- 1Unidad Académica de Sistemas Arrecifales, Instituto de Ciencias del Mar y Limnología, Universidad Nacional Autónoma de México, Puerto Morelos, Mexico
- 2Smithsonian Marine Station, Smithsonian Institution, Fort Pierce, FL, United States
- 3Laboratorio de Espectrometría de Masas, Centro Interdisciplinario de Ciencias Marinas, Instituto Politécnico Nacional, La Paz, Mexico
Caribbean coral reefs are undergoing massive degradation, with local increases of macroalgae and reduction of architectural complexity associated with loss of reef-building corals. We explored whether reef degradation affects the feeding ecology of two co-occurring spiny lobsters: Panulirus guttatus, which is an obligate reef-dweller, and Panulirus argus, which uses various benthic habitats including coral reefs. We collected lobsters of both species from the back-reef zones of two large reefs similar in length (∼1.5 km) but differing widely in level of degradation, at the Puerto Morelos Reef National Park (Mexico). We measured the carapace length (CL) and weight (W) of lobsters, estimated three condition indices (hepatosomatic index, HI; blood refractive index, BRI; and W/CL ratio), and analyzed their stomach contents and stable isotope values (δ15N and δ13C). All lobsters tested negative for the presence of the virus PaV1, which can affect nutritional condition. Stomach contents yielded 72 animal taxa, mainly mollusks and crustaceans, with an average of 35 taxa per species per reef, but with much overlap. In P. guttatus, CL, HI, BRI, and W/CL did not vary with reef, but mean isotopic values did. The isotopic niche of P. guttatus showed little overlap between reefs, reflecting differences in local carbon sources and underlining the habitat specialization of P. guttatus, which exhibited a higher trophic position on the more degraded reef. Overall, the trophic position of P. guttatus was higher than that of P. argus. In P. argus, none of the variables differed between reefs and the isotopic niche was wide and with great overlap between reefs, reflecting the broader foraging ranges of P. argus compared to P. guttatus. Additional isotopic values from 16 P. argus caught at a depth of 25 m in the fore reef suggest that these larger lobsters forage over different habitats and have a higher trophic position than their smaller conspecifics and congeners from the back reef. The feeding ecology of P. argus appears to be less influenced by coral reef degradation than that of P. guttatus, but our results suggest a buffering effect of omnivory against habitat degradation for both lobster species.
Introduction
Coral reefs are among the most biodiverse ecosystems around the world, but are being increasingly degraded due to the combined effect of multiple global and local stressors (Hughes et al., 2017). Since the 1980s in particular, populations of key reef building corals (i.e., massive boulder and branching corals) throughout the Caribbean basin have rapidly declined and are being replaced with macroalgae in response to disease, storms, and climate change (Bruno, 2014; Jackson et al., 2014). This phase shift eventually results in loss of structure (i.e., “flattening,” Álvarez-Filip et al., 2009; Bruno et al., 2009). Although most Caribbean reefs are degraded to some extent (Bruno, 2014), different reefs vary in their level of degradation and also in their recovery rates, resulting in wide variations in percent cover of live coral and other constituents of the benthic community (including various types of macroalgae) at the local and landscape scales (Emslie et al., 2008; Edmunds, 2014; Lozano-Álvarez et al., 2017).
There is concern about the potential effects of coral reef degradation on reef-associated communities, yet most studies have mainly focused on scleractinian corals and reef fishes. For example, the loss of structure has been predicted to change the size distribution of reef fishes, particularly those that are habitat-specialists (e.g., Pratchett et al., 2008; Álvarez-Filip et al., 2015). However, in order to understand reef dynamics under rapidly changing conditions, it is necessary to assess ecological changes in other groups, such as motile invertebrates, as these organisms serve as trophic links and can drive key ecological processes on coral reefs (Stella et al., 2011; Kramer et al., 2015). Although these groups have overall been less studied than corals and reef fishes, recent works have found little effects of reef degradation on the diversity or abundance of mobile reef-associated invertebrates in general and of crustaceans in particular (Head et al., 2015; Nelson et al., 2016; Lozano-Álvarez et al., 2017; González-Gómez et al., 2018). Reef degradation, however, may affect associated species in sub-lethal, more subtle ways, such as altering their trophic niche (Letourneur et al., 2017) or reducing their physiological condition due to changes or declines in food availability or quality (Pratchett et al., 2009; Hempson et al., 2018).
Spiny lobsters (Crustacea: Decapoda: Palinuridae) are among the largest and more abundant invertebrates in coral reef habitats (Briones-Fourzán and Lozano-Álvarez, 2013). They are also omnivorous mesopredators highly interlinked within their food webs, acting as consumers of smaller animals and as prey for larger predators. Spiny lobsters sustain important fisheries wherever they occur and many studies have addressed overfishing and management issues for spiny lobsters (reviewed in Phillips et al., 2013). Although spiny lobsters can respond to habitat characteristics (e.g., shelter or food availability) at local and landscape scales (Mai and Hovel, 2007; Wynne and Côté, 2007; MacArthur et al., 2011), little is known about the potential effects of coral reef degradation on the trophic ecology of tropical, reef-associated spiny lobsters (e.g., Lozano-Álvarez et al., 2017).
The congeners Panulirus argus (Caribbean spiny lobster) and P. guttatus (spotted spiny lobster) co-occur on coral reefs throughout the wider Caribbean region (Lozano-Álvarez et al., 2007), but differ in many traits (reviewed in Briones-Fourzán, 2014). For example, P. guttatus is a relatively small spiny lobster (<90 mm carapace length, CL) that is restricted to the coral reef habitat for its entire benthic life (Sharp et al., 1997) and exhibits limited movements, with adults having home ranges of around 100 m in radius (Lozano-Álvarez et al., 2002). In contrast, P. argus undergoes ontogenetic habitat shifts during its benthic life and is a highly mobile lobster, with home ranges tending to increase with lobster size (Herrnkind et al., 1975; Lozano-Álvarez et al., 2003; Bertelsen, 2013). The early juveniles of P. argus remain in the settlement habitat (coastal marine vegetation) for several months but then migrate to coral reef habitats as subadults (∼50 to 80 mm carapace length, CL) (Butler et al., 2006). Adult P. argus (∼80 to 200 mm CL) remain associated with coral reefs, but can occur to depths of 50–100 m (Lozano-Álvarez et al., 1991). These lobsters, usually larger than their conspecifics dwelling on the back-reef/crest zones, may occasionally return to forage on back-reef and reef lagoons areas due to their high mobility (Bertelsen, 2013; Briones-Fourzán and Lozano-Álvarez, 2013). P. argus sustains some of the most valuable fisheries throughout the wider Caribbean region, whereas P. guttatus is mostly targeted by recreational fishers, with small commercial fisheries in only some Caribbean islands. Another important difference between the two congeners is that P. argus, but not P. guttatus, is host to Panulirus argus Virus 1 (PaV1) (Shields and Behringer, 2004). This pathogenic virus can be lethal to juvenile lobsters (Butler et al., 2008) and affects the nutritional condition of the host lobsters (Briones-Fourzán et al., 2009), with potential consequences for populations of P. argus and the fisheries for this species (Behringer et al., 2011).
Both P. guttatus and P. argus have broad diets consisting of a wide variety of invertebrates, mainly small mollusks and crustaceans (e.g., Herrnkind et al., 1975; Colinas-Sánchez and Briones-Fourzán, 1990; Espinosa et al., 1990; Cox et al., 1997; Briones-Fourzán et al., 2003; Butler and Kintzing, 2016). Yet, a stable isotopes analysis (δ13C and δ15N) showed that despite great overlap in the diet of both species in terms of gross taxa, P. guttatus had a higher trophic position (mean δ15N value) than P. argus, and that both species had a very wide range in δ13C values, potentially reflecting differences in primary carbon sources at different spatial scales (Segura-García et al., 2016). In the Puerto Morelos Reef National Park, Mexico, Lozano-Álvarez et al. (2017) found that habitat complexity is an important factor determining the trophic ecology of P. guttatus. By comparing individuals of P. guttatus from two small isolated reef patches differing in architectural complexity, these authors found that P. guttatus from the less complex reef patch consumed proportionally more crustaceans than did conspecifics from the more complex (and less degraded) reef patch, which consumed proportionally more mollusks. Individuals from both patches had similar nutritional condition and δ15N values but differed in mean δ13C values, suggesting a similar diet quality but different carbon sources between reef patches. However, these authors acknowledged that the small size of the reef patches that they studied (1–1.4 ha) might not be representative of the impact of reef degradation on the trophic ecology of P. guttatus populations at larger scales.
Building upon these previous studies, we investigated the potential impacts of coral reef degradation on the feeding ecology of co-occurring P. guttatus and P. argus. As a first approach, our study focused on intra- and interspecific comparisons of stable isotopic niches and metrics, stomach contents, and three indices of nutritional condition between lobsters dwelling in an exceptionally well preserved reef versus lobsters dwelling in a highly degraded reef. We also did isotopic analyses in several individuals of P. argus captured by fishers in deeper waters along the fore-reef zone. Given the different life history traits of these species, we expected the feeding ecology of the habitat specialist (P. guttatus) to be more affected by habitat degradation than that of the generalist species (P. argus). In particular, we predicted that P. guttatus would have a smaller isotopic niche size and a lower mean δ15N value in the more degraded reef.
Materials and Methods
Study Area
The Puerto Morelos Reef National Park (PMRNP), located on the Mexican portion of the Mesoamerican Reef System (MRS, Western Caribbean) (see Figure 1), is a marine protected area that consists of a series of shallow reef units and patches constituting an extended fringing reef system separated from the shoreline by a shallow (<5 m) reef lagoon. The continental shelf is very narrow (<3 km) along most of the eastern coast of the Yucatan peninsula, but begins to widen in the Puerto Morelos area. This shelf configuration favors the development of coral communities on the reef crest and back-reef zone (i.e., the protected zone of the reef facing the continental coast). By contrast, on the fore-reef zone (the exposed zone of the reef facing the open sea) the coral cover is mostly of low relief and is limited to a depth of ∼25 m by a broad sand platform that extends to the edge of the continental slope (Jordán-Dahlgren, 1993). The present study is part of a larger research project into the impacts of coral reef degradation on local food webs and associated communities (González-Gómez et al., 2018; Morillo-Velarde et al., 2018). The research was conducted on two large coral reef units differing in level of degradation, “Limones” (centered at 20°59.1′ N, 86°47.9′ W) and “Bonanza” (centered at 20°57.6′ N, 86°48.9′ W). The two reef units are similar in size (∼1.5 km in length) and are separated from one another by a distance of ∼2 km. However, Limones is considered an exceptional site within the MRS because it contains abundant healthy populations of the Caribbean reef-building coral Acropora palmata, which has all but disappeared in many reefs along the MRS (Rodríguez-Martínez et al., 2014). In contrast, Bonanza is a highly degraded reef with a predominance of erect macroalgae and relic coral skeletons (Morillo-Velarde et al., 2018). Fishing activities have been banned at depths <5 m on both reefs since 1996, when the National Park was established. Bonanza is open to visitation, but Limones is closed to all types of touristic activities since 2014.
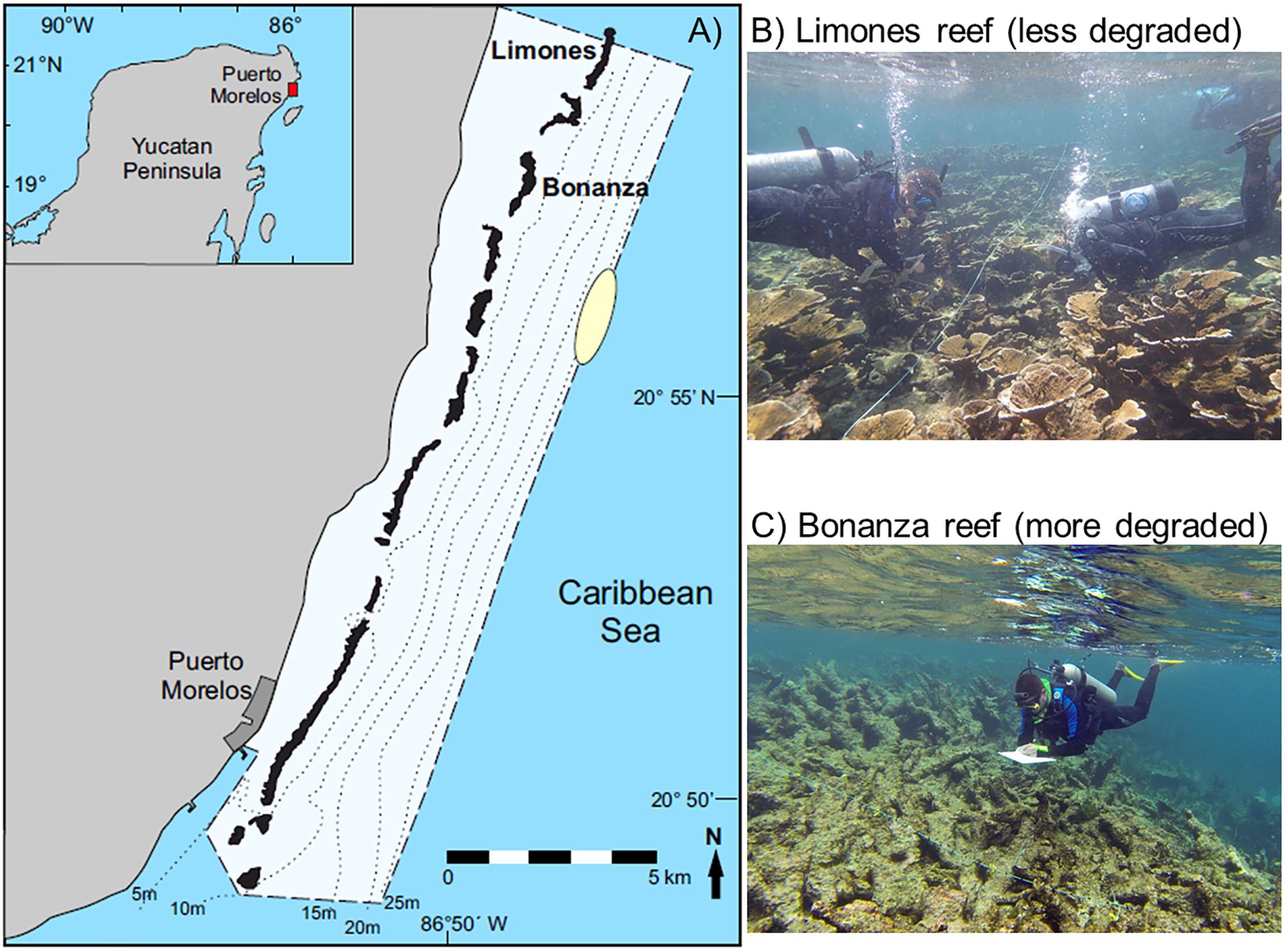
Figure 1. (A) Schematic map of the Puerto Morelos Reef National Park (PMRNP, dashed line), showing the location of the studied reefs, Limones and Bonanza. The dotted lines denote isobaths, and the yellow oval indicates the general area where the deep-habitat lobsters were caught. The inset shows the location of the PMRNP on the Caribbean coast of Mexico. Photographs showing the current state of (B) Limones and (C) Bonanza (Photo credits: Fernando Negrete-Soto).
Assessment of Reef Benthic Constituents
There is evidence that Bonanza has sustained considerably more degradation than Limones since a baseline study conducted in 1985 (Jordán-Dahlgren, 1993). Recently, Morillo-Velarde et al. (2018) assessed the cover of live coral and macroalgae on both reefs based on eight transects over the central part of each reef, whereas González-Gómez et al. (2018) did a similar analysis based on 24 transects on Limones and 21 on Bonanza, but exclusively over the back-reef zone. Here, we expand the benthic assessment by using data from 44 transects randomly laid throughout the back reef and crest zones of Limones and 35 throughout Bonanza. The transects were 10 m in length and marked every 10 cm to estimate the percent cover of various benthic constituents by means of the point intercept method (Risk, 1972). This method consists in recording the type of benthic constituent observed under every 10-cm mark, thus yielding 100 records per transect. We considered the following constituents: live hard coral, crustose coralline algae, algal turf, calcareous macroalgae, fleshy macroalgae, cyanobacteria, sessile invertebrates (sponges, soft corals, Millepora, zoanthids), and other substrates (sand, bare substrate, seagrass). To examine the similarity of benthic constituents between reefs, the percentage data were logit-transformed (Warton and Hui, 2011) and subjected to a principal components analysis (PCA). The transformed data for each constituent were then compared between reefs with General Linear Models (GLM) (Lozano-Álvarez et al., 2017).
Lobster Sampling
We haphazardly collected 15 individuals of P. guttatus and 15 of P. argus from the back-reef to crest zones along the central third of each reef, over a depth range of 1–5 m. The sample size was determined based on isotopic niche metrics from two previous studies conducted in the PMRNP: Segura-García et al. (2016), who found high among-individual niche variation in 12 lobsters of each species sampled from multiple reef sites, and Lozano-Álvarez et al. (2017), who found a clear separation along the δ13C axis but not along the δ15N axis between two samples of 20 P. guttatus each from two different reef sites. The entire lobster sampling on Limones and Bonanza took place over the course of 3 weeks, from October 7 to 29, 2015. Because lobsters hide in crevices during the day and leave their dens to forage after dusk, individual lobsters were collected with hand nets by SCUBA diving between 20:00 and 22:00 h to increase the probability of finding contents in their stomachs. Once on the boat, the lobsters were put into a container with ice slurry to slow digestion and transferred to the laboratory within 1 to 2 h of collection. Each lobster was measured (carapace length, CL, from between the rostral horns to the posterior edge of the carapace) with Vernier calipers (±0.1 mm) and weighed (W, in g) on a digital scale (0.01 g) after blotting excess water. The base of the fifth pereopod was swabbed with 70% ethanol to extract a sample of hemolymph using a sterile 1-ml disposable insulin syringe. A portion of the hemolymph sample was used immediately to determine the blood refractive index (see section “Stable Isotope Analyses”) and the rest was fixed in 95% ethanol and stored at -20°C for further molecular testing for the presence of DNA of P. argus Virus 1 (PaV1) (see section 2.8). Then, each lobster was dissected to obtain a sample of abdominal (tail) muscle and to extract the stomach and hepatopancreas (digestive gland). The hepatopancreas was weighed (HW, ±0.01 g) after blotting excess water. The stomach was preserved in 70% ethanol for further contents analyses.
Stomach Content Analyses
The quantitative analysis of stomach contents followed Briones-Fourzán et al. (2003) and Segura-García et al. (2016). For each individual, stomach contents were examined under a stereomicroscope and grouped into food categories corresponding to gross taxa. Percent frequency of occurrence was estimated for each food category (%F = number of stomachs containing the food category/total number of stomachs examined × 100). All the food categories of an individual stomach were placed on separate parts of a large Petri dish and gently pressed to a uniform depth. The Petri dish had a disk of millimetric paper glued to its exterior. The area (i.e., number of squares) for each food category, measured under a magnifying glass, was used to estimate its percent contribution by volume (%V) to the total volume of stomach contents (i.e., the sum of the areas of all food categories). This technique standardizes the volume estimates irrespective of the size of the lobsters (Joll and Phillips, 1984). With these data, an index of relative importance [IRI = (%V × %F)/100] was computed for each food category. IRI values range from 0 to 100, with values >40 denoting preferred prey, values between 10 and 40 denoting secondary prey, and values <10 denoting occasional or accidental prey. Horn’s index of overlap (Horn, 1966), computed using the %V of food categories, was used to determine diet overlap between conspecific lobsters from both reefs and between congeneric lobsters on the same reef. Horn’s index ranges from 0 (no overlap) to 1 (complete overlap). For interspecific and intraspecific comparisons, values ≤0.6 and ≤0.8, respectively, are considered indicative of major differences in diet (Cartes and Sardà, 1989; Briones-Fourzán et al., 2003).
A more qualitative analysis of the diet was conducted by identifying stomach contents to the lowest taxonomic level possible. In spiny lobsters, the combined action of the mouth parts and the gastric mill can result in highly fragmented contents; therefore, identification of prey was often based on remains such as shell and body fragments, opercula, spines, spicules, claws, appendages, and portions of exoskeletons and calcareous tests. Occasionally, small prey were found almost intact. The diet composition was analyzed using multivariate techniques with PRIMER 6 v6.1.9 (PRIMER-E Ltd.). Differences in the diet composition among the four groups of lobsters (P. argus and P. guttatus from Limones and Bonanza) were analyzed by non-metric multidimensional scaling (nMDS) on a presence/absence matrix using the Russell-Rao similarity coefficient (Jackson et al., 1989). The statistical significance of the observed differences in the diet composition among lobster groups was further tested with a one-way analysis of similarity (ANOSIM). This test provides an R-value indicative of the degree of difference between samples. R-values close to 0 are indicative of little difference while values close to 1 are indicative of a large difference in sample composition (Clarke and Warwick, 2001). We then did a similarity percentage analysis (SIMPER, Clarke, 1993) to identify those species responsible for the observed similarities in diet composition within each group of lobsters and differences in diet composition among the four groups.
Stable Isotope Analyses
Stable isotope analysis is based on naturally occurring differences in the ratios of nitrogen isotopes 15N/14N (δ15N) and carbon isotopes 13C/12C (δ13C) in tissues between consumers and their diet. These differences arise due to preferential retention of heavier isotopes and excretion of lighter isotopes, leading to relative enrichment in 15N and 13C with increasing trophic level (discrimination factors). The amount of 15N in tissues is indicative of an animal’s trophic position, whereas 13C content can be used to recognize primary sources of carbon (i.e., foraging habitat) (Post, 2002; Gulka et al., 2017). Samples of lobster abdominal (tail) muscle were rinsed with ultrapure MilliQ water, dried completely at 60°C and ground to a fine powder with agate mortar and pestle; then ∼1 mg of powdered tissue was transferred into tin capsules. The determination of the δ13C and δ15N composition was conducted at the Mass Spectrometry Laboratory, Centro Interdisciplinario de Ciencias Marinas, Instituto Politécnico Nacional, La Paz, Mexico, using an elemental analyzer (Elemental Combustion System, Costech model 4010) coupled to an isotope ratio mass spectrometer (Finnigan Delta V Plus, Thermo Scientific). The average precision across runs was 0.02‰ for δ13C and 0.1‰ for δ15N. Carbon and nitrogen ratios were expressed in delta (δ) notation, in units of per mil (‰): δ = 1000 × (R sample -R standard)/R standard, where R is the ratio of the heavier, rare isotope (13C or 15N) to the lighter, more common isotope (12C or 14N, respectively) (Post, 2002). Delta values are reported relative to the international standards of Vienna Pee-Dee Belemnite carbon and atmospheric nitrogen.
In contrast with P. guttatus, which does not occur outside the coral reef structure, P. argus occurs to depths in excess of 50 m on the continental shelf off Puerto Morelos (Lozano-Álvarez et al., 1991). For comparative purposes, we obtained a sample of 16 P. argus (mostly tails) caught by local fishers at an average depth of 25 m (range: 24–27 m) along the fore-reef edge. As fishers at Puerto Morelos commercialize only the lobster tails, these lobsters were only used to compare their stable isotope composition with their back-reef/crest conspecifics to examine whether they can also forage on the shallow reef habitats. We estimated the CL of these “deep habitat” lobsters using tail length-CL equations obtained for adult male and female P. argus from the Puerto Morelos area (Padilla-Ramos and Briones-Fourzán, 1997).
The isotopic (i.e., trophic) niche width for the five groups of lobsters (P. guttatus and P. argus from Limones and Bonanza, and P. argus from the deep habitat) was determined based on the isotopic dispersion of samples within a two-dimensional (δ13C and δ15N) space. A variety of niche metrics allows a more precise description of the isotopic niche of different species (Layman et al., 2007a); for example, CR and NR provide the range in δ13C and δ15N values for each group of lobsters, whereas the total area (TA) index, which is a measure of the area of a polygon drawn through the most extreme data points of the isotopic niche space (i.e., the convex hull), provides an estimation of the total trophic diversity of individuals of a given group in the isotopic biplot (Layman et al., 2007a). To estimate dietary differences among groups, we compared their Bayesian standard ellipse area (SEAc), corrected for small sample size by 2 standard deviations (n-1 for each axis), which contains the mean core of the population isotopic niche (40% of 10000 randomizations, Jackson et al., 2011). The trophic niche overlap was calculated as the overlap between each pair of SEAc. These metrics were estimated and plotted using the SIBER routine for the SIAR package in R (Jackson et al., 2011). We also estimated the ratio SEAc/TA, which is a measure of individual variability within the group (the lower the value of this ratio, the higher the individual variability; Letourneur et al., 2017).
To examine the contribution of different primary carbon sources to the diet of P. guttatus and P. argus from Limones and Bonanza we used stable isotopes data from particulate organic matter (POM), macroalgae (four species: Amphiroa tribulus, Caulerpa racemosa, Dictyota cervicornis, and Halimeda tuna), algal turf + epiphytes, and the seagrass Thalassia testudinum, sampled from the two reefs during the same period as our lobsters (see Morillo-Velarde et al., 2018). We did this analysis with Bayesian mixing models (Parnell et al., 2010) using the SIAR package in R (Jackson et al., 2011). Mixing models require the use of discrimination factors, the best of which are those derived from the species under study or, failing that, from closely related species (Gulka et al., 2017). In the absence of specific discrimination factors for P. argus or P. guttatus, we used a range of discrimination factors estimated by Waddington and MacArthur (2008) from tail tissue of spiny lobsters P. cygnus fed various diets (δ15N range 1.67–2.97‰; mean 2.57‰; δ13C range 2.92–3.60‰; mean 3.20‰). The estimated values of the dietary proportion were obtained via a Markov-Chain Monte Carlo (MCMC) simulation.
Indices of Nutritional Condition
Because there is no single best nutritional condition index for use in all situations (Gutzler and Butler, 2017; Hempson et al., 2018), we used three different indices to assess nutritional condition of lobsters: the weight/length (W/CL) ratio, the hepatosomatic index (HSI = HW/W × 100), and the blood (hemolymph) refraction index (BRI) (Lozano-Álvarez et al., 2017). In spiny lobsters, nutritional condition is positively related with both the W/CL ratio and the HSI, but for comparative purposes these indices need to be estimated on lobsters over a similar size range (Briones-Fourzán et al., 2009). The BRI was obtained by reading a portion of the hemolymph sample extracted from each lobster in a density refractometer. BRI is a proxy for physiological condition because hemolymph density is positively correlated with protein concentration in the serum of crustaceans (Lorenzon et al., 2011). However, as the molt cycle affects BRI (Rodríguez-García et al., 2015), we cut the distal third of one pleopod from each lobster to determine the molt stage by microscopy (Lyle and MacDonald, 1983). Only lobsters in intermolt (stage C of Drach’s scale) were used to estimate BRI.
Additional Statistical Analyses
For each lobster species, all response variables (CL, W/CL, BRI, HSI, δ13C and δ15N) were compared between reefs with General Linear Models (GLM), and separate factorial GLMs were further conducted to examine the effect of the two fixed factors (reef and species) on each response variable.
Testing for the Presence of DNA of PaV1
Upon infecting a lobster, PaV1 shows predilection for mesodermal cells, which include fixed phagocytes in the hepatopancreas (Li et al., 2008), and this organ becomes increasingly atrophied as the infection progresses, thus affecting the nutritional condition of the lobster host (Briones-Fourzán et al., 2009). Prevalence of PaV1 can reach 30% at some sites in the Puerto Morelos reef lagoon (Candia-Zulbarán, unpublished data), making it necessary to discard the presence of the virus in our lobsters. The diagnostic clinical sign of PaV1 (a milky hemolymph that does not clot) does not become evident until the lobsters are moderately to severely infected (Cruz-Quintana et al., 2011). Therefore, the presence of PaV1 was tested in hemolymph samples via molecular techniques (PCR) following the protocol of Huchin-Mian et al. (2013). Although P. guttatus does not appear to be affected by PaV1 (Butler et al., 2008), we also tested the hemolymph samples of P. guttatus for the presence of PaV1 to discard them as potential carriers.
Results
Assessment of Reef Benthic Constituents
The first three axes of the PCA analysis of the cover of benthic constituents explained 37.3, 58.6, and 72.3% of the cumulative variance, respectively. A biplot of the first two principal components illustrates how the benthic constituents were interrelated (Figure 2). The first axis was essentially a contrast between live hard coral (loading: -0.742) and fleshy macroalgae (0.608), whereas the second axis accounted for algal turf (loading: 0.707) and coralline algae (0.508) (Figure 2). The third axis (not shown) mostly accounted for the cover of “other substrates” (loading: -0.829). Most transects on Limones differed from those on Bonanza along the first axis. The univariate analyses showed that the cover of most benthic constituents (with the exception of coralline algae, other sessile invertebrates, and other substrates) differed significantly between reefs (Table 1). The cover of live hard coral and algal turf was far greater on Limones, whereas the cover of fleshy macroalgae, cyanobacteria, and calcareous macroalgae was greater on Bonanza (Table 1). Overall, total algal cover was significantly greater on Bonanza than on Limones.
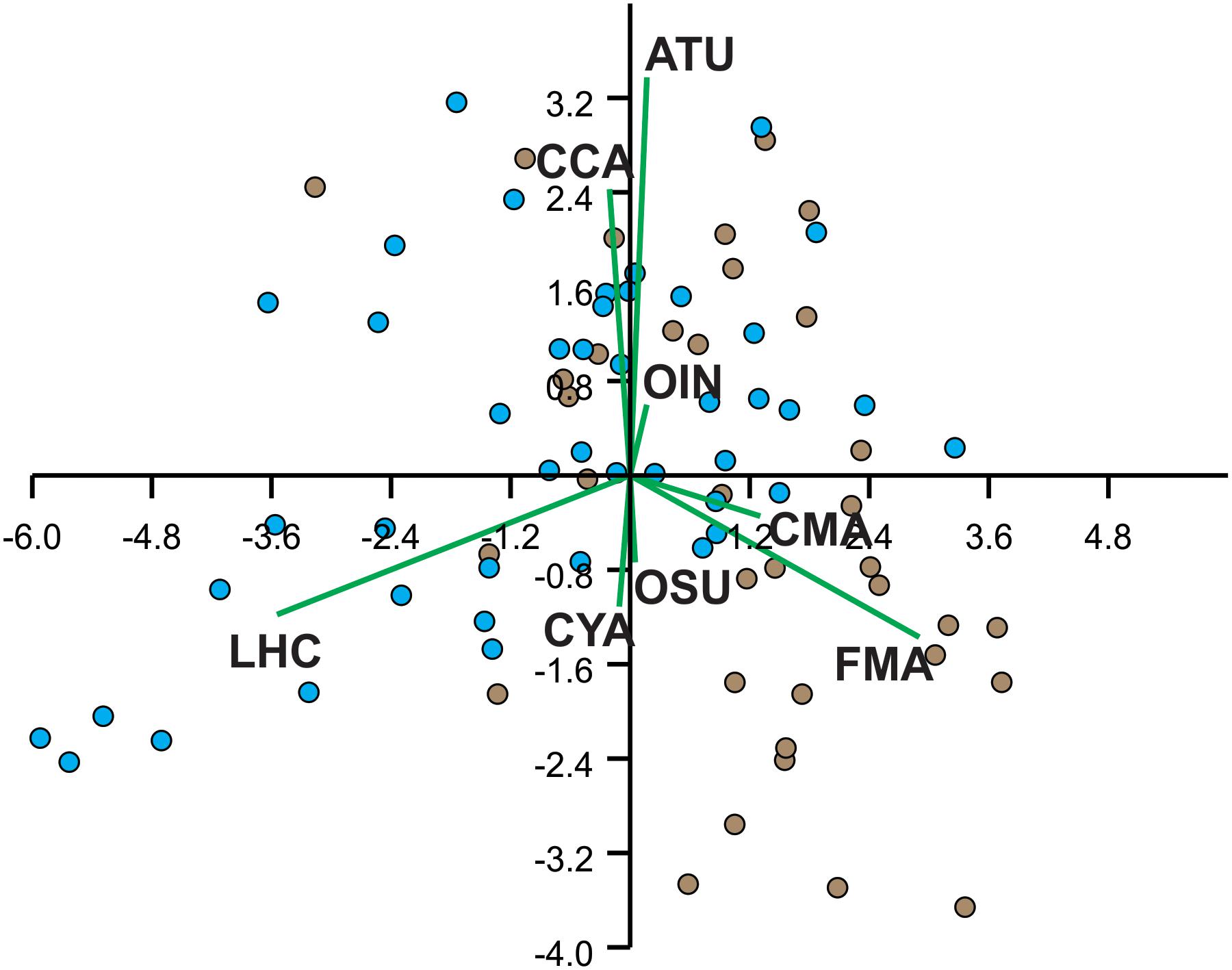
Figure 2. Principal components analysis biplot on logit-transformation of percent cover of benthic constituents over Limones reef (blue dots, N = 44 transects) and Bonanza reef (brown dots, N = 35 transects). Each dot represents one transect. ATU, algal turf; CCA, coralline algae; CMA, calcareous macroalgae; CYA, cyanobacterial mats; FMA, fleshy macroalgae; LHC, live hard coral; OIN, other invertebrates; OSU, other types of substrate.
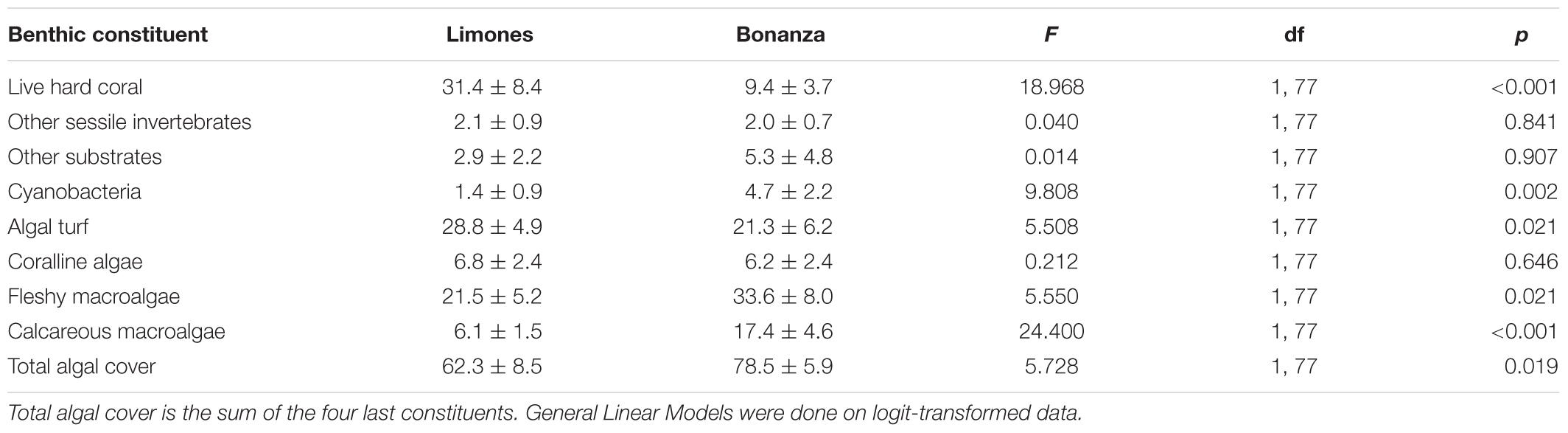
Table 1. Percent cover (mean ± 95% confidence interval) of benthic constituents on the studied reefs as measured in 44 transects on Limones reef and 35 transects on Bonanza reef.
PaV1
All 30 individuals of P. guttatus and all 30 individuals of P. argus tested negative for PaV1 by PCR. Therefore, all 60 lobsters were subjected to the rest of the analyses.
Stomach Contents Analyses
Of the 60 lobsters, only one P. argus from Limones and two P. guttatus from Bonanza had empty stomachs. On both reefs, lobsters of both species fed primarily on the food categories mollusks and crustaceans, followed generally by echinoderms, polychaetes, and sponges (Table 2). Thus, in terms of gross taxa, the diet of conspecific lobsters exhibited significant overlap between reefs (Horn’s index P. argus: 0.952; P. guttatus: 0.903), as did the diet between lobster species on each reef (Limones: 0.803, Bonanza: 0.940). At Limones, no gross taxa emerged as a preferred food category for either species, but at Bonanza, crustaceans were the preferred food category for P. guttatus (IRI = 47.0) and mollusks for P. argus (IRI = 44.7).
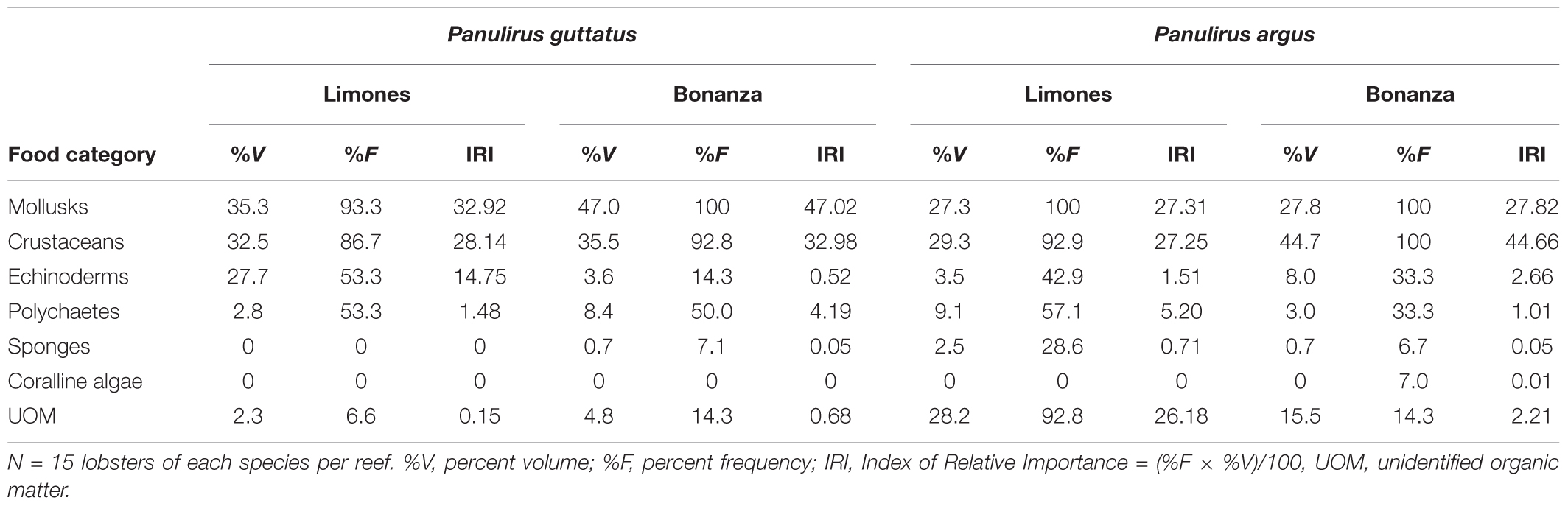
Table 2. Quantitative results of stomach content analyses for Panulirus guttatus and P. argus from Limones and Bonanza reefs.
Identification of stomach contents at a higher resolution (many to species level) yielded 72 different prey taxa, with 34–36 taxa per lobster species per reef, and 1–15 taxa per stomach (Supplementary Table S1). The nMDS 2D-ordination revealed a great overlap of the diet composition among the four groups of lobsters (Figure 3), reflecting their wide diet spectrum. The stress value (0.14) was moderately high, reflecting the presence/absence nature of the data (Clarke and Warwick, 2001). However, a 3D ordination plot (not shown) with a stress value of 0.09 confirmed the great overlap among the four groups of lobsters, which was further corroborated by ANOSIM (R = 0.142). Results of SIMPER (Supplementary Table S2) showed a low similarity in diet composition among conspecific individuals within each reef, ranging from 14.6% for P. guttatus on Limones to 28.5% for P. argus also on Limones, with nine to 11 prey species accounting for ∼90% of within-species similarity per reef. For P. guttatus, the three most common prey species per reef were the chiton Stenoplax purpurascens, the snail Tegula fasciata and the brittle star Ophiocoma wendtii on Limones (jointly accounting for 54.6% of the similarity among individuals), and the buttonsnail Modulus modulus, the stocky cerith Cerithium litteratum, and S. purpurascens on Bonanza (jointly accounting for 46.6% of the similarity). For P. argus, the three most common prey species per reef were T. fasciata, unidentified crabs, and S. purpurascens on Limones (accounting for 71.2% of the similarity), and T. fasciata, Cerithium sp., and unidentified bivalves on Bonanza (accounting for 44.9% of the similarity). Between reefs, dissimilarity in diet composition was higher for P. guttatus (83.9%) than for P. argus (77.4%), whereas within reefs, dissimilarity in diet composition between species was higher on Limones (83.1%) than on Bonanza (75.6%) (Supplementary Table S2).
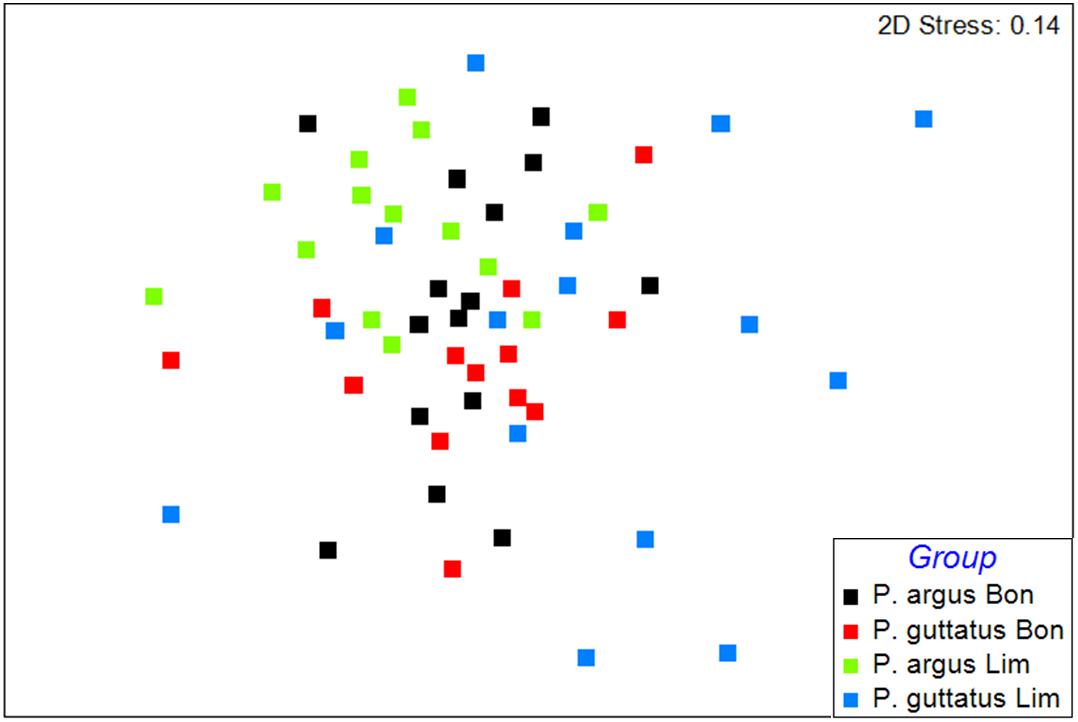
Figure 3. Non-metric multidimensional (nMDS) ordination of diet composition in stomach contents of Panulirus guttatus from Limones (blue squares) and Bonanza (red squares), and P. argus from Limones (green squares) and Bonanza (black squares) based on presence/absence of prey species. Each square denotes an individual lobster (N = 15 in every case).
Isotopic Niche and Metrics
The isotopic niches of the five groups of lobsters examined (P. guttatus from Limones and Bonanza; P. argus from Limones, Bonanza, and the deep habitat) showed some overlap along the δ13C axis but more differentiation along the δ15N axis (Figure 4 and Table 3). The P. argus from Bonanza exhibited the widest CR, which was reflected in a larger isotopic niche area (TA and SEAc, Table 3). The P. argus from both reefs showed the lower mean δ15N values despite their wide NR (Table 3). There was a 96.5% overlap between the SEAc of P. argus from Limones and Bonanza (Figure 4). The isotopic niches of P. guttatus from both reefs were much smaller than those of P. argus, but had little overlap (0.5%) because the P. guttatus from Bonanza had a narrower NR but a higher (more enriched) mean δ15N, and a broader CR but a lower (more depleted) mean δ13C than their conspecifics from Limones (Figure 4 and Table 3). Also, there was no overlap between the isotopic niches of P. guttatus and P. argus from Bonanza and Limones. The P. argus from the deep habitat had the lowest δ13C values but the highest δ15N values, with no overlap between the SEAc of this group and the SEAc of any of the other four groups of lobsters (Figure 4 and Table 3). All lobster groups exhibited moderate SEAc/TA ratio values (i.e., SEAc 2–2.5 times smaller than TA), indicating a relatively broad individual variability in isotopic values (Table 3).
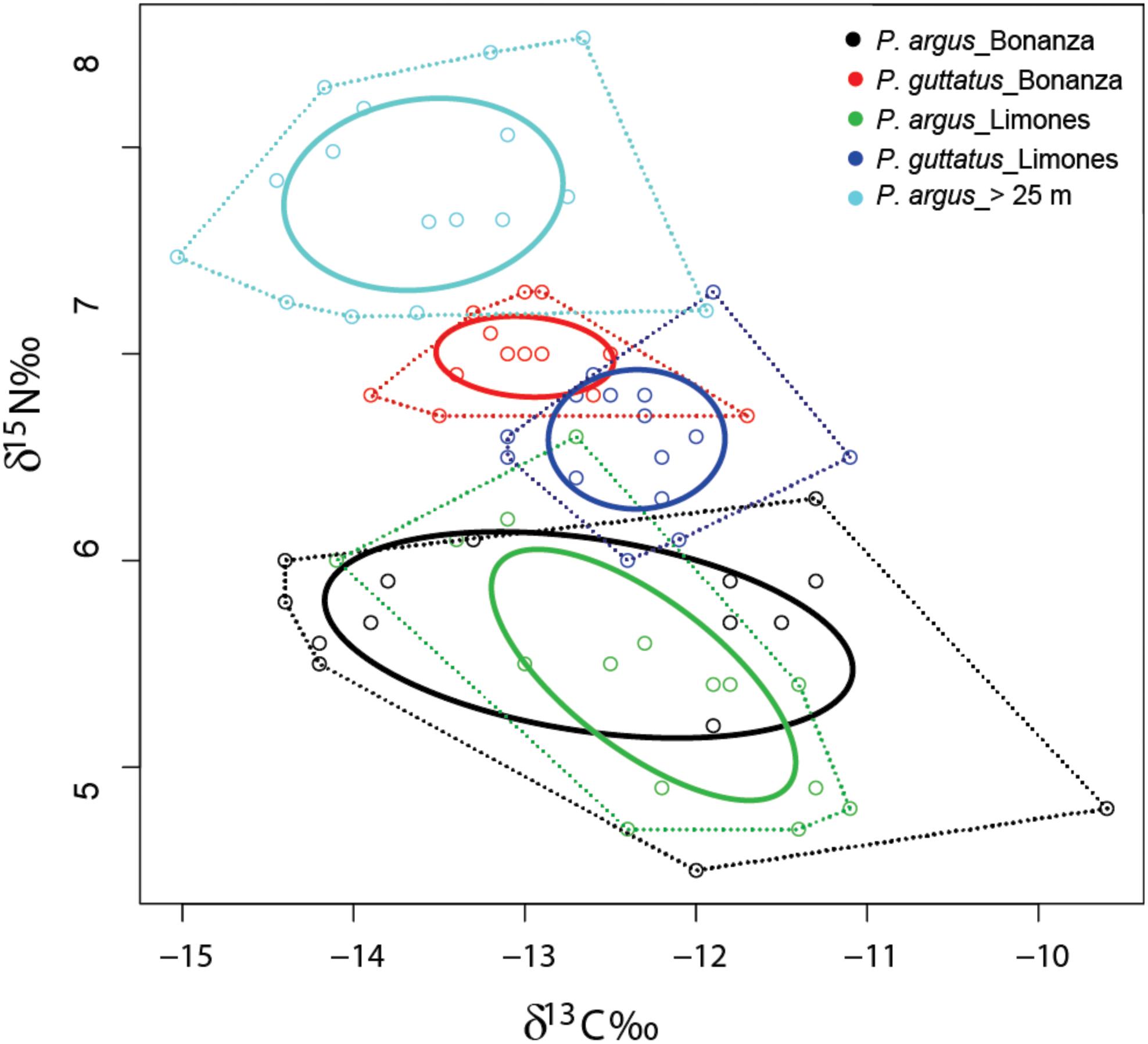
Figure 4. Isotopic niches. δ13C and δ15N values of individuals of P. guttatus from Limones (dark blue circles) and Bonanza (red circles), and of P. argus from Limones (green circles), Bonanza (black circles), and the deep habitat (light blue circles). Solid line of the same color as the dots: standard ellipse area corrected for small sample sizes (SEAc). Dotted line: convex hull area (TA).
Relative Importance of Primary Carbon Sources for Diet of Lobsters
At Bonanza, the main carbon source for both lobster species was POM, which contributed 65 and 70% to the isotopic signatures of P. argus (Figure 5A) and P. guttatus (Figure 5B), respectively. Seagrass emerged as a distant secondary carbon source, contributing ∼20% to the isotopic signature of both species on this reef, whereas the contribution of macroalgae and turf + epiphytes was close to nil (Figures 5A,B). On Limones, by contrast, turf + epiphytes contributed about 50% to the isotopic signature of both lobster species (Figures 5C,D). POM had a similar contribution to P. argus (∼45%), with virtually no contribution from either macroalgae or seagrass to this lobster species (Figure 5C). POM was the second most important contributor for P. guttatus on Limones (∼30%), followed at a distance by macroalgae (∼8%), whereas the contribution of seagrass was almost nil (Figure 5D). The isotopic niche of the large P. argus lobsters from the deep habitat clearly showed that these lobsters forage on habitats different from the shallower back-reef habitat (see section “Isotopic Niche and Metrics”); therefore, the mixed models did not include these lobsters.
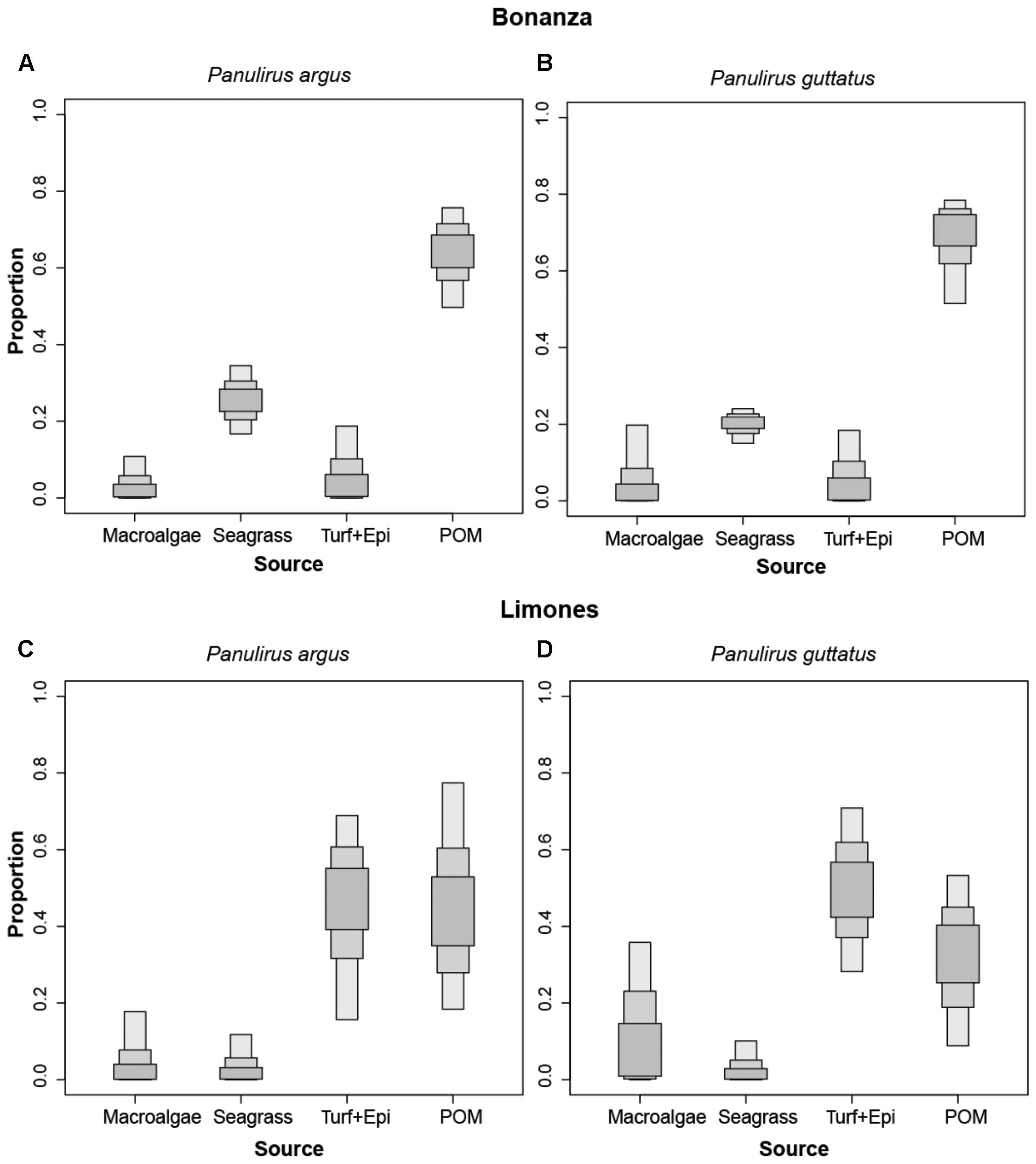
Figure 5. Relative importance of four different basal carbon sources for the spiny lobsters Panulirus argus (A,C) and P. guttatus (B,D) from Bonanza (A,B) and Limones (C,D) reefs. Boxes in different gray shading denote 95% (light), 75% (darker), and 50% (darkest) credibility intervals.
Size, Nutritional Condition, and Mean Stable Isotope Values
Individuals of P. guttatus from Limones (size range: 47.8–80.6 mm CL) and Bonanza (51.4–70.7 mm CL) did not differ significantly in mean size or any of the three nutritional indices, but differed significantly in mean δ15N and δ13C, with lobsters from Bonanza exhibiting higher values of δ15N and lower values of δ13C than lobsters from Limones (Table 4). Individuals of P. argus from Limones (size range: 33.3–80.6 mm CL) and Bonanza (38.5–81.3 mm CL) did not differ significantly in mean size or any of the three nutritional indices but, unlike P. guttatus, their mean values of δ15N and δ13C did not vary with reef (Table 5). The P. argus from the deep habitat (size range: 83.0–134.3 mm CL) had significantly larger mean size and values of δ15N, but lower values of δ13C, than the P. argus from Bonanza and Limones (Table 5).
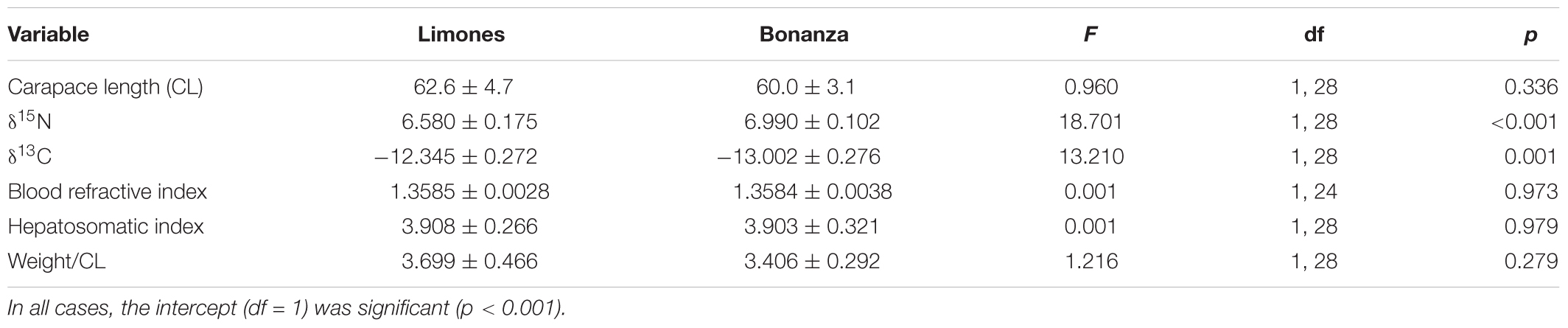
Table 4. Results of General Linear Models testing for effects of reef habitat (Limones, Bonanza) on main response variables for Panulirus guttatus.
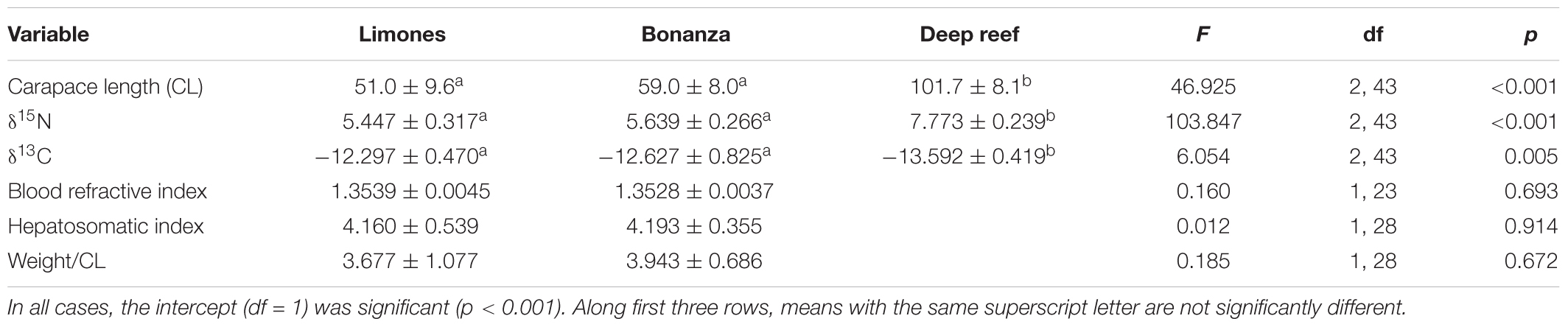
Table 5. Results of General Linear Models testing for effects of reef habitat (Limones, Bonanza, and the deep reef) on main response variables of Panulirus argus.
The factorial GLMs comparing each response variable between lobster species and reef units confirmed that mean values of CL, HSI, and W/CL did not vary with either species or reef (Table 6). BRI was not affected by reef but differed significantly between species, with higher mean values for P. guttatus than for P. argus on both reefs (the interaction term was not significant) (Table 6). Both main factors (reef and species) significantly affected δ15N, with higher values on Bonanza than on Limones and for P. guttatus than for P. argus. Finally, δ13C was not affected by species but varied with reef, with overall more depleted values in the entire sample of lobsters from Bonanza than for Limones. Raw data on individual lobster size and weight, three indices of nutritional condition, and stable isotope values appear in Supplementary Table S3.
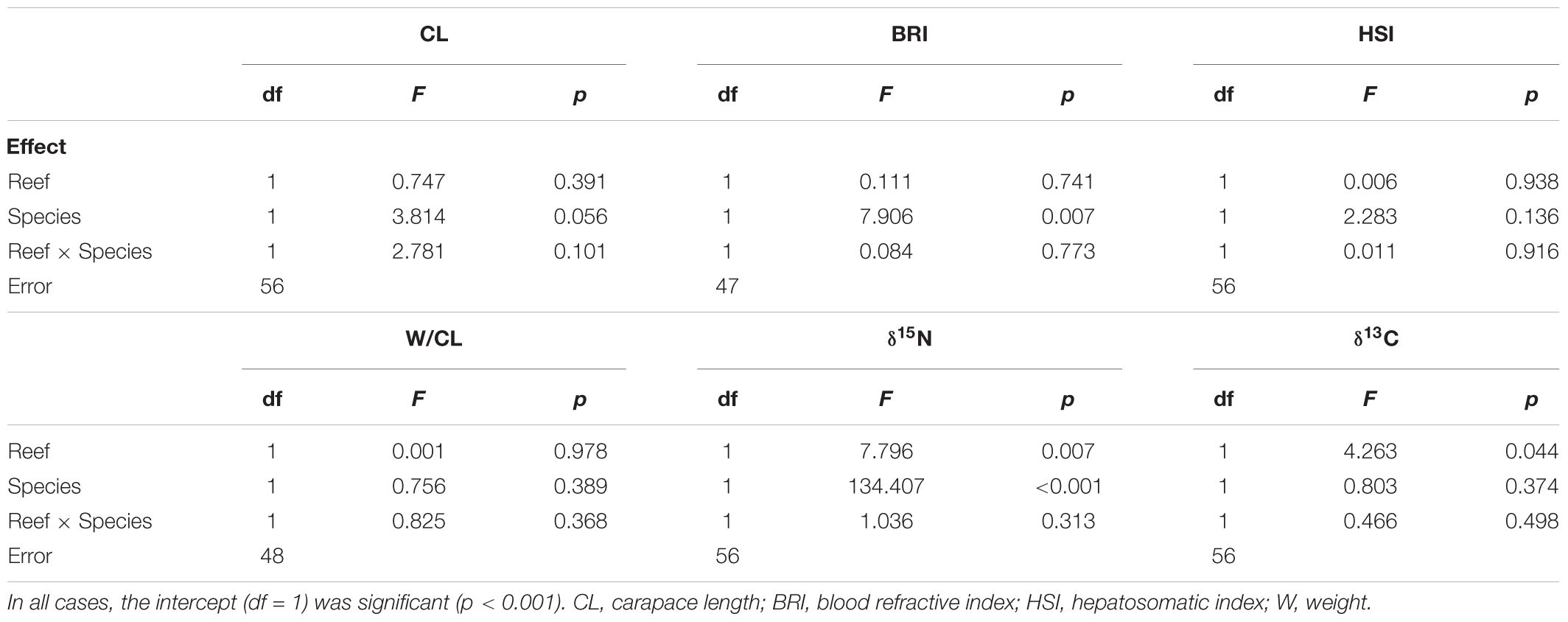
Table 6. Results of General Linear Models testing for effects of reef (Limones, Bonanza) and species (Panulirus guttatus, P. argus) on six response variables of lobsters.
Discussion
In marine systems, omnivorous mesopredators play an important role in the transmission and distribution of organic matter across food webs (Thompson et al., 2007; Long et al., 2011). Habitat degradation and fragmentation is known to affect the trophic ecology and isotopic niche of fish mesopredators (Layman et al., 2007b; Ashworth et al., 2014; Hempson et al., 2017; Letourneur et al., 2017), but here we examined the impact of coral reef degradation on the trophic ecology of two co-occurring, omnivorous mesopredatory spiny lobsters. Our analysis of benthic constituents confirmed that, of our two study reefs, Bonanza is more degraded, with far more cover of fleshy and calcareous macroalgae as well as cyanobacterial mats, whereas Limones still has abundant coral communities dominated by A. palmata (Rodríguez-Martínez et al., 2014; González-Gómez et al., 2018; Morillo-Velarde et al., 2018). We found that the trophic ecology of P. guttatus (the habitat specialist) appeared to be more impacted by reef degradation than that of P. argus. This impact was not evident through differences in stomach contents or nutritional condition but through changes in the isotopic niche, albeit not entirely as predicted. Although the P. guttatus from the more degraded reef (Bonanza) did have a smaller isotopic niche, they exhibited a higher trophic position (more enriched values of δ15N) than the P. guttatus from the less degraded reef (Limones).
Interestingly, these findings contrast with a previous study conducted in the same region in which P. guttatus lobsters from two relatively small reef patches (100 and 140 m in length) differing in rugosity and level of degradation, differed in their mean δ13C values but not in their δ15N values (Lozano-Álvarez et al., 2017). Compared to those small reef patches, however, Limones and Bonanza are much larger reef units (1.5 km in length each). Therefore, the differences in mean δ13C of P. guttatus between Limones and Bonanza likely reflect local differences in carbon sources, which in turn could be related with varying levels of degradation (Bruno, 2014), whereas the higher mean δ15N value of P. guttatus on Bonanza suggests that lobsters on this reef incorporate prey of higher trophic positions in their diet. These stable isotope results provide further evidence that foraging activity of individuals of P. guttatus is constrained to the reef on which they reside (Sharp et al., 1997; Lozano-Álvarez et al., 2002, 2017; Butler and Kintzing, 2016).
In contrast, we did not find a discernible impact of reef degradation on the trophic ecology of P. argus. Most of the P. argus sampled on both reefs were subadults (i.e., <80 mm CL), which, although less mobile than adults (Butler et al., 2006), can still exhibit considerable foraging movements (e.g., up to 400 m overnight, Lozano-Álvarez et al., 2003). Indeed, compared to P. guttatus, the mean δ13C values of P. argus did not vary significantly with reef, but their much broader and greatly overlapping ranges suggest that they may forage in various habitats adjacent to their home reefs, potentially including seagrass meadows, algal beds, rubble, or sandy bottoms of the reef lagoon (Herrnkind et al., 1975; Cox et al., 1997; Bertelsen, 2013). Thus, in contrast with P. guttatus, the isotopic niche of P. argus was larger on Bonanza, suggesting that some individuals from this reef may travel farther to seek food than conspecifics from Limones do. On the other hand, some P. argus lobsters had more depleted δ13C values on Bonanza than on Limones, a pattern similar to that of P. guttatus, suggesting that some P. argus do forage on the reef itself. This could reflect a greater availability of certain types of prey on Bonanza, such as bivalves or cerithiids, which were abundant in stomach contents of P. argus from Bonanza.
Habitat degradation alters trophic pathways of benthic communities. For example, for the same reefs studied here, Morillo-Velarde et al. (2018) showed that turf algae + epiphytes were the most important carbon source for all consumer categories on Limones, denoting a more benthic-derived carbon pathway, whereas particulate organic matter (POM) was the major carbon source for carnivores on Bonanza, denoting a more plankton-derived carbon pathway. We found that POM was indeed a major carbon source for both P. guttatus and P. argus on Bonanza, followed at a distance by seagrass, but on Limones the most important source for P. guttatus was turf + epiphytes, followed by POM, whereas both these sources were equally important for P. argus. Again, these results provide further evidence that P. guttatus do not forage outside their home reef and that P. argus can forage both on the reef and on adjacent habitats. Interestingly, seagrass contributed a proportionally larger fraction of basal carbon to both lobster species on Bonanza (∼25% for P. argus, 20% for P. guttatus) than on Limones (virtually zero), suggesting that seagrass-derived carbon propagates more along the food chain on the more degraded reef than on the healthier reef. In the case of P. argus, this would be consistent with some individuals from Bonanza feeding on nearby seagrass habitats. Although individuals of P. guttatus do not forage outside the coral reef habitat, lobsters of this species have been observed feeding along the reef-seagrass interface (Segura-García et al., 2016).
Unlike the comparatively smaller P. argus from Bonanza and Limones, which forage on the back-reef itself and adjacent habitats over the reef lagoon, the bigger P. argus from the deep habitat appear to forage over the deep fore-reef habitat and nearby sand platform, i.e., sites with a more plankton-derived carbon pathway (France, 1995), as suggested by their lower mean δ13C value. Also, their much higher mean δ15N value indicates that these bigger P. argus are more carnivorous than their smaller conspecifics from shallower reefs (as found for P. cygnus from western Australia, Waddington et al., 2008), and also than the habitat-specialist P. guttatus.
In terms of the relative importance of different food categories (by gross taxa), both P. argus and P. guttatus exhibited a more diverse diet on Limones, where neither species showed preference for a particular food category, than on Bonanza, where P. argus showed preference for crustaceans and P. guttatus for mollusks. Many invertebrates consumed by spiny lobsters (including some gastropods, chitons, and majoid crabs) feed on fleshy, calcareous, and coralline macroalgae (Stachowicz and Hay, 1996), and some can feed on cyanobacterial mats, which are becoming increasingly prominent on declining reefs (Charpy et al., 2012) such as Bonanza. Species of spiny lobsters living in cool-temperate ecosystems can exert control on populations of herbivores such as sea urchins (e.g., P. interruptus in California, United States, Tegner and Levin, 1983; P. penicillatus and P. gracilis in the Galapagos, Ecuador, Edgar et al., 2010; Jasus edwardsii in Tasmania, Australia, Ling et al., 2009; J. lalandii in South Africa, Blamey et al., 2014). This is less likely to occur in the more biodiverse tropical coral reefs, where the local spiny lobsters tend to be more omnivorous, although Butler and Kintzing (2016) postulated that P. guttatus could exert top-down control on highly degraded, but very small (131 m2 on average), patch reefs in the Florida Keys (United States). We found 72 different prey taxa from several phyla in gut contents of both lobster species, with an average of 35 prey taxa per lobster species per reef. These results indicate a very broad diet for both species, as confirmed by the lack of differences obtained in the multivariate analyses conducted on these data and in the SEAc/TA ratio values of all lobster groups. Other studies also identified many prey taxa in gut contents of P. argus (Espinosa et al., 1990; Cox et al., 1997; Martínez-Coello et al., 2015). These results indicate that both P. argus and P. guttatus feed opportunistically, shifting or diversifying their diet according to available resources.
Overall, δ15N values were lower for P. argus than for P. guttatus, indicating that, over time, herbivores contribute more to the diet of P. argus, as suggested by Segura-García et al. (2016). Indeed, some herbivorous mollusks (S. purpurascens, T. fasciata, Cerithium spp.) were among the most abundant prey in stomach contents of P. argus, but also of P. guttatus. Although the higher trophic position of P. guttatus at Bonanza suggests that, over time, these lobsters feed on prey of higher trophic positions than their conspecifics at Limones do, few of the identified carnivorous/scavenger prey species (e.g., the gastropods Calliostoma spp., Bulla striata, Conus spp., Naria spurca, and Vexillum albocinctum; the limpets Diodora spp.; the stomatopod Neogonodactylus oerstedii, and the ophiurid Ophiocoma wendtii) were among the most abundant in stomach contents of P. guttatus. Inconsistencies between the results of stomach contents and stable isotope analyses have been found in other studies (e.g., Ho et al., 2007; Ashworth et al., 2014), but this has been mostly ascribed to a low taxonomic resolution of the diet (Longenecker, 2007), which was not the case in the present study. However, stomach contents provide detailed information on the identity and relative abundance of recently ingested items, whereas stable isotope analyses can provide an indication of what was ingested and assimilated over several weeks; therefore, another potential source of inconsistencies could be the difference in turnover rates between muscle tissue and the different items in the stomach contents. In spiny lobsters, for example, the estimated half-time for muscle tissue is 147 days (Suring and Wing, 2009), whereas evacuation rates of prey vary from a few hours to a few days (Waddington, 2008).
We did not find significant differences in any of the three nutritional indices for either lobster species between Limones and Bonanza. Therefore, despite differences in some components of the diet and in the isotopic niche sizes, the overall quality of the diet appears to be similar between reefs for each species (Lozano-Álvarez et al., 2017). This may reflect the very wide spectrum of prey opportunistically consumed by these omnivorous spiny lobsters. Interestingly, though, P. guttatus had an overall higher BRI than P. argus, suggesting a higher level of serum protein for the habitat specialist, which would appear consistent with P. guttatus being more carnivorous than the P. argus co-occurring on the same reef habitats.
Coral reef degradation appears to be impacting the trophic ecology of P. guttatus more than that of P. argus, but our results suggest a potentially buffering effect of omnivory against reef degradation for both spiny lobster species. However, coral reef degradation is an ongoing process that is expected to continue in the years to come (Hughes et al., 2017), potentially resulting in lobsters having to travel farther to seek food, which may involve shifts in diet and in trophic pathways. Although habitat specialization does not equate with diet specialization, P. guttatus will probably be more affected by reef degradation because this species can neither forage outside the coral reef nor make a facultative use of other habitats. Reef degradation and its associated loss of complexity may also eventually affect these lobsters in other ways by decreasing available refuge or reproduction sites (Wynne and Côté, 2007; Lozano-Álvarez et al., 2017). Precisely because of these issues, productivity of reef fisheries is expected to decline with increasing coral reef degradation (Rogers et al., 2018). However, this might not necessarily be the case for P. argus given the greater movement ranges and colonization abilities of this species that allow it to use various habitats in addition to coral reefs (Briones-Fourzán and Lozano-Álvarez, 2013; Briones-Fourzán, 2014). To further understand the consequences of reef degradation on facultative species such as P. argus, studies involving a wider range of habitats and environmental conditions would be necessary.
Data Availability
All datasets for this study are included in the manuscript and the Supplementary Files.
Author Contributions
PB-F, EL-Á, LÁ-F, and IS-G conceived and designed the work. EL-Á acquired funding for the project. LÁ-F collected data on benthic constituents of reefs. FN-S, CB-O, and PM-V collected and prepared lobster samples for all types of analyses. CB-O conducted stomach contents analysis. AS-G was in charge of stable isotope analyses. PB-F, EL-Á, IS-G, LÁ-F, and AS-G analyzed and interpreted the data. PB-F drafted the work. EL-Á, LÁ-F, IS-G, PM-V, AS-G, FN-S, and CB-O revised the work critically for intellectual content. PB-F and EL-Á approved the final version of the manuscript. All authors agreed to be accountable for all aspects of the work in ensuring that questions related to the accuracy or integrity of the work are appropriately investigated and resolved.
Funding
This study received funding from Universidad Nacional Autónoma de México (Program UNAM-DGAPA-PAPIIT, project IN-205614), granted to EL-Á. UNAM-DGAPA also provided 2 years of financial support to PM-V through a postdoctoral fellowship.
Conflict of Interest Statement
The authors declare that the research was conducted in the absence of any commercial or financial relationships that could be construed as a potential conflict of interest.
Acknowledgments
We thank Sergio Aguíñiga-García for his support in conducting stable isotope analyses, Ángel H. Ruvalcaba-Díaz for his technical assistance in the stable isotope analyses, and Charlotte Eve Davies for testing all lobsters for PaV1. Roberto González-Gómez, Alí Espinosa-Magaña, Leslie Cid-González, Rubén Muñoz de Cote-Hernández, and Martha Oropesa-Ríos provided much appreciated assistance in field and/or laboratory activities. This is contribution No. 1104 of the Smithsonian Marine Station.
Supplementary Material
The Supplementary Material for this article can be found online at: https://www.frontiersin.org/articles/10.3389/fmars.2018.00516/full#supplementary-material
References
Álvarez-Filip, L., Dulvy, N. C., Gill, J. A., Côté, I. M., and Watkinson, A. R. (2009). Flattening of Caribbean coral reefs: region-wide declines in architectural complexity. Proc. R. Soc. B 276, 3019–3025.
Álvarez-Filip, L., Paddack, M. J., Collen, B., Robertson, D. R., and Côté, I. M. (2015). Simplification of Caribbean reef-fish assemblages over decades of coral reef degradation. PLoS One 10:e0126004. doi: 10.1371/journal.pone.0126004
Ashworth, E. C., Depczynski, M., Holmes, T. H., and Wilson, S. K. (2014). Quantitative diet analysis of four mesopredators from a coral reef. J. Fish Biol. 84, 1031–1045. doi: 10.1111/jfb.12343
Behringer, D. C., Butler, M. J. IV, Shields, J. D., and Moss, J. (2011). Review of Panulirus argus virus 1—a decade after its discovery. Dis. Aquat. Org. 94, 153–160. doi: 10.3354/dao02326
Bertelsen, R. D. (2013). Characterizing daily movements, nomadic movements, and reproductive migrations of Panulirus argus around the Western Sambo Ecological Reserve (Florida, USA) using acoustic telemetry. Fish. Res. 144, 91–102. doi: 10.1016/j.fishres.2012.12.008
Blamey, L. K., Plagányi, E. E., and Branch, G. M. (2014). Was overfishing of predatory fish responsible for a lobster-induced regime shift in the Benguela? Ecol. Model. 273, 140–150. doi: 10.1016/j.ecolmodel.2013.11.004
Briones-Fourzán, P. (2014). Differences in life history- and ecological traits between co-occurring Panulirus spiny lobsters (Decapoda: Palinuridae). ZooKeys 457, 289–311. doi: 10.3897/zookeys.457.6669
Briones-Fourzán, P., Baeza-Martínez, K., Lozano, and Álvarez, E. (2009). Nutritional indices of juvenile Caribbean spiny lobsters in a Mexican reef lagoon: are changes over a 10-year span related to the emergence of Panulirus argus virus 1 (PaV1)? J. Exp. Mar. Biol. Ecol. 370, 82–88. doi: 10.1016/j.jembe.2008.12.004
Briones-Fourzán, P., Castañeda-Fernández de Lara, V., Lozano-Álvarez, E., and Estrada-Olivo, J. (2003). Feeding ecology of the three juvenile phases of the spiny lobster Panulirus argus in a tropical reef lagoon. Mar. Biol. 142, 855–865. doi: 10.1007/s00227-003-1013-z
Briones-Fourzán, P., and Lozano-Álvarez, E. (2013). “Essential habitats for Panulirus spiny lobsters,” in Lobsters: Biology, Management, Aquaculture and Fisheries, 2nd Edn, ed. B. F. Phillips (Oxford: Wiley-Blackwell), 186–220. doi: 10.1002/9781118517444.ch7
Bruno, J. F., Sweatman, H., Precht, W. F., Selig, E. R., and Schutte, V. G. W. (2009). Assessing evidence of phase shifts from coral to macroalgal dominance on coral reefs. Ecology 90, 1478–1484. doi: 10.1890/08-1781.1
Butler, M. J. IV, Behringer, D. C., and Shields, J. D. (2008). Transmission of Panulirus argus virus 1 (PaV1) and its effect on the survival of juvenile Caribbean spiny lobster. Dis. Aquat. Org. 79, 173–182. doi: 10.3354/dao01899
Butler, M. J. IV, and Kintzing, M. D. (2016). An exception to the rule: top-down control of a coral reef macroinvertebrate community by a tropical spiny lobster. Bull. Mar. Sci. 92, 137–152. doi: 10.5343/bms.2015.1045
Butler, M. J. IV, Steneck, R. S., and Herrnkind, W. F. (2006). “Juvenile and adult ecology,” in Lobsters: Biology, Management, Aquaculture and Fisheries, ed. B. F. Phillips (Oxford: Blackwell), 263–309.
Cartes, J. E., and Sardà, F. (1989). Feeding ecology of the deep-water aristeid crustacean Aristeus antennatus. Mar. Ecol. Prog. Ser. 54, 229–238. doi: 10.3354/meps054229
Charpy, L., Casareto, B. E., Langlade, M. J., and Suzuki, Y. (2012). Cyanobacteria in coral reef ecosystems: a review. J. Mar. Biol. 2012:259571. doi: 10.1155/2012/259571
Clarke, K. R. (1993). Non-parametric multivariate analyses of changes in community structure. Aust. J. Ecol. 18, 117–143. doi: 10.1111/j.1442-9993.1993.tb00438.x
Clarke, K. R., and Warwick, R. M. (2001). Change in Marine Communities: An Approach to Statistical Analysis and Interpretation, 2nd Edn. Plymouth: PRIMER-E.
Colinas-Sánchez, F., and Briones-Fourzán, P. (1990). Alimentación de las langostas Panulirus guttatus y P. argus (Latreille, 1804) en el Caribe mexicano. An. Inst. Cienc. Mar. Limnol. Univ. Nal. Auton. Mexico 17, 89–106.
Cox, C., Hunt, J. H., Lyons, W. G., and Davis, G. E. (1997). Nocturnal foraging of the Caribbean spiny lobster (Panulirus argus) on offshore reefs of Florida, USA. Mar. Freshw. Res. 48, 671–679. doi: 10.1071/MF97198
Cruz-Quintana, Y., Rodríguez-Canul, R., and Vidal Martínez, V. M. (2011). First evidence of Panulirus argus virus 1 (PaV1) in spiny lobsters from cuba and clinical estimation of its prevalence. Dis. Aquat. Org. 93, 141–147. doi: 10.3354/dao02279
Edgar, G. J., Banks, S. A., Brandt, M., Bustamante, R. H., Chiriboga, A., Earle, S. A., et al. (2010). El Niño, grazers and fisheries interact to greatly elevate extinction risk for Galapagos marine species. Glob. Change Biol. 16, 2876–2890. doi: 10.1111/j.1365-2486.2009.02117.x
Edmunds, P. J. (2014). Landscape-scale variation in coral reef community structure in the United States Virgin Islands. Mar. Ecol. Prog. Ser. 509, 137–152. doi: 10.3354/meps10891
Emslie, M. J., Cheal, A. J., Sweatman, H., and Delean, S. (2008). Recovery from disturbance of coral and reef fish communities on the Great Barrier Reef, Australia. Mar. Ecol. Prog. Ser. 371, 177–190. doi: 10.3354/meps07657
Espinosa, J., Herrera, A., Brito, R., Ibarzábal, D., González, G., Díaz, E., et al. (1990). Los moluscos en la dieta de la langosta del Caribe Panulirus argus (Crustacea: Decapoda). Iberus 9, 127–139.
France, R. L. (1995). Carbon-13 enrichment in benthic compared to planktonic algae: foodweb implications. Mar. Ecol. Prog. Ser. 124, 307–312. doi: 10.3354/meps124307
González-Gómez, R., Briones-Fourzán, P., Álvarez-Filip, L., and Lozano-Álvarez, E. (2018). Diversity and abundance of conspicuous macrocrustaceans on coral reefs differing in level of degradation. PeerJ 6:e4922. doi: 10.7717/peerj.4922
Gulka, J., Carvalho, P. C., Jenkins, E., Johnson, K., Maynard, L., and Davoren, G. K. (2017). Dietary niche shifts of multiple marine predators under varying prey availability on the northeast Newfoundland coast. Front. Mar. Sci. 4:324. doi: 10.3389/fmars.2017.00324
Gutzler, B. C., and Butler, M. J. IV (2017). Comparison of methods for determining nutritional condition in spiny lobsters. J. Shellfish Res. 36, 175–179. doi: 10.2983/035.036.0118
Head, C. E. I., Bonsall, M. B., Koldewey, H., Pratchett, M. S., Speight, M., and Rogers, A. D. (2015). High prevalence of obligate coral-dwelling decapods on dead corals in the Chagos archipelago, central Indian Ocean. Coral Reefs 34, 905–915. doi: 10.1007/s00338-015-1307-x
Hempson, T. N., Graham, N. A. J., MacNeil, M. A., Bodin, N., and Wilson, S. K. (2018). Regime shifts shorten food chains for mesopredators with potential sublethal effects. Funct. Ecol. 32, 820–830. doi: 10.1111/1365-2435.13012
Hempson, T. N., Graham, N. A. J., MacNeil, M. A., Williamson, D. H., Jones, G. P., and Almany, G. R. (2017). Coral reef mesopredators switch prey, shortening food chains, in response to habitat degradation. Ecol. Evol. 7, 2626–2635. doi: 10.1002/ece3.2805
Herrnkind, W. F., van der Walker, J. A., and Barr, L. (1975). Population dynamics, ecology and behavior of spiny lobsters, Panulirus argus, of St John, U.S.V.I. IV. Habitation, patterns of movement and general behavior. Nat. Hist. Mus. Los Angeles Cty. Sci. Bull. 20, 31–45.
Ho, C. T., Kao, S. J., Dai, C. F., Hsieh, H. L., Shiah, F. K., and Jan, R. Q. (2007). Dietary separation between two blennies and the Pacific Gregory in northern Taiwan: evidence from stomach content and stable isotope analyses. Mar. Biol. 151, 729–736. doi: 10.1007/s00227-006-0517-8
Horn, H. S. (1966). Measurement of ‘overlap’ in comparative ecological studies. Am. Nat. 100, 419–424. doi: 10.1086/282436
Huchin-Mian, J. P., Rodríguez-Canul, R., Briones-Fourzán, P., and Lozano-Álvarez, E. (2013). Panulirus argus virus 1 (PaV1) infection prevalence and risk factors in a Mexican lobster fishery employing casitas. Dis. Aquat. Org. 107, 87–97. doi: 10.3354/dao02676
Hughes, T. P., Barnes, M. L., Bellwood, D. R., Cinner, J. E., Cumming, G. S., Jackson, J. B. C., et al. (2017). Coral reefs in the anthropocene. Nature 546, 82–90. doi: 10.1038/nature22901
Jackson, A. L., Inger, R., Parnell, A. C., and Bearhop, S. (2011). Comparing isotopic niche widths among and within communities: SIBER–Stable isotope bayesian ellipses in R. J. Anim. Ecol. 80, 595–602. doi: 10.1111/j.1365-2656.2011.01806.x
Jackson, D. A., Somers, K. M., and Harvey, H. H. (1989). Similarity coefficients: measures of co-occurrence and association or simply measures of occurrence? Am. Nat. 133, 436–453. doi: 10.1086/284927
Jackson, J. B. C., Donovan, M., Cramer, K., and Lam, V. (2014). Status and Trends of Caribbean Coral Reefs: 1970-2012. Gland: Global Coral Reef Monitoring Network, IUCN.
Joll, L. M., and Phillips, B. F. (1984). Natural diet and growth of juvenile western rock lobster Panulirus cygnus. J. Exp. Mar. Biol. Ecol. 75, 145–169. doi: 10.1016/0022-0981(84)90178-3
Jordán-Dahlgren, E. (1993). Atlas de los Arrecifes Coralinos del Caribe Mexicano. Chetumal: Centro de Investigaciones de Quintana Roo.
Kramer, M. J., Bellwood, O., Fulton, C. J., and Bellwood, D. R. (2015). Refining the invertivore: diversity and specialisation in fish predation on coral reef crustaceans. Mar. Biol. 162, 1779–1786. doi: 10.1007/s00227-015-2710-0
Layman, C. A., Arrington, D. A., Montaña, C. G., and Post, D. M. (2007a). Can stable isotope ratios provide for community-wide measures of trophic structure? Ecology 88, 42–48. doi: 10.1890/0012-9658(2007)88[42:CSIRPF]2.0.CO;2
Layman, C. A., Quattrochi, J. P., Peyer, C. M., and Allgeier, J. E. (2007b). Niche width collapse in a resilient top predator following ecosystem fragmentation. Ecol. Lett. 10, 937–944. doi: 10.1111/j.1461-0248.2007.01087.x
Letourneur, Y., Briand, M. J., and Graham, N. A. J. (2017). Coral reef degradation alters the isotopic niche of reef fishes. Mar. Biol. 164:224. doi: 10.1007/s00227-017-3272-0
Li, C., Shields, J. D., Ratzlaff, R. E., and Butler, M. J. (2008). Pathology and hematology of the Caribbean spiny lobster experimentally infected with Panulirus argus virus 1 (PaV1). Virus Res. 132, 104–113. doi: 10.1016/j.virusres.2007.11.005
Ling, S. D., Johnson, C. R., Frusher, S. D., and Ridgway, K. R. (2009). Overfishing reduces resilience of kelp beds to climate-driven catastrophic phase shift. Proc. Natl. Acad. Sci. U.S.A. 106, 22341–22345. doi: 10.1073/pnas.0907529106
Long, Z. T., Bruno, J. F., and Duffy, J. E. (2011). Food chain length and omnivory determine the stability of a marine subtidal food web. J. Anim. Ecol. 80, 586–594. doi: 10.1111/j.1365-2656.2010.01800.x
Longenecker, K. (2007). Devil in the details: high-resolution dietary analysis contradicts a basic assumption of reef-fish diversity models. Copeia 3, 543–555. doi: 10.1643/0045-8511(2007)2007[543:DITDHD]2.0.CO;2
Lorenzon, S., Martins, M., and Ferrero, E. A. (2011). Ecological relevance of hemolymph total protein concentration in seven unrelated crustacean species from different habitats measured predictively by a density-salinity refractometer. J. Mar. Biol. 2011:153654. doi: 10.1155/2011/153654
Lozano-Álvarez, E., Briones-Fourzán, P., and González-Cano, J. (1991). Pesca exploratoria de langostas con nasas en la plataforma continental del área de Puerto Morelos, Q.R. An. Inst. Cienc. Mar Limnol. Univ. Nac. Autón. México 18, 49–58.
Lozano-Álvarez, E., Briones-Fourzán, P., Osorio-Arciniegas, A., Negrete-Soto, F., and Barradas-Ortíz, C. (2007). Coexistence of congeneric spiny lobsters on coral reefs: differential use of shelter resources and vulnerability to predators. Coral Reefs 26, 361–373. doi: 10.1007/s00338-007-0207-0
Lozano-Álvarez, E., Briones-Fourzán, P., and Ramos-Aguilar, M. E. (2003). Distribution, shelter fidelity, and movements of subadult spiny lobsters (Panulirus argus) in areas with artificial shelters. J. Shellfish Res. 22, 533–540.
Lozano-Álvarez, E., Carrasco-Zanini, G., and Briones-Fourzán, P. (2002). Homing and orientation in the spotted spiny lobster, Panulirus guttatus (Decapoda, Palinuridae), towards a subtidal coral reef habitat. Crustaceana 75, 859–867. doi: 10.1163/156854002321210712
Lozano-Álvarez, E., Luviano-Aparicio, N., Negrete-Soto, F., Barradas-Ortiz, C., Aguíñiga-García, S., Morillo-Velarde, P. S., et al. (2017). Does reef architectural complexity influence resource availability for a large reef-dwelling invertebrate? J. Sea Res. 128, 84–91. doi: 10.1016/j.seares.2017.08.010
Lyle, W. G., and MacDonald, C. D. (1983). Molt stage determination in the Hawaiian spiny lobster Panulirus marginatus. J. Crust. Biol. 3, 208–216. doi: 10.2307/1548257
MacArthur, L. D., Phillips, D. L., Hyndes, G. A., Hanson, C. E., and Vanderklift, M. A. (2011). Habitat surrounding patch reefs influences the diet and nutrition of the western rock lobster. Mar. Ecol. Prog. Ser. 436, 191–205. doi: 10.3354/meps09256
Mai, T. T., and Hovel, K. A. (2007). Influence of local-scale and landscape-scale habitat characteristics on California spiny lobster (Panulirus interruptus) abundance and survival. Mar. Freshw. Res. 58, 419–428. doi: 10.1071/MF06141
Martínez-Coello, D., Lopeztegui-Castillo, A., and Amador-Marrero, U. (2015). Diferencias entre sexos en la composición de la dieta natural de la langosta Panulirus argus (Decapoda: Palinuridae) al este del golfo de Batabanó, Cuba. Cuad. Invest. UNED 7, 269–277.
Morillo-Velarde, P. S., Briones-Fourzán, P., Álvarez-Filip, L., Aguíñiga-García, S., Sánchez-González, A., and Lozano-Álvarez, E. (2018). Habitat degradation alters trophic pathways but not food chain length on shallow Caribbean coral reefs. Sci. Rep. 8:4109. doi: 10.1038/s41598-018-22463-x
Nelson, H. R., Kuempel, C. D., and Altieri, A. H. (2016). The resilience of reef invertebrate biodiversity to coral mortality. Ecosphere 7:e1399. doi: 10.1002/ecs2.1399
Padilla-Ramos, S. A., and Briones-Fourzán, P. (1997). Biological characteristics of the spiny lobsters (Panulirus spp.) from the commercial catch in Puerto Morelos, Quintana Roo, México. Cienc. Mar. 23, 175–193. doi: 10.7773/cm.v23i2.798
Parnell, A. C., Inger, R., Bearhop, S., and Jackson, A. L. (2010). Source partitioning using stable isotopes: coping with too much variation. PLoS One 5:e9672. doi: 10.1371/journal.pone.0009672
Phillips, B. F., Melville-Smith, R., Kay, M. C., and Vega-Velázquez, A. (2013). “Panulirus species,” in Lobsters: Biology, Management, Aquaculture and Fisheries, 2nd Edn, ed. B. F. Phillips (Oxford: Wiley-Blackwell), 289–325. doi: 10.1002/9781118517444.ch10
Post, D. M. (2002). Using stable isotopes to estimate trophic position: models, methods, and assumptions. Ecology 83, 703–718. doi: 10.1890/0012-9658(2002)083[0703:USITET]2.0.CO;2
Pratchett, M. S., Munday, P. L., Wilson, S. K., Graham, N. A. J., Cinner, J. E., Bellwood, D. R., et al. (2008). Effects of climate-induced coral bleaching on coral-reef-fishes: ecological and economic consequences. Oceanogr. Mar. Biol. Annu. Rev. 46, 251–296. doi: 10.1201/9781420065756.ch6
Pratchett, M. S., Wilson, S. K., Graham, N. A. J., Munday, P. L., Jones, G. P., and Polunin, N. V. C. (2009) “Coral bleaching and consequences for motile reef organisms: past, present and uncertain future effects” in Coral Bleaching, eds M. J. H. van Oppen and J. M. Lough (Berlin: Springer), 139–158. doi: 10.1007/978-3-540-69775-6_9
Risk, M. J. (1972). Fish diversity on a coral reef in the Virgin Islands. Atoll Res. Bull. 193, 1–6. doi: 10.5479/si.00775630.153.1
Rodríguez-García, O. U., Castañeda-Fernández de Lara, V., Rodríguez-Jaramillo, C., and Serviere-Zaragoza, E. (2015). Nutritional condition and gonad development of juvenile and subadult California spiny lobster Panulirus interruptus in two habitats. Rev. Biol. Mar. Oceanogr. 50, 261–270. doi: 10.4067/S0718-19572015000300005
Rodríguez-Martínez, R. E., Banaszak, A. T., McField, M. D., Beltrán-Torres, A. U., and Álvarez-Filip, L. (2014). Assessment of Acropora palmata in the Mesoamerican Reef System. PLoS One 9:e96140. doi: 10.1371/journal.pone.0096140
Rogers, A., Blanchard, J. L., and Mumby, P. J. (2018). Fisheries productivity under progressive coral reef degradation. J. Appl. Ecol. 55, 1041–1049. doi: 10.1111/1365-2664.13051
Segura-García, I., Briones-Fourzán, P., de Lestang, S., and Lozano-Álvarez, E. (2016). Dietary partitioning between sympatric species of spiny lobster in a coral reef system. Bull. Mar. Sci. 92, 355–369. doi: 10.5343/bms.2015.1073
Sharp, W. C., Hunt, J. H., and Lyons, W. G. (1997). Life history of the spotted spiny lobster, Panulirus guttatus, an obligate reef-dweller. Mar. Freshw. Res. 48, 687–698. doi: 10.1071/MF97125
Shields, J. D., and Behringer, D. C. Jr. (2004). A new pathogenic virus in the Caribbean spiny lobster Panulirus argus from the Florida Keys. Dis. Aquat. Org. 59, 109–118. doi: 10.3354/dao059109
Stachowicz, J. J., and Hay, M. E. (1996). Facultative mutualism between an herbivorous crab and a coralline alga: advantages of eating noxious seaweeds. Oecologia 105, 377–387. doi: 10.1007/BF00328741
Stella, J. S., Pratchett, M. S., Hutchings, P. A., and Jones, G. P. (2011). Coral-associated invertebrates: diversity, ecological importance and vulnerability to disturbance. Oceanogr. Mar. Biol. Annu. Rev. 49, 43–104. doi: 10.1201/b11009-3
Suring, E., and Wing, S. R. (2009). Isotopic turnover rate and fractionation in multiple tissues of red rock lobster (Jasus edwardsii) and blue cod (Parapercis colias): consequences for ecological studies. J. Exp. Mar. Biol. Ecol. 370, 56–63. doi: 10.1016/j.jembe.2008.11.014
Tegner, M. J., and Levin, L. A. (1983). Spiny lobsters and sea urchins: analysis of a predator-prey interaction. J. Exp. Mar. Biol. Ecol. 73, 125–150. doi: 10.1016/0022-0981(83)90079-5
Thompson, R. M., Hemberg, M., Starzomsky, B. M., and Shurin, J. B. (2007). Trophic levels and trophic tangles: the prevalence of omnivory in real food webs. Ecology 88, 612–617. doi: 10.1890/05-1454
Waddington, K. (2008). Variation in evacuation rates of different foods skew estimates of diet in the western rock lobster Panulirus cygnus. Mar. Freshw. Res. 59, 347–350. doi: 10.1071/MF07156
Waddington, K. I., Bellchambers, L. M., Vanderklift, M. A., and Walker, D. I. (2008). Western rock lobsters (Panulirus cygnus) in Western Australian deep coastal ecosystems (35–60 m) are more carnivorous than those in shallow coastal ecosystems. Estuar. Coast Shelf Sci. 79, 114–120. doi: 10.1016/j.ecss.2008.03.008
Waddington, K. I., and MacArthur, L. (2008). Diet quality and muscle tissue location influence consumer-diet discrimination in captive-reared rock lobsters (Panulirus cygnus). Mar. Biol. 154, 569–576. doi: 10.1007/s00227-008-0950-y
Warton, D. I., and Hui, F. K. C. (2011). The arcsine is asinine: the analysis of proportions in ecology. Ecology 92, 3–10. doi: 10.1890/10-0340.1
Keywords: Caribbean sea, habitat degradation, nutritional condition, omnivory, Panulirus argus, Panulirus guttatus, stable isotope analyses, stomach contents
Citation: Briones-Fourzán P, Álvarez-Filip L, Barradas-Ortíz C, Morillo-Velarde PS, Negrete-Soto F, Segura-García I, Sánchez-González A and Lozano-Álvarez E (2019) Coral Reef Degradation Differentially Alters Feeding Ecology of Co-occurring Congeneric Spiny Lobsters. Front. Mar. Sci. 5:516. doi: 10.3389/fmars.2018.00516
Received: 13 September 2018; Accepted: 21 December 2018;
Published: 11 January 2019.
Edited by:
Rochelle Diane Seitz, William & Mary’s Virginia Institute of Marine Science, College of William & Mary, United StatesReviewed by:
Gulnihal Ozbay, Delaware State University, United StatesKevin Alexander Hovel, San Diego State University, United States
Copyright © 2019 Briones-Fourzán, Álvarez-Filip, Barradas-Ortíz, Morillo-Velarde, Negrete-Soto, Segura-García, Sánchez-González and Lozano-Álvarez. This is an open-access article distributed under the terms of the Creative Commons Attribution License (CC BY). The use, distribution or reproduction in other forums is permitted, provided the original author(s) and the copyright owner(s) are credited and that the original publication in this journal is cited, in accordance with accepted academic practice. No use, distribution or reproduction is permitted which does not comply with these terms.
*Correspondence: Patricia Briones-Fourzán, YnJpb25lc0BjbWFybC51bmFtLm14
† Present address: Piedad S. Morillo-Velarde, CONACYT–Instituto de Ciencias Marinas y Pesquerías, Universidad Veracruzana, Veracruz, Mexico