- 1Department of Animal Ecology and Systematics, Faculty of Biology and Chemistry, Justus Liebig University Giessen, Giessen, Germany
- 2Departamento Ciencias Biológicas, Laboratorio de Biología Molecular Marina (BIOMMAR), Universidad de los Andes, Bogotá, Colombia
- 3Institute of Marine Sciences, Consejo Superior de Investigaciones Científicas, Barcelona, Spain
Gorgonian corals occurring in shallow waters are vulnerable to changing environmental conditions and human-related pressures such as pollution, overfishing, and diseases. However, anthropogenic effects on coral systems are difficult to quantify due to the lack of base-line data of unaffected populations. In order to assess the impact of global and local environmental parameters on gorgonian populations removed from direct anthropogenic impact, we evaluated demographic parameters and the health status of Pacifigorgia cairnsi (Gorgoniidae: Octocorallia) populations in Malpelo Island, a remote and pristine marine area in the Tropical Eastern Pacific of Colombia. Specifically, we studied P. cairnsi densities and population size structures under different habitat and local environmental conditions. We also studied whether ENSO events and local hydrodynamic features including locality, water depth, and upwelling conditions drive P. cairnsi growth rates. Finally, we evaluated the prevalence of the necrotic patch disease and rates of disease recovery. Major findings were that local hydrodynamic parameters shaped P. cairnsi size structures, that growth rates were affected by thermal anomalies associated to ENSO events and partly by water depth, that overall disease prevalence was low (6%) and that it did not correlate with the environmental parameters studied, and that most diseased colonies (57%) recovered via tissue breakage. The fact that P. cairnsi, a keystone species within the regional benthic food web, is affected by thermal anomalies remains of concern because these global events are predicted to increase in frequencies and severity in the future. Nonetheless, the low level of disease prevalence found indicates that the island’s pristine conditions might facilitate disease resistance. Moreover, the findings suggest an interesting trade-off between growth rates and colony recovery in shallow waters related to tissue breakage. This study provides crucial base-line data for future investigations aiming at understanding coral responses to anthropogenic pressures and the impact of global climate change on coral communities.
Introduction
Gorgonian corals are among the most vulnerable reef organisms (Goldberg and Wilkinson, 2004; Sánchez et al., 2019). Being long-lived and slow-growing species, they are particularly sensitive to natural disturbances (Linares et al., 2008). Moreover, gorgonian corals are increasingly affected by disease outbreaks and abundances of many species have declined worldwide (Bourne et al., 2009; Sánchez et al., 2011, 2014). This is of great concern given that these organisms play a key ecological role in benthic communities and are considered “engineering species,” shaping the habitat and increasing its complexity by forming three-dimensional structures (Jones et al., 1994; Ballesteros, 2006; Sánchez et al., 2016). Additionally, they provide substrate and habitat for associated biota, thus increasing biomass and biodiversity of benthic communities (Buhl-Mortensen and Mortensen, 2005; Sánchez et al., 2016). Therefore, changes in gorgonian abundances in response to natural and anthropogenic disturbances may have dramatic effects on entire communities (Garrabou and Harmelin, 2002).
Anthropogenic effects on coral systems, however, are often difficult to quantify due to the lack of base-line data for unaffected populations. Thus, information from populations largely removed from human impact, such as those on the offshore Island Malpelo located in the Tropical Eastern Pacific (TEP), is particularly valuable. This uninhabited island is considered to be under pristine conditions (Zapata and Vargas-Ángel, 2003; Quimbayo et al., 2016). While gorgonians are typically diverse in the TEP (Bayer, 1953; Van Oppen et al., 2005; Sánchez, 2016), rocky reef environments of Malpelo Island are mainly dominated by species of the genera Leptogorgia and Pacifigorgia (Sánchez et al., 2011; Sánchez, 2016). Of these, Pacifigorgia cairnsi, which is endemic to the TEP, is considered a keystone species in Malpelo. It dominates the infralittoral seascape by forming gorgonian forests on rocky outcrops and walls up to 30 m depth (Sánchez et al., 2011; Sánchez and Ballesteros, 2014). This species also plays an important ecological role due to, for instance, strong interactions with invertebrates such as ovulid gastropods and fishes including Oxycirrhites typus and Dermatolepis dermatolepis (Sánchez et al., 2016; Sánchez, 2016). Therefore, the loss or decrease of P. cairnsi in Malpelo would dramatically affect the entire ecosystem; a fact that makes its study and conservation a crucial matter (sensu Mills et al., 1993).
These gorgonian populations cope with cold waters and high levels of suspended matter during seasonal upwelling (from December to March), as well as anomalous increases in seawater temperature during sporadic El Niño-Southern Oscillation (ENSO) events (Glynn and Colgan, 1992; Zapata et al., 2010). Moreover, in recent years, P. cairnsi die-offs in Malpelo have been associated to disease outbreaks (Sánchez et al., 2011; Barrero-Canosa et al., 2013), namely the “necrotic-patch disease’ (NPD) (Quintanilla et al., 2018).
However, the impact of natural environmental stressors on P. cairnsi communities in the pristine island of Malpelo remains poorly understood, calling for coral response studies involving demographic traits such as growth rates, density, size structure, and disease susceptibility (sensu Bruno et al., 2011; Rico-Esenaro et al., 2019).
In this study, we therefore assessed the impact of ENSO events and local-scale environmental parameters on the demography and health status of gorgonian populations in the absence of direct anthropogenic pressures. First, we compared P. cairnsi densities and population size structures under different habitat and hydrodynamic settings to understand how local conditions drive these population parameters. We then identified the respective environmental features affecting growth rates. Finally, we evaluated NPD prevalence and colony recovery to assess their health status.
Materials and Methods
Study Site and Data Collection
The study was conducted in the TEP island Malpelo, which is located about 500 km off the Colombian coast (3°58′30″N, 81°34′48″W) (Figure 1). This Fauna and Flora Sanctuary is a marine protected area and a World Heritage Site that belongs to the Marine Conservation Corridor of the TEP together with Gorgona (Colombia), Cocos (Costa Rica), Coiba (Panamá), and Galapagos Islands (Ecuador). A total of 80 P. cairnsi colonies of different sizes were studied in two localities exposed to leeward or windward conditions depending on dry/wet seasons and wind patterns (Velásquez-Jiménez et al., 2016). The first locality, Arrecife, is located in the northeastern part of the island, which is constantly impacted by strong waves. P. cairnsi colonies are patchy distributed on large stones along the rocky reef. The second locality, Nevera, is located in a sheltered bay in the southwestern part of the island. P. cairnsi sea fans are continuously distributed along a wall up to depths of approximately 18–20 m (Figure 2). However, hydrodynamic conditions change considerably with water depth. Accordingly, depths of up to 10 m are influenced by strong waves and tidal movements (Velásquez-Jiménez et al., 2016). To reflect these different conditions, we studied in both sites populations in “shallow” (8 m) and “deep” (16 m) waters.
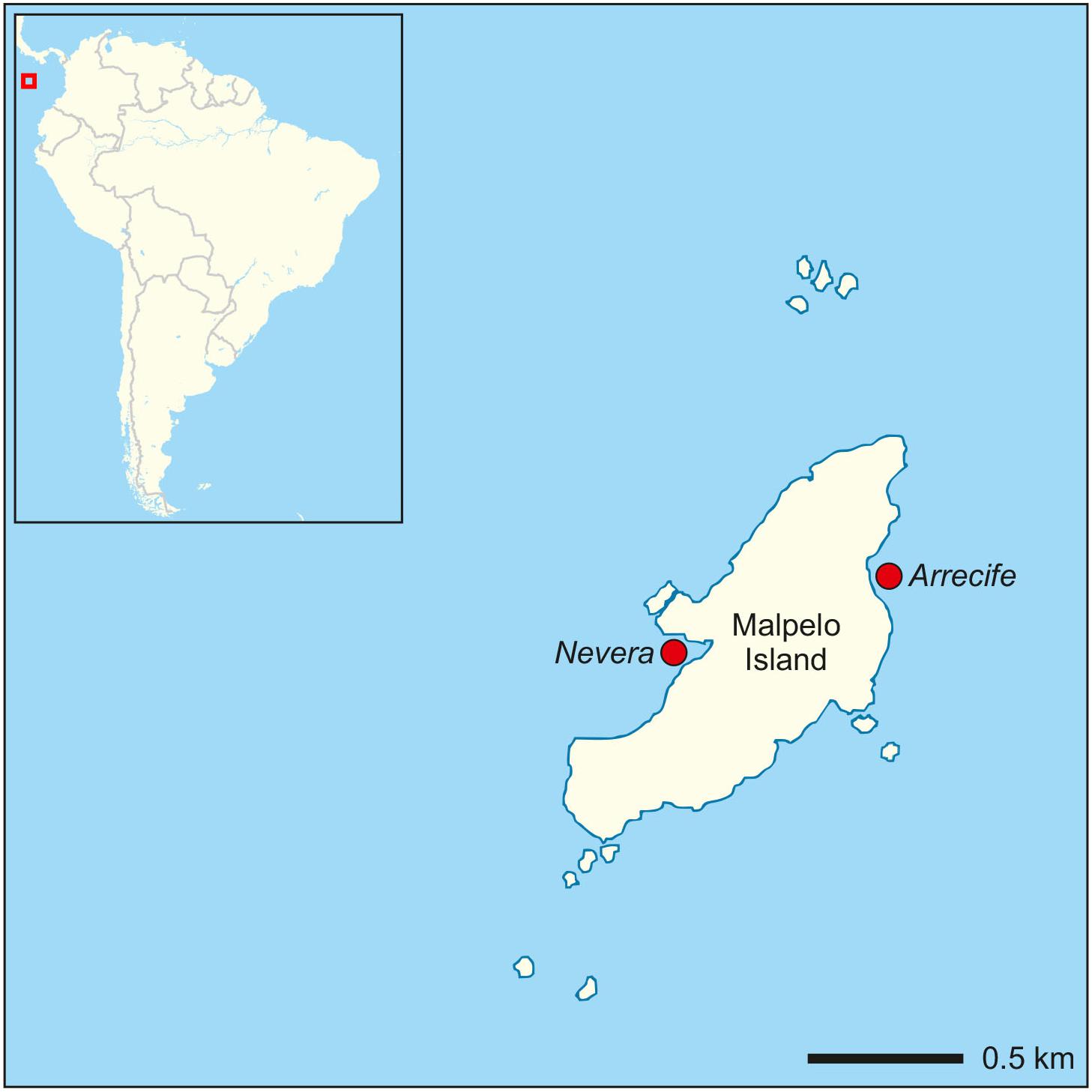
Figure 1. Geographical location map of Malpelo Island and the studied localities (Arrecife and Nevera).
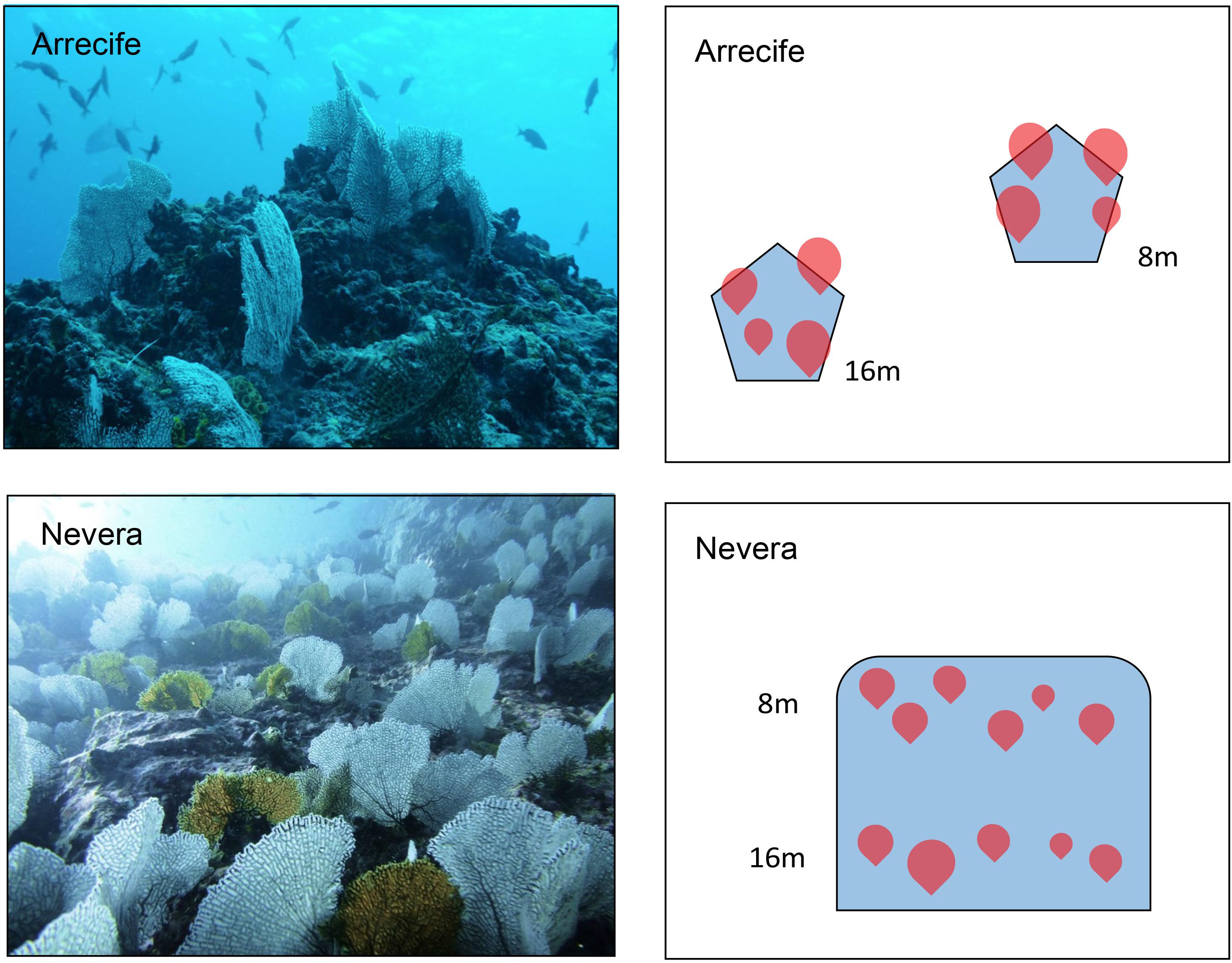
Figure 2. Images of the monitored localities of Pacifigorgia cairnsi in Malpelo Island (left). Diagram of sampling design showing an overview of the vertical/horizontal distribution of tagged colonies (right).
To assess the size structure, 10 quadrants each (50 × 50 cm) were randomly placed at the two depths (8 and 16 m) in the Arrecife and Nevera localities (40 quadrants in total) in June 2016. All colonies within each quadrant were counted and their total areas were registered. Underwater pictures (PowerShotG12, Canon) were taken together with an acrylic board containing a defined scale. Most underwater photographs had to be corrected for skewedness by using a standardized grid as they were taken with a deviation from the vertical plane of the colony. Total colony area was obtained by contouring the colony perimeter of each corrected photograph using Image J® software (Research Services Branch – NIMH, Bethesda, MD, United States). Colonies measuring <5 cm2 were considered recruits as defined by Gomez et al. (2014) and six size classes were delimited: <5, 5–100, 101–200, 201–300, 301–400, and >400 cm2.
To study gorgonian growth rates, the total area of each tagged colony was registered by underwater pictures during eight consecutive monitoring times, covering a 3-year study period: July 2013, December 2013, March 2014, July 2014, March 2015, September 2015, March 2016, and June 2016. Growth rates for each colony were obtained by calculating the difference in area (cm2) between two consecutive monitoring times and expressed as cm2 month–1. Additionally, tissue breakage, colony recovery, and disease prevalence (% of diseased colonies) were reported over the study period.
According to the National Oceanic and Administration (NOAA)1, abnormal positive seawater temperatures associated to ENSO events ranged from +0.5 to +2.3°C during the monitoring period. As the periods July 2014–March 2015 and March 2016–June 2016 included more months with abnormal positive temperatures than with normal ones, they were considered as ENSO periods (Table 1). “La Niña” events (the cool phase of ENSO) did not occur during the study period.
Data Analyses
Differences in size structures and densities between localities and water depths were assessed using Kruskal–Wallis and Mann–Whitney tests as implemented in PAST v3.12 (Hammer et al., 2001). The significant level was adjusted for the number of comparisons tested by Bonferroni correction. To evaluate the effect of five factors [locality, water depth, upwelling conditions (local thermal conditions), ENSO events (global thermal conditions), and colony area] on growth rates and disease prevalence, linear mixed effect (LME) models were constructed using the R v.3.1.2 (R Studio Team, 2015) package nlme (Pinheiro et al., 2009). Colony and periods were treated as random effects; locality, water depth, local and global thermic conditions, and colony area as fixed effects. Full and reduced models were fitted using a restricted maximum likelihood (REML) approach and models were compared using Akaike’s Information Criterion (AIC). To understand site-specific effects, LME models were also applied individually to the Arrecife and Nevera datasets.
Results
Density and Size Structure
The average P. cairnsi density observed was 18.5 (±7.92) col m–2 and did not differ between localities (Mann–Whitney test, P > 0.05, P = 0.96) or water depths (Mann–Whitney pairwise test, P > 0.008, P = 0.93) (Table 2). Images from a total of 179 P. cairnsi colonies were analyzed to study the size structure in June 2016. Overall, mean colony areas did not show significant differences between Arrecife and Nevera (Mann–Whitney test, P > 0.05), but displayed significant differences between shallow sites (Mann–Whitney pairwise test, P < 0.008). In particular, the Arrecife shallow site showed lower proportions of large colonies and higher percentage of young colonies (<100 cm2) than Nevera shallow. Additionally, the percentage of recruits (<5 cm2) and big colonies (>300 cm2) were higher in Nevera deep than in any other site (Figure 3).
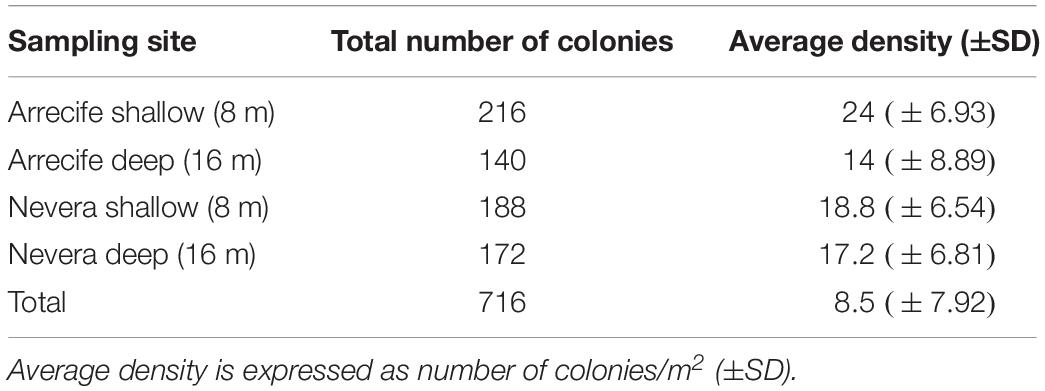
Table 2. Number of Pacifigorgia cairnsi colonies and average densities in Malpelo Island in June 2016.
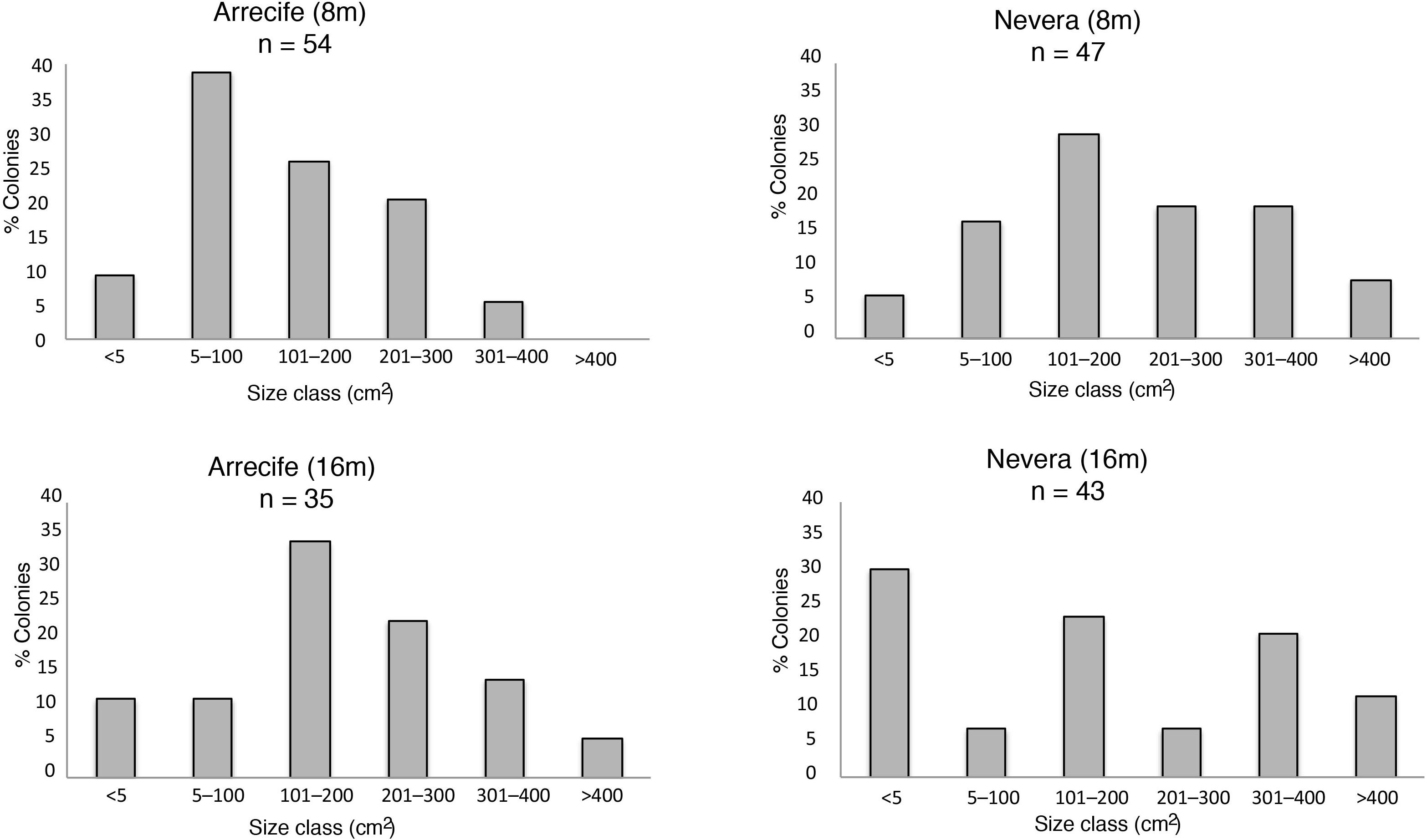
Figure 3. Size-frequency distribution of Pacifigorgia cairnsi colonies in shallow and deep water sites at Arrecife and Nevera localities in Malpelo Island in June 2016 (n = number of colonies).
Growth Rates
The average growth rate measured for P. cairnsi was 6.16 cm2 month–1. Visual examination of residuals of the best-fit LME models indicated that the assumptions of normality, homogeneity, and independence were all met. Global thermal conditions (ENSO events) and water depth had a significant effect on Nevera P. cairnsi growth rates (P = 0.001 and P = 0.045, respectively). Growth rates of colonies from Arrecife were not significantly affected by the studied parameters. Considering both localities together, only ENSO events had an effect on growth rates (Table 3).
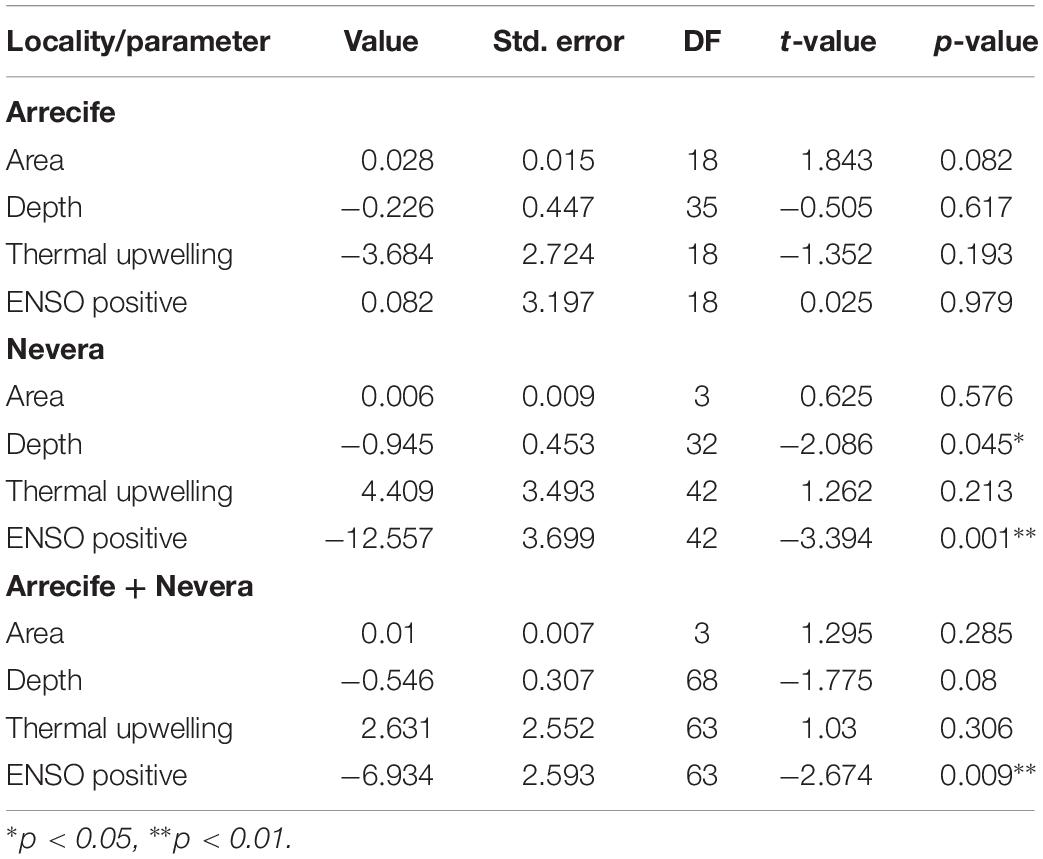
Table 3. Results of the best-fitted linear mixed effect model for parameters driving Pacifigorgia cairnsi growth rates in Malpelo Island.
Disease Prevalence, Tissue Breakage, and Colony Recovery
None of the studied parameters had a significant effect on NPD prevalence. Overall, mean disease prevalence registered during the eight monitoring times was 6.02% (± 9.49%) (Table 4). Overall, 37.80% of the healthy colonies showed tissue breakage at some point during the study period. In particular, the Arrecife shallow and deep sites displayed 33.34 and 15.78% of healthy-broken colonies, respectively, and Nevera shallow and Nevera deep sites 61.12 and 42.85%, respectively. 57% of diseased colonies recovered from NPD; all of them showed signs of tissue breakage (Figure 4).

Table 4. NPD prevalence of Pacifigorgia cairnsi (% diseased colonies) in Malpelo Island during eight monitoring times (July 2013, December 2013, March 2014, July 2014, March 2015, September 2015, March 2016, and June 2016).
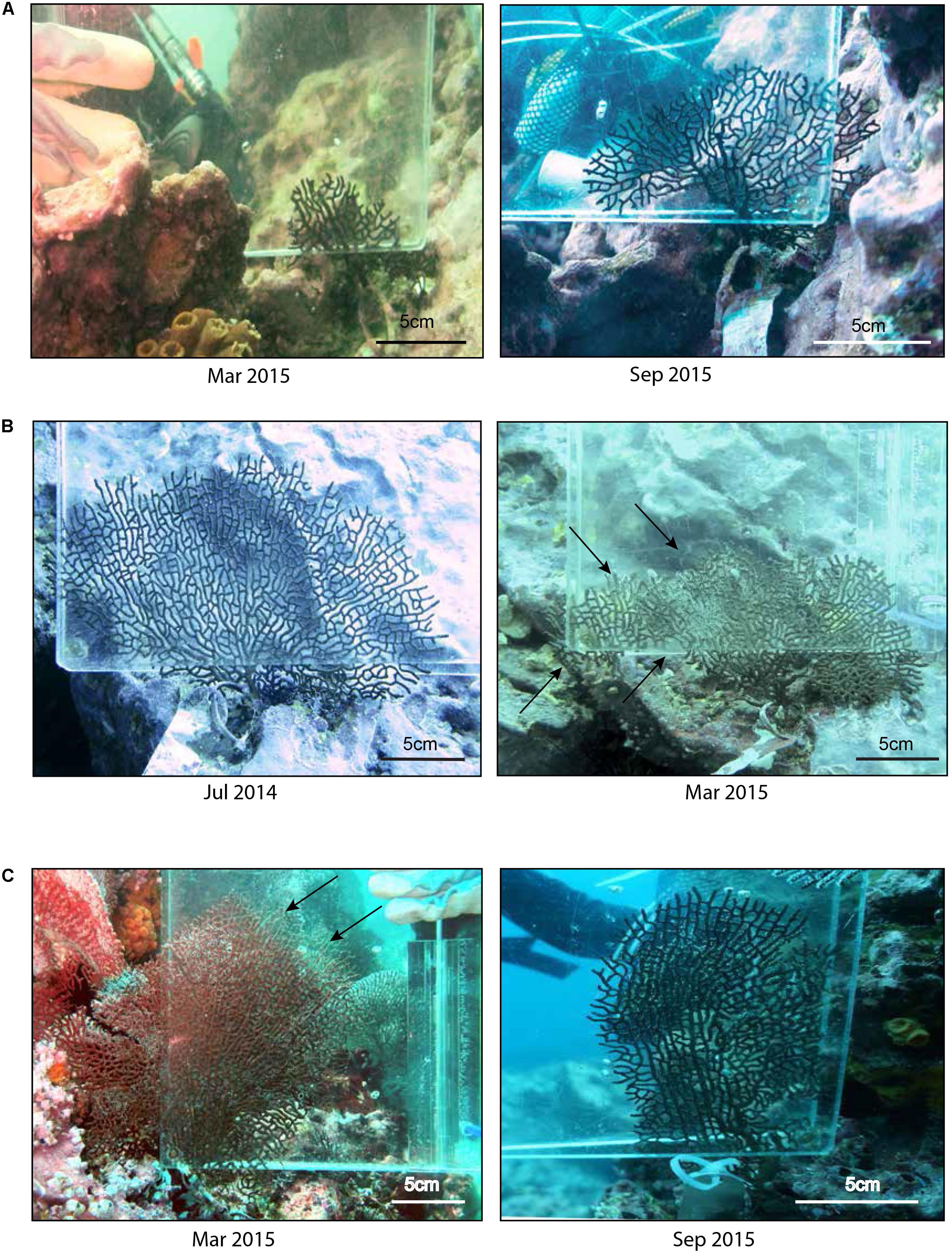
Figure 4. Growth (A), tissue breakage (B), and colony recovery by tissue breakage (C) of a Pacifigorgia cairnsi colony in Malpelo Island during two consecutive monitoring times. Arrows show areas of tissue breakage from a healthy (B) and diseased colony (necrotic tissue) (C).
Discussion
In this study, we assessed the effects of global (i.e., ENSO events) and local hydrodynamic parameters on the demography of P. cairnsi populations and evaluated their health status in a remote marine area, currently not directly affected by anthropogenic processes. Our major findings were: (i) local hydrodynamics defined P. cairnsi size structures, (ii) growth rates were driven by thermal anomalies and partly by water depth, (iii) disease prevalence was low and did not correlate with any of the studied environmental parameters, and (iv) most diseased colonies recovered via tissue breakage.
Pacifigorgia cairnsi densities did not differ between the localities and sites (water depth) studied. Although this pattern is consistent with those from Coiba Island (Panamanian TEP), the average density of P. cairnsi in Malpelo Island (18 col m–2) was considerably higher than in Coiba Island (1.2 col m–2) (Gomez et al., 2014). Patchy species distribution of gorgonians within TEP areas is attributed to variable oceanographic and environmental conditions (e.g., upwelling, freshwater and runoff sediment inputs, suspended matter, etc.) (Zapata et al., 2010; Barrero-Canosa et al., 2013; Gomez et al., 2014).
However, size structures of P. cairnsi colonies in Malpelo Island differed among localities and sites. Arrecife shallow showed both the highest proportion of small colonies and the lowest percentage of large colonies. As hydrodynamic forces may cause dislodgement and tissue breakage (Lasker, 1990; Chang et al., 2007), the permanent exposition of this locality to strong water motions might make local P. cairnsi colonies particular vulnerable. Additionally, the Nevera deep site exhibited the greatest percentage of both recruits and large colonies. Abiotic factors including light intensity, temperature, and water movement may considerably change with water depth (Garrabou et al., 2002; Mass et al., 2007) and thus modulate demographic parameters of benthic organisms such as gorgonian corals. Sheltered environments associated with deep sublittoral habitats in the Mediterranean Sea, for example, allow Eunicella singularis gorgonian populations to reach larger colony sizes than in shallow waters (Gori et al., 2011). Similarly, larger Gorgonia ventalina colonies were found at deeper environments in the Gulf of Mexico, where water motion decreases significantly with water depth (Jordán-Dahlgren, 1989). Therefore, local perturbations such as high hydrodynamic forces in shallow waters may explain the higher percentage of broken colonies both in Nevera and Arrecife shallow sites compared to Nevera deep site. Additionally, the latter site showed the highest recruitment rate, suggesting that reproductive success increases with colony size (Coma et al., 1995).
We also found that growth rates of P. cairnsi were affected by thermal anomalies associated with ENSO events. Average temperatures are a major factor controlling coral growth rates (Chindapol et al., 2013). Specifically, elevated seawater temperatures related to ENSO episodes exercise stress on corals as there is a thermal optimum for coral growth (Lough and Cooper, 2011). Coral responses to thermal stress are well studied in zooxanthellate reef-building corals and include changes in coral physiology, reduction of growth rates, up-regulation of heat-shock proteins, coral bleaching, and ultimately die-offs of entire coral reefs (Ainsworth et al., 2008; Voolstra et al., 2009; Lough and Cooper, 2011). In particular, recurrent ENSO episodes have increased thermal stress in Caribbean and Eastern Pacific reef-building corals, leading to bleaching and growth declines (Glynn et al., 2001; Manzello, 2010; Hetzinger et al., 2016). However, while thermal stress response (e.g., up-regulation of stress proteins and bleaching; Prada et al., 2009; Dias and Gondim, 2015; Lõhelaid et al., 2015) has also been observed in zooxanthellate octocorals, the latter organisms seem to be more resilient to environmental stressors and show higher recovery rates after ENSO episodes than reef-building corals (Schleyer and Benayahu, 2010; Tsounis and Edmunds, 2017).
Nevertheless, responses of azooxanthellate octocorals, such as P. cairnsi, to thermal stress remain largely elusive. Despite the assumption that octocorals represent the most resistant coral organisms in regard to thermal stress (Tsounis and Edmunds, 2017), our findings indicate that azooxanthellate gorgonians are also vulnerable (as zooxanthellate ones) to abnormal temperatures and changes in productivity and nutrient levels associated with ENSO events (Harvell et al., 2001; Turk et al., 2011). This is of particular relevance as these global-scale events are predicted to increase in frequency and severity over the next decades (Santoso et al., 2013; Cai et al., 2014). It has also been shown that the coral recovery capacity declines with increasing frequency of ENSO episodes; exacerbated by the fact that ENSO-associated effects on coral communities may last for months or years following initial impact (Cai et al., 2014; Glynn et al., 2017). This is of great concern for benthic communities in Malpelo Island as P. cairnsi is a keystone species there, and its decline might have severe adverse effects on the entire benthic food web.
Apart from temperature, water motion and light are also main environmental parameters affecting coral growth (Todd, 2008; Chindapol et al., 2013). Colonies in deeper waters, for example, display decreased growth rates, minimizing metabolic demand under low-light conditions (Grigg, 2006; Kahng et al., 2010). However, P. cairnsi lacks zooxanthellae and light may thus not be a major limiting factor. In fact, our results suggest that hydrodynamic forces might be the main parameter affecting growth rates. Due to wave-induced tissue breakage, shallow-water colonies have to allocate considerable energy resources to tissue repair (Lartaud et al., 2016). Accordingly, the fact that depth did not contribute significantly to decreased growth rates in Arrecife deep suggests that these colonies, as the shallow ones, are probably allocating energy in tissue repair due to wave-induced tissue breakage. In contrast, deeper (and larger) colonies from Nevera deep might be able to allocate more energy to recruitment rather than to tissue repair and growth due to the sheltered environment as noted in other gorgonian corals (Coma et al., 1995; Heino and Kaitala, 1999).
Our results also showed that the prevalence of NPD affecting P. cairnsi in Malpelo Island was low (i.e., 6%) compared to coral diseases in other areas. For example, aspergillosis affects 6–39% of G. ventalina colonies throughout the Caribbean Sea (Weil, 2004; Toledo-Hernández et al., 2007). These high levels of disease prevalence in the Caribbean Sea, compared to the TEP, have been attributed to higher levels of long-term thermal and anthropogenic stress in the former region (Barton and Casey, 2005; Ruiz-Moreno et al., 2012).
The low level of NPD prevalence observed in our study sites might thus be related to the pristine conditions of Malpelo Island. Moreover, the fact that we did not find correlations between local/global environmental parameters and NPD prevalence suggests a random and occasional behavior of the disease in P. cairnsi. This assumption is also supported by the fact that NPD affecting P. cairnsi in Malpelo Island behaves opportunistically and is likely in a state of microbial dysbiosis (Quintanilla et al., 2018). Interestingly, while tissue breakage in shallow, wave-exposed waters is energetically costly and affects growth rates, it also facilitates recovery from NPD via physical loss of affected, peripheral tissues. Overall, the NPD patterns observed in P. cairnsi colonies in Malpelo Island indicate that coral diseases are also present in pristine marine areas, being part of a natural population dynamic.
Conclusion
Our work provides crucial base-line data on demographic and health parameters of gorgonian populations in a remote and pristine marine area removed from direct anthropogenic impact. Overall, colonies developed into mature gorgonian populations, showing high-density levels and size structures driven mostly by local hydrodynamics. However, global thermal conditions such as ENSO events negatively affected P. cairnsi growth rates. This is of great concern as the taxon represents a key stone species within the benthic food web and as these global-scale events are predicted to increase in frequencies and severity under conditions of climate change. Nonetheless, the low levels of disease prevalence found suggest that the island’s pristine conditions might facilitate disease resistance. Moreover, our results indicate an interesting trade-off between growth rates and colony recovery in shallow waters related to tissue breakage. Finally, this study facilitates future research aimed at understanding coral responses to anthropogenic pressures and the impact of global climate change on coral communities.
Data Availability Statement
All datasets generated for this study are included in the article/Supplementary Material.
Author Contributions
EQ, TM, TW, and JS contributed to the design of the study, revised the manuscript, and approved the final text. EQ conducted the sampling, generated and analyzed the data, and wrote the manuscript. EQ and TM interpreted and discussed the data results.
Funding
This study was funded by the Facultad de Ciencias (Universidad de los Andes) and the Center of Excellence in Marine Sciences (CEMarin) in Bogotá.
Conflict of Interest
The authors declare that the research was conducted in the absence of any commercial or financial relationships that could be construed as a potential conflict of interest.
Acknowledgments
We thank the Fundación Malpelo and Parques Nacionales Naturales (PNN) in Colombia for organizing the expeditions to Malpelo Island, especially Sandra Bessudo and Nancy Murillo. We also acknowledge the colleagues at the Laboratorio de Biología Molecular Marina (BIOMMAR, Universidad de los Andes) for their support in the field. We are especially grateful to Torsten Hauffe (Justus Liebig University Giessen) for his advice and support with the statistics.
Supplementary Material
The Supplementary Material for this article can be found online at: https://www.frontiersin.org/articles/10.3389/fmars.2019.00694/full#supplementary-material
Footnotes
References
Ainsworth, T. D., Hoegh-Guldberg, O., Heron, S. F., Skirving, W. J., and Leggat, W. (2008). Early cellular changes are indicators of pre-bleaching thermal stress in the coral host. J. Exp. Mar. Biol. Ecol. 364, 63–71. doi: 10.1016/j.jembe.2008.06.032
Ballesteros, E. (2006). Mediterranean coralligenous assemblages: a synthesis of present knowledge. Oceanogr. Mar. Biol. 44, 123–195. doi: 10.1201/9781420006391.ch4
Barrero-Canosa, J., Dueñas, L. F., and Sánchez, J. A. (2013). Isolation of potential fungal pathogens in gorgonian corals at the Tropical Eastern Pacific. Coral Reefs 32, 35–41. doi: 10.1007/s00338-012-0972-2
Barton, A. D., and Casey, K. S. (2005). Climatological context for large-scale coral bleaching. Coral Reefs 24, 536–554. doi: 10.1007/s00338-005-0017-1
Bayer, F. M. (1953). Zoogeography and evolution in the octocorallian family gorgoniidae. Bull. Mar. Sci. Gulf Caribb. 3, 110–119.
Bourne, D. G., Garren, M., Work, T. M., Rosenberg, E., Smith, G. W., and Harvell, C. D. (2009). Microbial disease and the coral holobiont. Cell Press 17, 554–562. doi: 10.1016/j.tim.2009.09.004
Bruno, J. F., Ellner, S. P., Vu, I., Kim, K., Harvell, C. D., and Ellner, P. (2011). Impacts of aspergillosis on sea fan coral demography: modeling a moving target. Ecol. Monogr. 81, 123–139. doi: 10.1890/09-1178.1
Buhl-Mortensen, L., and Mortensen, P. B. (2005). “Distribution and diversity of species associated with deep-sea gorgonian corals off Atlantic Canada,” in Cold-Water Corals and Ecosystems, eds A. Freiwald, and J. Roberts, (Berlin: Springer), 849–879. doi: 10.1007/3-540-27673-4_44
Cai, W., Borlace, S., Lengaigne, M., Van Rensch, P., Collins, M., and Vecchi, G. (2014). Increasing frequency of extreme El Niño events due to greenhouse warming. Nat. Clim. Chang. 4, 111–116. doi: 10.1038/nclimate2100
Chang, W. L., Chi, K. J., Fan, T. Y., and Dai, C. F. (2007). Skeletal modification in response to flow during growth in colonies of the sea whip, Junceella fragilis. J. Exp. Mar. Biol. Ecol. 347, 97–108. doi: 10.1016/j.jembe.2007.03.011
Chindapol, N., Kaandorp, J. A., Cronemberger, C., Mass, T., and Genin, A. (2013). Modelling growth and form of the scleractinian coral pocillopora verrucosa and the influence of hydrodynamics. PLoS Comput. Biol. 9:e1002849. doi: 10.1371/journal.pcbi.1002849
Coma, R., Ribes, M., Zabala, M., and Gilil, J.-M. (1995). Reproduction and cycle of gonadal development in the mediterranean gorgonian Paramuricea clavata. Mar. Ecol. Prog. Ser. 117, 173–183. doi: 10.3354/meps117173
Dias, T. L., and Gondim, A. I. (2015). Bleaching in scleractinians, hydrocorals, and octocorals during thermal stress in a northeastern brazilian reef. Mar. Biodivers. 46, 303–307. doi: 10.1007/s12526-015-0342-8
Garrabou, J., Ballesteros, E., and Zabala, M. (2002). Structure and dynamics of north-western mediterranean rocky benthic communities along a depth gradient. Estuar. Coast. Shelf Sci. 55, 493–508. doi: 10.1006/ecss.2001.0920
Garrabou, J., and Harmelin, J.-G. (2002). A 20-year study on life-history traits of a harvested long-lived temperate coral in the NW mediterranean: insights into conservation and management needs. J. Anim. Ecol. 71, 966–978. doi: 10.1046/j.1365-2656.2002.00661.x
Glynn, P., Mones, A., Podestá, G., Colbert, A., and Colgan, M. (2017). El Niño-Southern Oscillation: Effects on Eastern Pacific Coral reefs and Associated Biota Coral Reefs of the Eastern Tropical Pacific. Dordrecht: Springer.
Glynn, P. W., and Colgan, M. W. (1992). Sporadic disturbances in fluctuating coral reef environments: el niño and coral reef development in the Eastern Pacific. Am. Zool. 32, 707–718. doi: 10.1093/icb/32.6.707
Glynn, P. W., Maté, J., Baker, A. C., and Calderon, M. O. (2001). Coral bleaching and mortality in panama and ecuador during the 1997-1998 el niño-southern oscillation event: spatial/temporal patterns and comparisons with the 1982-1983 Event. Bull. Mar. Sci. 69, 79–109.
Goldberg, J., and Wilkinson, C. (2004). “Global threats to coral reefs: coral bleaching, global climate change, disease, predator plagues and invasive species,” in Status of Coral Reefs of the World, ed. C. Wilkinson, (Townsville: James Cook University), 67–92.
Gomez, G. C., Guzman, M. H., Gonzalez, A., and Breedy, O. (2014). Survival, growth, and recruitment of octocoral species (Coelenterata: Octocorallia) in coiba National Park. Pacific panama. Bull. Mar. Sci. 90, 623–650. doi: 10.5343/bms.2012.1092
Gori, A., Rossi, S., Linares, C., Berganzo, E., Orejas, C., Dale, M. R. T., et al. (2011). Size and spatial structure in deep versus shallow populations of the mediterranean gorgonian Eunicella singularis (Cap de creus, northwestern mediterranean sea). Mar. Biol. 158, 1721–1732. doi: 10.1007/s00227-011-1686-7
Grigg, R. W. (2006). Depth limit for reef building corals in the Au’au channel. S.E. Hawaii. Coral Reefs 25, 77–84. doi: 10.1007/s00338-005-0073-6
Hammer, O., Harper, D. A., and Ryan, P. (2001). Paleontological statistics software: package for education and data analysis. Paleontol. Electron. 4:9.
Harvell, D., Kim, K., Quirolo, C., Weir, J., and Smith, G. (2001). Coral bleaching and disease: contributors to 1998 mass mortality in Briareum asbestinum (Octocorallia. Gorgonacea). Hydrobiologia 460, 97–104. doi: 10.1007/978-94-017-3284-0_8
Heino, M., and Kaitala, V. (1999). Evolution of resource allocation between growth and reproduction in animals with indeterminate growth. J. Evol. Biol. 12, 423–429. doi: 10.1046/j.1420-9101.1999.00044.x
Hetzinger, S., Pfeiffer, M., Dullo, W.-C., Zinke, J., and Garbe-Schönberg, D. (2016). A change in coral extension rates and stable isotopes after El Niño- induced coral bleaching and regional stress events. Sci. Rep. 6:32879.
Jones, C. G., Lawton, J. H., and Shachak, M. (1994). Organisms as ecosystem engineers. Oikos 69, 373–386.
Jordán-Dahlgren, E. (1989). Gorgonian community structure and reef zonation patterns on yucatan coral reefs. Bull. Mar. Sci. 45, 678–696.
Kahng, S. E., Garcia, J. R., Spalding, H. L., Brokovich, E., Wagner, D., Weil, E., et al. (2010). Community ecology of mesophotic coral reef ecosystems. Coral Reefs 29, 255–275. doi: 10.1007/s00338-010-0593-6
Lartaud, F., Galli, G., Raza, A., Priori, C., Benedetti, M. C., Cau, A., et al. (2016). “Growth patterns in long-lived coral species,” in Marine Animal Forests, ed. S. Rossi, (Switzerland: Springer International Publishing).
Lasker, H. R. (1990). Clonal propagation and population dynamics of a gorgonian coral. Ecology 71, 1578–1589. doi: 10.2307/1938293
Linares, C., Coma, R., Garrabou, J., Díaz, D., and Zabala, M. (2008). Size distribution, density and disturbance in two mediterranean gorgonians: Paramuricea clavata and Eunicella singularis. J. Appl. Ecol. 45, 688–699. doi: 10.1111/j.1365-2664.2007.01419.x
Lõhelaid, H., Teder, T., and Samel, N. (2015). Lipoxygenase-allene oxide synthase pathway in octocoral thermal stress response. Coral Reefs 34, 143–154. doi: 10.1007/s00338-014-1238-y
Lough, J. M., and Cooper, T. F. (2011). New insights from coral growth band studies in an era of rapid environmental change. Earth Sci. Rev. 108, 170–184. doi: 10.1016/j.earscirev.2011.07.001
Manzello, D. P. (2010). Coral growth with thermal stress and ocean acidification: lessons from the eastern tropical Pacific. Coral Reefs 29, 749–758. doi: 10.1007/s00338-010-0623-4
Mass, T., Einbinder, S., Brokovich, E., Shashar, N., Vago, R., Erez, J., et al. (2007). Photoacclimation of Stylophora pistillata to light extremes: metabolism and calcification. Mar. Ecol. Prog. Ser. 334, 93–102. doi: 10.3354/meps334093
Mills, S. L., Soulé, M. E., and Doak, D. F. (1993). The keystone-species concept in ecology and conservation. Bioscience 43, 219–224. doi: 10.2307/1312122
Pinheiro, J., Bates, D., DebRoy, S., Deepayan, S., and Team, R. C. (2009). nlme: Linear and Nonlinear Mixed Effects Models. R Packag Version.
Prada, C., Weil, E., and Yoshioka, P. (2009). Octocoral bleaching during unusual thermal stress. Coral Reefs 29, 41–45. doi: 10.1007/s00338-009-0547-z
Quimbayo, J. P., Mendes, T. C., Kulbicki, M., Floeter, S. R., and Zapata, F. A. (2016). Unusual reef fish biomass and functional richness at Malpelo, a remote island in the Tropical Eastern Pacific. Environ. Biol. Fishes 100, 149–162. doi: 10.1007/s10641-016-0557-y
Quintanilla, E., Ramírez-Portilla, C., Adu-Oppong, B., Walljasper, G., Glaeser, S., Wilke, T., et al. (2018). Local confinement of disease-related microbiome facilitates recovery of gorgonian sea fans from necrotic-patch disease. Sci. Rep. 8:14636. doi: 10.1038/s41598-018-33007-8
Rico-Esenaro, S. D., Sanchez-cabeza, J.-A., Carricart-Ganivet, J. P., Montagna, P., and Ruiz-fernández, A. C. (2019). Uncertainty and variability of extension rate, density and calcification rate of a hermatypic coral (Orbicella faveolata). Sci. Total Environ. 650, 1576–1581. doi: 10.1016/j.scitotenv.2018.08.397
Ruiz-Moreno, D., Willis, B. L., Page, A. C., Weil, E., Cróquer, A., Vargas-Angel, B., et al. (2012). Global coral disease prevalence associated with sea temperature anomalies and local factors. Dis. Aquat. Org. 100, 249–261. doi: 10.3354/dao02488
Sánchez, J. A. (2016). “Diversity and evolution of octocoral animal forests at both sides of Tropical America,” in Marine Animal Forests, eds S. Rossi, L. Bramanti, and A. Gori, (Berlin: Springer International Publishing), 1–33. doi: 10.1007/978-3-319-17001-5_39-1
Sánchez, J. A., Ardila, N. E., Andrade, J., Dueñas, L. F., Navas, R., and Ballesteros, D. (2014). Octocoral densities and mortalities in Gorgona Island, Colombia, Tropical Eastern Pacific. Rev. Biol. Trop. 62, 209–219.
Sánchez, J. A., and Ballesteros, D. C. (2014). The invasive snowflake coral (carijoa riisei) in the tropical eastern pacific Colombia. Rev. Biol. Trop. 62, 197–201.
Sánchez, J. A., Dueñas, L. F., Rowley, S. J., Gonzalez-zapata, F. L., Vergara, D. C., Montaño-salazar, S. M., et al. (2019). “Gorgonian corals,” in Mesophotic Coral Ecosystems of the World, eds Y. Loya, and K. A. Puglise, (New York, NY: Springer), 729–747.
Sánchez, J. A., Fuentes-Pardo, A. P., Ní Almhain, I., Ardila-Espitia, N. E., Cantera-Kintz, J., and Forero-Shelton, M. (2016). The masquerade game: marine mimicry adaptation between egg-cowries and octocorals. PeerJ 4:e2051. doi: 10.7717/peerj.2051
Sánchez, J. A., Gómez, C. E., Escobar, D., and Dueñas, L. F. (2011). Diversidad, abundancia y amenazas de los octocorales de la isla malpelo, pacífico oriental Tropical, Colombia. Bol. Invest. Mar. Cost. 40, 139–154.
Santoso, A., Mcgregor, S., Jin, F., Cai, W., England, M. H., An, S., et al. (2013). Late-twentieth-century emergence of the el niño propagation asymmetry and future projections. Nature 504, 126–130.
Schleyer, M. H., and Benayahu, Y. (2010). Pre- and post-1998 ENSO records of shallow-water octocorals (Alcyonacea) in the chagos archipelago. Mar. Pollut. Bull. 60, 2197–2200. doi: 10.1016/j.marpolbul.2010.08.019
R Studio Team, (2015). Integrated Development for R. RStudio, Inc. Available at: http://www.rstudio.com (accessed June 2019).
Todd, P. A. (2008). Morphological plasticity in scleractinian corals. Biol. Rev. 83, 315–337. doi: 10.1111/j.1469-185x.2008.00045.x
Toledo-Hernández, C., Sabat, A. M., and Zuluaga-Montero, A. (2007). Density, size structure and aspergillosis prevalence in gorgonia ventalina at six localities in puerto rico. Mar. Biol. 152, 527–535. doi: 10.1007/s00227-007-0699-8
Tsounis, G., and Edmunds, P. J. (2017). Three decades of coral reef community dynamics in St. John, USVI: a contrast of scleractinians and octocorals. Ecosphere doi: 10.1002/ecs2.1646 [Epub ahead of print].
Turk, D., Meinen, C. S., Antoine, D., McPhaden, M. J., and Lewis, M. R. (2011). Implications of changing El Niño patterns for biological dynamics in the equatorial Pacific Ocean. Geophys. Res. Lett. 38, 1–6.
Van Oppen, M. J. H., Mieog, J. C., Sánchez, C. A., and Fabricius, K. E. (2005). Diversity of algal endosymbionts (zooxanthellae) in octocorals: the roles of geography and host relationships. Mol. Ecol. 14, 2403–2417. doi: 10.1111/j.1365-294x.2005.02545.x
Velásquez-Jiménez, L., Acosta, A., Cortés-Chong, N., and García, S. (2016). Population structure of megabalanus peninsularis in malpelo island. Colombia. Rev. Biol. Mar. Oceanogr. 51, 461–468. doi: 10.4067/s0718-19572016000200024
Voolstra, C. R., Schnetzer, J., Peshkin, L., Randall, C. J., Szmant, A. M., and Medina, M. (2009). Effects of temperature on gene expression in embryos of the coral Montastraea faveolata. BMC Genomics doi: 10.1186/1471-2164-10-627 [Epub ahead of print].
Weil, E. (2004). “Coral reef disease in the wider caribbean,” in Coral Health and Disease, eds E. Rosenberg, and Y. Loya, (Berlin: Springer), 358–364.
Zapata, F. A., Rodríguez-Ramírez, A., Caro-Zambrano, C., and Garzón-Ferreira, J. (2010). Mid-term coral-algal dynamics and conservation status of a Gorgona Island (Tropical Eastern Pacific) coral reef. Rev. Biol. Trop. 58, 81–94.
Keywords: gorgonian corals, Tropical Eastern Pacific, disease prevalence, growth rates, ENSO
Citation: Quintanilla E, Madurell T, Wilke T and Sánchez JA (2019) Dynamic Interplay of ENSO Events and Local Hydrodynamic Parameters Drives Demography and Health Status of Gorgonian Sea Fan Populations on a Remote Tropical Eastern Pacific Island. Front. Mar. Sci. 6:694. doi: 10.3389/fmars.2019.00694
Received: 14 June 2019; Accepted: 29 October 2019;
Published: 19 November 2019.
Edited by:
Michael Sweet, University of Derby, United KingdomCopyright © 2019 Quintanilla, Madurell, Wilke and Sánchez. This is an open-access article distributed under the terms of the Creative Commons Attribution License (CC BY). The use, distribution or reproduction in other forums is permitted, provided the original author(s) and the copyright owner(s) are credited and that the original publication in this journal is cited, in accordance with accepted academic practice. No use, distribution or reproduction is permitted which does not comply with these terms.
*Correspondence: Elena Quintanilla, ZS5xdWludGFuaWxsYTEwQHVuaWFuZGVzLmVkdS5jbw==