- 1Scripps Institution of Oceanography, La Jolla, CA, United States
- 2The Manta Trust, Dorchester, Dorset, United Kingdom
- 3Sydney Institute of Marine Science, Mosman, NSW, Australia
- 4Department of Biological Sciences, Macquarie University, Sydney, NSW, Australia
- 5School of Biomedical Sciences, The University of Queensland, St. Lucia, QLD, Australia
- 6Marine Megafauna Foundation, Truckee, CA, United States
- 7Université de Brest, CNRS, IRD, Ifremer, UMR 6539 LEMAR, Plouzané, France
- 8Coastal Conservation Action Lab, University of California, Santa Cruz, Santa Cruz, CA, United States
- 9Hawaii Association of Marine Education and Research, Lahaina, HI, United States
- 10Department of Biology and Environmental Science, Linnaeus University, Kalmar, Sweden
- 11Blue Resources Trust, Colombo, Sri Lanka
- 12Murdoch University, Perth, WA, Australia
- 13Inter-American Tropical Tuna Commission, La Jolla, CA, United States
- 14Laboratory of Evolutionary Biology and Bioinformatics, University of Barcelona, Barcelona, Spain
- 15Molecular Ecology and Fisheries Genetics Laboratory, Bangor University, Bangor, United Kingdom
- 16Center for Fisheries, Aquaculture & Aquatic Sciences, Southern Illinois University Carbondale, Carbondale, IL, United States
- 17Okeanos Research Centre of the University of the Azores, Horta, Portugal
- 18AZTI - Tecnalia Marine Research Divison, Pasaia, Spain
- 19Tethys Research Institute, Milan, Italy
- 20Instituto Politécnico Nacional (CICIMAR), La Paz, Mexico
- 21The School of Biological Sciences and the Oceans Institute, The University of Western Australia, Perth, WA, Australia
- 22Save Our Seas Foundation –D'Arros Research Centre, Geneva, Switzerland
- 23The Australian Institute of Marine Science, Crawley, WA, Australia
- 24Centre for Applications in Natural Resource Mathematics (CARM), School of Mathematics and Physics, The University of Queensland, St. Lucia, QLD, Australia
- 25CSIRO Oceans and Atmosphere, Ecosciences Precinct, Dutton Park, Brisbane, QLD, Australia
- 26Pacific Manta Research Group, Santa Rosa, CA, United States
- 27School of Science and Engineering, University of the Sunshine Coast, Hervey Bay, QLD, Australia
Manta and devil rays are filter-feeding elasmobranchs that are found circumglobally in tropical and subtropical waters. Although relatively understudied for most of the Twentieth century, public awareness and scientific research on these species has increased dramatically in recent years. Much of this attention has been in response to targeted fisheries, international trade in mobulid products, and a growing concern over the fate of exploited populations. Despite progress in mobulid research, major knowledge gaps still exist, hindering the development of effective management and conservation strategies. We assembled 30 leaders and emerging experts in the fields of mobulid biology, ecology, and conservation to identify pressing knowledge gaps that must be filled to facilitate improved science-based management of these vulnerable species. We highlight focal research topics in the subject areas of taxonomy and diversity, life history, reproduction and nursery areas, population trends, bycatch and fisheries, spatial dynamics and movements, foraging and diving, pollution and contaminants, and sub-lethal impacts. Mobulid rays remain a poorly studied group, and therefore our list of important knowledge gaps is extensive. However, we hope that this identification of high priority knowledge gaps will stimulate and focus future mobulid research.
Introduction
Manta and devil rays (collectively known as mobulids) are medium to large batoid fishes, reaching wingspans of up to seven meters and occupying primarily pelagic, offshore marine habitats (Couturier et al., 2012). These filter-feeding species are found circumglobally in tropical and subtropical waters where they exploit a broad range of oceanographic regimes, from productive equatorial upwelling systems to oligotrophic tropical seas (Couturier et al., 2012; Stewart et al., 2017a). Although relatively understudied for most of the Twentieth century, public awareness, and scientific research on these species has increased dramatically in recent years. Much of this attention has been in response to targeted fisheries (White et al., 2006; Croll et al., 2016), international trade in mobulid products (O'Malley et al., 2016), and a growing concern over the fate of exploited populations (Ward-Paige et al., 2013; Lewis et al., 2015; White et al., 2015). Over the past decade, research advances on the biology and ecology of mobulid rays have supported a number of international, national, and local management actions focused on halting or reversing population declines (Croll et al., 2016).
The majority of previous research efforts have focused on manta rays (previously genus Manta but now included in genus Mobula; White et al., 2017), due in part to their popularity with the dive community and high ecotourism value (O'Malley et al., 2013). Mobula alfredi is the best studied member of the family due to its coastal distribution (Kashiwagi et al., 2011) and relatively predictable visitation to coastal aggregation sites such as cleaning stations (Marshall et al., 2011; Jaine et al., 2012). These attributes facilitate field research activities and allow for long-term monitoring of populations that is critical for studying the ecology, life history, and population dynamics of the species (e.g., Stevens, 2016). In contrast, the species in the family more associated with offshore habitats (i.e., many devil rays) are challenging to study in the field and are better known from specimens captured in fisheries (White et al., 2006; Fernando and Stevens, 2011; Croll et al., 2016; Pardo et al., 2016a; Stewart et al., 2017a). Recent public and conservation interest in devil rays has resulted in more targeted field efforts to study these species, leading to a growing understanding of their movements and habitat use (e.g., Croll et al., 2012; Thorrold et al., 2014).
Despite progress in mobulid research over the past decade, major knowledge gaps still exist. Considerable action has been taken to curb targeted fisheries for these species, but bycatch remains a persistent threat (Croll et al., 2016). Fisheries impacts are currently the most acute threat to mobulids and are the main focus of management strategies (Lawson et al., 2017). However, little management attention has been directed toward the impacts of pollution, habitat degradation, and climate change on already-depleted mobulid populations. To develop effective management strategies and prioritize conservation actions for mobulid rays, a number of pressing knowledge gaps must be filled on the life history, biology, ecology, taxonomic status, and population dynamics of these species.
Here we describe priority questions for future mobulid ray research. Our aim is to identify the most critical questions for conservation scientists to address in the coming decade. We have organized these priority questions around nine themes that we consider vital to improving different aspects of management of mobulids: 1. Taxonomy and diversity; 2. Life history; 3. Reproduction and nursery areas; 4. Population trends; 5. Bycatch and fisheries; 6. Spatial dynamics and movements; 7. Foraging and diving; 8. Pollution and contaminants; and 9. Sub-lethal impacts. (See Figure 1 for a graphical table of contents). Within each theme, we describe methods that have been successfully applied to other taxa and provide suggestions about how these could be adapted for use in future mobulid research. Mobulid rays remain a poorly studied group, and therefore our list of important knowledge gaps is extensive. However, we hope that this identification of high priority knowledge gaps will stimulate and focus future mobulid research.
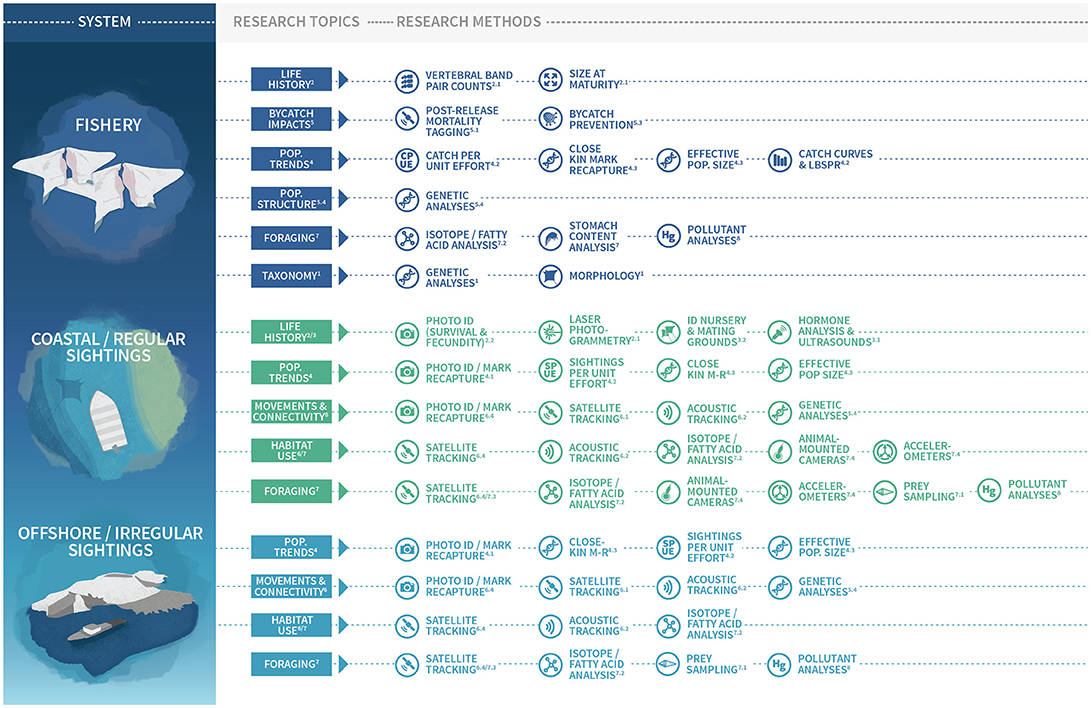
Figure 1. Graphical table of contents/research topics and methods for use in different mobulid study systems. Superscript numbers refer to sections in the paper with detailed descriptions and recommendations for future research addressing each topic and using each method. Figure design by Madeline Wukusick www.communique.design.
1. Taxonomy and Diversity
Taxonomic clarity is a fundamental requirement for species conservation and management. Without it, human impacts on evolutionary and ecologically distinct groups cannot be accurately evaluated. Key advances in this field include an initial revisionary study of the genus Mobula (Notarbartolo di Sciara, 1987), the resurrection of M. alfredi, splitting the genus Manta into two species (Marshall et al., 2009), a redescription of M. kuhlii (Notarbartolo di Sciara et al., 2017), and a recent phylogenetic reclassification of the mobulid ray family (Mobulidae) that proposes a single genus (Mobula) and eight species where there were previously 11 (White et al., 2017). Despite considerable progress over the past three decades, more work is needed to fully characterize diversity within the Mobulidae.
1.1. Clarifying Existing Taxonomy
There is an urgent requirement to resolve species boundaries further in the genus Mobula to underpin conservation and management. White et al. (2017) synonymized M. rochebrunei and M. hypostoma, M. eregoodootenkee and M. kuhlii, and M. japanica and M. mobular, and proposed a single genus, lumping Manta into Mobula. Previous work supports the monophyly of the genus Mobula (Adnet et al., 2012; Poortvliet et al., 2015) and the lumping of M. mobular and M. japanica (Notarbartolo di Sciara, 1987; Poortvliet et al., 2015). It is important to note that in all cases of synonymy in White et al. (2017), the observed genetic relatedness between the lumped species is of comparable magnitude to that detected for M. alfredi and M. birostris. However, a more detailed population level study previously revealed them to be distinct species that had experienced introgression, obscuring genetic separation (Kashiwagi et al., 2012). In the case of M. kuhlii and M. eregoodootenkee, White et al. (2017) suggest that such a population level study might provide the evidence needed to recognize them as distinct species. Investigation into intra-specific variation using genomic data is lacking for most of the mobulid species. We therefore strongly recommend comprehensive population level studies of these species complexes as currently recognized, with the inclusion of morphological considerations. Such studies should also make use of genomic techniques and multi-locus analysis such as Restriction site Associated DNA Sequencing (e.g., Davey and Blaxter, 2010; Peterson et al., 2012). There is also a need to consider interactions between species given current evidence for secondary contact in manta rays (Kashiwagi et al., 2012; Walter et al., 2014), and recent divergence times within the group (Poortvliet et al., 2015). The challenge, therefore, will be distinguishing distinct species where hybridization and historical introgression may occur.
Samples from across the known ranges of species of interest, including replicates within localities, should be collected and analyzed to minimize the introduction of ascertainment bias, and encompass intra-specific variation. In addition, given the morphological similarities among species, samples should be accompanied by photographs that allow for confident species identification and documentation of intra-specific variation. Clarifying the taxonomy of the M. hypostoma species complex (including those specimens formerly attributed to M. rochebrunei) will be particularly challenging, as few historical samples of M. rochebrunei were ever collected along the West African coastline. Given the scarcity of modern sightings and samples of M. rochebrunei and high rates of fishing in the region (Agnew et al., 2009), this collapse may lead to an underestimate of the species' vulnerability to extinction, and highlights the susceptibility of mobulid rays to Illegal, Unreported and Unregulated (IUU) fishing. Future research should include a comprehensive survey of the West African coastline to collect specimens and investigate diversity within the region. For the purposes of this paper, we identify existing research and knowledge gaps for M. eregoodootenkee and M. rochebrunei, and urge further taxonomic attention to resolve the M. kuhlii cf. eregoodootenkee and M. hypostoma cf. rochebrunei species complexes.
1.2. Cryptic Diversity
In addition to the currently recognized M. birostris and M. alfredi, a putative third species of manta ray in the Atlantic has been hypothesized for almost a decade (Marshall et al., 2009; Hinojosa-Alvarez et al., 2016; Stewart et al., 2018b). Conclusive molecular support and clear morphological descriptions based on sufficient population and genomic sampling are still lacking (Hinojosa-Alvarez et al., 2016). However, a formal description of M. cf. birostris is urgently needed to determine whether this is a distinct species or a subspecies of M. birostris, which will have implications for management. Rediscovery of cryptic diversity within manta rays in the early Twenty-first Century was the result of detailed morphological observation of many individuals of several populations combined with genetic analyses of multiple genes (Marshall et al., 2009; Kashiwagi et al., 2012). The lack of equivalent studies for the remaining devil rays indicates that currently undetected cryptic diversity within the group cannot be ruled out, and thus we recommend additional detailed taxonomic studies for mobulid rays generally. Further, population level sampling enables opportunities for studies to define Evolutionarily Significant Units (ESUs) for conservation prioritization. To support future morphometric and meristic-based studies, we strongly encourage research groups and collections to improve reference material at the population level to effectively catalog mobulid diversity. These should include tissue samples for molecular analysis; whole specimens where possible; partial specimens of species- and region-specific characters such as gill plates, spiral valves, and tooth-bands; and high-quality images with a scale bar of the dorsal, ventral, mouth, tail/dorsal fin, and spiracle views.
2. Life History
Life-history information is needed to estimate extinction risk, maximum population growth rates in data-poor species, and for performing stock assessments (Musick, 1999; Pardo et al., 2016a,b). Many chondrichthyans have highly conservative life-history characteristics, and mobulid rays have some of the most conservative traits, making them vulnerable to overfishing (Cortés, 2002; Dulvy et al., 2014; Croll et al., 2016). These slow-growing rays have very low rates of reproduction and long maturation times (Notarbartolo di Sciara, 1988; Marshall and Bennett, 2010; Dulvy et al., 2014; Pardo et al., 2016a; Stevens, 2016). Key life history parameters such as age at maturity, growth rate, lifespan, mortality (both natural and fisheries-induced) and fecundity are lacking for most mobulid species (Table 1). Estimating these parameters is crucial for assessing the vulnerability and demography of exploited populations (Campana et al., 2002; Goldman, 2005; Cailliet et al., 2006; García et al., 2008; Dulvy et al., 2014). Wherever possible, life-history parameter estimates should be obtained and applied to management at the population level, as biological characteristics are likely to vary both among species and locations with variable environmental conditions. Regional differences may be especially relevant in broadly distributed species such as M. mobular, M. thurstoni, M. tarapacana, and M. birostris. Available estimates of mobulid extinction risk suggest that mobulid ray populations are unlikely to withstand current levels of fishing mortality, even in small-scale artisanal fisheries (Dulvy et al., 2014; Pardo et al., 2016a). Therefore, accurate estimates of life history parameters are urgently needed to assess the effectiveness of potential fisheries management scenarios.
2.1. Age and Growth
In elasmobranchs, growth is usually modeled by estimating the age of individuals of known size (disc width in rays) by counting growth bands on vertebral centra (Figure 2) or other hard parts such as fin spines (Cailliet et al., 2006). The vertebral centra of devil rays tend to be poorly calcified, which has hindered the use of this technique so far. Currently, only one data set has provided size-at-age estimates to model growth rates using vertebrae from the caudal region of M. mobular (previously M. japanica) from Mexico (Cuevas-Zimbrón et al., 2013; Pardo et al., 2016a). Further efforts should assess this method in other species and locations, since the extent of calcification may vary. Researchers should strive to obtain samples from a broad size-range and estimate growth parameters using a multi-model approach (i.e., including von Bertalanffy, Gompertz, or Logistic models) (Smart et al., 2016). In data-poor situations or when a size-class is poorly represented, back-calculation, or Bayesian methods may allow for adequate estimates (Cailliet et al., 2006; Pardo et al., 2016a). An important assumption is that each pair of opaque and translucent bands is deposited annually (Cailliet et al., 2006). Validating this assumption in mobulid rays could be attempted by injection of a fluorochrome label (e.g., calcein) into specimens in aquaria and confirming band periodicity post-mortem (Huveneers et al., 2013), although aquarium specimens may show atypical band deposition due to constant environmental conditions. Alternatively, radiocarbon (14C) dating of archival devil ray hard parts could provide a method of age validation by tracing this dated chemical marker released in high concentrations during atomic bomb testing in the 60–70 s (Campana et al., 2002). Additionally, researchers could evaluate the feasibility of using cesium isotopes released during the Fukushima nuclear accident to assist with age validation for mobulids in the western Pacific (Neville et al., 2014). Until validation is achieved, researchers can test band-pair deposition consistency across years as a means of verification (Cailliet et al., 2006).
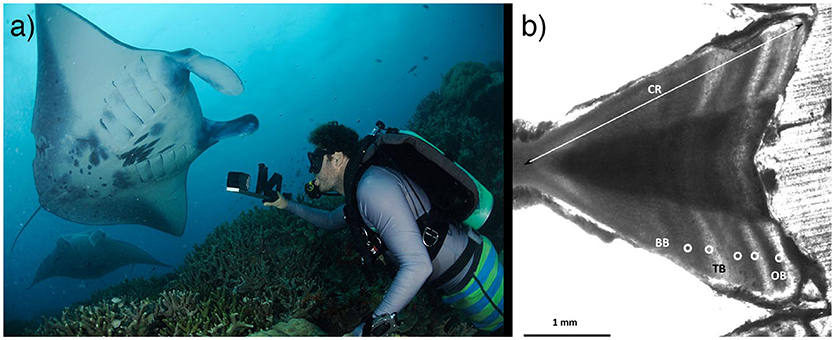
Figure 2. Age and growth in mobulid rays. (a) Stereo-video photogrammetry can be used to measure individuals in the field, providing insights into growth, size at maturity, and other life history characteristics. Photo © Guy Stevens, used with consent. (b) In fisheries scenarios, vertebral centra have been successfully used to age mobulids, although validation of band-pair deposition rates are needed. Photo © Betty Laglbauer. (See section 2).
Aging elasmobranchs using vertebrae relies on access to fished specimens and therefore is not appropriate in many wild mobulid ray populations. Direct estimates of growth rate can be obtained in-situ from morphometric measurements of resighted individuals over known time increments, based on visual size estimates (Kitchen-Wheeler et al., 2012; Kashiwagi, 2014; Stevens, 2016), paired laser photogrammetry (Deakos, 2010), or stereo-video photogrammetry (Shortis et al., 2009) (Figure 2). These mark-recapture data with associated size estimates can be used to fit growth curves (e.g., Perry et al., 2018), estimate size at maturity, and study factors influencing growth rates across life stages, regions and populations. Therefore, further efforts should aim to obtain accurate size estimates over longer time frames to estimate growth rates. It will also be important to obtain accurate estimates of size-at-birth from neonates or late-term embryos across populations since these are necessary to fit two-parametric growth models, which are preferable to three-parametric models that sometimes provide unrealistic estimates of size at birth in elasmobranchs (Smart et al., 2016).
2.2. Mortality
Mortality is an essential parameter to understand population dynamics, and it is important for demographic models and stock assessments to separate natural mortality from fishing mortality. There are a number of methods researchers can use to estimate mortality in mobulid rays depending on both data availability and type. In data-rich wild populations, mark-recapture (via photo identification) and acoustic telemetry can be used to estimate survival and natural mortality of specific life stages (e.g., Heupel and Simpfendorfer, 2002). In fisheries scenarios, catch curve analysis of landed specimens allows for direct estimates of total mortality (natural plus fishing mortality) (e.g., Simpfendorfer, 1999; Pardo et al., 2016a). Many indirect methods exist to estimate mortality based on other life history parameters (Pauly, 1980; Kenchington, 2014). The most relevant approaches to mobulid research likely include estimation of natural mortality from maximum age (e.g., Hoenig, 1983) and average age (e.g., Pardo et al., 2016a), or from the von Bertalanffy growth coefficient K (e.g., Beverton and Holt, 1959; Charnov, 1993; Jensen, 1996).
2.3. Population Viability
The life history information highlighted in this section is important for developing management strategies that prioritize life stages for conservation, and for testing the ability of proposed conservation approaches to enhance population viability. Matrix models are a common tool for population viability analysis, and can be particularly useful in data-poor scenarios that are common among mobulid rays (Heppell et al., 2000). As key life history data become available, future research on mobulids should use sensitivity, elasticity, and perturbation analyses to identify life stages with significant contributions to overall population viability in order to direct management actions (Heppell, 1998; Frisk et al., 2005). Additionally, researchers could assess the impact of specific management approaches by quantitatively evaluating the increase in the growth rate of a population given reduced mortality of specific life stages (e.g., Gerber and Heppell, 2004; Smart et al., 2017). Further, in cases where time-series of abundance or relative abundance are not available (which is the case for many mobulid populations), these life history parameters can be used to calculate the maximum growth rate of a population (Pardo et al., 2016b). This can be compared with total mortality to determine population trajectories and extinction risk (Pardo et al., 2016a).
3. Reproduction and Nursery Areas
The reproductive biology of mobulid rays is known mostly from the reef manta ray (M. alfredi). Courtship and copulation in reef manta rays are common around cleaning stations and reef habitats (Marshall and Bennett, 2010; Deakos et al., 2011; Deakos, 2012; Stevens, 2016; Stevens et al., 2018b). Observations of courtship and mating remain rare in other mobulid species (Yano et al., 1999; Duffy and Scott, 2017; Stevens et al., 2018a). A gestation period of 1 year has been observed in both wild and captive M. alfredi, but remains unknown for other mobulids (Uchida et al., 2008; Marshall and Bennett, 2010; Couturier et al., 2012; Deakos, 2012; Stevens, 2016). All mobulids are thought to give birth to a single large pup, with rare observations of twins (Marshall et al., 2009; Couturier et al., 2012). Parturition is often followed immediately by copulation in captive and wild M. alfredi (Uchida et al., 2008; Stevens, 2016); a reproductive strategy that is likely to be found in other mobulid species, as well (Stevens, 2016; Duffy and Scott, 2017). In wild populations of M. alfredi, interbirth intervals of 2–7 years have been reported, presumably dependent on environmental conditions and food availability (Marshall and Bennett, 2010; Deakos, 2012; Kashiwagi, 2014; Stevens, 2016). The fecundities of mobulids other than M. alfredi have not been determined, and previous risk assessments and demographic analyses have assumed that mobulids share similar key reproductive parameters (Dulvy et al., 2014; Pardo et al., 2016a). Size segregation appears to be common across mobulid species, with juveniles absent from most field studies or present at different times or locations than adults (Notarbartolo di Sciara, 1988; Deakos, 2010), with the exception of M. alfredi in Mozambique where young-of-year individuals were occasionally observed within the general population (Marshall and Bennett, 2010). In terms of movements, habitat use and general life history data, the juvenile life stage of all mobulid species remains poorly studied.
3.1. Age at Maturity & Fecundity
Maturity assessments of mobulids are based primarily on external morphological cues, such as changes in clasper morphology in males, and the presence of visual signs of mating or pregnancy in females (Deakos, 2010; Marshall and Bennett, 2010; Stevens, 2016) (Figure 3). However, physiological sexual maturity may not coincide with the external signs of sexual maturation. Physiological markers such as changes in steroid levels have been successfully adopted as indicators of reproductive status in elasmobranchs (Sulikowski et al., 2007; Prohaska et al., 2013). Ultrasonography has also been widely adopted to define gonadal maturity in a variety of fish species (Bryan et al., 2007; Novelo and Tiersch, 2012) including elasmobranchs (Carrier et al., 2003; Whittamore et al., 2010). Future research addressing mobulid reproduction and fecundity could consider applying these methods, particularly in species where individual identification to track reproductive status through time is not possible. If used on a sufficiently large scale, these approaches could help estimate the proportion of females within a population contributing to annual recruitment and, for data collected over extended periods of time, may provide information on the timing and seasonality of the reproductive cycle (Whittamore et al., 2010). In combination with photogrammetry, ultrasonography and endocrinology would enable more reliable estimates of age and size at maturity.
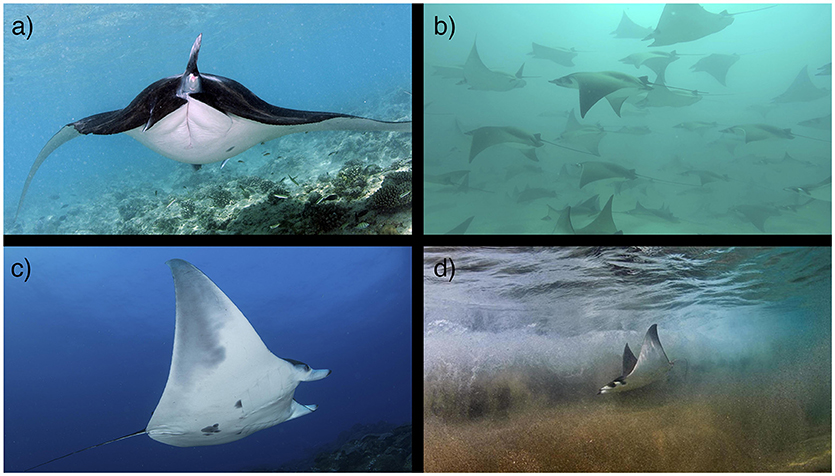
Figure 3. Mobulid reproduction. Mating, pupping and nursery grounds are not well defined for mobulids. (a) A pregnant reef manta ray (M. alfredi) in the Maldives. Photo © Guy Stevens (b) A school of pregnant M. munkiana in the Galapagos Islands. Photo © Walter Pisco. (c) A juvenile male M. cf. birostris at the Flower Garden Banks in the Gulf of Mexico. Photo © NOAA/FGBNMS/G.P. Schmahl. (d) A juvenile M. munkiana in the Sea of Cortez. Photo © Octavio Aburto. (See section 3).
3.2. Mating, Pupping, and Nursery Areas
In several mobulid species, reproductive activity peaks seasonally and may occur more often at social aggregation sites such a seamounts or cleaning stations (Yano et al., 1999; Marshall and Bennett, 2010; Stevens, 2016; Stevens et al., 2018b). However, significant knowledge gaps remain in our understanding of mobulid courtship and mating behavior. Long-term monitoring of areas where opportunistic courtship activity has been documented previously may reveal more seasonal mating grounds for certain species, and targeted surveillance of social aggregation sites would be a natural starting place to undertake these studies. Little is known about parturition in mobulid rays. To date, no natural wild birth for any mobulid species has been observed. Advanced technology may be required to determine if specific pupping grounds exist, or if females give birth in any area with suitable conditions. Researchers could use satellite and acoustic tags to evaluate the movements and habitat use of near-term females and reveal behavioral changes preceding pupping events. Additionally, animal-borne video camera systems (e.g., Stewart et al., 2017b; Fontes et al., 2018) may also reveal changes in behavior preceding parturition and allow for more detailed examinations of environments being used.
In many locations, juvenile M. alfredi and M. birostris have been observed in coastal shoreline and lagoon habitats (McCauley et al., 2014; Stevens, 2016; M. Deakos, G. Stevens unpublished) and occasionally in offshore habitats (R. Rubin unpublished) segregated from adult populations. Additionally, juvenile M. thurstoni have been observed in coastal regions of the Gulf of California (Notarbartolo di Sciara, 1988), indicating that protected coastal habitats may be important to juvenile mobulids in general. To date two locations have been identified that meet established criteria for elasmobranch nursery habitats (Heupel et al., 2007; Martins et al., 2018) (M. birostris in the Gulf of Mexico, Stewart et al., 2016b; M. munkiana in the Sea of Cortez, Palacios et al. in prep; Figure 3), although other locations are currently undergoing similar assessments. Future work should target locations where juvenile mobulids are known to aggregate or are frequently present, and determine whether these sites meet the criteria for formal classification as a nursery habitat as defined by Heupel et al. (2007) and Martins et al. (2018). Given the apparent affinity for protected coastal and lagoon habitats in several species, aerial drone surveys could be an efficient and cost-effective method for identifying candidate sites. The identification of mating and pupping grounds may also aid in the identification of juvenile habitats and nursery grounds. For example, large annual aggregations of M. munkiana in the Gulf of California, Mexico are presumed to be mating aggregations. Juvenile and potential young-of-year individuals have been observed along the coast adjacent to these aggregations (Notarbartolo di Sciara, G. Stevens & J. Stewart, pers. obs; Palacios et al., in prep), suggesting that pupping and nursery grounds may exist in close proximity to mating grounds. If mating pupping, and nursery habitats are identified, these critical habitats should be protected to prevent changes in natural behavior, to ensure that pathways to these areas are not obstructed and to ensure the safety of individuals within these areas.
4. Population Trends
With increasing concern for the general status of global mobulid populations, estimates of abundance, and breeding stock along with monitoring of long-term population trends are crucial measures required to develop effective management and conservation strategies. Current population information for manta rays is predominantly gathered through diver counts and photo-identification (photo-ID) surveys of targeted populations at predictable aggregation sites. When photographs are collected in a rigorous sampling design, they can provide an index of sighting per unit effort over time and/or be incorporated into mark-recapture statistical models to generate estimates of abundance and parameters of population trends (Marshall et al., 2011; Marshall and Pierce, 2012; Couturier et al., 2014). However, photo-ID is limited to species with individually unique markings (e.g., M. birostris, M. alfredi, M. tarapacana; Figure 4) and is most effective in populations with coastal distributions that are accessible by divers. Therefore, there is a need to investigate additional methods to assess population trends, especially for devil rays.
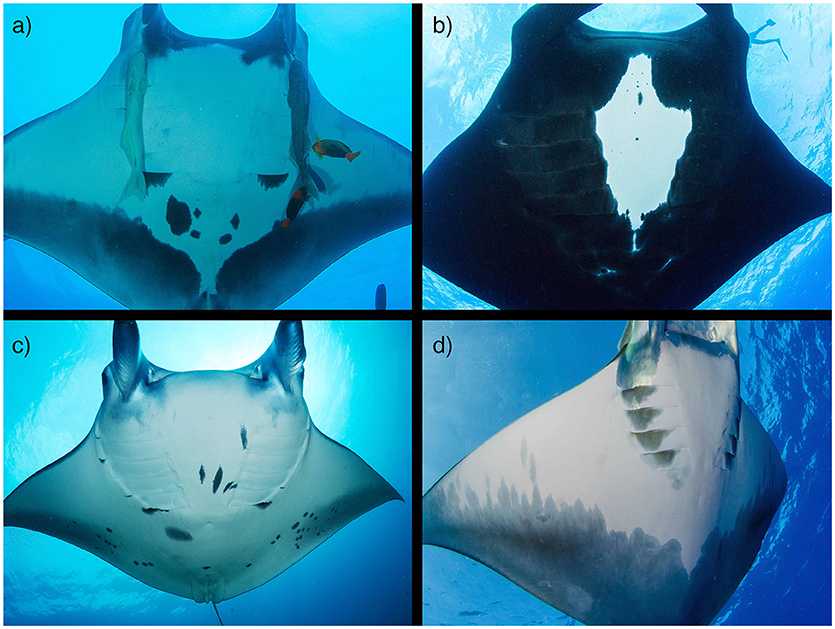
Figure 4. Photo identification of mobulids. Oceanic manta rays (M. birostris; a,b) and reef manta rays (M. alfredi; c) have unique spot patterns that remain constant through time and can be used to identify individuals for mark-recapture studies. Both species have chevron (a,c) and black (b) morphs. (d) The posterior shading of the ventral surface of M. tarapacana may also allow for individual identification. Photo copyrights: (a,b) Joshua Stewart; (c) Guy Stevens; (d) Tom Burd www.tomburd.co.uk. (See section 4).
4.1. Photo Identification
Of all mobulid species, M. alfredi is the most studied using photo-ID methods (Deakos et al., 2011; Marshall et al., 2011; Kitchen-Wheeler et al., 2012; Couturier et al., 2014; Kashiwagi, 2014) and therefore has the most robust estimates of population size. The large variation in body pigmentation patterns, presumed to be unique to each individual from birth, facilitates the cataloging of individuals and the creation of photo-ID databases (Kumli and Rubin, 2010; Kitchen-Wheeler et al., 2012; Marshall and Pierce, 2012). The popularity of in-water interactions with mobulids has also resulted in a high incidence of citizen science contributions to regional photo-ID databases from recreational divers (Kumli and Rubin, 2010; Couturier et al., 2011, 2014; Germanov and Marshall, 2014; Stevens, 2016). In addition to benefiting researchers by expanding data collection spatially and temporally without additional cost or resource use, citizen science promotes public awareness, and engagement in conservation actions (Bonney et al., 2009). The opportunistic manner by which these sightings are reported, however, can impede the use of mark-recapture analyses that require precise sampling designs to control for effort and information on absence as well as presence of individuals (Cooch and White, 2006). Researchers should carefully consider the analytical methods they plan to employ and the key parameters they wish to recover, and plan their data collection accordingly rather than relying purely on opportunistic sightings. Furthermore, a key limiting factor to mark-recapture analysis is recapture rate: the probability of observing an individual animal on subsequent events. Patchy data often result in low recapture rates, and this may lead to non-estimable parameters or low precision around estimates, precluding the determination of trends. The use of auxiliary data within mark-recapture frameworks can help to overcome limitations with low recapture rates. For example, the incorporation of acoustic tagging data into mark-recapture analysis of tagged seven-gill sharks (Notorhynchus cepedianus) facilitated abundance estimation for this species (Dudgeon et al., 2015). Several mobulid research programs already include tagging studies (see Movements section) and future research programs could consider integrating these study designs to enable best use of data across multiple studies. Rigorous sampling designs can also increase the utility of citizen science data. For example, focusing citizen science efforts during particular time periods can increase data input into analysis frameworks, as well as investigating presence-only models to include opportunistic sightings (Mengersen et al., 2017). Ongoing surveys of well-studied populations should be continued to enable long-term trends to be assessed.
4.2. Relative Abundance, Data Poor Methods, and Population Projections
For many mobulid species, assessing population trends through individual identification (photo-ID, tag, and release) is impossible due to extremely low recapture probabilities resulting from infrequent encounters, diver avoidance behaviors, or lack of distinct physical markings. Other approaches therefore need to be considered. In populations of mobulids that may exhibit high site affinity and recapture rates, researchers could evaluate the efficacy of external tags or implanted PIT tags (Chapman et al., 2009) in facilitating traditional mark-recapture methods for abundance estimation. Indirect or relative estimates of abundance can be generated through: a) count surveys (SPUE: sighting per unit effort) of live animals (e.g., Rohner et al., 2013), b) observer data of capture rates (CPUE: catch per unit effort or BPUE: bycatch per unit effort), or c) landed animals at fish markets (CPUE). As life history parameters and population estimates become available for mobulids, researchers should use traditional stock assessment methods to evaluate the status and trends of populations (Methot and Wetzel, 2013). However, this will most likely only be possible in fisheries that have high observer coverage or bycatch reporting (e.g., Inter-American Tropical Tuna Commission (IATTC) purse seine fishery). In more common data-poor scenarios, researchers could take advantage of existing length- and age-based assessment methods such as catch curve analysis (Pardo et al., 2016a) and the length based spawning potential ratio (Hordyk et al., 2015) to evaluate the status of mobulid populations. Where robust estimates of current population sizes are available (e.g., Couturier et al., 2014), researchers should use population projection models (Meyer-Gutbrod and Greene, 2018) and extinction risk analyses (Dulvy et al., 2004) to evaluate future population trends under varying management schemes, environmental conditions, and climate change scenarios.
4.3. Genetic Approaches to Estimating Abundance
New developments in genomic approaches show promise for facilitating population size estimates for mobulids. DNA can be extracted from small amounts of tissue collected from live or dead animals as well as preserved components such as gill plates. High throughput sequencing enables thousands of genetic markers to be sequenced (most commonly focusing on single nucleotide polymorphisms—SNPs), which can then be used in multiple population genetic analyses. One approach is estimating genetic effective population size (Ne), which provides the number of breeding individuals to be used as a metric of population viability. Strong concordance has been demonstrated between ecological and genetic estimates of abundance for some elasmobranch species (Portnoy et al., 2009; Dudgeon and Ovenden, 2015) with preliminary work for mobulids also demonstrating concordance for M. alfredi in Japan (Kashiwagi, 2014). Investigations are ongoing for M. alfredi from Australia (A. Armstrong unpublished) and Mozambique (S. Venables unpublished). The advantage of Ne is that only a single population sample is required to generate a point estimate. Theoretically, changes in Ne with temporal sampling can demonstrate population trends (e.g., Kuparinen et al., 2016). However, given that demography, ecology, and reproductive mode can all influence Ne estimates (Wang, 2005), future research is needed to investigate the sensitivity of Ne to these biological features in mobulids and provide guidelines on minimum sampling requirements. Another emerging molecular approach to estimating population size is close-kin mark-recapture (CKMR; Bravington et al., 2016a,b). Genomic data are used to identify kin pairs including parent-offspring, full siblings and half-siblings. Marking occurs during reproduction and the juvenile genomic signatures represent “recaptures” of the parents (Hillary et al., 2018). CKMR has been applied to natural populations of bluefin tuna (Bravington et al., 2016a) and white sharks (Hillary et al., 2018). As with Ne, CKMR only provides information about adults and requires sampling a substantial proportion of the population to identify kinship pairs and generate abundance estimates (Bravington et al., 2016b). However, combining CKMR methods with auxiliary data, such as acoustic telemetry data, can provide estimates of key demographic parameters needed to estimate total population size (Hillary et al., 2018). With few published examples for CKMR, sampling guidelines are not yet available, however several elasmobranch studies are underway (Ovenden et al., in review) and their outcomes will be informative for designing studies for mobulids.
5. Bycatch and Fisheries
The greatest contributors to direct mortality of mobulid rays across their range are targeted and bycatch fisheries (Croll et al., 2016). At least 13 fisheries in 12 countries specifically target mobulids, and at least 30 fisheries in 23 countries capture mobulids as bycatch (Hall and Roman, 2013; Croll et al., 2016). They are targeted or retained for the Asian medicine trade as well as consumption of meat (Croll et al., 2016; O'Malley et al., 2016) (Figure 5), which has contributed to declines in mobulid landings in the Philippines, Indonesia, Mexico, India, and Mozambique (Couturier et al., 2012). The attraction of mobulids to productive tropical and subtropical habitats where target species such as tunas aggregate, along with their distribution in the epipelagic zone, make them vulnerable to fisheries capture (Croll et al., 2016). Mobulids are targeted or caught as bycatch in virtually every fishing gear type, including small-scale fisheries characterized by the use of driftnets, gillnets, harpoons, gaffs, traps, trawls, and longlines; and large-scale fisheries using driftnets, trawls and purse seines (Croll et al., 2016). The level of bycatch depends greatly on the fishing method used, with the highest bycatch rates reported from gillnets and purse seiners (Croll et al., 2016; Alfaro-Cordova et al., 2017). The slow population growth rates of mobulid populations largely exclude them from considerations of sustainable targeted fisheries (Dulvy et al., 2014; Pardo et al., 2016a). Consequently, future research should focus on assessing bycatch risks and mitigation efforts as well as the impacts of existing fisheries on mobulid populations.
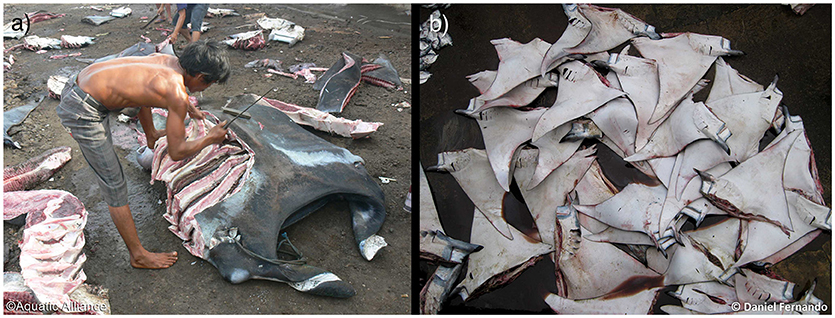
Figure 5. Mobulid fisheries. Mobulid rays are captured incidentally in virtually all fishing gear types, and are also targeted for their meat and gill plates in some countries. (a) A fisherman removes the gill plates of an oceanic manta ray (M. birostris) in Tanjung Luar, Indonesia. Photo © Peter Bassett, Aquatic Alliance, used with consent. (b) A pile of M. mobular and M. tarapacana at a fish market in Sri Lanka. Photo © Daniel Fernando. (See section 5).
5.1. Post-release Mortality
While fisheries regulations have sought to prevent the retention and landing of mobulid rays, the vast majority of mobulid captures are a result of unintentional bycatch (Croll et al., 2016). Physical and biological traits of rays (i.e., lack of a rigid skeleton to protect internal organs, relatively high metabolic rates) make them particularly vulnerable to handling while they are out of water (Poisson et al., 2014). In the past, onboard handling practices have resulted in high levels of post-release mortality (Hall and Roman, 2013; Poisson et al., 2014). Efforts to create a comprehensive “best practices” bycatch mitigation plan for mobulids have defined suitable technical and behavioral recommendations aboard vessels (Poisson et al., 2014; Hutchinson et al., 2017). Recent resolutions prohibiting harmful handling practices like gaffing and punching holes in the bodies of rays were approved by the IATTC, and have begun to be applied in some purse seine and longline fisheries (IATTC Resolution C-15-04; IOTC Resolution 2017-S21-PropC (E)). Further work to identify and implement less harmful handling practices could prevent a significant amount of onboard mobulid mortality. Evaluating survival rates of mobulids released alive in non-target fisheries will help guide management decisions, as simply banning landings will not be an effective strategy in cases where a high proportion of individuals die after release. Initial studies of M. mobular released from tuna purse seine fisheries in New Zealand found mortality rates of 50–60% (Francis and Jones, 2016). Researchers should evaluate the post-release mortality of mobulids captured incidentally in a variety of gear types, as fishing gear such as gill nets with long soak times may have considerably higher post-release mortality rates than long lines or purse seines. Additionally, future studies should evaluate the impact of handling and release methods and relevant environmental and operational covariates on mobulid post-release mortality in order to develop release guidelines that maximize survival. Post-release mortality can be evaluated using pop-off satellite tags (Francis and Jones, 2016) or blood chemistry analyses (Hutchinson et al., 2015) in offshore fisheries. In coastal fisheries where released mobulids are more likely to remain in range of acoustic receivers, researchers could also consider the use of acoustic tags to evaluate survivorship rates (Skomal, 2007). Accelerometer tags may also be useful in the quantification of post-release behavior and mortality and the impacts of handling methods in cases where instrument recovery is feasible (Whitney et al., 2016). As relationships between directly observable covariates (e.g., time on deck, behavior after release) and survivorship are identified, observer programs in commercial fisheries should strive to collect fishery-wide data on these covariates, which will allow managers to estimate the total impact of a given fishery on mobulid populations.
5.2. Species Distributions and Fishery Data Standardization
Identifying areas of overlap between mobulid hotspots and fisheries could help reduce mobulid bycatch rates. Concurrent satellite tracking of focal species and vessel monitoring systems can provide insights into bycatch risk and identify key locations for mitigation and management action (Queiroz et al., 2016). Onboard fisheries observers provide far more data-rich scenarios in which to assess bycatch risk, especially in commercial fisheries with high observer coverage such as the IATTC tuna purse seine fleet (Hall and Roman, 2013; Croll et al., 2016). These observer records allow for detailed species distribution models that can reveal relationships between species abundance and key environmental variables, both for bycatch and target species (Scales et al., 2017; Hazen et al., 2018). Future studies should identify the regions with both the highest overall mobulid bycatch rates, and the highest mobulid bycatch to target catch ratios. This information will allow managers to develop spatio-temporal (and ideally dynamic) management approaches with the greatest ecological and conservation value for mobulids and the lowest economic loss from reduced catches of target species. However, to take full advantage of fisheries observer data to develop species-specific habitat and distribution models, data collection protocols need to be standardized and species identification training for observers emphasized. Researchers could work with regional fisheries management organizations (RFMOs) to develop a comprehensive, standardized data collection manual for mobulids that ensures all relevant variables, including release methods, are collected and can be compared among regions and fisheries. Additionally, RFMOs should be encouraged to implement detailed mobulid identification training courses, and researchers could follow up with molecular identification of captured specimens to determine identification error rates. Unfortunately, these approaches will not cover mobulid mortality onboard smaller tuna purse seine vessels that don't carry observers, or for fisheries targeting species outside the purview of the current RFMOs (e.g., clupeoid seine fisheries), and this mortality is not accounted for in current bycatch estimates. Many of these vessels operate in nearshore productive waters where mobulids aggregate, and are likely to represent substantial unreported mobulid bycatch. Further research is necessary to describe the nature and quantity of this bycatch (e.g., Alfaro-Cordova et al., 2017).
5.3. Bycatch Prevention and Mitigation
Bycatch mitigation methods have not been adequately explored for mobulids, and proposals for preventing interactions between fishing gear and mobulids through technological innovations or gear modifications are needed. Strong associations with the thermocline in M. birostris (Stewart et al., 2016b) suggest that setting fishing gear above the thermocline depth could reduce bycatch rates of this species, and incorporating water column dynamics into species distribution models could help identify important bycatch hotspots (Brodie et al., 2018a). However, the feasibility of limiting gear depth in commercial fisheries is questionable, and therefore alternate proposals should be developed and tested for mobulids more generally. Bycatch mitigation has been developed for many non-target marine species such as seabirds in long-line fisheries (e.g., streamers, bird-scaring lines, weighted lines) (Melvin et al., 2014), and turtles in shrimp trawl fisheries (e.g., turtle excluder devices) (Crowder et al., 1995). For elasmobranchs more generally, there have been various trials, such as the (apparently unsuccessful) use of rare-earth metals to deter sharks from baited hooks (Jordan et al., 2011; Godin et al., 2013), and use of “deterrent” colors and patterns (Jordan et al., 2013). Some studies suggest that the use of light-emitting diodes in or near the ultraviolet range may reduce bycatch of elasmobranchs in gill net fisheries (Jordan et al., 2013), and trials employing similar approaches are currently underway with mobulid rays in Indonesia (Rosady, pers. comm.).
5.4. Fisheries Impacts on Genetic Diversity
Although in-depth research into population genetic structure is lacking for mobulids, the limited studies of genetic connectivity indicate that populations are spatially structured (Stewart et al., 2016a). Consequently, fisheries management of mobulid rays may be more effective and relevant at the stock level, rather than simply along political or geographic boundaries (Reiss et al., 2009). More genetic studies are needed to identify genetically distinct populations of mobulid rays to support regional management strategies. As traditional gene sequencing methods may not reveal population structure in mobulids (Kashiwagi et al., 2012), we recommend high-throughput genomic methods (Peterson et al., 2012; Catchen et al., 2013) applied to samples of representative coverage within and among populations to generate robust estimates of population structure. Given that many of the smaller mobulid species are challenging to find and sample in typical field expeditions, fisheries may provide the best opportunities to obtaining such sample representation. For example, tissue, or tail samples collected by observers in tuna fisheries could provide the extensive geographic coverage and the large sample sizes necessary for investigating genetic connectivity. Additionally, there is strong evidence for a positive correlation between genetic diversity and a population's resilience to extinction (Frankham, 2005; von der Heyden, 2017) and overexploited fish species have been shown to have lower genetic diversity than closely related species that are not overharvested (Pinsky and Palumbi, 2014). We therefore encourage researchers to evaluate genetic diversity across a gradient of fishing pressure to establish if and where fishing has led to a loss of diversity and population bottlenecks (e.g., Hauser et al., 2002). This will help prioritize vulnerable populations for conservation and management action. Studies of general patterns of effective population sizes and descriptions of the demographic histories of mobulids can establish valuable baseline reference points.
6. Spatial Dynamics and Movements
Understanding the spatial dynamics and habitat use patterns of animal populations is key to successful conservation and management (Cooke, 2008; Ogburn et al., 2017). This theme has been a dominant focus of mobulid ray research over the past decade, with 17 published studies documenting movements of individuals using a combination of photographic mark-recapture methods and electronic tracking. Using acoustic and satellite telemetry, movements of 1,008 individual rays of 6 species have been investigated in 10 distinct regions throughout their range (Figure 6). However, the majority of this effort has been focused on manta rays (n = 863), with devil rays representing only 14.4% of tracked animals (n = 145). Together, these studies highlight the large movement capacity of manta and devil rays and their use of broad geographic ranges including coastal and pelagic waters (Croll et al., 2012; Jaine et al., 2014; Thorrold et al., 2014; Francis and Jones, 2016). High rates of site residency and fidelity have been demonstrated, particularly in manta rays (Jaine et al., 2014; Stewart et al., 2016a; Couturier et al., 2018), with examples of seasonal migration between known aggregation sites up to 750 km apart (Couturier et al., 2011; Germanov and Marshall, 2014). The drivers underlying these large seasonal aggregations, common to both manta and devil rays, remain elusive. Identifying common physical and biological processes that underlie movements and aggregative behavior across species will allow for improved identification and characterization of critical habitats. Studies of fine-scale habitat use at aggregation sites have revealed that individuals visit these areas to attend shallow cleaning stations, engage in courtship behavior, and forage on ephemeral food resources (Dewar et al., 2008; Jaine et al., 2012; Weeks et al., 2015; Armstrong et al., 2016; Burgess, 2017; Couturier et al., 2018).
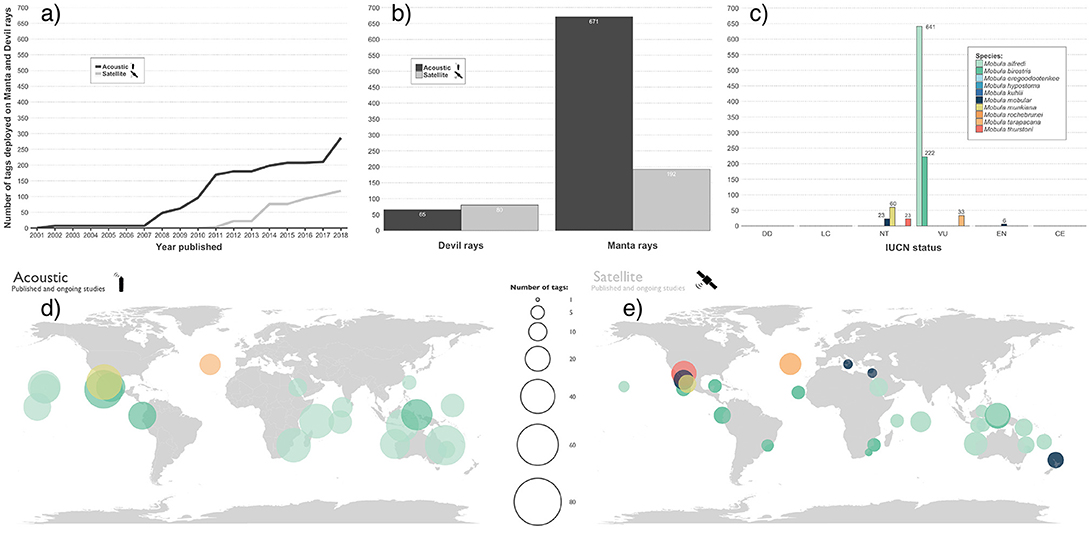
Figure 6. Mobulid telemetry effort to date. (a) Cumulative number of acoustic and satellite tags deployed on all mobulid rays from published literature by year. (b) Comparison of tagging effort to date for manta and devil rays. (c) Total tags deployed by species. Note that we specify tagging effort by IUCN species classifications, but use the same color for M. japanica and M. mobular, which have been lumped into M. mobular but have different IUCN Red List classifications. (d) Distribution of acoustic tag deployments on mobulid rays by species. (e) Distribution of satellite tag deployments on mobulid rays by species. The mobulid tagging effort information was sourced from published literature on the topic as well as from other unpublished mobulid tracking studies known to the authors and their collaborators (summarized in Table S1). (See section 6).
6.1. Satellite Tagging
Technological advances in satellite tagging (Musyl et al., 2011; Hussey et al., 2015) are providing tools to explore topics of direct relevance to mobulid rays such as fine-scale habitat use (e.g., Fastloc technology, Wildlife Computers) and fishing mortality (e.g., mark-report or survivorship tags, Wildlife Computers). Most studies of mobulid movements have employed pop-up satellite archival transmitting (PSAT) tags (Table S1), which typically yield long retention times but coarse position estimates (i.e., one or two positions per day estimated from light levels and SST measurements) (Braun et al., 2015). Using these, mobulid rays have been successfully tracked for periods of up to a year, revealing regional philopatric movements and strong affinity to shelf edge habitats in manta rays (Braun et al., 2014; Jaine et al., 2014; Stewart et al., 2016a) as well as long-distance offshore movements in devil rays (i.e., > 1,500 km, Thorrold et al., 2014; Francis and Jones, 2016). In contrast, towed Argos-linked tags require long tethers to facilitate surface satellite communications, leading to shorter deployments (poor tag retention) but higher resolution position estimates whenever the tag surfaces. These have been successfully used to investigate short-term (i.e., up to 64 days) foraging movements of manta rays in the Gulf of Mexico (Graham et al., 2012). More recently, towed tags enabled with fast-acquisition GPS technology (e.g., Fastloc, Wildlife Computers) have produced valuable data on the fine-scale movements of manta rays by providing both light-based geolocation estimates as well as accurate GPS positions upon surfacing, for periods of up to 6 months (M. Erdmann pers. com.). Fastloc positions require less surface time, allowing for shorter tethers and longer retention times than Argos-linked tags, but do not transmit archived high-resolution GPS positions until the tag detaches from the animal. Recent improvements to geolocation and subsequent state space models are quantifying and reducing the levels of uncertainty associated with light-based geolocation (Patterson et al., 2008; Jonsen et al., 2013; Gredzens et al., 2014; Braun et al., 2018). It is likely that with the increasing capacity to make fine-scale observations, movement patterns will indicate significant overlap with anthropogenic threats or sensitive areas (Braun et al., 2015; Kessel et al., 2017; Pikesley et al., 2018). Researchers could therefore incorporate metrics such as the Human Impact Index (Halpern et al., 2008) in future mapping of core mobulid utilization areas to identify time periods and locations where mobulid populations are likely to be impacted by human activities. Fine-scale habitat use assessments will be facilitated by increased use of Argos-linked and Fastloc tags, and researchers could make efforts to improve retention times for these tag types, perhaps by implementing new attachment methods such as dorsal fin mounts (e.g., Kessel et al., 2017). In addition to contributing important spatial information about the conservation needs of these species, such information will enhance our understanding of the global connectivity of mobulid populations with respect to gene flow and potential isolation.
6.2. Acoustic Tagging
Compared with satellite tags, the affordability and longer battery life of acoustic tags enables the collection of larger datasets on mobulid movements, site fidelity and habitat use than studies employing satellite tags. The increased battery life of acoustic transmitters (up to 10 years) will also greatly benefit efforts to monitor site fidelity and residency in mobulid species that are rarely encountered and are often only tagged opportunistically (e.g., M. mobular). However, the long-term attachment of external tags on mobulid rays over months to years remains a challenge. Future studies should consider internal tagging where possible (e.g. Kessel et al., 2017; Croll unpublished). Additionally, acoustic tags only provide positions when they are in range of acoustic receiver stations, limiting their utility for wide-ranging mobulids that routinely travel outside of receiver arrays. Researchers could make use of collaborative regional acoustic sensor networks to expand monitoring capacity (e.g., Australia's Integrated Marine Observing System—Animal Tracking Facility network, The Atlantic Cooperative Telemetry Network, The Ocean Tracking Network, iTAG network in the Gulf of Mexico). Large scale collaborative efforts will inform the design of management strategies on national (or smaller) scales (Lea et al., 2016) and facilitate the protection of key aggregation sites, especially for the philopatric manta species (Graham et al., 2012; Jaine et al., 2014; Braun et al., 2015; Stewart et al., 2016a; Kessel et al., 2017).
6.3. Critical Habitats
The ability of researchers to identify and predict areas of critical habitat for mobulid rays will be improved with increasing amounts of movement data collected through telemetry studies (Hays et al., 2016; Sequeira et al., 2018). Density maps (e.g., kernel utilization density, minimum convex polygon) can be constructed from horizontal movement data to reflect the core area use of mobulid species (Croll et al., 2012; Kessel et al., 2017). Historical observations of mobulid presence and aggregation events may provide novel and temporal insight into species distributions. Researchers should consider Unmanned Aerial Vehicle (UAV) technology or aerial surveys to provide additional opportunities to collect quantitative and behavioral data of mobulids (Hodgson et al., 2013; Girondot et al., 2014; Notarbartolo di Sciara et al., 2015; Weeks et al., 2015; Schofield et al., 2017; Pikesley et al., 2018). It is highly likely that oceanographic features and their influence on the distribution of patchy prey resources play a key role in driving the behavior of planktivores (Sims et al., 2006; Rohner et al., 2014; Weeks et al., 2015). Studies should include oceanographic and biological information, such as prey field density and frontal systems, in their spatial analyses to expand our current understanding of mobulid movement patterns (e.g., Papastamatiou et al., 2012; McCauley et al., 2014). Together, these data can be used in ecosystem niche modeling to identify potential new regions of importance to mobulid species (Panigada et al., 2017). The position uncertainty inherent in light-based geolocation tags impedes the incorporation and comparison of oceanographic data with movements as prey fields and oceanographic features can vary widely within the error radius of a single position estimate. Increased use of Argos-linked transmitters with more precise position estimates may facilitate the inclusion of these important physical and biological features into spatial analyses. It is crucial to gain a broader understanding of mobulid habitat use to better understand the effects of anthropogenic activities (Graham et al., 2012) and shifts in plankton communities associated with predicted climate-driven changes in ocean circulation (Hays et al., 2005; Harley et al., 2006; Richardson, 2008). Ontogenetic shifts in movements and habitat use are common in elasmobranchs (Grubbs, 2010). Juvenile mobulids are rarely seen and presumably exhibit size or age segregation until they approach maturity (Stewart et al., 2018b; Palacios et al. in prep). Consequently, critical habitat use and movements likely vary among life stages in mobulid rays. Future studies could seek to address these ontogenetic differences, in particular by filling knowledge gaps in the movements and habitat use of juvenile and young-of-year mobulids.
6.4. Large Collaborative Efforts
Ongoing efforts to monitor mobulid movements through photo-ID (Kumli and Rubin, 2010; Couturier et al., 2011; Germanov and Marshall, 2014; Stevens, 2016), telemetry (Croll et al., 2012; Jaine et al., 2014; Stewart et al., 2016a) and genetic studies are collectively beneficial to the future of mobulid research and conservation, and should be continued with an emphasis on collaboration among research groups at a global scale to maximize the likelihood of detecting population connectivity (Andrzejaczek et al., 2016). Fortunately, strong collaborative momentum within the scientific community and developments in analytical tools are now allowing for telemetry studies to take place at unprecedented scales, tracking movements of a multitude of marine species across oceans and over several years (e.g., Brodie et al., 2018b; Sequeira et al., 2018). Acoustic and satellite telemetry studies continue to provide insights into the movement and spatial use patterns of mobulids (Croll et al., 2012; Jaine et al., 2014; Stewart et al., 2016a; Couturier et al., 2018), although the majority of research has comprised single-species and single location studies. This site and species specificity in combination with limited sample sizes does not sufficiently support our understanding of broad-scale patterns at the population or species level. We recommend that future studies emphasize multi-species and multi-region tagging efforts to define critical habitats, resolve patterns in movement and connectivity among populations, improve our understanding of species-specific drivers of movement, and support efforts for management and bycatch reduction.
7. Foraging and Diving
Determining drivers of mobulid aggregative and migratory behavior and how they are linked to foraging opportunities can help identify where these species are most susceptible to direct or incidental capture in fisheries (Rohner et al., 2017; Stewart et al., 2017a) and predict their response to climate-driven changes in prey distribution. Mobulids are restricted to tropical and subtropical oceans, which in many regions are oligotrophic and may have lower zooplankton biomass than temperate and polar systems (Moriarty and O'Brien, 2013). This means that many populations of mobulids must find high biomass food patches in a dilute food environment. Stomach content analysis suggests that mobulids are opportunistic feeders that exploit zooplankton and, sometimes, teleost prey that are available in high densities (Figure 7). For example, in Mexico and the Philippines, several mobulid species (M. birostris, M. mobular, M. thurstoni, and M. tarapacana) feed on seasonally abundant euphausiids, with little to no niche partitioning observed in co-occurring species (Notarbartolo di Sciara, 1988; Rohner et al., 2017). Similarly, studies evaluating the prey community alongside feeding mobulids showed that M. alfredi and M. birostris engage in feeding activities when high biomass prey are available and when zooplankton meet specific density thresholds (Armstrong et al., 2016; Burgess, 2017). Results from studies using stable isotopes and fatty acids as trophic tracers support direct observation data that mobulids are predominantly consumers of zooplankton, but that fishes make up a notable portion of their diet in some regions (Sampson et al., 2010; Couturier et al., 2013; Burgess et al., 2016; Stewart et al., 2017a). Further, some studies have suggested that M. birostris feed on mesopelagic (Burgess et al., 2016, 2018; Rohner et al., 2017) and M. alfredi feed on demersal (Couturier et al., 2013) prey. Diving capacities and behaviors of several mobulid species also support the hypothesis that these animals can exploit prey in deep oceanic layers (Canese et al., 2011; Braun et al., 2014; Jaine et al., 2014; Thorrold et al., 2014; Stewart et al., 2016b). To help predict spatiotemporal distributions of mobulids, future studies should aim to identify common characteristics among their prey species such as community composition, biomass, size spectra and swimming capacity. Further, examination of diving capacities of mobulids will provide a better understanding of different foraging strategies across their range and how these are affected by environmental variability (e.g., water stratification, oxygen minimum zone). Knowledge of prey landscapes alongside drivers and types of movement is key to habitat modeling efforts and the implementation of effective conservation measures for mobulids.
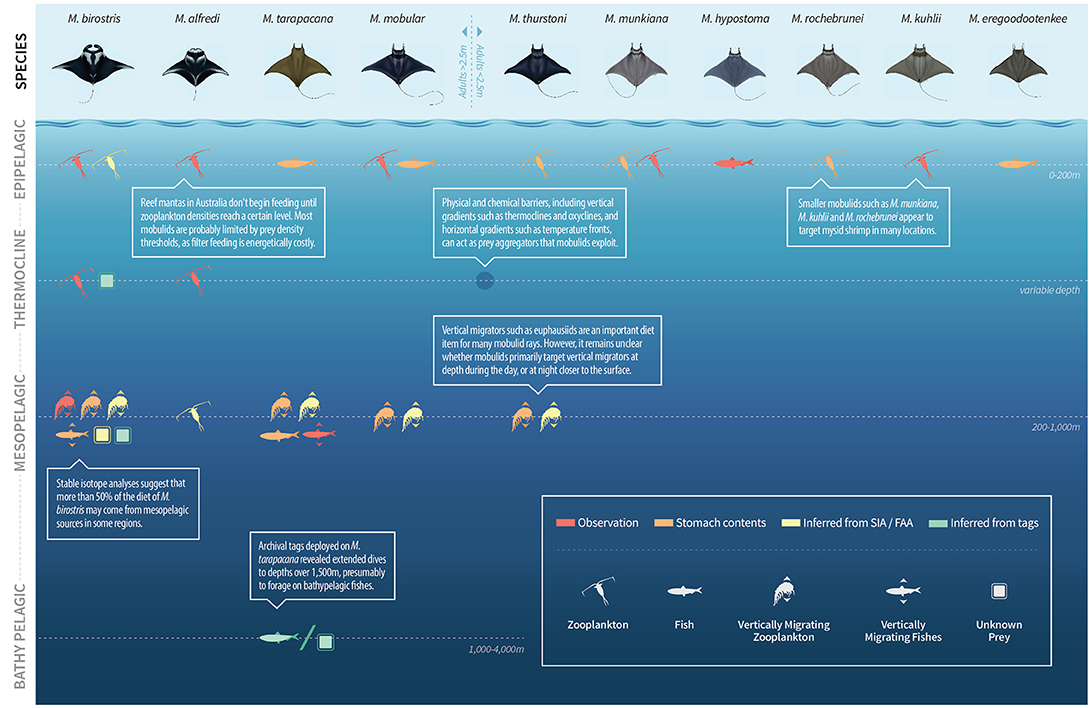
Figure 7. Foraging behavior of mobulid rays. The majority of direct observations of foraging are from epipelagic waters. However, a broad range of methods including isotope and fatty acid analysis, archival satellite tagging, stomach content analysis and submersible observations indicate that mesopelagic prey are important diet items for mobulids. References for this figure are listed in Table S2. Mobulid illustrations © Marc Dando. Figure design by Madeline Wukusick www.communique.design. (See section 7).
7.1. Incorporating Prey Data Into Mobulid Studies
Understanding oceanographic mechanisms that influence the distribution of mobulids will be important for identifying critical habitats that warrant special protection (see section 6.3. Movements: Critical habitats). However, the temporal lag between primary productivity, which can be observed via satellite, and secondary productivity (i.e., zooplankton), which presumably drives mobulid movements and diving behavior, can limit inferences on mobulid feeding ecology from remotely-sensed datasets (Lambert et al., 2014). Incorporating prey (e.g., zooplankton, mesopelagic fishes) and oceanographic data into distribution and foraging studies will be essential for identifying the environmental and food web patterns that drive mobulid movements and distributions. When possible, researchers should strive to collect prey data concurrently with mobulid sightings and behavioral data (McCauley et al., 2014; Armstrong et al., 2016; Burgess, 2017). However, in studies using telemetry and fisheries data this may not be feasible. Instead, researchers could take advantage of existing oceanographic datasets with high-resolution prey data (e.g., Bograd et al., 2003). We encourage researchers to consider what prey data are available at or near their proposed study region, and even to consider specifically targeting regions with large oceanographic datasets for future mobulid research. Additionally, we encourage researchers to collaborate with oceanographers and cruise planners to help ensure that large-scale zooplankton data collection efforts are most relevant to studies of higher trophic level organisms including mobulids. Bridging the gap between oceanographic data and mobulid movements and foraging behavior will allow for more robust analyses of distribution, critical habitat use, bycatch risk, and climate impacts.
7.2. Improving Inference From Isotope and Fatty Acid Analyses
Given the limitations of temporal coverage in stomach content analyses, a number of studies have employed stable isotope analysis and fatty acid profiling to examine the diet of mobulid rays (Sampson et al., 2010; Couturier et al., 2013; Burgess et al., 2016, 2018; Stewart et al., 2017a). While diet-tissue discrimination values and turnover rates have been examined for several elasmobranchs (Hussey et al., 2010; Kim et al., 2012a,b), they have not been determined for any mobulid species to date, meaning that inferences of diet and trophic position in mobulids have been based entirely on estimated values. Captive feeding experiments on mobulids under controlled conditions in aquaria are needed to evaluate turnover times, diet tissue discrimination factors, and validate the use of other tissue types that can be sampled by non-invasive means for biochemical analyses, such as mucus (Burgess et al., 2017). Standardized protocols for sample preservation (e.g., ethanol vs. frozen) and analyses should also be developed to allow for direct comparisons among studies to improve general knowledge on mobulid feeding ecology. Future biochemical work on mobulid species could also consider compound-specific isotope analysis, a relatively new analytic tool in dietary studies (Mcmahon et al., 2015; McMahon et al., 2016). This technique provides a finer resolution on the origin of individual components (e.g., selected fatty acids, amino acids, methyl mercury) and contributes to discriminating essential food sources in the species' diet that cannot be determined through other methods (Bec et al., 2011). In all isotopic studies, adequate sampling of the food web and candidate diet items identified in observational studies and stomach contents is necessary to parameterize mixing models and quantify dietary contributions (Burgess et al., 2016; Stewart et al., 2017a). By combining several biochemical techniques and using large datasets, future studies will bring new understanding on trophic markers resulting from the different analyses and allow for more robust data interpretations on the dynamics of energy transfer in the pelagic environment.
7.3. Linking Diving Behavior and Mesopelagic Prey
Over the past decade, there has been an increased effort in using tagging technology to assess the vertical movement of mobulids and associated mesopelagic foraging ability. Telemetry studies of other marine megafauna species have identified that the deep scattering layer (Goldbogen et al., 2015), water stratification (Houssard et al., 2017), the oxygen minimum layer (Carlisle et al., 2017), temperature (Andrzejaczek et al., 2018) and prey availability (Womble et al., 2014) can influence diving behavior as well as depth distribution. For mobulid species, all telemetry studies have shown that individuals spend most of their time within the upper 50 m of the water column where the likelihood of encountering fishing gear is greatest. All monitored species also display capacities to occupy mesopelagic depths, with some even able to reach bathypelagic regions (1,000 m+) (Canese et al., 2011; Jaine et al., 2014; Thorrold et al., 2014; Francis and Jones, 2016; Stewart et al., 2016b). Several studies suggest that mesopelagic food sources comprise a major part of mobulid diet (Burgess et al., 2016, 2018; Rohner et al., 2017; Stewart et al., 2017a), but mesopelagic prey are yet to be defined in terms of composition and availability relative to alternative food sources such as surface zooplankton (Couturier et al., 2013; Burgess et al., 2016, 2018). Additionally, vertical migrators such as euphausiids and myctophids that appear to be an important prey source for mobulids may allow them to feed on mesopelagic prey sources without accessing mesopelagic environments (Burgess et al., 2018; Stewart et al., 2018a) This may be especially relevant in shelf-edge environments, where prey such as euphausiids, large copepods and myctophids undergo large vertical migrations from mesopelagic depths to the epipelagic (Hopkins, 1982; Kaltenberg et al., 2007; Hazen and Johnston, 2010). Many manta ray aggregation sites are adjacent to shelf breaks, and telemetry data suggest that individuals may depart coastal habitats at night (Burgess, 2017; Couturier et al., 2018), possibly to access vertical migrators in deeper waters. Obtaining new information on mesopelagic prey sources may require targeted field expeditions that sample prey at depth, which can be done with opening-closing nets, or could employ technology such as submersibles (Stewart et al., 2016b), remotely operated vehicles or remote cameras. A better understanding of mobulids' reliance on these food sources and habitats will lead to improved characterization of aggregation sites, as well as habitat and species distribution models.
7.4. New Technology in Foraging Studies
As technology develops, so too does the ability to track and monitor mobulids in remote and deep realms of the ocean. Animal-borne cameras (Stewart et al., 2017b; Fontes et al., 2018; Stewart unpublished) and accelerometers facilitate short-term visual surveys of an individuals' feeding behavior and prey choice, and the characterization and recording of feeding events, respectively. In studies of the diving behavior of other marine species, accelerometers have been successfully used to remotely assess diving energetics in the whale shark (Gleiss et al., 2013), and to identify feeding events in penguins (Watanabe and Takahashi, 2013). We therefore encourage researchers to apply accelerometers to future studies of mobulid feeding and diving behavior. This will require advances in attachment methods that allow accelerometer orientation to remain stable on mobulids, and exploring options for longer-term deployments and instrument recovery. New sensory systems can also be incorporated into existing telemetry devices. For example, fluorometers embedded into satellite tags can provide in situ measurements of phytoplankton fluorescence that can be used to calculate chlorophyll-a concentrations and to assess primary productivity levels alongside animal movement data (Lander et al., 2015), with additional dissolved oxygen sensors able to associate dive and oxygen profiles along with temperature and salinity measurements (Bailleul et al., 2015; Coffey and Holland, 2015). The use of “sonar tags” that are capable of recording prey field density concurrently with movement tracks would allow for detailed studies of mobulid movements and prey targeting, although this currently requires tag recovery (Lawson et al., 2015), which may limit applications to coastal species such as reef manta rays.
8. Pollution and Contaminants
Mobulid rays, as filter feeders, may be susceptible to ingesting marine pollutants and contaminants such as persistent organic pollutants (POPs), heavy metals, and microplastics. Pollutants enter the marine environment through wastewater, poor industry practices, and degradation of marine debris, among other sources. Microplastics contain added pollutants and toxins such as phthalates, bisphenol A, flame retardants, styrenes, and adsorb and concentrate persistent organic pollutants (POPs) and heavy metals from the marine environment (Worm et al., 2017). Many dissolved pollutants are lipophilic, persistent in the environment, and have the capacity to bio accumulate up the food chain, with large bodied, long-lived species at high risk to exposure (Niimi, 1996). Mobulid habitats, including foraging grounds, overlap with microplastic pollution hotspots in many cases (Germanov et al., 2018). However, rates of plastic ingestion by mobulids, bioaccumulation of pollutants, and the impacts of plastic pollution on mobulid biology, ecology, and population viability have not been studied. Future research should work toward identifying the scale of these problems and quantifying impacts so they can be evaluated in a management context.
8.1. Identifying Trophic Interactions With Pollutants
In locations with high densities of floating microplastics, mobulids may directly ingest microplastics (Figure 8). Additionally, zooplankton can be contaminated with pollutants and toxins (Fossi et al., 2014) as well as ingest microplastics and nanoplastics (Cole et al., 2013; Setälä et al., 2014). This suggests that mobulids may be secondary consumers of microplastics and associated pollutants even if they are foraging in locations (or at depths) that do not have high densities of floating microplastics. Future research should seek to quantify ingestion rates of microplastics in mobulids. This could be accomplished by evaluating microplastic densities and contamination in prey assemblages by sampling prey alongside feeding mobulids. Additionally, measuring the abundance of microplastic particles in stomach contents of mobulids landed in fisheries could provide estimates of direct ingestion. In order to evaluate whether ingestion leads to bioaccumulation in tissues, researchers should measure phthalate, heavy metal, and POP levels in mobulid tissue biopsies from wild populations and samples collected from fisheries. Previous studies found elevated levels of some heavy metals in mobulid tissues (Essumang, 2009, 2010; Ooi et al., 2015), but low levels of POPs (Germanov et al., in prep; Fernando, unpublished). Phthalates and/or POPs have been recorded in tissue samples of baleen whales, basking sharks and whale sharks in areas with high levels of microplastic pollution (Fossi et al., 2014, 2016, 2017), indicating that filter feeding organisms are likely bioaccumulating these pollutants as a result of plastic ingestion. Future research in mobulids could evaluate levels of phthalates, POPs and heavy metals in mobulid tissues across gradients of microplastic pollution to determine how bioaccumulation scales with pollutant levels in the environment and to identify heavily impacted populations. Sources and aggregating mechanisms of plastic pollution vary between coastal and offshore environments, leading to differing levels of overlap between microplastic hotspots and mobulid habitats (Germanov et al., 2018). Researchers could investigate differences in pollutant contamination between coastal mobulids (e.g., M. alfredi, M. munkiana) and offshore species, as well as species with different foraging strategies to identify risk factors for plastic ingestion. Once trophic interactions between mobulids and pollutants are better understood, researchers could endeavor to identify high-risk areas for mobulids following existing approaches for other taxa such as sea turtles (Schuyler et al., 2016).
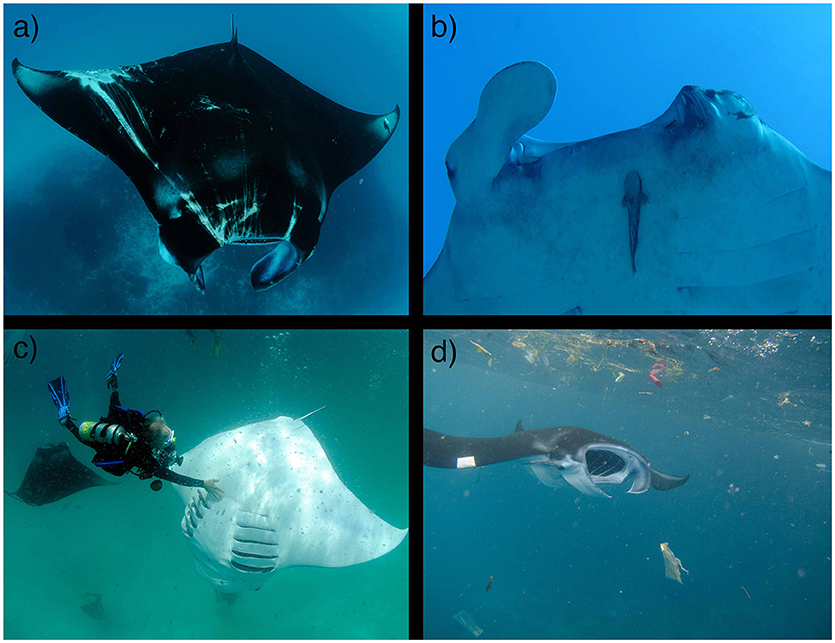
Figure 8. Sub-lethal impacts on mobulids. Entanglement in fishing gear can cause substantial damage and scarring (a), including amputations of cephalic fins and damage to eyes (b). (c) Harassment by tourists and heavy tourism pressure at important feeding and cleaning sites may lead to changes in behavior, especially for resident coastal species such as M. alfredi. (d) As filter feeders, mobulids may be vulnerable to ingestion of microplastics and other pollutants. The impacts of these stressors on mobulid foraging success, reproductive output, and population viability are poorly studied. Photo copyrights: (a) Guy Stevens; (b) Lydie Couturier; (c) Guy Stevens, used with consent; (d) Elitza Germanov. (See sections 8 and 9).
8.2. Quantifying Impacts From Pollutants
A number of recent studies have demonstrated that microplastics, POPs and heavy metals impact regular cellular and system functioning, including endocrine disruption, leading to knock-on negative impacts on reproductive output with the potential to alter populations and ecological assemblages of marine species (Jakimska et al., 2011; Rochman, 2013; Rochman et al., 2014; Galloway and Lewis, 2016; Sussarellu et al., 2016; Germanov et al., 2018). The implications of exposure to pollution and contaminants in mobulids is still speculative, especially at the level of individual fitness and population viability. Demonstrating that mobulids ingest microplastics and other pollutants is important for assessing the extent of these threats, but will be insufficient for evaluating the true impact of pollutants and their importance as a management consideration. Future studies should seek to quantify the impacts of plastic pollution on mobulids by, for example, comparing pollutant concentrations in the environment and in mobulid tissues with metrics of individual and population health such as body condition, reproductive output, population trends, and individual survival. Studies that compare populations across a pollutant gradient, or evaluate a single population through time as pollutant levels fluctuate could provide insights into the true impacts of plastics and other pollutants and their importance as an emerging management consideration.
9. Sub-Lethal Impacts
Sub-lethal threats such as entanglement, tourism pressure, habitat destruction, and climate change are likely to considerably impact mobulid survival throughout their range (Couturier et al., 2012) (Figure 8). Anthropogenic injuries resulting from foul hooking, entanglement in fishing gear, and boat strikes are evident in every monitored mobulid population across the globe, including severe injuries such as amputation or deformity of cephalic and pectoral fins, and damage to the eyes (Deakos et al., 2011). While these threats are known, the extent to which these impacts may affect individual health and overall population fitness is unclear (Couturier et al., 2012; Croll et al., 2016). Mobulid-focused tourism activities are increasingly promoted as a non-consumptive and more sustainable alternative to fishing practices, particularly in developing countries (Anderson et al., 2010; O'Malley et al., 2013; Venables et al., 2016b). Potential impacts of unregulated tourism activities at mobulid aggregation sites have been widely recognized. Initial investigations observed short-term behavioral changes including the termination of feeding or cleaning behaviors during tourism interactions with M. alfredi in Australia (Venables et al., 2016a) and the Maldives (Murray et al., in review). Future research priorities lie in accurately documenting and quantifying sub-lethal stressors, while determining the severity of different types of impacts to prioritize mitigation measures and develop effective management strategies.
9.1. Injuries and Entanglement
For well-studied, resident populations, documenting how well and how quickly injuries heal over time will be informative. Additionally, researchers could track metrics such as body size, growth rates, and pregnancy rates between injured and healthy individuals to determine the impacts of different types of injuries on an individual's fitness. Researchers could evaluate the frequency of injuries in different age classes and sexes in order to characterize ontogenetic variability of impacts. Comparing differences in the magnitude of the threats among populations of the same species will guide appropriate management strategies or codes of conduct that specifically address local needs and effectively mitigate regional threats. For example, certain populations may more commonly exhibit surface ram feeding to target a preferred food source, subjecting individuals to a greater threat of collision with boats or propeller injuries. Other populations may inhabit an environment littered with fishing nets due to regional fishing practices, creating a greater threat of entanglement. Proper characterization and quantification of these threats and their individual and population-level impacts is critical for effective, local management of mobulid populations.
9.2. Tourism Impacts
As the nature and intensity of tourism activities vary considerably among locations, specific aggregation sites and focal species, we recommend site-specific assessments and long-term monitoring of local tourism industries to determine their effect on mobulid populations. Researchers could focus on identifying and quantifying avoidance behaviors and their causes, determining carrying capacities to set limits for divers/boats, assessments of diver damage to cleaning stations, and identification of vessel limitation or exclusion zones. Aerial and underwater drone technology or unmanned stationary cameras could be used as passive observation methods to avoid observers or boats impacting natural behaviors and confounding results (e.g., Garrud, 2016). Visits to cleaning stations provide many perceived benefits to the fitness of visiting clients including parasite removal, wound cleaning and even improved cognitive performance (Grutter, 1999; Binning et al., 2018), while serving as settings for important social and reproductive interactions (Marshall and Bennett, 2010; Stevens, 2016). Future research could aim to determine whether short-term disturbances lead to biologically significant impacts, such as decreased health (e.g., body condition), reduced energy intake leading to slower growth rates, or declines in reproductive success (Lusseau, 2004; Bejder et al., 2006; Williams et al., 2006). The implications of tourism activities using artificial lighting to attract plankton (Osada, 2010) could be tested through long-term comparative studies quantifying growth and pregnancy rates as fitness indicators for individuals that do and do not partake in nighttime feeding activities. Additionally, telemetry methods can be used to determine if individuals preferentially visit provisioning sites, altering natural behavior (Hammerschlag et al., 2017). Passive interactions and adherence to a code of conduct have been recommended as precautionary measures to decrease the likelihood of disturbance (Garrud, 2016; Venables et al., 2016a; Murray et al., in review). Assessments of the effectiveness of established codes of conduct during different interaction scenarios are essential to refine and improve regulations (Murray et al., in review).
9.3. Habitat Degradation and Climate Change
Habitat destruction and degradation can directly impact species survivorship and responses to environmental change, making it a leading cause of biodiversity loss (Dobson et al., 2006). Anthropogenic pressures on coastal environments such as aquaculture, dredging and coastal runoff are potential stressors to marine communities, including pelagic visitors. Coastal reefs in tropical and subtropical waters are preferred habitats for several mobulid species where individuals can show high site fidelity and in some cases long-term residency (Marshall and Bennett, 2010; Deakos et al., 2011; Jaine et al., 2012; Couturier et al., 2018; F. McGregor pers. obs.). Lagoon habitats, which may serve as important nursery areas, are especially sensitive to habitat degradation, pollution, and sedimentation (McCauley et al., 2012, 2014). Future research could investigate habitat selectivity of mobulid rays to determine if individuals preferentially occupy and use healthy versus degraded reef habitat, and how these preferences affect behaviors such as feeding, cleaning and socializing. This information can then be used to inform which regions require additional management actions, such as marine protected areas, or reduction of upstream aquaculture and agricultural activities.
Climate change is expected to cause further disturbances in marine ecosystems, affecting the reproductive biology, abundance and survival of many species. Warming oceans cause changes in ocean acidity, oxygen content, oceanic circulation and primary productivity dynamics, ultimately affecting food web structure and the distribution and availability of mobulid prey (Moloney et al., 2011). The major impact of climate change on mobulids is likely to be the projected decline in zooplankton biomass in tropical waters. Biogeochemical models project a decline in zooplankton biomass in the future of about 10% globally (Chust et al., 2014; Stock et al., 2014; Woodworth-Jefcoats et al., 2017), but some regions, particularly those in the tropics, could experience >50% declines (Stock et al., 2014). While it is unknown how this broad-scale decline in zooplankton biomass at the tropics could impacts local areas where mobulids feed, the most likely outcome is that there will be lower zooplankton biomass available for mobulids and other zooplanktivores. Further work is needed to identify the relative importance of different mobulid feeding environments. For example, if it is confirmed that feeding at the shelf-edge or open ocean on vertically migrating zooplankton from the mesopelagic zone is more important than feeding on zooplankton concentrated by local oceanographic processes around small-scale aggregation sites, then it will be easier to project future changes in zooplankton biomass from biogeochemical models, which are better at projecting large-scale rather than local-scale changes.
Conclusion
In addition to prioritizing critical knowledge gaps, there are several more general considerations that will make the results of future mobulid research most impactful and relevant to management. Perhaps the most important of these is methodological consistency. We have argued that understanding general patterns of movement, habitat use, and foraging behavior across species and regions will be essential for developing management strategies and mitigating bycatch. In many cases this will require aggregating data across study sites or incorporating previously published data into new studies and analyses. This becomes increasingly difficult and in some cases impossible when different methodologies have been used to address similar questions. To maximize the utility of future research efforts, researchers should make every effort to coordinate methodological approaches across studies and maintain methodological consistency with previously published studies, even if this means adding complexity to an existing or proposed study. This may include, for example, standardized measurement methods, tissue collection protocols, sequencing the same genomic regions for comparability across genetic datasets, sample preservation methods for isotope analysis (e.g., ethanol vs. frozen), archival tag programming, or field survey methods. In addition, we recommend the development of guidelines for standardized capture and handling protocols to minimize stress to animals and maximize sampling efficiency. Achieving this will require improved and ongoing communication among research groups and would benefit from a coordinated effort or working group aimed at creating standardized research protocols.
Second, long-term data sets are critical for answering many pressing questions about mobulid ecology and biology. For example, multi-year or multi-decadal telemetry datasets are required to understand interannual variability and environmental drivers of movements and habitat use in mobulids. For such long-lived animals, long-term (ideally multi-decadal) photo-ID databases are necessary to accurately estimate key parameters such as survival and fecundity, and to capture the variability that is important for stochastic population projection models. We encourage researchers to make every effort to continue long-running research programs that have the potential to answer key questions proposed in this paper, even at the expense of initiating new projects. There are many situations where multiple research groups working in the same region may be able to pool resources to maintain key project areas for extended periods.
Finally, to make their work directly applicable to regional management actions, researchers should involve resource managers in planning discussions and even research activities where applicable. Management priorities will vary by region, and having an understanding of local needs and knowledge gaps will help guide research programs and maximize their efficacy. We encourage newcomers and established experts in the field of mobulid research to use the priority areas outlined in this paper as a roadmap for future research activities. However, this should be adapted to local and regional management needs and will depend greatly on the specimens and study systems available. We believe that if progress is made in these research areas over the next decade, it will result in significant contributions to the management and conservation of these threatened species.
Author Contributions
JS, FJ, and GS conceived of the paper and figure concepts. JH led the Taxonomy & diversity section. BL led the Life history section. JS led the Reproduction & nursery areas section. CD led the Population trends section. DF led the Fisheries & bycatch section. AJA led the Spatial dynamics & movements section. KB led the Foraging & diving section. EG led the Pollution & contaminants section. SV led the Sub-lethal impacts section. All authors contributed to writing and editing the manuscript.
Conflict of Interest Statement
NL-O was affiliated with AZTI. DF was affiliated with Blue Resources Trust. EG, TK, AM, and SV were affiliated with the Marine Megafauna Foundation. JS, DF, NF, LP, and GS were affiliated with The Manta Trust.
The remaining authors declare that the research was conducted in the absence of any commercial or financial relationships that could be construed as a potential conflict of interest.
Acknowledgments
JS was supported by NOAA ONMS Nancy Foster Scholarship NA15NOS4290068. LC is supported by the Laboratoire d'Excellence LabexMER (ANR-10-LABX-19). SV is supported by the Bruce & Betty Green Scholarship. AJA, AOA, MB, CD, FM, AR and KT were all supported by an Australian Research Council Linkage grant #LP150100669. JH is supported by a NERC CASE studentship through the ENVISION DTP (CASE partner - Royal Zoological Society of Scotland) and has received grants from the Save Our Seas Foundation, The People's Trust for Endangered Species, the Fisheries Society of the British Isles and the Genetics Society. EG is supported by Australian Postgraduate Award & Murdoch International Top Up, Ocean Park Conservation Foundation, Fortuna Foundation, the PADI Foundation, and Idea Wild. The Manta Trust supported publishing fees for this open access article.
Supplementary Material
The Supplementary Material for this article can be found online at: https://www.frontiersin.org/articles/10.3389/fmars.2018.00314/full#supplementary-material
References
Adnet, S., Cappetta, H., Guinot, G., and Di Sciara, G. N. (2012). Evolutionary history of the devilrays (Chondrichthyes: Myliobatiformes) from fossil and morphological inference. Zool. J. Linn. Soc. 166, 132–159. doi: 10.1111/j.1096-3642.2012.00844.x
Agnew, D. J., Pearce, J., Pramod, G., Peatman, T., Watson, R., Beddington, J. R., et al. (2009). Estimating the worldwide extent of illegal fishing. PLoS ONE 4:e4570. doi: 10.1371/journal.pone.0004570
Alfaro-Cordova, E., Del Solar, A., Alfaro-Shigueto, J., Mangel, J. C., Diaz, B., Carrillo, O., et al. (2017). Captures of manta and devil rays by small-scale gillnet fisheries in northern Peru. Fish. Res. 195, 28–36. doi: 10.1016/j.fishres.2017.06.012
Anderson, R. C., Adam, M. S., Kitchen-Wheeler, A. M., and Stevens, G. (2010). Extent and economic value of manta ray watching in Maldives. Tour. Mar. Environ. 7, 15–27. doi: 10.3727/154427310X12826772784793
Andrzejaczek, S., Gleiss, A. C., Jordan, L. K. B., Pattiaratchi, C. B., Howey, L. A., Brooks, E. J., et al. (2018). Temperature and the vertical movements of oceanic whitetip sharks, Carcharhinus longimanus. Sci. Rep. 8:8351. doi: 10.1038/s41598-018-26485-3
Andrzejaczek, S., Meeuwig, J., Rowat, D., Pierce, S., Davies, T., Fisher, R., et al. (2016). The ecological connectivity of whale shark aggregations in the Indian Ocean: a photo-identification approach. R. Soc. Open Sci. 3:160455. doi: 10.1098/rsos.160455
Armstrong, A. O., Armstrong, A. J., Jaine, F. R. A., Couturier, L. I. E., Fiora, K., Uribe-Palomino, J., et al. (2016). Prey density threshold and tidal influence on reef manta ray foraging at an aggregation site on the Great Barrier Reef. PLoS ONE 11:e0153393. doi: 10.1371/journal.pone.0153393
Bailleul, F., Vacquie-Garcia, J., and Guinet, C. (2015). Dissolved oxygen sensor in animal-borne instruments: an innovation for monitoring the health of oceans and investigating the functioning of marine ecosystems. PLoS ONE 10:e0132681. doi: 10.1371/journal.pone.0132681
Bec, A., Perga, M. E., Koussoroplis, A., Bardoux, G., Desvilettes, C., Bourdier, G., et al. (2011). Assessing the reliability of fatty acid-specific stable isotope analysis for trophic studies. Methods Ecol Evol. 2, 651–659. doi: 10.1111/j.2041-210X.2011.00111.x
Bejder, L., Samuels, A., Whitehead, H., Gales, N., Mann, J., Connor, R., et al. (2006). Decline in relative abundance of bottlenose dolphins exposed to long-term disturbance. Conserv. Biol. 20, 1791–1798. doi: 10.1111/j.1523-1739.2006.00540.x
Beverton, R. J. H., and Holt, S. J. (1959). “A review of the lifespans and mortality rates of fish in nature, and their relation to growth and other physiological characteristics,” in Ciba Foundation Symposium-The Lifespan of Animals (Colloquia on Ageing), Vol. 5 (Chichester: John Wiley & Sons, Ltd.).
Bigelow, H., and Schroeder, W. C. (1953). Fishes of the western North Atlantic. Sawfishes, guitarfishes, skates, rays, and chimaeroids. Mem. Sears. Found. Mar. Res. 1, 500–514.
Binning, S. A., Roche, D. G., Grutter, A. S., Colosio, S., Sun, D., Miest, J., et al. (2018). Cleaner wrasse indirectly affect the cognitive performance of a damselfish through ectoparasite removal. Proc. R. Soc. B 285:20172447. doi: 10.1098/rspb.2017.2447
Bograd, S. J., Checkley, D. A., and Wooster, W. S. (2003). CalCOFI: A half century of physical, chemical, and biological research in the California Current System. Deep-sea Res. II Top. Stud. Oceanogr. 50, 2349–2353. doi: 10.1016/S0967-0645(03)00122-X
Bonney, R., Cooper, C. B., Dickinson, J., Kelling, S., Phillips, T., Rosenberg, K. V., et al. (2009). Citizen science: a developing tool for expanding science knowledge and scientific literacy. BioScience 59, 977–984. doi: 10.1525/bio.2009.59.11.9
Braun, C. D., Galuardi, B., and Thorrold, S. R. (2018). HMMoce: an R package for improved geolocation of archival-tagged fishes using a hidden Markov method. Methods Ecol. Evol. 2018, 1212–1220. doi: 10.1111/2041-210X.12959
Braun, C. D., Skomal, G. B., Thorrold, S. R., and Berumen, M. L. (2014). Diving behavior of the reef manta ray links coral reefs with adjacent deep pelagic habitats. PLoS ONE 9:e88170. doi: 10.1371/journal.pone.0088170
Braun, C. D., Skomal, G. B., Thorrold, S. R., and Berumen, M. L. (2015). Movements of the reef manta ray (Manta alfredi) in the Red Sea using satellite and acoustic telemetry. Mar. Boil. 162. doi: 10.1007/s00227-015-2760-3
Bravington, M. V., Grewe, P. M., and Davies, C. R. (2016a). Absolute abundance of southern bluefin tuna estimated by close-kin mark-recapture. Nat. Commun. 7, 1–8. doi: 10.1038/ncomms13162
Bravington, M. V., Skaug, H. J., and Anderson, E. C. (2016b). Close-Kin Mark-Recapture. Stat. Sci. 31, 259–274. doi: 10.1214/16-STS552
Broadhurst, M. K., Laglbauer, B. J. L., Burgess, K. B., and Coleman, M. A. (2018). Reproductive biology and range extension for Mobula kuhlii cf. eregoodootenkee. Endanger Species Res. 35, 71–80. doi: 10.3354/esr00876
Brodie, S., Jacox, M. G., Welch, H., Dewar, H., Scales, K. L., et al. (2018a). Integrating dynamic subsurface habitat metrics into species distribution models. Front. Mar. Sci. 5:219. doi: 10.3389/fmars.2018.00219
Brodie, S., Lédée, E. J. I., Heupel, M. R., Babcock, R. C., Campbell, H. A., Gledhill, D. C, et al. (2018b). Continental-scale animal tracking reveals functional movement classes across marine taxa. Sci. Rep. 8:3717. doi: 10.1038/s41598-018-21988-5
Bryan, J. L., Wildhaber, M. L., Papoulias, D. M., Delonay, A. J., Tillitt, D. E., and Annis, M. L. (2007). Estimation of gonad volume, fecundity, and reproductive stage of shovelnose sturgeon using sonography and endoscopy with application to the endangered pallid sturgeon. J. Appl. Ichthyol. 23, 411–419. doi: 10.1111/j.1439-0426.2007.00889.x
Burgess, K. B. (2017). Feeding Ecology and Habitat Use of the Giant Manta Ray Manta birostris at a Key Aggregation Site off Mainland Ecuador. Ph.D. thesis, Faculty of Medicine, The University of Queensland, Queensland. doi: 10.14264/uql.2018.157
Burgess, K. B., Couturier, L. I. E., Marshall, A. D., Richardson, A. J., Weeks, S., and Bennett, M. B. (2016). Manta birostris, predator of the deep? insight into the diet of the giant manta ray through stable isotope analysis. R. Soc. Open Sci. 3:160717. doi: 10.1098/rsos.160717
Burgess, K. B., Guerrero, M., Marshall, A. D., Richardson, A. J., Bennett, M. B., and Couturier, L. I. E. (2018). Novel signature fatty acid profile of the giant manta ray suggests reliance on an uncharacterised mesopelagic food source low in polyunsaturated fatty acids. PLoS ONE 13:e0186464. doi: 10.1371/journal.pone.0186464
Burgess, K. B. A., Guerrero, M. C., Richardson, A. J. D., and Bennett, M. B. A. (2017). Use of epidermal mucus in elasmobranch stable isotope studies: a pilot study using the giant manta ray (Manta birostris). Mar. Freshwater Res. 69, 336–342. doi: 10.1071/MF16355
Cadenat, J. (1960). Notes d'Ichthyologie ouest-africaine. XXIX. Les Mobulidae de la côte occidentale d'Afrique. Bulletin de l'Institut Français d'Afrique Noire 22, 1053–1084.
Cailliet, G. M., Smith, W. D., Mollet, H. F., and Goldman, K. J. (2006). Age and growth studies of chondrichthyan fishes: the need for consistency in terminology, verification, validation, and growth function fitting. Environ. Biol. Fishes 77, 211–228. doi: 10.1007/s10641-006-9105-5
Campana, S. E., Natanson, L. J., and Myklevoll, S. (2002). Bomb dating and age determination of large pelagic sharks. Can. J. Fish. Aquat. Sci. 59, 450–455. doi: 10.1139/f02-027
Canese, S., Cardinali, A., Romeo, T., Giusti, M., Salvati, E., Angiolillo, M., et al. (2011). Diving behavior of the giant devil ray in the Mediterranean Sea. Endangered Species Res. 14, 171–176. doi: 10.3354/esr00349
Carlisle, A. B., Kochevar, R. E., Arostegui, M. C., Ganong, J. E., Castleton, M., Schratwieser, J., et al. (2017). Influence of temperature and oxygen on the distribution of blue marlin (Makaira nigricans) in the Central Pacific. Fish. Oceanogr. 26, 34–48. doi: 10.1111/fog.12183
Carrier, J. C., Murru, F. L., Walsh, M. T., and Pratt, H. L. (2003). Assessing reproductive potential and gestation in nurse sharks (Ginglymostoma cirratum) using ultrasonography and endoscopy: an example of bridging the gap between field research and captive studies. Zoo Biol. 22, 179–187. doi: 10.1002/zoo.10088
Catchen, J., Hohenlohe, P. A., Bassham, S., Amores, A., and Cresko, W. A. (2013). Stacks: an analysis tool set for population genomics. Mol. Ecol. 22, 3124–3140. doi: 10.1111/mec.12354
Chapman, D. D., Babcock, E. A., Gruber, S. H., Dibattista, J. D., Franks, B. R., Kessel, S. A., et al. (2009). Long-term natal site-fidelity by immature lemon sharks (Negaprion brevirostris) at a subtropical island. Mole. Ecol. 18, 3500–3507. doi: 10.1111/j.1365-294X.2009.04289.x
Charnov, E. L. (1993). Life History Invariants: Some Explorations of Symmetry in Evolutionary Ecology, Vol. 6. Oxford University Press.
Chust, G., Allen, J. I., Bopp, L., Schrum, C., Holt, J., Tsiaras, K., et al. (2014). Biomass changes and trophic amplification of plankton in a warmer ocean. Global Change Biol. 20, 2124–2139. doi: 10.1111/gcb.12562
Clark, T. B. (2010). Abundance, Home Range, and Movement Patterns of Manta Rays (Manta alfredi, M. birostris) in Hawai‘i. Dissertation, University of Hawaii at Manoa, Honolulu.
Coffey, D. M., and Holland, K. N. (2015). First autonomous recording of in situ dissolved oxygen from free-ranging fish. Anim. Biotelem. 3, 1–9. doi: 10.1186/s40317-015-0088-x
Cole, M., Lindeque, P., Fileman, E., Halsband, C., Goodhead, R., Moger, J., et al. (2013). Microplastic ingestion by zooplankton. Environ. Sci. Technol. 47, 6646–6655. doi: 10.1021/es400663f
Coles, R. J. (1916). Natural history notes on the Devil Fish, Manta birostris (Walbaum) and Mobula olfersi (Müller). Bull. Am. Museum Nat. Hist. 35, 649–657.
Compagno, L. (1999). “Systematics and body form,” in Sharks, Skates and Rays: The Biology of Elasmobranch Fishes, ed W. C. Hamlett (Baltimore, MD: John Hopkins University Press), 1–42.
Compagno, L. J. V., and Last, P. R. (1999). “Mobulidae,” in FAO Species Identification Guide for Fishery Purposes, The Living Marine Resources of the Western Central Pacific, Vol. 3, Batoid Fishes, Chimaeras and Bony Fishes, Part 1 (Elopidae to Linophyrnidae), eds K. E. Carpenter and V. H. Niem (Rome: FAO), 1524–1529.
Cooch, E., and White, G. (2006). Program MARK: A Gentle Introduction. Ithaca, NY: Cornell University.
Cooke, S. J. (2008). Biotelemetry and biologging in endangered species research and animal conservation: relevance to regional, national, and IUCN Red List threat assessments. Endangered Species Res. 4, 165–185. doi: 10.3354/esr00063
Cortés, E. (2002). Incorporating uncertainty into demographic modeling: application to shark populations and their conservation. Conser. Biol. 16, 1048–1062. doi: 10.1046/j.1523-1739.2002.00423.x
Couturier, L. I. E., Dudgeon, C. L., Pollock, K. H., Jaine, F. R., a, Bennett, M. B., Townsend, K., et al. (2014). Population dynamics of the reef manta ray Manta alfredi in eastern Australia. Coral Reefs 33, 329–342. doi: 10.1007/s00338-014-1126-5
Couturier, L. I. E., Jaine, F. R. A., Townsend, K. A., Weeks, S. J., Richardson, A. J., and Bennett, M. B. (2011). Distribution, site affinity and regional movements of the manta ray, Manta alfredi (Krefft, 1868), along the east coast of Australia. Mar. Freshwater Res. 62, 628–637. doi: 10.1071/MF10148
Couturier, L. I. E., Marshall, A. D., Jaine, F. R. A., Kashiwagi, T., Pierce, S. J., Townsend, K. A., et al. (2012). Biology, ecology and conservation of the Mobulidae. J. Fish Biol. 80, 1075–1119. doi: 10.1111/j.1095-8649.2012.03264.x
Couturier, L. I. E., Newman, P., Jaine, F. R. A., Bennett, M. B., Venables, W. N., Cagua, E. F., et al. (2018). Variation in occupancy and habitat use of Mobula alfredi at a major aggregation site. Mar. Ecol. Prog. Series. 599:125–145. doi: 10.3354/meps12610
Couturier, L. I. E., Rohner, C. A., Richardson, A. J., Marshall, A. D., Jaine, F. R. A., Bennett, M. B., et al. (2013). Stable isotope and signature fatty acid analyses suggest reef manta rays feed on demersal zooplankton. PLoS ONE 8:e77152. doi: 10.1371/journal.pone.0077152
Croll, D. A., Dewar, H., Dulvy, N. K., Fernando, D., Francis, M. P., Galván-Magaña, F., et al. (2016). Vulnerabilities and fisheries impacts: the uncertain future of manta and devil rays. Aqua. Conserv. Mar. Freshw. Ecosyst. 26, 562–575. doi: 10.1002/aqc.2591
Croll, D. A., Newton, K. M., Weng, K., Galván-Magaña, F., O'Sullivan, J., and Dewar, H. (2012). Movement and habitat use by the spine-tail devil ray in the Eastern Pacific Ocean. Mar. Ecol. Prog. Series 465, 193–200. doi: 10.3354/meps09900
Crowder, L. B., Hopkins-Murphy, S. R., and Royle, J. A. (1995). Effects of turtle excluder devices (TEDs) on Loggerhead sea turtle strandings with implications for conservation. Nature 4, 773–779. doi: 10.2307/1447026
Cuevas-Zimbrón, E., Sosa-Nishizaki, O., Pérez-Jiménez, J. C., and O'Sullivan, J. B. (2013). An analysis of the feasibility of using caudal vertebrae for ageing the spinetail devilray, Mobula japanica (Müller and Henle, 1841). Environ. Biol. Fishes 96, 907–914. doi: 10.1007/s10641-012-0086-2
Davey, J. L., and Blaxter, M. W. (2010). RADseq: next-generation population genetics. Brief. Funct. Genomics 9, 416–423. doi: 10.1093/bfgp/elq031
Deakos, M. H. (2010). Paired-laser photogrammetry as a simple and accurate system for measuring the body size of free-ranging manta rays Manta alfredi. Aquat. Biol. 10, 1–10. doi: 10.3354/ab00258
Deakos, M. H. (2012). The reproductive ecology of resident manta rays (Manta alfredi) off Maui, Hawaii, with an emphasis on body size. Environ. Biol. Fishes 94, 443–456. doi: 10.1007/s10641-011-9953-5
Deakos, M. H., Baker, J. D., and Bejder, L. (2011). Characteristics of a manta ray Manta alfredi population off Maui, Hawaii, and implications for management. Mar. Ecol. Prog. Series 429, 245–260. doi: 10.3354/meps09085
Dewar, H., Mous, P., Domeier, M., Muljadi, A., Pet, J., and Whitty, J. (2008). Movements and site fidelity of the giant manta ray, Manta birostris, in the Komodo Marine Park, Indonesia. Mar. Biol. 155, 121–133. doi: 10.1007/s00227-008-0988-x
Dobson, A., and McGlade, J. (2006). Habitat loss, trophic collapse, and the decline of ecosystem services. Ecology 87, 1915–1924. doi: 10.1890/0012-9658(2006)87[1915:HLTCAT]2.0.CO;2
Dudgeon, C. L., and Ovenden, J. R. (2015). The relationship between abundance and genetic effective population size in elasmobranchs: an example from the globally threatened zebra shark Stegostoma fasciatum within its protected range. Conserv. Genet. 16, 1443–1454. doi: 10.1007/s10592-015-0752-y
Dudgeon, C. L., Pollock, K. H., Braccini, J. M., Semmens, J. M., and Barnett, A. (2015). Integrating acoustic telemetry into mark–recapture models to improve the precision of apparent survival and abundance estimates. Oecologia 178, 761–772. doi: 10.1007/s00442-015-3280-z
Duffy, C. A. J., and Scott, C. T. (2017). First observation of the courtship behaviour of the giant devil ray Mobula mobular (Myliobatiformes: Mobulidae). N. Z. J. Zool. 1–8. doi: 10.1080/03014223.2017.1410850
Dulvy, N. K., Ellis, J. R., Goodwin, N. B., Grant, A., Reynolds, J. D., and Jennings, S. (2004). Methods of assessing extinction risk in marine fishes. East 5, 255–276. doi: 10.1111/j.1467-2679.2004.00158.x
Dulvy, N. K., Pardo, S. A., Simpfendorfer, C. A., and Carlson, J. K. (2014). Diagnosing the dangerous demography of manta rays using life history theory. PeerJ 2:e400. doi: 10.7717/peerj.400
Essumang, D. K. (2009). Analysis and human health risk assessment of arsenic, cadmium, and mercury in Manta birostris (Manta ray) caught along the Ghanaian coastline. Hum. Ecol. Risk Assess. 15, 985–998. doi: 10.1080/10807030903153451
Essumang, D. K. (2010). First determination of the levels of platinum group metals in Manta birostris (Manta Ray) caught along the Ghanaian coastline. Bull. Environ. Contam. Toxicol. 84, 720–725. doi: 10.1007/s00128-010-0019-8
Fernando, D., and Stevens, G. (2011). A Study of Sri Lanka's Manta & Mobula Ray Fishery. Dorchester, UK: The Manta Trust.
Fontes, J., Baeyaert, J., Prieto, R., Graca, G., Buyle, F., and Afonso, P. (2018). New non-invasive methods for short-term electronic tagging of pelagic sharks and rays. Mar. Biol. 165:34. doi: 10.1007/s00227-018-3289-z
Fossi, M. C., Baini, M., Panti, C., Galli, M., Jiménez, B., Muñoz-Arnanz, J., et al. (2017). Are whale sharks exposed to persistent organic pollutants and plastic pollution in the Gulf of California (Mexico)? first ecotoxicological investigation using skin biopsies. Comp. Biochem. Physiol. C Toxicol. Pharmacol. 199, 48–58. doi: 10.1016/j.cbpc.2017.03.002
Fossi, M. C., Coppola, D., Baini, M., Giannetti, M., Guerranti, C., Marsili, L., et al. (2014). Large filter feeding marine organisms as indicators of microplastic in the pelagic environment: the case studies of the Mediterranean basking shark (Cetorhinus maximus) and fin whale (Balaenoptera physalus). Mar. Environ. Res. 100, 17–24. doi: 10.1016/j.marenvres.2014.02.002
Fossi, M. C., Marsili, L., Baini, M., Giannetti, M., Coppola, D., Guerranti, C., et al. (2016). Fin whales and microplastics: the Mediterranean Sea and the Sea of Cortez scenarios. Environ. Pollut. 209, 68–78. doi: 10.1016/j.envpol.2015.11.022
Francis, M. P., and Jones, E. G. (2016). Movement, depth distribution and survival of spinetail devilrays (Mobula japanica) tagged and released from purse-seine catches in New Zealand. Aquat. Conserv. Mar. Freshw. Ecosyst. 27, 219–236. doi: 10.1002/aqc.2641
Frankham, R. (2005). Ecosystem recovery enhanced by genotypic diversity. Heredity 95:183. doi: 10.1038/sj.hdy.6800706
Frisk, M. G., Miller, T. J., and Dulvy, N. K. (2005). Life histories and vulnerability to exploitation of elasmobranchs: inferences from elasticity, perturbation and phylogenetic analyses. J. Northwest Atl. Fish. Sci. 35, 27–45. doi: 10.2960/J.v35.m514
Galloway, T. S., and Lewis, C. N. (2016). Marine microplastics spell big problems for future generations. Proc. Nat. Acad. Sci. U.S.A. 113, 2331–2333. doi: 10.1073/pnas.1600715113
García, V. B., Lucifora, L. O., and Myers, R. A. (2008). The importance of habitat and life history to extinction risk in sharks, skates, rays and chimaeras. Proc. R Soc. B Biol. Sci. 275, 83–89. doi: 10.1098/rspb.2007.1295
Garrud, E. (2016). Does Tourist Behaviour Affect Reef Manta Ray Feeding Behaviour? An Analysis of Human and Manta alfredi Interactions in Baa Atoll, the Maldives. York, UK: University of York.
Gerber, L. R., and Heppell, S. S. (2004). The use of demographic sensitivity analysis in marine species conservation planning. Biol. Conserv. 120, 121–128. doi: 10.1016/j.biocon.2004.01.029
Germanov, E. S., and Marshall, A. D. (2014). Running the gauntlet: regional movement patterns of Manta alfredi through a complex of parks and fisheries. PLoS ONE 9:e110071. doi: 10.1371/journal.pone.0110071
Germanov, E. S., Marshall, A. D., Bejder, L., Fossi, M. C., and Loneragan, N. R. (2018). Microplastics: no small problem for filter-feeding megafauna. Trends Ecol Evol. 33, 227–232. doi: 10.1016/j.tree.2018.01.005
Girondot, M., Bédel, S., Delmoitiez, L., Russo, M., Chevalier, J., Guéry, L., et al. (2014). Spatio-temporal distribution of Manta birostris in French Guiana waters. J. Mar. Biol. Assoc. U.K. 95, 153–160. doi: 10.1017/S0025315414001398
Gleiss, A. C., Wright, S., Liebsch, N., Wilson, R. P., and Norman, B. (2013). Contrasting diel patterns in vertical movement and locomotor activity of whale sharks at Ningaloo Reef. Mar. Biol. 160, 2981–2992. doi: 10.1007/s00227-013-2288-3
Godin, A. C., Wimmer, T., Wang, J. H., and Worm, B. (2013). No effect from rare-earth metal deterrent on shark bycatch in a commercial pelagic longline trial. Fish. Res. 143, 131–135. doi: 10.1016/j.fishres.2013.01.020
Goldbogen, J. A., Hazen, E. L., Friedlaender, A. S., Calambokidis, J., DeRuiter, S. L., Stimpert, A. K., et al. (2015). Prey density and distribution drive the three-dimensional foraging strategies of the largest filter feeder. Funct. Ecol. 29, 951–961. doi: 10.1111/1365-2435.12395
Goldman, K. J. (2005). “Age and growth of elasmobranch fishes,” in Management Techniques for Elasmobranch Fisheries, FAO Fisheries Tech. Paper 474, eds J. A. Musick and R. Bonfil.(Rome: Food and Agriculture Organization), 97–132.
Graham, R. T., Witt, M. J., Castellanos, D. W., Remolina, F., Maxwell, S., Godley, B. J., et al. (2012). Satellite tracking of manta rays highlights challenges to their conservation. PLoS ONE 7:e36834. doi: 10.1371/journal.pone.0036834
Gredzens, C., Marsh, H., Fuentes, M. M. P. B., Limpus, C. J., Shimada, T., and Hamann, M. (2014). Satellite tracking of sympatric marine megafauna can inform the biological basis for species co-management. PLoS ONE 9:e98944. doi: 10.1371/journal.pone.0098944
Grubbs, R. D. (2010). “Ontogenetic shifts in movements and habitat use,” in Sharks and Their Relatives II: Biodiversity, Adaptive Physiology, and Conservation, eds J. C. Carrier, J. A. Musick, and M. R. Heithaus (Boca Raton, FL: CRC Press), 319–350.
Hall, M., and Roman, M. (2013). Bycatch and Non-Tuna Catch in the Tropical Tuna Purse Seine Fisheries Of The World. La Jolla, CA: FAO Technical Paper, 568, 249.
Halpern, B. S., Walbridge, S., Selkoe, K. A., Kappel, C. V., Micheli, F., D'Agrosa, C., et al. (2008). A global map of human impact on marine ecosystems. Science 319, 948–953. doi: 10.1126/science.1149345
Hammerschlag, N., Gutowsky, L. F. G., Gallagher, A. J., Matich, P., and Cooke, S. J. (2017). Diel habitat use patterns of a marine apex predator (tiger shark, Galeocerdo cuvier) at a high use area exposed to dive tourism. J. Exp. Mar. Biol. Ecol. 495, 24–34. doi: 10.1016/j.jembe.2017.05.010
Harley, C. D. G., Hughes, A. R., Hultgren, K. M., Miner, B. G., Sorte, C. J. B., Thornber, C. S., et al. (2006). The impacts of climate change in coastal marine systems. Ecol. Lett. 9, 228–241. doi: 10.1111/j.1461-0248.2005.00871.x
Hauser, L., Adcock, G. J., Smith, P. J., Bernal Ramirez, J. H., and Carvalho, G. R. (2002). Loss of microsatellite diversity and low effective population size in an overexploited population of New Zealand snapper (Pagrus auratus). Proc. Natl. Acad. Sci. U.S.A. 99, 11742–11747. doi: 10.1073/pnas.172242899
Hays, G. C., Ferreira, L. C., Sequeira, A. M. M., Meekan, M. G., Duarte, C. M., Bailey, H., et al. (2016). Key questions in marine megafauna movement ecology. Trends Ecol. Evol. 31, 463–475. doi: 10.1016/j.tree.2016.02.015
Hays, G. C., Richardson, A. J., and Robinson, C. (2005). Climate change and marine plankton. Trends Ecol. Evol. 20, 337–344. doi: 10.1016/j.tree.2005.03.004
Hazen, E. L., and Johnston, D. W. (2010). Meridional patterns in the deep scattering layers and top predator distribution in the central equatorial Pacific. Fish. Oceanogr. 19, 427–433. doi: 10.1111/j.1365-2419.2010.00561.x
Hazen, E. L., Scales, K. L., Maxwell, S. M., Briscoe, D. K., Welch, H., Bograd, S. J., et al. (2018). A dynamic ocean management tool to reduce bycatch and support sustainable fisheries. Sci. Adv. 4:eaar3001. doi: 10.1126/sciadv.aar3001
Heppell, S. S. (1998). Application of life-history theory and population model analysis to turtle conservation. Copeia 1998, 367–375. doi: 10.2307/1447430
Heppell, S. S., Hal, C., and Crowder, L. B. (2000). Life histories and elasticity patterns: perturbation analysis for species with minimal demographic data. Ecology 81, 654–665. doi: 10.1890/0012-9658(2000)081[0654:LHAEPP]2.0.CO;2
Heupel, M. R., Carlson, J. K., and Simpfendorfer, C. A. (2007). Shark nursery areas: concepts, definition, characterization and assumptions. Mar. Ecol. Prog. Series 337, 287–297. doi: 10.3354/meps337287
Heupel, M. R., and Simpfendorfer, C. A. (2002). Estimation of mortality of juvenile blacktip sharks, Carcharhinus limbatus, within a nursery area using telemetry data. Can. J. Fish. Aquat. Sci. 59, 624–632. doi: 10.1139/f02-036
Hillary, R. M., Bravington, M. V., Patterson, T. A., Grewe, P., Bradford, R., Feutry, P., et al. (2018). Genetic relatedness reveals total population size of white sharks in eastern Australia and New Zealand. Sci. Rep. 8:2661. doi: 10.1038/s41598-018-20593-w
Hinojosa-Alvarez, S., Walter, R. P., Diaz-Jaimes, P., Galván-Magaña, F., and Paig-Tran, E. M. (2016). A potential third Manta Ray species near the Yucatán Peninsula? evidence for a recently diverged and novel genetic Manta group from the Gulf of Mexico. PeerJ 4:e2586. doi: 10.7717/peerj.2586
Hodgson, A., Kelly, N., and Peel, D. (2013). Unmanned aerial vehicles (UAVs) for surveying Marine Fauna: a dugong case study. PLoS ONE 8:e79556. doi: 10.1371/journal.pone.0079556
Hoenig, J. M. (1983). Empirical use of longevity data to estimate mortality rates. Fish. Bull. 81, 898–903.
Homma, K., Maruyama, T., Itoh, T., Ishihara, H., and Uchida, S. (1999). “Biology of the manta ray, Manta birostris Walbaum, in the Indo-Pacific,” in Proceedings of the 5th Indo-Pacific Fish Conference, Noumea, 1997, eds B. Seret and J.-Y. Sire (Paris: SocieteFrancaise d'Ichthyologie), 209–216.
Hopkins, T. L. (1982). The vertical distribution of zooplankton in the eastern Gulf of Mexico. Deep Sea Res. Oceanogr. Res. Pap. 29, 1069–1083. doi: 10.1016/0198-0149(82)90028-0
Hordyk, A., Ono, K., Valencia, S., Loneragan, N., and Prince, J. (2015). A novel length-based empirical estimation method of spawning potential ratio (SPR), and tests of its performance, for small-scale, data-poor fisheries. ICES J. Mar. Sci. 72, 217–231. doi: 10.1093/icesjms/fsu004
Houssard, P., and Grahamd. B. S Menkese, C. E. (2017). Trophic position increases with thermocline depth in yellowfin and bigeye tuna across the Western and Central Pacific Ocean. Prog. Oceanogr. 154, 49–63. doi: 10.1016/j.pocean.2017.04.008
Hussey, N. E., Brush, J., McCarthy, I. D., and Fisk, A. T. (2010). δ15N and δ13C diet-tissue discrimination factors for large sharks under semi-controlled conditions. Comp. Biochem. Physiol. Mole. Integrat. Physiol. 155, 445–453. doi: 10.1016/j.cbpa.2009.09.023.
Hussey, N. E., Kessel, S. T., Aarestrup, K., Cooke, S. J., Cowley, P. D., Fisk, A. T., et al. (2015). Aquatic animal telemetry: a panoramic window into the underwater world. Science 348:1255642. doi: 10.1126/science.1255642
Hutchinson, M., Poisson, F., and Swimmer, Y. (2017). Developing Best Handling Practice Guidelines to Safely Release Mantas, Mobulids and Stingrays Captured in Commercial Fisheries. US Department of Commerce, National Oceanic and Atmospheric Administration, National Marine Fisheries Service, Pacific Islands Fisheries Science Center.
Hutchinson, M. R., Itano, D. G., Muir, J. A., and Holland, K. N. (2015). Post-release survival of juvenile silky sharks captured in a tropical tuna purse seine fishery. Mar. Ecol. Prog. Series 521, 143–154. doi: 10.3354/meps11073
Huveneers, C., Stead, J., Bennett, M. B., Lee, K. A., and Harcourt, R. G. (2013). Age and growth determination of three sympatric wobbegong sharks: How reliable is growth band periodicity in Orectolobidae? Fish. Res. 147, 413–425. doi: 10.1016/j.fishres.2013.03.014
Jaine, F. R. A, Couturier, L. I. E., Weeks, S. J., Townsend, K. A., Bennett, M. B., Fiora, K., et al. (2012). When giants turn up: sighting trends, environmental influences and habitat use of the manta ray Manta alfredi at a Coral Reef. PLoS ONE 7:e46170. doi: 10.1371/journal.pone.0046170
Jaine, F. R. A., Rohner, C. A., Weeks, S. J., Couturier, L. I. E., Bennett, M. B., Townsend, K. A., et al. (2014). Movements and habitat use of reef manta rays off eastern Australia: offshore excursions, deep diving and eddy affinity revealed by satellite telemetry. Mar. Ecol. Prog. Series 510, 73–86. doi: 10.3354/meps10910
Jakimska, A., Konieczka, P., Skóra, K., and Namieśnik, J. (2011). Bioaccumulation of metals in tissues of marine animals, part I: the role and impact of heavy metals on organism. Pol. J. Enviro. Studies 20, 1127–1146.
Jensen, A. L. (1996). Beverton and holt life history invariants result from optimal trade-off of reproduction and survival. Can. J. Fish. Aquat. Sci. 53, 820–822. doi: 10.1139/f95-233
Jonsen, I. D., Basson, M., Bestley, S., Bravington, M. V., Patterson, T. A., Pedersen, M. W., et al. (2013). State-space models for bio-loggers: A methodological road map. Deep-sea Res. II. Top. Stud. Oceanogr. 88–89, 34–46. doi: 10.1016/j.dsr2.2012.07.008
Jordan, L. K., Mandelman, J. W., and Kajiura, S. M. (2011). Behavioral responses to weak electric fields and a lanthanide metal in two shark species. J. Exp. Mar. Biol. Ecol. 409, 345–350. doi: 10.1016/j.jembe.2011.09.016
Jordan, L. K., Mandelman, J. W., McComb, D. M., Fordham, S. V., Carlson, J. K., and Werner, T. B. (2013). Linking sensory biology and fisheries bycatch reduction in elasmobranch fishes: a review with new directions for research. Conserv. Physiol. 1, 1–20. doi: 10.1093/conphys/cot002
Kaltenberg, A. M., Biggs, D. C., and DiMarco, S. F. (2007). Deep scattering layers of the Northern Gulf of Mexico observed with a shipboard 38-kHz acoustic doppler current profiler. Gulf Mex. Sci. 2, 97–108. doi: 10.18785/goms.2502.01
Kashiwagi, T. (2014). Conservation Biology And Genetics of the Largest Living Rays: Manta Rays. Brisbane, QLD: University of Queensland.
Kashiwagi, T., Marshall, A. D., Bennett, M. B., and Ovenden, J. R. (2011). Habitat segregation and mosaic sympatry of the two species of manta ray in the Indian and Pacific Oceans: Manta alfredi and M. birostris. Mar. Biodivers. Rec. 4, 1–8. doi: 10.1017/S1755267211000479
Kashiwagi, T., Marshall, A. D., Bennett, M. B., and Ovenden, J. R. (2012). The genetic signature of recent speciation in manta rays (Manta alfredi and M. birostris). Mol. Phylogenet. Evol. 64, 212–218. doi: 10.1016/j.ympev.2012.03.020
Kenchington, T. J. (2014). Natural mortality estimators for information-limited fisheries. Page Fish. Fish. 15, 533–562. doi: 10.1111/faf.12027
Kessel, S. T., Elamin, N. A., Yurkowski, D. J., Chekchak, T., Walter, R. P., Klaus, R., et al. (2017). Conservation of reef manta rays (Manta alfredi) in a UNESCO world heritage site: large-scale island development or sustainable tourism? PLoS ONE 12:e0185419. doi: 10.1371/journal.pone.0185419
Kim, S. L., Casper, D. R., Galván-Magaña, F., Ochoa-Díaz, R., Hernández-Aguilar, S. B., and Koch, P. L. (2012a). Carbon and nitrogen discrimination factors for elasmobranch soft tissues based on a long-term controlled feeding study. Environ. Biol. Fishes 95, 37–52. doi: 10.1007/s10641-011-9919-7
Kim, S. L., del Rio, C. M., Casper, D., and Koch, P. L. (2012b). Isotopic incorporation rates for shark tissues from a long-term captive feeding study. J. Exp. Biol. 215, 2495–2500. doi: 10.1242/jeb.070656
Kitchen-Wheeler, A. M., Ari, C., and Edwards, A. J. (2012). Population estimates of Alfred mantas (Manta alfredi) in central Maldives atolls: North Male, Ari and Baa. Environ. Biol. Fishes 93, 557–575. doi: 10.1007/s10641-011-9950-8
Kumli, K. R., and Rubin, R. D. (2010). Photo-Identification of the Manta Ray, Manta birostris, in the Revillagigedos Islands. Quebec: Page Joint Meeting of Ichthyologists and Herpetologists.
Kuparinen, A., Hutchings, J. A., and Waples, R. S. (2016). Harvest-induced evolution and effective population size. Evol. Appl. 9, 658–672. doi: 10.1111/eva.12373
Lambert, C., Mannocci, L., Lehodey, P., and Ridoux, V. (2014). Predicting cetacean habitats from their energetic needs and the distribution of their prey in two contrasted tropical regions. PLoS ONE 9:e105958. doi: 10.1371/journal.pone.0105958
Lander, M. E., Lindstrom, T., Rutishauser, M., Franzheim, A., and Holland, M. (2015). Development and field testing a satellite-linked fluorometer for marine vertebrates. Anim. Biotelem. 3:40. doi: 10.1186/s40317-015-0070-7
Lawson, G. L., Hückstädt, L. A., Lavery, A. C., Wiebe, P. H., Fincke, J. R., et al. (2015). Development of an animal-borne “sonar tag” for quantifying prey availability: Test deployments on northern elephant seals. Anim. Biotelem 3:22. doi: 10.1186/s40317-015-0054-7
Lawson, J. M., Fordham, S. V., O'Malley, M. P., Davidson, L. N., Walls, R. H., Heupel, M. R., et al. (2017). Sympathy for the devil: a conservation strategy for devil and manta rays. PeerJ 5:e3027. doi: 10.7717/peerj.3027
Lea, J. S. E., Humphries, N. E., von Brandis, R. G., Clarke, C. R., and Sims, D. W. (2016). Acoustic telemetry and network analysis reveal the space use of multiple reef predators and enhance marine protected area design. Proc. R. Soc. B. Biol. Sci. 283:20160717. doi: 10.1098/rspb.2016.0717
Lewis, S. A., Setiasih, N., Fahmi, F., Dharmadi, D., O'Malley, M. P., Campbell, S. J., et al. (2015). Assessing Indonesian manta and devil ray populations through historical landings and fishing community interviews. PeerJ PrePrints. 6:e1334v1. doi: 10.7287/peerj.preprints.1334v1
Lopez, J. N. S. (2009). Estudio Comparativo de la Reproduccion de tres Especies del Genero Mobula (Chondrichthyes: Mobulidae) en el Suroeaste del Golfo de California, Mexico. La Paz: Instituto Politecnico Nacional.
Lusseau, D. (2004). The hidden cost of tourism: detecting long-term effects of tourism using behavioral information. Ecol. Soc. 9:2. doi: 10.5751/ES-00614-090102
Marshall, A. D., and Bennett, M. B. (2010). Reproductive ecology of the reef manta ray Manta alfredi in southern Mozambique. J. Fish Biol. 77, 169–190. doi: 10.1111/j.1095-8649.2010.02669.x
Marshall, A. D., Compagno, L. J. V., and Bennett, M. B. (2009). Redescription of the genus Manta with resurrection of Manta alfredi. Zootaxa 28, 1–28.
Marshall, A. D., Dudgeon, C. L., and Bennett, M. B. (2011). Size and structure of a photographically identified population of manta rays Manta alfredi in southern Mozambique. Mar. Biol. 158, 1111–1124. doi: 10.1007/s00227-011-1634-6
Marshall, A. D., and Pierce, S. J. (2012). The use and abuse of photographic identification in sharks and rays. J. Fish Biol. 80, 1361–1379. doi: 10.1111/j.1095-8649.2012.03244.x
Martins, A. P. B., Heupel, M. R., Chin, A., and Simpfendorfer, C. A. (2018). Batoid nurseries : definition, use and importance. Mar. Ecol. Prog. Series 595, 253–267. doi: 10.3354/meps12545
McCauley, D. J., Desalles, P. A., Young, H. S., Dunbar, R. B., Dirzo, R., Mills, M. M., et al. (2012). From wing to wing: the persistence of long ecological interaction chains in less-disturbed ecosystems. Sci. Rep. 2:409. doi: 10.1038/srep00409
McCauley, D. J., DeSalles, P. A., Young, H. S., Papastamatiou, Y. P., Caselle, J. E., Deakos, M. H., et al. (2014). Reliance of mobile species on sensitive habitats: a case study of manta rays (Manta alfredi) and lagoons. Mar. Biol. 161, 1987–1998. doi: 10.1007/s00227-014-2478-7
McEachran, J. D., and Carvalho, M. R. (2002). “Mobulidae,” in The Living Marine Resources of the Western Central Atlantic, ed K. E. Carpenter (Rome: FAO), 586–589.
Mcmahon, K. W., Thorrold, S. R., Elsdon, T. S., and Mccarthy, M. D. (2015). Trophic discrimination of nitrogen stable isotopes in amino acids varies with diet quality in a marine fish. Limnol. Oceanogr. 60, 1076–1087. doi: 10.1002/lno.10081
McMahon, K. W., Thorrold, S. R., Houghton, L. A., and Berumen, M. L. (2016). Tracing carbon flow through coral reef food webs using a compound-specific stable isotope approach. Oecologia 180, 809–821. doi: 10.1007/s00442-015-3475-3
Melvin, E. F., Guy, T. J., and Read, L. B. (2014). Best practice seabird bycatch mitigation for pelagic longline fisheries targeting tuna and related species. Fish. Res. 149, 5–18. doi: 10.1016/j.fishres.2013.07.012
Mengersen, K., Peterson, E. E., Clifford, S., Ye, N., Kim, J., Bednarz, T., et al. (2017). Modelling imperfect presence data obtained by citizen science. Environmetrics 28, 1–29. doi: 10.1002/env.2446
Methot, R., and Wetzel, C. (2013). Stock synthesis: a biological and statistical framework for fish stock assessment and fishery management. Fish. Res. 142, 86–99. doi: 10.1016/j.fishres.2012.10.012
Meyer-Gutbrod, E. L., and Greene, C. H. (2018). Uncertain recovery of the North Atlantic right whale in a changing ocean. Global Change Biol. 24, 455–464. doi: 10.1111/gcb.13929
Moloney, C. L., St John, M. A., Denman, K. L., Karl, D. M., Köster, F. W., Sundby, S., et al. (2011). Weaving marine food webs from end to end under global change. J. Mar. Syst. 84, 106–116. doi: 10.1016/j.jmarsys.2010.06.012
Moriarty, R., and O'Brien, T. D. (2013). Distribution of mesozooplankton biomass in the global ocean. Earth Syst. Sci. Data 5, 45–55. doi: 10.5194/essd-5-45-2013
Musick, J. A. (1999). Criteria to define extinction risk in marine fishes: the american fisheries society initiative. Fisheries 24, 6–14.
Musyl, M. K., Domeier, M. L., Nasby-Lucas, N., Brill, R. W., McNaughton, L. M., Swimmer, J. Y., et al. (2011). Performance of pop-up satellite archival tags. Mar. Ecol. Prog. Series 433, 1–28. doi: 10.3354/meps09202
Neville, D. R., Phillips, A. J., Brodeur, R. D., and Higley, K. A. (2014). Trace levels of fukushima disaster radionuclides in east pacific albacore. Environ. Sci. Technol. 48, 4739–4743. doi: 10.1021/es500129b
Niimi, A. J. (1996). Evaluation of PCBs and PCDDFs retention by aquatic organisms. Sci. Total Environ. 2, 123–150. doi: 10.1016/S0048-9697(96)05306-5
Notarbartolo di Sciara, G. (1987). A revisionary study of the genus Mobula Rafinesque, 1810 (Chondrichthyes: Mobulidae) with the description of a new species. Zool. J. Linn. Soc. 91, 1–91. doi: 10.1111/j.1096-3642.1987.tb01723.x
Notarbartolo di Sciara, G. (1988). Natural history of the rays of the genus Mobula in the Gulf of California. Fish. Bul. 86, 45–66.
Notarbartolo di Sciara, G., Fernando, D., Adnet, S., Cappetta, H., and Jabado, R. W. (2017). Devil rays (Chondrichthyes: Mobula) of the Arabian Seas, with a redescription of Mobula kuhlii (Valenciennes in Müller and Henle, 1841). Aquat. Conserv. Mar. Freshw. Ecosyst. 27, 197–218. doi: 10.1002/aqc.2635
Notarbartolo di Sciara, G., Lauriano, G., Pierantonio, N., Cañadas, A., Donovan, G., and Panigada, S. (2015). The devil we don't know: investigating habitat and abundance of endangered giant devil rays in the North-Western Mediterranean Sea. PLoS ONE 10:e0141189. doi: 10.1371/journal.pone.0141189
Notarbartolo di Sciara, G., and Serena, F. (1988). Term embryo of Mobula mobular (Bonnaterre, 1788) from the northern Tyrrhenian Sea (Chondrichthyes: Mobulidae). Atti della Societa Italiana di Scienze Naturali e del Museo Civico di Storia Naturale in Milano 129, 396–400.
Novelo, N. D., and Tiersch, T. R. (2012). A review of the use of ultrasonography in fish reproduction. North Am. J. Aquaculture 74, 169–181. doi: 10.1080/15222055.2012.672370
Nozu, R., Murakumo, K., Matsumoto, R., Matsumoto, Y., Yano, N., Nakamura, M., et al. (2017). High-resolution monitoring from birth to sexual maturity of a male reef manta ray, Mobula alfredi, held in captivity for 7 years: changes in external morphology, behavior, and steroid hormones levels. BMC Zool. 2:14. doi: 10.1186/s40850-017-0023-0
Ogburn, M. B., Harrison, A. L., Whoriskey, F. G., Cooke, S. J., Mills Flemming, J. E., and Torres, L. G. (2017). Addressing challenges in the application of animal movement ecology to aquatic conservation and management. Front. Mar. Sci. 4:70. doi: 10.3389/fmars.2017.00070
O'Malley, M. P., Lee-Brooks, K., and Medd, H. B. (2013). The global economic impact of manta ray watching tourism. PLoS ONE 8:e65051. doi: 10.1371/journal.pone.0065051
O'Malley, M. P., Townsend, K. A., Hilton, P., Heinrichs, S., and Stewart, J. D. (2016). Characterization of the trade in manta and devil ray gill plates in China and Southeast Asia through trader surveys. Aquat. Conserv. Mar. Freshw. Ecosyst. 1–41. doi: 10.1002/aqc.2670
Ooi, M. S. M., Townsend, K. A., Bennett, M. B., Richardson, A. J., Fernando, D., Villa, C. A., et al. (2015). Levels of arsenic, cadmium, lead and mercury in the branchial plate and muscle tissue of mobulid rays. Mar. Pollut. Bull. 94, 251–259. doi: 10.1016/j.marpolbul.2015.02.005
Osada, K. (2010). Relationship Of Zooplankton Emergence, Manta Ray Abundance And Scuba Diver Usage Kona Hawai'i. Hilo: University of Hawai'i.
Panigada, S., Donovan, G. P., Druon, J. N., Lauriano, G., Pierantonio, N., Pirotta, E., et al. (2017). Satellite tagging of Mediterranean fin whales: Working towards the identification of critical habitats and the focussing of mitigation measures. Sci. Rep. 7:3365. doi: 10.1038/s41598-017-03560-9
Papastamatiou, Y. P., DeSalles, P. A., and McCauley, D. J. (2012). Area-restricted searching by manta rays and their response to spatial scale in lagoon habitats. Mar. Ecol. Prog. Series 456, 233–244. doi: 10.3354/meps09721
Pardo, S. A., Kindsvater, H. K., Cuevas-Zimbrón, E., Sosa-Nishizaki, O., Pérez-Jiménez, J. C., and Dulvy, N. K. (2016a). Growth, productivity, and extinction risk of a data-sparse devil ray. Sci. Rep. 6:33745. doi: 10.1038/srep33745
Pardo, S. A., Kindsvater, H. K., Reynolds, J. D., and Dulvy, N. K. (2016b). Maximum intrinsic rate of population increase in sharks, rays, and chimaeras: the importance of survival to maturity. Can. J. Fish. Aquat. Sci. 73, 1159–1163. doi: 10.1139/cjfas-2016-0069
Patella, R., and Bullard, S. A. (2013). Hexabothriids of devil rays (Mobulidae): new genus and species from gill of Mobula hypostoma in the Northern Gulf of Mexico and redescription of a congener from Mobula rochebrunei in the Eastern Atlantic Ocean. J. Parasitol. 99, 856–867. doi: 10.1645/12-153.1
Patterson, T. A., Evans, K., Carter, T. I., and Gunn, J. S. (2008). Movement and behaviour of large southern bluefin tuna (Thunnus maccoyii) in the Australian region determined using pop-up satellite archival tags. Fish. Oceanogr. 17, 352–367. doi: 10.1111/j.1365-2419.2008.00483.x
Pauly, D. (1980). On the interrelationships between natural mortality, growth parameters, and mean environmental temperature in 175 fish stocks. ICES J. Mar. Sci. 39, 175–192. doi: 10.1093/icesjms/39.2.175
Perry, C. T., Figueiredo, J., Vaudo, J. J., and Shivji, M. S. (2018). Comparing length-measurement methods and estimating growth parameters of free-swimming whale sharks (Rhincodon typus) near the South Ari Atoll, Maldives. Mar. Freshw. Res. doi: 10.1071/MF17393. [Epub ahead of print].
Peterson, B. K., Weber, J. N., Kay, E. H., Fisher, H. S., and Hoekstra, H. E. (2012). Double digest RADseq: an inexpensive method for de novo SNP discovery and genotyping in model and non-model species. PloS ONE 7:e37135. doi: 10.1371/journal.pone.0037135
Pikesley, S. K., Agambouec, P. D., Bayetd, J. P., Bibange, J. N., Bongunof, E. A., Boussambag, F., et al. (2018). A novel approach to estimate the distribution, density and at-sea risks of a centrally-placed mobile marine vertebrate. Biol. Conserv. 221, 246–256. doi: 10.1016/j.biocon.2018.03.011
Pinsky, M. L., and Palumbi, S. R. (2014). Meta-analysis reveals lower genetic diversity in overfished populations. Mol. Ecol. 23, 29–39. doi: 10.1111/mec.12509
Poisson, F., Séret, B., Vernet, A. L., Goujon, M., and Dagorn, L. (2014). Collaborative research: development of a manual on elasmobranch handling and release best practices in tropical tuna purse-seine fisheries. Mar. Pol. 44, 312–320. doi: 10.1016/j.marpol.2013.09.025
Poortvliet, M., Olsen, J. L., Croll, D. A., Bernardi, G., Newton, K., Kollias, S., et al. (2015). A dated molecular phylogeny of manta and devil rays (Mobulidae) based on mitogenome and nuclear sequences. Mol. Phyl. Evol. 83, 72–85. doi: 10.1016/j.ympev.2014.10.012
Portnoy, D. S., McDowell, J. R., McCandless, C. T., Musick, J. A., and Graves, J. E. (2009). Effective size closely approximates the census size in the heavily exploited western Atlantic population of the sandbar shark, Carcharhinus plumbeus. Conser. Genet. 10, 1697–1705. doi: 10.1007/s10592-008-9771-2
Prohaska, B. K., Tsang, P. C. W., Driggers, I. I. I. W. B, Hoffmayer, E. R., Wheeler, C. R., Brown, A. C., et al. (2013). Assessing reproductive status in elasmobranch fishes using steroid hormones extracted from skeletal muscle tissue Conserv. Physiol. 1:cot028. doi: 10.1093/conphys/cot028
Queiroz, N., Humphries, N. E., Mucientes, G., Hammerschlag, N., Lima, F. P., Scales, K. L., et al. (2016). Ocean-wide tracking of pelagic sharks reveals extent of overlap with longline fishing hotspots. Proc. Nat. Acad. Sci. U.S.A. 113, 1582–1587. doi: 10.1073/pnas.1510090113
Rambahiniarison, J. M., Lamoste, M. J., Rohner, C. A., Murray, R., Snow, S., Labaja, J., et al. (2018). Life history, growth, and reproductive biology of four mobulid species in the bohol sea, philippines. Front. Mar. Sci. 5:269. doi: 10.3389/fmars.2018.00269
Reiss, H., Hoarau, G., Dickey-Collas, M., and Wolff, W. J. (2009). Genetic population structure of marine fish: mismatch between biological and fisheries management units. Fish. Fish. 10, 361–395. doi: 10.1111/j.1467-2979.2008.00324.x
Richardson, A. (2008). In hot water: zooplankton and climate change. ICES J. Mar. Sci. 65, 279–295. doi: 10.1093/icesjms/fsn028
Rochman, C. M. (2013). Plastics and priority pollutants: a multiple stressor in aquatic habitats. Environ. Sci. Technol. 47, 2439–2440. doi: 10.1021/es400748b
Rochman, C. M., Kurobe, T., Flores, I., and Teh, S. J. (2014). Early warning signs of endocrine disruption in adult fish from the ingestion of polyethylene with and without sorbed chemical pollutants from the marine environment. Sci. Total Environ. 493, 656–661. doi: 10.1016/j.scitotenv.2014.06.051
Rohner, C. A., Burgess, K. B., Rambahiniarison, J. M., Stewart, J. D., Ponzo, A., and Richardson, A. J. (2017). Mobulid rays feed on euphausiids in the Bohol Sea. R. Soc. Open Sci. 4:161060. doi: 10.1098/rsos.161060
Rohner, C. A., Pierce, S. J., Marshall, A. D., Weeks, S. J., Bennett, M. B., and Richardson, A. J. (2013). Trends in sightings and environmental influences on a coastal aggregation of manta rays and whale sharks. Mar. Ecol. Prog. Series 482, 153–168. doi: 10.3354/meps10290
Rohner, C. A., Weeks, S. J., Richardson, A. J., Pierce, S. J., Magno-Canto, M. M., Feldman, G. C., et al. (2014). Oceanographic influences on a global whale shark hotspot in southern Mozambique. PeerJ PrePrints. 2:e661v1. doi: 10.7287/peerj.preprints.661v1
Sampson, L., Galván–Magaña, F., De Silva-Dávila, R., Aguiniga-Garcia, S., and O'Sullivan, J. B. (2010). Diet and trophic position of the devil rays Mobula thurstoni and Mobula japanica as inferred from stable isotope analysis. J. Mar. Biol. Assoc. U.K. 90, 969–976. doi: 10.1017/S0025315410000548
Scales, K. L., Hazen, E. L., Maxwell, S. M., Dewar, H., Kohin, S., Jacox, M. G., et al. (2017). Fit to predict? Eco-informatics for predicting the catchability of a pelagic fish in near real time. Ecol. Appl. 27, 2313–2329. doi: 10.1002/eap.1610
Schofield, G., Katselidis, K. A., Lilley, M. K. S., Reina, R. D., and Hays, G. C. (2017). Detecting elusive aspects of wildlife ecology using drones: new insights on the mating dynamics and operational sex ratios of sea turtles. Funct. Ecol. 31, 2310–2319. doi: 10.1111/1365-2435.12930
Schuyler, Q. A., Wilcox, C., Townsend, K. A., Wedemeyer-Strombel, K. R., Balazs, G., van Sebille, E., et al. (2016). Risk analysis reveals global hotspots for marine debris ingestion by sea turtles. Global Change Biol. 22, 567–576. doi: 10.1111/gcb.13078
Sequeira, A. M. M., Rodríguez, J. P., Eguíluz, V. M., Harcourt, R., Hindell, M., Sims, D. W., et al. (2018). Convergence of marine megafauna movement patterns in coastal and open oceans. Proc. Nat. Acad. Sci. U.S.A. 115, 3072–3077. doi: 10.1073/pnas.1716137115
Setälä, O., Fleming-Lehtinen, V., and Lehtiniemi, M. (2014). Ingestion and transfer of microplastics in the planktonic food web. Environ. Pollut. 185, 77–83. doi: 10.1016/j.envpol.2013.10.013
Shortis, M., Harvey, E., and Abdo, D. (2009). A review of underwater stereo-image measurement for marine biology and ecology applications. Oceanogr. Mar. Biol. 47, 257–292. doi: 10.1201/9781420094220.ch6
Simpfendorfer, C. A. (1999). Mortality estimates and demographic analysis for the Australian sharpnose shark, Rhizoprionodon taylori, from northern Australia. Fish. Bull. 97, 978–986.
Sims, D. W., Witt, M. J., Richardson, A. J., Southall, E. J., and Metcalfe, J. D. (2006). Encounter success of free-ranging marine predator movements across a dynamic prey landscape. Proc. R. Soc. B. Biol. Sci. 273, 1195–1201. doi: 10.1098/rspb.2005.3444
Skomal, G. B. (2007). Evaluating the physiological and physical consequences of capture on post-release survivorship in large pelagic fishes. Fish. Manag. Ecol. 14, 81–89. doi: 10.1111/j.1365-2400.2007.00528.x
Smart, J. J., Chin, A., Tobin, A. J., and Simpfendorfer, C. A. (2016). Multimodel approaches in shark and ray growth studies: strengths, weaknesses and the future. Fish. Fish. 17, 955–971. doi: 10.1111/faf.12154
Smart, J. J., Chin, A., Tobin, A. J., White, W. T., Kumasi, B., and Simpfendorfer, C. A. (2017). Stochastic demographic analyses of the silvertip shark (Carcharhinus albimarginatus) and the common blacktip shark (Carcharhinus limbatus) from the Indo-Pacific. Fish. Res. 191, 95–107. doi: 10.1016/j.fishres.2017.03.002
Stevens, G. M. W. (2016). Conservation and Population Ecology of Manta Rays in the Maldives. York, UK: University of York.
Stevens, G. M. W., Fernando, D., Dando, M., and Notobarlo di Sciara, G. (2018a). Guide to the Manta and Devil Rays of the World. Wild Nature Press.
Stevens, G. M. W., Hawkins, J. P., and Roberts, C. M. (2018b). Courtship and mating behaviour of manta rays Mobula alfredi and M. birostris in the Maldives. J. Fish Biol. doi: 10.1111/jfb.13768. [Epub ahead of print].
Stewart, J. D., Barroso, A., Butler, R. H., and Munns, R. J. (2018a). Caught at the surface: myctophids make easy prey for dolphins and devil rays. Ecology. 99, 1894–1896. doi: 10.1002/ecy.2348
Stewart, J. D., Beale, C. S., Fernando, D., Sianipar, A. B., Burton, R. S., Semmens, B. X., et al. (2016a). Spatial ecology and conservation of manta birostris in the indo-pacific. Biol. Conserv. 200, 178–183. doi: 10.1016/j.biocon.2016.05.016
Stewart, J. D., Hoyos-Padilla, E. M., Kumli, K. R., and Rubin, R. D. (2016b). Deep-water feeding and behavioral plasticity in Manta birostris revealed by archival tags and submersible observations. Zoology 119, 406–413. doi: 10.1016/j.zool.2016.05.010
Stewart, J. D., Nuttall, M., Hickerson, E. L., and Johnston, M. A. (2018b). Important juvenile manta ray habitat at flower garden banks national marine sanctuary in the northwestern Gulf of Mexico. Mar. Biol. 165:111. doi: 10.1007/s00227-018-3364-5
Stewart, J. D., Rohner, C. A., Araujo, G., Avila, J., Fernando, D., Forsberg, K., et al. (2017a). Trophic overlap in mobulid rays: insights from stable isotope analysis. Mar. Ecol. Prog. Series 580, 131–151. doi: 10.3354/meps12304
Stewart, J. D., Stevens, G. M. W., Marshall, G. J., and Abernathy, K. (2017b). Are mantas self aware or simply social? a response to Ari and D'Agostino 2016. J. Ethol. 35, 145–147. doi: 10.1007/s10164-016-0491-7
Stock, C. A., Dunne, J. P., and John, J. G. (2014). Drivers of trophic amplification of ocean productivity trends in a changing climate. Biogeosciences 11, 7125–7135. doi: 10.5194/bg-11-7125-2014
Sulikowski, J. A., Driggers, W. B., Ingram, G. W., Kneebone, J., Ferguson, D. E., and Tsang, P. C. W. (2007). Profiling plasma steroid hormones: a non-lethal approach for the study of skate reproductive biology and its potential use in conservation management. Environ. Biol. Fishes 80, 285–292. doi: 10.1007/s10641-007-9257-y
Sussarellu, R., Suquet, M., Thomas, Y., Lambert, C., Fabioux, C., Pernet, M. E., et al. (2016). Oyster reproduction is affected by exposure to polystyrene microplastics. Proc. Nat. Acad. Sci. U.S.A. 113, 2430–2435. doi: 10.1073/pnas.1519019113
Thorrold, S. R., Afonso, P., Fontes, J., Braun, C. D., Santos, R. S., Skomal, G. B., et al. (2014). Extreme diving behaviour in devil rays links surface waters and the deep ocean. Nat. Commun. 5:4274. doi: 10.1038/ncomms5274
Uchida, S., Toda, M., and Matsumoto, Y. (2008). “Captive records of manta rays in Okinawa Churaumi Aquarium,” in Joint Meeting of Ichthyologists and Herpetologists (Montreal, QC), 23–28.
Venables, S., McGregor, F., Brain, L., and van Keulen, M. (2016a). Manta ray tourism management, precautionary strategies for a growing industry: a case study from the Ningaloo Marine Park, Western Australia. Pacific Conserv. Biol. 22, 295–300. doi: 10.1071/PC16003
Venables, S., Winstanley, G., Bowles, L., and Marshall, A. D. (2016b). A giant opportunity: the economic impact of manta rays on the Mozambican tourism industry — an incentive for increased management and protection. Tour. Mar. Environ. 12, 51–68. doi: 10.3727/154427316X693225
Villavicencio-Garayzar, C. J. (1991). Observations on Mobula munkiana (Chondrichthyes: Mobulidae) in the Bajia de la Paz, B.C.S., Mexico. Rev. Invest. Cient. 2, 78–81.
von der Heyden, S. (2017). Making evolutionary history count: biodiversity planning for coral reef fishes and the conservation of evolutionary processes. Coral Reefs 36, 183–194. doi: 10.1007/s00338-016-1512-2
Walter, R. P., Kessel, S. T., Alhasan, N., Fisk, A. T., Heath, D. D., Chak, T. C., et al. (2014). First record of living Manta alfredi × Manta birostris hybrid. Mar. Biodiver. 44, 1–2. doi: 10.1007/s12526-013-0183-2
Wang, J. (2005). Estimation of effective population sizes from data on genetic markers. Philos. Trans. R Soc. B Biol. Sci. 360, 1395–1409. doi: 10.1098/rstb.2005.1682
Ward-Paige, C. A., Davis, B., and Worm, B. (2013). Global population trends and human use patterns of Manta and Mobula rays. PLoS ONE 8:e74835. doi: 10.1371/journal.pone.0074835
Watanabe, Y. Y., and Takahashi, A. (2013). Linking animal-borne video to accelerometers reveals prey capture variability. Proc. Nat. Acad. Sci. U.S.A. 110, 2199–2204. doi: 10.1073/pnas.1216244110
Weeks, S. J., Magno-Canto, M. M., Jaine, F. R. A., Brodie, J., and Richardson, A. J. (2015). Unique sequence of events triggers manta ray feeding frenzy in the Southern Great Barrier Reef, Australia. Remote Sens. 7:3138–3152. doi: 10.3390/rs70303138
White, E. R., Myers, M. C., Flemming, J. M., and Baum, J. K. (2015). Shifting elasmobranch community assemblage at Cocos Island-an isolated marine protected area. Conserv. Biol. 29, 1186–1197. doi: 10.1111/cobi.12478
White, W. T., Corrigan, S., Yang, L., Henderson, A. C., Bazinet, A. L., Swofford, D. L., et al. (2017). Phylogeny of the manta and devilrays (Chondrichthyes: mobulidae), with an updated taxonomic arrangement for the family. Zool. J. Linn. Soc. 82, 65–73. doi: 10.1093/zoolinnean/zlx018
White, W. T., Giles, J., and Dharmadi, P. I. (2006). Data on the bycatch fishery and reproductive biology of mobulid rays (Myliobatiformes) in Indonesia. Fish. Res. 82, 65–73. doi: 10.1016/j.fishres.2006.08.008
Whitney, N. M., White, C. F., Gleiss, A. C., Schwieterman, G. D., Anderson, P., Hueter, R. E., et al. (2016). A novel method for determining post-release mortality, behavior, and recovery period using acceleration data loggers. Fish. Res. 183, 210–221. doi: 10.1016/j.fishres.2016.06.003
Whittamore, J. M., Bloomer, C., Hanna, G. M., and McCarthy, I. D. (2010). Evaluating ultrasonography as a non-lethal method for the assessment of maturity in oviparous elasmobranchs. Mar. Biol. 157, 2613–2624. doi: 10.1007/s00227-010-1523-4
Williams, R., Lusseau, D., and Hammond, P. S. (2006). Estimating relative energetic costs of human disturbance to killer whales (Orcinus orca). Biol. Conserv. 133, 301–311. doi: 10.1016/j.biocon.2006.06.010
Womble, J. N., Blundell, G. M., Gende, S. M., Horning, M., Sigler, M. F., and Csepp, D. J. (2014). Linking marine predator diving behavior to local prey fields in contrasting habitats in a subarctic glacial fjord. Mar. Biol. 161, 1361–1374. doi: 10.1007/s00227-014-2424-8
Woodworth-Jefcoats, P. A., Polovina, J. J., and Drazen, J. C. (2017). Climate change is projected to reduce carrying capacity and redistribute species richness in North Pacific pelagic marine ecosystems. Global Change Biol. 23, 1000–1008. doi: 10.1111/gcb.13471
Worm, B., Lotze, H. K., Jubinville, I., Wilcox, C., and Jambeck, J. (2017). Plastic as a persistent marine pollutant. Ann. Rev. Environ. Res. 42, 1–26. doi: 10.1146/annurev-environ-102016-060700
Keywords: manta, mobula, devil ray, elasmobranch, management
Citation: Stewart JD, Jaine FRA, Armstrong AJ, Armstrong AO, Bennett MB, Burgess KB, Couturier LIE, Croll DA, Cronin MR, Deakos MH, Dudgeon CL, Fernando D, Froman N, Germanov ES, Hall MA, Hinojosa-Alvarez S, Hosegood JE, Kashiwagi T, Laglbauer BJL, Lezama-Ochoa N, Marshall AD, McGregor F, Notarbartolo di Sciara G, Palacios MD, Peel LR, Richardson AJ, Rubin RD, Townsend KA, Venables SK and Stevens GMW (2018) Research Priorities to Support Effective Manta and Devil Ray Conservation. Front. Mar. Sci. 5:314. doi: 10.3389/fmars.2018.00314
Received: 27 June 2018; Accepted: 15 August 2018;
Published: 18 September 2018.
Edited by:
Mark Meekan, Australian Institute of Marine Science (AIMS), AustraliaReviewed by:
Camrin Braun, MIT/WHOI Joint Program in Oceanography, United StatesNuno Queiroz, Centro de Investigação em Biodiversidade e Recursos Genéticos (CIBIO), Portugal
Yannis Peter Papastamatiou, Florida International University, United States
Copyright © 2018 Stewart, Jaine, Armstrong, Armstrong, Bennett, Burgess, Couturier, Croll, Cronin, Deakos, Dudgeon, Fernando, Froman, Germanov, Hall, Hinojosa-Alvarez, Hosegood, Kashiwagi, Laglbauer, Lezama-Ochoa, Marshall, McGregor, Notarbartolo di Sciara, Palacios, Peel, Richardson, Rubin, Townsend, Venables and Stevens. This is an open-access article distributed under the terms of the Creative Commons Attribution License (CC BY). The use, distribution or reproduction in other forums is permitted, provided the original author(s) and the copyright owner(s) are credited and that the original publication in this journal is cited, in accordance with accepted academic practice. No use, distribution or reproduction is permitted which does not comply with these terms.
*Correspondence: Joshua D. Stewart, am9zaEBtYW50YXRydXN0Lm9yZw==