions on the treatment days; pyrethroid bath treatments (with well boats or tarp usage) were likely administered such that dispersion resulted in accumulation on one side of the site.
At some salmon farms, concentrations of Cu and Zn in sediment below cages range between 48 and 150 mg·kg−1 (ppm) dry weight for Cu, and can be as high as 400 mg·kg−1 (ppm) for Zn (Debourg et al., 1993; Burridge et al., 1999; Chou et al., 2002; Brooks and Mahnken, 2003). The Cu values measured at our fallowed site ranged between 27.40 and 64.00 ppm, close to what has been measured in active farms elsewhere and at our production site (39.3–66.0). Similarly, Zn concentrations at the fallowed site varied between 58.4 and 664.0 ppm (299.0–726.5 ppm at production site) with a significant decrease with distance to cage showing a potential depositional gradient of fish wastes. Newer salmon feed formulations include lower concentrations of Zn, as manufacturers have switched to using zinc methionine, a form which is more bioavailable (Nash, 2001; Skretting Informa, 2014). Therefore, the amount of Zn introduced to marine environments should be decreasing with time (Burridge et al., 2010). The remaining Cu and Zn flocculent concentrations after 2 years of fallow highlight the persistence of aquaculture wastes. However, sedimentary Zn remediation potential varies: at some sites, post-fallow sedimentary concentrations of Zn and other contaminants under cages declined to background levels (Brooks and Mahnken, 2003), whereas elsewhere, post-fallow concentrations remained relatively similar over 5 years (Smith et al., 2005). In NL, the hard-bottom substrates and nature of the flocculent matter precludes direct comparisons with soft bottom sites.
Other elements measured as part of this study (Fe, Co, Ni, Se, Cr, Pb) were not correlated to OM, distance to cage, or P and Ca but were correlated with each other. Without a detailed composition of potential natural depositional flows feeding into the OM collected at aquaculture sites, statements on their exact origin remain speculative and require further investigation.
Chemotherapeutants
Degradation half-lives of chemotherapeutants can differ according to sediment type (Ernst et al., 2001; Meyer et al., 2013), presence of bacteria/opportunistic species, OM concentration and oxygenation state. Deltamethrin was shown to degrade slowly at 10°C, whether incubated by itself or under its commercial formulation (t1/2 = 330 ± 107 and 201 ± 27.1 d respectively). At colder incubation temperatures (4°C), deltamethrin only degraded significantly when in its commercial formulation (t1/2 = 285 ± 112 d) (Benskin et al., 2016). Cypermethrin adsorbs extensively and rapidly to organic carbon, with an overall mean organic carbon adsorption partition coefficient of 350,000 (Maund et al., 2002). Cypermethrin degradation was shown to be influenced by soil type: experiments indicated that this compound breaks down more rapidly in soils that are OM-poor or comprised of sandy clay and sandy loam compared to clay (US Environmental Protection Agency, 1989). The half-life of cypermethrin in soil is 2–8 weeks under aerobic conditions, with greater persistence under anaerobic conditions (US Environmental Protection Agency, 1989). The sediment concentrations obtained in our study varied between 0.00 and ~4.00 ng·g−1, with two higher values of cypermethrin (66.82 and 70.35 ng·g−1) below cages at the fallowed site. This indicated persistence beyond timelines described by some authors, considering that our site has been in fallow for 2 years. Depletion of deltamethrin was observed in sediments treated to hinder bacterial activity at 10°C, indicating that abiotic pathways were involved in its transformation (Benskin et al., 2016). Abiotic processes are predominant under reducing conditions (Tribovillard et al., 2006); these processes may lead to the depletion or formation of complexes, and the precipitation of sulfides and/or insoluble oxyhydroxides (Tribovillard et al., 2006). While deltamethrin degradation can occur through microbial action as well as hydrolysis and photolysis (Farghaly et al., 2013; Meyer et al., 2013), it may degrade less rapidly once adsorbed to OM-rich sediments (similarly to cypermethrin) (Benskin et al., 2016). The sorption of pyrethroids to flocculent matter is also highlighted by the observed correlation between pyrethroids and both OM content and distance to cage.
Benskin et al. (2016) found that the shortest calculated half-life for EB was of 404 days (for SLICE in inert sediments, at 4°C) and that EB biodegradation differed in incubations maintained with and without oxygen. Our results confirm the persistence of EB beyond two years (some might have been used during a previous production cycle) within the conditions of NL hard-bottom sites (low temperatures, low oxygen, and persistent OM). At other salmon aquaculture sites, sedimentary EB concentrations of 0.051–35 ng·g−1 wet weight were measured within 50–100 m from cages (Ikonomou and Surridge, 2013). These values are comparable to the active site (ng·g−1 dry weight in our study) and are slightly higher than what has been observed at the fallowed site.
The β-lactam antibiotics (amoxicillin) occur naturally in the environment and should undergo oxidation via biological and physicochemical pathways (Armstrong et al., 2005). However, it has been argued that antibacterial agents such as antibiotics resist microbial degradation in sediment, but are mainly eliminated by washing out (Samuelsen, 1989) or other mechanisms including abiotic photolysis and hydrolysis (Pouliquen et al., 2007). The persistence of amoxicillin in flocculent samples at the fallowed site remains surprising even if at low levels (<1 ppm). In contrast florfenicol, if present, was below the detection limit (i.e. < 16.2 μg·L−1). Smith and Samuelsen (1996) show that levels of other antibiotics such as oxytetracycline in farm sediments reached 11 μg·g−1 with estimated half-lives ranging from 9 to 415 days. The persistence of amoxicillin requires further investigation.
Potential Toxicity of Compounds
Trace Metals and Other Elements
Flocculent matter and fish wastes can be a food source for benthic organisms, given their accessibility and high nutritive value (i.e., enrichment in lipids and ω3 and ω6 fatty acids; Salvo et al., 2015b; White et al., 2016, 2017). Copper values above 50–150 mg·kg−1 exceed levels considered safe for various animal species (Debourg et al., 1993; Burridge et al., 1999); similarly Zn values above the threshold of 260 μg·g−1 exceed the apparent effects value (Burridge et al., 2010). This suggests that Cu values measured in our study are below toxic thresholds but Zn might have toxic potential. However, the chemical form or structure of Cu and Zn, and the degree to which these metals are bound to other ligands greatly affect their toxicity (Newman and Unger, 2003). Because elevated sedimentary concentrations of Cu and Zn co-occur below salmon cages, they may interact with each other (Burridge et al., 2010). While in some studies, the effects of Cu and Zn were additive, they more frequently appeared to act in an antagonistic manner, with Zn decreasing the toxicity of Cu (Finlayson and Verrue, 1982; Newman and Unger, 2003). In addition, any information on toxicity would have to be related to the non-target species inhabiting aquaculture areas. Our values suggest no Pb or Cd toxicity with probable effect thresholds in marine sediments of 112 and 4.2 mg·kg−1 dry weight respectively (Canadian Council of Ministers of the Environment (CCME), 1999). Iron can also exert adverse effects on aquatic organisms (value of 19,136 ppm at the fallowed site); the chemical form of Fe, as well as local pH and dissolved oxygen content influence those effects by mediating bioavailability, speciation and the physical form of iron. Conversely, iron plays a role in the reoxidation and precipitation of sulfides accumulated below fish farms (Holmer et al., 2005). Therefore, thresholds of iron toxicity in sediments/flocculent might be difficult to establish. We should also bear in mind that decreasing concentrations of sedimentary metals are indicative of transport rather than degradation. One of the mechanisms for the decline of metals in sediments is release into the water column, which will increase their bioavailability to other pelagic organisms (Burridge et al., 2010).
Chemotherapeutants
Chemotherapeutants are designed to target key biochemical patterns of groups of organisms and are not always species specific. The mode of treatment (bath vs. fish feed) also affects exposure of organisms and accumulation at the seafloor. When discussing our results, we can only report studies that have investigated chemotherapeutant toxicity to non-target groups/species that are not always applicable to the NL ecosystems.
In laboratory studies, arthropods were shown to be highly sensitive to pyrethroids, while molluscs, echinoderms and fish showed less pronounced effects (e.g., Burridge and Haya, 1997; Burridge et al., 1999). Marine amphipods exposed to contaminated sediments showed effects within 2–4 days; 10-d LC50s for deltamethrin and cypermethrin were 16 and 80 ng·g−1 (dry weight), respectively (Van Geest et al., 2014). The toxicity of deltamethrin is greater than that of cypermethrin, with tests revealing 3–8 times higher thresholds for Excis® than for AlphaMax® (Van Geest et al., 2014). The SEPA predicted-no-effect-concentration for cypermethrin is of 2.2 ng·g−1 compared to 0.33 ng.g−1 for deltamethrin (Scottish Environmental Protection Agency (SEPA), 2008). Other than at two locations below cages at the fallowed site, the pyrethroid values measured in this study suggest no significant toxicity on organisms as per the studies cited above. However, toxicity thresholds described in the literature do not always take into account OM content and composition. Cypermethrin toxicity in Hyalella azteca (amphipod) and Chironomus tentans (diptera) show decreases in toxicity observed with increasing organic carbon content (Maund et al., 2002). This clearly indicates that more studies are needed with local species and sediments with different organic loads to properly characterize toxicity thresholds. The lethality of EB to aquatic invertebrates such as Nephrops norvegicus (Norway lobster) and Crangon crangon (shrimp) was established at >68 mg·kg−1 (Scottish Environmental Protection Agency (SEPA), 1999). For the marine amphipod Corophium volutator the estimated 10-day LC50 of avermectin in sediment was 180 μg·kg−1 dry weight (DW) (Davies et al., 1998). Arenicola marina (polychaete) and Asterias rubens (seastar) showed 10 d LC50s of 23 and 23,6 mg·kg−1 DW, respectively (Thain et al., 1997). Based on these values, and similarly to pyrethroids, our results suggest low potential toxicity of EB in the flocculent samples at the fallowed site. However, sublethal effects and the consequences of long-term exposure of local organisms to these compounds remain poorly known.
At aquaculture sites, antibiotics are usually administered in feed, and can reach bacteria in the surrounding environment following gut transit (Romero et al., 2012). Shellfish and wild fish from nearby waters could also come into contact with antibiotics; harvesting those resources could affect food safety (Samuelsen et al., 1992). We found no information suggesting toxicity of amoxicillin, the only antibiotic detectable as part of this study. Antibiotics may select for antibiotic resistance in a diversity of bacteria, and it is important to assess the risk posed by antibiotic use at aquaculture sites (Romero et al., 2012).
Overall, our results show persistence of flocculent matter at an aquaculture site in fallow for two years. The chemical signature of the samples confirms that Cu, Zn, Ca, and P are direct markers of aquaculture wastes and present around fish cages. Persistence of two pyrethroids, one avermectin, and one antibiotic in grab samples show a potentially lasting association between these chemicals and the OM from fish feed and wastes even after two years. Overall, the concentrations measured do not indicate a direct lethal toxicity on marine organisms (as per studies described in literature) except for Zn. Nevertheless, the long-term persistence of a flocculent mixture rich in chemicals and the lack of information on sublethal and synergistic effects on hard-bottom communities call for caution and additional studies. Detailed infauna analyses of samples that could be collected by grabs around aquaculture sites could also be informative within this context.
Author Contributions
DH secured the funding, led the research and analyses and wrote the first draft. FS led the sampling, completed the statistical analyses and contributed to the writing. SE completed the chemical analyses and contributed to the writing. AM-R completed the chemical analyses and contributed to part of the writing. RK completed the Organic matter analyses and QA/QC of trace element analyses. GP provided history of aquaculture sites and important context information. CB provided guidance on chemical analyses procedures and reviewed the manuscript. SD completed QA/QC of trace element analyses and protocols and reviewed the manuscript.
Conflict of Interest Statement
The authors declare that the research was conducted in the absence of any commercial or financial relationships that could be construed as a potential conflict of interest.
Acknowledgments
This work was funded by the Program of Regulatory Aquaculture Research (PARR). We thank the aquaculture industry for allowing us access to sites.
References
Anderson, M., Gorley, R. N., and Clarke, R. K. (2008). Permanova+ for Primer: Guide to Software and Statistical Methods. Plymouth, MN: Primer-E Limited.
Anderson, M. R., Tlusty, M. F., and Pepper, V. A. (2005). Organic enrichment at cold water aquaculture sites—the Case of Coastal Newfoundland. Handb. Environ. Chem. 5, 99–113. doi: 10.1007/b136006
Armstrong, S. M., Hargrave, B. T., and Haya, K. (2005). Antibiotic use in finfish aquaculture: modes of action, environmental fate and microbial resistance. Handb. Environ. Chem. 5(Pt M), 341–357. doi: 10.1007/b136017
Arndt, S., Jørgensen, B. B., LaRowe, D. E., Middelburg, J. J., Pancost, R. D., and Regnier, P. (2013). Quantifying the degradation of organic matter in marine sediments: a review and synthesis. Earth. Sci. Rev. 123, 53–86. doi: 10.1016/j.earscirev.2013.02.008
Benskin, J. P., Ikonomou, M. G., Surridge, B. D., Dubetz, C., and Klaassen, E. (2016). Biodegradation potential of aquaculture chemotherapeutants in marine sediments. Aquaculture Res. 47, 482–497. doi: 10.1111/are.12509
Brooks, K. M., and Mahnken, C. V. (2003). Interactions of Atlantic salmon in the Pacific Northwest environment. III. Accumulation of Zinc and Copper. Fish. Res. 62, 295–305. doi: 10.1016/S0165-7836(03)00065-1
Brooks, K. M., Stierns, A. R., and Backman, C. (2004). Seven year remediation study at the Carrie Bay Atlantic salmon (Salmo salar) farm in the Broughton Archipelago, British Columbia Canada. Aquaculture 239, 81–123. doi: 10.1016/j.aquaculture.2003.12.024
Burridge, L. E., Doe, K., Haya, K., Jackman, P. M., Lindsay, G., and Zitko, V. (1999). Chemical Analysis and Toxicity Tests on Sediments Under Salmon Net Pens in the Bay of Fundy. Canadian Technical Report of Fisheries and Aquatic Sciences, 2291.
Burridge, L. E., and Haya, K. (1997). The lethality of pyrethrins to larvae and post-larvae of the American lobster (Homarus americanus). Ecotoxicol. Environ. Saf. 38, 150–154. doi: 10.1006/eesa.1997.1571
Burridge, L. E., Weis, J. S., Cabello, F., Pizarro, J., and Bostick, K. (2010). Chemical use in salmon aquaculture: a review of current practices and possible environmental effects. Aquaculture 306, 7–23. doi: 10.1016/j.aquaculture.2010.05.020
Canadian Council of Ministers of the Environment (CCME) (1999). “Canadian sediment quality guidelines for the protection of aquatic life: lead,” in Canadian Environmental Quality Guidelines (Winnipeg, MB: Canadian Council of Ministers of the Environment).
Chou, C. L., Haya, K., Paon, L. A., Burridge, L., and Moffatt, J. D. (2002). Aquaculture-related trace metals in sediments and lobsters and relevance to environmental monitoring program ratings for near-field effects. Mar. Pollut. Bull. 44, 1259–1268. doi: 10.1016/S0025-326X(02)00219-9
Clarke, K. R., Gorley, R. N., Somerfield, P. J., and Warwick, R. M. (2014). Change in Marine Communities: An Approach to Statistical Analysis and Interpretation, 3rd Edn. Plymouth: PRIMER-E.
Corner, R. A., Brooker, A. J., Telfer, T. C., and Ross, L. G. (2006). A fully integrated GIS-based model of particulate waste distribution from marine fish-cage sites. Aquaculture 258, 299–311. doi: 10.1016/j.aquaculture.2006.03.036
Cromey, C. J., Nickell, T. D., Black, K. D., Provost, P. G., and Griffiths, C. R. (2002). Validation of a fish farm water resuspension model by use of particulate tracer of discharged from a point source in a coastal environment. Estuaries 25, 916–929. doi: 10.1007/BF02691340
Davies, I. M., Gillibrand, P. A., McHenery, J. G., and Rae, G. H. (1998). Environmental risk of ivermectin to sediment dwelling organisms. Aquaculture 163, 29–46. doi: 10.1016/S0044-8486(98)00211-7
Debourg, C., Johnson, A., Lye, C., Tornqvist, L., and Unger, C. (1993). Antifouling Products Pleasure Boats, Commercial Vessels, Net, Fish Cages, and Other Underwater Equipment. KEM Report, No 293. The Swedish National Chemicals Inspectorate.
Department of Fisheries Oceans (DFO) (2012). Standard Operating Procedures (SOP) for Underwater Video Camera System. Available online at: http://www.dfo-mpo.gc.ca/library/347683.pdf
Ernst, W., Jackman, P., Doe, K., Page, F., Julien, G., Mackay, K., et al. (2001). Dispersion and toxicity to non-target aquatic organisms of pesticides used to treat sea lice on salmon in net pen enclosures. Mar. Pollut. Bull. 42, 433–444. doi: 10.1016/S0025-326X(00)00177-6
Farghaly, M. F., Zayed, S. M., and Soliman, S. M. (2013). Deltamethrin degradation and effects on soil microbial activity. J. Environ. Sci. Health B. 48, 575–581. doi: 10.1080/03601234.2013.774900
Finlayson, B. J., and Verrue, K. M. (1982). Toxicity of copper, zinc and cadmium mixtures to juvenile chinook salmon. Trans. Am. Fish. Soc. 111, 645–650. doi: 10.1577/1548-8659(1982)111<645:TOCZAC>2.0.CO;2
Friel, J. K., Skinner, C. S., Jackson, S. E., and Longerich, H. P. (1990). Analysis of biological reference materials, prepared by microwave dissolution, using inductively coupled plasma mass spectrometry. Analyst 115, 269–273. doi: 10.1039/AN9901500269
Hamoutene, D., Salvo, F., Bungay, T., Mabrouk, G., Couturier, C., Ratsimandresy, A., et al. (2015). Assessment of finfish aquaculture effect on Newfoundland epibenthic communities through video monitoring. North Am. J. Aquac. 77, 117–127. doi: 10.1080/15222055.2014.976681.
Hamoutene, D., Salvo, F., Donnet, S., and Dufour, S. C. (2016). The usage of visual indicators in regulatory monitoring at hard-bottom finfish aquaculture sites in Newfoundland (Canada). Mar. Pollut. Bull. 108, 232–241. doi: 10.1016/j.marpolbul.2016.04.028
Hamoutene, D., Sheppard, L., Mersereau, J., Oldford, V., Bungay, T., Salvo, F., et al. (2014). Applicability of the Use of Visual Indicators [Presence of Beggiatoa and/or Opportunistic Polychaete Complexes (OPC)] to Identify Benthic Changes Due to Aquaculture on Various Substrates. DFO Canadian Science Advisory Secretariat Research Document 2014/063 v+17 pp.
Health Canada-Pest Management Regulatory Agency (HC-PMRA) (2010). AlphaMax® 2010–3648. Available online at: http://pr-rp.hc-sc.gc.ca/pi-ip/adoc-ddoc-eng.php?p_app_id=2010-3648
Holmer, M., Wildish, D., and Hargrave, B. (2005). Organic enrichment from marine finfish aquaculture and effects on sediments biogeochemical processes. Handb. Environ. Chem. 5 (Pt M), 181–206. doi: 10.1007/b136010
Ikonomou, M. G., and Surridge, B. D. (2013). Ultra-trace determination of aquaculture chemotherapeutants and degradation products in environmental matrices by LC-MS/MS. Intl. J. Env. Anal. Chem. 93, 183–198. doi: 10.1080/03067319.2012.673222
Keeley, N. B., Forrest, B. M., and Macleod, C. K. (2015). Benthic recovery and re-impact responses from salmon farm enrichment: implications for farm management. Aquaculture 435, 412–423. doi: 10.1016/j.aquaculture.2014.10.007
Krieger, R. (ed.). (2010). Hayes' Handbook of Pesticide Toxicology, 3rd Edn. San Diego, CA: Elsevier.
Langford, K. H., Øxnevad, S., Schøyen, M., and Thomas, K. V. (2014). Do antiparasitic medicines used in aquaculture pose a risk to the Norwegian aquatic environment? Environ. Sci. Technol. 48, 7774–7780. doi: 10.1021/es5005329
Lunestad, B. T., and Samuelsen, O. (2008). “Veterinary drug use in aquaculture,” in Improving Farmed Fish Quality and Safety, ed O. Lie (Cambrirge, MA: Woodhead Publishing), 97–127.
Mabrouk, G., Bungay, T., Drover, D., and Hamoutene, D. (2014). Use of Remote Video Survey Methodology in Monitoring Benthic Impacts From Finfish Aquaculture on the South Coast of Newfoundland (Canada). DFO Canadian Science Advisory Secretariat Research Document 2014/039.
Maund, S. J., Hamer, M. J., Lane, M. C. G., Farrelly, E., Rapley, J. H., Goggin, U. M., et al. (2002). Partitioning, bioavailability, and toxicity of the pyrethroid insecticide cypermethrin in sediments. Environ. Toxicol. Chem. 21, 9–15. doi: 10.1002/etc.5620210102
Mendiguchía, C., Moreno, C., Mánuel-Vez, M. P., and García-Vargas, M. (2006). Preliminary investigation on the enrichment of heavy metals in marine sediments originated from intensive aquaculture effluents. Aquaculture 254, 317–325. doi: 10.1016/j.aquaculture.2005.10.049
Meyer, B. N., Lam, C., Moore, S., and Jones, R. L. (2013). Laboratory degradation rates of 11 pyrethroids under aerobic and anaerobic conditions. J. Agric. Food Chem. 61, 4702–4708. doi: 10.1021/jf400382u
Milligan, T. G., and Law, B. A. (2005). The effect of marine aquaculture on fine sediment dynamics in coastal inlets. Handb. Environ. Chem. 5 (Pt M), 239–251. doi: 10.1007/b136013
Miranda, C. D., and Rojas, R. (2007). Occurrence of florfenicol resistance in bacteria associated with two Chilean salmon farms with different history of antibacterial usage. Aquaculture 266, 39–46. doi: 10.1016/j.aquaculture.2007.02.007
Nash, C. E. (2001). The Net Pen Salmon Farming Industry in the Pacific Northwest. U.S. Department of Commerce, NOAA Technical Memorandum NMFS series, NWFSC-49.
Naylor, S. J., Moccia, R. D., and Durant, G. M. (1999). The chemical composition of settleable solid fish waste (manure) from commercial Rainbow Trout farms in Ontario, Canada. N. Am. J. Aquac. 61, 21–26. doi: 10.1577/1548-8454(1999)061<0021:TCCOSS>2.0.CO;2
Newman, M. C., and Unger, M. A. (2003). Fundamentals of Ecotoxicology. Boca Raton FL: CRC Press; Lewis Publishers.
Pouliquen, H., Delépée, R., Larhantec-Verdier, M., Morvan, M. L., and Le Bris, H. (2007). Comparative hydrolysis and photolysis of four antibacterial agents (oxytetracycline oxolinic acid, flumequine and florfenicol) in deionised water, freshwater and seawater under abiotic conditions. Aquaculture 262, 23–28. doi: 10.1016/j.aquaculture.2006.10.014
Richardson, N. L., Higgs, D. A., Beams, R. M., and McBride, J. R. (1985). Influence of Dietary Calcium, Phosphorus, Zinc and Sodium Phytate Level on Cataract Incidence, Growth and Histopathology in Juvenile Chinook Salmon (Oncorhynchus tshawytscha). J. Nutr. 115, 553–567. doi: 10.1093/jn/115.5.553
Romero, J., Feijoo, C. G., and Navarrete, P. (2012). “Antibiotics in aquaculture – use, abuse and alternatives,” in Health and Environment in Aquaculture, ed E. Carvalho (InTech). Available online at: http://www.intechopen.com/books/health-and-environment-in-aquaculture/antibiotics- in-aquaculture-use-abuse-and-alternatives-
Roy, W. J., Sutherland, I. H., Rodger, H. D. M., and Varma, K. J. (2000). Tolerance of Atlantic salmon, Salmo salar L. and rainbow trout, Oncorhynchus mykiss (Walbaum), to emamectin benzoate, a new orally administered treatment for sea lice. Aquaculture 184, 19–29. doi: 10.1016/S0044-8486(99)00307-5
Salcedo-Castro, J., and Ratsimandresy, A. W. (2013). Oceanographic response to the passage of hurricanes in Belle Bay, Newfoundland. Estuar. Coast. Shelf Sci. 133, 224–234. doi: 10.1016/j.ecss.2013.08.031
Salvo, F., Dufour, S. C., and Hamoutene, D. (2017b). Temperature thresholds of opportunistic annelids used as benthic indicators of aquaculture impact in Newfoundland (Canada). Ecol. Indic. 79, 103–105. doi: 10.1016/j.ecolind.2017.03.057
Salvo, F., Dufour, S. C., Hamoutene, D., and Parrish, C. C. (2015b). Lipid classes and fatty acids in Ophryotrocha cyclops, a dorvilleid from Newfoundland aquaculture sites. PLoS ONE 10:e0136772. doi: 10.1371/journal.pone.0136772
Salvo, F., Hamoutene, D., and Dufour, S. C. (2015a). Trophic analyses of opportunistic polychaetes (Ophryotrocha cyclops) at salmonid aquaculture sites. J. Mar. Biol. Assoc. UK 95, 1–10. doi: 10.1017/S0025315414002070
Salvo, F., Mersereau, J., Hamoutene, D., Belley, R., and Dufour, S. C. (2017a). Spatial and temporal changes in epibenthic communities at deep, hard bottom aquaculture sites in Newfoundland. Ecol. Indic. 76, 207–218. doi: 10.1016/j.ecolind.2017.01.019
Samuelsen, O. B. (1989). Degradation of oxytetracycline in seawater at two different temperatures and light intensities and the persistence of oxytetracycline in the sediment from a fish farm. Aquaculture 83, 7–16. doi: 10.1016/0044-8486(89)90056-2
Samuelsen, O. B., Lunestad, B. T., Husevg, B., Hølleland, T., and Ervik, A. (1992). Residues of oxolinic acid in wild fauna following medication in fish farms. Dis. Aquat. Organ. 12, 111–119. doi: 10.3354/dao012111
Schwinghamer, P., Hawryluk, M., Powell, C., and MacKenzie, C. H. (1994). Resuspended hypnozygotes of Alexandrium fundyense associated with winter occurrence of PSP in inshore Newfoundland waters. Aquaculture 122, 171–179. doi: 10.1016/0044-8486(94)90508-8
Scottish Environmental Protection Agency (SEPA) (1998). SEPA Policy on the Use of Cypermethrin in Marine Fish Farming Risk Assessment, EQS and Recommendations. Policy No. 30. Available online at: http://www.sepa.org.uk/aquaculture/policies/index.htm
Scottish Environmental Protection Agency (SEPA) (1999). Emamectin Benzoate, an Environmental Assessment. Available online at: http://www.sepa.org.uk/policies/index.htm
Scottish Environmental Protection Agency (SEPA) (2008). Attachment XIV Guidance Note on the Licensing of Discharges of AMX (Deltamethrin) at Marine Cage Fish Farms. Available onlien at: http://www.sepa.org.uk/water/water_regulation/regimes/aquaculture/marine_aquaculture/fish_farm_manual.aspx
Smith, J. N., Yeats, P. A., and Milligan, T. G. (2005). “Sediment geochronologies for fish farm contaminants in Lime Kiln Bay, Bay of Fundy,” in Environmental Effects of Marine Finfish Aquaculture. Handbook of Environmental Chemistry, Vol. 5M, ed B. T. Hargrave (Berlin: Springer), 221–238.
Smith, P., and Samuelsen, O. B. (1996). Estimates of the significance of out-washing of oxytetracycline from sediments under Atlantic salmon sea-cages. Aquaculture 144, 17–26. doi: 10.1016/S0044-8486(96)01318-X
Sutherland, T., and Yeats, P. F. (2011). Elemental indicators of benthic organic enrichment associated with marine finfish aquaculture. Dyn. Biochem. Process Biotechnol. Mol. Biol. 5, 66–75. Available online at: http://www.globalsciencebooks.info/Online/GSBOnline/OnlineDBPBMB_5_SI1.html
Thain, J. E., Davies, I. M., Rae, G. H., and Allen, Y. T. (1997). Acute toxicity of ivermectin to the lugworm Arenicola marina. Aquaculture 159, 47–52. doi: 10.1016/S0044-8486(97)00210-X
Tribovillard, N., Algeo, T. J., Lyons, T., and Riboulleau, A. (2006). Trace metals as paleoredox and paleoproductivity proxies: an update. Chem. Geol. 232, 12–32. doi: 10.1016/j.chemgeo.2006.02.012
US Environmental Protection Agency (1989). Pesticide Fact Sheet Number 199: Cypermethrin. US EPA, Office of Pesticide Programs, Registration Division, Washington, DC.
Van Geest, J. L., Burridge, L. E., and Kidd, K. A. (2014). Toxicity of two pyrethroid-based anti-sea lice pesticides, AlphaMax® and Excis®, to a marine amphipod in aqueous and sediment exposures. Aquaculture 434, 233–240. doi: 10.1016/j.aquaculture.2014.08.025
White, C. A., Dworjanyn, S. A., Nichols, P. D., Mos, B., and Dempster, T. (2016). Future aquafeeds may compromise reproductive fitness in a marine invertebrate. Mar. Environ. Res. 122, 67–75. doi: 10.1016/j.marenvres.2016.09.008
White, C. A., Nichols, P. D., Ross, D. J., and Dempster, T. (2017). Dispersal and assimilation of an aquaculture waste subsidy in a low productivity coastal environment. Mar. Pollut. Bull. 120, 309–321. doi: 10.1016/j.marpolbul.2017.05.042
Wildish, D. J., Dowd, M., Sutherland, T. F., and Levings, C. D. (2004). Environmental Fate and Effect of Chemicals Associated with Canadian Freshwater Aquaculture. Canadian Technical Report of Fisheries and Aquatic Sciences.
Keywords: salmon, aquaculture, chemotherapeutant, flocculent matter, hard-bottom substrate, organic matter
Citation: Hamoutene D, Salvo F, Egli SN, Modir-Rousta A, Knight R, Perry G, Bottaro CS and Dufour SC (2018) Measurement of Aquaculture Chemotherapeutants in Flocculent Matter Collected at a Hard-Bottom Dominated Finfish Site on the South Coast of Newfoundland (Canada) After 2 Years of Fallow. Front. Mar. Sci. 5:228. doi: 10.3389/fmars.2018.00228
Received: 07 February 2018; Accepted: 12 June 2018;
Published: 02 July 2018.
Edited by:
Marty Riche, Florida Atlantic University, United StatesReviewed by:
Yi Zhou, Institute of Oceanology (CAS), ChinaLaura Ribeiro, Instituto Português do Mar e da Atmosfera (IPMA), Portugal
Copyright © 2018 Hamoutene, Salvo, Egli, Modir-Rousta, Knight, Perry, Bottaro and Dufour. This is an open-access article distributed under the terms of the Creative Commons Attribution License (CC BY). The use, distribution or reproduction in other forums is permitted, provided the original author(s) and the copyright owner(s) are credited and that the original publication in this journal is cited, in accordance with accepted academic practice. No use, distribution or reproduction is permitted which does not comply with these terms.
*Correspondence: Dounia Hamoutene, dounia.hamoutene@dfo-mpo.gc.ca