- 1CONACyT-Instituto de Ciencias del Mar y Limnología, Universidad Nacional Autónoma de México, Mexico City, Mexico
- 2Alfred-Wegener-Institut, Helmholtz-Zentrum für Polar-und Meeresforschung, Bremerhaven, Germany
- 3Instituto de Ciencias Marinas y Pesquerías, Universidad Veracruzana, Veracruz, Mexico
Many benthic dinoflagellates are known or suspected producers of lipophilic polyether phycotoxins, particularly in tropical and subtropical coastal zones. These toxins are responsible for diverse intoxication events of marine fauna and human consumers of seafood, but most notably in humans, they cause toxin syndromes known as diarrhetic shellfish poisoning (DSP) and ciguatera fish poisoning (CFP). This has led to enhanced, but still insufficient, efforts to describe benthic dinoflagellate taxa using morphological and molecular approaches. For example, recently published information on epibenthic dinoflagellates from Mexican coastal waters includes about 45 species from 15 genera, but many have only been tentatively identified to the species level, with fewer still confirmed by molecular criteria. This review on the biodiversity and biogeography of known or putatively toxigenic benthic species in Latin America, restricts the geographical scope to the neritic zones of the North and South American continents, including adjacent islands and coral reefs. The focus is on species from subtropical and tropical waters, primarily within the genera Prorocentrum, Gambierdiscus/Fukuyoa, Coolia, Ostreopsis and Amphidinium. The state of knowledge on reported taxa in these waters is inadequate and time-series data are generally lacking for the prediction of regime shift and global change effects. Details of their respective toxigenicity and toxin composition have only recently been explored in a few locations. Nevertheless, by describing the specific ecosystem habitats for toxigenic benthic dinoflagellates, and by comparing those among the three key regions - the Gulf of Mexico, Caribbean Sea and the subtropical and tropical Pacific coast, insights for further risk assessment of the global spreading of toxic benthic species is generated for the management of their effects in Latin America.
Introduction to Benthic Dinoflagellates
Benthic dinoflagellates are important components of marine ecosystems, implicated in benthic food webs and species interactions, including bacterial associations, and also contribute to community diversity. Such species are common in shallow waters, and are frequently found attached to diverse substrates through the production of polysaccharide filaments and mucous layers (Honsell et al., 2013). In natural habitats, they tend to thrive in undisturbed locations and accumulate under calm water conditions. Benthic dinoflagellates do not create “blooms” in the conventional sense, as planktonic species do in the water column, but often yield dense aggregations or colonies of attached cells. In cases where dense cell aggregations are associated with harmful events, they are frequently referred to as “benthic harmful algal blooms” (or bHABs) but this terminology is not precisely defined. Whereas benthic dinoflagellates are better known and more completely described from tropical and subtropical waters, species are found globally even from temperate, sub-arctic and polar environments. Although optimal growth conditions are still unknown for many benthic species, their high relative cell abundance and diversity in shallow subtropical and tropical waters indicates that higher cell growth is favored at elevated water temperatures. In the Caribbean, there is an apparent correlation between high water temperature and Gambierdiscus Adachi & Fukuyo cell abundance (Tester et al., 2010), but this relationship is not clear for other benthic dinoflagellates.
Many benthic species are considered to be low-light (“shade”) adapted, and can therefore flourish in deeper waters within tropical environments with higher water transparency, while some species can even tolerate high irradiance (Richlen and Lobel, 2011). Tropical waters with abundant benthic dinoflagellate communities are usually characterized by high salinity, but there is little direct evidence from culture experiments or observations of natural populations that such high salinities are necessary for growth.
Benthic dinoflagellates comprise both phototrophic and heterotrophic species, and mixotrophic capacity is also common. In fact, it is likely that all benthic species are capable of facultative heterotrophy and may be less dependent on inorganic nutrient concentration than phototrophic pelagic species, since they can acquire organic nutrients not only from the surrounding waters but also from the sediments or other surfaces upon which they live (Litaker et al., 2010).
Although benthic and, in particular, epiphytic dinoflagellates are designated as such because of their typical preference for substrate attachment, the vegetative cells of free-living species are flagellated and are fully capable of detachment and motility. They can occupy a wide variety of micro-niches provided by these alternative substrate habitats, compared to plankton species in the water column. For example, the genus Ostreopsis Schmidt is widely distributed in both tropical and temperate waters, often in shallow sheltered embayments or reefs, adhering to different substrates such as macroalgae, seagrasses, pebbles, rocks, coral rubble, mussel shells, soft sediments and invertebrates, but can also be found in the water column (Tindall and Morton, 1998; Totti et al., 2010; Accoroni et al., 2015). Many benthic dinoflagellates are photosynthetic endosymbionts (“zooxanthellae”), e.g., Symbiodinium spp. or the species from other recently described closely related genera (LeJeunesse et al., 2018), among corals, sea anemones, nudibranchs and other sessile and vagile macrobenthic invertebrates, but none of those endosymbionts are known to be toxigenic.
A comprehensive understanding of the diverse life history strategies of benthic dinoflagellates is still lacking and is even more limited than that available for free-living planktonic species. A few life history studies have been performed on Gambierdiscus and Ostreopsis (Bravo et al., 2014), for which both meiosis and gametogenesis have been described, but much remains unresolved with reference to sexual and asexual cycles. High apparent morphological variability, e.g., within Ostreopsis, could correspond to alternative life history stages, but this has not been confirmed.
Ocean Environment, Human Health and Socio-Economic Consequences
Among the plethora of benthic dinoflagellates, several species collected primarily from seagrasses and macroalgal substrates are known to produce potent biotoxins (Boisnoir et al., 2018). In fact, as a proportion of the described taxa of free-living marine dinoflagellates, epiphytic and sand-dwelling species are heavily represented among known toxigenic genotypes. Such toxins from benthic dinoflagellates are often found within complex toxic species assemblages and are therefore of great concern worldwide (Ben-Gharbia et al., 2016), but associations of individual dinoflagellate species with particular toxin chemotypes is often unclear. There is a critical lack of research on bHABs, based on the objective knowledge of the real threat that these organisms represent. This is particularly true in Latin America, although there have been significant improvements in addressing these phenomena by regional research institutions (Barón-Campis et al., 2014) and the associated toxin syndromes by health authorities. Major global efforts have now been initiated to describe and understand the dynamics and biodiversity of bHAB populations (Berdalet et al., 2016) and to develop effective strategies to monitor and mitigate the consequences.
Benthic HABs are of particular significance in tropical and subtropical regions, for coastal and island communities where the ocean is crucial for seafood production for local inhabitants, and also for global trade. These regions are frequently threatened by human illnesses and marine faunal toxicity associated with toxic benthic and epiphytic dinoflagellates (Berdalet et al., 2017). The toxic effects on humans and other organisms often occurs when bHABs are present in persistently high cell abundances, but with certain species, these effects can be expressed via biomagnification even when highly toxigenic populations are present at low cell densities (Berdalet et al., 2016).
In Latin America, several human intoxication syndromes are caused by benthic dinoflagellates due to the bioaccumulation of their toxins within the food web and transfer into seafood species. For example, on the northwestern Pacific coast of Mexico, several cases of human poisoning were a direct result of the consumption of fish species of the families Serranidae and Lutjanidae (Sierra-Beltrán et al., 1998). In general, in Latin America there is a high local consumption of fish and shellfish from commercial and artisanal fisheries, including non-common species such as octopus and sea cucumber with poorly described accumulation kinetics, and from emerging aquaculture activities (Barón-Campis et al., 2014). The economic consequences of bHABs on human health and losses to the fishing, aquaculture and tourism industries in Latin America have not been comprehensively investigated, but informal estimates suggest that these are substantial (Cuellar-Martinez et al., 2018).
Monitoring programs for bHAB species and their toxins in seafood are sporadic on a global scale. In Latin America only a few countries have implemented routine monitoring programs and these focus exclusively on commercial species (Cuellar-Martinez et al., 2018). With such inadequate monitoring protocols to protect human health, some fish species have been banned for human consumption, e.g., in Australia and French Polynesia, because of the risk of CFP (Lehane, 2000). This is also the case in Quintana Roo on the Caribbean coast of Mexico, where fishing, selling and consumption of the great barracuda (Sphyraena barracuda Walbaum), a known ciguateric fish, is forbidden (García-Mendoza et al., 2016; López, 2018). In spite of the health risks posed by bHAB toxins, many toxins are still not well characterized structurally or toxicologically, and reliable quantitative analysis and assay methods for such toxins are still emerging.
Biogeography of Toxigenic Benthic Dinoflagellates
The distribution of benthic dinoflagellates within Latin America is expected to reflect adaptive capacity, tolerance and resilience of species within ecological niches defined by the ambient environmental conditions. For these reasons, the distribution patterns are considered herein from the perspective of linked or contiguous functional ecosystems, which can only be roughly defined, rather than by geographical or geopolitical boundaries (Table 1 and Figure 1). The temperate and Arctic eastern coasts of North America (north of Mexico) are therefore not considered within the purview of this review on toxigenic benthic dinoflagellates. On a cautionary note, it should be recognized that reported species distribution may more accurately reflect the intensity of regional surveys rather than a true biogeographical pattern. Taxonomic revisions can also bias interpretation of biogeographical species patterns. For example, Prorocentrum rhathymum is considered here as distinct from P. mexicanum because these taxa are morphologically and ecologically separable (Cortés-Altamirano and Sierra-Beltrán, 2003; An et al., 2010), although it has been claimed that they are synonymous (Steidinger and Tangen, 1997; Faust and Gulledge, 2002; Lim et al., 2013; Gómez et al., 2017a; Steidinger, 2018). A final caveat regarding biogeographical description of benthic dinoflagellate species distribution is due to incomplete knowledge of the linkage between species descriptors, either morphological or molecular, and actual or potential toxigenicity at the local population level.

Table 1. Potentially toxic benthic dinoflagellate species reported in Latin America and adjacent subtropical waters.
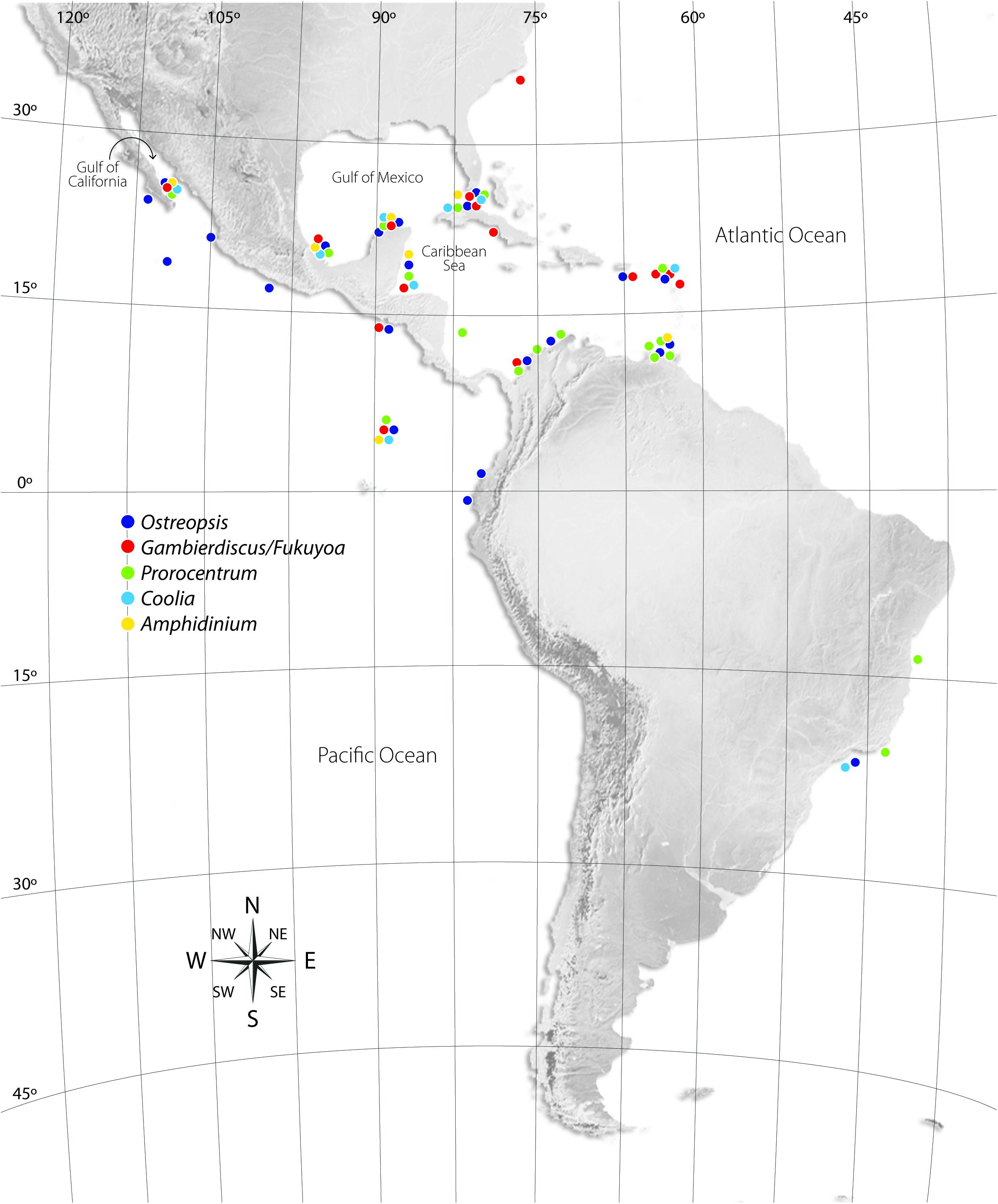
Figure 1. Known biogeographical distribution of dinoflagellate genera with toxigenic benthic members from tropical and subtropical waters of Latin America, as well as the Caribbean Sea and adjacent coasts of the Americas. Genera including toxigenic benthic species were obtained from the IOC-Harmful Microalgal Species list (Moestrup et al., 2009), but the map may include taxa not yet confirmed as toxigenic at the specified locations.
Eastern Pacific Coast of Latin America
With respect to biogeographical distribution of potentially toxigenic species of the eastern Pacific of Latin America, the region is defined to include the coast of Mexico, Guatemala, El Salvador, Honduras, Nicaragua, Costa Rica, Panama, Colombia, Ecuador, Peru and Chile.
The Pacific coastal waters of Baja California Sur, Mexico, have been relatively well explored for the distribution of benthic dinoflagellates. Four putatively toxic Ostreopsis species have been found: O. lenticularis, O. marina, O. ovata and O. siamensis (Sierra-Beltrán et al., 1998; Núñez-Vázquez et al., 2000; Cortés-Lara et al., 2005; Gárate-Lizárraga, 2005; Núñez-Vázquez, 2005; Okolodkov and Gárate-Lizárraga, 2006). The latter species was also reported from Isabel Island, Nayarit, 70 km from the mainland (Cortés-Lara et al., 2005; Cortés-Altamirano et al., 2011). Gambierdiscus toxicus has been found in several studies in the adjacent waters of Baja California Sur [see references in Okolodkov and Gárate-Lizárraga (2006)]. Some benthic dinoflagellate species isolated from Bahía de La Paz and Bahía Concepción in the southern Gulf of California are maintained in culture: Prorocentrum belizeanum, P. cf. concavum, P. lima, P. maculosum, O. cf. ovata and P. rhathymum, (Morquecho-Escamilla et al., 2016), and confirmation of toxin production is currently underway. Further macroalgal samples collected at Isla San José and Bahía de La Paz in 2011, 2014, and 2016, yielded cultures of Amphidinium operculatum, Bysmatrum gregarium, Coolia canariensis, Fukuyoa yasumotoi, G. cf. carolinianus, G. cf. carpenteri, O. marina, O. ovata, P. concavum, P. fukuyoi, P. lima and P. rhathymum (Morquecho-Escamilla et al., 2017). Ostreopsis ovata has also been observed along the Pacific coastal state of Guerrero (Gallegos-Mendiola et al., 2017). At San Juan de la Costa and Isla Gaviota in Bahía de La Paz, A. cf. carterae, C. malayensis, O. cf. lenticularis and Prorocentrum sp. were found associated with brown algae (Sepúlveda-Villarraga et al., 2017). In the waters of the Archipielago de Revillagigedo (Isla San Benedicto, Isla Socorro and Isla Roca Partida), state of Colima, about 720–970 km west of the continent, O. lenticularis was also reported in 2001–2017 (Gárate-Lizárraga et al., 2018).
The Pacific coast of Central America has only been sporadically explored for benthic dinoflagellates. Nevertheless, both toxigenic genera Gambierdiscus and Ostreopsis (not identified to species) were found among macroalgal samples from the Los Cóbanos reef zone along the west coast of El Salvador, whereas P. lima was the most abundant species (Quintanilla and Amaya, 2016). Analysis of samples taken in Costa Rica (2006–2011) at Cocos Island, 532 km southwest of the continental mainland, revealed A. carterae, C. tropicalis, C. cf. areolata, unidentified benthic Gambierdiscus (presumably two species), P. concavum and O. siamensis (Vargas-Montero et al., 2012). The first evidence of O. cf. ovata in the eastern tropical Pacific Ocean was provided from the Ecuadorian coast at Estero del Plátano (Esmeraldas Province) and Playa Mateo (Manabí Province) and multiple isolates have been successfully cultured from the region (Carnicer et al., 2016). Recently, a study from the Galapagos Marine Reserve and the Marine Reserve of Galera de San Francisco, in Ecuador, reported the genus Ostreopsis as a common inhabitant in both places, showing a great abundance and diversity of species in the Galapagos. They also reported members of the genera Gambierdiscus, Prorocentrum Ehrenb., Amphidinium Clap. & J. Lachm., and Coolia Meunier (Yépez-Rendón et al., 2018).
The paucity of information on toxigenic benthic dinoflagellates from the Chilean coast and the absence from the distributional map (Figure 1) may be derived from multiple but not mutually exclusive factors. First, the cold-water regime defined by the Humboldt Current influence may be less favorable to the development of bHABs. In consequence, the lack of bHAB events has reduced the relative urgency for research and monitoring of these species and their toxins in fisheries and aquaculture operations. This should not be interpreted to conclude, however, that these toxigenic benthic species are necessarily absent from the region.
Subtropical and Tropical Atlantic Ocean and the Caribbean Sea
In the Northern Hemisphere, the subtropical North Atlantic Ocean and the Caribbean Sea are not discontinuous in terms of water mass movement motility of benthic and epiphytic species and therefore cannot be reliably separated in terms of ecological niches and ecotypes of benthic dinoflagellates. For this reason, subtropical and tropical regions belonging to this biogeographical cluster are considered to include the Eastern Florida coast and Keys, Bahamas, Turks and Caicos above the Caribbean proper, the large Caribbean islands of the Greater Antilles (Cuba, Haiti/Dominican Republic, Puerto Rico, Jamaica) and a myriad of other islands belonging to the Lesser Antilles, such as Anguilla, Antigua and Barbuda, Aruba, Barbados, Curaçao, Guadalupe, Dominica, Martinique, Montserrat, and St. Lucia, etc., and island chains continuing toward the South American coast. The mainland coasts of Belize, Venezuela and Colombia are also linked to this system.
In the northern Caribbean from Cuba, Prorocentrum belizeanum, P. concavum and P. mexicanum were associated with nine macroalgal species collected at Bahía de Cienfuegos and at Jaimanitas Inlet, northeast of Havana (Delgado et al., 2002). Within the annual cycle from 2011 to 2012, in a shallow-sheltered reef lagoon offshore Bahía de Cienfuegos, the reportedly toxic and potentially toxic benthic dinoflagellates included Gambierdiscus caribaeus, Ostreopsis cf. lenticularis (dominant), P. hoffmannianum, P. lima and P. mexicanum (Moreira-González et al., 2016). Along the southern Cuban coast, a high diversity of Gambierdiscus species has been revealed by a molecular method (Díaz-Asencio et al., 2016), but the qPCR technique has not been widely applied in Latin America for comparison. The epiphytic dinoflagellate taxocoenosis was studied during two annual cycles (1999 to 2000 and 2001 to 2002) at Jaimanitas (Delgado et al., 2002; Delgado-Miranda, 2005); G. toxicus and P. lima were reported as the dominant species, but the former is a misidentification, according to Litaker et al. (2009, 2010). Coolia monotis, O. lenticularis and P. belizeanum were also found from Jaimanitas and the latter species is maintained in culture (Morquecho-Escamilla et al., 2016). An early publication on epibenthic dinoflagellates from Puerto Rico (Tosteson et al., 1986) identified two species: G. toxicus and O. lenticularis, collected at La Parguera on the southwest coast, but, evidently, G. toxicus was misidentified.
The first studies on ciguatera from waters around the Caribbean were conducted in the British and United States Virgin Islands (Coral Bay) of the Lesser Antilles (Carlson and Tindall, 1985). Both epibenthic and planktonic dinoflagellates were collected biweekly throughout a year; five dominant epibenthic species that may contribute to ciguatera were identified: Gambierdiscus sp. (referred to as G. toxicus), O. lenticularis, P. rhathymum, P. concavum, and P. lima. Coolia sp. (referred to as C. monotis) was reported among the dominant putatively non-toxic benthic species. Much later, G. caribaeus and G. belizeanus were collected and brought into culture from St. Tomas, United States Virgin Islands and Saint-Barthélemy Island (Litaker et al., 2009; Rains and Parsons, 2015; Boisnoir et al., 2019). Recently, based on morphometric and phylogenic analyses, two new toxic species, Coolia palmyrensis and C. santacroce were described from the northern coast of the Dominican Republic, and the United States Virgin Islands (Saint Croix), respectively (Karafas et al., 2015; Karafas and Tomas, 2015). The latter species was also later found further north in the Bahamas.
From the northeastern coast of Venezuela, near Tortuga Island, Mochima Bay, and the Gulf of Cariaco, epiphytic dinoflagellates associated with the seagrass Thalassia testudinum Banks ex König from 0.5 to 30 m water depth were found sporadically over two years (Valerio-González and Díaz-Ramos, 2008). Prorocentrum lima, P. rhathymum and P. compressum were reported as the most abundant putatively toxic species. In the Gulf of Cariaco (Turpialito Bay), epibenthic dinoflagellates were also sampled biweekly for six months from different substrates (T. testudinum, two brown algae, three green seaweeds and plastic bottles). Ostreopsis siamensis, P. rhathymum and P. lima were present at the highest abundances among nine species observed (Navarro-Vargas et al., 2014). Typically, planktonic species, P. minimum and P. micans, were also found, but they are not considered part of the epibenthic taxocoenosis, and in any case are presumably non-toxigenic. Several potentially toxic benthic species were found in the sand of two beaches of Margarita Island: Amphidinium carterae, O. ovata, O. siamensis, O. lenticularis and P. lima, among which P. lima, O. ovata and O. siamensis were the most abundant (Marchan-Álvarez et al., 2017).
Epibenthic dinoflagellate taxocoenosis studies from the seagrass T. testudinum along the Caribbean Sea coast of Colombia, from Chengue Bay and Chengue Lagoon, found O. cf. ovata, P. hoffmannianum, P. lima and P. rhathymum as the most common among 14 species in these two localities (Arbelaez et al., 2017). At San Andres Island, 12 species collected from drifting brown macroalgae and seagrass samples revealed P. emarginatum and P. belizeanum at the highest cell concentrations (Mancera-Pineda et al., 2014). In the Gulf of Morrosquillo, six dinoflagellate species were found attached to 26 macroalgal and seagrass species (Quintana-M and Mercado-Gómez, 2017). In general, with respect to the species composition and cell abundances of epibenthic dinoflagellates, the potentially toxic species, O. lenticularis, O. ovata and P. lima were the most abundant. The Gambierdiscus taxon found at San Andres Island was referred to as G. toxicus, as illustrated in two SEM micrographs in antapical view, but the taxonomic and biogeographic revision of the genus Gambierdiscus (Litaker et al., 2009, 2010) was not taken into account. The illustrated species may be G. belizeanus, judging from the shape of the 1p thecal plate and the cell width/depth ratio (Y.B. Okolodkov, pers. observation).
Intensive taxonomic observations of benthic dinoflagellate species from mangrove environments along the western Caribbean coast of Belize have led to the description of an array of new morphological species of Coolia, Gambierdiscus, Ostreopsis, and Prorocentrum (Faust, 1990b, 1993c,d, 1994, 1995, 1997, 1999; Faust and Morton, 1996; Faust et al., 2008; Litaker et al., 2009). Known species from Belize comprise: C. tropicalis (referred to as C. monotis); members of the Gambierdiscus group (Fukuyoa ruetzleri = G. ruetzleri, G. caribaeus, G. carpenteri, and including toxigenic G. belizeanus); Ostreopsis spp. of unknown toxicity or considered non-toxic in the region (O. labens, O. marina, O. belizeana, O. caribbeana); and Prorocentrum species (P. caribbaeum, P. elegans, P. foraminosum, P. formosum, P. norriseanum, P. reticulatum, P. ruetzlerianum, P. sabulosum, P. sculptile, P. tropicalis), with several known toxigenic species (P. arenarium, P. belizeanum, P. hoffmannianum, P. levis, P. maculosum). Cultures developed from the material collected at South Water Cay and Carrie Bow Cay were particularly useful in the description of the new species, G. caribaeus, G. carpenteri and F. ruetzleri (Litaker et al., 2009). Benthic dinoflagellate taxocoenosis in Belize has been studied from a variety of habitats including floating detritus, coral rubble, sand sediment, lagoon water, seaweeds and seagrasses from intertidal mangrove islands in the barrier reef ecosystem (Faust, 1993b, 1995, 2000, 2004; Faust and Morton, 1996; Morton and Faust, 1997; Faust and Gulledge, 2002; Faust et al., 2005). Ontogenetic, morphological and autoecological studies have focused mainly on toxic P. lima (Faust, 1990a, 1991, 1993a), Coolia sp. [referred to as C. monotis (Faust, 1992)], and the non-toxigenic species Bysmatrum subsalsum (Faust, 1996; Faust and Steidinger, 1998). Information on the problems in species identification, and details on the morphology, ecology and toxicity of benthic dinoflagellates from Belize is also included in the accompanying reviews (Faust et al., 1999; Faust and Gulledge, 2002).
The morphotaxonomy of epibenthic dinoflagellates from the Caribbean Sea coast of Mexico has been more thoroughly investigated than from other Caribbean areas, with the possible exception of Belizean reef ecosystems. Three key species linked to ciguatera fish poisoning (CFP), Gambierdiscus sp. (referred to as G. toxicus), G. belizeanus, and Fukuyoa yasumotoi have been identified from both epibenthos and net phytoplankton samples collected along the eastern Yucatán Peninsula coast, including the northern part of the Mesoamerican Reef System (Hernández-Becerril and Almazán Becerril, 2004). In addition to these potentially ciguateric species, descriptions and illustrations of four benthic Prorocentrum species have also been provided (Almazán-Becerril, 2000). Later, 24 epiphytic dinoflagellate species, including thirteen Prorocentrum species, were found from the same area (Almazán-Becerril et al., 2015; Irola-Sansores et al., 2018). A list of potentially toxic species from the northern portion of the Mesoamerican Reef System includes A. cf. carterae, C. cf. tropicalis, O. heptagona, O. siamensis and various Prorocentrum species, among which P. arenarium, P. belizeanum, P. concavum, P. emarginatum, P. hoffmannianum, P. lima, P. maculosum and P. rhathymum have been reported as toxigenic.
Gulf of Mexico
The Gulf of Mexico is linked hydrodynamically to the Caribbean Sea and adjacent waters and via the Florida Straits to the western Atlantic coasts of Florida and the Carolinas. The latter coasts are not technically part of Latin America but can be considered as a warm water sub-regional extension for investigating the biogeography and taxocoenosis of toxigenic benthic dinoflagellates.
The coastal waters of northern Yucatan, along with the Florida Keys, are the most studied in the Greater Caribbean Region with respect to benthic dinoflagellate biogeography. Most investigations focusing primarily on seasonal changes in benthic dinoflagellate taxocoenosis have been conducted along the southern Gulf of Mexico in the coastal waters of the Mexican states of Veracruz and Yucatan. In 2005, at two sampling sites in the Sistema Arrecifal Veracruzano National Park, 17 dinoflagellate species were found associated with 23 macroalgal and two seagrass species (Okolodkov et al., 2007). More recently, Amphidinium cf. carterae, Amphidinium cf. operculatum, Bysmatrum cf. caponii, Cabra cf. matta, Coolia cf. monotis, Ostreopsis sp., Prorocentrum cf. lima, and P. rhathymum were found in a reef zone at Chachalacas, Veracruz (Estrada-Vargas et al., 2017). In 2008–2009, seasonal changes were followed at ten sampling sites in the Chelem lagoon system and in the area exposed to the open sea at Dzilam de Bravo in the coast of Yucatan (Okolodkov et al., 2014). Prorocentrum lima and P. rhathymum were the most abundant species among 20 dinoflagellate taxa identified and associated with four seagrass and 33 macroalgal species. Gambierdiscus toxicus reported in Yucatan waters (Okolodkov et al., 2009) should be reconsidered as G. caribaeus, based on the description of the latter new species (Litaker et al., 2009), and known low toxicity (Litaker et al., 2017). A survey in 2011 of benthic dinoflagellates along the northern coast of the Yucatan Peninsula, within a wide range of habitats (exposed coast, semi-closed brackish water and hypersaline lagoons, fringe mangroves) from Celestún to El Cuyo found 26 dinoflagellate taxa associated with macroalgae, seagrasses, sponges and surface sediments (Aguilar-Trujillo et al., 2014). Changes in the benthic dinoflagellate taxocoenosis (composed of 25 species) were followed in August–December 2011, in the coastal waters of the northern Yucatan Peninsula (Dzilam de Bravo – San Crisanto area), before and after a pelagic bloom that lasted about 150 days (Aguilar-Trujillo, 2017; Aguilar-Trujillo et al., 2017). Prorocentrum rhathymum and P. cf. sipadanensis were co-dominant before the bloom, but only the latter remained dominant after the bloom. Along the northern Yucatan coast, unidentified species of Gambierdiscus, Ostreopsis and Prorocentrum have also been found (Barón-Campis et al., 2014). Monitoring of the seagrass Thalassia testudinum over an annual cycle (2012 to 2013) revealed 16 epiphytic dinoflagellate species, with P. lima and P. cf. sipadanensis as the most abundant (Martínez-Cruz et al., 2016).
The benthic habitat in the northwestern Gulf of Mexico is not considered to be suitable for optimal growth of benthic dinoflagellates–muddy bottoms characterize the region, but thousands of oil platforms and hundreds of artificial reefs along this basin provide substrates for these organisms to attach to and to reproduce. In 2007, members of the genus Gambierdiscus were reported as epiphytes on six oil platforms (Villareal et al., 2007). This study was of particular interest regarding how human activities can allow for the expansion of toxic species into places where they were not found previously, or where the natural substrate was not suitable for survival and growth. In the northern Gulf of Mexico, United States, a great diversity of species of the genus Gambierdiscus has been reported, including G. belizeanus, G. caribaeus, G. carolinianus, G. carpenteri and Gambierdiscus ribotype 2 (Tester et al., 2013). This study revealed that some Gambierdiscus species are able to grow throughout the year at the Flower Garden Banks National Marine Sanctuary located 185 km offshore of Galveston, Texas in the northwestern Gulf of Mexico.
Florida Straits, Keys and Southeastern Atlantic Coast of the United States
The annual cycle (1983 to 1984) of the benthic dinoflagellate taxocoenosis (<38 μm size-fraction) was studied at five locations in the Florida Keys: the Upper Matecumbe Key, Long Key, Knight Key, Bahia Honda Key, and Raccoon Key (Mitchell, 1985). Amphidinium klebsii, Coolia monotis and Prorocentrum lima were the dominant species found together with P. rhathymum, P. emarginatum, and Bysmatrum subsalsum; their autecology was studied in detail, with respect to seasonality, spatial heterogeneity and preference for macrophyte substrates among 15 macroalgal species and Thalassia testudinum. The seasonality of Gambierdiscus sp. (referred to as G. toxicus) was investigated from field sub-samples taken at Knight Key (Bomber et al., 1988); the influence of temperature, salinity and light on seasonality, growth rate and toxicity was determined from unialgal culture experiments under controlled laboratory conditions. The field samples collected at Knight Key in 1983 to 1984 (38–250 μm size-fraction) also served a study of substrate specificity and the nutrition of five benthic dinoflagellate species (Gambierdiscus sp. referred to as G. toxicus, Ostreopsis heptagona, O. siamensis, P. lima, P. rhathymum and P. concavum) from the seagrass T. testudinum and 14 macroalgal species (Bomber et al., 1989). Cultures developed from single-cell isolates from samples collected from Marathon Key were assigned to Gambierdiscus belizeanus, and isolates from Cape Fear, North Carolina were the basis for descriptions of the new species, G. carolinianus and F. ruetzleri (Litaker et al., 2009).
Southwestern Atlantic
Along the Brazilian coasts, Ostreopsis cf. ovata and Coolia malayensis were reported in the coastal waters of Ubatuba, east of São Paulo (Gómez et al., 2017b). A new toxic epiphytic species, Prorocentrum caipirignum, was described (Nascimento et al., 2017) from isolates collected from Rio de Janeiro (Arraial do Cabo) and Bahía (Garapuá, Ilha de Tinharé, Cairu). The lack of reports of toxigenic benthic dinoflagellates (and absence from Figure 1) from the Argentine coast further south, likely reflects the low relative cell abundance in colder waters influenced by the Malvinas Current. The limited or negligible impacts of toxigenic benthic dinoflagellates on aquaculture and fisheries activities should not be interpreted, however, to conclude that such species are absent.
Taxonomy and Biodiversity
A comprehensive worldwide guide for identifying marine benthic dinoflagellates includes 190 described species from 45 genera (Hoppenrath et al., 2014), which is less than a tenth of the known extant dinoflagellate species diversity (Taylor et al., 2008; Hoppenrath et al., 2009). Confirmed toxigenic dinoflagellates with well-defined toxin composition are all marine free-living obligate or facultative photoautotrophs, although many have mixotrophic capabilities. In contrast, among freshwater dinoflagellates, only a single putatively toxic species (Naiadinium polonicum (Wołosz.) Carty) has been described, and it is planktonic (Moestrup and Calado, 2018). Toxigenic dinoflagellates comprise more than 90 species (Moestrup and Calado, 2018), including 22 athecate members from the Gymnodiniales and Amphidiniales. There are at least 34 toxigenic benthic species and therefore in comparison to planktonic forms, toxic benthic and epiphytic species are heavily represented among free-living marine dinoflagellates. The known toxigenic marine benthic species are found frequently in Latin America (Table 1 and Figure 1), and often in high relative cell abundance, but toxigenicity and toxin composition are typically unconfirmed.
Morphological Taxonomy
The first described benthic dinoflagellate species (Amphidinium operculatum, Coolia monotis, Ostreopsis siamensis, and Prorocentrum lima) were characterized morphologically as early as the late 19th to beginning of the 20th century. A few more species were described throughout the 1940s and 1950s (e.g., Sinophysis microcephalus Nie and Wang, 1944, Roscoffia capitata Balech, 1956, Amphidinium carterae Hulburt 1957), but most benthic dinoflagellates have been described based upon morphological characteristics since the late 1970s. These species include well-known and widely distributed taxa such as Gambierdiscus toxicus, P. rhathymum, P. concavum, P. emarginatum, O. lenticularis, and O. heptagona and comprise all the major known toxigenic phenotypes. Originally, new species descriptions were based exclusively on the vegetative cell morphology and thecal plate tabulations, and this has continued during the first decade of the 21th century.
The vegetative cells of most benthic dinoflagellates are relatively large (>10 μm) and often heavily armored (but not always, e.g., athecate Amphidinium spp.) and thus preserve well for optical- and electron-microscopic analysis. It is perhaps surprising, therefore, that an expert dinoflagellate taxonomist can usually distinguish planktonic taxa at species level easier than benthic ones. This may be due to the unusually high degree of intraspecific morphological plasticity among populations of benthic dinoflagellate species. Furthermore, most cells of benthic dinoflagellates are bilaterally symmetrical and strongly dorso-ventrally compressed - a probable adaptation to their specific habitats (surfaces of seagrasses, macroalgae or invertebrates, narrow spaces between sand particles, etc). These morphological constraints create difficulties for detailed microscopic analysis of the diagnostic apical platelets of genera such as Prorocentrum Ehrenb. (in the periflagellar area), Amphidiniopsis Wołosz., Cabra Murray & Patterson emend. Chomerat, Couté & Nézan, Plagiodinium Faust & Balech, Planodinium Saunders & Dodge, Sinophysis Nie & Wang, Thecadinium Kof. & Skogsb., i.e., genera with reduced epitheca.
Until the past decade, identification of benthic dinoflagellates at species level has been substantially, if not exclusively, based on the theca morphology, in particular, on the plate arrangement (typically Kofoidean tabulation notation). According to Hoppenrath et al. (2013), asymmetry or symmetry of the theca, in combination with the shape of the periflagellar area, is constant within a species; however, the stability of pore patterns is not known to date. The high degree of morphological variability and intraspecific plasticity in details of the thecal structure and ornamentation highlight the difficulties in relying exclusively on these phenotypic characteristics for distinguishing benthic dinoflagellate species. Lately, high cryptic diversity has been revealed for some previously considered “good” species, such as P. lima, O. ovata or C. monotis, presently referred to as species complexes (Leaw et al., 2010; Zhang et al., 2015; Accoroni and Totti, 2016). Problems in the strict application of the morphospecies concept, particularly for desmokont dinoflagellates, are well illustrated by the uncertainty in species boundaries of potentially toxic species such as P. hoffmannianum, P. belizeanum, P. maculosum and P. faustiae (Hoppenrath et al., 2013). Patterns of the periflagellar platelets seem to be species-specific for most benthic Prorocentrum species, as shown in Hoppenrath et al. (2013). Nevertheless, confirmed identification of P. lima, one of the most common toxigenic epibenthic species of the genus and widely distributed in both temperate and tropical regions, is particularly problematic. The morphospecies P. lima comprises high cryptic diversity; thus, referring to it as the “Prorocentrum lima complex” is recommended (Aligizaki, 2009). Our morphological observations of recent isolates from the Caribbean and Gulf of Mexico coast of 23 monoclonal cultures of the Prorocentrum lima complex under an epifluorescence microscope showed much greater variability in cell shape than expected; some cells could be easily (if not incorrectly) identified as another Prorocentrum species but the thecal pore pattern remained stable. The fact that most species of benthic dinoflagellates belong to Prorocentrum also contributes to the above-mentioned methodological difficulties in morphologically discriminating between benthic and planktonic thecate species.
The problem of distinguishing between P. rhathymum and P. mexicanum (both reported as toxic) still remains. Although they are usually regarded as distinct morphospecies, P. rhathymum is sometimes considered a junior synonym to P. mexicanum. In addition to subtle morphological differences, the former species is epibenthic whereas the latter is typically planktonic (Cortés-Altamirano and Sierra-Beltrán, 2003). If this habitat distinction is valid, most, if not all, records of P. mexicanum in benthic habitats are likely misidentifications. Species boundaries between P. emarginatum and P. sculptile and between P. faustiae and P. concavum (all reported as toxigenic except for P. sculptile) are not clear, and more investigations are required to compare specimens from natural populations and cultured isolates of these taxa (Hoppenrath et al., 2014; Lassus et al., 2016).
Ostreopsis species are in general difficult to distinguish because they have the same thecal plate tabulation (Kofoidean formula) and that is similar to the genus Coolia; within Ostreopsis there may be only slight differences in cell shape and sometimes in the presence of only one thecal pore type (as in most species) or two types (as in O. lenticularis). Identification at species level is particularly problematic due to imprecision in the original descriptions and confusion in subsequent interpretations (Hoppenrath et al., 2014). The first apical (1’) plate is the most critical for identification. Probably, only O. heptagona has the plate features that would allow a specialist to identify it unequivocally - a long 2’ plate that has a wide contact with the 3’ plate on the epitheca, and a very characteristic 1p plate that narrows from the sulcus toward the center of the hypotheca.
After re-investigation of the original description of Coolia species (Hoppenrath et al., 2014), the species are mainly distinguished on the basis of small differences in the shape and size of the 1’ and 7’ plates on the epitheca, in combination with the presence or absence of a suture between the 2”’ and 2”” plates on the hypotheca.
Gambierdiscus toxicus, the type species of the genus, has been re-described (Litaker et al., 2009), and subsequently, the following morphological features have been used to distinguish between species: shape (narrow or broad) and size of the 1p plate on the hypotheca; a smooth or areolated thecal surface; and the shape of 2’ plate, which can be quantified by the ratio of the 2’/2” suture length to the 2’/4” suture length (Hoppenrath et al., 2014). Within the morphologically related genus Fukuyoa F. Gómez, D. Qiu, R.M. Lopes & S. Lin, the species are mainly distinguished by the cell size and shape (globular or laterally flattened, unlike anterior-posteriorly compressed Gambierdiscus sensu stricto), the proportions between the cell length, width and depth, the shape of the 2”” plate (Litaker et al., 2009), and the shape of the 1’ plate (Gómez et al., 2015).
Amphidinium is an athecate genus, with poorly defined morphological characteristics. The genus is found in a wide variety of marine habitats including benthic sandy and epiphytic substrates, and within the water column. Vegetative cells are generally dorsoventrally compressed with a minute, triangular or crescent-shaped, usually left-deflected epicone. The type species of the genus, A. operculatum, was re-described, and the genus was redefined; based on morphological and molecular phylogenetic analyses, six distinct species were identified (Jørgensen et al., 2004; Murray et al., 2004). Furthermore, the genus Prosoaulax Calado & Moestrup was erected for some freshwater species previously ascribed to Amphidinium (Calado and Moestrup, 2005). Among toxigenic benthic Amphidinium species, the two most frequently reported, A. carterae and A. cf. operculatum are distinguished mainly by the cell size and shape of the epicone. Furthermore, some authors consider that A. operculatum and A. klebsii are synonyms (Dodge, 1982); Karafas et al. (2017); however, recommended elimination of A. klebsii as an accepted taxonomic entity. Examination of live Amphidinium cells is always preferable; otherwise, for morphological study, Lugol’s iodine-fixed cells are better than formalin- or ethanol-fixed ones. The number and the shape of chloroplasts and the position of the ring-shaped starch-sheathed pyrenoid can be additional useful features to identify species and to distinguish among other Amphidinium species (Karafas et al., 2017).
Molecular Genetic Approaches to Taxonomy and Phylogenetic Reconstruction
The advent of molecular technologies, particularly metagenomics and targeted gene sequencing, has revolutionized our understanding of biodiversity of microeukaryotes in marine ecosystems, revealing cryptic species and permitting definition of biogeographical distributional patterns of species occurrence. Within the last decade, morphological descriptors are frequently supplemented by molecular methods applied to sequencing key genes (rDNA, ITS) for taxonomic and phylogenetic reconstruction for benthic dinoflagellates (e.g., Litaker et al., 2009). Among toxigenic HAB taxa, molecular approaches have been essential in establishing the structure and function of toxin biosynthetic genes and the mechanisms whereby gene expression is regulated. As of yet, there are no diagnostic gene probes for the polyketide synthase (PKS) genes presumably responsible for the biosynthesis of the array of polyether toxins produced among various benthic dinoflagellates.
Application of ecogenomics and phylogenetic reconstruction approaches to bHAB taxa (almost all dinoflagellates) has been rather sporadic and often geographically limited, with very little information available for toxigenic benthic dinoflagellates from Latin America. Nevertheless, a few key studies have addressed this issue from a global perspective, but which involve populations from the Americas. Molecular evidence supports the contention that both P. belizeanum and P. hoffmannianum belong to the P. hoffmannianum species complex in which clades are separated by geographical region. Thus, four ribotypes can be distinguished from diverse geographical locations: from Belize, Florida-Cuba, India and Australia (Herrera-Sepúlveda et al., 2015). Similarly, application of molecular tools to the genus Gambierdiscus, originally believed to be monotypic, has resolved several cryptic taxa within this genus into distinct species, and is adding to an ever increasing list of new descriptions (Larsson et al., 2018). Recently, combined morphological and molecular genetic analysis was also successful in separating a new genus, Fukuyoa, with putative toxigenicity, and similar to but distinct from Gambierdiscus (Gómez et al., 2015).
Establishing the link between toxigenicity and phylogenetic relatedness among benthic dinoflagellates continues to be hampered by the lack of targeted gene probes for toxin biosynthetic genes and limited analysis of toxin spectra from natural populations and cultured isolates. Nevertheless, there are ongoing attempts to define patterns of toxigenicity and taxonomic affiliations. Currently, three genetic clades of Ostreopsis cf. ovata have been shown to occur in overlapping geographical areas (Penna et al., 2005), and several different toxicity patterns have been described (Carnicer et al., 2016). In this investigation, thirteen isolates from the coast of Ecuador in the eastern Pacific Ocean, including a geographical area from which no information existed, were analyzed for Ostreopsis genotypes and toxicity of the O. cf. ovata complex. By analyzing the ITS and 5.8S rDNA, the isolates were found to be identical and to cluster within the Atlantic/Indian/Pacific clade.
Beyond the contribution to resolution of taxonomic issues and cryptic species, functional genomic approaches will play an increasing role in tracking shifts in gene frequency of HAB species over seasonal and multi-annual cycles. Although such work has already been initiated for planktonic HAB species at the population level, e.g., for diversity of Alexandrium minutum along the coast of France (Le Gac et al., 2016), to our knowledge there are no published corresponding studies for bHAB species within tropical or subtropical ecosystems in Latin America. Such studies are critical to understanding the population dynamics of bHABs and associated toxin distribution because the ecological niche definitions and contributions to community structure are expected to be rather different for benthic species compared with their planktonic counterparts.
Determination of Toxin Composition, Occurrence and Associated Syndromes
Most Latin American countries have developed and implemented some capability for monitoring potentially toxic phytoplankton blooms and/or the presence of their toxins above regulatory limits in production and harvest zones for mollusks for human consumption (Secretaría de Salud, 2015). Phycotoxin monitoring programs in Latin America have tended to focus on quantitative assay or analysis of hydrophilic toxins in shellfish, primarily the paralytic shellfish toxins (PSTs) (or saxitoxins) and domoic acid, associated with planktonic blooms of dinoflagellates (Alexandrium spp., Gymnodinium catenatum, Pyrodinium bahamense) or chain-forming diatoms (Pseudo-nitzschia spp.), respectively. In Latin America, somewhat less monitoring attention has been directed to the lipophilic phycotoxins from planktonic dinoflagellate blooms, such as the diarrhetic shellfish toxins (DSTs; okadaic acid/dinophysistoxins) from Dinophysis spp., and brevetoxins from Karenia brevis, mainly in the Gulf of Mexico. With respect to the toxins generated by bHABs and the associated benthic dinoflagellate species, there are no comprehensive monitoring programs implemented within Latin America.
Benthic dinoflagellates are capable of synthesizing a wide array of bioactive molecules, particularly cyclic polyether compounds, often considered as “toxins” because of their potent effects in mammalian systems and/or cytotoxicity. These toxins are, in general, lipophilic compounds, and thus highly soluble in organic solvents, but their polarities are extremely variable (Blanco et al., 2005), and among analogs they differ markedly in specific toxicity and species occurrence (Figures 2–5 and Table 2).
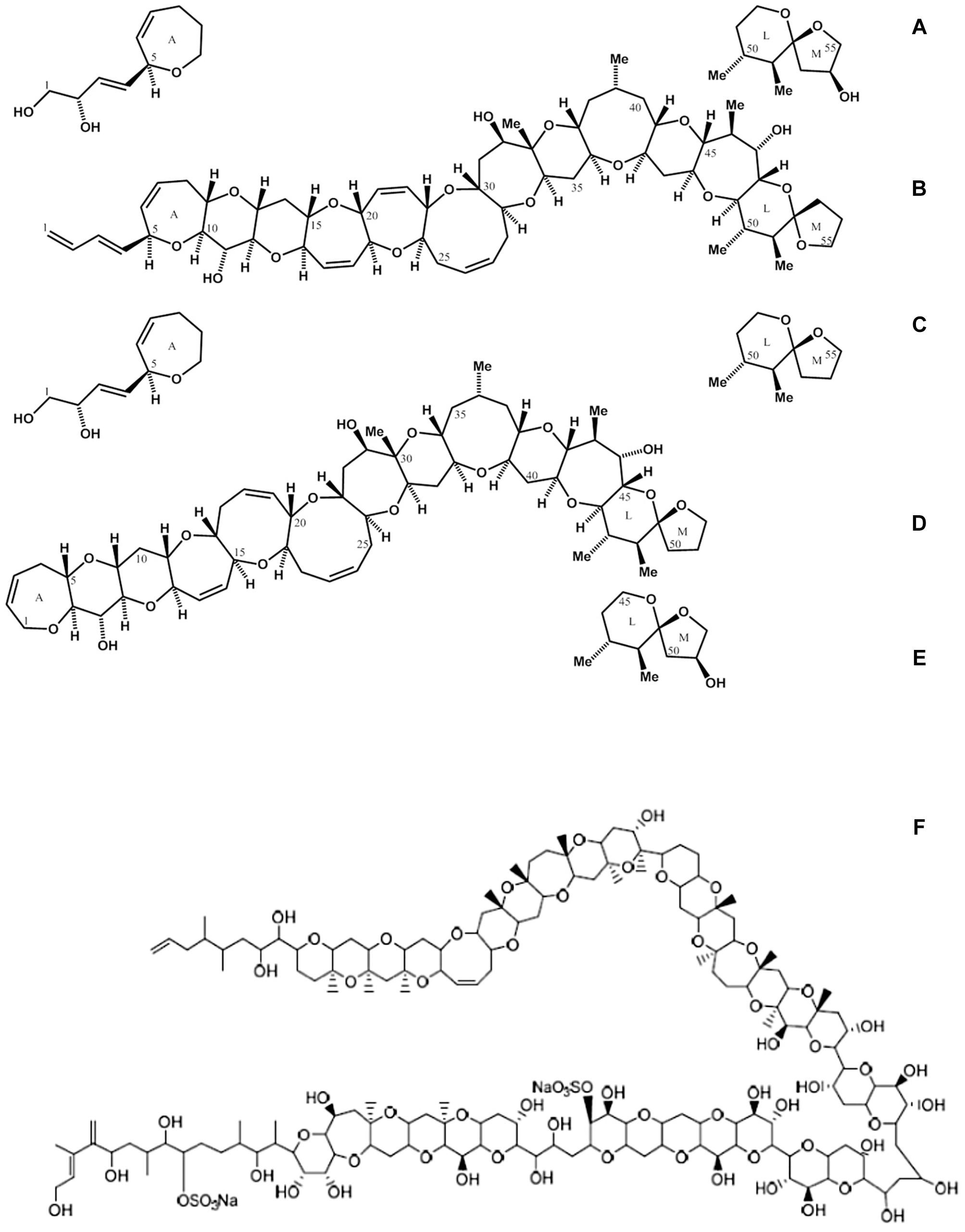
Figure 2. Some toxins associated with ciguatera fish poisoning (CPF) and originating from members of the genus Gambierdiscus and/or Fukuyoa: (A) CTX1B; (B) CTX4A; (C) epideoxyCTX1B; (D) CTX3C; (E) 51OH-CTX3C; (F) MTX. Structures modified from Ajani et al. (2017) and Kato and Yasumoto (2017).
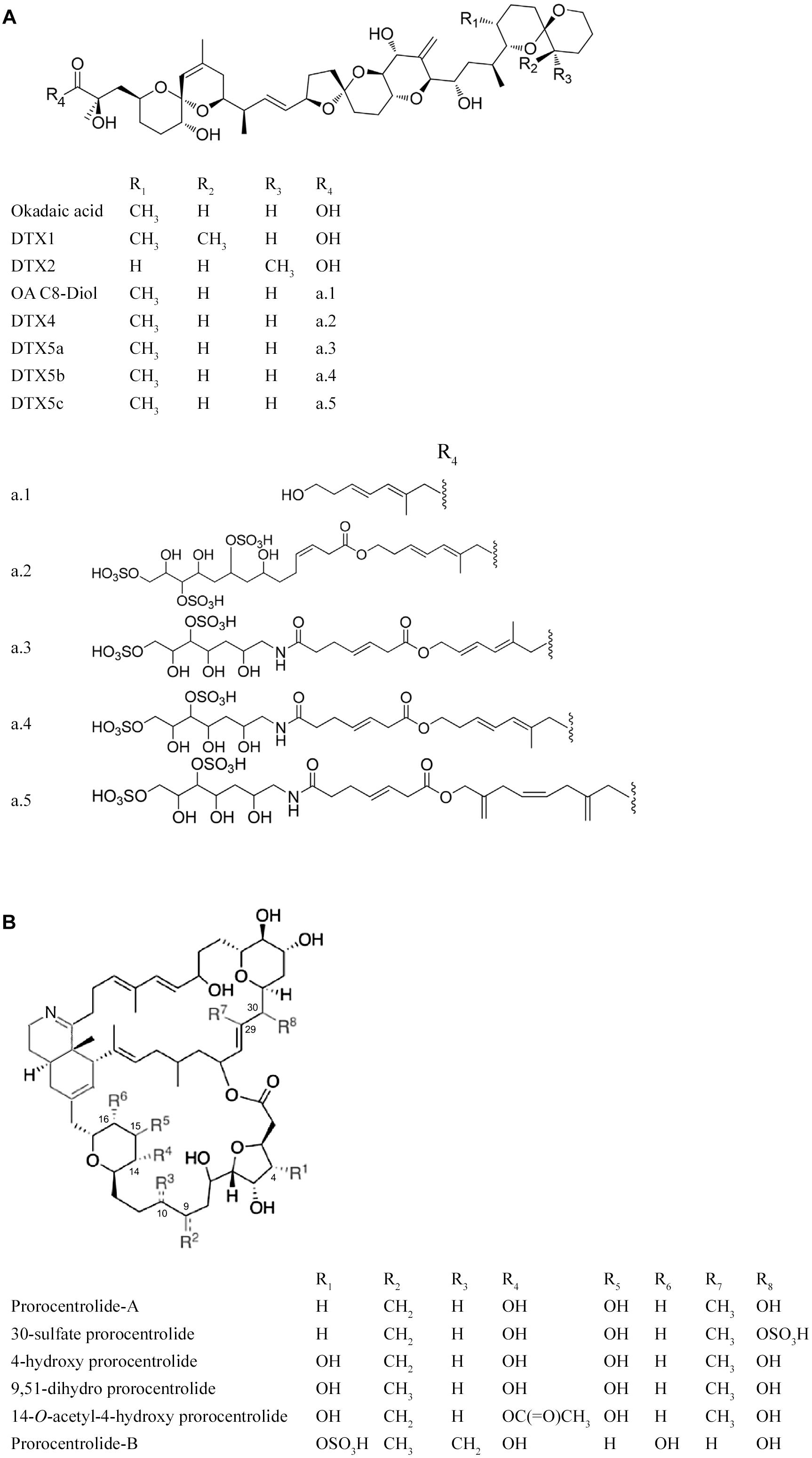
Figure 3. (A) Okadaic acid (OA) and dinophysistoxins (DTXs) produced by benthic members of the genus Prorocentrum (Twiner et al., 2016); a.1 to a.5 C8-diol ester and sulfated diesters of OA. (B) Prorocentrolides and analogs reported to date. Modified from Amar et al. (2018).
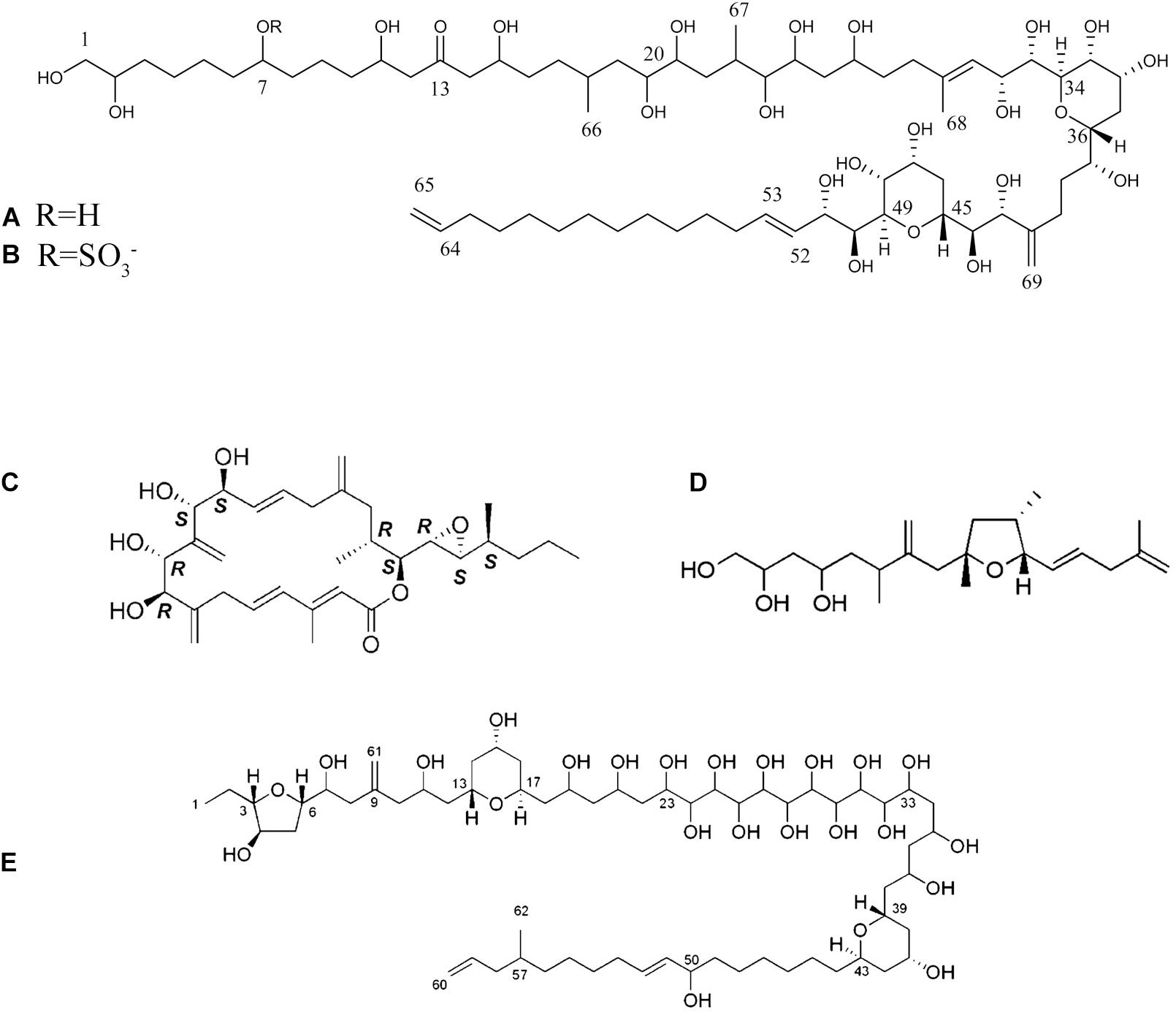
Figure 4. (A,B) Amphidinols A and B; (C) Amphidinolide A; (D). Amphidinin; (E) Amphezonol (Kobayashi and Kubota, 2007; Cutignano et al., 2017). Reprinted with permission from Kobayashi and Kubota (2007).
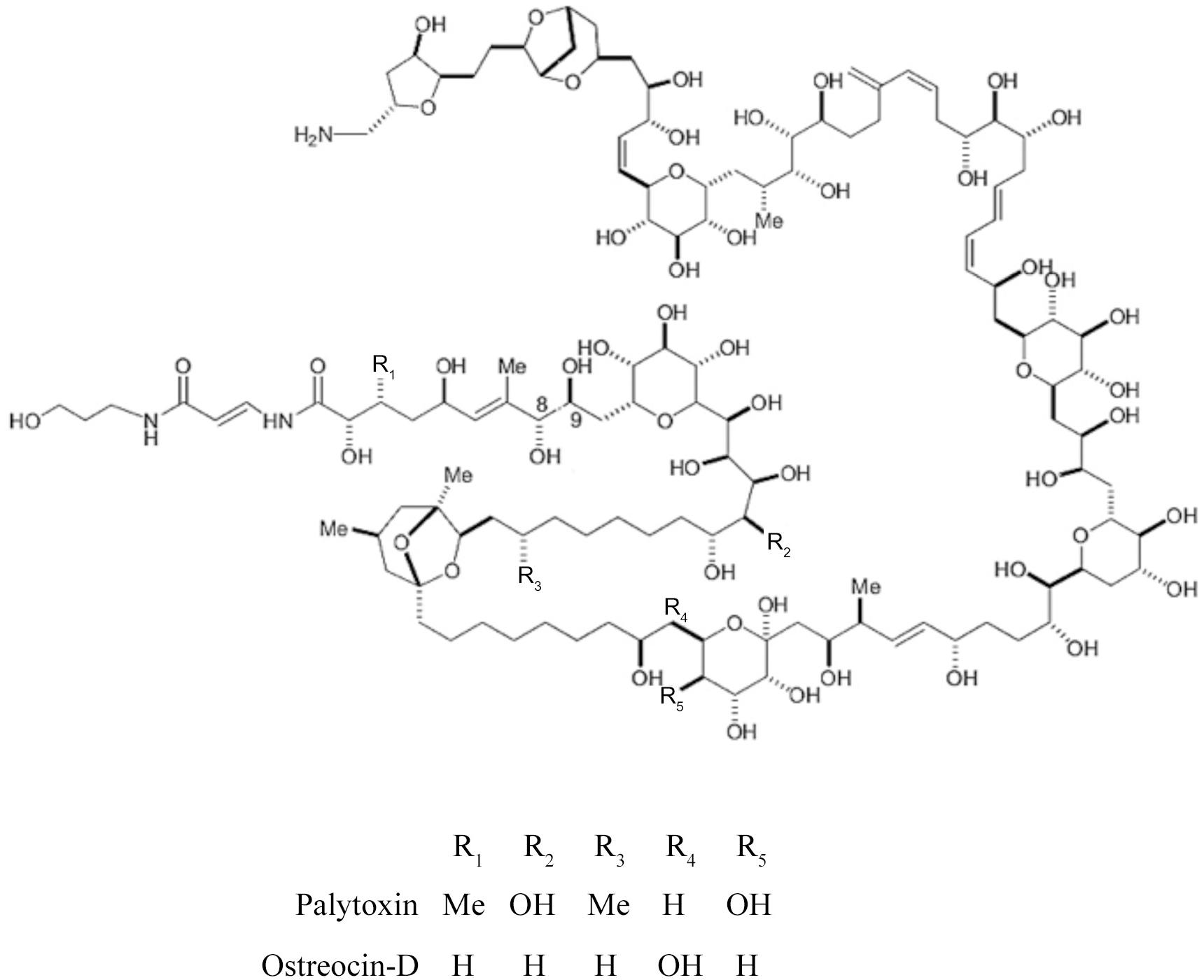
Figure 5. Structures of palytoxin (PLTX) and ostreocin-D, produced by certain benthic members of the genus Ostreopsis (Amzil et al., 2012).
Ciguatoxins (CTXs) (Figures 2A–E) have been directly implicated in CFP (Lehane, 2000), but early studies also presumed that maitotoxins (MTXs) (Figure 2F) were involved in the diverse CFP syndrome (Yasumoto and Murata, 1993). Ciguatoxins are known to act on site 5 of the voltage-gated sodium channels (NaV) with great affinity, affecting normal cellular function through the elevation of cytoplasmic Na+ and Ca2+ concentrations. Other biological activities have also been reported, such as the blockage of voltage-gated potassium channels (KV), activation of L-type Ca2+ channels, and accumulation of nitric oxide in cells (Kumar-Roiné et al., 2008).
Maitotoxins (Figure 2F) are water-soluble polyethers, structurally similar to CTXs but more hydrophilic due to numerous hydroxyl and sulfate groups. When injected intraperitoneally into mice, MTX is among the most potent known natural toxins (Estacion, 2000), with toxicity even higher than for CTX, but less potent when administered orally (Wang, 2008; Kohli et al., 2014). The role of MTX analogs in CFP has not been clearly established, although accumulation in carnivorous fish tissues such as viscera, liver and muscle has been confirmed (Kohli et al., 2014). Recent toxicological studies have shown that MTXs are probably not a significant factor in CFP because of their low oral potency and higher water solubility (compared to CTXs).
Circumstantial association of CFP with toxigenic benthic dinoflagellates has led to the historical view that the toxin syndrome represents a synergistic effect of a complex suite of diverse metabolites produced by various benthic dinoflagellates (primarily Gambierdiscus, Amphidinium, Coolia, Ostreopsis, and Prorocentrum species). Gambierdiscus species are still considered the ultimate origin of most toxins involved in CFP, but structural elucidation of many CFP toxins has traditionally been conducted on tissue extracts of fish and other marine fauna, rather than directly from the putative toxigenic dinoflagellates. With the advent of advanced mass spectrometric and NMR approaches, it is now confirmed that members of the genus Gambierdiscus (and closely related Fukuyoa) produce multiple analogs of toxins such as CTXs and MTXs, known to be associated with CFP, whereas the other implicated genera do not. For some years, the genus Gambierdiscus was thought to be monotypic, but increased interest and the use of molecular tools for taxonomic identification have shown that this genus comprises multiple species, and an increasing list of new taxa (Larsson et al., 2018). A new related toxigenic genus, Fukuyoa, has also been described (Gómez et al., 2015) but few strains of this genus have been established in culture, and hence there is little autoecological information, and only scarce knowledge on natural distribution and toxin production. Research on F. ruetzleri from Belize, North Carolina and the United States coast of the Gulf of Mexico found CTX and CTX-like compounds (Holland et al., 2013; Lewis et al., 2016; Litaker et al., 2017; Pisapia et al., 2017). A study on F. paulensis from Australia, detected no CTX or CTX-related bioactivity, but a putative MTX analog, known as MTX-3 was found (Larsson et al., 2018), though this species has not been identified in Latin America.
Diarrhetic shellfish poisoning (DSP) is a human intoxication syndrome caused by consumption of shellfish contaminated with okadaic acid (OA) and/or structurally related dinophysistoxins (DTXs) (Figure 3A). The OA toxin group comprises lipophilic polyether toxins that can accumulate in suspension-feeding shellfish such as oysters, mussels, scallops, cockles and clams. Some known analogs, such as DTX3, are considered almost non-toxic in their natural form (Braga et al., 2016), but could be hydrolyzed after ingestion and thereby converted into more potent compounds, such as OA, DTX1 and DTX2 (Doucet et al., 2007). These toxins are potent inhibitors of the serine/threonine phosphatases PP1, PP2A, and PP2B (Bialojan and Takai, 1988; Hu et al., 2017). Since diarrheic episodes have not been explicitly confirmed as linked with PP inhibition, other modes of action could be involved in the intoxication process, probably related to a neuropeptide that protects against diarrhea by the inhibition of intestinal mobility and water and electrolyte secretion (Louzao et al., 2015).
On a global basis, production of DSP toxins and associated HAB events are usually associated with members of the phytoplankton community belonging to the genus Dinophysis, but these toxins are also produced by several species of benthic dinoflagellates of the genus Prorocentrum (Valdiglesias et al., 2013). Benthic Prorocentrum species known to produce OA and toxic DTX analogs include P. borbonicum, P. caipirignum, P. cassubicum, P. concavum, P. cordatum, P. emarginatum, P. faustiae, P. hoffmannianum, P. lima, P. maculosum, P. mexicanum, and P. texanum (Moestrup et al., 2009). Even though many of these toxigenic species have been reported from Latin American coasts (Tables 1, 2), except for earlier work in Cuba (Delgado et al., 2002, 2005) and Mexico (Heredia-Tapia et al., 2002), few studies have been conducted to corroborate their toxicity in the region.
To our knowledge, there are no confirmed records of DSP caused by toxins associated with benthic dinoflagellates in Latin America, although there is mention of one case which could be attributable to such toxins (Heredia-Tapia et al., 2002). In general, there are no reliable data of incidence or etiology of DSP episodes in the region (Acuña, 2015). In most cases of putative DSP, affected people are not attended to by medical services or, even when this is the case, because the major DSP symptoms include diarrhea, abdominal pain, gastrointestinal distress and sometimes fever (James et al., 2010), they are easily confused with other food-borne gastrointestinal syndromes.
Bioactive cyclic imine compounds, known as prorocentrolides, may be produced by P. lima (Figure 3B), but the human health risk has not been established. Members of this family of macrocyclic imines are known as “fast-acting toxins” due to the rapid onset of neurological symptoms, followed by paralysis and death after intraperitoneal injection into mice (Amar et al., 2018). At least one benthic dinoflagellate species found in Latin America, P. caipirignum, is capable of producing both OA and prorocentrolides (Nascimento et al., 2017). Recent studies including competition-binding assays, electrophysiological techniques and in silico simulations have been performed with prorocentrolide-A to determine potency and biological activity (Amar et al., 2018). Such cyclic imines have been reported to be antagonists of muscle and neuronal types of nicotinic acetylcholine receptors (nAChRs) (Molgó et al., 2017), but their exact mode of action remains unknown.
Cytotoxic macrolides produced by members of the primarily benthic dinoflagellate genus Amphidinium, and hence called amphidinolides (Figures 4A–E), have shown hemolytic activity (Yasumoto et al., 1987), as well as evidence of cytotoxicity against cancer cell lines, and antifungal properties (Kobayashi et al., 1991; Bauer et al., 1995a,b; Kobayashi et al., 2002; Tsuda et al., 2007; Espiritu et al., 2017). Within the last decade, interest in the toxigenicity of this genus has increased because some species, namely the type species A. carterae Hulburt 1957 and members of the A. operculatum Claparède & Lachmann, 1859 species complex, are known to produce ichthyotoxic substances (Kobayashi et al., 1991; Satake et al., 1991; Tsuda et al., 1994; Bauer et al., 1995a,b). In most cases, bioactivity (and in higher doses toxicity) is assumed to be related to various polyketides and macrolides isolated from different species of the genus (Kobayashi et al., 1991; Bauer et al., 1995a,b; Kobayashi et al., 2002; Tsuda et al., 2007; Espiritu et al., 2017). Nevertheless, many of the pioneering studies lacked sophisticated chemical analysis for structural elucidation and toxin potency confirmation was often conducted only on semi-purified material.
No human illnesses or marine faunal mortalities have been directly linked to bHABs of Amphidinium or via toxins transferred in marine food webs (Holmes et al., 2014), but some fish kills have been circumstantially attributed to Amphidinium. If confirmed, such cases are probably due to amphidinol and its analogs, because they are structurally similar to karlotoxins, known ichthyotoxins produced by planktonic Karlodinium species (Place et al., 2012). In any case, to date there are no published data on the toxin composition or associated ichthyotoxicity of cultured isolates or natural populations of Amphidinium species found in Latin America.
Members of the genus Coolia are known to produce bioactive secondary metabolites (and putative toxins), but there is still confusion about both their toxigenic potency and taxonomic affinities within the genus. The genus is undergoing rapid taxonomic revisions, and two new toxic species have been recently added to the list: C. palmyrensis and C. santacroce (Karafas et al., 2015; Karafas and Tomas, 2015); it is also likely that certain Amphidinium species have been previously misidentified as C. monotis and C. malayensis. According to the IOC-UNESCO Taxonomic Reference List of Harmful Micro Algae (Moestrup et al., 2009), C. tropicalis is the only confirmed toxin producing species among of the five Amphidinium species on the list (C. monotis, C. tropicalis, C. areolata, C. canariensis, and C. malayensis). Most members of the genus are apparently non-toxigenic, although in any early report (Holmes et al., 1995) based on a mass spectrometric analysis, C. monotis was found to produce cooliatoxin, a probable monosulfated analog of yessotoxin. Extracts and supernatants of cultured C. monotis have showed hemolytic activity, toxicity to mice, and sodium channel activity (Rhodes and Thomas, 1997), but the specific compounds responsible for such bioactivity have not been structurally elucidated. Until now, no human intoxications caused by metabolites produced by members of the genus Coolia have been reported, and many studies have failed to detect toxicity in any species (Holmes et al., 2014). There is no published research on bioactive metabolites (including toxins) produced by this genus in Latin America.
Palytoxins (PLTXs) are complex polyhydroxylated polyketides with both lipophilic and hydrophilic moieties (Figure 5; Ramos and Vasconcelos, 2010); PLTX is considered to be among the most potent non-proteinaceous toxins, second only to MTX with respect to intraperitoneal toxicity in mice. Recently, members of the genus Ostreopsis have been found to produce PLTX, and almost 20 analogs are known to date (Ajani et al., 2017). Four structural sub-groups of these toxins have been defined: palytoxins, ostreocins, ovatoxins and mascarenotoxins (Tubaro et al., 2012), but toxigenicity of most individual analogs remains undetermined. Blooms of Ostreopsis are now well known for causing respiratory distress in humans in coastal areas, particularly in southern Europe and the Mediterranean Sea, because of toxic aerosol formation, but the problem has not yet become evident in Latin America. Isolates of the O. cf. ovata complex from the coast of Ecuador were judged to be non-toxic according to hemolytic cell assays, and the absence of PLTX-like compounds was confirmed by liquid chromatography-mass spectrometry (LC-MS/MS) (Carnicer et al., 2016). Whether lack of human toxic events associated with Ostreopsis in Latin America is primarily due to the relatively low magnitude of the blooms, hydrodynamic factors associated with aerosol formation, lack of human exposure in bHABs affected areas or to the non-toxigenicity of local populations remains to be established.
Studies on benthic dinoflagellates in Latin America have traditionally relied on microscopic taxonomic analysis of samples collected from benthic or epiphytic substrates; if species known to be toxic elsewhere are detected and morphologically confirmed, local populations are often assumed to be toxigenic as well. Such populations are then considered to be the causative agent of toxic events associated with bHAB toxins found nearby, e.g., in reef fish associated with ciguatera fish poisoning (CFP) even without proving toxicity of the local strains (Delgado-Miranda, 2005). Ciguatera chemistry in Latin America is focused mostly on public safety, e.g., fish tissues are analyzed or assayed by governmental laboratories. Toxin chemistry research is scarce, and most scientific data are presented only at local or national conferences (Barón-Campis et al., 2014; Ley-Martinez et al., 2014; Núñez-Vázquez et al., 2018).
The necessary methods for the determination of toxin composition, most notably LC-MS/MS, are not widely applied for regulatory toxin surveillance in seafood or in research programs within Latin America. Many known toxins have not yet been confirmed from benthic dinoflagellates or food web compartments and seafood from the region. In some cases, such as for the CTXs, neither certified analytical standards nor reliable calibration reference materials are available for these assays and analyses (Dechraoui-Bottein and Clausing, 2017). Nevertheless, several Latin American countries, namely Brazil, Chile, Argentina and Mexico among others, have recently established LC-MS/MS facilities for phycotoxin analysis, including certain lipophilic analogs of highest relevance for toxigenic benthic dinoflagellates and contaminated seafood. These are now being applied to both research questions and toxin monitoring activities on a limited basis.
In most of Latin America, the conventional method for quick decisions on fisheries and aquaculture closures for public health protection remains the mouse bioassay (MBA). Variations of the standardized AOAC MBA for hydrophilic and lipophilic toxins, respectively, are widely applied in laboratories for seafood quality control throughout Latin America. In certain countries, the MBA has been largely replaced by chemical analytical methods for hydrophilic toxins, in particular by liquid chromatography coupled to ultraviolet detection [LC-UV] for domoic acid, and by LC coupled with fluorescence detection (LC-FD) for PSTs. Alternative diagnostic techniques, such as the phosphatase inhibition assay for DSTs or immunoassays for PSTs and domoic acid are often available, but they are typically applied for research or limited toxin screening purposes and are not common in regulatory protocols (Ministerio de Salud, 2008; NOM-242-SSA1, 2009; Secretaría de Salud, 2015; Hardison et al., 2016; Lewis et al., 2016). The MBA allows for rapid results to decide on closures for shellfish and finfish harvesting but has a high detection limit and is rather imprecise regarding toxicity.
Biochemical and functional biological assay methods are primarily useful for semi-quantitative screening for specific toxin groups. For example, in vitro biological assays such as the neuroblastoma (N2A) assay, and the radioligand/fluorescent receptor-binding assay (RBA) have been successfully applied for detection of certain lipophilic neurotoxins. The latter technique has been developed for screening the NaV site 5 specific neurotoxins, including the brevetoxins (PbTxs) and CTXs (Dechraoui-Bottein and Clausing, 2017). In Cuba, an optimized receptor binding assay (RBA) has been used to detect CTXs (Díaz-Asencio et al., 2016), and the protocol supports the validation of the assay. This will allow the implementation of a monitoring program for CTXs in this country, but to date such technological approaches have not been widely deployed in Latin America (Cuellar-Martinez et al., 2018). In any case, in addition to the drawback of the lack of certified reference materials for many toxin analogs, none of these assay methods are adequate for full qualitative and quantitative determination of lipophilic bHAB toxin analogs in either plankton or seafood matrices.
Among the phycotoxin syndromes associated with benthic dinoflagellates in Latin America, human illnesses linked to CFP are clearly the most acute and socio-economically relevant, but little etiological information is available on a regional scale. Within Mexico, 25 relatively well documented cases of CFP have been reported between 1979 and 2014 (Cuellar-Martinez et al., 2018), and CTX has been found in tissues of several fish species in the Caribbean (Ley-Martinez et al., 2014). Ciguateric areas and fishes are well-known by inhabitants of Caribbean coasts, in particular, through experience from historical and recent cases of CFP. Ciguatoxins are accumulated through the food webs in many tropical marine ecosystems, but to date little research has been conducted on the kinetics and mechanisms of toxin transfer from benthic dinoflagellates to carnivorous fish in Latin America and metabolism and toxin composition in various ecological compartments remains poorly understood. Registration of human cases and confirmed CFP intoxications is inconsistent in the region; in the Caribbean it has been estimated that almost half of the cases are not reported to medical facilities (Olsen et al., 1984), but public health surveillance and awareness are gradually improving.
In the absence of reliable diagnostic procedures for toxin detection, in many cases, fish that are known to be potentially toxic based upon historical medical or anecdotal data are simply avoided or banned for human consumption, such as the barracuda (Sphyraena spp.) in the Mexican Caribbean state of Quintana Roo (Olsen et al., 1984; García-Mendoza et al., 2016). Traditionally, fish are sold directly to the consumers by fisherman, or through cooperatives and fish markets; fish purchase is considered a hazardous activity, such that even hotels and restaurants in the Virgin Islands have refused to serve local fish (Olsen et al., 1984).
A key study carried out in Cuba on three coastal fishing communities showed a circumstantial link between macroalgal biofouling enhanced by thermal pollution that caused coral bleaching, and ciguatera cases (Morrison et al., 2008). More importantly, the social–ecological resilience of the community was demonstrated by linking through the local fishermen organizations via communication related to ciguatera and ciguatoxic fish in the fishery areas, and the associated CFP cases. In a well-organized fishing community, where this information was provided and shared, the number of CFP cases remained low, whereas in a larger non-organized community, where the relevant information scarcely reached the fishermen, intoxication cases increased during the same time period. This highlights the critical importance of local education, networking among seafood producers, regulatory authorities and public health personnel, and information exchange in ciguateric areas.
Current and Future Perspectives on Benthic Harmful Algal Blooms and Global Change
On a global scale, HABs and their environmental and human health consequences appear to be more frequent, intense and widespread within the last several decades (Hallegraeff, 2010). Toxic incidents caused by polyether phycotoxins are increasing worldwide, with an apparent expansion in the distributional range of toxin-producing benthic dinoflagellates, perhaps driven by climatic shifts and anthropogenic factors. Current assumptions are that this apparent increase is primarily related to global climate change, anthropogenic impacts in the coastal zones and enhanced public and scientific awareness via improved toxin and bloom surveillance programs (Accoroni et al., 2011). Evidence for the “global spreading hypothesis” has been acquired primarily for planktonic HABs, as they have historically received more research attention than bHABs, which tend to be cryptic and less spectacular. Recent studies, however, have shown that marine benthic and epiphytic dinoflagellates are also expanding their biogeographical ranges, with frequent species occurrences at latitudes where they were formerly unknown or uncommon (Shears and Ross, 2009). Benthic and epiphytic toxic dinoflagellates are now emerging as a critical issue due to their range expansion from tropical and subtropical waters to temperate coasts (Aligizaki, 2009; Shears and Ross, 2009).
This global spreading phenomenon was noted several decades ago for phytoplankton (Smayda, 1990; Hallegraeff, 2010), and more recently for species associated with HAB events linked to benthic systems (GEOHAB, 2012; Kibler et al., 2015). In particular, whereas bHABs were often previously considered to be restricted to discrete zones (e.g., coral reefs, mangrove coastal waters) of tropical ecosystems there have been increasing reports of toxigenic benthic species and bHAB events in subtropical and even warm temperate regions (GEOHAB, 2012; Kibler et al., 2015).
The implementation of more effective and comprehensive surveillance programs for detection of putatively toxigenic species and better linkages to environmental and human health consequences undoubtedly accounts for some of this apparent increase in the magnitude, frequency and biogeographical distribution of bHABs. Nevertheless, there is now empirical evidence of shifts in distributional range of bHAB-associated taxa that can be circumstantially linked to global change and the mesoscale consequences in local and regional ecosystems. For example, expansion into temperate waters by members of the genus Gambierdiscus has been reported (Jeong et al., 2012). The associated risk of exposure to CFP is usually higher in the tropics (Lewis, 1986), but as sea surface temperatures continue to rise, it is likely to become more prevalent in temperate regions (Kibler et al., 2015). The positive correlation between water temperature and abundance of Gambierdiscus cells could lead to expansion into areas where this genus was never or rarely previously reported.
Blooms of Ostreopsis have emerged within the last decade as a major problem in southern Europe, particularly in the Mediterranean Sea, where they are associated with palytoxins and toxic aerosols causing respiratory problems in humans in affected coastal areas (Lemée et al., 2012). Closely related, if not identical Ostreopsis species, are commonly found in Latin America but not yet associated with toxic events. Knowledge of toxigenicity of Ostreopsis populations in Latin America is also lacking and this must be regarded as a critical issue to be addressed in the near future. Sudden expansion of bloom magnitude within local areas and/or range extension into more temperate waters could yield severe impacts to the tourist industry and shellfish production in Latin America.
Global climate change is frequently invoked to explain shifts in biogeographical distribution of marine microeukaryotes, with increased temperatures considered the primary driver of range expansions. Correlations between climate variability and CFP incidence has led to preliminary conclusions that climate change is already responsible for global increases in CFP cases and that this trend will continue in the future (Tester et al., 2010; Gingold et al., 2014). In addition to the caveat that correlation is not necessarily causality, the assumption that climate-dependent biogeographical shifts in bHAB species distribution is a direct function of sea surface temperature increases is clearly an oversimplification and perhaps not generally valid. Furthermore, although higher water temperatures at benthic interfaces should lead to slightly higher growth rates and range expansion within temperature limits, evidence that such temperature regimes will selectively favor genotypes of bHAB dinoflagellates over other members of benthic communities is lacking. Global change scenarios for consequences on bHAB biodiversity and biogeography must consider not only direct temperature effects, but also anthropogenic consequences due to deforestation, destruction of mangroves, urbanization and altered land use strategies in coastal zones, which can contribute to nutrient loading (eutrophication), organic and heavy metal pollution, ocean acidification, alteration of run-off patterns and volumes, changes in sediment deposition and coastal erosion processes. Anthropogenic disturbances, such as pollution, dredging and construction activities close to reefs have already had a dramatic impact on the health and diversity of reef ecosystems and presumably could influence the pattern and intensity of bHAB events (Chinain et al., 2010b; Rongo and van Woesik, 2011). In addition to these direct anthropogenic environmental stressors, extreme weather events (hurricanes, typhoons, tornados, etc.), whether they are linked to global climate change or not, creates short-term habitat disruption and hydrodynamic shifts that could account for permanent changes in bHAB species biogeography.
The integration of all these oceanographic processes leads to a complex expression of regime shifts yielding changes in the dynamics and distribution of bHABs. Such challenges must be addressed at the appropriate temporal and spatial level, e.g., submesoscale. Perhaps, it is premature to consider constructing predictive models of bHAB dynamics in Latin America, given the current lack of the knowledge of the functional ecology of these complex benthic ecosystems. Nevertheless, it is not too soon to generate testable hypotheses on the interactions among bHAB dinoflagellates and their shifting environmental regime, and the extent to which growth, survival and toxigenesis are determined by external “bottom-up” abiotic mechanisms versus internally regulated acclimation and adaptive responses. Warning signs are required to improve surveillance and research on these bHABs and addressing these hypotheses will generate knowledge to support risk assessment and bHAB dynamic modeling.
The urgency of the requirements for enhanced research and monitoring strategies is underscored by the fact that thousands of cases of human illnesses linked to seafood consumption, primarily CFP caused by transfer of phycotoxins into fish, but also other toxin syndromes originating from benthic dinoflagellates occur annually and often in tropical regions with poorly developed human health and research infrastructure. In Latin America the socio-economic impacts of bHABs, including increases in public health expenses, reduced productivity, lost tourism revenue, the need to seek alternative seafood resources, increases in the cost of monitoring bHAB events and toxins (Berdalet et al., 2017) are often not taken into account and are rarely systematically addressed. Collaborative work among public health authorities, fishermen, aquaculturists and scientists is required to address the challenges posed by bHABs at the local and regional level within Latin America. On a global scale, the integrated research and observational program GlobalHAB has been established to enhance research on these harmful algal species, to understand their relationships within the ecosystem and to determine their future trends in a changing world (Berdalet et al., 2017). Such international programs will provide detailed knowledge about toxins, public health risks, microalgal metabolism, and oceanographic processes and mechanisms related to HAB dynamics, with the ultimate goal of developing strategies for decreasing the impacts of HABs on human health and wellbeing (Berdalet et al., 2016).
In the Latin American context, it is encouraging that bHABs are considered as a major focus of the international research agenda. With the exception of the Gulf of Mexico and the Florida coasts of the United States, and a few isolated islands in the Caribbean region, biogeography and biodiversity of toxigenic benthic dinoflagellates in the Americas is virtually unexplored and poorly understood. In Latin America, the current lack of knowledge on bHABs is at least partially attributable to the paucity of advanced technology (e.g., gene sequencing facilities for phylogenomics and molecular taxonomy; liquid chromatography-mass spectrometry for toxin analysis) and trained personnel applied to biodiversity studies. Yet such capacity building and technology transfer is now underway and successful research initiatives on biogeography and biodiversity will assist in designing effective management strategies for bHAB events in Latin America.
Author Contributions
All authors LD-R, AC, and YO contributed actively in reviewing the literature and interpreting the experimental aspects cited in this review and co-wrote the manuscript with particular focus on their areas of scientific expertise, i.e., toxin chemistry, chemical ecology, ecophysiology and bloom dynamics, and taxonomy, respectively, of marine dinoflagellates.
Funding
Financial contribution for the preparation of this manuscript and associated research activities was provided to AC via the PACES II Research Program (Topic II Coast: WP3) of the Alfred-Wegener-Institut, Helmholtz Zentrum für Polar- und Meeresforschung under the research theme Earth and Environment, Helmholtz Gemeinschaft, Germany.
Conflict of Interest Statement
The authors declare that the research was conducted in the absence of any commercial or financial relationships that could be construed as a potential conflict of interest.
Acknowledgments
The authors thank Mario Fernando Sánchez-Bernal for the graphic design. All authors are members of RedFAN and SOMEFAN, national scientific societies of Mexico for the study of harmful algal blooms.
References
Accoroni, S., Glibert, P. M., Pichierri, S., Romagnoli, T., Marini, M., and Totti, C. (2015). A conceptual model of annual Ostreopsis cf. ovata blooms in the northern Adriatic Sea based on the synergic effects of hydrodynamics, temperature, and the N:P ratio of water column nutrients. Harmful Algae 45, 14–25. doi: 10.1016/j.hal.2015.04.002
Accoroni, S., Romagnoli, T., Colombo, F., Pennesi, C., Di Camillo, C. G., Marini, M., et al. (2011). Ostreopsis cf. ovata bloom in the northern Adriatic Sea during summer 2009: ecology, molecular characterization and toxin profile. Mar. Poll. Bull. 62, 2512–2519. doi: 10.1016/j.marpolbul.2011.08.003
Accoroni, S., and Totti, C. (2016). The toxic benthic dinoflagellates of the genus Ostreopsis in temperate areas: a review. Adv. Oceanogr. Limnol. 7, 1–15. doi: 10.4081/aiol.2016.5591
Acuña, R. (2015). Diarrea aguda. Revista Médica Clínica Las Condes 26, 676–686. doi: 10.1016/j.rmclc.2015.09.010
Aguilar-Trujillo, A. C. (2017). Variación de grupos funcionales de dinoflagelados epifíticos/bentónicos en la costa norte de la Península de Yucatán. Ph.D. Thesis, CINVESTAV-IPN Unidad Mérida, Mérida.
Aguilar-Trujillo, A. C., Okolodkov, Y. B., Herrera-Silveira, J. A., Merino-Virgilio, F. D. C., and Galicia-García, C. (2017). Taxocoenosis of epibenthic dinoflagellates in the coastal waters of the northern Yucatan Peninsula before and after the harmful algal bloom event in 2011–2012. Mar. Poll. Bull. 119, 396–406. doi: 10.1016/j.marpolbul.2017.02.074
Aguilar-Trujillo, A. C., Okolodkov, Y. B., Merino-Virgilio, F., Osorio-Moreno, I., and Herrera-Silveira, J. (2014). “Variación espacial de dinoflagelados bentónicos/epifíticos en aguas costeras del norte de Yucatán (agosto de 2011),” in Golfo de México. Contaminación, Impacto Ambiental, Diagnóstico y Tendencias, 3 Edn, eds A. V. Botello, J. Rendón Von Osten, J. A. Benítez, and G. Gold-Bouchot (México: UNAM-ICMYL), 147–160.
Ajani, P., Harwood, D. T., and Murray, S. A. (2017). Recent trends in marine phycotoxins from Australian coastal waters. Mar. Drugs 15:33. doi: 10.3390/md15020033
Aligizaki, K. (2009). “Spread of potentially toxic benthic dinoflagellates in the Mediterranean Sea: A response to climate change?,” in Phytoplankton Response to Mediterranean Environmental Change, ed. F. Briand (Paris: CIESM),57–61.
Almazán-Becerril, A. (2000). Estudio taxonómico de algunos dinoflagelados potencialmente tóxicos en el Caribe Mexicano. M.Sc. Thesis, Universidad Nacional Autónoma de México, Ciudad Universitaria, México.
Almazán-Becerril, A., Escobar-Morales, S., Rosiles-González, G., and Valadez, F. (2015). Benthic-epiphytic dinoflagellates from the northern portion of the Mesoamerican Reef System. Bot. Mar. 58, 115–128.
Amar, M., Aráoz, R., Iorga, B. I., Yasumoto, T., Servent, D., and Molgó, J. (2018). Prorocentrolide-A from cultured Prorocentrum lima dinoflagellates collected in Japan blocks sub-types of nicotinic acetylcholine receptors. Toxins 10:97. doi: 10.3390/toxins10030097
Amzil, Z., Sibat, M., Chomerat, N., Grossel, H., Marco-Miralles, F., Lemee, R., et al. (2012). Ovatoxin-a and palytoxin accumulation in seafood in relation to Ostreopsis cf. ovata blooms on the French Mediterranean coast. Mar. Drugs 10, 477–496. doi: 10.3390/md10020477
An, T., Winshell, J., Scorzetti, G., Fell, J. W., and Rein, K. S. (2010). Identification of okadaic acid production in the marine dinoflagellate Prorocentrum rhathymum from Florida Bay. Toxicon 55, 653–657. doi: 10.1016/j.toxicon.2009.08.018
Arbelaez, N., Pineda, J. E. M., and Reguera, B. (2017). Dinoflagelados epífitos de Thalassia testudinum en dos sistemas costeros del Caribe colombiano. Bol. Invest. Mar. Cost. 46, 9–40. doi: 10.25268/bimc.invemar.2017.46.2.725
Balech, E. (1956). Étude des Dinoflagellés du sable de Roscoff. Revue Algologique, Nouvelle Serie 2, 29–52.
Barón-Campis, S. A., Okolodkov, Y. B., Ríos-Lara, G. V., Vázquez-Gómez, N., Rubio-Sánchez, V., Arce-Rocha, G., et al. (2014). “Dinoflagelados nocivos y ciguatoxinas en la costa norte de Yucatán: un riesgo para el futuro desarrollo de la maricultura,” in Proceedings of the XXI Congreso Nacional de Ciencia y Tecnología del Mar, México City.
Bauer, I., Maranda, L., Young, K. A., Shimizu, Y., Fairchild, C., Cornell, L., et al. (1995a). Isolation and structure of caribenolide I, a highly potent antitumor macrolide from a cultured free-swimming Caribbean dinoflagellate, Amphidinium sp. S1-36-5. J. Org. Chem. 60, 1084–1086. doi: 10.1021/jo00109a050
Bauer, I., Maranda, L., Young, K. A., Shimizu, Y., and Huang, S. (1995b). The isolation and structures of unusual 1, 4-polyketides from the dinoflagellate, Amphidinium sp. Tetrahedron Lett. 36, 991–994.
Ben-Gharbia, H., Yahia, O. K.-D., Amzil, Z., Chomérat, N., Abadie, E., Masseret, E., et al. (2016). Toxicity and growth assessments of three thermophilic benthic dinoflagellates (Ostreopsis cf. ovata, Prorocentrum lima and Coolia monotis) developing in the southern Mediterranean basin.Toxins 8:297.
Berdalet, E., Fleming, L. E., Gowen, R., Davidson, K., Hess, P., Backer, L. C., et al. (2016). Marine harmful algal blooms, human health and wellbeing: challenges and opportunities in the 21st century. J. Mar. Biol. Assoc. 96, 61–91. doi: 10.1017/S0025315415001733
Berdalet, E., Tester, P. A., Chinain, M., Fraga, S., Lemée, R., Litaker, W., et al. (2017). Harmful algal blooms in benthic systems: recent progress and future research. Oceanography 30, 36–45. doi: 10.5670/oceanog.2017.108
Bialojan, C., and Takai, A. (1988). Inhibitory effect of a marine-sponge toxin, okadaic acid, on protein phosphatases. specificity and kinetics. Biochem. J. 256, 283–290. doi: 10.1042/bj2560283
Blanco, J., Moroño,Á., and Fernández, M. L. (2005). Toxic episodes in shellfish, produced by lipophilic phycotoxins: an overview. Galician J. Mar. Resour. 1, 1–70.
Boisnoir, A., Pascal, P.-Y., Cordonnier, S., and Lemée, R. (2018). Depth distribution of benthic dinoflagellates in the Caribbean Sea. J. Sea Res. 135, 74–83. doi: 10.1016/j.seares.2018.02.001
Boisnoir, A., Pascal, P.-Y., Cordonnier, S., and Lemée, R. (2019). Spatio-temporal dynamics and biotic substrate preferences of benthic dinoflagellates in the Lesser Antilles, Caribbean sea. Harmful Algae 81, 18–29. doi: 10.1016/j.hal.2018.11.012
Bomber, J. W., Morton, S. L., Babinchak, J. A., Norris, D. R., and Morton, J. G. (1988). Epiphytic dinoflagellates of drift algae—another toxigenic community in the ciguatera food chain. Bull. Mar. Sci. 43, 204–214.
Bomber, J. W., Rubio, M. G., and Norris, D. R. (1989). Epiphytism of dinoflagellates associated with the disease ciguatera: substrate specificity and nutrition. Phycologia 28, 360–368. doi: 10.2216/i0031-8884-28-3-360.1
Braga, A. C., Alves, R. N., Maulvault, A. L., Barbosa, V., Marques, A., and Costa, P. R. (2016). In vitro bioaccessibility of the marine biotoxin okadaic acid in shellfish. Food Chem. Toxicol. 89, 54–59. doi: 10.1016/j.fct.2016.01.008
Bravo, I., Figueroa, R. I., and Fraga, S. (2014). Cellular and nuclear morphological variability within a single species of the toxigenic dinoflagellate genus Gambierdiscus: relationship to life-cycle processes. Harmful Algae 40, 1–8. doi: 10.1016/j.hal.2014.09.009
Calado, A. J., and Moestrup, Ø (2005). On the freshwater dinoflagellates presently included in the genus Amphidinium, with a description of Prosoaulax gen. nov. Phycologia 44, 112–119. doi: 10.2216/0031-8884(2005)44[112:OTFDPI]2.0.CO;2
Carlson, R. D., and Tindall, D. R. (1985). “Distribution and periodicity of toxic dinoflagellates in the Virgin Islands,” in Toxic dinoflagellates, eds D. M. Anderson, A. W. White, and D. G. Baden (New York, NY: Elsevier Sci), 171–176.
Carnicer, O., García-Altares, M., Andree, K. B., Diogène, J., and Fernández-Tejedor, M. (2016). First evidence of Ostreopsis cf. ovata in the eastern tropical Pacific Ocean, Ecuadorian coast. Bot. Mar. 59, 267–274. doi: 10.1515/bot-2016-0022
Chinain, M., Darius, H. T., Ung, A., Cruchet, P., Wang, Z., Ponton, D., et al. (2010a). Growth and toxin production in the ciguatera-causing dinoflagellate Gambierdiscus polynesiensis (Dinophyceae) in culture. Toxicon 56, 739–750. doi: 10.1016/j.toxicon.2009.06.013
Chinain, M., Darius, H. T., Ung, A., Fouc, M. T., Revel, T., Cruchet, P., et al. (2010b). Ciguatera risk management in French Polynesia: the case study of Raivavae Island (Australes Archipelago). Toxicon 56, 674–690. doi: 10.1016/j.toxicon.2009.05.032
Chinain, M., Faust, M. A., and Pauillac, S. (1999). Morphology and molecular analyses of three toxic species of Gambierdiscus (Dinophyceae): G. pacificus, sp. nov., G. australes, sp. nov., and G. polynesiensis, sp. nov. J. Phycol. 35, 1282–1296. doi: 10.1046/j.1529-8817.1999.3561282.x
Ciminiello, P., Dell’Aversano, C., Iacovo, E. D., Fattorusso, E., Forino, M., Tartaglione, L., et al. (2013). Investigation of toxin profile of Mediterranean and Atlantic strains of Ostreopsis cf. siamensis (Dinophyceae) by liquid chromatography–high resolution mass spectrometry. Harmful Algae 23, 19–27. doi: 10.1016/j.hal.2012.12.002
Ciminiello, P., Dell’Aversano, C., Iacovo, E. D., Fattorusso, E., Forino, M., Tartaglione, L., et al. (2014). First finding of Ostreopsis cf. ovata toxins in marine aerosols. Environ. Sci. Technol. 48, 3532–3540. doi: 10.1021/es405617d
Cortés-Altamirano, R., Sierra-Beltrán, A., and Cortés-Lara, M. C. (2011). “Ultraestructura de Ostreopsis siamensis y O. ovata (Dinophyceae): referencia especial a la placa 2′, el poro apical (Po) y poros tecales,” in Proceedings of the XIV Congreso Latino-Americano de Ciências de Mar, COLACMAR, Camboriú.
Cortés-Altamirano, R., and Sierra-Beltrán, A. P. (2003). Morphology and taxonomy of Prorocentrum mexicanum and reinstatement of Prorocentrum rhathymum (Dinophyceae). J. Phycol. 39, 221–225. doi: 10.1046/j.1529-8817.2003.01112.x
Cortés-Lara, M. C., Cortés-Altamirano, R., Sierra-Beltrán, A., and Reyes-Juárez, A. (2005). Ostreopsis siamensis (Dinophyceae) a new tychoplanktonic record from Isabel Island National Park, Pacific Mexico. Harmful Algae News 28, 4–5.
Cuellar-Martinez, T., Ruiz-Fernández, A. C., Alonso-Hernández, C., Amaya-Monterrosa, O., Quintanilla, R., Carrillo-Ovalle, H. L., et al. (2018). Addressing the problem of harmful algal blooms in Latin America and the Caribbean-a regional network for early warning and response. Front. Mar. Sci. 5:409. doi: 10.3389/fmars.2018.00409
Cutignano, A., Nuzzo, G., Sardo, A., and Fontana, A. (2017). The missing piece in biosynthesis of amphidinols: first evidence of glycolate as a starter unit in new polyketides from Amphidinium carterae. Mar. Drugs 15:157. doi: 10.3390/md15060157
Dechraoui-Bottein, M. Y., and Clausing, R. J. (2017). Receptor-binding assay for the analysis of marine toxins: detection and mode of action. Comp. Anal. Chem. 78, 277–301. doi: 10.1016/bs.coac.2017.08.004
Delgado, G., Popowski, G., García, C., Lagos, N., and Lechuga-Devéze, C. H. (2005). Presence of DSP-toxins in Prorocentrum lima (Ehrenberg) Dodge in Cuba. Rev. Invest. Mar. 26, 229–234.
Delgado, G., Popowski, G., and Pombo, M. D. C. (2002). Nuevos registros de dinoflagelados tóxicos epibenticos en Cuba. Rev. Invest. Mar. 23, 229–232.
Delgado-Miranda, G. (2005). Dinoflagelados bentónicos tóxicos asociados a la ciguatera: abundancia, toxicidad y relación con los factores ambientales en un área de litoral noroccidental de Cuba. Ph. D. Thesis, Centro de Investigaciones Biológicas del Noroeste, La Paz, B.C.S., Mexico.
Díaz-Asencio, L., Chamero-Lago, D., Gómez-Batista, M., Vandersea, M. W., García-Chamero, A., and Moreira-González, A. (2016). “Progress on ciguatera risk assessment in South Central Cuba,” in Proceedings of the Abstract Book of the 17th International Conference on Harmful Algae, Florianópolis.
Dodge, J. D. (1982). Marine Dinoflagellates of the British Isles. London: Her Majesty’s Stationery Office.
Dominguez, H. J., Paz, B., Daranas, A. H., Norte, M., Franco, J. M., and Fernández, J. J. (2010). Dinoflagellate polyether within the yessotoxin, pectenotoxin and okadaic acid toxin groups: characterization, analysis and human health implications. Toxicon 56, 191–217. doi: 10.1016/j.toxicon.2009.11.005
Doucet, E., Ross, N. N., and Quilliam, M. A. (2007). Enzymatic hydrolysis of esterified diarrhetic shellfish poisoning toxins and pectenotoxins. Anal. Bioanal. Chem. 389, 335–342. doi: 10.1007/s00216-007-1489-3
Echigoya, R., Rhodes, L., Oshima, Y., and Satake, M. (2005). The structures of five new antifungal and hemolytic amphidinol analogs from Amphidinium carterae collected in New Zealand. Harmful Algae 4, 383–389. doi: 10.1016/j.hal.2004.07.004
Espiritu, R. A., Tan, M. C. S., and Oyong, G. G. (2017). Evaluation of the anti-cancer potential of amphidinol 2, a polyketide metabolite from the marine dinoflagellate Amphidinium klebsii. Jordan J. Biol. Sci. 10,297–302.
Estacion, M. (2000). “Ciguatera toxins: Mechanism of action and pharmacology of maitotoxin,” in Seafood and Freshwater toxins. Pharmacology, Physiology and Detection, ed. L. Botana (New York, NY: Marcel Dekker), 473–504. doi: 10.1201/9780203909539.ch22
Estrada-Vargas, L., Okolodkov, Y., Aké-Castillo, J. A., Pérez-España, H., Morquecho-Escamilla, L., and Martínez-Serrano, I. (2017). “Dinoflagelados epibentónicos en la zona arrecifal de Punta Norte, Veracruz,” in 4o Congreso Nacional de la Sociedad Mexicana para el Estudio de los Florecimientos Algales Nocivos A.C. y 2a Reunión de la Asociación Latinoamericana para el Estudio de Algas Nocivas: Sociedad Mexicana para el Estudio de los Florecimientos Algales Nocivos A.C, Cancún.
Faust, M. A. (1990a). “Cysts of Prorocentrum marinum (Dinophyceae) in floating detritus at Twin Cays, Belize mangrove habitats,” in Proceedings of the IV International Conference on Toxic Marine Phytoplankton, eds E. Granéli, B. Sundström, L. Edler, and D. M. Anderson (New York, NY: Elsevier), 138–143.
Faust, M. A. (1990b). Morphologic details of six benthic species of Prorocentrum (Pyrrophyta) from a mangrove island, Twin Cays, Belize, including two new species. J. Phycol. 26, 548–558. doi: 10.1111/j.0022-3646.1990.00548.x
Faust, M. A. (1991). Morphology of ciguatera-causing Prorocentrum lima (Pyrrophyta) from widely differing sites. J. Phycol. 27, 642–648. doi: 10.1111/j.0022-3646.1991.00642.x
Faust, M. A. (1992). Observations on the morphology and sexual reproduction of Coolia monotis (Dinophyceae). J. Phycol. 28, 94–104. doi: 10.1111/j.0022-3646.1992.00094.x
Faust, M. A. (1993a). “Alternate asexual reproduction of Prorocentrum lima in culture,” in Toxic Phytoplankton Blooms in the Sea, eds T. J. Smayda and Y. Shimizu (New York, NY: Elsevier Sci Publ), 115–120.
Faust, M. A. (1993b). “Ecology of toxic benthic, toxic dinoflagellates: an overview,” in Proceedings of the VI International Conference on Toxic Marine Phytoplankton, eds P. Lassus, G. Arzul, E. Erard-Le Denn, P. Gentien, and C. Marcaillou-Le Baut (Paris: Intercept), 847–854.
Faust, M. A. (1993c). Prorocentrum belizeanum, Prorocentrum elegans, and Prorocentrum caribbaeum, three new benthic species (Dinophyceae) from a mangrove island, Twin Cays, Belize. J. Phycol. 29, 100–107. doi: 10.1111/j.1529-8817.1993.tb00287.x
Faust, M. A. (1993d). Three new benthic species of Prorocentrum (Dinophyceae) from Twin Cays, Belize: P. maculosum sp. nov., P. foraminosum sp. nov. and P. formosum sp. nov. Phycologia 32, 410–418. doi: 10.1111/j.1529-8817.2007.00450.x
Faust, M. A. (1994). Three new benthic species of Prorocentrum (Dinophyceae) from Carry Bow Cay, Belize: P. sabulosum sp. nov., P. sculptile sp. nov., and P. arenarium sp. nov. J. Phycol. 30, 755–763. doi: 10.1111/j.0022-3646.1994.00755.x
Faust, M. A. (1995). Observation of sand-dwelling toxic dinoflagellates (Dinophyceae) from widely differing sites, including two new species. J. Phycol. 31, 996–1003. doi: 10.1111/j.0022-3646.1995.00996.x
Faust, M. A. (1996). Dinoflagellates in a mangrove ecosystem, Twin Cays, Belize. Nova Hedwigia 112, 447–460.
Faust, M. A. (1997). Three new benthic species of Prorocentrum (Dinophyceae) from Belize: P. norrisianum sp. nov., P. tropicalis sp. nov., and P. reticulatum sp. nov. J. Phycol. 33, 851–858. doi: 10.1111/j.0022-3646.1997.00851.x
Faust, M. A. (1999). Three new Ostreopsis species (Dinophyceae): O. marinus sp. nov., O. belizeanus sp. nov., and O. caribbeanus sp. nov. Phycologia 38, 92–99. doi: 10.2216/i0031-8884-38-2-92.1
Faust, M. A. (2000). Dinoflagellate associations in a coral reef-mangrove ecosystem: pelican and associated Cays, Belize. Atoll. Res. Bull. 473, 135–147. doi: 10.5479/si.00775630.473.137
Faust, M. A. (2004). The dinoflagellates of Twin Cays, Belize: biodiversity, distribution and vulnerability. Atoll. Res. Bull. 514, 1–20. doi: 10.5479/si.00775630.514.1
Faust, M. A., and Gulledge, R. A. (1996). Associations of microalgae and meiofauna in floating detritus at a mangrove island, Twin Cays, Belize. J. Exp. Mar. Biol. Ecol. 197, 159–175. doi: 10.1016/0022-0981(95)00159-X
Faust, M. A., and Gulledge, R. A. (2002). Identifying harmful marine dinoflagellates. Contr. U.S Natl. Herb. 42, 1–144.
Faust, M. A., Larsen, J., and Moestrup, Ø (1999). “Potentially toxic phytoplankton. 3. Genus Prorocentrum (Dinophyceae),” in ICES Identification Leaflets for Plankton, ed. J. A. Lindley (Copenhagen: International Council for the Exploration of the Sea), 1–23.
Faust, M. A., Litaker, R. W., Vandersea, M. V., Kibler, S. R., and Tester, P. A. (2005). Dinoflagellate, diversity and abundance in two Belizean coral reef-mangrove lagoons: a test of Margalef’s Mandala. Atoll. Res. Bull. 534, 105–131. doi: 10.5479/si.00775630.534.105
Faust, M. A., and Morton, S. L. (1996). Morphology and ecology of the marine dinoflagellate Ostreopsis labens sp. nov. (Dinophyceae). J. Phycol. 32, 456–463.
Faust, M. A., and Steidinger, K. A. (1998). Bysmatrum gen. nov.(Dinophyceae) and three new combinations for benthic scrippsielloid species. Phycologia 37, 47–52. doi: 10.2216/i0031-8884-37-1-47.1
Faust, M. A., Vandersea, M. W., Kibler, S. R., Tester, P. A., and Litaker, R. W. (2008). Prorocentrum levis, a new benthic species (Dinophyceae) from a mangrove island, Twin Cays, Belize. J. Phycol. 44, 232–240. doi: 10.1111/j.1529-8817.2007.00450.x
Ferron, P.-J., Hogeveen, K., Fessard, V., and Hégarat, L. L. (2014). Comparative analysis of the cytotoxic effects of okadaic acid-group toxins on human intestinal cell lines. Mar. Drugs 12, 4616–4634. doi: 10.3390/md12084616
Gallegos-Mendiola, V., Pérez-Cruz, B., Díaz-Ortiz, J. A., Morán-Vega, D. E., Huante-Catalán, R., and Gárate-Lizárraga, I. (2017). “Ocurrencia de Ostreopsis ovata en la playa Piedra de Tlalcoyunque en la región Costa Grande, Guerrero, México,” in 4o Congreso Nacional de la Sociedad Mexicana para el Estudio de los Florecimientos Algales Nocivos A.C. y 2a Reunión de la Asociación Latinoamericana para el Estudio de Algas Nocivas, Cancún, 96.
Gárate-Lizárraga, I. (2005). “Florecimientos algales nocivos,” in Perspectivas para el desarrollo acuacultural del sistema lagunar de Bahía Magdalena-Almejas, B.C.S., México, ed. B. H. Anguas-Vélez (Mexico: Comité Editorial del Instituto Politécnico Nacional), 41–48.
Gárate-Lizárraga, I. (2012). Proliferation of Amphidinium carterae (Gymnodiniales: Gymnodiniaceae) in Bahía de La Paz, Gulf of California. CICIMAR Oceánides 27, 37–49.
Gárate-Lizárraga, I., Band-Schmidt, C. J., Verdugo-Díaz, G., Muñetón-Gómez, M. d. S., and Féliz-Pico, E. F. (2007). “Dinoflagelados (Dinophyceae) del sistema lagunar Magdalena-Almejas,” in Estudios Ecológicos de Bahía Magdalena, eds R. Funes-Rodríguez, J. Gómez-Gutierrez, and R. Palomares-García (Mexico City: Instituto Politécnico Nacional), 145–174.
Gárate-Lizárraga, I., González-Armas, R., and Okolodkov, Y. B. (2018). Occurrence of Ostreopsis lenticularis (Dinophyceae: Gonyaulacales) from the Archipielago de Revillagigedo, Mexican Pacific. Mar. Poll. Bull. 128, 390–395. doi: 10.1016/j.marpolbul.2018.01.020
García-Mendoza, E., Núñez-Vázquez, E., and Almazán Becerril, A. (2016). Legislación sanitaria asociada al control de ficotoxinas marinas en México. La Jornada Ecológica. Available at: https://www.jornada.com.mx/2016/07/25/eco-i.html
GEOHAB (2012). “Global ecology and oceanography of harmful algal blooms,” in GEOHAB Core Research Project: HABs in Benthic Systems, eds E. Berdalet, P. Tester, and A. Zingone, (Paris: IOC-UNESCO), 64.
Gingold, D. B., Strickland, M. J., and Hess, J. J. (2014). Ciguatera fish poisoning and climate change: analysis of National Poison Center data in the United States, 2001–2011. Environ. Health Perspect. 122, 580–586. doi: 10.1289/ehp.1307196
Gómez, F., Qiu, D., and Lin, S. (2017a). The synonymy of the toxic dinoflagellates Prorocentrum mexicanum and P. rhathymum and the description of P. steidingerae sp. nov. (Prorocentrales, Dinophyceae). J. Eukaryot Microbiol. 64, 668–677. doi: 10.1111/jeu.12403
Gómez, F., Qiu, D., Lopes, R. M., and Lin, S. (2017b). Morphological and molecular characterization of the toxic dinoflagellate Ostreopsis cf. ovata (Gonyaulacales: Dinophyceae) from Brazil (South Atlantic Ocean). Rev. Biol. Trop. 65, 1022–1032. doi: 10.15517/rbt.v65i3.29436
Gómez, F., Qiu, D., Lopes, R. M., and Lin, S. (2015). Fukuyoa paulensis gen. et sp. nov., a new genus for the globular species of the dinoflagellate Gambierdiscus (Dinophyceae). PLoS One 10:e0119676. doi: 10.1371/journal.pone.0119676
Hallegraeff, G. M. (2010). Ocean climate change, phytoplankton community responses, and harmful algal blooms: a formidable predictive challenge. J. Phycol. 46, 220–235. doi: 10.1111/j.1529-8817.2010.00815.x
Hardison, D. R., Holland, W. C., Mccall, J. R., Bourdelais, A. J., Baden, D. G., Darius, H. T., et al. (2016). Fluorescent receptor binding assay for detecting ciguatoxins in fish. PLoS One 11:e0153348. doi: 10.1371/journal.pone.0153348
Heredia-Tapia, A., Arredondo-Vega, B., Nuñez-Vázquez, E., Yasumoto, T., Yasuda, M., and Ochoa, J. (2002). Isolation of Prorocentrum lima (Syn. Exuviaella lima) and diarrhetic shellfish poisoning (DSP) risk assessment in the Gulf of California, Mexico. Toxicon 40, 1121–1127. doi: 10.1016/S0041-0101(02)00111-3
Hernández-Becerril, D., and Almazán Becerril, A. (2004). Especies de dinoflagelados del género Gambierdiscus (Dinophyceae) del Mar Caribe mexicano. Rev. Biol. Trop. 52, 77–87.
Herrera-Sepúlveda, A., Medlin, L. K., Murugan, G., Sierra-Beltrán, A. P., Cruz-Villacorta, A. A., and Hernández-Saavedra, N. Y. (2015). Are Prorocentrum hoffmannianum and Prorocentrum belizeanum (Dinophyceae, Prorocentrales), the same species? An integration of morphological and molecular data. J. Phycol. 51, 173–188. doi: 10.1111/jpy.12265
Holland, W. C., Litaker, R. W., Tomas, C. R., Kibler, S. R., Place, A. R., Davenport, E. D., et al. (2013). Differences in the toxicity of six Gambierdiscus (Dinophyceae) species measured using an in vitro human erythrocyte lysis assay. Toxicon 65, 15–33. doi: 10.1016/j.toxicon.2012.12.016
Holmes, M. J., Brust, A., and Lewis, R. J. (2014). “Dinoflagellate toxins: an overview,” in Seafood and Freshwater Toxins: Pharmacology, Physiology, and Detection, ed. L. Botana (Boca Raton, FL: CRC Press), 3–38.
Holmes, M. J., Lewis, R. J., Jones, A., and Hoy, A. W. W. (1995). Cooliatoxin, the first toxin from Coolia monotis (Dinophyceae). Nat. Toxins 3, 355–362. doi: 10.1002/nt.2620030506
Honsell, G., Bonifacio, A., De Bortoli, M., Penna, A., Battocchi, C., Ciminiello, P., et al. (2013). New insights on cytological and metabolic features of Ostreopsis cf. ovata Fukuyo (Dinophyceae): a multidisciplinary approach. PLoS One 8:e57291. doi: 10.1371/journal.pone.0057291
Hoppenrath, M., Chomérat, N., Horiguchi, T., Schweikert, M., Nagahama, Y., and Murray, S. (2013). Taxonomy and phylogeny of the benthic Prorocentrum species (Dinophyceae)—A proposal and review. Harmful Algae 27, 1–28. doi: 10.1016/j.hal.2013.03.006
Hoppenrath, M., Elbrächter, M., and Drebes, G. (eds) (2009). Marine phytoplankton. Selected microphytoplankton species from the North Sea around Helgoland and Sylt. Stuttgart: E. Schweizerbart’sche Verlagsbuchhandlung.
Hoppenrath, M., Murray, S. A., Chomérat, N., and Horiguchi, T. (2014). Marine Benthic Dinoflagellates-unveiling Their Worldwide Biodiversity. Stuttgart: E. Schweizerbart’sche Verlagsbuchhandlung.
Hu, T., Leblanc, P., Burton, I. W., Walter, J. A., Mccarron, P., Melanson, J. E., et al. (2017). Sulfated diesters of okadaic acid and DTX-1: self-protective precursors of diarrhetic shellfish poisoning (DSP) toxins. Harmful Algae 63, 85–93. doi: 10.1016/j.hal.2017.01.012
Irola-Sansores, E. D., Delgado-Pech, B., Garcia-Mendoza, E., Núñez-Vázquez, E. J., Olivos-Ortiz, A., and Almazán-Becerril, A. (2018). Population dynamics of benthic-epiphytic dinoflagellates on two macroalgae from coral reef systems of the northern Mexican Caribbean. Front. Mar. Sci. 5:487. doi: 10.3389/fmars.2018.00487
James, K., Carey, B., O’halloran, J., and Škrabáková, Z. (2010). Shellfish toxicity: human health implications of marine algal toxins. Epidemiol. Infect. 138, 927–940. doi: 10.1017/S0950268810000853
Jeong, H. J., Lim, A. S., Jang, S. H., Yih, W. H., Kang, N. S., Lee, S. Y., et al. (2012). First report of the epiphytic dinoflagellate Gambierdiscus caribaeus in the temperate waters off Jeju Island, Korea: morphology and molecular characterization. J. Eukaryot Microbiol. 59, 637–650. doi: 10.1111/j.1550-7408.2012.00645.x
Jørgensen, M. F., Murray, S., and Daugbjerg, N. (2004). Amphidinium revisited. I. Redefinition of Amphidinium (Dinophyceae) based on cladistic and molecular phylogenetic analyses. J. Phycol. 40, 351–365. doi: 10.1111/j.1529-8817.2004.03131.x
Karafas, S., Teng, S. T., Leaw, C. P., and Alves-De-Souza, C. (2017). An evaluation of the genus Amphidinium (Dinophyceae) combining evidence from morphology, phylogenetics, and toxin production, with the introduction of six novel species. Harmful Algae 68, 128–151. doi: 10.1016/j.hal.2017.08.001
Karafas, S., York, R., and Tomas, C. (2015). Morphological and genetic analysis of the Coolia monotis species complex with the introduction of two new species, Coolia santacroce sp. nov. and Coolia palmyrensis sp. nov. (Dinophyceae). Harmful Algae 46, 18–33. doi: 10.1016/j.hal.2015.05.002
Karafas, S. J., and Tomas, C. R. (2015). Further observations on the genetics and morphometrics of Coolia santacroce (Dinophyceae). Algae 30, 275–280. doi: 10.4490/algae.2015.30.4.275
Kato, T., and Yasumoto, T. (2017). Quantification of representative ciguatoxins in the Pacific using quantitative nuclear magnetic resonance spectroscopy. Mar. Drugs 15:309. doi: 10.3390/md15100309
Kibler, S. R., Tester, P. A., Kunkel, K. E., Moore, S. K., and Litaker, R. W. (2015). Effects of ocean warming on growth and distribution of dinoflagellates associated with ciguatera fish poisoning in the Caribbean. Ecol. Model. 316, 194–210. doi: 10.1016/j.ecolmodel.2015.08.020
Kobayashi, J. I., and Kubota, T. (2007). Bioactive macrolides and polyketides from marine dinoflagellates of the genus Amphidinium. J. Nat. Prod. 70, 451–460. doi: 10.1021/np0605844
Kobayashi, J. I., Shimbo, K., Sato, M., and Tsuda, M. (2002). Amphidinolides H2- H5, G2, and G3, new cytotoxic 26-and 27-membered macrolides from dinoflagellate Amphidinium sp. J. Org. Chem. 67, 6585–6592. doi: 10.1021/jo016222c
Kobayashi, J. I., Tsuda, M., Ishibashi, M., Shigemori, H., Yamasu, T., Hirota, H., et al. (1991). Amphidinolide F, a new cytotoxic macrolide from the marine dinoflagellate Amphidinium sp. J. Antibiot. 44, 1259–1261. doi: 10.7164/antibiotics.44.1259
Kohli, G. S., Farrell, H., and Murray, S. A. (2015). “Gambierdiscus, the cause of ciguatera fish poisoning: An increased human health threat influenced by climate change,” in Climate Change and Marine and Freshwater Toxins, eds L. Botana, C. Louzao, and N. Vilariño (Boston, MA: Walter de Gruyter GmbH), 273–312.
Kohli, G. S., Papiol, G. G., Rhodes, L. L., Harwood, D. T., Selwood, A., Jerrett, A., et al. (2014). A feeding study to probe the uptake of maitotoxin by snapper (Pagrus auratus). Harmful Algae 37, 125–132. doi: 10.1016/j.hal.2014.05.018
Kumar-Roiné, S., Matsui, M., Chinain, M., Laurent, D., and Pauillac, S. (2008). Modulation of inducible nitric oxide synthase gene expression in RAW 264.7 murine macrophages by Pacific ciguatoxin. Nitric Oxide 19, 21–28. doi: 10.1016/j.niox.2008.03.001
Larsson, M. E., Harwood, T. D., Lewis, R. J., Swa, H., and Doblin, M. A. (2018). Toxicological characterization of Fukuyoa paulensis (Dinophyceae) from temperate Australia. Phycol. Res. 67, 65–71. doi: 10.1111/pre.12349
Lassus, P., Chomérat, N., Hess, P., and Nézan, E. (2016). Toxic and Harmful Microalgae of the World Ocean–Micro-Algues Toxiques et Nuisibles de L’océan Mondial. Copenhagen: Intergovernmental Oceanographic Commission of UNESCO.
Le Gac, M., Metegnier, G., Chomérat, N., Malestroit, P., Quéré, J., Bouchez, O., et al. (2016). Evolutionary processes and cellular functions underlying divergence in Alexandrium minutum. Mol. Ecol. 25, 5129–5143. doi: 10.1111/mec.13815
Leaw, C. P., Lim, P. T., Cheng, K. W., Ng, B. K., and Usup, G. (2010). Morphology and molecular characterization of a new species of thecate benthic dinoflagellate, Coolia malayensis sp. nov. (Dinophyceae). J. Phycol. 46, 162–171. doi: 10.1111/j.1529-8817.2009.00778.x
LeJeunesse, T. C., Parkinson, J. E., Gabrielson, P. W., Jeong, H. J., Reimer, J. D., Voolstra, C. R., et al. (2018). Systematic revision of Symbiodiniaceae highlights the antiquity and diversity of coral endosymbionts. Curr. Biol. 28, 2570–2580. doi: 10.1016/j.cub.2018.07.008
Lemée, R., Mangialajo, L., Cohu, S., Amzil, Z., Blanfune, A., Chomerat, N., et al. (2012). Interactions between scientists, managers and policy makers in the framework of the French MediOs project on Ostreopsis (2008–2010). Cryptogam. Algol. 33, 137–142. doi: 10.7872/crya.v33.iss2.2011.137
Lewis, N. D. (1986). Disease and development: ciguatera fish poisoning. Soc. Sci. Med. 23, 983–993. doi: 10.1016/0277-9536(86)90255-8
Lewis, R. J., Inserra, M., Vetter, I., Holland, W. C., Hardison, D. R., Tester, P. A., et al. (2016). Rapid extraction and identification of maitotoxin and ciguatoxin-like toxins from Caribbean and Pacific Gambierdiscus using a new functional bioassay. PLoS One 11:e0160006. doi: 10.1371/journal.pone.0160006
Ley-Martinez, T. C., Nuñez-Vázquez, E. J., Almazán Becerril, A., Barón-Campis, S. A., Ramírez-Camarena, C., Caballero-Vázquez, J. A., et al. (2014). “Bioprospección de toxinas tipo ciguatoxinas en peces carnívoros del Caribe mexicano y aguas adyacentes,” in XXI Congreso Nacional de Ciencia y Tecnología del Mar. Quintana Roo: Dirección General de Educación en Ciencia y Tecnología del Mar 1–13.
Lim, A. S., Jeong, H. J., Jang, T. Y., Kang, N. S., Lee, S. Y., Du Yoo, Y., et al. (2013). Morphology and molecular characterization of the epiphytic dinoflagellate Prorocentrum cf. rhathymum in temperate waters off Jeju Island, Korea. Ocean Sci. J. 48, 1–17. doi: 10.1007/s12601-013-0001-6
Litaker, R. W., Holland, W. C., Hardison, D. R., Pisapia, F., Hess, P., Kibler, S. R., et al. (2017). Ciguatoxicity of Gambierdiscus and Fukuyoa species from the Caribbean and Gulf of Mexico. PLoS One 12:e0185776. doi: 10.1371/journal.pone.0185776
Litaker, R. W., Vandersea, M. W., Faust, M. A., Kibler, S. R., Chinain, M., Holmes, M. J., et al. (2009). Taxonomy of Gambierdiscus including four new species, Gambierdiscus caribaeus, Gambierdiscus carolinianus, Gambierdiscus carpenteri and Gambierdiscus ruetzleri (Gonyaulacales, Dinophyceae). Phycologia 48, 344–390. doi: 10.3390/md16010007
Litaker, R. W., Vandersea, M. W., Faust, M. A., Kibler, S. R., Nau, A. W., Holland, W. C., et al. (2010). Global distribution of ciguatera causing dinoflagellates in the genus Gambierdiscus. Toxicon 56, 711–730. doi: 10.1016/j.toxicon.2010.05.017
López, C. (2018). COFEPRIS mantiene alerta por venta de barracuda. La Jornada Maya, domingo 8 de abril de 2018. Available at: https://www.lajornadamaya.mx/2018-04-08/Cofepris-mantiene-alerta-por-venta-de-barracuda
Louzao, M. C., Fernández, D. A., Abal, P., Fraga, M., Vilariño, N., Vieytes, M. R., et al. (2015). Diarrhetic effect of okadaic acid could be related with its neuronal action: changes in neuropeptide Y. Toxicol. Lett. 237, 151–160. doi: 10.1016/j.toxlet.2015.06.004
Mancera-Pineda, J. E., Montalvo-Talaigua, M., and Gavio, B. (2014). Dinoflagelados potencialmente tóxicos asociados a material orgánico flotante (drift) en San Andrés Isla, Reserva Internacional de la Biosfera-Seaflower: potentially toxic dinoflagellates associated to drift in San Andrés Island, International Biosphere Reservation-Seaflower. Caldasia 36, 139–156. doi: 10.15446/caldasia.v36n1.43896
Maranda, L., and Shimizu, Y. (1996). Amphidinium operculatum var. nov. gibbosum (Dinophyceae), a free-swimming marine species producing cytotoxic metabolites. J. Phycol. 32, 873–879. doi: 10.1111/j.0022-3646.1996.00873.x
Marchan-Álvarez, J. G., Valerio-González, L., Troccoli-Ghinaglia, L., and López-Monroy, F. (2017). Dinoflagelados bentónicos nocivos, asociados con el sedimento arenoso en dos playas de la isla de Margarita, Venezuela. Rev. Biodivers. Neotrop. 7, 156–168.
Martínez-Cruz, M. E., Okolodkov, Y. B., Aguilar-Trujillo, A. C., and Herrera-Silveira, J. A. (2016). Epiphytic dinoflagellates on the seagrass Thalassia testudinum at Dzilam, southeastern Gulf of Mexico. Cymbella 1, 2–9.
Meng, Y., Van Wagoner, R. M., Misner, I., Tomas, C., and Wright, J. L. (2010). Structure and biosynthesis of amphidinol 17, a hemolytic compound from Amphidinium carterae. J. Nat. Prod. 73, 409–415. doi: 10.1021/np900616q
Ministerio de Salud, G. D. C. (2008). Programa Nacional de Vigilancia y Control de las Intoxicaciones Por Fenómenos Algales Nocivos (“marea roja”). Santiago: Departamento de Alimentos y Nutrición.
Mitchell, L. E. (1985). Ecological Studies of Benthic Dinoflagellates Associated with Ciguatera in the Florida Keys: the 0-38 Micrometer Size Fraction. MSc, Florida Institute of Technology, Florida.
Moestrup,Ø, Akselmann, R., Fraga, S., Hoppenrath, M., Iwataki, M., Komárek, J., et al. (2009). IOC-UNESCO Taxonomic Reference List of Harmful Micro Algae. Available at: http://www.marinespecies.org/hab/aphia.php?p=taxdetails&id=531446
Moestrup,Ø, and Calado, A. J. (2018). Süßwasserflora von Mitteleuropa, Bd. 6-Freshwater Flora of Central Europe, Dinophyceae, Vol. 6. Berlin: Springer-Verlag GmbH. doi: 10.1007/978-3-662-56269-7
Mohammad-Noor, N., Moestrup,Ø, Lundholm, N., Fraga, S., Adam, A., Holmes, M. J., et al. (2013). Autecology and phylogeny of Coolia tropicalis and Coolia malayensis (Dinophyceae), with emphasis on taxonomy of C. tropicalis based on light microscopy, scanning electron microscopy and LSU rDNA. J. Phycol. 49, 536–545. doi: 10.1111/jpy.12062
Molgó, J., Marchot, P., Aráoz, R., Benoit, E., Iorga, B. I., Zakarian, A., et al. (2017). Cyclic imine toxins from dinoflagellates: a growing family of potent antagonists of the nicotinic acetylcholine receptors. J. Neurochem. 142, 41–51. doi: 10.1111/jnc.13995
Moreira-González, A. R., Felicio-Fernandes, L., Peraza-Escarrá, R., Díaz-Asencio, L., Rodriguez, F., Riobó, P., et al. (2016). “Notes on morphology, phylogeny and toxicity of a dominant community of toxic benthic dinoflagellates from southern-central coast of Cuba,” in Proceedings of the 17th International Conference on Harmful Algae, eds L. A. O. Proença and G. M. Hallegraeff (Florianopolis: International Society for the Study of Harmful Algae), 88.
Morquecho-Escamilla, L., Reyes-Salinas, A., and Okolodkov, Y. (2016). Illustrated Taxonomic Guide of the Marine Dinoflagellate Collection (CODIMAR) Guía Taxonómica Ilustrada de la Colección de Dinoflagelados Marinos (CODIMAR). Mexico: Centro de Investigaciones Biológicas del Noreste, 147.
Morquecho-Escamilla, L., Reyes-Salinas, A., Okolodkov, Y. B., Gárate-Lizárraga, I., Mazariegos-Villarreal, A., and Galicia-García, C. (2017). “Aislamiento y caracterización taxonómica de dinoflagelados epífitos en Bahía de la Paz e Isla San José, Golfo de California,” in 4o Congreso Nacional de la Sociedad Mexicana para el Estudio de los Florecimientos Algales Nocivos A.C. y 2a Reunión de la Asociación Latinoamericana para el Estudio de Algas Nocivas, Cancún.
Morrison, K., Prieto, P. A., Domínguez, A. C., Waltner-Toews, D., and Fitzgibbon, J. (2008). Ciguatera fish poisoning in La Habana, Cuba: a study of local social–ecological resilience. EcoHealth 5, 346–359. doi: 10.1007/s10393-008-0188-7
Morsy, N., Houdai, T., Matsuoka, S., Matsumori, N., Adachi, S., Oishi, T., et al. (2006). Structures of new amphidinols with truncated polyhydroxyl chain and their membrane-permeabilizing activities. Bioorg. Med. Chem. 14, 6548–6554. doi: 10.1016/j.bmc.2006.06.012
Morton, S. L., and Bomber, J. W. (1994). Maximizing okadaic acid content from Prorocentrum hoffmannianum Faust. J. Appl. Phycol. 6, 41–44. doi: 10.1007/BF02185903
Morton, S. L., and Faust, M. A. (1997). Survey of toxic epiphytic dinoflagellates from the Belizean barrier reef ecosystem. J. Mar. Sci. 61, 899–906.
Murakami, Y., Oshima, Y., and Yasumoto, T. (1982). Identification of okadaic acid as a toxic component of a marine dinoflagellate Prorocentrum lima. Nippon Suisan Gakkaishi 48, 69–72. doi: 10.2331/suisan.48.69
Murray, S., Flø Jørgensen, M., Daugbjerg, N., and Rhodes, L. (2004). Amphidinium revisited. II. Resolving species boundaries in the Amphidinium operculatum species complex (Dinophyceae), including the descriptions of Amphidinium trulla sp. nov. and Amphidinium gibbosum. comb. nov. J. Phycol. 40, 366–382. doi: 10.1046/j.1529-8817.2004.03132.x
Nakajima, I., Oshima, Y., and Yasumoto, T. (1981). Toxicity of benthic dinoflagellates found in coral reef-II. Toxicity of benthic dinoflagellates in Okinawa. Nippon Suisan Gakkaishi 47, 1029–1033. doi: 10.2331/suisan.47.1029
Napolitano, J. G., Norte, M., Padrón, J. M., Fernández, J. J., and Hernández Daranas, A. (2009). Belizeanolide, a cytotoxic macrolide from the dinoflagellate Prorocentrum belizeanum. Angew. Chem. Int. Ed. 48, 796–799. doi: 10.1002/anie.200804023
Nascimento, S. M., Mendes, M. C. Q., Menezes, M., Rodríguez, F., Alves-De-Souza, C., Branco, S., et al. (2017). Morphology and phylogeny of Prorocentrum caipirignum sp. nov.(Dinophyceae), a new tropical toxic benthic dinoflagellate. Harmful Algae 70, 73–89. doi: 10.1016/j.hal.2017.11.001
Nascimento, S. M., Purdie, D. A., and Morris, S. (2005). Morphology, toxin composition and pigment content of Prorocentrum lima strains isolated from a coastal lagoon in southern UK. Toxicon 45, 633–649. doi: 10.1016/j.toxicon.2004.12.023
Navarro-Vargas, G., Díaz-Ramos, J. R., Troccoli-Ghinalgia, L., and Subero-Pino, S. (2014). Dinoflagelados epibentónicos presentes en diferentes sustratos en la Bahía Turpialito, golfo de Cariaco, Venezuela. Bol. Inst. Oceanogr. Venezuela 53, 161–170.
Nie, D., and Wang, C.-C. (1944). Dinoflagellata of the Hainan Region. VIII. On Sinophysis microcephalus, a new genus and species of Dinophysidae. Sinensia 15, 145–151.
NOM-242-SSA1 (2009). Norma Oficial Mexicana NOM-242-SSA1. Diario Oficial de la Federación. Available at: http://www.dof.gob.mx/normasOficiales/4295/salud2a/salud2a.htmNOM
Núñez-Vázquez, E. (2005). Biotoxinas Marinas en Peces Comestibles de Baja California Sur, México: Origen, Identificación y Cuantificación. México: Bachelor, Universidad Autónoma de Baja California Sur.
Núñez-Vázquez, E., Almazán-Becerril, A., Heredia-Tapia, A., Hernández-Becerril, D., Troccoli-Ghinaglia, L., Arredondo-Vega, B., et al. (2000). “Incidencia del envenenamiento por ciguatera en México,” in 4a. Reunión de Expertos en Envenenamientos por Animales Ponzoñosos: Instituto de Biotecnología-UNAM, International Society on Toxicology-Sección Panamericana, Instituto Bioclon., Laboratorio Silanes, Cuernavaca, 49–50.
Núñez-Vázquez, E. J., Poot-Delgado, C. A., Turner, A., Ley-Martínez, T. C., Domínguez-Solís, G., Cahuich-Sánchez, Y., et al. (2018). “Presencia de toxinas marinas en peces comerciales de las costas de Campeche, México,” in IX Foro Científico de Pesca Ribereña, Mazatlán.
Okolodkov, Y. B., Campos-Bautista, G., Gárate-Lizárraga, I., González-González, J. A. G., Hoppenrath, M., and Arenas, V. (2007). Seasonal changes of benthic and epiphytic dinoflagellates in the Veracruz reef zone, Gulf of Mexico. Aquat. Microb. Ecol. 47, 223–237. doi: 10.3354/ame047223
Okolodkov, Y. B., and Gárate-Lizárraga, I. (2006). An annotated checklist of dinoflagellates (Dinophyceae) from the Mexican Pacific. Acta Bot. Mex. 74, 1–154. doi: 10.21829/abm74.2006.1008
Okolodkov, Y. B., Merino-Virgilio, F. D. C., Herrera-Silveira, J. A., Espinosa-Matías, S., and Parsons, M. L. (2009). Gambierdiscus toxicus in the southeastern Gulf of Mexico. Harmful Algae News 40, 12–14.
Okolodkov, Y. B., Virgilio, F. D. C. M., Castillo, J. A. A., Trujillo, A. C. A., Espinosa-Matías, S., and Silveira, A. H. (2014). Seasonal changes in epiphytic dinoflagellate assemblages near the northern coast of the Yucatan Peninsula, Gulf of Mexico. Acta Bot. Mex. 107, 121–151. doi: 10.21829/abm107.2014.204
Olsen, D. A., Nellis, D. W., and Wood, R. S. (1984). Ciguatera in the eastern Caribbean. Mar. Fish Rev. 46, 13–18.
Penna, A., Vila, M., Fraga, S., Giacobbe, M. G., Andreoni, F., Riobó, P., et al. (2005). Characterization of Ostreopsis and Coolia (Dinophyceae) isolates in the Western Mediterreanean Sea based on morphology, toxicity and internal transcribed spacer 5.8 S rDNA sequences. J. Phycol. 41, 212–225. doi: 10.1111/j.1529-8817.2005.04011.x
Pisapia, F., Sibat, M., Herrenknecht, C., Lhaute, K., Gaiani, G., Ferron, P.-J., et al. (2017). Maitotoxin-4, a novel MTX analog produced by Gambierdiscus excentricus. Mar. Drugs 15:220. doi: 10.3390/md15070220
Place, A. R., Bowers, H. A., Bachvaroff, T. R., Adolf, J. E., Deeds, J. R., and Sheng, J. (2012). Karlodinium veneficum—The little dinoflagellate with a big bite. Harmful Algae 14, 179–195. doi: 10.1016/j.hal.2011.10.021
Quintana-M, H., and Mercado-Gómez, J. (2017). Composición de dinoflagelados epífitos y forófitos en la Costa norte del golfo de Morrosquillo, Sucre, Colombia. Rev. Colomb. Cienc. Anim. 9, 129–140. doi: 10.24188/recia.v9.n2.2017.550
Quintanilla, C. R., and Amaya, O. (2016). “First report of the epiphytic genera Gambierdiscus and Ostreopsis from the coast of El Salvador, Eastern Tropical Pacific,” in Proceedings of the 17th International Conference on Harmful Algae, eds L. A. O. Proença and G. M. Hallegraeff (Florianopolis: International Society for the Study of Harmful Algae), 80–82.
Rains, L. K., and Parsons, M. L. (2015). Gambierdiscus species exhibit different epiphytic behaviors toward a variety of macroalgal hosts. Harmful Algae 49, 29–39. doi: 10.1016/j.hal.2015.08.005
Ramos, V., and Vasconcelos, V. (2010). Palytoxin and analogs: biological and ecological effects. Mar Drugs 8, 2021–2037. doi: 10.3390/md8072021
Rhodes, L. L., Smith, K. F., Munday, R., Selwood, A. I., McNabb, P. S., Holland, P. T., et al. (2010). Toxic dinoflagellates (Dinophyceae) from Rarotonga, Cook Islands. Toxicon 56, 751–758. doi: 10.1016/j.toxicon.2009.05.017
Rhodes, L. L., and Thomas, A. E. (1997). Coolia monotis (Dinophyceae): a toxic epiphytic microalgal species found in New Zealand. N. Z. J. Mar. Freshw. Res. 31, 139–141. doi: 10.1080/00288330.1997.9516751
Richlen, M. L., and Lobel, P. S. (2011). Effects of depth, habitat, and water motion on the abundance and distribution of ciguatera dinoflagellates at Johnston Atoll, Pacific Ocean. Mar. Ecol. Prog. Ser. 421, 51–66. doi: 10.3354/meps08854
Rongo, T., and van Woesik, R. (2011). Ciguatera poisoning in Rarotonga, southern Cook islands. Harmful Algae 10, 345–355. doi: 10.1016/j.toxicon.2012.12.018
Satake, M., Murata, M., Yasumoto, T., Fujita, T., and Naoki, H. (1991). Amphidinol, a polyhydroxy-polyene antifungal agent with an unprecedented structure, from a marine dinoflagellate, Amphidinium klebsii. J. Am. Chem. Soc. 113, 9859–9861. doi: 10.1021/ja00026a027
Secretaría de Salud (2015). “Plan de contingencia para el control de biotoxinas marinas,” in Comisión Federal para la Protección contra Riesgos Sanitarios, (Mexico City: COFEPRIS). Available at: https://www.gob.mx/cms/uploads/attachment/file/178793/Plan_de_contigencia_MB_ES_20151208_ESP.pdfq
Sepúlveda-Villarraga, M., Band-Schmidt, C. J., Leyva-Valencia, I., Hernández-Carmona, G., and Almazán-Becerril, A. (2017). “Dinoflagelados potencialmente tóxicos asociados a macroalgas en la Bahía de La Paz, B.C.S,” in 4o Congreso Nacional de la Sociedad Mexicana para el Estudio de los Florecimientos Algales Nocivos A.C. y 2a Reunión de la Asociación Latinoamericana para el Estudio de Algas Nocivas, Cancún, 83.
Shears, N. T., and Ross, P. M. (2009). Blooms of benthic dinoflagellates of the genus Ostreopsis; an increasing and ecologically important phenomenon on temperate reefs in New Zealand and worldwide. Harmful Algae 8, 916–925. doi: 10.1016/j.hal.2009.05.003
Sierra-Beltrán, A., Cruz, A., Nuñez, E., Del Villar, L., Cerecero, J., and Ochoa, J. (1998). An overview of the marine food poisoning in Mexico. Toxicon 36, 1493–1502. doi: 10.1016/S0041-0101(98)00139-1
Smayda, T. J. (1990). “Novel and nuisance phytoplankton blooms in the sea: evidence for a global epidemic,” in Toxic Marine Phytoplankton, eds E. Granéli, B. Sundström, L. Edler, and D. M. Anderson (New York: Elsevier), 29–40.
Steidinger, K., and Tangen, K. (1997). “The Planktonic Marine Flagellates,” in Identifying Marine Phytoplankton. ed. C. R. Tomas (San Diego, CA: Academic Press), 591–730.
Steidinger, K. A. (2018). “Chapter III. Toxic and potentially harmful dinoflagellates in the Gulf of Mexico,” in Guide to the Identification of Harmful Microalgae in the Gulf of Mexico, eds K. A. Steidinger and M. E. Meave Del Castillo (St. Peterburg, FL: Florida Institute of Oceanography), 211–342.
Taylor, F., Hoppenrath, M., and Saldarriaga, J. F. (2008). Dinoflagellate diversity and distribution. Biodivers. Conserv. 17, 407–418. doi: 10.1007/s10531-007-9258-3
Tester, P. A., Feldman, R. L., Nau, A. W., Kibler, S. R., and Litaker, R. W. (2010). Ciguatera fish poisoning and sea surface temperatures in the Caribbean Sea and the West Indies. Toxicon 56, 698–710. doi: 10.1016/j.toxicon.2010.02.026
Tester, P. A., Vandersea, M. W., Buckel, C. A., Kibler, S. R., Holland, W. C., Davenport, E. D., et al. (2013). Gambierdiscus (Dinophyceae) species diversity in the Flower Garden Banks National Marine Sanctuary, Northern Gulf of Mexico, USA. Harmful Algae 29, 1–9. doi: 10.1016/j.hal.2013.07.001
Tindall, D., Miller, D., and Tindall, P. (1990). “Toxicity of Ostreopsis lenticularis from the British and United States Virgin Islands,” in Toxic Marine Phytoplankton, eds E. Granéli, L. Edler, and D. M. Anderson (New York,NY: Elsevier), 424–429.
Tindall, D. R., and Morton, S. L. (1998). “Community dynamics and physiology of epiphytic/benthic dinoflagellates associated with ciguatera,” in Physiological Ecology of Harmful Algal Blooms, eds D. A. Anderson, A. D. Cembella, and G. M. Hallegraeff (Berlin: Springer-Verlag), 293–314.
Tosteson, T., Ballantine, D., Tosteson, C., Bardales, A., Durst, H., and Higerd, T. (1986). Comparative toxicity of Gambierdiscus toxicus, Ostreopsis cf. lenticularis, and associated microflora. Mar. Fish Rev. 48, 57–60.
Totti, C., Accoroni, S., Cerino, F., Cucchiari, E., and Romagnoli, T. (2010). Ostreopsis ovata bloom along the Conero Riviera (northern Adriatic Sea): relationships with environmental conditions and substrata. Harmful Algae 9, 233–239. doi: 10.1016/j.hal.2009.10.006
Tsuda, M., Oguchi, K., Iwamoto, R., Okamoto, Y., Kobayashi, J. I., Fukushi, E., et al. (2007). Iriomoteolide-1a, a potent cytotoxic 20-membered macrolide from a benthic dinoflagellate Amphidinium species. J. Org. Chem. 72, 4469–4474. doi: 10.1021/jo070414b
Tsuda, M., Sasaki, T., and Kobayashi, J. (1994). Amphidinolide L, a new cytotoxic 27-membered macrolide from the cultured dinoflagellate Amphidinium sp. J. Org. Chem. 59, 3734–3737. doi: 10.3390/molecules16075422
Tubaro, A., Sosa, S., and Hungerford, J. (2012). “Toxicology and diversity of marine toxins,” in Veterinary Toxicology (Second Edition), ed. R. C. Gupta (San Diego, CA: Elsevier), 896–934.
Twiner, M. J., Doucette, G. J., Pang, Y., Fang, C., Forsyth, C. J., and Miles, C. O. (2016). Structure–activity relationship studies using natural and synthetic okadaic acid/dinophysistoxin toxins. Mar. Drugs 14:207. doi: 10.3390/md14110207
Ukena, T., Satake, M., Usami, M., Oshima, Y., Naoki, H., Fujita, T., et al. (2001). Structure elucidation of ostreocin D, a palytoxin analog isolated from the dinoflagellate Ostreopsis siamensis. Biosci. Biotech. Biochem. 65, 2585–2588. doi: 10.1271/bbb.65.2585
Valdiglesias, V., Prego-Faraldo, M., Pásaro, E., Méndez, J., and Laffon, B. (2013). Okadaic acid: more than a diarrheic toxin. Mar. Drugs 11, 4328–4349. doi: 10.3390/md11114328
Valerio-González, L., and Díaz-Ramos, J. (2008). Distribución de dinoflagelados epifitos potencialmente tóxicos asociados a praderas de Thalassia testudinum en la isla la Tortuga, la Bahía de Mochima y Golfo de Cariaco, Venezuela. Bol. Inst. Oceanogr. Venezuela 47, 47–58.
Vargas-Montero, M., Morales, A., and Cortés, J. (2012). Primer informe del género Gambierdiscus (Dinophyceae) y otros dinoflagelados bentónicos en el Parque Nacional Isla del Coco, Costa Rica, Pacífico Tropical Oriental. Rev. Biol. Trop. 60, 187–199.
Villareal, T., Hanson, S., Qualia, S., Jester, E., Granade, H., and Dickey, R. (2007). Petroleum production platforms as sites for the expansion of ciguatera in the northwestern Gulf of Mexico. Harmful Algae 6, 253–259. doi: 10.1016/j.hal.2006.08.008
Wang, D. Z. (2008). Neurotoxins from marine dinoflagellates: a brief review. Mar. Drugs 6, 349–371. doi: 10.3390/md20080016
Yasumoto, T., and Murata, M. (1993). Marine toxins. Chem. Rev. 93, 1897–1909. doi: 10.1021/cr00021a011
Yasumoto, T., Seino, N., Murakami, Y., and Murata, M. (1987). Toxins produced by benthic dinoflagellates. Biol. Bull. 172, 128–131. doi: 10.2307/1541612
Yépez-Rendón, J. B., Keith, I., Ramírez-Sarmiento, A. K., Chávez-Rodríguez, N. F., and Carnicer-Castaño, O. (2018). Presencia de microalgas epibentónicas en el Pacífico este tropical. Hallazgos 21, 1–23.
Keywords: benthic dinoflagellates, polyether toxins, dinoflagellate biodiversity, phycotoxins, chemical ecology
Citation: Durán-Riveroll LM, Cembella AD and Okolodkov YB (2019) A Review on the Biodiversity and Biogeography of Toxigenic Benthic Marine Dinoflagellates of the Coasts of Latin America. Front. Mar. Sci. 6:148. doi: 10.3389/fmars.2019.00148
Received: 30 November 2018; Accepted: 08 March 2019;
Published: 05 April 2019.
Edited by:
Juan Jose Dorantes-Aranda, University of Tasmania, AustraliaReviewed by:
Gustaaf Marinus Hallegraeff, University of Tasmania, AustraliaPatricia A. Tester, Ocean Tester, LLC., United States
Copyright © 2019 Durán-Riveroll, Cembella and Okolodkov. This is an open-access article distributed under the terms of the Creative Commons Attribution License (CC BY). The use, distribution or reproduction in other forums is permitted, provided the original author(s) and the copyright owner(s) are credited and that the original publication in this journal is cited, in accordance with accepted academic practice. No use, distribution or reproduction is permitted which does not comply with these terms.
*Correspondence: Lorena María Durán-Riveroll, bG9yZW5hLmR1cmFuQGF3aS5kZQ==; bGR1cmFuQGNvbmFjeXQubXg=