- 1School of Urban and Environmental Engineering, Ulsan National Institute of Science and Technology, Ulsan, South Korea
- 2Division of Polar Climate Sciences, Korea Polar Research Institute, Incheon, South Korea
- 3Major in Polar Science, University of Science and Technology, Daejeon, South Korea
- 4Division of Polar Ocean Sciences, Korea Polar Research Institute, Incheon, South Korea
- 5School of Civil, Environmental and Chemical Engineering, Changwon National University, Changwon, South Korea
Antarctic shelf systems play an important role in organic matter circulation on Earth; hence, identifying the characteristics of dissolved organic matter (DOM) can be a good indicator for understanding its origin, as well as climate change. In this study, to identify the characteristics of DOM in the ice shelf systems, surface water was collected from the open sea (OS) and Marian cove (fjord, FJ). Although there were no differences in DOM characteristics between sampling sites in the quantitative analyses, the DOM in the surface water of each region seemed to be more affected by terrestrial than marine biological sources in optical and molecular properties. This finding indicates that the terrestrial DOM related to mosses based on the results of molecular properties: high levels of lipid-like (35–39%) and unsaturated hydrocarbon-like (UH; 27–34%) in both the OS and the FJ regions and significantly higher tannin-like substance and condensed aromatic structures in the FJ than in the OS region. When comparing the FJ transect samples, those nearest to a glacier (FJ1; 0.93 km from the glacier) showed relatively low salinity, high dissolved organic carbon, and high chromophoric DOM, indicating that terrestrial DOM (possibly produced by moss) inflow occurred with the runoff from the freshly melting land ice and glacier. However, no significant differences in molecular composition were detected, suggesting that terrestrial DOM is introduced into the ice shelf systems by melting land ice, and glacier runoff could be a major source of DOM-rich seawater during austral fall when low marine biological activity occurs. This study has a great significance as background data for DOM characteristics in the ice shelf systems due to the enhanced biological activity during the austral summer.
Introduction
The ice shelf systems in Antarctica are one of the important organic carbon reservoirs on Earth. Annually, 2 Pg of carbon is absorbed by the seawaters of the world – approximately half is absorbed by the ice shelf systems, which is significant to the global carbon cycle (Takahashi et al., 2009). Moreover, the ice shelf systems are known to carry algae and microorganisms, which are a major source of organic carbon in seawaters (Nagata and Kirchman, 1992). Additionally, dissolved organic matter (DOM) in seawater is directly emitted from the seawater surface into the atmosphere via bubble bursting (i.e., primary marine aerosols or sea spray aerosols), leading to the production of organic-rich particles in the atmosphere over the Antarctica and the Arctic (O’Dowd and De Leeuw, 2007; Rastelli et al., 2017; Park J. et al., 2019). These organic aerosols can constitute large fractions (approximately 80%) of the submicron particle mass during a phytoplankton bloom (Park et al., 2014; Park J. et al., 2019). Therefore, the characterization of DOM in the Antarctic seawater can be a good indicator for understanding its origin as well as contribute to the understanding of marine ecosystem changes and climate change.
Diverse analytical techniques [i.e., UV spectrophotometer, total organic carbon (TOC) analysis, chromatography, and separation of hydrophilic and hydrophobic components using resin] have been generally used for DOM characterization in seawaters (e.g., determination of mass accuracy and unique spectral properties); however, these conventional analysis methods are limited by complicated and time-consuming measurement processes (Hur et al., 2006). The compositional differences and the characteristics of marine DOM are still largely unidentified because the very low concentrations of DOM in seawater, compared to the massive amounts of inorganic sea salts, present a major challenge (Hansell and Carlson, 2002; Park S. et al., 2019). Recently, high-resolution mass spectrometry (HRMS) has been applied to identify the molecular signatures of DOM (Koch et al., 2008; Phungsai et al., 2016; Baek et al., 2019). HRMS has far higher sensitivity and resolving power in identifying mass spectral peaks than the existing mass spectrometry (MS) methods (Koch et al., 2008). In particular, Orbitrap-MS can determine over 1,500 unknown molecular formulae and masses of DOM with high accuracy (even with <0.5 ppm) and resolution (up to 240,000) (D’Andrilli et al., 2015). Orbitrap analysis could improve our understanding of DOM by identifying the possible formulae of DOM assigned with few elements, such as C, H, O, N, and S; other elements are disregarded as they are rare in natural organic matter (Phungsai et al., 2016).
Numerous studies have been conducted in various regions of the hydrosphere on the molecular composition of DOM by HRMS, and different dominant formulae have been identified. For example, unsaturated compounds (70.4 ± 0.6%) were highly dominant in the Mediterranean Sea (Martínez-Pérez et al., 2017), and lipid and lignin were the most abundant in river waters (Sleighter and Hatcher, 2008; Derrien et al., 2017). Particularly, Sleighter and Hatcher (2008) reported a decrease of aromaticity toward offshore from inshore using Fourier-transform ion cyclotron resonance MS. Recently, studies on molecular compositions using HRMS were conducted in arctic areas and reported 38–71% terrestrial components (i.e., lignin) due to the influence of river discharge or melting snow and ice runoff (Hodgkins et al., 2016; Mangal et al., 2017; Brogi et al., 2018). There have also been several studies on DOM in the Antarctic (Dittmar and Koch, 2006; D’Andrilli et al., 2015); however, the samples were collected in austral spring and summer, such that the results were geographically and seasonally limited to cover the whole season of Antarctica. Therefore, the compositions of the Antarctic DOM and its temporal or spatial variations at the molecular level are required to deepen our understanding of Antarctica and its role in global carbon cycle and climate change.
We studied the chemical composition of DOM in the ice shelf systems of King George Island onboard the Korean icebreaker R/V Araon from April to May 2018 (austral fall). For this, we collected surface water samples (sampling depth: 0–1 m) from open sea (OS) and fjord (FJ) areas in Antarctica and analyzed the characteristics of the DOM in the samples. The main objectives of this study were (1) to analyze the optical signatures of chromophoric and fluorescent DOM (CDOM and FDOM, respectively, using spectrophotometry, (2) to qualitatively evaluate the chemical properties and the components of DOM on a molecular level using an Orbitrap, and (3) to predict the potential sources of DOM based on the environmental conditions of the Antarctic seawater.
Materials and Methods
Sample Collection and Preparation
The Antarctic seawater sampling was conducted onboard the Korean icebreaker R/V Araon from April to May 2018 (austral fall). Surface seawater samples were collected from two open sea locations (OS1 and OS2) and four fjord locations (FJ1–FJ4), as shown in Figure 1. The open sea areas, OS1 and OS2, were located between the Antarctic peninsula and King George Island (KGI), where the east part of Bransfield Strait meets the Antarctic Slope Front (Thompson et al., 2009). Samples FJ1 to FJ4 (0.94 to 7.05 km from the innermost part of a glacier) were collected from Marian cove, which is a glacier fjord (approximately 4 km in length and 1.5 km in width) located in Maxwell bay on the southwest side of KGI. Tidewater glaciers with a catchment area of approximately 15 km2 are well developed in the inner cove (especially for sample FJ1). Glacier calving occurs, introducing significant volumes of meltwater and icebergs into the seawater. This glacier retreated approximately 1,700 m from 1956 to 2006 (Lee et al., 2008). In this study, the latitude, the longitude, the temperature, and the salinity of the water samples at each site were measured using a probe for measuring conductivity–temperature–depth connected to a rosette sampler (Supplementary Table S1).
Two-liter surface samples were collected at each site and immediately frozen and stored at –20°C in the dark during transport to our laboratory at Ulsan National Institute of Science and Technology in South Korea. Spencer et al. (2007) reported that freezing and thawing steps caused decreased DOM concentration and varying DOM composition. However, DOM is a heterogeneous and complex substance because of its various sources and surrounding conditions. The specific characteristics of DOM often generate contrary results in the same analysis. Otero et al. (2007) performed experiments regarding the effects of freezing/thawing on DOM in salt marsh pore water, but they found no variation in DOM fluorescence properties. In addition, Yamashita et al. (2007) and Walker et al. (2009) reported that no problematic differences in DOM characteristics existed between fresh and frozen samples. Consequently, freezing is a commonly used storage method in marine DOM studies (Amon et al., 2003; Stedmon et al., 2011; Chen et al., 2018). The transported samples were then filtered through 0.45-μm cellulose acetate membrane filters (Advantec, Japan) for DOM characterization.
Dissolve Organic Carbon, Dissolved Organic Nitrogen, and Chromophoric DOM Characterization
Filtered samples were loaded into precombusted borosilicate glass vials. The concentrations of dissolved organic carbon (DOC) and dissolved organic nitrogen (DON) were analyzed using a TOC analyzer (TOC-VCPH; Shimadzu, Japan) equipped with a total nitrogen measuring unit (TNM-1; Shimadzu, Japan) and autosampler (ASI-V; Shimadzu, Japan) by the high-temperature combustion method. All DOC and DON samples (5 ml) were analyzed in duplicate or triplicate, and their standard deviations are presented as error bars in Figures 2–5.
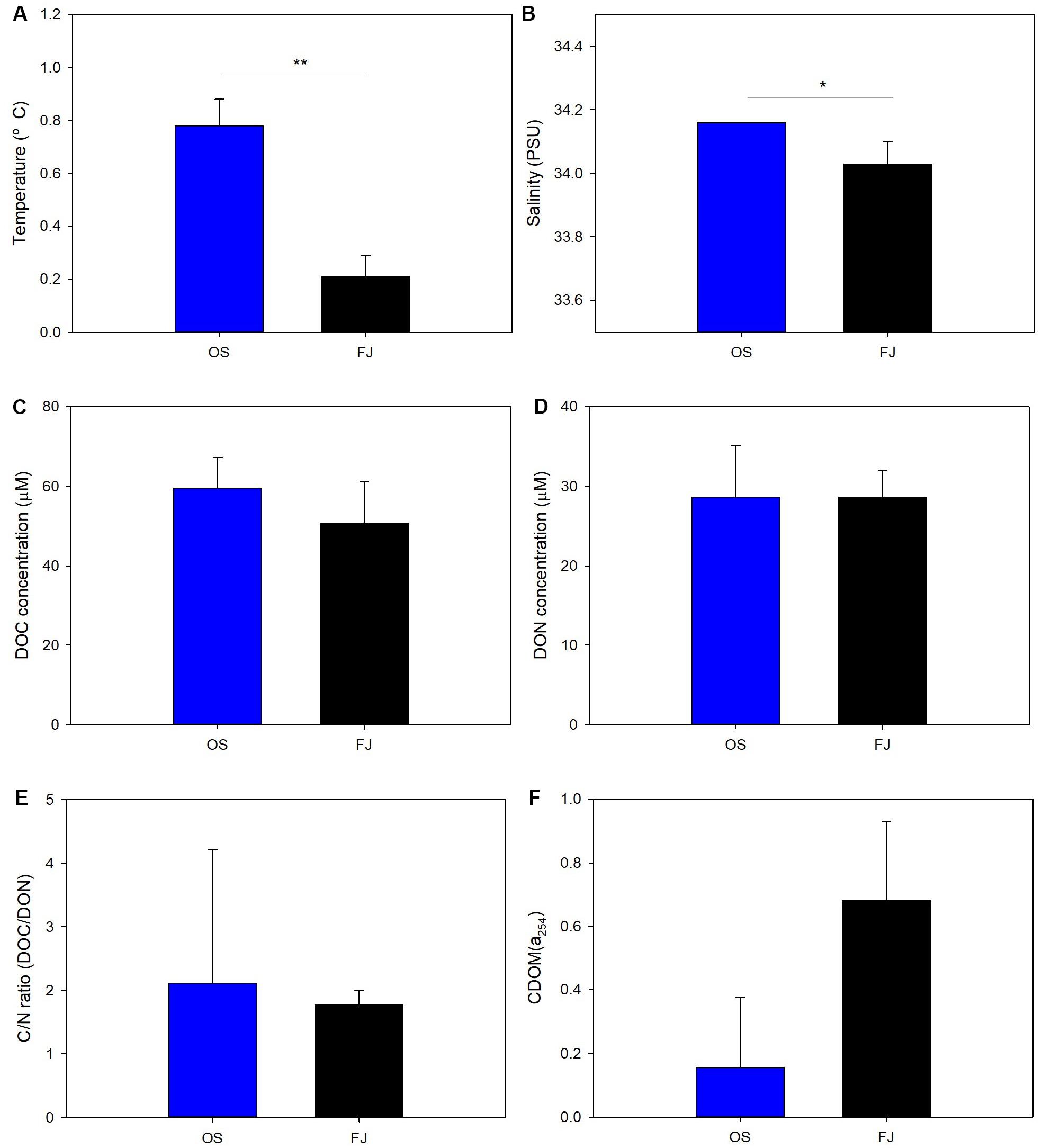
Figure 2. Physical characteristics: (A) temperature, (B) salinity, dissolved organic matter (DOM) abundances [(C) dissolved organic carbon concentrations, (D) dissolved organic nitrogen concentrations, (E) C/N ratio], and (F) chromophoric DOM absorption coefficient of surface seawater. The blue and black bars indicate open sea and fjord, respectively. The error bars refer to the standard deviation. *p-value < 0.05, **p-value < 0.01.
The absorbance of CDOM was measured with a 10-cm quartz cuvette using an ultraviolet–visible spectrometer (Scinco, South Korea). Ultrapure water (18 Mohm) was used as a reference, and the spectra were measured from 190 to 1,100 nm at 1-nm intervals. The optical density at a specific wavelength of the measured absorbance was converted to the CDOM absorption coefficient using the following equation (Bricaud et al., 1981):
In the above equation, a(λ) is the absorption coefficient (m–1) at λ wavelength, 2.303 is the transform coefficient, A(λ) is the optical density at λ wavelength, and l is the optical path length (m). In this study, we derived the CDOM value at 254 nm [i.e., a(254)], which is widely used as a proxy for terrestrial DOC in CDOM and DOM studies (Asmala et al., 2012; Zhao et al., 2016).
Fluorescence Characterization
The excitation–emission matrixes (EEMs) of the filtered samples and ultrapure water (18 Mohm) were scanned using a fluorescence spectrometer (Varian, United States). The excitation wavelength range was between 250 and 500 nm, and the emission was between 280 and 600 nm at 10-nm increments. A blank ultrapure water EEM spectrum was extracted from the sample EEMs, and water Raman peak normalization was performed. In this study, the five major fluorescent components were classified based on the criteria of Coble (2007) and quantified based on the equations in Chen et al. (2003), that is: peaks A (terrestrial humic-like; λEx 260 nm/λEm 400–460 nm), M (marine humic-like; λEx 290–310 nm/λEm 370–410 nm), C (terrestrial humic-like; λEx 320–360 nm/λEm 420–460 nm), B (tyrosine, protein-like; λEx 270 nm/λEm 310 nm), and T (tryptophan, protein-like; λEx 270 nm/λEm 340 nm). Moreover, three indicators as proxies of sources–fluorescence index (FI), humification index (HIX), and biological index (BIX) – were calculated using the excitation and the emission intensities, as described in Supplementary Table S2. The FI is known as a proxy for humic substances in aquatic environments and represents the fluorescence intensity ratio at wavelengths λEx 370 nm/λEm 470 nm and λEx 370 nm/λEm 520 nm (McKnight et al., 2001). When its FI value is larger than 1.9, DOM is considered to originate from microorganisms and contain few aromatic structures. In comparison, FI values smaller than 1.4 are considered as highly correlated with terrestrial DOM, which has highly aromatic characteristics (McKnight et al., 2001). BIX is an index showing the intensity ratio at wavelengths λEx 250 nm/λEm 300–340 nm and λEx 250 nm/λEm 430–480 nm. DOM with BIX values of 0.6 or less are generally of terrestrial origin, while that with values larger than 1 are predominantly of marine biological origin. Furthermore, HIX values are indices of the intensities at wavelengths λEx 310 nm/λEm 380 nm and λEx 310 nm/λEm 430 nm and indicate the degradability of organic matter (Zsolnay et al., 1999).
Liquid Chromatography–Orbitrap Mass Spectrometry
To concentrate the marine DOM, solid phase extraction (SPE) was conducted as a pretreatment step for analysis by Orbitrap-MS. One liter of each pre-filtered water sample was passed through a hydrophilic–lipophilic balanced (HLB) SPE cartridge (6 cc, 500 mg, Oasis-HLBTM, Waters, United States) under vacuum for DOM extraction. The HLB cartridge was water-wettable and reversed-phase, with the capacity to extract both hydrophobic and hydrophilic compounds, and was therefore used to extract all unknown organic matter in this study. The extraction steps were performed based on the methods described by Hao et al. (2006). Briefly, the SPE cartridges were preconditioned before use by washing with high-performance liquid chromatography-grade methanol and equilibrating with ultrapure water (18 Mohm). For the elution step, the cartridge was first washed with 10 ml of 5% methanol in water; then, the retained DOM was eluted with 10 ml of 100% methanol. The eluates were evaporated under a gentle flow of nitrogen gas at 34°C, reconstituted with 1 ml of methanol, and filtered with syringe filter (0.2 μm, 13 mm, Advantec). The filtered samples were stored at −20°C in the dark before analysis.
The filtered SPE extracts (10 μl) were directly injected into an Orbitrap Q-Exactive spectrometer (Thermo Fisher Scientific, Germany) coupled with liquid chromatography (Ultimate 3000, Dionex Co., United States). The AcclaimTM C18 column (2.1 × 50 mm, 2.2 μm, 120 Å, Thermo Fisher Scientific, United States) was used for chromatographic separation, with methanol as mobile phase at a flow rate of 0.3 ml min–1 for 20 min. The negative electrospray ion mode using a heated electrospray ionization source was operated under the following conditions: the flow rate of ultrapure nitrogen sheath gas remained at 7 a.u., the spray needle voltage was maintained at 4.5 kV, and the capillary temperature was 320°C. To obtain mass spectra, a consistent flow rate of 300 μl/min, resolution of 70,000 with full scan mode over the range of m/z 100–1,500, and automatic gain control target of 1 × 106 were set. The LC-Orbitrap-MS was calibrated with PierceTM ESI positive ion calibration solution before analysis to ensure high accuracy. Milli-Q water (18 Mohm) was analyzed following the same procedure as that for the blank test (Supplementary Figure S3). Mass spectra and formulae assignment were conducted using a Thermo Xcalibur Qual Browser (ver. 2.5).
The molecular formulae of the m/z 100–900 range with a relative abundance were calculated with MATLAB R2017a. We visualized the molecular formulae using a van Krevelen diagram because it is a powerful graphical approach for explaining the compositions in DOM samples and has recently been applied in DOM characterization studies (Kim et al., 2003; Kim et al., 2004; Herzsprung et al., 2012; Choi et al., 2019). The van Krevelen diagrams were displayed using a FTMS Visualization (i-van Krevelen) package in Python 2.7 for subsequent analysis of ranges 0 < H/C < 2.5 and 0 < O/C < 1.5. The molecular formulae in the van Krevelen diagrams were divided into seven categories based on previous studies (Hockaday et al., 2009; Hodgkins et al., 2016): lipid-like (H/C = 1.6–2.0, O/C ≤ 0.29), protein-like (H/C = 1.5–2.0, O/C = 0.29–0.6), carbohydrate-like (H/C = 1.5–2.0, O/C = 0.6–1.0), unsaturated hydrocarbon-like (H/C = 1.0–1.6, O/C ≤ 0.29), lignin-like (H/C = 0.7–1.5, O/C = 0.29–0.65), tannin-like (H/C = 0.5–1.5, O/C = 0.65–1.0), and condensed aromatic structures (H/C ≤ 0.7, O/C ≤ 0.4).
Statistical Analysis
The samples were grouped according to spatial variability and assessed by one-way analysis of variance (ANOVA). In the ANOVA, the p-value indicates the regional difference for each area; the significant differences are considered to be represented by a p-value of <0.05. The one-way ANOVA and a t-test for post hoc analysis were calculated using SigmaPlot (Systat, United States).
Results and Discussion
Water Chemistry and DOM Abundance
The water quality parameters for each sampling station are summarized in Figure 2 and Supplementary Table S1. The surface seawater temperatures, ranging between 0.12 and 0.85°C (at 0–1 m sampling depths), for the OS and FJ samples were measured, with significantly higher temperatures being measured in the OS than in the FJ samples (t-test, p < 0.01; Figure 2A). The temperatures were close to zero in the FJ samples, with the lowest being FJ1 (Supplementary Table S1) – that nearest to the glacier – indicating that surface seawater temperature is susceptible to the influence of ice or glacier. Moreover, the salinity of surface seawater varied between 33.89 and 34.17 PSU, and the average salinity values for the OS and the FJ samples were 34.16 ± 0.00 and 34.03 ± 0.07 PSU, respectively (Supplementary Table S1). Slightly lower salinities were observed in the FJ samples, with significant differences between stations (t-test, p < 0.05; Figure 2B).
Figures 2C,D show the DOC and DON concentrations in the OS and the FJ samples. The average values of DOC and DON for all samples were 53.71 ± 9.79 and 28.62 ± 3.97 μM, respectively, with insignificant differences between OS and FJ. It was similar to the DOC levels in previous studies conducted during austral fall: 43.3 ± 2 μM in April (Carlson et al., 2000) and 45.1 ± 1.6 μM from February to April in Ross Sea (Bercovici et al., 2017). Moreover, from spring to summer, large ranges of DOC values have been recorded in the Antarctic seawater; for example, 41–64 μM DOC was detected in samples collected from November to February (Fang et al., 2020), whereas DOC varied between 40 and 102 μM in the upper mixed layer (Doval et al., 2002). The DOC values measured in this study were 1.1 to 1.9 times lower than those in the austral summer. DOC accumulates in the euphotic zone of the seawater during summer, indicating that the high concentration of DOC was produced via biological activities in the previous seasons. Meanwhile, the C/N ratio (DOC/DON) ranged between 1.63 and 2.26, and the mean value was 1.88 ± 0.26 (Figure 2E), which is lower than that in a previous research in which the C/N ratios were between 3 and 95 in Antarctica in the summer season (Thomas et al., 2001). C/N ratios below 5 usually represent a scarcity of algae and other endogenous DOM in the aquatic environment (Ni and Wang, 2015). Thus, it seems that the DOM content in the austral fall of the present study was less influenced by marine biological activity.
Optical Properties of DOM
The CDOM absorption coefficient values were calculated (Figure 2F and Supplementary Table S2), and the fluorescence intensities of each peak (A, M, C, B, and T) were calculated as percentages (Figure 3 and Supplementary Table S2) based on previous studies (Chen et al., 2003; Coble, 2007). There were no significant differences between the OS and the FJ areas in both CDOM and FDOM values (t-test, p > 0.05), indicating that the surface seawater DOM compositions in open sea and in fjord areas are not different. By the fluorescence peaks, the intensities of humic substances (i.e., peaks A, M, and C) were found to be 1.8 to 5.7 times greater than those of protein-like substances (i.e., peaks B and T, Figure 3, and Supplementary Table S2). The DOM in fresh water is mainly derived from the degradation of aquatic organic matter from forest and riparian zones where humic substances are high (Baker and Inverarity, 2004). In the case of protein-like substances (i.e., peaks B and T), those are characterized as amino-acid-like DOM derived from marine biological sources. As such, they could be more labile in abundance, depending on the marine biological activity (D’Andrilli et al., 2015). In this study, peaks B and T showed the lowest fluorescent intensity compared to the humic peaks in all sampling site groups (Figure 3), which may be related to the low C/N ratios and scarcity of microorganisms. Kang et al. (1997) reported that the biomass of microalgae in the Antarctic is very low during the austral winter due to the lack of radiance. Additionally, protein-like substances – usually characterized by the biochemical degradation of biologically derived metabolites – are generally found in the surface layer during bloom periods (Coble, 2007). Moreover, a comprehensive analysis of the fluorescence intensities of the humic and the protein regions of the EEMs – as well as the FI, BIX, and HIX – indicates that the DOM of the OS and the FJ areas are dominantly derived from terrestrial sources (Supplementary Figure S1).
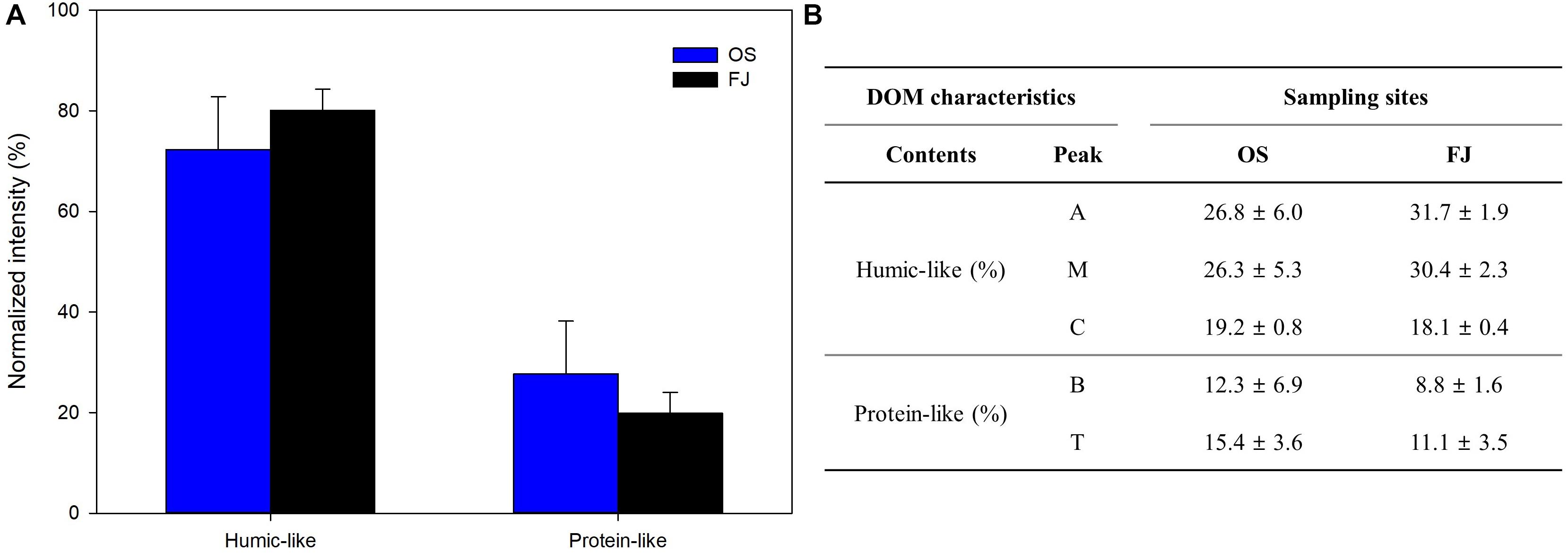
Figure 3. The fluorescence intensities of humic-like and protein-like dissolved organic matter: A, terrestrial humic-like; M, marine humic-like; C, humic-like; B, tyrosine-like; and T, tryptophan-like. The error bars refer to the standard deviation.
Molecular Properties of DOM and Identification of Source
General Properties of Molecular Compositions by Orbitrap Measurement
To characterize the DOM on a molecular level, it was extracted from the samples using SPE cartridges and then analyzed by Orbitrap. Figure 4 shows the van Krevelen spectra of the Antarctic seawater samples in the range of 100–900 m/z; a total of more than 3,500 different molecular formulae were identified. The diagrams for all samples exhibited high intensities of lipid-like (35–39%) and unsaturated hydrocarbon-like (UH; 27–34%) substances, as shown in Figure 4B. As reported in a previous study, the source of lipid-like and UH-like substances in the Antarctic waters seem to be related to the prevalence of cryptogamic organisms (e.g., moss, lichen, and algae) across Antarctica (Matsumoto et al., 2010). The authors explained that the long chains of hydrocarbons (both saturated and unsaturated) and lipids are likely from the detritus of lichen and/or vascular plants, while the short chains are related to marine biological debris (Matsumoto et al., 2010). However, previously observed results and a satellite map of seasonal variations of chlorophyll-a concentrations in the ice shelf systems showed a rapid decrease after the occurrence of a phytoplankton bloom in March—equivalent to the austral summer (Kang et al., 1997; Vernet et al., 2012; Supplementary Figure S2). Additionally, it has been reported that DOM is dependent upon vegetation when marine biological activity is low at temperatures lower than 5°C (Malik and Gleixner, 2013; Roth et al., 2015). Therefore, although further research is needed to determine whether the lipid-like and UH-like substances were affected by the bloom or the plant-derived DOM, the Antarctic surface seawater seems to be more affected by the terrestrial DOM of non-algae origin based on the results of the optical properties.
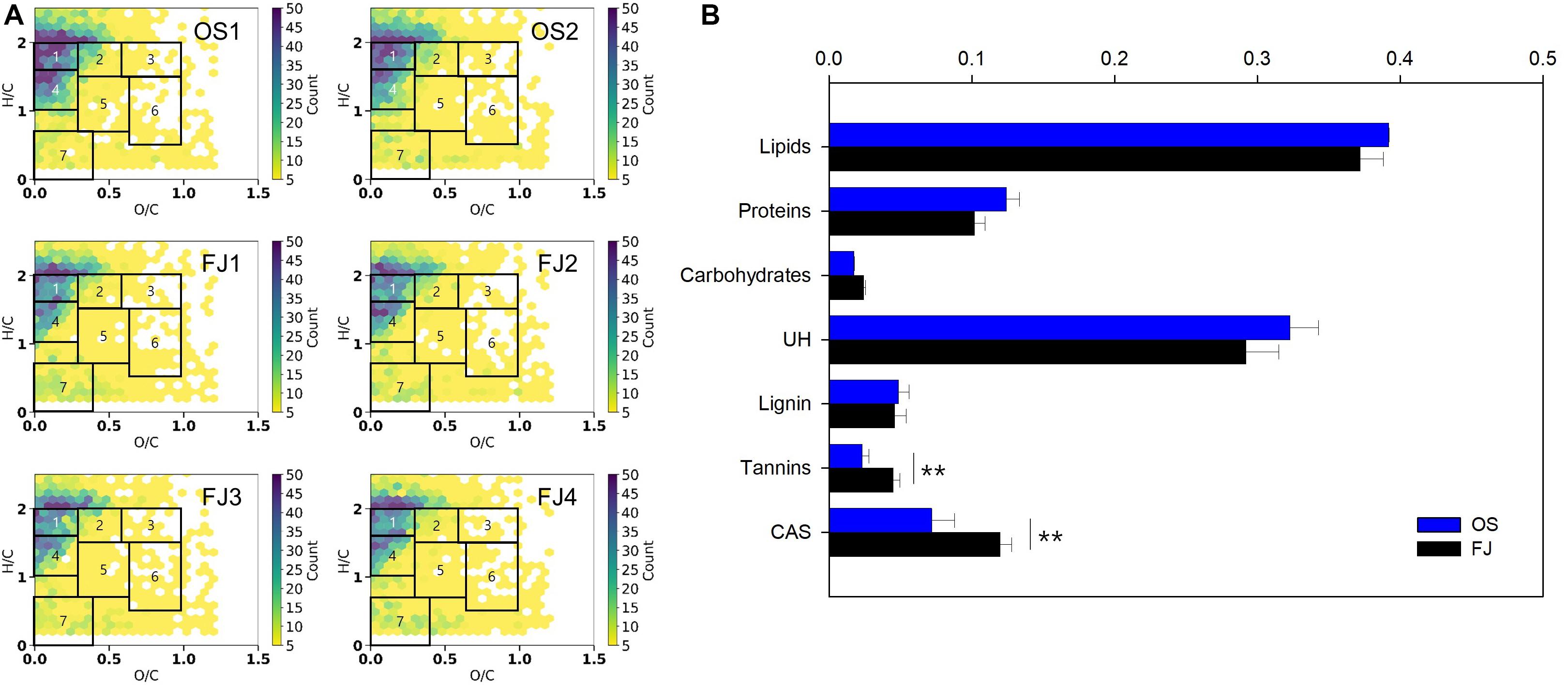
Figure 4. (A) The van Krevelen diagram of the Antarctic seawater samples in the range of 100–900 m/z and (B) the seven compound classes divided in the diagram and their normalized intensities (1, lipid-like; 2, protein-like; 3, carbohydrate-like; 4, unsaturated hydrocarbon-like; 5, lignin-like; 6, tannin-like; and 7, condensed aromatic structures). The error bars refer to the standard deviation. **p-value < 0.01.
In other studies, DOM in old accumulated snow or long-established glacier ice from the Antarctic region was analyzed, and a large portion of lignin was reported (Table 1; Grannas et al., 2006; Antony et al., 2017). Similar to these studies, in the Arctic, lignin was found to be one of the dominant components in various environments during spring flooding, when DOM deposited in snow or ice was mobilized with melting waters (Table 1). The differences between this result and those of previous studies may be influenced by the different sampling seasons (Table 1). The DOM in this study was collected in the austral fall. It has been reported that the Antarctic ice pack increases up to 21%, and melting rate decreases from the austral fall to winter (Gloersen et al., 1993; St-Laurent et al., 2015). In these circumstances, transference of DOM from ice to seawater is reduced. As such, the difference between this result and that of previous studies in dominant composition is caused by the fact that the DOM that has accumulated in ice or glacier over long periods of time does not flow into the seawater.
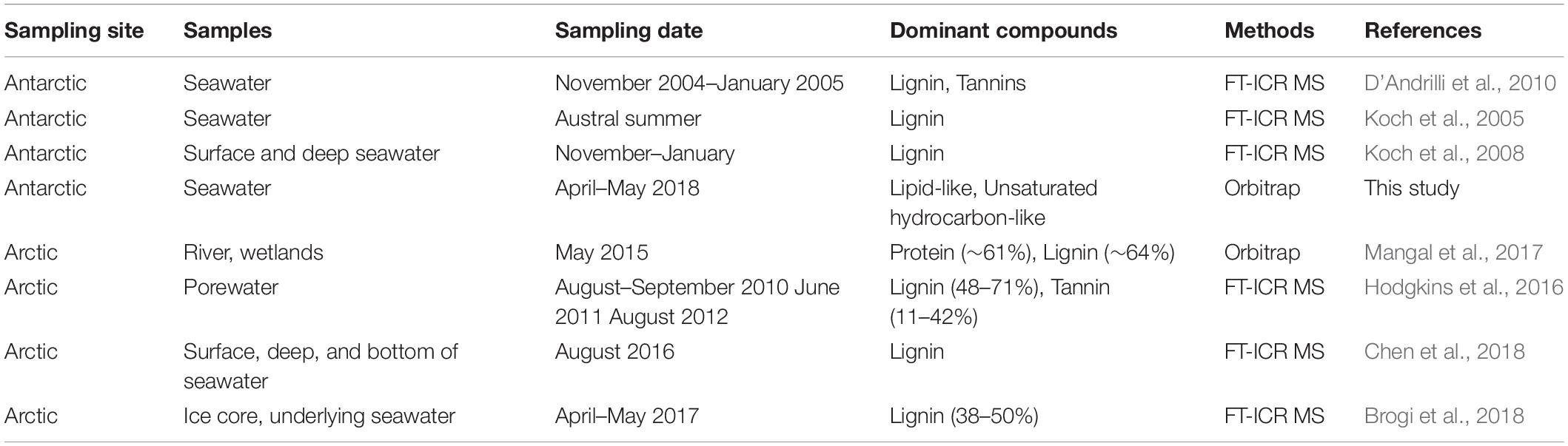
Table 1. Studies on dominant compounds in various environments of Antarctica and the Arctic by using high-resolution mass spectrometry (HRMS).
Tannin-like substance and condensed aromatic structures (CAS) were not found in high amounts but were significantly higher in the FJ area than in the OS (Figure 4B). This indicates that these substances originated from land rather than from sea. This result is consistent with previous studies in which only compounds originating from plants were found during low marine biological activity and low temperatures in winter (Malik and Gleixner, 2013; Porcal et al., 2015; Roth et al., 2015). Specifically, in glacier areas, DOM has been known to be introduced from the surrounding vegetation and soil, which is captured during the advancement of the glacier or ice sheet in the austral winter (Barker et al., 2009). Among terrestrial sources, tannin-like substance and CAS have been shown to be more related to moss than root plants (Hodgkins et al., 2014). Antarctica is covered with a variety of living organisms adapted to extreme weather conditions—one of the major flora being moss (Fowbert and Smith, 1994; Matsumoto et al., 2010; Singh et al., 2018). Despite the very slow growth rate of moss—1 mm per year—moss-covered green areas in Antarctica continue to grow due to climate change. According to a 26-year monitoring study, populations of Deschampsia antarctica and Colobanthus quitensis, native cryptogamic organisms, increased by 25 and five times, respectively, with increasing temperature (Fowbert and Smith, 1994). Moreover, a recent study, which investigated moss in a 600-km transect in Antarctica, found that moss growth rates are four to five times faster than those in the 1950s (Amesbury et al., 2017). Therefore, the refractory terrestrial DOM—such as tannin-like substance and CAS—could rapidly increase in the seawaters in Antarctica in the future with the increased growth rate of mosses and melting of land ice and glaciers due to climate change. Furthermore, the findings in this study implicate background results for high marine biological activity in summer.
Influence of the Runoff Water From Freshly Melting Land Ice and Glacier
The concentration and properties of the DOM were investigated according to its distance from a glacier to determine if it was of terrestrial origin. In salinity, it was found that the salinity of FJ1 was lower than that of FJ2–FJ4 (Figure 5A). Moreover, there were increasing trends in the concentrations of DOC and CDOM toward the FJ1 site (Figures 5B,D). Salinity also negatively correlated with DOC (r2 = 0.53, Figure 5E) and CDOM (r2 = 0.54, Figure 5E) values, although the regressions were not significant (p-values > 0.05). There were no significant trends in DON except for FJ4 (Figures 5C,E). These results indicate that DOM created by living organisms on the ground entered the seawater with runoff from the freshly melting land ice and glacier. Previous studies have observed continuous crevassing and erosion of the glacier at the head of Marian cove (Khim et al., 2007; Yoo et al., 2015). The surface waters in this cove are not readily affected by resuspension because the inner basin is deep (120 m), whereas terrestrial and supraglacial meltwaters from the glacier influenced the surface water of the cove, specifically in austral summer (Yoo et al., 2015; Llanillo et al., 2019). Surface water was actively exchanged between Marian cove and Maxwell Bay, and the bay mouth is connected to Bransfield Strait (Yoo et al., 2015). Consequently, terrestrial DOM can be transported to offshore KGI by tides.
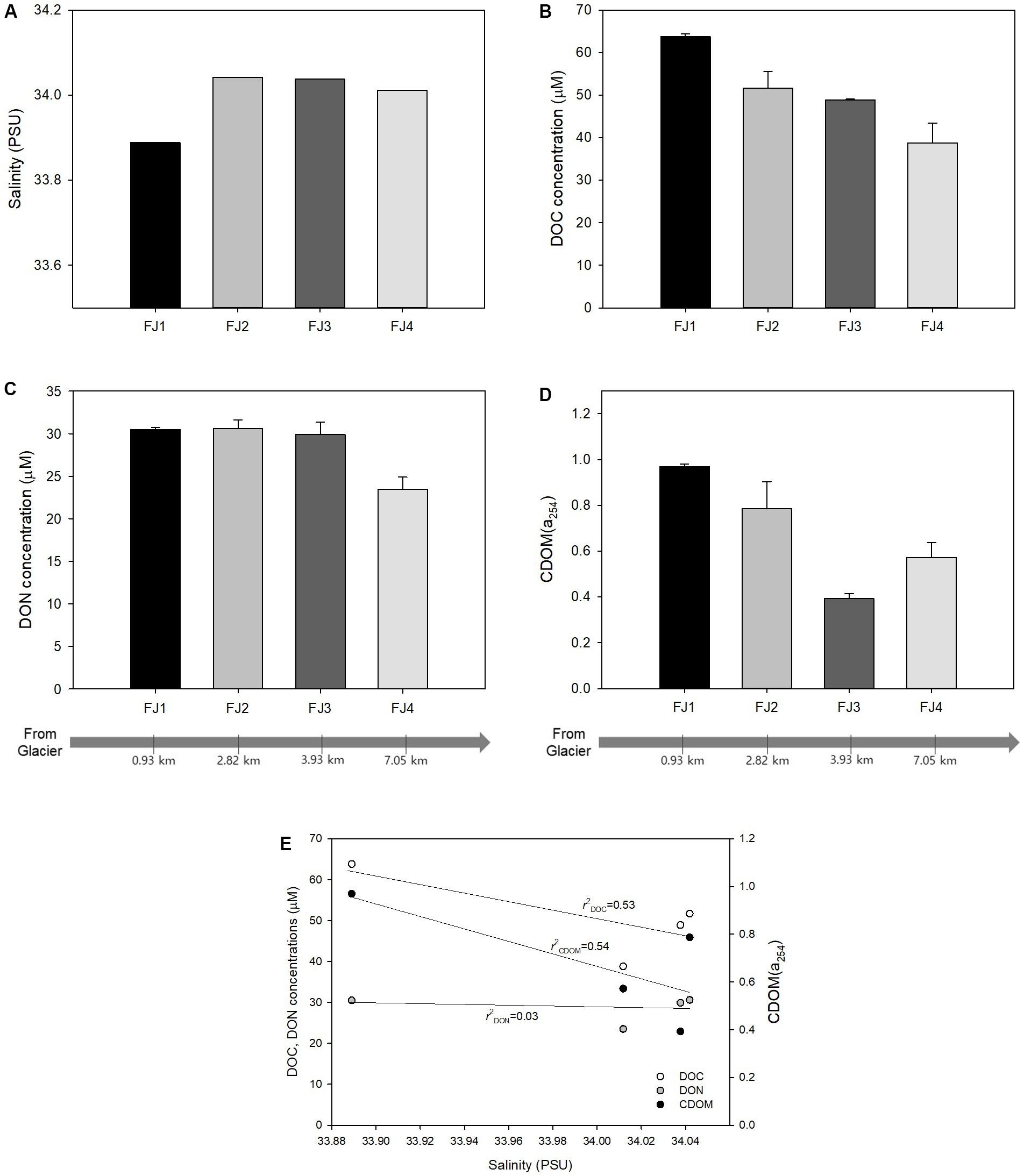
Figure 5. (A) Salinity, (B) dissolved organic carbon concentration, (C) dissolved organic nitrogen concentration, (D) chromophoric dissolved organic matter (DOM) at 254 nm in the fjord area, and (E) relationships between salinity and DOM. The error bars refer to the standard deviation.
Furthermore, there were no trends of FDOM or molecular composition in the FJ area (FJ1–FJ4; Figure 6), indicating that there was no change in molecular composition. The biological characteristics were not significant since there were not enough factors that could affect DOM degradation. According to previous studies, the factors that affect DOM degradation include photo-oxidation, marine biological activity, and temperature. These studies support our findings that low light levels and temperatures in winter undermine marine biological decomposition (Porcal et al., 2015; Roth et al., 2015). Particularly, in Marian Cove, the number of microorganisms was lower in austral winter, when water temperatures were relatively low, than in austral spring–summer (Kang et al., 2000, 2002).
To summarize, the DOM concentrations in the ice shelf systems seem to increase by the impact of DOM originating from land and introduced via fresh melt runoff from the land ice and glacier. However, there were no significant changes in composition resulting from low marine biological degradation due to low light and temperature. Therefore, we expected that the effect of terrestrial DOM on marine DOM would increase, especially due to increased land ice and glacier runoff caused by climate warming and increased temperature in the austral summer. The increased DOM could increase primary organic-rich aerosol production, which could exacerbate climate warming. Park J. et al. (2019) recently found that the large amount of freshwater from river runoff may substantially impact primary aerosol production, possibly affecting cloud radiative forcing. We further showed that riverine organic matter, especially terrestrial DOC, can be directly emitted from surface seawater to the Arctic atmosphere via bubble bursting (Park J. et al., 2019).
Conclusion and Environmental Implication
We investigated the characteristics of DOM from the Antarctic surface seawaters using optical spectrometry and Orbitrap-MS and obtained the following results:
• The DOM in the Antarctic seawater was more affected by terrestrial than marine biological sources due to the lower marine biological activities in the austral fall.
• Both lipid-like and UH-like substances were dominant in the ice shelf systems of King George Island, which is believed to be the result of non-algal and terrestrial organisms such as cryptogams spread across Antarctica.
• The DOM in the ice shelf systems was found to be affected by non-algal and terrestrial organisms such as cryptogams without any change in molecular composition, which are introduced via land ice and glacier runoff in the austral fall.
Climate warming has caused the rapid growth of cryptogamic organisms and extended their habitat. We could not evaluate the contribution of cryptogamic organisms to increasing DOM in the Antarctic Ocean due to the insufficient sampling numbers (six samples) and sampling location (King George Island). Nonetheless, the effects of terrestrial DOM on marine DOM are expected to increase, especially due to the effects of increased cryptogamic organisms and increased land ice and glacier runoff in the austral summer. Moreover, DOM originating from terrestrial organisms is expected to significantly impact the global atmosphere by increasing the primary organic aerosol production, which could contribute to climate warming. Therefore, more sampling and molecular-level DOM analysis over the Antarctic Ocean should be conducted to provide a much clearer evidence of the contribution of cryptogamic organisms.
Data Availability Statement
All datasets generated for this study are included in the article/Supplementary Material.
Author Contributions
JJ carried out the experiments of the Antarctic seawater samples came from the institute involving JiP, SA, K-TP, and S-YH. JiP and KC contributed to the conception of this study and editing the manuscript. K-TP, S-YH, and JoP also contributed to the conception of the study and the interpretation of results. SA collected samples in the Antarctica and helped method section. JJ wrote the manuscript with contributions and comments from all co-authors.
Funding
This research was supported by the KOPRI projects (PE20060 and PE20120), the Basic Science Research Program (No. 2017R1D1A1B04033074), and the Global Ph.D. Fellowship Program (No. 2019H1A2A1074315) through the National Research Foundation of Korea, funded by the Ministry of Education.
Conflict of Interest
The authors declare that the research was conducted in the absence of any commercial or financial relationships that could be construed as a potential conflict of interest.
Acknowledgments
We are grateful to the captain and crew of R/V Araon for their enthusiastic assistance during the cruise.
Supplementary Material
The Supplementary Material for this article can be found online at: https://www.frontiersin.org/articles/10.3389/fmars.2020.00339/full#supplementary-material
References
Amesbury, M. J., Roland, T. P., Royles, J., Hodgson, D. A., Convey, P., Griffiths, H., et al. (2017). Widespread biological response to rapid warming on the Antarctic Peninsula. Curr. Biol. 27, 1616–1622. doi: 10.1016/j.cub.2017.04.034
Amon, R. M., Budéus, G., and Meon, B. (2003). Dissolved organic carbon distribution and origin in the Nordic Seas: exchanges with the Arctic Ocean and the North Atlantic. J. Geophys. Res. Oceans 108:14. doi: 10.1029/2002JC001594
Antony, R., Willoughby, A. S., Grannas, A. M., Catanzano, V., Sleighter, R. L., Thamban, M., et al. (2017). Molecular insights on dissolved organic matter transformation by supraglacial microbial communities. Environ. Sci. Technol. 51, 4328–4337. doi: 10.1021/acs.est.6b05780
Asmala, E., Stedmon, C. A., and Thomas, D. N. (2012). Linking CDOM spectral absorption to dissolved organic carbon concentrations and loadings in boreal estuaries. Estuar. Coast. Shelf Sci. 111, 107–117. doi: 10.1016/j.ecss.2012.06.015
Baek, S., Jeon, J., Lee, H., Park, J., and Cho, K. H. (2019). Investigating influence of hydrological regime on organic matters characteristic in a Korean Watershed. Water 11:512. doi: 10.3390/w11030512
Baker, A., and Inverarity, R. (2004). Protein-like fluorescence intensity as a possible tool for determining river water quality. Hydrol. Process. 18, 2927–2945. doi: 10.1002/hyp.5597
Barker, J., Sharp, M., and Turner, R. (2009). Using synchronous fluorescence spectroscopy and principal components analysis to monitor dissolved organic matter dynamics in a glacier system. Hydrol. Process. Intern. J. 23, 1487–1500. doi: 10.1002/hyp.7274
Bercovici, S. K., Huber, B. A., DeJong, H. B., Dunbar, R. B., and Hansell, D. A. (2017). Dissolved organic carbon in the ross sea: deep enrichment and export. Limnol. Oceanogr. 62, 2593–2603. doi: 10.1002/lno.10592
Bricaud, A., Morel, A., and Prieur, L. (1981). Absorption by dissolved organic matter of the sea (yellow substance) in the UV and visible domains. Limnol. Oceanogr. 26, 43–53. doi: 10.4319/lo.1981.26.1.0043
Brogi, S. R., Ha, S.-Y., Kim, K., Derrien, M., Lee, Y. K., and Hur, J. (2018). Optical and molecular characterization of dissolved organic matter (DOM) in the Arctic ice core and the underlying seawater (Cambridge Bay, Canada): implication for increased autochthonous DOM during ice melting. Sci. Total Environ. 627, 802–811. doi: 10.1016/j.scitotenv.2018.01.251
Carlson, C. A., Hansell, D. A., Peltzer, E. T., and Smith, W. O. (2000). Stocks and dynamics of dissolved and particulate organic matter in the southern Ross Sea, Antarctica. Deep Sea Res. Part II Top. Stud. Oceanogr. 47, 3201–3225. doi: 10.1016/S0967-0645(00)00065-65
Chen, M., Jung, J., Lee, Y. K., and Hur, J. (2018). Surface accumulation of low molecular weight dissolved organic matter in surface waters and horizontal off-shelf spreading of nutrients and humic-like fluorescence in the Chukchi Sea of the Arctic Ocean. Sci. Total Environ. 639, 624–632. doi: 10.1016/j.scitotenv.2018.05.205
Chen, W., Westerhoff, P., Leenheer, J. A., and Booksh, K. (2003). Fluorescence excitation- emission matrix regional integration to quantify spectra for dissolved organic matter. Environ. Sci. Technol. 37, 5701–5710. doi: 10.1021/es034354c
Choi, J., Jang, E., Yoon, Y., Park, J., Kim, T. W., Becagli, S., et al. (2019). Influence of biogenic organics on the chemical composition of Arctic aerosols. Glob. Biogeochem. Cycles 33, 1238–1250. doi: 10.1029/2019GB006226
Coble, P. G. (2007). Marine optical biogeochemistry: the chemistry of ocean color. Chem. Rev. 107, 402–418. doi: 10.1021/cr050350+
D’Andrilli, J., Cooper, W. T., Foreman, C. M., and Marshall, A. G. (2015). An ultrahigh-resolution mass spectrometry index to estimate natural organic matter lability. Rapid Commun. Mass Spectrom. 29, 2385–2401. doi: 10.1002/rcm.7400
D’Andrilli, J., Dittmar, T., Koch, B. P., Purcell, J. M., Marshall, A. G., and Cooper, W. T. (2010). Comprehensive characterization of marine dissolved organic matter by Fourier transform ion cyclotron resonance mass spectrometry with electrospray and atmospheric pressure photoionization. Rapid Commun. Mass Spectrom. 24, 643–650. doi: 10.1002/rcm.4421
Derrien, M., Lee, Y., and Hur, J. (2017). Comparing the spectroscopic and molecular characteristics of different dissolved organic matter fractions isolated by hydrophobic and anionic exchange resins using fluorescence spectroscopy and FT-ICR-MS. Water 9:555. doi: 10.3390/w9080555
Dittmar, T., and Koch, B. P. (2006). Thermogenic organic matter dissolved in the abyssal ocean. Mar. Chem. 102, 208–217. doi: 10.1016/j.marchem.2006.04.003
Doval, M. D., Álvarez-Salgado, X. A., Castro, C. G., and Pérez, F. F. (2002). Dissolved organic carbon distributions in the bransfield and gerlache straits, Antarctica. Deep Sea Res. Part II Top. Stud. Oceanogr. 49, 663–674. doi: 10.1016/S0967-0645(01)00117-5
Fang, L., Lee, S., Lee, S.-A., Hahm, D., Kim, G., Druffel, E. R., et al. (2020). Removal of refractory dissolved organic carbon in the Amundsen Sea, Antarctica. Sci. Rep. 10, 1–8. doi: 10.1038/s41598-020-57870-6
Fowbert, J. A., and Smith, R. I. L. (1994). Rapid population increases in native vascular plants in the Argentine Islands, Antarctic Peninsula. Arctic Alpine Res. 26, 290–296. doi: 10.2307/1551941
Gloersen, P., Campbell, W., Cavalieri, D., Comiso, J., Parkinson, C., and Zwally, H. (1993). Arctic and Antarctic Sea Ice, 1978–1987. Satellite Passive-Microwave Observations and Analysis 290. Available online at: https://www.igsoc.org/annals/17/igs_annals_vol17_year1993_pg149-154.pdf
Grannas, A. M., Hockaday, W. C., Hatcher, P. G., Thompson, L. G., and Mosley-Thompson, E. (2006). New revelations on the nature of organic matter in ice cores. J. Geophys. Res. Atmos. 111:D04304. doi: 10.1029/2005JD006251
Hansell, D. A., and Carlson, C. A. (2002). Biogeochemistry of Marine Dissolved Organic Matter. Cambridge, MA: Academic Press.
Hao, C., Lissemore, L., Nguyen, B., Kleywegt, S., Yang, P., and Solomon, K. (2006). Determination of pharmaceuticals in environmental waters by liquid chromatography/electrospray ionization/tandem mass spectrometry. Anal. Bioanal. Chem. 384, 505–513. doi: 10.1007/s00216-005-0199-y
Herzsprung, P., von Tümpling, W., Hertkorn, N., Harir, M., Büttner, O., Bravidor, J., et al. (2012). Variations of DOM quality in inflows of a drinking water reservoir: linking of van krevelen diagrams with EEMF spectra by rank correlation. Environ. Sci. Technol. 46, 5511–5518. doi: 10.1021/es300345c
Hockaday, W. C., Purcell, J. M., Marshall, A. G., Baldock, J. A., and Hatcher, P. G. (2009). Electrospray and photoionization mass spectrometry for the characterization of organic matter in natural waters: a qualitative assessment. Limnol. Oceanogr. Methods 7, 81–95. doi: 10.4319/lom.2009.7.81
Hodgkins, S. B., Tfaily, M. M., McCalley, C. K., Logan, T. A., Crill, P. M., Saleska, S. R., et al. (2014). Changes in peat chemistry associated with permafrost thaw increase greenhouse gas production. Proc. Natl. Acad. Sci. U.S.A. 111, 5819–5824. doi: 10.1073/pnas.1314641111
Hodgkins, S. B., Tfaily, M. M., Podgorski, D. C., McCalley, C. K., Saleska, S. R., Crill, P. M., et al. (2016). Elemental composition and optical properties reveal changes in dissolved organic matter along a permafrost thaw chronosequence in a subarctic peatland. Cell 187, 123–140. doi: 10.1016/j.gca.2016.05.015
Hur, J., Shin, J.-K., and Park, S.-W. (2006). Characterizing fluorescence properties of dissolved organic matter for water quality management of rivers and lakes. J. Korea. Soc. Environ. Eng. 28, 940–948.
Kang, J., Kang, S., Lee, J., Choi, D., and Lee, S. (2000). Seasonal variation of microalgae in the surface water of Marian Cove, King George Island, the Antarctic 1998/1999. J. Korea. Soc. Environ. Eng. 18:1.
Kang, J.-S., Kang, S.-H., Lee, J. H., and Lee, S. (2002). Seasonal variation of microalgal assemblages at a fixed station in King George Island, Antarctica, 1996. Mar. Ecol. Prog. Ser. 229, 19–32. doi: 10.3354/meps229019
Kang, S.-H., Kang, J.-S., Chung, K.-H., Lee, M.-Y., Lee, B.-Y., Chung, H., et al. (1997). Seasonal variation of nearshore Antarctic microalgae and environmental factors in Marian Cove, King George Island, 1996. Korea. J. Pol. Res. 8, 9–27.
Khim, B., Shim, J., Yoon, H., Kang, Y., and Jang, Y. (2007). Lithogenic and biogenic particle deposition in an Antarctic coastal environment (Marian Cove, King George Island): seasonal patterns from a sediment trap study. Estuar. Coast. Shelf Sci. 73, 111–122. doi: 10.1016/j.ecss.2006.12.015
Kim, S., Kaplan, L. A., Benner, R., and Hatcher, P. G. (2004). Hydrogen-deficient molecules in natural riverine water samples—evidence for the existence of black carbon in DOM. Mar. Chem. 92, 225–234. doi: 10.1016/j.marchem.2004.06.042
Kim, S., Kramer, R. W., and Hatcher, P. G. (2003). Graphical method for analysis of ultrahigh-resolution broadband mass spectra of natural organic matter, the van krevelen Diagram. Anal. Chem. 75, 5336–5344. doi: 10.1021/ac034415
Koch, B. P., Ludwichowski, K.-U., Kattner, G., Dittmar, T., and Witt, M. (2008). Advanced characterization of marine dissolved organic matter by combining reversed-phase liquid chromatography and FT-ICR-MS. Mar. Chem. 111, 233–241. doi: 10.1016/j.marchem.2008.05.008
Koch, B. P., Witt, M., Engbrodt, R., Dittmar, T., and Kattner, G. (2005). Molecular formulae of marine and terrigenous dissolved organic matter detected by electrospray ionization Fourier transform ion cyclotron resonance mass spectrometry. Geochim. Cosmochim. Acta 69, 3299–3308. doi: 10.1016/j.gca.2005.02.027
Lee, J., Jin, Y., Hong, J., Yoo, H., and Shon, H. (2008). Simulation of a tidewater glacier evolution in marian cove, king george Island, Antarctica. Geosci. J. 12, 33–39. doi: 10.1007/s12303-008-0005-x
Llanillo, P., Aiken, C., Cordero, R., Damiani, A., Sepúlveda, E., and Fernández-Gómez, B. (2019). oceanographic variability induced by tides, the intraseasonal cycle and Warm Subsurface Water intrusions in Maxwell Bay, King George island (West-Antarctica). Sci. Rep. 9, 1–17. doi: 10.1038/s41598-019-54875-8
Malik, A., and Gleixner, G. (2013). Importance of microbial soil organic matter processing in dissolved organic carbon production. FEMS Microbiol. Ecol. 86, 139–148. doi: 10.1111/1574-6941.12182
Mangal, V., Shi, Y., and Guéguen, C. (2017). Compositional changes and molecular transformations of dissolved organic matter during the arctic spring floods in the lower Churchill watershed (Northern Manitoba, Canada). Biogeochemistry 136, 151–165. doi: 10.1007/s10533-017-0388-8
Martínez-Pérez, A. M., Nieto-Cid, M., Osterholz, H., Catalá, T. S., Reche, I., Dittmar, T., et al. (2017). Linking optical and molecular signatures of dissolved organic matter in the Mediterranean Sea. Sci. Rep. 7:3436. doi: 10.1038/s41598-017-03735-4
Matsumoto, G. I., Honda, E., Sonoda, K., Yamamoto, S., and Takemura, T. (2010). Geochemical features and sources of hydrocarbons and fatty acids in soils from the McMurdo Dry Valleys in the Antarctic. Pol. Sci. 4, 187–196. doi: 10.1016/j.polar.2010.04.001
McKnight, D. M., Boyer, E. W., Westerhoff, P. K., Doran, P. T., Kulbe, T., and Andersen, D. T. (2001). Spectrofluorometric characterization of dissolved organic matter for indication of precursor organic material and aromaticity. Limnol. Oceanogr. 46, 38–48. doi: 10.4319/lo.2001.46.1.0038
Nagata, T., and Kirchman, D. L. (1992). Release of dissolved organic matter by heterotrophic protozoa: implications for microbial food webs. Arch. Hydrobiol. Beih. Ergebn. Limnol. 35, 99–109.
Ni, Z., and Wang, S. (2015). Economic development influences on sediment-bound nitrogen and phosphorus accumulation of lakes in China. Environ. Sci. Pollut. Res. 22, 18561–18573. doi: 10.1007/s11356-015-5171-6
O’Dowd, C. D., and De Leeuw, G. (2007). Marine aerosol production: a review of the current knowledge. Philos. Trans. R. Soc. A 365, 1753–1774. doi: 10.1098/rsta.2007.2043
Otero, M., Mendonça, A., Válega, M., Santos, E., Pereira, E., Esteves, V. I., et al. (2007). Fluorescence and DOC contents of estuarine pore waters from colonized and non-colonized sediments: effects of sampling preservation. Chemosphere 67, 211–220. doi: 10.1016/j.chemosphere.2006.10.044
Park, J., Dall’Osto, M., Park, K., Kim, J.-H., Park, J., Park, K.-T., et al. (2019). Arctic primary aerosol production strongly influenced by riverine organic matter. Environ. Sci. Technol. 53, 8621–8630. doi: 10.1021/acs.est.9b03399
Park, S., Nam, T., You, J., Kim, E.-S., Choi, I., Park, J., et al. (2019). Evaluating membrane fouling potentials of dissolved organic matter in brackish water. Water Res. 149, 65–73. doi: 10.1016/j.watres.2018.10.079
Park, J. Y., Lim, S., and Park, K. (2014). Mixing state of submicrometer sea spray particles enriched by insoluble species in bubble-bursting experiments. J. Atmos. Ocean. Technol. 31, 93–104. doi: 10.1175/JTECH-D-13-00086.1
Phungsai, P., Kurisu, F., Kasuga, I., and Furumai, H. (2016). Molecular characterization of low molecular weight dissolved organic matter in water reclamation processes using Orbitrap mass spectrometry. Water Res. 100, 526–536. doi: 10.1016/j.watres.2016.05.047
Porcal, P., Dillon, P. J., and Molot, L. A. (2015). Temperature dependence of photodegradation of dissolved organic matter to dissolved inorganic carbon and particulate organic carbon. PLoS One 10:e0128884. doi: 10.1371/journal.pone.0128884
Rastelli, E., Corinaldesi, C., Dell’Anno, A., Martire, M. L., Greco, S., Facchini, M. C., et al. (2017). Transfer of labile organic matter and microbes from the ocean surface to the marine aerosol: an experimental approach. Sci. Rep. 7:11475. doi: 10.1038/s41598-017-10563-z
Roth, V.-N., Dittmar, T., Gaupp, R., and Gleixner, G. (2015). The molecular composition of dissolved organic matter in forest soils as a function of pH and temperature. PLoS One 10:e0119188. doi: 10.1371/journal.pone.0119188
Singh, J., Singh, R. P., and Khare, R. (2018). Influence of climate change on Antarctic flora. Pol. Sci. 18, 94–101. doi: 10.1016/j.polar.2018.05.006
Sleighter, R. L., and Hatcher, P. G. (2008). Molecular characterization of dissolved organic matter (DOM) along a river to ocean transect of the lower Chesapeake Bay by ultrahigh resolution electrospray ionization Fourier transform ion cyclotron resonance mass spectrometry. Mar. Chem. 110, 140–152. doi: 10.1016/j.marchem.2008.04.008
Spencer, R. G., Bolton, L., and Baker, A. (2007). Freeze/thaw and pH effects on freshwater dissolved organic matter fluorescence and absorbance properties from a number of UK locations. Water Res. 41, 2941–2950. doi: 10.1016/j.watres.2007.04.012
Stedmon, C. A., Thomas, D. N., Papadimitriou, S., Granskog, M. A., and Dieckmann, G. S. (2011). Using fluorescence to characterize dissolved organic matter in Antarctic sea ice brines. J. Geophys. Res. Biogeosci. 116:G03027. doi: 10.1029/2011JG001716
St-Laurent, P., Klinck, J., and Dinniman, M. (2015). Impact of local winter cooling on the melt of Pine Island Glacier, Antarctica. J. Geophys. Res. Oceans 120, 6718–6732. doi: 10.1002/2015JC010709
Takahashi, T., Sutherland, S. C., Wanninkhof, R., Sweeney, C., Feely, R. A., Chipman, D. W., et al. (2009). Climatological mean and decadal change in surface ocean pCO2, and net sea–air CO2 flux over the global oceans. Deep Sea Res. Part II 56, 554–577. doi: 10.1016/j.dsr2.2008.12.009
Thomas, D. N., Kattner, G., Engbrodt, R., Giannelli, V., Kennedy, H., Haas, C., et al. (2001). Dissolved organic matter in Antarctic sea ice. Ann. Glaciol. 33, 297–303. doi: 10.3189/172756401781818338
Thompson, A. F., Heywood, K. J., Thorpe, S. E., Renner, A. H., and Trasviña, A. (2009). Surface circulation at the tip of the Antarctic Peninsula from drifters. J. Phys. Oceanogr. 39, 3–26. doi: 10.1175/2008JPO3995.1
Vernet, M., Kozlowski, W. A., Yarmey, L. R., Lowe, A. T., Ross, R. M., Quetin, L. B., et al. (2012). Primary production throughout austral fall, during a time of decreasing daylength in the western Antarctic Peninsula. Mar. Ecol. Prog. Ser. 452, 45–61. doi: 10.3354/meps09704
Walker, S. A., Amon, R. M., Stedmon, C., Duan, S., and Louchouarn, P. (2009). The use of PARAFAC modeling to trace terrestrial dissolved organic matter and fingerprint water masses in coastal Canadian Arctic surface waters. J. Geophys. Res. Biogeosci. 114:G00F06. doi: 10.1029/2009JG000990
Yamashita, Y., Tsukasaki, A., Nishida, T., and Tanoue, E. (2007). Vertical and horizontal distribution of fluorescent dissolved organic matter in the Southern Ocean. Mar. Chem. 106, 498–509. doi: 10.1016/j.marchem.2007.05.004
Yoo, K.-C., Lee, M. K., Yoon, H. I., Lee, Y. I., and Kang, C. Y. (2015). Hydrography of marian COVE, King George Island, West Antarctica: implications for ice-proximal sedimentation during summer. Antarct. Sci. 27, 185–196. doi: 10.1017/S095410201400056X
Zhao, Y., Song, K., Li, S., Ma, J., and Wen, Z. (2016). Characterization of CDOM from urban waters in Northern-Northeastern China using excitation-emission matrix fluorescence and parallel factor analysis. Environ. Sci. Pollut. Res. 23, 15381–15394. doi: 10.1007/s11356-016-6703-4
Keywords: dissolved organic matter, terrestrial DOM, glacier runoff, ice shelf systems, King George Island, Orbitrap
Citation: Jang J, Park J, Ahn S, Park K-T, Ha S-Y, Park J and Cho KH (2020) Molecular-Level Chemical Characterization of Dissolved Organic Matter in the Ice Shelf Systems of King George Island, Antarctica. Front. Mar. Sci. 7:339. doi: 10.3389/fmars.2020.00339
Received: 09 January 2020; Accepted: 22 April 2020;
Published: 09 June 2020.
Edited by:
Johnna M. Holding, Aarhus University, DenmarkReviewed by:
Sarah Bercovici, University of Oldenburg, GermanySarah Ellen Johnston, University of Lethbridge, Canada
Copyright © 2020 Jang, Park, Ahn, Park, Ha, Park and Cho. This is an open-access article distributed under the terms of the Creative Commons Attribution License (CC BY). The use, distribution or reproduction in other forums is permitted, provided the original author(s) and the copyright owner(s) are credited and that the original publication in this journal is cited, in accordance with accepted academic practice. No use, distribution or reproduction is permitted which does not comply with these terms.
*Correspondence: Jiyeon Park, anlwYXJrQGtvcHJpLnJlLmty; Kyung Hwa Cho, a2hjaG9AdW5pc3QuYWMua3I=