- 1Department for the Monitoring and Protection of the Environment and for Biodiversity Conservation, Higher Institute for Environmental Protection and Research (ISPRA), Chioggia, Italy
- 2Department for the Monitoring and Protection of the Environment and for Biodiversity Conservation, Higher Institute for Environmental Protection and Research (ISPRA), Rome, Italy
- 3National Institute of Oceanography and Applied Geophysics (OGS), Trieste, Italy
- 4Institute of Oceanography and Fisheries (IOF), Split, Croatia
- 5Hellenic Centre for Marine Research (HCMR), Anavyssos, Greece
- 6National Institute of Biology (NIB), Marine Biology Station, Piran, Slovenia
- 7Ruder Boskovic Institute (RBI), Zagreb, Croatia
- 8University of Montenegro - Institute of Marine Biology (UoM-IMBK), Kotor, Montenegro
- 9Region of Western Greece (RWG), Patras, Greece
- 10Slovenian Environment Agency (ARSO), Ljubljana, Slovenia
- 11Faculty of Agriculture and Environment, Agriculture University of Tirana (AUT), Tirana, Albania
The Adriatic-Ionian region (ADRION Region) shows strong development in terms of urban expansion in coastal and inland areas as well as increasing maritime traffic and offshore hydrocarbon extraction activities. A serious risk of pollution arises from hazardous substances requiring reliable and coherent monitoring and assessment programs. EU Directives (WFD – Water Framework Directive, MSFD – Marine Strategy Framework Directive) and Barcelona Convention protocols, aim to assess the level of pollution with the objective to implement measures to prevent and/or mitigate impacts on the marine environment. This high level integration process has to be based on common and agreed protocols for monitoring of contaminants. Aiming to share best practices to encourage a harmonized implementation of monitoring and assessment of contaminants, an extensive review of monitoring and analytical protocols adopted by six EU and non-EU countries along the Adriatic and Ionian seas was carried out in the framework of the Interreg Adrion project HarmoNIA (Interreg V-B Adriatic-Ionian (ADRION), 2018–2020). This paper presents a methodological proposal to define a common protocol for the evaluation of the metal contamination of seawater, sediment and biota. Contaminants have been chosen following preliminary consultations among countries of the ADRION area, considering objectives of WFD and MSFD, as well as Environmental Impact Assessment (EIA) procedures for offshore platforms. Information was gathered relative to matrix characteristics and quality assurance/quality control of the analytical performance (sample preservation, analytical methodology, reference materials, limit of detection, and limit of quantification, accuracy, reproducibility, etc.). The comparison of information provided by laboratories of nine institutions highlighted the request for harmonization in terms of sampling procedures, matrix characterization, preservation procedures, analytical methods and LOQ values. Although appropriate environmental quality standards for biota and sediment matrices should be established at national level and also through regional and sub-regional cooperation, as required by the WFD and MSFD, the proposed LOQ values, even if challenging, represent a benchmark and a stimulus to optimize analytical performance, to ensure the best level of protection to the coastal and offshore environment in the ADRION Region.
Introduction
According to the Marine Strategy Framework Directive 2008/56/EC, 2008, European Union Member States should develop and follow a common approach in environmental monitoring at the level of marine region or sub-region, as well as in definition and assessment of good environmental status (GES). The new Commission Decision 2017/848/Eu (2017) has recently revised criteria and methodological standards for monitoring and assessment of GES. For contaminants, full compliance is required with Water Framework Directive (WFD) through the application of Environmental Quality Standards fixed by EU Directive 2013/39/EU, 2013 or, where these are not available, Member States shall establish threshold values through regional or sub-regional cooperation. Furthermore, countries of the Mediterranean region ratified the Mediterranean Action Plan of United Nations Environment Program (UNEP-MAP), aiming to reach and maintain GES toward the Ecosystem Approach (EcAp) by implementing an Integrated Monitoring and Assessment Programme (IMAP) coherent and consistent with MSFD for EU countries. In the Adriatic-Ionian Sea region (ADRION Region), a serious risk of pollution arises from hazardous substances due to the development of coastal urbanization, riverine inputs, increase in marine transport and offshore oil and gas extraction. EU Directives (WFD, MSFD), Barcelona Convention protocols (Dumping Protocol, Land Based Sources Protocol LBS, Offshore Protocol, Prevention and Emergency Protocol, Hazardous Wastes Protocol) and control and prevention plans defined at national level aim to assess the level of pollution with the main objective to plan, agree and implement measures to prevent, mitigate or remove impacts on the marine environment. Such measures usually require to modify the Business as Usual (BAU) scenario with socio-economic costs on human activities, so coherence and consistency between countries in the monitoring and the assessment methodology is of the utmost importance to avoid different level of environmental protection and application of the precautionary and “polluter pay” principles.
In fact, since the ratification of Barcelona Convention by Mediterranean Contracting Parties, specific monitoring and assessment programs have been developed for each Protocol with a high level of heterogeneity between them. The implementation process of WFD and MSFD, started in 2000 and 2008, respectively, by EU countries, has added a new set of activities, many of which overlap with those required by Barcelona Convention Protocols. In order to assure coherence between EU and non-EU countries in the Mediterranean region, Barcelona Convention has developed and adopted a fully and integrated ecosystem approach (EcAp) through the definition of Common Indicators grouped by Ecological Objectives. This strictly resembles MSFD Descriptors with the sole exception of the coastal zone, which is not specifically addressed by any of the EU Directives. Monitoring protocols and assessment for each of the Common Indicators have been further developed in the Integrated Monitoring and Assessment Programme (IMAP, 2016). The recent Commission Decision 2017/848/Eu (2017) on criteria and methodological standards on MSFD good environmental status has reinforced an integrated and intelligible assessment process between MSFD and WFD, aiming to harmonize the two directives regarding the spatial scale and rules of procedure to assess a “good chemical status” for the coastal and marine environment.
However, such high level integration approach would be useless if not soundly based on common and agreed protocols for monitoring contaminants. Such issue is far from being standardized for marine environment due to the different methodologies available and adopted during each monitoring survey and laboratories involved therein, with differences in sampling, storage, laboratory quality assurance (QA) process and so on.
In this context, the Interreg ADRION project HarmoNIA (Harmonization and Networking for contaminant assessment in the Ionian and Adriatic Seas) aimed to share best practices to encourage a harmonized implementation of marine environmental directives in countries bordering Adriatic-Ionian Seas. For such a scope, information on sampling procedures, analytical methodologies and quality assurance/quality control (QA/QC) procedures were collected for a list of selected pollutants in water, sediment and biota, by a questionnaire submitted to the project partners, located in 6 countries bordering the ADRION Region (Italy, Slovenia, Croatia, Montenegro, Albania and Greece). Among the various contaminants selected for the investigation, heavy metals represent a group of particular importance, considering their introduction into the environment from widespread sources, such as atmospheric fall out, riverine input and point sources pollution from coastal cities and industrial plants (Gallmetzer et al., 2017; Joksimovic et al., 2020; Cukrov et al., 2011; Igwe et al., 2013). In fact the Directive 2013/39/EU, 2013 and amended Directive 2008/105/EC, 2008 and Directive 2000/60/Ec (2000), imposed environmental quality standards (EQS – the concentration of a contaminant that should not be exceeded to protect human health and environment) for cadmium (Cd), mercury (Hg), lead (Pb), nickel (Ni) and their compounds in water. Moreover, Cd and Hg have been recognized as priority hazardous substances and EQS for mercury in biota has been introduced. Therefore, such heavy metals play a major role in GES assessment according to Descriptor 8 of MSFD, which requires that concentrations of contaminants be at levels not giving rise to pollution effects. Hg, Cd and Pb, as well as their organic compounds, are considered hazardous substances of priority also in the framework of Barcelona Convention (UNEP-MAP). Assessment criteria, such as Background Concentration (Med BCs), Background Assessment Criteria (Med BACs) and Environmental Assessment Criteria (EACs) for mussel (Mytilus galloprovincialis), fish and sediments have been also developed by UNEP-MAP as an instrument to assess and monitor the achievement of GES.
This paper has the purpose to propose a harmonized methodology for sampling procedures, matrix characterization, sample preservation procedures, analytical methods and limit of quantification (LOQ) values for selected metals, derived from the information provided by the laboratories of the six ADRION countries and assuring coherence with EU legislation and decisions adopted by countries of Barcelona Convention. We hope that such a proposal will pave the way for future legislation and regulations in the ADRION basin.
Materials and Methods
Study Area
The Adriatic-Ionian Sea region has a strategic geographical position, being located on the intersection of main ways of transport system between Mediterranean, Eastern Europe and Asia. Its rich heritage, both natural and cultural, makes it one of the world’s most attractive destinations for tourism. However, this region shows significant differences. On the one hand, some areas are characterized by high competitiveness due to excellent performance in terms of research, development and product quality certification systems. On the other hand, in the region there are numerous territories characterized by limited research capacity and low manufacturing productivity. Some regions suffer from the increase in the production of household waste and poor air quality. Imbalances are present in transport links between different states in the region (Interreg V-B Adriatic-Ionian (ADRION), 2018–2020). Despite these differences, the whole Adriatic-Ionian basin suffers from pollution of marine and coastal environment due to human activities such as the exploitation of various resources, agriculture (land runoff), urban development in the coastal area and activities related to maritime transport (harbor activities, ballast water management etc.). In particular, heavy metal pollution in ADRION Region originates from sources such as land mining activities, like mercury mine of Idrija, Slovenia (Horvat et al., 2014; Gallmetzer et al., 2017), metallurgic industries, like Taranto industrial plants (Di Leo et al., 2013), oil refinery plants (Cukrov et al., 2011; Traven et al., 2015), old-type chlor-alkali plants (Mikac et al., 2006; Kljaković-Gašpić et al., 2006; Acquavita et al., 2012), municipal-sewage outflows (Cozzi et al., 2008; Cukrov et al., 2011; Joksimovic et al., 2020), harbors (container terminals, Cukrov et al., 2011), cruise tourism (Carić and Mackelworth, 2014; Joksimovic et al., 2019), by means of produced water discharge in offshore oil and gas extraction plants (Igwe et al., 2013). The presence of metals in the coastal environment of the Adriatic-Ionian basin is also due to natural, geogenic sources: the metals contained in the eroded rocky material are transported through rivers, as observed, for example, in the case of nickel in Koper Bay, Slovenia (Rogan Šmuc et al., 2018), or in the case of chromium and nickel in the lagoon area of Amvrakikos Gulf, Greece (Vasileiadou et al., 2016).
Six countries of ADRION Region (Italy, Slovenia, Croatia, Montenegro, Albania, and Greece) contributed to the comparative study on sampling procedures, analytical methods and QA/QC procedures adopted in contaminant analysis, with the scope to develop a common protocol according to EQSD (Environmental Quality Standard Directive) requirements. The laboratories of the following nine institutions, belonging to research and monitoring sectors, shared their information: Italian Institute for Environmental Protection and Research (ISPRA, Italy), National Institute of Biology and Slovenian Environment Agency (NIB and ARSO, Slovenia), Institute of Oceanography and Fisheries and Ruder Boskovic Institute (IOF and RBI, Croatia), University of Montenegro – Institute of Marine Biology and Center for Eco-Toxicological Research (UoM-IMBK and CETI, Montenegro), Agriculture University of Tirana (AUT, Albania), Hellenic Center for Marine Research (HCMR, Greece) (Figure 1).
Questionnaire Structure
The investigation was performed through a questionnaire, prepared considering both the contaminants monitored routinely for achieving the objectives of the WFD and MSFD, and those included in the Environmental Impact Assessment (EIA) procedures of offshore platforms. The questionnaires distributed to the participants were divided into three parts, related to environmental matrices of common interest: seawater, sediment and biota (see Supplementary Material). The list of metals included in the comparative study for different matrices was reported in Table 1.
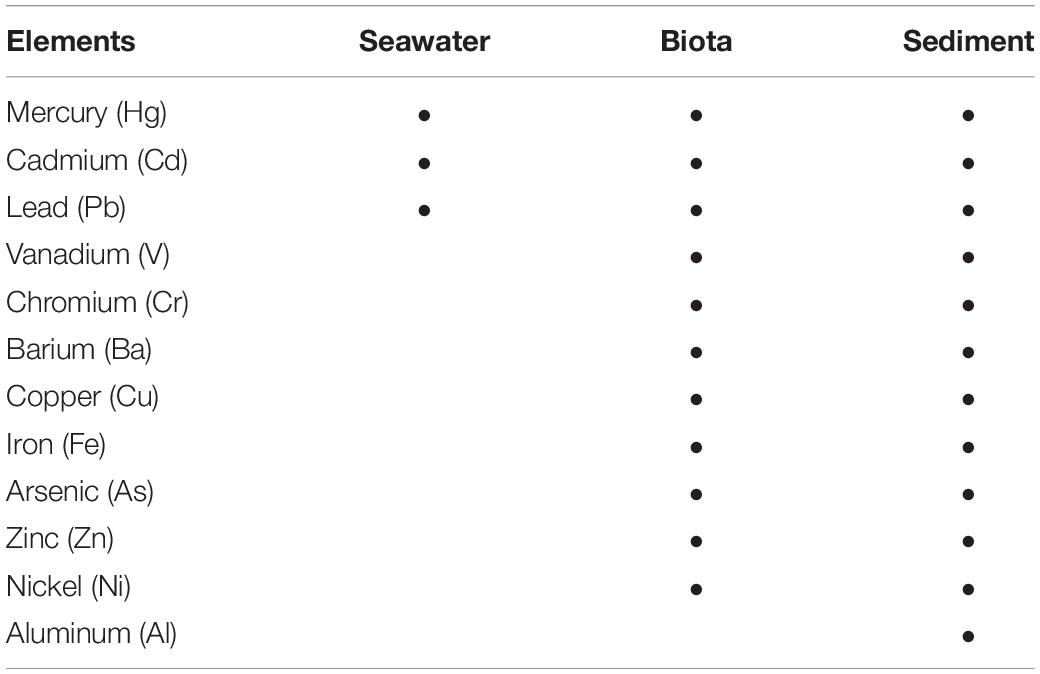
Table 1. Metals considered for comparative analysis of sampling, analytical, and QA/QC procedures in different matrices.
Among types of information requested, those related to QA/QC procedures (limit of detection – LOD, limit of quantification – LOQ, use of reference materials, participation to intercalibration circuits, accuracy and reproducibility), as well as storage and analytical methods, were common for all three matrices. Furthermore, some specific information characteristic for each matrix is reported in Table 2.
The information reported by nine laboratories, from six countries of the ADRION area was compared. Most of laboratories work in respect of requirements of ISO/IEC 17025:2005. Not all of them performed the assessment of all contaminants in all three matrices. Beside the review of data collected, for the development of harmonized protocols, several technical and legislative references, such as Directive 2013/39/EU, 2013, Oslo-Paris Convention (OSPAR), Barcelona Convention documents (UNEP) and European Commission Regulations 2006/1881/EC, 2006 and its amendments), were taken into account.
Results
Seawater Matrix
For the seawater matrix the only metals required by questionnaires were Hg, Cd, and Pb. Information on Cd and Pb was provided by one institution for each of the six countries, while information on Hg was provided by 5 of them. One of the laboratories reported additional data for nickel, copper and zinc. Among reporting laboratories, Niskin sampler made of PVC with internal silicone elastics was the most used tool for seawater sampling. Only one laboratory reported to use a home-made sampler, made of plastic such as HDPE and plexiglass with silicone seals and external closure system. Samplers employed contained external metallic parts. An acid cleaning pretreatment of the samplers was reported only by two participants. Regarding sampling cables, metal wires were mostly used, except in one case, where Kevlar cable was used. Most of the participants analyzed dissolved phase concentrations, as required by the Directive 2013/39/EU, 2013 (Figure 2), and one laboratory analyzed both dissolved and reactive particulate fraction. Only Hg was analyzed predominantly as total concentration.
All the participants who analyzed metals on dissolved phase, used to filter the sample through 0.45 μm pore size filters, but only one reported what type of filter was employed (cellulose acetate). Regarding storage, information was purchased by almost all reporting laboratories (only one did not provide data), highlighting that acidification and storage at 4°C were the most frequently employed procedures. Three types of analytical methods were used for Hg analysis and Cold Vapor Atomic Absorption Spectrometry (CV-AAS) was the most common. Regarding Cd and Pb, most laboratories employed Inductively Coupled Plasma Mass Spectrometry (ICP-MS), but voltammetry was also used (Figure 3).
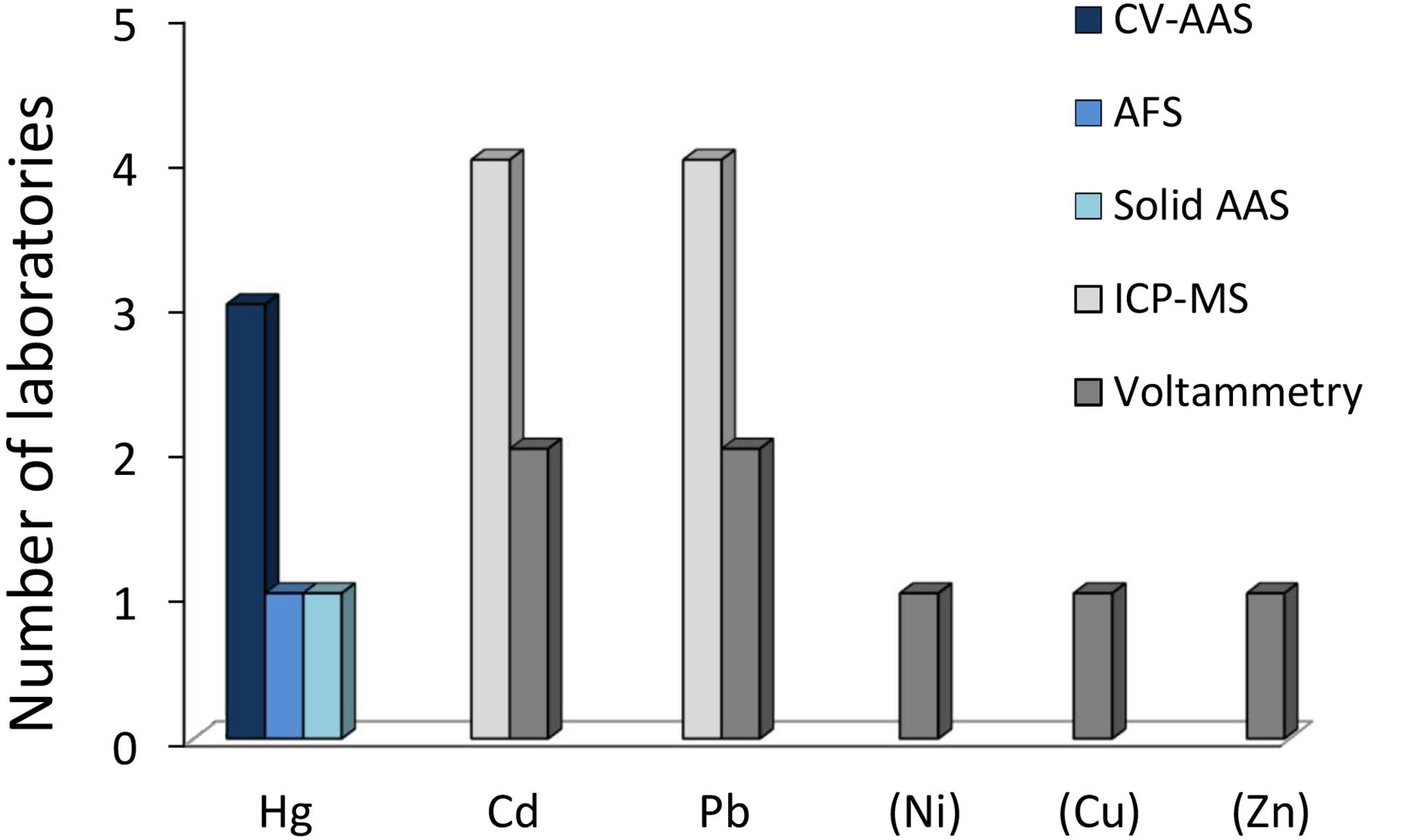
Figure 3. Analytical methods used for metal analysis in seawater by different laboratories. CV-AAS, Cold Vapor Atomic Absorption Spectrometry; CV-AFS, Cold Vapor Atomic Fluorescence Spectrometry; Solid AAS, Solid Atomic Absorption Spectrometry; ICP-MS, Inductively Coupled Plasma Mass Spectrometry.
LOD values were reported by only two participants for Hg (in the range 0.005–0.1 μg/l) and by three of them for Cd and Pb (0.001–1 μg/l and 0.026–2 μg/l, respectively). On the contrary, all participants reported LOQ for requested metals. That is the reason why the minimum LOQ value may appear lower than the minimum LOD, like in the case of Hg. The ranges of LOQ values were very wide, reaching up to 3 orders of magnitude (Table 3).
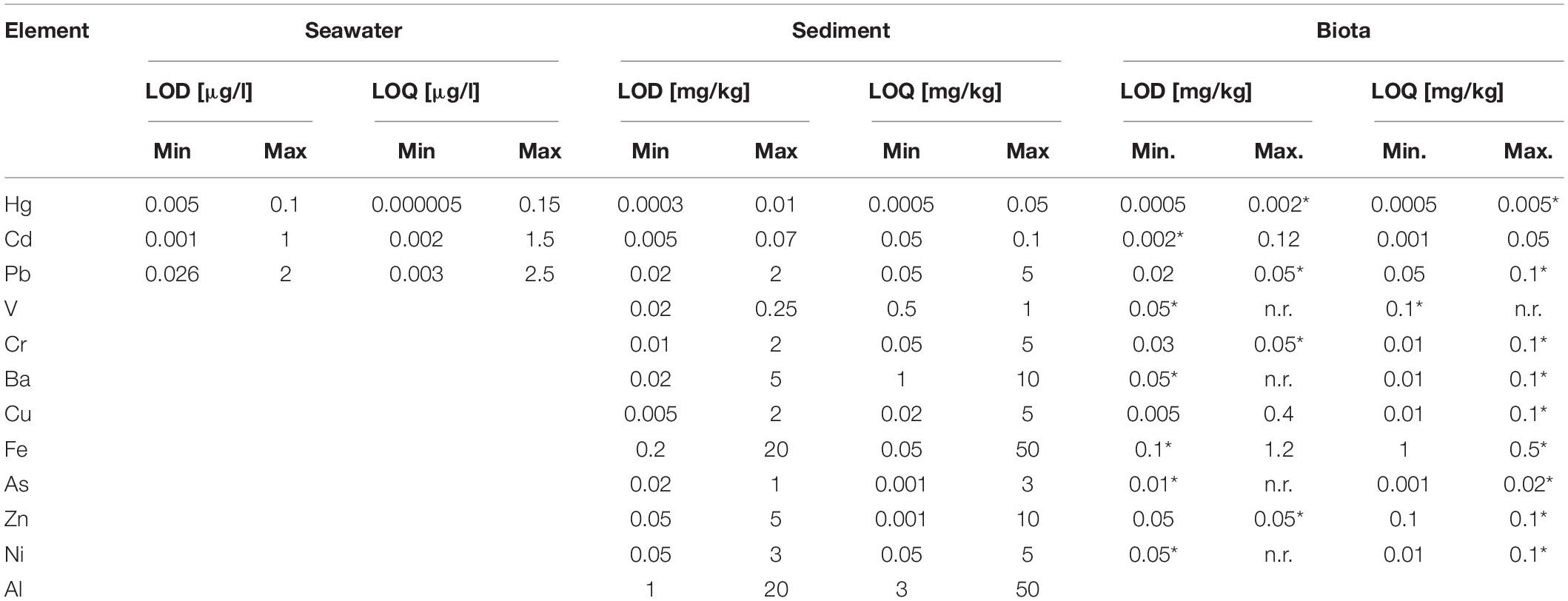
Table 3. Minimum and maximum values of LOD and LOQ values for metals in seawater, sediment and biota (Mytilus galloprovincialis) among reporting laboratories; *values expressed as wet weight (to be multiplied by a factor of 5 for the comparison with d.w. units), otherwise as dry weight (for sediment and biota); n.r. – not reported.
Different reference materials were used for determination of heavy metals in seawater in each institution. Two institutions reported to use reference materials for Hg: coastal seawater BCR-579 (JRC) and NIST SRM 1641d. Three different reference materials were employed by four laboratories for metals such as Cd and Pb: coastal seawater CASS-5 (NRC-CNRC), estuarine water SLEW-3 (NRC-CNRC) and open ocean seawater NASS-6 (NRC-CNRC).
Regarding the participation in intercalibration exercises, among institutions analyzing metals in seawater, only two laboratories reported to take part in such circuits – one participated in Quasimeme, while other referred to Acquacheck S11 and IELAB circuits.
Information on accuracy has been expressed in two different modes: as percentage of recovery with respect to certified value, or as the percentage of difference between the test result and the reference value. Only one laboratory responded regarding Hg: 101% with respect to certified value. For Cd and Pb, two participating institutions referred on accuracy: 96 and 100%, respectively (as% recovery), and 19 and 33%, respectively (as% difference). More information was presented regarding method reproducibility, expressed as relative standard deviation (% RSD), as three laboratories referred to produce data with reproducibility lower than 10%, and one institution declared 20% RSD at LOQ level. As additional information, one laboratory reported on measurement uncertainty: 33, 36, and 7%, respectively for Hg, Cd, and Pb.
Sediment Matrix
For the sediment matrix the metals considered were reported in Table 1. In addition, Co and Mn were added as supplementary parameters by two and one institution, respectively. Metal concentrations in the sediment matrix were expressed as mg/kg on dry weight basis. Seven among nine participating institutions analyzed some of the metals, while only one performed analyses of all elements included in the questionnaire. Only Pb, Cr, Cu, and Zn were analyzed by all reporting laboratories, followed by Hg, Fe, Ni (6 laboratories), and Cd (5 laboratories), while less importance was given to the rest of the elements.
Sampling of sediments was mostly performed using box corer, followed by Van Veen grab, Ponar grab and gravity corer. The thickness of the sampled sediment layer varied among participants, while one institution reported the use of two methods for some elements. Most institutes sampled a 10 cm layer, whereas, 5 and 2 cm-thick layers were also sampled. Different grain size fractions have been chosen for metals analysis and the frequency of use was in the order 63 μm > 2 mm = unsieved > 0.5 mm. One of the laboratories measured some elements analyzing two grain size fractions. Regarding storage conditions, two laboratories reported the application of the SIST ISO 5667-15 method, whereas, others apply different approaches regarding both the temperature of storage (refrigeration at 1–5°C or freezing at −20°C or −40°C) and the lyophilization of samples (some referred to freeze-dry the sediments, some used the untreated sample).
More homogeneity was found among institutions in relation to the analytical instrumentation, given that for Hg most of the analyses were carried out with CV-AAS, while for the other metals the most used technique was ICP-MS, even though flame and graphite furnace AAS and Inductively Coupled Plasma Optical Emission Spectrometry (ICP-OES) have also been used (Figure 4). One of the laboratories applied two analytical techniques for some metals.
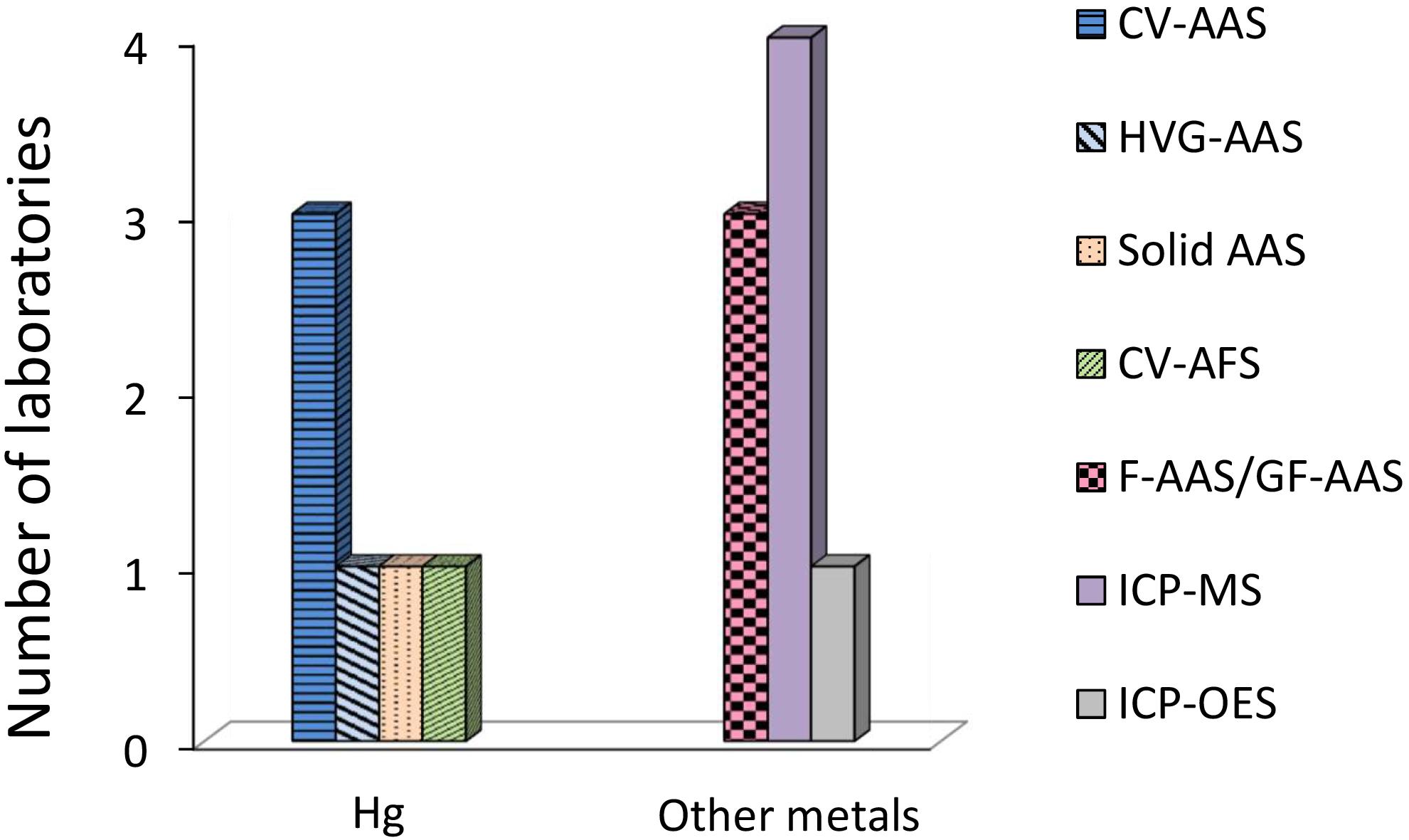
Figure 4. Analytical methods used for metal analysis in sediment by the different laboratories. CV-AAS, Cold Vapor Atomic Absorption Spectrometry; HVG-AAS, Hydride Vapor Generation Atomic Absorption Spectrometry; Solid AAS, Solid Atomic Absorption Spectrometry; CV-AFS, Cold Vapor Atomic Fluorescence Spectrometry; F-AAS, Flame Atomic Absorption Spectrometry; GF-AAS, Graphite Furnace Atomic Absorption Spectrometry; CP-MS, Inductively Coupled Plasma Mass Spectrometry; ICP-OES, Inductively Coupled Plasma Optical Emission Spectrometry.
Regarding the method performance, not all laboratories referred to LOD and LOQ of the applied methods, whereas, differences of up to two orders of magnitude were observed between the reported values (Table 3). As some laboratories purchased only LOD or LOQ, for several elements small differences were observed between minimum values of both limits.
Certified reference materials (CRM) for metals in sediment were used by five institutions, which, however, used a different CRM, such as marine sediments IAEA 158 and IAEA 458 (International Atomic Energy Agency), SRM 2702 (NIST), estuarine sediment MESS-1 (NRCC) and loam soil ERM-CC141 (EU-JRC). One of the reporting laboratories used an internal reference material. Moreover, four laboratories participated in intercalibration exercises organized by IAEA, SETOC, or UNICHIM networks.
Only four laboratories reported on accuracy and/or reproducibility. Rather good accuracy was observed among all reporting laboratories; when accuracy was expressed as % recovery, it ranged between 80 and 115%, while when expressed as% difference, the values were all under 12%. Good analytical performances were confirmed also by reproducibility data, as three of four metal-analyzing institutions reported RSD values lower than 10% for all metals. Even if not requested in the questionnaire, one laboratory reported data on uncertainty lower than 20% for most metals.
Biota Matrix
For biota, the metals considered in the questionnaire are listed in Table 1, and additional elements (manganese, selenium, and cobalt) were reported by only one laboratory. Six laboratories gave their contribution on the biota matrix. Cd, Cu, and Zn were analyzed by all participants, followed by Hg and Cr (5 laboratories), while less institutions determined other elements.
Participants were enquired to express their data as mg/kg. Four of them expressed their results on dry weight basis and two on wet weight basis. Mussel Mytilus galloprovincialis was the organism employed by all participants. Analysis was performed on whole mussel tissue by almost all institutions – only one specified that mussel muscle was used. Various institutes gave different importance to the number of analytical replicates, which ranged from 1 to 5, however, the most popular choice was 2. Regarding storage conditions, most laboratories reported keeping their samples frozen at −20°C, even if −40°C freezing and refrigeration under 5°C were also applied. Only one laboratory specified to freeze-dry the biota samples, and another to store the untreated frozen tissue.
Some commonalities were observed regarding analytical techniques used for metal determination: for Hg various atomic absorption methods were applied. Other metals were analyzed both by means of AAS (graphite furnace or flame) and ICP-MS or ICP-OES (Figure 5).
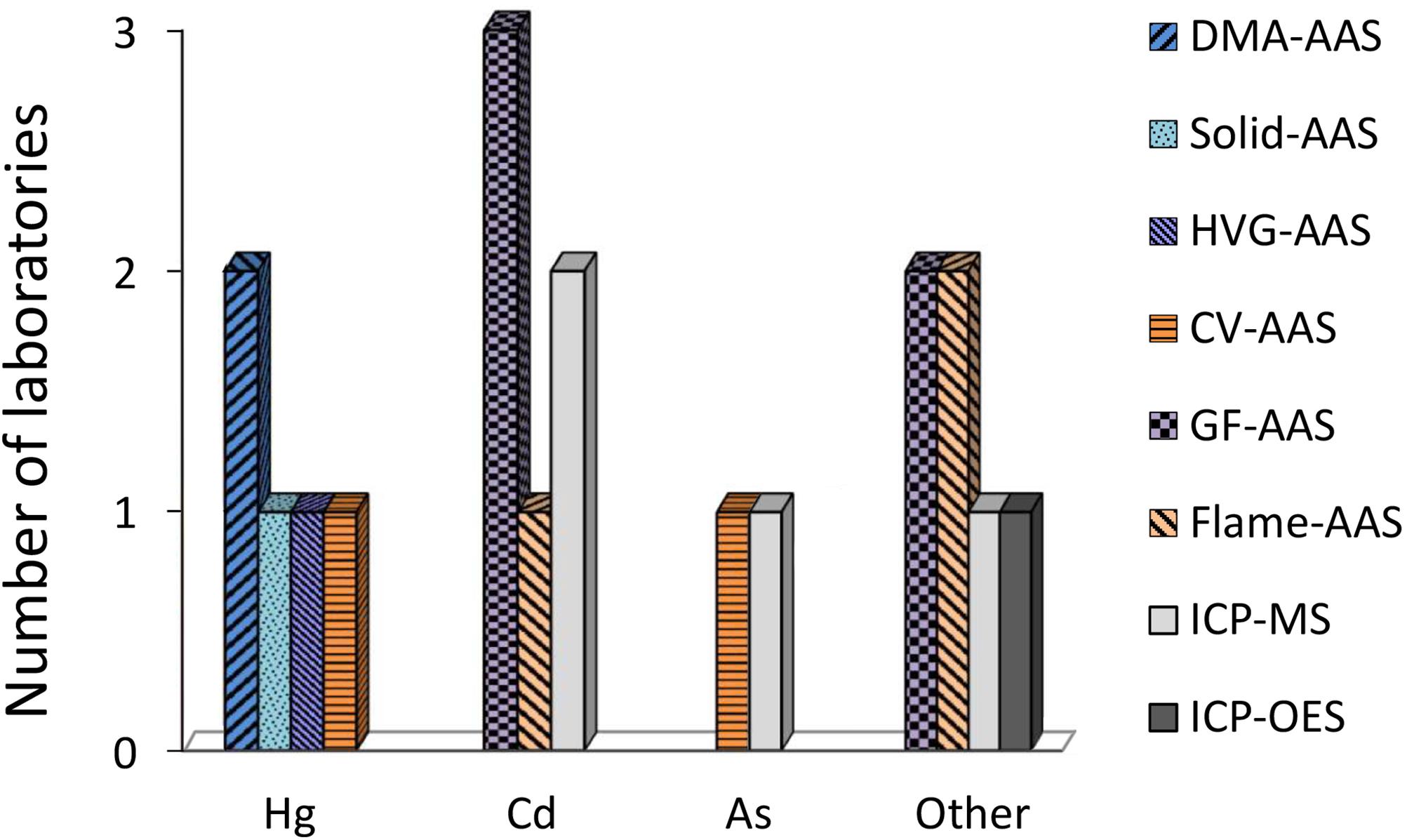
Figure 5. Analytical methods used for metal analysis in biota by different laboratories. DMA, Direct Mercury Analyzer; Solid AAS, Solid Atomic Absorption Spectrometry; HVG-AAS, Hydride Vapor Generation Atomic Absorption Spectrometry; CV-AAS, Cold Vapor Atomic Absorption Spectrometry; GF-AAS, Graphite Furnace Atomic Absorption Spectrometry; F-AAS, Flame Atomic Absorption Spectrometry; ICP-MS, Inductively Coupled Plasma Mass Spectrometry; ICP-OES, Inductively Coupled Plasma Optical Emission Spectrometry.
Only four laboratories reported values of LOD and/or LOQ. The direct comparison was not possible, as some values were expressed on dry and other on wet weight basis. Only with the scope of comparing the values, the dry/wet weight conversion factor of 20% was assumed (w.w. units = d.w. units/5). However, w.w. data were not transformed in d.w. units, as real d.w./w.w. ratios were not known. Considering this, the minimum and maximum values of LOD and LOQ are presented in Table 3. The differences among LOQ values were in the range of one order of magnitude, reflecting major homogeneity of analytical performances in biota matrix. As some laboratories purchased only LOD or LOQ, for several elements values of both limits are very similar, and in some cases LOD is apparently higher than LOQ.
Certified reference materials were employed by all laboratories analyzing biota and mussel tissue SRM 2976 (NIST) was the most used. Other CRMs used were: fish tissue IAEA 407, fish protein DORM-2 and DORM-4 (NRC-CNRC), oyster tissue SRM 1566 (NIST) and mussel tissue BCR 278 (JRC). Three participants took part in intercalibration exercises (QUASIMEME, IAEA, FAPAS). Accuracy and reproducibility data were reported by four laboratories. Good analytical performances were evidenced, as accuracy values for all metals were in the range of 92–106% (as % recovery with respect to certified values), or under 12% (as % difference between the test result and the reference value). All the reproducibility data were lower than 15%. Additional information on measurement uncertainty for Hg, Cd and Pb was reported by one of the participants (21, 20, 25%, respectively).
Discussion
The comparison between sampling, analytical and QA protocols adopted by laboratories of nine institutions from six countries of the ADRION Region revealed high level of heterogeneity, pointing out the necessity to develop common and harmonized protocols for monitoring procedures to be applied for the assessment of good environmental status, in order to fulfill the requirements of both Marine Strategy Framework Directive 2008/56/EC, 2008 and UNEP/MAP Integrated Monitoring and Assessment Programme (IMAP). In order to establish a common protocol for the evaluation of the metal contamination, several targets for harmonization have been identified, such as matrix characteristics, measurement units, sampling procedures, and sample storage, methods of analysis and LOQ values.
Seawater
Regarding seawater matrix, according to the Directive 2013/39/EU, 2013, measurement unit to be used is μg/l and concentrations of metals have to be determined in dissolved phase of water sample, achieved by filtration through 0.45 μm filter (or equivalent treatment), so the use of total sample has to be avoided. According to the ISO 5667-3 standard (2018), samples for metal analysis should be acidified to pH in the range 1–2 with concentrated nitric acid (possibly with purity grade for trace metals analysis), and stored cooled at 4°C. For Cd and Pb, SPE pre-concentration step may be applied, even if only one institution reported to apply this procedure. It has to be kept in mind that the risk of seawater contamination by metals has to be minimized. At this regard, the most critical step is sampling, as multiple sources of contamination may be present on the vessel such as wires, rust or antifouling paints. For this reason, it is recommended to use plastic tools and devices, preferentially made in Teflon or Teflon-coated (sampling bottles, submersible pumps). Internal metallic springs or silicone elastics should be avoided in favor of external closure systems. Non-metallic wires should be employed (made, for example, of Kevlar). On-board handling should be possibly carried out in a clean area, like a mobile laboratory container equipped with ultrapure water production system and laminar flow hoods. All sampling and storage containers, filtration apparatus and plastic material employed should be acid cleaned and rinsed with ultrapure water, and finally conditioned with seawater to be sampled (Capodaglio et al., 1995). Regarding analytical method, following the majority of questionnaire responses, CV-AAS was the preferential method for Hg determination in seawater samples, while Cd and Pb were analyzed by ICP-MS or voltammetry. Considering the wide range of LOQ values observed for metals in seawater, LOQ proposal was “harmonized” taking into account that the Article 4 of the Directive 2009/90/EC, 2009 requires that LOQ have to be equal or below 30% of environmental quality standard (EQS) values. The Directive 2013/39/EU, 2013 (EQSD) imposes MAC-EQS (maximum allowable concentration EQS for “other surface waters”) of 0.07 μg/l for Hg and its compounds and AA-EQS (annual average EQS) of 0.2 and 1.3 μg/l for Cd and Pb, respectively (Table 4). Taking into account LOQ values reported in questionnaires by different laboratories from ADRION Region, the proposed limits should be easily reached for Hg, while for Cd and Pb some laboratories will need to improve their methods.
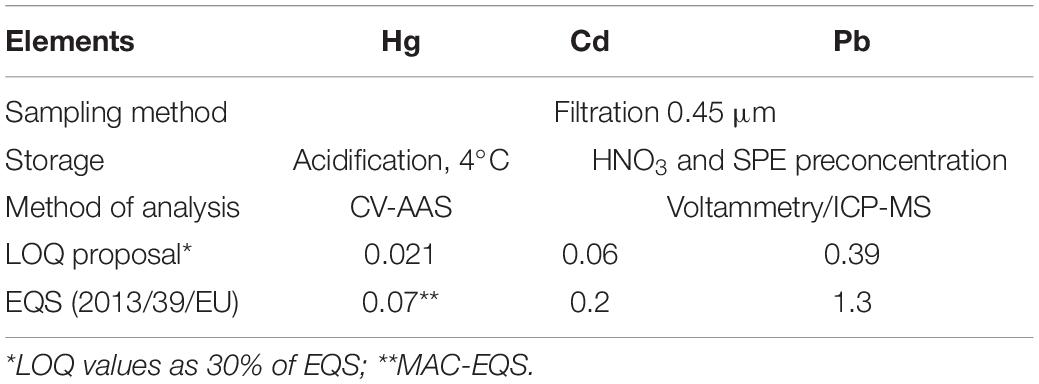
Table 4. Proposal of harmonized monitoring procedures of metals in seawater: LOQ and EQS are expressed as μg/l.
Sediment
As regards the harmonization proposal for sediment matrix, consensus was reached that data should be expressed as mg/kg d.w. In view of different sampling devices used by ADRION Region laboratories, box corer was chosen as the most appropriate sampling tool, considering that its use causes minimal disturbance to the sample regardless of the sampling conditions (weather/sea state, water depth, sediment composition, etc.) (Blomqvist, 1991). According to Guidance on chemical monitoring of sediment and biota under the WFD (European Commission, 2010), the top 5–10 cm of sediment is the most dynamic layer resulting from sedimentation and physical and biological interactions, reflecting the actual status of pollution. However, the choice of the sediment layer thickness should depend on the sediment accumulation rate of the sampling site. ADRION laboratories agreed that in coastal as well as offshore areas the top 0–2 cm sediment layer should be sampled in order to evaluate the recent temporal trends. Even thinner depths should be sampled if measured sedimentation rates are low, such as in open water environments or coastal water with low particle deposition. Considering that various grain size classes were analyzed by different laboratories, and that one of the HarmoNIA objectives was to improve the comparability of data, it was agreed that it is advisable to analyze the grain size fraction of <2 mm. It is well known that most contaminants are preferentially linked to the fine fraction (<63 μm) and to the related organic matter, however, in areas of high hydrodynamic energy, such as coastal and offshore marine areas, fine particulate matter is mixed with coarser material (European Commission, 2010), and in sandy bottoms the < 63 μm fraction would represent a negligible fraction not representative of the bulk sediments. Nonetheless, for meaningful comparisons, it is requested to obtain also information on granulometry as metadata, in order to allow normalization to the fine fraction. Following the OSPAR approach, it is recommended to analyze Al also for the normalization of the metal concentrations (Ospar Commission, 2009). Alternatively, other elements, like Fe, may be successfully employed for normalizing the heavy metal concentrations (Villares et al., 2003; Sakan et al., 2015). For the scope of harmonization, ICP-MS technique was selected for metals determination due to its multi-element character and capacity to determine very low concentrations (Thomas, 2013); in fact, the lowest LOQ values, reported among laboratories, were obtained using this technique.
Both WFD (2000/60/EC) and MSFD (2008/56/EC), as well as their amendments and implementing decrees, consider water and biota for the assessment of GES. According to the Directive 2013/39/EU, 2013, no EQS values were laid down for sediment matrix, however, Member States have the flexibility to set EQS for alternative matrices, as long as they provide at least the same level of protection, in order to take advantage of their monitoring approach. These national EQS should be established according to WFD indications of Guidance document no. 27 (European Commission, 2011, 2018 revision). According to collected information, only Italy has set a national EQS for metals in the sediment matrix (Legislative Decree, 2015). Italian EQS values have been derived on the basis of direct hazards for the benthic communities as well as for human health via seafood consumption and the ecotoxicological criterion of Threshold Effect Level (TEL) was used as reference. Chemical and toxicological data were collected along the Italian coast in the period 2001–2008 (Tornero et al., 2019). In other ADRION countries, no national standards for sediment matrix are in force, but some international references such as Med BACs, Effect Range Low (ERL) or TEL are applied for the sediment quality assessment (Table 5).
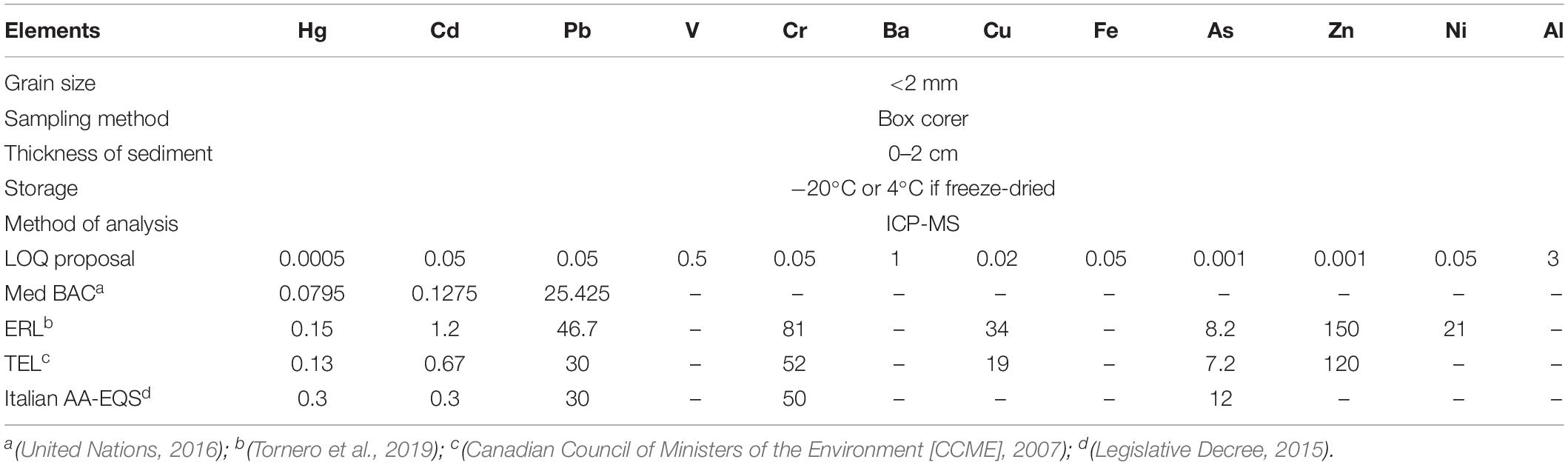
Table 5. Proposed harmonized monitoring procedures of metals in sediment. LOQ values and international reference thresholds are expressed as mg/kg d.w.
Med BACs criterion was developed on the basis of Oslo-Paris Convention for the Protection of the Marine Environment of the North-East Atlantic (OSPAR) and by criteria defined by the scientific studies in the Mediterranean Sea (United Nations, 2011 and references therein). Med BACs have been calculated for the Mediterranean Sea as a whole, using Med BCs, concentrations at a pristine or remote site (United Nations, 2016; United Nations, 2019). For the purpose of this study, Med BACs for the whole Mediterranean Sea were applied as reference, even if specific BACs for Adriatic, Aegean-Levantine, Central Mediterranean and Western Mediterranean regions have been assessed. Another threshold value, often used as reference, is ERL (or ER-Low), developed by the United States Environmental Protection Agency (US EPA), defined as the lower tenth percentile of the dataset of concentrations in sediments associated with biological effects. At concentrations lower than ERL adverse effects on organisms occur rarely, thus this threshold is, in some way, of parallel significance to WFD EQS and OSPAR EACs, representing the contaminant concentration below which no chronic effects are expected to arise even in most sensitive marine species, even if these criteria are derived in a very different way (Ospar Commission, 2009). TEL, on the other hand, is a threshold value below which the negative effects on biota are expected to arise only rarely and was developed by the Canadian Council of Ministers of the Environment. In the framework of HarmoNIA project, in order to ensure the best analytical performance and maintain a precautionary approach, LOQ values proposed for sediment matrix (Table 5) were based on the lowest values reported by laboratories in the questionnaires, considering also that values proposed would be sufficient to determine concentrations at international threshold levels. The proposed LOQ values for sediments may be challenging to reach, considering that differences of 2 orders of magnitude were observed among values reported in the questionnaires by the ADRION Region laboratories, however, until adequate EQS for the sediment matrix are developed, it seems appropriate to stimulate the development and optimization of analytical methods.
Biota
With regard to biota, it was agreed to express the concentrations of contaminants on the basis of wet weight, in order to favor the comparison with both the EQS values of Directive 2013/39/EU, 2013 and the maximum levels for metals in foodstuffs of the Commission Regulation 2006/1881/Ec (2006) and Commission Regulation 2008/629/Ec (2008). However, considering that the differences in the water content could affect the comparison of the different samples, it is always recommended to carefully measure both wet and dry weight (Guidance No. 25 - European Commission, 2010) and include this information among metadata. Since all laboratories use bivalve mollusk M. galloprovincialis for the evaluation of metal contamination in the biota matrix, it was shared that the analysis must be carried out on total samples (whole soft tissues), as indicated by the Guidance No. 25 (European Commission, 2010). However, considering that the threshold values for metals established by legislation are related to fish (EQS for Hg of Directive 2013/39/EU, 2013) or to fish and bivalve mollusks (Commission Regulation 2006/1881/Ec, 2006; Commission Regulation 2008/629/Ec, 2008), it is important to remember that the choice of the tissue to be analyzed must be relative to the purpose of the monitoring (European Commission, 2010). If the objective is to protect the ecosystem, the use of the whole fish tissue is suggested, while if the aim of monitoring is the human health protection, edible tissues should be analyzed. In this context, it has to be underlined that muscle concentrations reflect long-term accumulation processes and do not reveal current bioaccumulation nor recent temporal changes in contamination level, which on the contrary are better reflected in the liver tissue (Guidance No. 25 European Commission, 2010). According to WFD Common Implementation Strategy, for each sample 3–5 replicates should be prepared and stored at −20°C, and consensus was reached that analysis should be performed on at least 3 replicates. For each sample, biometric measurements of each individual should be registered (weight and length of shell, weight of tissues). Considering that different analytical techniques were applied by different institutions, the use of CV-AAS was proposed to harmonize Hg determination, as this technique features good sensitivity, relative freedom of interferences, simplicity of use and low cost (Krata and Bulska, 2005; Ferreira et al., 2015), while ICP-MS and ICP-OES were recommended for other metal analyses, due to their multi-elemental character coupled with good precision, accuracy and recovery (Thomas, 2013; Park et al., 2018). Hg (and its compounds) is the only metal with biota EQS imposed by the Directive 2013/39/EU, 2013 (20 μg/kg w.w. in fish tissue) thus, according to what already discussed for water matrix, the LOQ proposed for its harmonized assessment was 30% of EQS, according to the Directive 2009/90/EC, 2009 (Table 6). For other metals, the lowest values reported among questionnaires, as listed in Table 3, were proposed as LOQ in order to obtain the best analytical performance in terms of QA/QC and to maintain the precautionary approach. Considering that Hg, Cd and Pb are trace metals of common concern among Commission Regulation 2006/1881/Ec, 2006, OSPAR Convention and UNEP-MAP, it has been taken into account that the proposed LOQs are sufficient to determine these metals’ concentrations at the maximum levels in the foodstuffs and at the levels of OSPAR BACs and Med BACs (Table 6).
According to the Common Implementation Strategy of WFD, isotopic analysis of biota should be performed to determine the trophic levels if different species have to be monitored (Guidance No. 25 European Commission, 2010).
General Indications
In addition to the harmonization indications described above, some general proposals have been suggested regarding all matrices. Laboratories involved in the comparison from all around the ADRION Region were invited to make an effort to attempt the analysis of all the substances included in the questionnaires, with the aim of allowing the evaluation of the relative contamination in the whole area. Although the use of certified reference materials was quite common, especially in sediment and biota, all participants were invited to use adequate reference materials, similar to the sample matrix, in order to be able to verify analytical performances, especially considering the scarcity of information related to essential characteristics of method validation, such as accuracy and reproducibility. In this regard, it is important to remember, that according to the Association of Official Analytical Chemists (AOAC) recommendations, for concentrations from 0.1 to 10 mg/kg the mean recovery is expected in the range of 80–110%, with relative standard deviation (RSD%) of 15–7.3%, while for concentrations from 10 to 100 mg/kg the mean recovery should remain in the narrower range of 90–107%, with RSD of 7.3–5.3% (Aoac, 2016). In order to ensure that assessment of contaminants is coherent in the region, it was also suggested that all involved laboratories take part in the intercalibration tests or proficiency tests.
Conclusion
A general lack of homogeneity emerged from the investigation on the sampling and preservation protocols and on the analytical methods used by various laboratories responsible for research and monitoring of heavy metals in different environmental compartments in the ADRION region, also due to the lack of completeness of some information. In particular, differences were related to the sampling characteristics (filtration for seawater, sample thickness and grain size for sediments, tissue and weight basis for biota), to the storage procedures (preservative addition, cooling, freezing, freeze-drying), to the analytical technique and to the achievable LOQ values. In order to enable the coherent assessment of the state of the environment and of the impacts on the coastal and off-shore areas of the Adriatic and Ionian Seas, a harmonized methodological proposal was developed and shared based on the requirements of the EU legislation and taking into account the decisions adopted by Barcelona Convention (UNEP-MAP) and OSPAR. Although appropriate environmental quality standards for biota and sediment matrices should be established at national level through regional and sub-regional cooperation, as required by the WFD and MSFD, the proposed LOQ values, even if challenging, represent a benchmark and a stimulus to optimize analytical performance, to ensure the best level of protection of the coastal and offshore environment in the ADRION Region.
Data Availability Statement
The raw data supporting the conclusions of this article will be made available by the authors, without undue reservation, to any qualified researcher.
Author Contributions
GG, DB, and ML directed this part of the project. DB, GG, ML, and FR conceived the form of this study. DB, CG, FR, and MF gathered and confronted the data. MG contributed to the discussion of the results. MF, DB, CG, FR, GG, BT, and ML wrote the manuscript and designed figures and tables with input and revisions from all authors. All authors contributed to the article and approved the submitted version.
Funding
This work was financed by the Adriatic-Ionian Program INTERREG V-B Transnational 2014-2020, 2018-2020 within the Interreg Adrion project HarmoNIA (Harmonization and Networking for contaminant assessment in the Ionian and Adriatic Seas) granted to ML and BT. The content of the publication is the sole responsibility of beneficiaries of the project and can under no circumstances be regarded as reflecting the position of the European Union and/or ADRION programme authorities.
Conflict of Interest
The authors declare that the research was conducted in the absence of any commercial or financial relationships that could be construed as a potential conflict of interest.
Supplementary Material
The Supplementary Material for this article can be found online at: https://www.frontiersin.org/articles/10.3389/fmars.2020.00717/full#supplementary-material
References
Acquavita, A., Covelli, S., Emili, A., Berto, D., Faganeli, J., Giani, M., et al. (2012). Mercury in the sediments of the marano and grado lagoon (northern Adriatic Sea): sources, distribution and speciation. Estuar. Coast Shelf Sci. 113, 20–31. doi: 10.1016/j.ecss.2012.02.012
Aoac (2016). Appendix F: Guidelines for Standard Method Performance Requirements, 20th Ed. of the Official Methods of Analysis of AOAC International. Washington, D.C: AOAC.
Blomqvist, S. (1991). Quantitative sampling of soft-bottom sediments: problems and solutions. Mar. Ecol. Prog. Ser. 72, 295–304. doi: 10.3354/meps072295
Capodaglio, G., Scarponi, G., Toscano, G., Barbante, C., and Cescon, P. (1995). Speciation of trace metals in seawater by anodic stripping voltammetry: critical analytical steps. Fresenius J. Anal. Chem. 351, 386–392. doi: 10.1007/BF00322907
Carić, H., and Mackelworth, P. (2014). Cruise tourism environmental impacts - The perspective from the Adriatic Sea. Ocean Coast. Manag. 102, 350–363. doi: 10.1016/j.ocecoaman.2014.09.008
Canadian Council of Ministers of the Environment [CCME] (2007). “Protocol for the derivation and use of sediment quality guidelines for the protection of aquatic life,” in Report prepared by the Task Force on Water Quality Guidelines of the Canadian Council of Ministers of the Environment Water Quality Branch, Environment Canada, Ottawa.
Commission Decision 2017/848/Eu (2017). 2017/848/EU Laying Down Criteria and Methodological Standards on Good Environmental Status of Marine Waters and Specifications and Standardised Methods for Monitoring and Assessment, and Repealing Decision 2010/477/EU. EU: EEA.
Commission Regulation 2006/1881/Ec (2006). (EC) No 1881/2006 Setting Maximum Levels for Certain Contaminants in Foodstuffs. EU: EEA.
Commission Regulation 2008/629/Ec (2008). (EC) No 629/2008 Amending Regulation (EC) No 1881/2006 Setting Maximum Levels for Certain Contaminants in Foodstuffs. EU: EEA.
Cozzi, S., Reisenhofer, E., Di Monte, L., Cantoni, C., and Adami, G. (2008). Effect of environmental forcing on the fate of nutrients, dissolved organic matter and heavy metals released by a coastal wastewater pipeline. Chem. Ecol. 24, 87–107. doi: 10.1080/02757540801919354
Cukrov, N., Frančišković-Bilinski, S., Hlača, B., and Barišić, D. (2011). A recent history of metal accumulation in the sediments of Rijeka harbor, Adriatic Sea, Croatia. Mar. Pollut. Bull. 62, 154–167. doi: 10.1016/j.marpolbul.2010.08.020
Di Leo, A., Annicchiarico, C., Cardellicchio, N., Spada, L., and Giandomenico, S. (2013). Trace metal distributions in Posidonia oceanica and sediments from Taranto Gulf (Ionian Sea. Southern Italy). Mediterranean Mar. Sci. 14, 204–213. doi: 10.12681/mms.316
Commission Directive 2009/90/Ec (2009). Laying Down, Pursuant to Directive 2000/60/EC of the European Parliament and of the Council, Technical Specifications for Chemical Analysis and Monitoring of Water Status. Brussels: EU.
Directive 2000/60/Ec (2000). Directive 2000/60/EC of the European Parliament and of the Council establishing a framework for Community action in the field of water policy. EU: EEA.
Directive 2008/56/Ec (2008). Directive 2008/56/EC of the European Parliament and of the Council establishing a framework for community action in the field of marine environmental policy (Marine Strategy Framework Directive). Brussels: EU.
Directive 2008/105/Ec, (2008). Directive 2008/105/EC of the European Parliament and of the Council on environmental quality standards in the field of water policy, amending and subsequently repealing Council Directives 82/176/EEC, 83/513/EEC, 84/156/EEC, 84/491/EEC, 86/280/EEC and amending Directive 2000/60/EC of the European Parliament and of the Council. Brussels: EU.
Directive 2013/39/Eu (2013). Directive 2013/39/EU of the European Parliament and of the Council amending Directives 2000/60/EC and 2008/105/EC as regards priority substances in the field of water policy. Brussels: EU.
European Commission (2010). Common Implementation Strategy for the Water Framework Directive (2000/60/EC). Guidance Document No. 25. Guidance on chemical monitoring of sediment and biota under the Water Framework Directive. Brussels: EU.
European Commission (2011). Common Implementation Strategy for the Water Framework Directive-Guidance Document No. 27-Technical Guidance for Deriving Environmental Quality Standards; European Commission Technical Report; Brussels: EU.
European Commission (2018). Common Implementation Strategy for the Water Framework Directive (2000/60/EC). Guidance Document No: 27, Updated Version 2018, Document endorsed by EU Water Directors at their meeting in Sofia on 11-12 June 2018. Technical Guidance for Deriving Environmental Quality Standards.
Ferreira, S. L. C., Lemos, V. A., Silva, L. O. B., Queiroz, A. F. S., Souza, A. S., da Silva, E. G. P., et al. (2015). Analytical strategies of sample preparation for the determination of mercury in food matrices — a review. Microchem. J. 121, 227–236. doi: 10.1016/j.microc.2015.02.012
Gallmetzer, I., Haselmair, A., Tomašovych, A., Stachowitsch, M., and Zuschin, M. (2017). Responses of molluscan communities to centuries of human impact in the northern Adriatic Sea. PLoS One 12:e0180820. doi: 10.1371/journal.pone.0180820
Horvat, M., Degenek, N., Lipej, L., Snoj, Tratnik, J., and Faganeli, J. (2014). Trophic transfer and accumulation of mercury in ray species in coastal waters affected by historic mercury mining (Gulf of Trieste, northern Adriatic Sea). Environ. Sci. Pollut. Res. 21, 4163–4176. doi: 10.1007/s11356-013-2262-0
Igwe, C. O., Saadi, A. A. L., and Ngene, S. E. (2013). Optimal options for treatment of produced water in offshore petroleum platforms. J. Pollut. Eff. Cont. 1:102. doi: 10.4172/2375-4397.1000102
IMAP (2016). Integrated Monitoring and Assessment Programme of the Mediterranean Sea and Coast and Related Assessment Criteria, UNEP/MAP. Athens: United Nations.
Interreg V-B Adriatic-Ionian (ADRION) (2018–2020). Cooperation Programme Avaliable online at: https://www.adrioninterreg.eu/
Joksimovic, D., Castelli, A., Pestorić, B., and Perosevic, A. (2019) An assessment of trace metal contamination in surface sediments of the Montenegrin coast by using pollution indexes and statistical analysis. Fresenius Environ. Bull. 28, 879–884.
Joksimovic, D., Perošević, A., Castelli, A., Pestorić, B., Šuković, D., and Ðurović, D. (2020). Assessment of heavy metal pollution in surface sediments of the Montenegrin coast: a 10-year review. J. Soils Sediments 20, 2598–2607. doi: 10.1007/s11368-019-02480-7
Kljaković-Gašpić, Z., Odžak, N., Ujević, I., Zvonarić, T., Horvat, M., and Barić, A. (2006). Biomonitoring of mercury in polluted coastal area using transplanted mussels. Sci. Total Environ. 368, 199–209. doi: 10.1016/j.scitotenv.2005.09.080
Krata, A., and Bulska, E. (2005). Critical evaluation of analytical performance of atomic absorption spectrometry and inductively coupled plasma mass spectrometry for mercury determination. Spectrochim. Acta B 60, 345–350. doi: 10.1016/j.sab.2004.11.011
Legislative Decree of 13 October 2015, no. 172. Implementation of Directive 2013/39/EU amending Directive 2000/60/EC as regards priority substances in the field of water policy. (15G00186) (GU General Series No. 250 of 27-10-2015)]. Rome: Ministry of Economy and Finance.
Mikac, N., Foucher, D., Kwokal, Ž, and Barišić, D. (2006). Mercury and radionuclides in sediments of the Kaštela bay (Croatia) – evaluation of the sediment pollution history. Croat. Chem. Acta 79, 85–93.
Park, Y. M., Choi, J. Y., Nho, E. Y., Lee, C. M., Hwang, I. M., Khan, N., et al. (2018). Determination of macro and trace elements in canned marine products by inductively coupled plasma—optical emission spectrometry (ICP-OES) and ICP—mass spectrometry (ICP-MS). Anal. Lett. 52, 1018–1030. doi: 10.1080/00032719.2018.1510938
Rogan Šmuc, N., Dolenec, M., Kramar, S., and Mladenović, A. (2018). Heavy metal signature and environmental assessment of nearshore sediments: port of koper (Northern Adriatic Sea). Geosciences 8:398. doi: 10.3390/geosciences8110398
Sakan, S., Dević, G., Relić, D., Anelković, I., Sakan, N., and Ðorević, D. (2015). Evaluation of sediment contamination with heavy metals: the importance of determining appropriate background content and suitable element for normalization. Environ. Geochem. Health 37, 97–113. doi: 10.1007/s10653-015-9766-0
Thomas, R. (2013). Practical guide to ICP-MS: a tutorial for beginners. 3rd edition. Boca Raton, FL: CRC Press.
Tornero, V., and Hanke, G. the Msfd Expert Network on Contaminants. (2019). Marine chemical contaminants – support to the harmonization of MSFD D8 methodological standards: Matrices and threshold values/reference levels for relevant substances. Luxembourg: Publications Office of the European Union.
Traven, L., Furlan, N., and Cenov, A. (2015). Historical trends (1998–2012) of nickel (Ni), copper (Cu) and chromium (Cr) concentrations in marine sediments at four locations in the Northern Adriatic Sea. Mar. Pollut. Bull. 98, 289–294. doi: 10.1016/j.marpolbul.2015.07.001
United Nations (2016). UNEP(DEPI)/MED WG.427/7. Agenda item 5. Review of Proposed Background Concentrations (BC)/Background Assessment Criteria (BACs)/Environmental Assessment Criteria (EACs) for Contaminants and Biomarkers at Mediterranean and Regional Scales. Proposal of Assessment Criteria for Hazardous Substances and Biological Markers in the Mediterranean Sea Basin and its Regional Scales. Marseille:UNEP.
Vasileiadou, K., Pavloudi, C., Kalantzi, I., Apostolaki, E., Chatzigeorgiou, G., Chatzinikolaou, E., et al. (2016). Environmental variability and heavy metal concentrations from five lagoons in the Ionian Sea (Amvrakikos Gulf. W Greece). Biodivers. Data J. 4, e8233. doi: 10.3897/BDJ.4.e8233
Keywords: hazardous substances, harmonized protocols, integrated approach, monitoring, assessment of contaminants, heavy metals, Adriatic-Ionian Sea region
Citation: Berto D, Formalewicz M, Giorgi G, Rampazzo F, Gion C, Trabucco B, Giani M, Lipizer M, Matijevic S, Kaberi H, Zeri C, Bajt O, Mikac N, Joksimovic D, Aravantinou AF, Poje M, Cara M and Manfra L (2020) Challenges in Harmonized Assessment of Heavy Metals in the Adriatic and Ionian Seas. Front. Mar. Sci. 7:717. doi: 10.3389/fmars.2020.00717
Received: 08 June 2020; Accepted: 05 August 2020;
Published: 04 September 2020.
Edited by:
Senthil Kumar Ponnusamy, SSN College of Engineering, IndiaReviewed by:
Paolo Cocci, University of Camerino, ItalyThadickal V. Joydas, King Fahd University of Petroleum and Minerals, Saudi Arabia
Copyright © 2020 Berto, Formalewicz, Giorgi, Rampazzo, Gion, Trabucco, Giani, Lipizer, Matijevic, Kaberi, Zeri, Bajt, Mikac, Joksimovic, Aravantinou, Poje, Cara and Manfra. This is an open-access article distributed under the terms of the Creative Commons Attribution License (CC BY). The use, distribution or reproduction in other forums is permitted, provided the original author(s) and the copyright owner(s) are credited and that the original publication in this journal is cited, in accordance with accepted academic practice. No use, distribution or reproduction is permitted which does not comply with these terms.
*Correspondence: Daniela Berto, ZGFuaWVsYS5iZXJ0b0Bpc3ByYW1iaWVudGUuaXQ=