- 1CPR Survey, Marine Biological Association, Nanaimo, BC, Canada
- 2The Cyprus Institute, Nicosia, Cyprus
- 3Japan Agency for Marine-Earth Science and Technology (JAMSTEC), Yokohama, Japan
- 4UNEP-WCMC, Cambridge, United Kingdom
- 5CPR Survey, Marine Biological Association, Plymouth, United Kingdom
- 6Regional Centre, CSIR-National Institute of Oceanography, Kochi, India
- 7Department of the Environment and Energy, Australian Antarctic Division, Kingston, TAS, Australia
- 8BOREA Research Unit Biology of Aquatic Organisms and Ecosystems, Sorbonne University, Paris, France
- 9Centre for Marine and Conservation Policy Research, University of Plymouth, Plymouth, United Kingdom
- 10Laboratório de Zooplâncton, Universidade Federal do Rio Grande - FURG, Rio Grande, Brazil
- 11CSIRO Oceans and Atmosphere, Brisbane, QLD, Australia
- 12Faculty of Science, The University of Queensland, Brisbane, QLD, Australia
- 13National Institute of Water and Atmospheric Research, Christchurch, New Zealand
- 14National Institute of Polar Research, Tokyo, Japan
- 15Department of Biological Sciences, Marine Research Institute, University of Cape Town, Cape Town, South Africa
- 16Oceans and Coasts Research, Department of Environmental Affairs, Cape Town, South Africa
Plankton are the base of marine food webs, essential to sustaining fisheries and other marine life. Continuous Plankton Recorders (CPRs) have sampled plankton for decades in both hemispheres and several regional seas. CPR research has been integral to advancing understanding of plankton dynamics and informing policy and management decisions. We describe how the CPR can contribute to global plankton diversity monitoring, being cost-effective over large scales and providing taxonomically resolved data. At OceanObs09 an integrated network of regional CPR surveys was envisaged and in 2011 the existing surveys formed the Global Alliance of CPR Surveys (GACS). GACS first focused on strengthening the dataset by identifying and documenting CPR best practices, delivering training workshops, and developing an integrated database. This resulted in the initiation of new surveys and manuals that enable regional surveys to be standardized and integrated. GACS is not yet global, but it could be expanded into the remaining oceans; tropical and Arctic regions are a priority for survey expansion. The capacity building groundwork is done, but funding is required to implement the GACS vision of a global plankton sampling program that supports decision-making for the scientific and policy communities. A key step is an analysis to optimize the global sampling design. Further developments include expanding the CPR for multidisciplinary measurements via additional sensors, thus maximizing the ship-of-opportunity platform. For example, defining pelagic ecoregions based on plankton and ancillary data could support high seas Marine Protected Area design. Fulfillment of Aichi Target 15, the United Nation’s Sustainable Development Goals, and delivering the Essential Ocean Variables and Essential Biodiversity Variables that the Global Ocean Observing System and Group on Earth Observation’s Biodiversity Observation Network have, respectively, defined requires the taxonomic resolution, spatial scale and time-series data that the CPR approach provides. Synergies with global networks exploiting satellite data and other plankton sensors could be explored, realizing the Survey’s capacity to validate earth observation data and to ground-truth emerging plankton observing platforms. This is required for a fully integrated ocean observing system that can understand global ocean dynamics to inform sustainable marine decision-making.
The Need for Global Plankton Observations
The pelagic zone is the largest biome on Earth. Plankton are found throughout the ∼1 billion km3 of living space in the pelagic zone, and are extremely abundant; one group, the copepods, could be three orders of magnitude more abundant than insects (Schminke, 2007). Plankton underpin almost all marine food webs and provide the link between the physical environment and the fish, marine birds and mammals that society values and which forms the basis of much of the blue economy. Furthermore, plankton are responsible for ∼46% of the planetary photosynthesis, the first step in a series of complex biogeochemical processes in the ocean that make up the biological pump, which involves the export of carbon and other elements from the atmosphere via surface waters into the ocean’s interior. The many and varied roles of plankton make them essential candidates for measuring the health of our oceans in the Anthropocene.
There is an increasing emphasis on globally coordinated marine science strategies toward “conserving and sustainably using the oceans, seas and marine resources for sustainable development” as laid out in the United Nation’s sustainable development goal 14 (SDG14). Plankton are an ideal indicator for sustainably managing our oceans, as they are sensitive to the environment and they are not yet fished to any great extent, meaning that measured changes in plankton communities unambiguously reflect environmental changes and not the amount of harvesting, which complicates analyses of fish stock data.
Plankton: An Essential Ocean and Biodiversity Variable
The Global Ocean Observing System (GOOS) advocates for sustained observations that describe the current ocean state. The initial focus on physical oceanography now informs weather and climate forecasts through a suite of observing platforms (e.g., moorings, voluntary observing ships, satellites, and Argo) to measure the temperature and salinity of the oceans. A more recent focus has been on the biological properties of the ocean, developed from the Framework for Ocean Observation (Lindstrom et al., 2012), with GOOS establishing a Biology and Ecosystems Panel in 2015. Its remit is to promote a global, sustained, and targeted ecosystem observing program based on essential ocean variables (EOVs). Plankton (abundance and diversity) were identified as EOVs with moderate to high relative impact for addressing societal drivers and pressures (Miloslavich et al., 2018).
The Group on Earth Observations Biodiversity Observation Network (GEO BON) has developed Essential Biodiversity Variables (EBVs) to “play the role of brokers between monitoring initiatives and decision makers” with a focus on the status and trend in biodiversity. EBVs include taxonomic diversity to inform policy makers on community composition and secondary productivity as well as plankton functional type variables to inform on ecosystem structure and function.
A key challenge in observing plankton in the pelagic zone over the vast expanses of the ocean is to estimate the zooplankton component. For nearly four decades, phytoplankton have been observed from space. Satellites not only provide estimates of phytoplankton biomass (chlorophyll-a), but also of some phytoplankton functional types (Brewin et al., 2010), although phytoplankton species composition remains elusive. However, zooplankton, the intermediate trophic link between phytoplankton and fish, cannot be observed from satellites. Zooplankton can readily be monitored over local scales using nets and modern imaging and laser systems, but sampling zooplankton over large spatial scales – both abundance and species composition – remains challenging.
Continuous Plankton Recorder Surveys
First routinely deployed in 1931 the Continuous Plankton Recorder (CPR) survey is the longest running, most extensive, marine biological sampling program (Richardson et al., 2006). Uniquely, the CPR collects in situ samples over large spatial scales, allowing species-level identification of plankton composition and abundance. This is possible because the CPR is sufficiently robust to be deployed from commercial ships (ships of opportunity), unaccompanied by researchers, making sample collection cost-efficient over large ocean tracts, although the species-level identification currently necessitates relatively high processing costs per sample. Full technical details of the CPR can be found in Batten et al. (2003a).
The first CPR sampling took place in the North Sea in 1931, followed by a network of transects around the United Kingdom which extended over the European shelf by the late 1940s. Further expansion to the western North Atlantic occurred next, followed by the first independent regional survey in 1961 off the east coast of the United States. Over time, additional regional surveys have extended CPR operations to northern and southern hemispheres, the North Atlantic and Pacific Oceans as well as smaller regional seas (Figure 1).
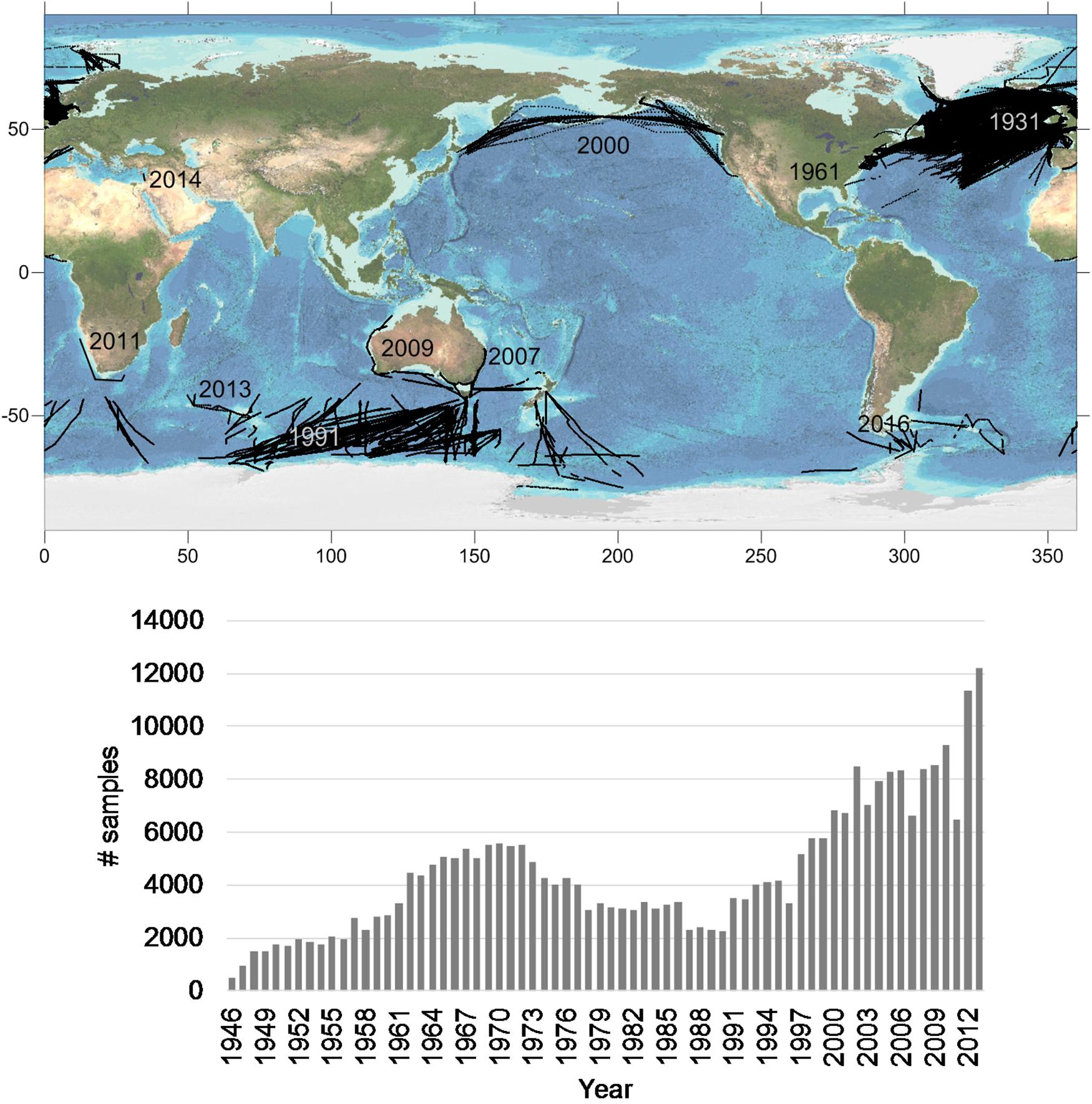
Figure 1. Upper panel shows the CPR transect locations together with the year of inception of that local survey. Lower panel shows the combined total number of CPR samples that have had plankton counts determined.
Strengths and limitations of CPR sampling are well documented in the CPR literature (e.g., Richardson et al., 2006) and will not be repeated here but its specifications mean that only a portion of the entire plankton community is sampled, a fact that is common to all plankton sampling strategies (Wiebe and Benfield, 2003). The CPR filters plankton from the seawater using a mesh with a nominal size of 270 μm [although because of the silk weave it commonly captures phytoplankton down to ∼10 μm (Richardson et al., 2006)]. The seawater entrance aperture has sides of 1.2 cm. There are, therefore, upper and lower size limits of organisms that can be effectively captured and retained. The preservative used is formaldehyde which works well for some species, but not for others. The high speed of sampling (up to 25 knots) means that fragile and gelatinous groups are often damaged or destroyed. The CPR is towed at a fixed near-surface depth (5–10 m) meaning it only captures those taxa that spend some of their time in the mixed layer. Despite these limitations the sampler has changed relatively little since its inception and is internally consistent. Many hundreds of taxa are routinely identified from CPR samples, resulting in a rich ecological dataset of unparalleled spatial extent allowing the identification of changes in plankton communities over large space and time scales, such as multi-decadal and ocean basin. There is a diverse scientific literature based on these data of over 1,000 peer-reviewed publications (a selection is presented in Table 1). It should also be noted that the CPR is not an appropriate sampler for very shallow, near-shore regions, where transect lengths are less than about 100 km or for station-based sampling. However, through collaborative approaches suggested in section “The Future,” CPR data could provide larger scale context or link distant sampling locations where other samplers are used.
Initiating the Global Alliance of CPR Surveys
At the 2009 Global Ocean Ecosystem Dynamics (GLOBEC) Open Science Meeting in Victoria, Canada, CPR users from regional surveys met to examine new results and to begin discussions on stronger links between surveys and how it may be possible to integrate their products (Batten and Burkill, 2010). Two years later, in September 2011, the Global Alliance of CPR surveys (GACS) had its first meeting and signed a Memorandum of Understanding to work toward providing an integrated data set derived from the several national CPR Surveys that currently operated or were planned in the near future. It was anticipated that each of these surveys would continue to operate independently but with increasing emphasis for their contribution to the global perspective. There were six objectives that were laid out as targets;
(1) A common aim “to understand changes in plankton biodiversity at ocean basin scales through a global alliance of CPR Surveys”.
(2) Adoption of common standards and procedures wherever possible.
(3) The generation of a plankton biodiversity database that would ultimately be made freely available to the science community.
(4) The setting up of a website for publicity and data access.
(5) The production of a regular Ecological Status Report on Global Plankton Biodiversity.
(6) An interface between plankton biodiversity and other global ocean observation programs.
Current Status: Successes and Stumbling Blocks
Nine independent regional CPR surveys currently exist which are members of GACS (Figure 1, upper panel). One survey has ceased operation since GACS was formed (the east coast of the United States) but some sampling has been maintained there by the United Kingdom CPR survey. The proportion of collected samples that are analyzed for taxonomic abundance differs between surveys but the lower panel of Figure 1 shows the annual total of analyzed samples, globally, to the year when all surveys have reported data. Samples that are collected but not analyzed are for the most part archived and can be used for additional studies. The total of collected and archived samples is about twice the number shown in Figure 1.
Many of the surveys have started relatively recently; however, there are now almost two decades with more than 5,000 analyzed samples per year that are spread over at least 3 regions (regional seas or ocean basins) and both hemispheres. Funding is the largest limitation to further expansion of CPR surveys; there is strong competition for available funds and there is a (false) perception that it takes many years to realize the benefits of a new CPR survey. There are many issues apart from long-term changes that can be addressed by young CPR surveys (see section “The Diversity of Applications of CPR Data” for examples). CPR surveys that have ceased operation have done so not because of lack of scientific merit, but because of a paucity of funding.
Resources have also limited the speed at which the global CPR database has been developed. Building the infrastructure to link the regional surveys relies not just on physical hardware and person-time in the GACS host institution but also person-time and expertise at each regional survey to format and deliver the data, some of which consist of only one Principal Investigator with many competing requirements for their time. While the benefits to participating in GACS and contributing to a global system are clear to all the scientists involved it is nonetheless not a small task for most to isolate resources for this process when the funding source may have an entirely national or regional focus. Creation of the CPR global database is a significant step for marine ecology as the only other plankton data at a similar spatial scale is currently from remote sensing. Although satellite data have global ocean coverage, they can only provide information on phytoplankton biomass and a few key functional groups, and cannot be used to examine oceanic or trans-oceanic changes in species and, therefore, biodiversity. Once the global CPR database is fully developed it can be interrogated to support integrated global analyses of CPR data, furthering understanding of near-global scale plankton dynamics and of inter-regional connectivity of pelagic habitats.
Defining Best Practices, Capacity Building and New Surveys
Continuous Plankton Recorder surveys share many methodological similarities. These include the CPR device itself [the same design is used by all surveys except the Southern Ocean (SO) surveys, which use a slightly modified version for deployment in sea ice], the silk mesh for capturing plankton (all sourced from the parent organization), and methods for phytoplankton counting [see Richardson et al. (2006) for details]. New surveys, however, are not constrained by maintaining the consistency of a time series and can modify the methods to better address their primary research questions. For example, surveys have different frequencies of deployment and this is not only related to cost: some surveys (e.g., in the North Atlantic) tow monthly to address questions concerning phenology and succession in temperate/polar regions, while other surveys e.g., Australian CPR survey (AusCPR) tow every 3 months in (less seasonal) tropical regions to address inter-annual variation. Another difference among surveys is the method of zooplankton counting: some surveys in (diverse) tropical regions wash the plankton off the mesh for identification of taxa to a higher taxonomic level because the focus is on changes in diversity with climate change (e.g., AusCPR) or to avoid the need for a purpose-built microscope (e.g., New Zealand CPR and SO-CPR), while other surveys complete on-mesh analysis (which is more challenging for species-level identification of smaller species) that is well-suited to the larger organisms and lower diversity of temperate regions (e.g., the North Atlantic). Therefore, surveys in different regions have bespoke research questions, and thus some of the detailed methodology has been modified accordingly, precluding the use of identical methods across all surveys. However, these methodological differences can be viewed as being akin to measuring temperature in the ocean on different platforms (e.g., satellites, moorings, XBTs, or Argo floats) at different spatial and temporal resolutions – each platform by itself is useful for answering specific questions, but their data can be successfully integrated into global temperature products. The similarity of the same sampling device – the CPR – and the species-level plankton identification – are key to the comparability of the data. This comes with some caveats though, as abundances from the CPR are semi-quantitative, providing consistent information on spatial and temporal variation, but not on absolute abundance, which is usually better measured with other sampling techniques (Clark et al., 2001; John et al., 2001; Batten et al., 2003a; Richardson et al., 2004, 2006; Lewis et al., 2006).
An early focus of GACS has been to prepare manuals and materials to document the standard procedures used in all aspects of CPR deployment, maintenance and sample processing and archiving by the North Atlantic survey at the Marine Biological Association, United Kingdom [now home to the former Sir Alister Hardy Foundation for Ocean Science (SAHFOS) that also hosts GACS]. Technicians from several nations that have subsequently initiated new surveys have attended training courses held by the North Atlantic survey. A short-term goal is to make those documents accessible to all through the Ocean Best Practices Repository.
The Diversity of Applications of CPR Data
Continuous Plankton Recorder data have been used to study a multitude of scientific and societal questions (Table 1). The assessment of global issues such as climate change (e.g., Hinder et al., 2014) and fisheries (e.g., Batten et al., 2018) provide the means to recognize similar trends in different areas. The survey has also been successful in evaluating regional stressors (e.g., Vezzulli et al., 2016) or distinct events with globally applicable results or methodologies (Hallegraeff et al., 2014). Here (Table 1) we do not aim to provide an exhaustive list of the CPR bibliography but rather a sample of the breadth and depth of knowledge produced through the various surveys.
Review of the Different Funding Models
Historically, the major challenge facing sustained ocean observing programs is to attract long-term funding (Duarte et al., 2009; Koslow and Couture, 2013). Physical oceanographic components of GOOS have been funded by national governments rather than by international organizations such as the UN or World Bank, and this has also been the case for CPR surveys. CPR surveys have a range of successful funding models, but the most common is some type of funding by national governments through a dedicated program, supplemented by competitive grants and industry collaboration. One successful funding model within Australia has been for the small biological observing community to team up with the physical oceanographic observing community to push for a large and integrated observing system. Thus the Integrated Marine Observing System (IMOS) was born, with ∼$US 11 million per year from the Australian Government and $US14 million per year in matching co-investment (Hill et al., 2010; Moltmann, 2011). All platforms, from physical to biological, have benefitted from being part of a larger integrated and coordinated system, allowing more direct lines of communication and influence with government. The disadvantage is that this single funding source means the program is vulnerable to fluctuating Government budgets.
Another successful model has been industry collaboration. Individual routes in some surveys are supported by the oil and gas industry, in proactive collaborations. For example, British Petroleum has funded an AusCPR route across the Great Australian Bight in southern Australia – a region of developing oil and gas interests – to establish environmental baselines and understand ecosystem connectivity. In the North Atlantic, the CPR route from Aberdeen to the Shetland Islands is funded by the oil and gas exploration company Nexen because it passes close to their drilling platform. Arguably the most challenging pot of money to access is that of national competitive grants, but this has provided additional funding for specific hypothesis-driven research such as work on marine fungal blooms (Australian Research Council funding) and viruses in CPR samples (United Kingdom Natural Environmental Research Council). The North Pacific CPR Survey has been partially funded by the Exxon Valdez Oil Spill (EVOS) program for the past 16 years. This has provided sustained funding toward a North–South route along the United States and Canadian west coasts. Although large-scale environmental disasters are thankfully rare, they do sometimes provide the opportunity for initiating long-term ocean observation. Supplemented by funding from the North Pacific Research Board and the Canadian Government’s Department of Fisheries and Oceans through a consortium the North Pacific Survey has maintained two lengthy transects (including a trans-Pacific route) for 19 years.
It is clear there is no single best way to fund CPR surveys, but having close links with the national research community involved in ocean observation, being responsive to short-term local funding priorities, and partnering with industry have all been fruitful approaches for long-term sustained funding.
The First CPR-Based Ecological Status Reports
The translation of scientific jargon into non-technical language is an important challenge in disseminating scientific results to policy makers. An important driver behind the North Atlantic survey’s strategy was to transfer scientific information revealed by CPR data to decision makers in an accessible and useful format. To address this challenge, in the early 2000’s the CPR program (Sir Alister Hardy Foundation for Ocean Science Annual Report, 2002) published its first annual Ecological Status Report. The Ecological Status Reports apply an indicator approach to summarize the status of North Atlantic plankton using data and research from the CPR survey. The indicators were initially developed to monitor annual changes in key attributes of planktonic systems with a particular emphasis on indicators that were relevant to evolving United Kingdom policy and marine ecosystem management. This strategy of using indicators to clearly communicate the relevance of the CPR was particularly important to the funders of the North Atlantic CPR Survey such as the United Kingdom’s Department of Environment, Food, and Rural Affairs (Defra). Defra continue to be the major funder of the North Atlantic CPR survey, with CPR data and science integral to informing United Kingdom and EU policy (see also policy section, “CPR-Derived Metrics in Marine Policy”).
Marine management drivers continue to influence research using CPR Survey data. Management and policy drivers have co-evolved with the survey, from purely a fisheries perspective in the 1940s to a whole ecosystem approach to management in the 21st century (Edwards et al., 2010). This close alignment with management and policy needs and the continued relevance of the CPR survey in providing large-scale evidence of marine ecosystem and anthropogenic changes (Figure 2) is one of the reasons why the North Atlantic CPR Survey has survived for 80 years, when many time-series have not lasted more than a decade (Koslow and Couture, 2013). CPR data (as summary metrics) from several regional surveys have also contributed to the International Group for Marine Ecological Time Series (IGMETS) report. An ongoing effort, this presents an analysis and overview of oceanic trends based on a collection of over 340 in situ marine ecological time series1, and supplemented with satellite-based spatio-temporal SST and chlorophyll background fields (UNESCO, 2017).
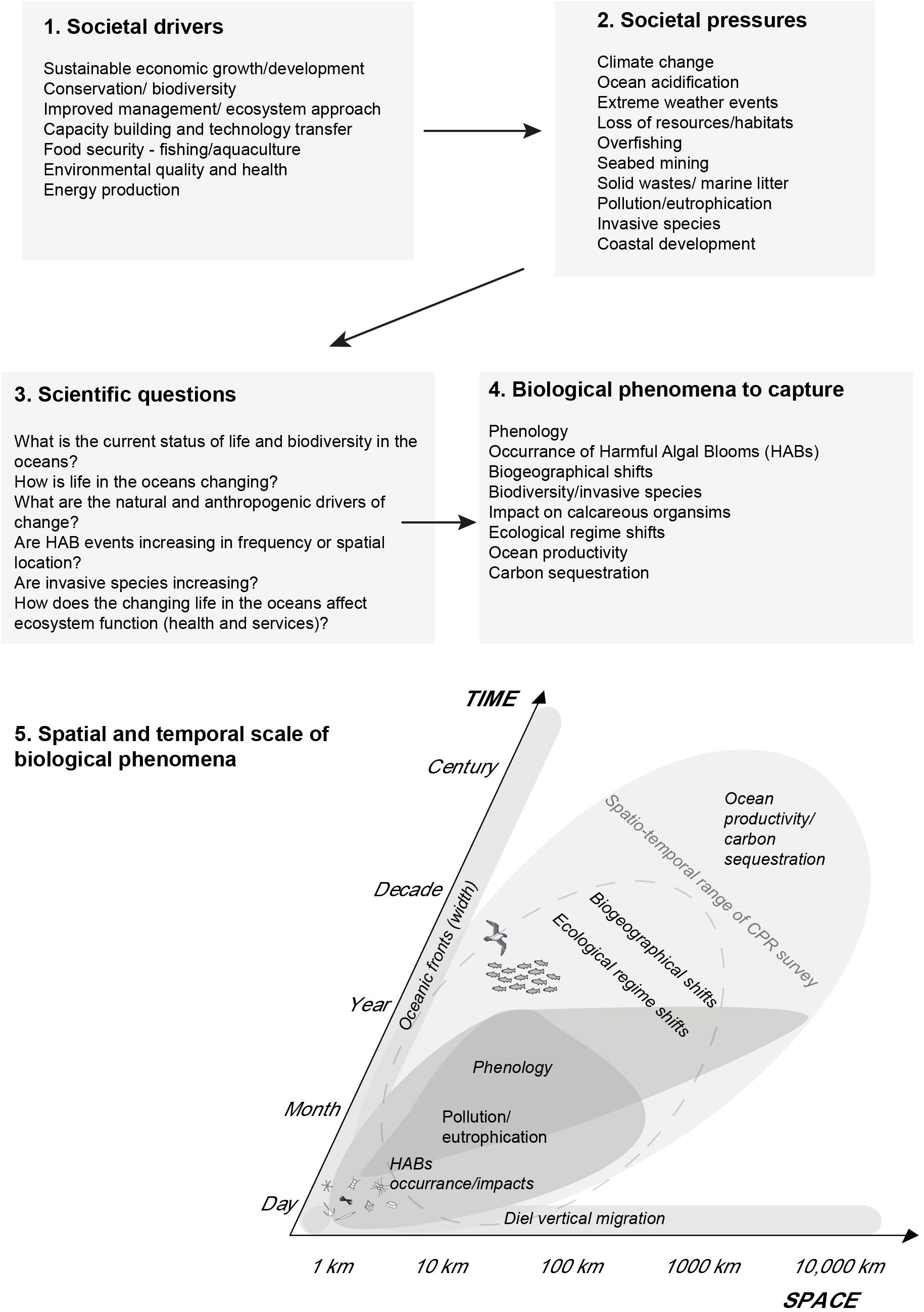
Figure 2. Key societal drivers and pressures on the marine environment and the aspects of plankton dynamics used to capture their impacts on the oceans. The aspects of plankton dynamics addressed occur at multiple spatial and temporal scales and therefore require monitoring by a system, such as the CPR survey network, which operates at similar scales. Based on Edwards et al. (2010).
Since the formation of GACS in 2011 the CPR Ecological Status Reports have been used to report on changes by all the CPR regional surveys and give the international community a global perspective on plankton community change (Edwards et al., 2016). These Global Ecological Status Reports maintain the indicator approach, quantifying marine climate change impacts (biogeographical shifts and phenology), changes in ecosystem health (water quality and marine pathogens), changes in ecosystem state (biodiversity and invasive species). They continue to evolve and adopt new indicators, such as ocean acidification and marine microplastics, as new anthropogenic issues emerge (Edwards et al., 2016). Looking to the future, the Global Ecological Status Reports will be used to report metrics to global initiatives such as the EOVs and EBVs for GOOS, the marine component of GEO BON (MBON, Marine Biodiversity Observation Network) and the IPCC. They also support the recent recommendations made by G7 Ministers of Science which include to “Support an enhanced system of ocean assessment through the UN Regular Process for Global Reporting and Assessment of the State of the Marine Environment that would help develop a consensus view on the state of the oceans on a regular timescale.” This would in turn enable sustainable management strategies to be developed and implemented across the G7 group and beyond.
CPR-Derived Metrics in Marine Policy
The CPR Survey has co-evolved with policy drivers and through the Survey’s development of policy-relevant applied indicators, the CPR has played an integral part in providing relevant, targeted evidence for United Kingdom, European and international decision-makers (Edwards et al., 2010). While CPR data and science have contributed to national ecosystem state assessments in the United Kingdom (United Kingdom Marine Monitoring, and Assessment Strategy [Ukmmas], 2010), United States (e.g., Zador and Yasumiishi, 2017), Canada (Chandler et al., 2017), and Australia (Richardson et al., 2015; Evans et al., 2016), the survey’s transboundary nature has enabled it to play a key role in supporting regional policy and management initiatives. The European Union’s Marine Strategy Framework Directive (MSFD) takes an ecosystem approach to achieving Good Environmental Status in Europe’s regional seas. Uniquely, the CPR survey’s pan-European nature supports collection of plankton data at this regional scale. CPR data were, therefore, fundamental to the conception, development, and delivery of two Northeast Atlantic-wide pelagic habitats indicators for the EU’s Marine Strategy Framework Directive (OSPAR, 2017). The first indicator uses plankton functional groups, or lifeforms, to reveal change in plankton communities while the second uses the Phytoplankton Colour Index and total copepod abundance to assess changes in plankton biomass and abundance (McQuatters-Gollop et al., 2015). Both indicators are dependent on the taxonomic data collected by the CPR, with the underlying genus- and species-level information integral to interpreting indicator change to inform a program of management measures in the United Kingdom and the OSPAR (Northeast Atlantic Regional Seas Commission) region (McQuatters-Gollop et al., 2017).
Another example of a CPR survey’s contribution to regional decision-making is through the SCAR Southern Ocean CPR survey (SO-CPR), which is the major zooplankton monitoring program in the Antarctic region and supports the management of Antarctic biodiversity and resources. The SO-CPR survey includes members from Australia, Japan, Germany, New Zealand, France, South Africa, Brazil, Chile, United Kingdom, United States and Russia and, as of 2018, has collected over 250,000 nautical miles of zooplankton samples (see Hosie et al., 2014, and the SO-CPR Database metadata record at Australian Antarctic Data Center2). The Survey provides data and advice for use by the general Antarctic research community, notably via the Scientific Committee on Antarctic Research (SCAR) as well as to national and intergovernmental organizations within the Antarctic Treaty System such as the Commission for the Conservation of Antarctic Marine Living Resources (CCAMLR), the Committee on Environmental Protection (CEP), the Southern Ocean Observing System (SOOS), the Monitoring program of the Japanese Antarctic Research Expedition (JARE); and the Ministry for Primary Industries project of New Zealand (Robinson et al., 2014).
The extensive spatial scale, multi-decadal time-series, and taxonomic richness of the CPR survey have placed CPR science at the forefront of evidence provision for high-level policy and management advice. Data and research from the survey have informed high profile and strategic global marine assessments such as the IPCC status reports (Pörtner et al., 2014) and the United Nations World Ocean Assessment (Innes et al., 2016). These international assessments are key to raising the profile of marine ecosystem change and are widely read by those on both sides of the science-policy interface. Integrating and analyzing data holistically across the GACS network through a global database with open access to data products would further increase the global impact of CPR data and research.
Instrumenting the CPR
The CPR surveys are best known for taxonomic plankton data based on microscopy including many phytoplankton, hard-shelled microzooplankton and meso-zooplankton. With the developments in technology that have occurred during its history, most notably in the most recent two decades, there has been a push to add supplemental instrumentation which can also collect oceanographic data. Using the CPR itself as a platform takes full advantage of the sampling infrastructure already in place and can extend the types of data collected, both enhancing the understanding of in situ conditions for the plankton communities sampled and maximizing the information that can be gained from having the instrument in the water.
The North Atlantic CPR survey has developed and used a water sampler installed on the CPR body, so far the only automated water sampler that can be deployed on a vessel, external to the ship, whilst still moving. The water samples thus obtained from the English Channel were used to successfully identify planktonic organisms using metagenetic approaches (Stern et al., 2015), revealing a range of unseen diversity not detected by microscopic methods. Additionally, abundance of different size-classes of plankton from flow cytometry analysis of the water samples has been shown to be robust and has revealed new patterns of abundance. Genetic and size-classified biomass data can enhance existing CPR datasets to better model biotic responses to the environment. Alongside a range of “PlankTags” (self-powered instruments that can telemeter data in real-time) and off-the-shelf CTD instruments that measure temperature, salinity and fluorescence, there are a number of biogeochemical sensors that are being tested on the CPR, which can, for example, measure the concentration of carbon dioxide in the seawater.
The Future
Synergies With Satellite Observations
Continuous Plankton Recorder surveys are the program that can provide basin-scale data similar to satellites, but although the temporal scale is far less frequent, CPRs have the advantage of providing species-level taxonomic data. Studies have been carried out that combine CPR and satellite data. Batten et al. (2003b) and Raitsos et al. (2013) used satellite fluorescence data to positively validate the CPR’s Phytoplankton Colour Index, showing that although a simple index, it reveals seasonal and long-term trends in phytoplankton communities. Rêve-Lamarche et al. (2017) used CPR diatom taxonomic data to associate diatom assemblages with specific spectral anomalies (from PHYSAT) for regions of the English Channel and North Sea. The ability to ground-truth satellite-derived phytoplankton functional groups from different regions around the world sampled with CPRs is an attractive idea. Through collaboration with groups such as the International Ocean-Colour Coordinating Group (IOCCG) this is an area that should be further exploited as a short-term goal.
Adding Value to Development of New Sensors and Platforms
There are other simultaneous efforts to improve and extend the measurement of global plankton. The Scientific Committee on Oceanic Research (SCOR) Working Group 154 “Integration of Plankton-Observing Sensor Systems to Existing Global Sampling Programs,” for example, is reviewing the current inventory of state-of-the-art, validated, plankton-related measurements and off-the-shelf sensors. This review will identify those that could be implemented/installed on board research vessels that are operating on other globally coordinated ocean monitoring networks (Boss et al., 2018) such as the Global Ocean Shipped-Based Hydrographic Investigations Program (GO-SHIP), http://www.go-ship.org/ and OceanSITES, http://www.oceansites.org/. GOSHIP co-ordinates trans-basin ship surveys that are repeated at least once every 10 years per transect (frequency of sampling varies). OceanSITES co-ordinates full ocean depth time series observations from moorings and repeat ship visits. The WG will also identify the required resources to support those measurements, as well as the data-dissemination infrastructure, and make recommendations. The WGs approach is to minimize the impact on these existing sampling programs that do not yet record plankton by using self-contained instrumentation. GACS data will be invaluable in providing links between such new measurements that may be temporally sparse (GO-SHIP) or spatially restricted (OceanSITES) with nearby well-established CPR time series as well as provide an historical context. CPR data also provide the species-level information that is often missing with more automated approaches. Many of the developing autonomous systems (boats, gliders, subs, underway systems etc.) still require a significant amount of person time to set-up, supervise and recover. Emerging plankton observing technologies are quickly developing but are often not (yet) robust enough to operate at vessel speeds of more than a few knots, or be deployed unattended for thousands of kilometers. The time will come when they are ready to complement traditional observation systems, but collaboration between networks is essential if we are to link existing and new time series to fully recognize the magnitude of pelagic ecosystem changes.
Instrumentation and Analyses Achievable in the Next Decade
The CPR Survey has already developed qualitative and quantitative assays for microbial pathogens, harmful algae and overall plankton diversity that can be used for indicator development of water quality and ecosystem health. Additionally, assays have been developed to genetically capture plankton diversity from CPR samples, despite their preservation in formaldehyde, allowing for greater scope to fully detect pelagic biodiversity (e.g., Vezzulli et al., 2016). Using an improved filter-based capture method will allow the sampling of greater water volumes which will improve detection rates of species, together with metagenetic detection methods, would provide a new automated method for rapidly monitoring diversity.
The CPR Survey currently deploys PlankTags on nine routes within the North Sea, English Channel and in the N.E. Atlantic. The next generation PlankTags will be able to measure a greater suite of biogeochemical proxies (Conductivity, Temperature, Depth, Chl-a fluorescence) and are designed for trans-oceanic deployment. A methodology for “Macro” FlowCam processing is also being developed in order to explore the size and abundance spectra of zooplankton and plastics from CPR samples (or discrete water samples).
Quantifying the distribution and abundance of plastics within the world ocean has become a necessary demand due to increased concern over potential marine and human health impacts. GACS provides a promising platform to achieve the global coverage required and to develop the CPR protocol further for monitoring large and small plastics that get caught within the CPR. As new technologies capable of identifying the composition of microplastics continue to develop (such as the use of hyper-spectral cameras), these may be able to provide a method to retrospectively analyze historic CPR samples and create a more complete picture and consistent monitoring of the global plastics problem.
Becoming Truly Global
New Surveys to Fill Gaps
A frequently asked question of members of existing CPR surveys is why you would choose to use a CPR when there are many newer plankton samplers in use and under development? The CPR is the method-of-choice for large-scale plankton surveys, which is what is needed for a global program, and no other device currently available delivers similar information at a similar cost. There are four main reasons:
(a) Cost: Research vessel costs for large-scale surveys, (e.g., fisheries surveys) are tens of thousands of dollars a day in most countries. Other than a small gratuity to the crew of the merchant ships, CPR sampling is essentially free. Most current plankton samplers are far too fragile for Ships of Opportunity (SOOP) and require dedicated research ship time, making them far too expensive for long-term, large-scale surveys. While autonomous samplers that can cover reasonably large distances are in the pilot phase (e.g., Ohman et al., 2019) there are still significant start-up, maintenance, and data processing costs. The expense of microscopy required to process CPR samples is offset by the longevity of the instrument. With servicing, the CPR can last decades even when it is deployed monthly on SOOPs and it is highly reliable with a success rate of over 90%. It can also easily be moved between vessels since only a towing point needs to be added, there is no alteration of a ship’s water intake system. Many modern instruments require regular calibration and technician time. Longevity, reliability and low-cost sampling make the CPR particularly good value-for-money.
(b) Species-level taxonomy: Most other modern instruments for zooplankton or phytoplankton do not collect species-level taxonomy. No autonomous vehicle can currently identify phytoplankton or zooplankton to species level. Molecular approaches can identify species, but cannot estimate abundance very well, which is relatively easy with microscopy. Molecular approaches also do not distinguish juveniles from adults, and females from males, which is relatively straightforward with microscopy. So, whilst somewhat labor-intensive, the CPR approach provides highly resolved taxonomic data together with abundances. This is essential for effective biodiversity monitoring.
(c) A physical sample: Many other plankton sampling techniques, such as the Video Plankton Recorder, Optical Plankton Counter, autonomous vehicles, and Imaging Flow Cytobot, extract a measure of the plankton community, but do not collect a sample. Having a physical sample, especially when archived and curated, allows for many additional analyses such as molecular studies, other biochemical assays (stable isotope measurement for example), as well as analyses of taxa that were not able to be counted at the time of sample processing. There are very likely new techniques in the future that are not currently imagined that can also be applied to an archive of physical samples.
(d) Comparative analyses: There is an archive of standardized samples and data from other CPR surveys around the globe for comparison with new results. Wiebe and Benfield (2003) reported that there were then over 150 different zooplankton samplers, with no acknowledged global standard other than the CPR. The ability to place a new regions’ results into a global context will increase the ability to understand a local system.
For all of these reasons, the CPR is the only reliable, robust sampler that can be used over large space and long-time scales – and remains the method-of-choice for new plankton surveys.
Designing the Sampling
An important current gap in the GACS vision of having a global CPR survey is the sampling design. One way to envision such a design is to consider the different bioregions of the ocean. There are several classification systems in use that define marine biogeochemical provinces for the pelagic realm in terms of major oceanographic and ecological patterns: (a) the Longhurst Biogeochemical Provinces (BGCP; Longhurst, 2007), (b) the Marine Ecoregions of the World (MEOW; Spalding et al., 2007), (c) the Large Marine Ecosystems (LME for coastal systems; Sherman, 2005) which also includes socio-economic factors in the delineations. Adding ecological complexity and dynamics to such essentially static systems by combining satellite data and in situ observations has been proposed by Kavanaugh et al. (2016). However, probably the most currently accepted global bio-regionalization for the open ocean sampled by the CPR is the “Longhurst Provinces” (first presented in Longhurst, 1998), which are 56 ecoregions based primarily on the major oceanographic regimes (Figure 3). For a global plankton survey, we might aim for a network that covers all of these provinces. Currently, CPRs sample provinces in the North Atlantic (e.g., NECS, NADR, SARC, and NASE), the Southern Ocean (SANT, ANTA, and NEWZ), around Australia (AUSE, AUSW, and TASM), and the North Pacific (CCAL, PSAE, and NPPF). These are relatively well sampled, but most of the biogeographical provinces are not sampled (Figure 3), including whole parts of the ocean including the South Pacific (SPSG) and the southern Indian Ocean north of the Southern Ocean (ISSG). Coverage of CPR sampling will continue to grow, but we can stimulate its development by learning from the approach of the physical oceanographic research community to building the global observing system for climate. Beginning in 1997, the community released a blueprint for what the global observing system for ocean climate would look like, detailing the needed temporal and spatial coverage of its major platforms (National Research Council, 1997). Not only was this global system designed through community discussion, but by simulated sampling of temperature and salinity by different platforms from output of hydrographic models. This enabled an objective design of the system, based on the needed precision of the data products. It also provided a target that could be tracked through time – motivating the research community and focusing the attention of funding bodies. For example, the ARGO network, which had only 544 floats in 2002, reached its design specification of 3,000 floats globally in 2007, and has maintained this coverage ever since.
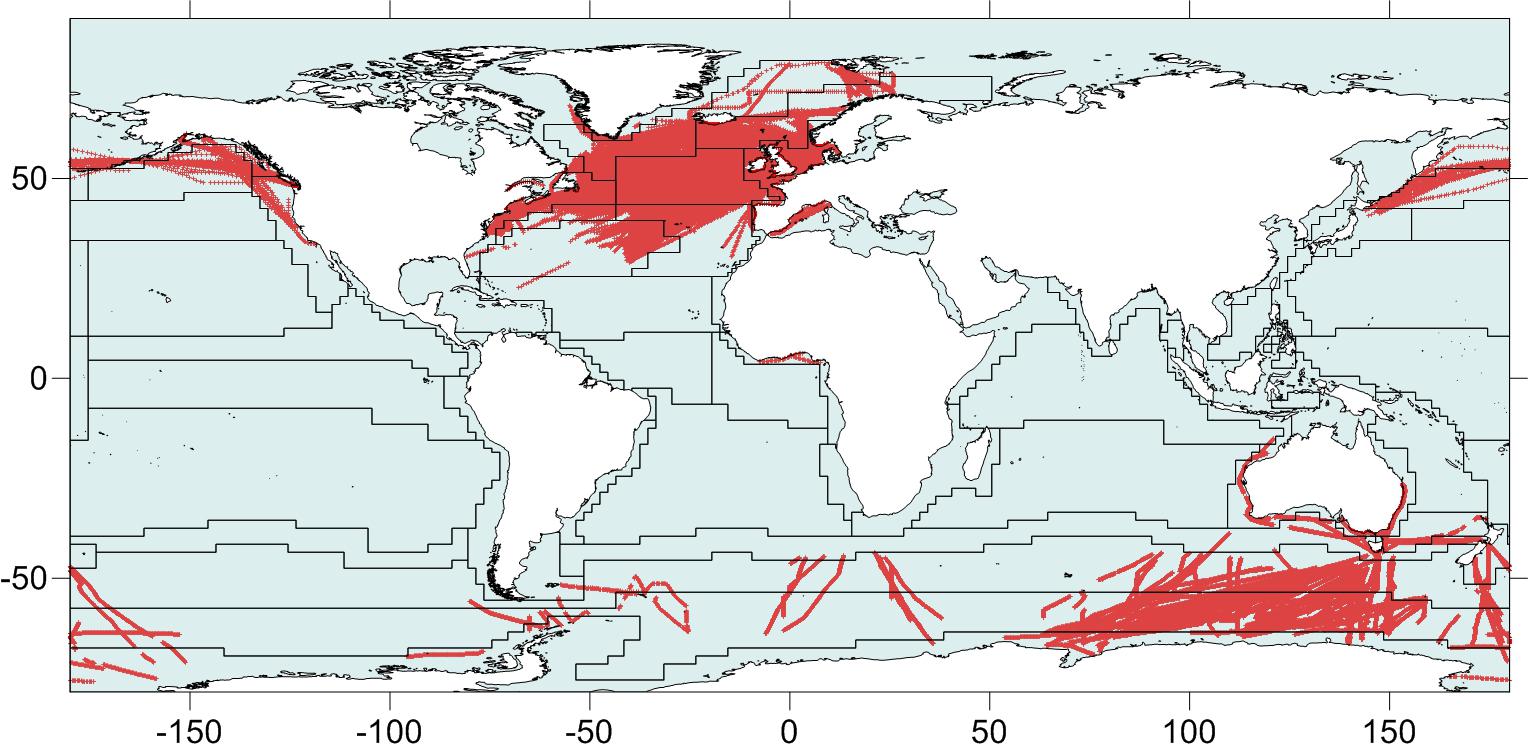
Figure 3. Longhurst Provinces (Flanders Marine Institute, 2009) overlaying CPR sample locations (red). Names of provinces are not shown to avoid clutter but can be found at http://www.marineregions.org/images/boundaries/Longhurst_crop.png.
As the physical oceanographic research community used hydrodynamic models that capture the time and space scales of variation in temperature and salinity to design the physical components of GOOS, so the biological oceanographic research community can use global biogeochemical and ecosystem models that incorporate plankton functional types to inform the design of a global plankton observing system. There might be different designs for different objectives. For example, a key objective might be to measure the planktonic component of the carbon cycle, and we could use biogeochemical models to estimate the global coverage and frequency of observations of the critical zooplankton functional groups. Another key objective might be to measure zooplankton productivity supporting fisheries and we could use ecosystem models that include plankton and fish for this purpose. In this way, we could develop a design – or an amalgamation of a few designs – for a global plankton observing system. Different plankton sampling methods (say time series from nets or zooplankton size spectra from LOPC) can be integrated into a global observing program, although they each measure different yet complementary aspects of the zooplankton community, as do the different oceanographic platforms that currently measure temperature and salinity in GOOS. The key might not be the design itself, but that there is a coherent, defensible vision that the international community could own and promote. Such a design would also provide target against which progress could be measured.
Delivering Indicators for Global Marine Policy
The CPR survey’s scale is approaching global coverage and so the survey is uniquely placed to inform transboundary, basin-scale, ocean-scale, and even global-scale management efforts. Besides climate change impacts, which are indeed global, many human induced pressures on the marine environment and biodiversity are transboundary across the EEZs of multiple-countries and from EEZs to the high seas as well, e.g., chemical and debris pollution, fisheries, maritime operations and offshore industries. International policy mechanisms should be established to ensure effective conservation and management planning of global marine ecosystems. The need for such a globally integrated mechanism has been further recognized since the Ocean Summit in 2017 where the UN agreed their commitment to maintain and achieve a “healthy ocean.” The spatial extent of the Global Alliance of CPR Surveys enables coherence between different projects for assessments contributing to international high-level policy and management initiatives.
Several opportunities for contributions of the CPR to global policy mechanisms exist in UN led agendas such as the 2030 Agenda on Sustainable Development Goals (SGDs3), Convention of Biological Diversity (CBD) post-2020 global biodiversity framework (Convention on Biological Diversity, 2017), and the conservation and sustainable use of marine biological diversity Beyond Boundaries of National Jurisdiction (BBNJ4). For SDG14: Life Below Water, CPR data can provide scientific evidence useful in development of global indicators to report the achievement for the Goal 14.1 on pollution, 14.2 on ecosystem-based approaches, 14.3 on ocean acidification, and 14.5 on marine protected areas. Such indicators could be developed and assessed at the regional or basin scale and reported through national mechanisms, enabling direct comparability between seas and national waters and allowing examination of change in a global context. Plankton information including the CPR data are currently not used in the global indicator suites of the current CBD framework or Aichi Targets despite the fact that the CPR’s scientific quality and data coverage could exceed the requirement of these indicators (Chiba et al., 2018). This issue may be solved in the post-2020 framework in which a more harmonized collaboration of different UN organizations, such as IOC-GOOS and UNEP, will be expected.
It is worth noting that both in the SDGs and CBD many of the established or proposed global indicators are to indicate the “response” of society, while the development of robust “state” indicators to indicate the status of the ecosystem and biodiversity, and which are needed to fill the gap of the indicator suites particularly in the marine realm, have not yet been specified. One way to promote this will be by establishing protocols that streamline GOOS EOVs to global indicators. Robust indicators will be developed by coupling plankton EOVs and physical and biogeochemical EOVs (part of the GOOS 2030 strategy, in prep.). This will also strengthen the current Framework for Ocean Observing scheme of global ocean observation (Lindstrom et al., 2012), which has not yet identified the explicit methodology/strategy in feedback of the observation outcome (data) to policy.
Finally, global CPR data can provide scientific evidence useful for negotiating BBNJ where a lack of biodiversity data in the High Seas makes assessment of ecosystems in the High Seas difficult (United Nations, 2017), and has been one of the obstacles for establishment of the internationally agreed (Wright et al., 2016), effective conservation and management policy of BBNJ.
Conclusion
“Locally Strong, Globally Connected” is the rationale that underpins GACS and it remains the best way to develop a global plankton diversity monitoring network. The decade since OceanObs 2009 has seen dramatic changes in the coverage of CPR surveys, collaborative studies and in the degree to which the data are applied to marine resource management policies. As the biological focus of the GOOS matures during the next decade, and with the UN Decade of Ocean Science for Sustainable Development (2021–2030) about to start, the importance of extending GACS and realizing its full potential could not be greater, nor more timely. A global network of CPR surveys has been initiated. What is needed now is a coordinated approach; to fill gaps in current coverage of large ocean tracts, integrate with other plankton sampling programs that operate in regions not appropriate for CPRs, ground-truth emerging technologies and satellite observations, and integrate with other Essential Ocean Variables to build an efficient global observing program for the open ocean.
Author Contributions
SB contributed to significant pieces of text throughout the study. AM-G and SC contributed to the policy sections. ME contributed to the ESR section. RA-A contributed to Table 1. AR contributed to the sections “Review of the Different Funding Models” and “Becoming Truly Global.” GG, RJ, JK, PK, EM, CO, KR, KT, HV, and WW contributed to shorter contributions sections throughout the study.
Conflict of Interest Statement
The authors declare that the research was conducted in the absence of any commercial or financial relationships that could be construed as a potential conflict of interest.
Acknowledgments
The authors are grateful to the past and present volunteer ships and their officers and crews that have towed CPRs and contributed to the GACS dataset. They are grateful to the two reviewers and the editor whose comments have helped in considerable improvement of the manuscript.
Footnotes
- ^ https://igmets.net/report
- ^ https://data.aad.gov.au/metadata/records/AADC-00099
- ^ https://www.un.org/sustainabledevelopment/sustainable-development-goals/
- ^ https://www.un.org/bbnj/
References
Batten, S. D., Allen, R. J. S., and Wotton, C. O. M. (1998). The effects of the Sea Empress oil spill on the plankton of the Southern Irish Sea. Mar. Pollut. Bull. Pergamon 36, 764–774. doi: 10.1016/S0025-326X(98)00039-3
Batten, S. D., and Burkill, P. H. (2010). The continuous plankton recorder: towards a global perspective. J. Plankt. Res. 2010, 1619–1621. doi: 10.1093/plankt/fbq140
Batten, S. D., Clarke, R. A., Flinkman, J., Hays, G. C., John, E. H., John, A. W. G., et al. (2003a). CPR sampling – The technical background, materials and methods, consistency and comparability. Prog. Oceanogr. 58, 193–215. doi: 10.1016/j.pocean.2003.08.004
Batten, S. D., Walne, A. W., Edwards, M., and Groom, S. B. (2003b). Phytoplankton biomass from continuous plankton recorder data: an assessment of the phytoplankton colour index. J. Plankt. Res. 25, 697–702. doi: 10.1093/plankt/25.7.697
Batten, S. D., Ruggerone, G. T., and Ortiz, I. (2018). Pink Salmon induce a trophic cascade in plankton populations in the southern Bering Sea and around the Aleutian Islands. Fish. Oceanogr. 27, 548–559. doi: 10.1111/fog.12276
Beaugrand, G., Brander, K. M., Lindley, J. A., Souissi, S., and Reid, P. C. (2003). Plankton effect on cod recruitment in the North Sea. Nature 426, 661–664. doi: 10.1038/nature02164
Beaugrand, G., Reid, P. C., Ibanez, F., Lindley, A. J., and Edwards, M. (2002). Reorganization of North Atlantic marine copepod biodiversity and climate. Science 296, 1692–1694. doi: 10.1126/science.1071329
Bedford, J., Johns, D., Greenstreet, S., and McQuatters-Gollop, A. (2018). Plankton as prevailing conditions: a surveillance role for plankton indicators within the marine strategy framework Directive. Mar. Policy 89, 109–115. doi: 10.1016/j.marpol.2017.12.021
Boalch, G. T., and Harbour, D. S. (1977). Unusual diatom off the coast of south-west England and its effect on fishing. Nature 269, 687–688. doi: 10.1038/269687a0
Boss, E., Waite, A., Muller-Karger, F., Yamazaki, H., Wanninkhof, R., Uitz, J., et al. (2018). Beyond chlorophyll fluorescence: the time is right to expand biological measurements in ocean observing programs. Limnol. Oceanogr. Bull. 27, 89–90. doi: 10.1002/lob.10243
Brewin, R. J. W., Sathyendranath, S., Hirata, T., Lavender, S. J., Barciela, R., and Hardman-Mountford, N. J. (2010). A three-component model of phytoplankton size class for the Atlantic Ocean. Ecol. Model. 221, 1472–1483. doi: 10.1016/j.ecolmodel.2010.02.014
Chandler, P. C., King, S. A., and Boldt, J. (eds) (2017). State of the physical, biological and selected fishery resources of Pacific Canadian marine ecosystems in 2016. Can Tech. Rep. Fish. Aquat. Sci. 3225:243.
Chiba, S., Batten, S., Martin, C. S., Ivory, S., Miloslavich, P., and Weatherdon, L. V. (2018). Zooplankton monitoring to contribute towards addressing global biodiversity conservation challenges. J. Plankt. Res. 40, 509–518. doi: 10.1093/plankt/fby030
Clark, R. A., Frid, C. L. J., and Batten, S. (2001). A critical comparison of two long-term zooplankton time series from the central-west North Sea. J. Plank. Res. 23, 27–39. doi: 10.1093/plankt/23.1.27
Convention on Biological Diversity (2017). Recommendation Adopted by the Subsidiary Body on Science, Technical and Technological Advice. Available at: https://www.cbd.int/doc/recommendations/sbstta-21/sbstta-21-rec-01-en.pdf (accessed October, 2018).
Di Cesare, A., Petrin, S., Fontaneto, D., Losasso, C., Eckert, E. M., Tassistro, G., et al. (2018). ddPCR applied on archived continuous plankton recorder samples reveals long-term occurrence of class 1 integrons and a sulphonamide resistance gene in marine plankton communities. Environ. Microbiol. Rep. 10, 458–464. doi: 10.1111/1758-2229.12665
Duarte, C. M., Conley, D. J., Carstensen, J., and Sánchez-Camacho, M. (2009). Return to neverland: shifting baselines affect eutrophication restoration targets. Estuar. Coasts 32, 29–36. doi: 10.1007/s12237-008-9111-2
Edwards, M., Beaugrand, G., Hays, G. C., Koslow, J. A., and Richardson, A. J. (2010). Multi-decadal oceanic ecological datasets and their application in marine policy and management. Trends Ecol. Evol. 25, 602–610. doi: 10.1016/j.tree.2010.07.007
Edwards, M., Helaouet, P., Alhaija, R. A., Batten, S., Beaugrand, G., Chiba, S., et al. (2016). Global Marine Ecological Status Report: Results from the Global CPR Survey 2014/2015. SAHFOS Technical Report, 11, 1–32. Plymouth.
Edwards, M., John, A. W. G., Johns, D. G., and Reid, P. C. (2001). Case history and persistence of the non-indigenous diatom Coscinodiscus wailesii in the north-east Atlantic. J. Mar. Biol. Assoc. 81, 207–211. doi: 10.1017/S0025315401003654
Edwards, M., Johns, D. G., Richardson, A. J., Leterme, S. C., Svendsen, E., and Richardson, A. J. (2006). Regional climate change and harmful algal blooms in the northeast Atlantic. Limnol. Oceanogr. 51, 820–829. doi: 10.4319/lo.2006.51.2.0820
Evans, K., Bax, N. J., and Smith, D. C. (2016). Australia state of the environment. Mar. Environ. 226, 134–146. doi: 10.1093/biosci/biw180
Hallegraeff, G., Coman, F., Davies, C., Hayashi, A., Mcleod, D., Slotwinski, A., et al. (2014). Australian dust storm associated with extensive Aspergillus sydowii Fungal “Bloom” in Coastal Waters. Appl. Environ. Microbiol. 80, 3315–3320. doi: 10.1128/AEM.04118-13
Hill, K., Moltmann, T., Proctor, R., and Allen, S. (2010). The Australian integrated marine observing system: delivering data streams to address national and international research priorities. Mar. Tech. Soc. J. 44, 65–72. doi: 10.403/MTSJ.44.6.13
Hinder, S. L., Gravenor, M. B., Edwards, M., Ostle, C., Bodger, O. G., Lee, P. L. M., et al. (2014). Multi-decadal range changes vs. thermal adaptation for north east Atlantic oceanic copepods in the face of climate change. Glob. Change Biol. 20, 140–146. doi: 10.1111/gcb.12387
Hosie, G., Mormede, S., Kitchener, J., Takahashi, K., and Raymond, B. (2014). “Near surface zooplankton communities,” in The CAML/SCAR-MarBIN Biogeographic Atlas of the Southern Ocean, eds C. De Broyer, P. Koubbi, H. Griffiths, et al. (Cambridge: Scientific Committee on Antarctic Research).
Innes, L., Simcock, A., Ajawin, A. Y., Alcala, A. C., Bernal, P., Calumpong, H. P., et al. (2016). The First Global Integrated Marine Assessment: World Ocean Assessment 1. New York, NY: United Nations.
John, E. H., Batten, S. D., Harris, R. P., and Hays, G. C. (2001). Comparison between zooplankton data collected by the Continuous Plankton Recorder survey in the English Channel and by WP-2 nets at station L4, Plymouth (UK). J. Sea Res. 46, 223–232. doi: 10.1016/S1385-1101(01)00085-5
Kavanaugh, M. T., Oliver, M. J., Chavez, F. P., Letelier, R. M., Muller-Karger, F. E., and Doney, S. C. (2016). Seascapes as a new vernacular for pelagic ocean monitoring, management and conservation. ICES J. Mar. Sci. 73, 1839–1850. doi: 10.1093/icesjms/fsw086
Koslow, J. A. (1983). Zooplankton community structure in the north sea and northeast atlantic: development and test of a biological model. Can. J. Fish. Aquat. Sci. 40, 1912–1924. doi: 10.1139/f83-222
Koslow, J. A., and Couture, J. (2013). Ocean sciences: follow the fish. Nature 502, 163–164. doi: 10.1038/502163a
Lewis, K., Allen, J. I., Richardson, A. J., and Holt, J. T. (2006). Error quantification of a high resolution coupled hydrodynamic-ecosystem coastal-ocean model: part 3, validation with continuous plankton recorder data. J. Mar. Syst. 63, 209–224. doi: 10.1016/j.jmarsys.2006.08.001
Lindstrom, E., Gunn, J., Fischer, A., McCurdy, A., and Glover, L. K. (2012). A Framework for Ocean Observing. By the Task Team for an Integrated Framework for Sustained Ocean Observing. Paris: UNESCO.
McQuatters-Gollop, A., Edwards, M., Helaouët, P., Johns, D. G., Owens, N. J. P., Raitsos, D. E., et al. (2015). The continuous plankton recorder survey: how can long-term phytoplankton datasets deliver good environmental status? Estuar. Coast. Shelf Sci. 162, 88–97. doi: 10.1016/j.ecss.2015.05.010
McQuatters-Gollop, A., Johns, D. G., Bresnan, E., Skinner, J., Rombouts, I., Stern, R. F., et al. (2017). From microscope to management: the critical value of plankton taxonomy to marine policy and biodiversity conservation. Mar. Policy 83, 1–10. doi: 10.1016/j.marpol.2017.05.022
McQuatters-Gollop, A., Raitsos, D. E., Edwards, M., Pradhan, Y., Mee, L. D., Lavender, S. J., et al. (2007). A long-term chlorophyll dataset reveals regime shift in North Sea phytoplankton biomass unconnected to nutrient levels. Limnol. Oceanogr. 52, 635–648. doi: 10.4319/lo.2007.52.2.0635
Miesner, A. K., and Payne, M. R. (2018). Oceanographic variability shapes the spawning distribution of blue whiting (Micromesistius poutassou). Fish. Oceanogr. 27, 623–638. doi: 10.1111/fog.12382
Miloslavich, P., Bax, N. J., Simmons, S. E., Klein, E., Appeltans, W., Aburto-Oropeza, O., et al. (2018). Essential ocean variables for global sustained observations of biodiversity and ecosystem changes. Glob. Change Biol. 2018, 2416–2433. doi: 10.1111/gcb.14108
Moltmann, T. (2011). “Ocean observing systems a national perspective from Australia,” in Proceedings of the GEOSS Workshop XLII - Oceans, (Kona, HI: IEEE), doi: 10.1109/GEOSS-XLII.2011.6105429
National Research Council (1997). The Global Ocean Observing System: Users, Benefits, and Priorities. Washington, DC: The National Academies Press, doi: 10.17226/5580
Ohman, M. D., Davis, R. E., Sherman, J. T., Grindley, K. R., Whitmore, B. M., Nickels, C. F., et al. (2019). Zooglider: An autonomous vehicle for optical and acoustic sensing of zooplankton. Limnol. Oceanogr. Methods 17, 69–86. doi: 10.1002/lom3.10301
OSPAR (2017). Intermediate Assessment 2017. Available at: https://oap.ospar.org/en/ospar-assessments/intermediate-assessment-2017 (accessed October, 2018).
Planque, B., and Fromentin, J. M. (1996). Calanus and environment in the eastern North Atlantic.1. Spatial and temporal patterns of C-finmarchicus and C-helgolandicus. Mar. Ecol. Prog. Ser. 134, 101–109. doi: 10.3354/meps134101
Pörtner, H.-O., Karl, D. M., Boyd, P. W., Cheung, W. W. L., Lluch-Cota, S. E., Nojiri, Y., et al. (eds) (2014). “Ocean systems,” in Climate Change 2014: Impacts, Adaptation, and Vulnerability. Part A: Global and Sectoral Aspects. Contribution of Working Group II to the Fifth Assessment Report of the Intergovernmental Panel on Climate Change, eds C. B. Field, V. R. Barros, D. J. Dokken, (Cambridge: Cambridge University Press),411–484.
Raitsos, D. E., Walne, A., Lavender, S. J., Licandro, P., Reid, P. C., and Edwards, M. (2013). A 60-year ocean colour data set from the continuous plankton recorder. J. Plankt. Res. 35, 158–164. doi: 10.1093/plankt/fbs079
Reid, P. C., Battle, E. J. V., Batten, S. D., and Brander, K. M. (2000). Impacts of fisheries on plankton community structure. ICES J. Mar. Sci. 57, 495–502. doi: 10.1006/jmsc.2000.0740
Rêve-Lamarche, A.-H., Séverine, A., Racault, M.-F., Dessailly, D., Guiselin, N., Jamet, C., et al. (2017). Estimation of the potential detection of diatom assemblages based on ocean color radiance anomalies in the North Sea. Front. Mar. Sci. 4:408. doi: 10.3389/fmars.2017.00408
Richardson, A. J., Eriksen, R. S., and Rochester, W. (2015). Plankton 2015: State of Australia’s oceans. CSIRO Report. Canberra: CSIRO.
Richardson, A. J., John, E. H., Irigoien, X., Harris, R. P., and Hays, G. C. (2004). How well does the Continuous Plankton Recorder (CPR) sample zooplankton? A comparison with the Longhurst Hardy Plankton Recorder (LHPR) in the northeast Atlantic. Deep Sea Res. Part 1 Oceanogr. Res. Pap. 51, 1283–1294. doi: 10.1016/j.dsr.2004.04.002
Richardson, A. J., Walne, A. W., John, A. W. G., Jonas, T. D., Lindley, J. A., Sims, D. W., et al. (2006). Using continuous plankton recorder data. Prog. Oceanogr. 68, 27–74. doi: 10.1016/j.pocean.2005.09.011
Richardson, A. J., and Schoeman, D. S. (2004). Climate impact on plankton ecosystems in the northeast Atlantic. Science 305, 1609–1612. doi: 10.1126/science.1100958
Robinson, K. V., Pinkerton, M. H., Hall, J. A., and Hosie, G. W. (2014). Continuous Plankton Recorder Time Series. New Zealand Aquatic Environment and Biodiversity Report No. 128. Wellington: Ministry for PrimaryIndustries.
Schartup, A. T., Qureshi, A., Dassuncao, C., Thackray, C. P., Harding, G., and Sunderland, E. M. (2018). A model for Methylmercury uptake and trophic transfer by marine plankton. Environ. Sci. Technol. 52, 654–662. doi: 10.1021/acs.est.7b03821
Schminke, H. K. (2007). Entomology for the copepodologist. J. Plankt. Res. 29, i149–i162. doi: 10.1093/plankt/fbl073
Sherman, K. (2005). “The large marine ecosystem approach for assessment and management of ocean coastal waters,” in Sustaining Large Marine Ecosystems: the Human Dimension. eds T. Hennessey and J. Sutinen (Amsterdam: Elsevier), 3–16. doi: 10.1016/s1570-0461(05)80025-4
Skerratt, J. H., Mongin, M., Wild-Allen, K. A., Baird, M. E., Robson, B. J., Schaffelke, B., et al. (2019). Plankton and nutrient dynamics on the Great Barrier Reef: Skill assessment and analysis of the eReefs biogeochemical model. J. Mar. Syst. 192, 51–74.
Spalding, M. D., Fox, H. E., Allen, G. R., Davidson, N., Ferdaña, Z. A., Finlayson, M. A. X., et al. (2007). Marine ecoregions of the World: a bioregionalization of coastal and shelf areas. BioScience 57, 573–583. doi: 10.1641/b570707
Stern, R. F., Picard, K. T., Hamilton, K. M., Walne, A., Tarran, G. A., Mills, D., et al. (2015). Novel lineage patterns from an automated water sampler to probe marine microbial biodiversity with ships of opportunity. Prog. Oceanogr. 137, 409–420. doi: 10.1016/j.pocean.2015.04.015
Thompson, R. C., Olsen, Y., Mitchell, R. P., Davis, A., Steven, J., John, A. W. G., et al. (2004). Lost at sea: where is all the plastic? Science 304:838. doi: 10.1126/science.1094559
UNESCO (2017). What are Marine Ecological Time Series telling us about the ocean? A status report, eds T. D. O’Brien, L. Lorenzoni, K. Isensee, and L. Valdés (Paris: IOC-UNESCO), 171–190.
United Kingdom Marine Monitoring, and Assessment Strategy [Ukmmas] (2010). Charting Progress 2: The state of UK seas. An overview. London: Defra.
United Nations (2017). The First Global Integrated Marine Assessment. Cambridge: Cambridge University Press.
Vezzulli, L., Grande, C., Reid, P. C., Hélaouët, P., Edwards, M., Höfle, M. G., et al. (2016). Climate influence on Vibrio and associated human diseases during the past half-century in the coastal North Atlantic. Proc. Natl. Acad. Sci. U.S.A. 113, E5062–E5071. doi: 10.1073/pnas.1609157113
Wiebe, P. H., and Benfield, M. C. (2003). From the Hensen net toward four-dimensional biological oceanography. Prog. Oceanogr. 56, 7–136. doi: 10.1016/S0079-6611(02)00140-4
Wright, G., Rochette, J., Druel, E., and Gjerde, K. (2016). The Long and Winding Road Continues: Towards a New Agreement on High Seas Governance. Paris: IDDRI.
Keywords: Continuous Plankton Recorder, zooplankton, phytoplankton, global monitoring, biodiversity, ocean observing, essential ocean variables
Citation: Batten SD, Abu-Alhaija R, Chiba S, Edwards M, Graham G, Jyothibabu R, Kitchener JA, Koubbi P, McQuatters-Gollop A, Muxagata E, Ostle C, Richardson AJ, Robinson KV, Takahashi KT, Verheye HM and Wilson W (2019) A Global Plankton Diversity Monitoring Program. Front. Mar. Sci. 6:321. doi: 10.3389/fmars.2019.00321
Received: 28 October 2018; Accepted: 28 May 2019;
Published: 14 June 2019.
Edited by:
Frank Edgar Muller-Karger, University of South Florida, United StatesReviewed by:
Jaimie Rojas-Marquez, La Salle Foundation of Natural Sciences, VenezuelaTodd D. O’Brien, National Oceanic and Atmospheric Administration (NOAA), United States
Copyright © 2019 Batten, Abu-Alhaija, Chiba, Edwards, Graham, Jyothibabu, Kitchener, Koubbi, McQuatters-Gollop, Muxagata, Ostle, Richardson, Robinson, Takahashi, Verheye and Wilson. This is an open-access article distributed under the terms of the Creative Commons Attribution License (CC BY). The use, distribution or reproduction in other forums is permitted, provided the original author(s) and the copyright owner(s) are credited and that the original publication in this journal is cited, in accordance with accepted academic practice. No use, distribution or reproduction is permitted which does not comply with these terms.
*Correspondence: Sonia D. Batten, c29uaWEuYmF0dGVuQG1iYS5hYy51aw==