Age and Growth of Striped Venus Clam Chamelea gallina (Linnaeus, 1758) in the Mid-Western Adriatic Sea: A Comparison of Three Laboratory Techniques
- 1Department of Biological, Geological and Environmental Sciences, University of Bologna, Bologna, Italy
- 2Institute for Biological Resources and Marine Biotechnologies, National Research Council, Ancona, Italy
Age and growth studies provide critical data for clam fishery management. Three aging techniques, thin sections and acetate peel replicas – which involve shell sectioning – and surface growth rings were used to estimate the age and growth of Chamelea gallina populations in the mid-western Adriatic Sea. Their results were compared to identify the most reliable and least time-consuming approach. There were no significant differences between the two shell sectioning techniques (χ2 = 4.66, df = 3, p = 0.198), which were described by the same von Bertalanffy (VBF) growth curve parameters (L8 = 43.9, k = 0.26, t0 = −0.84), whereas significantly different L8 and k values were found between the two shell sectioning techniques and surface growth rings (L8: χ2 = 13.62, df = 1, p < 0.001; k: χ2 = 9.18, df = 1, p < 0.002; these statistics refer to the comparison between acetate peels and surface growth rings). The latter approach proved unreliable and error-prone, as it underestimated age and overestimated the growth rate (L8 = 26.4, k = 1.91, t0 = −0.11). Although the thin sections and acetate peel techniques both provide reliable age and growth estimates, the former approach was less time-consuming. Our analyses demonstrated that shell growth is slower in the cold season and in older specimens and that it has slowed down over the past few decades.
Introduction
Chamelea gallina (Linnaeus, 1758) is an infaunal filter-feeder clam of the Veneridae family (Bivalvia: Lamellibranchiata: Veneridae) that inhabits the fine well-sorted sand biocenosis described by Péres and Picard (1964). It is widespread in the Mediterranean and Black Seas and along the eastern Atlantic coast. The striped venus clam tolerates limited salinity and temperature variations and requires sandy and muddy–sandy sediments (Moschino and Marin, 2006). In Italy it inhabits a narrow coastal strip at depths ranging from 0 to 12 m up to 1–2 nautical miles (nm) off the coast (Morello et al., 2006). It is particularly abundant in the central and northern Adriatic Sea, where the massive Po River outflow and the currents flowing along the Italian coast provide abundant nutrients, particles and organic matter (Orban et al., 2007).
Chamelea gallina is a major edible bivalve species throughout the Mediterranean, especially in Italy, Spain, Turkey, and Morocco. In Italy, the fishery consists of 636 hydraulic dredgers employing ca. 1500 workers and totaling annual landings of about 10,000 metric tons and revenues approaching €12 million (DGPEMAC, 2019). C. gallina is managed by detailed national and international regulations. In Italian territorial waters its Minimum Conservation Reference Size (MCRS) has been reduced from 25 mm total length (TL) [Council Regulation (CE) No. 1967/2006, 2006 of the European Community (EC)] to 22 mm TL [Delegated Regulation (UE) No. 2016/2376, 2016 of the European Union (EU) and Commission Delegated Regulation (EU) 2020/3, 2020].
Studies of bivalve population dynamics require a thorough knowledge of their growth rate and age and are essential to develop effective fishery management measures (Mancuso et al., 2019). Age and growth have extensively been investigated for bivalve mollusks in different geographical areas with a variety of methods, including mark and recapture (Jones et al., 1978; Ropes et al., 1984; Adjei-Boateng and Wilson, 2013), size-frequency distribution analysis (Froglia, 2000; Herrmann et al., 2009), shell surface growth rings (Fiori and Morsán, 2004; Adjei-Boateng and Wilson, 2013), thin sections (Christian et al., 2000; Dalgiç et al., 2010), acetate peel replicas (Ramón and Richardson, 1992; Gibson et al., 2001; Gaspar et al., 2004; Masu et al., 2008) and isotope analysis (Keller et al., 2002; Mancuso et al., 2019).
Most of these methods involve some disadvantages. Mark and recapture experiments require lengthy procedures to obtain the data (especially in slow-growing species) and involve marking a large number of specimens. Size-frequency distribution analysis is suitable only for fairly young clams since the slower growth of older specimens makes the statistical modes undistinguishable; in addition, it is unsuitable for species with a relatively long annual recruitment period and/or highly variable growth within age classes (Gaspar et al., 2004). In C. gallina gonad development and gamete emission are closely related to water temperature, as reported for various venerids living in temperate areas (Tirado and Salas, 1998; Rizzo et al., 2011). Polenta (1993) also described a broad growth variability for this species. In the Adriatic Sea, its spawning season, which peaks in late spring-early summer, spans approximately early spring to early autumn (Cordisco et al., 2003; Rizzo et al., 2010; Scopa et al., 2014). The shell surface growth ring approach often yields conflicting results, due to the difficulty of distinguishing true annual rings from false ones, which are generally caused by disturbances such as changes in environmental parameters (e.g., salinity, oxygen concentration, temperature, food availability, pollution, predation), endogenous factors (e.g., reproduction, disease) and even dredger-related stress (Carlucci et al., 2015). In addition, in older specimens the most recent rings are deposited close to one another and near the ventral margin, hampering their distinction, especially if the margin is eroded.
Techniques that overcome these problems, such as acetate peel replicas, thin sections and isotope analyses, have been developed to assess the age and growth of C. gallina as well as for several other bivalve species (e.g., Jones et al., 1990; Moura et al., 2009; Versteegh et al., 2010; Ezgeta-Balić et al., 2011; Hernández-Otero et al., 2014), but they are more expensive and time-consuming.
Given the sedentary habits of bivalves and the influence exerted by environmental and endogenous conditions on growth in different geographical areas (Gaspar et al., 2004), the assessment of age population structure for management purposes must be performed on the local scale. This study examines the age structure and growth rate of C. gallina populations harvested in the mid-western Adriatic Sea by three techniques – thin sections, acetate peel replicas and shell surface growth rings – to identify the most accurate and least time-consuming method. The results are discussed in the light of current regulations and in particular compared with those obtained by similar studies conducted in the same area.
Materials and Methods
Sampling
Chamelea gallina individuals were obtained from November 2018 to October 2019 from commercial hydraulic dredges conducting normal fishing operations in the Ancona and San Benedetto del Tronto Maritime Districts (mid-western Adriatic Sea; Figure 1). The dredges exploited fishing grounds characterized by sandy sediment located more than 0.3 NM from the coast at depths ranging from 5 to 12 m. An unsieved sample of about 30 specimens comprising all the available size classes, including individuals under the MCRS, was obtained monthly from the dredge collecting box. Only clams with undamaged valves were studied. For each specimen, the TL (maximum distance along the anterior-posterior axis) and height (H; maximum distance along the dorsoventral axis) of the shell were measured to the nearest 0.1 mm using a digital vernier caliper. The valves were then opened with a cutter to remove the flesh, air-dried and numbered for further processing.
Thin Sections
The right valves of 353 specimens were placed in a silicon mold and embedded in polyester resin as described by Rhoads and Lutz (1980). Once hardened, the blocks were cut radially into 1-mm-thick sections, from the umbo to the ventral margin along the axis of maximum growth, using a high-speed saw equipped with a diamond blade. The surfaces of each section were ground flat using successive finger grits (800, 1000, 1200 μm) and wet-polished with a polishing compound to obtain the required texture and thickness. In each thin section, the number of annual growth rings was determined by counting the alternating opaque (carbonate matrix) and translucent (carbonate-organic matrix) increments visible on the shell cross-section (Arneri et al., 1995) using a dissecting microscope under reflected light at low magnification (6.4 X). Assuming that the growth rings are laid down yearly, the age of each clam was estimated by counting all the translucent zones after marking them with a black marker pen along the shell section. The distance between the umbo and the ventral margin of each growth ring was measured with a vernier caliper. For edge analysis, the distance between the last annual growth ring and the ventral margin of the shell was measured using a video analysis system connected to the dissecting microscope, to determine how the shell margin extent (opaque zone) varied over the months.
Acetate Peels
The other halves of the resin blocks, which were processed to obtain the thin sections, were used to prepare the acetate peel replicas. For this time-consuming analysis we only prepared a subsample, which yielded a total number of 118 readable slides, encompassing all the available size classes but not all the months. After grounding flat and polishing their surfaces as described above, the half blocks were immersed for 2 min in 1% HCl solution, which dissolves the carbonate parts but preserves the organic part of the matrix, resulting in a relief on the sectioned shell surface. Acetate peel replicas were prepared as described by Rhoads and Pannella (1970) and Richardson et al. (1979). The pattern emerging after treatment in HCl solution was transferred to a 0.1 mm thick sheet of cellulose acetate, previously immersed in an ethyl-acetate solution and then laid on the shell surface block. Once dried, the acetate peels were placed on a microscope slide and photographed under a light microscope connected to a video analysis system, to identify the annual growth rings. Each annual ring was marked directly on the peel using a black marker pen. The distance between the umbo and each ring was measured with a digital vernier caliper.
Surface Growth Rings
All the left valves of the 353 specimens were examined for the annual growth rings, which appeared as smooth clefts on the shell surface and as strong pigmented lines across the anterior-posterior axis. The distance between the umbo and each ring was measured using a digital vernier caliper along the dorsoventral axis.
Data Analysis
Since the measurements taken with all three techniques were relative to shell height (H), the height-at-age data were converted to shell TL using an equation resulting from the height-length relationship, as follows:
The mean length-at-age was calculated and compared among techniques. The annual increments were calculated by subtracting the mean TL of the younger age class from the mean TL of the older class immediately above it.
The age of each individual was calculated at half a year, based on the capture date and on the assumption that the translucent bands form in winter, considering as conventional birthday the 1st of July. Accordingly, half a year was added to all the specimens collected from 1st January to 30th June and to all those aged 0+. The age readings were performed by the same reader 2 weeks apart. Their accuracy was estimated by calculating and comparing the average percent error (APE) and average coefficient of variation (ACV) of each technique.
To describe the growth patterns, the following von Bertalanffy Growth Function (VBGF) was fitted to the length-at-age data:
where E[L]t is the mean length-at-age t and L8 (theoretical maximum length), k (growth coefficient) and t0 (theoretical age at length zero) are the parameters to be estimated.
The LogLikelihood ratio test was applied to increasingly less complex nested models by setting and varying the parameters of the two VBGFs (Ogle, 2016), to compare the VBGF parameters of pairs of techniques. The first two models to be tested were the ones which showed different and equal values of the von Bertalanffy (VBF) growth curve parameters (L8, k, t0). Bias plots were built according to Campana et al. (1995) and Muir et al. (2008) to compare the age estimates obtained by the two shell sectioning methods. All analyses were performed using the free software R (R Core Team, 2013) and the FSA package (Ogle et al., 2020).
Results
Thin Sections and Acetate Peels
The specimens analyzed using the thin sections technique ranged in size from 12.4 to 37.7 mm. The annual growth rings, consisting of wide opaque bands (light zones) laid down in summer (fast growth period) and of narrow translucent bands (dark zones) laid down in winter (slow growth period) were easily detected (Figure 2A). Since the bands are deposited parallel to the ventral margin of the shell, they could sometimes be seen also in the region of the umbo (Figure 2B), which helped the counts when the sections were not clearly readable. The method provided age estimates ranging from 0.5 to 6.5 years (Figure 3A). Indices of age precision within readings performed by the same reader were very low (APE = 1.22%; ACV = 4.34%), reflecting good method consistency and reproducibility. The mean length-at-age and the standard error (SE) are reported in Table 1. In particular, 1-year-old specimens reached 14.57 ± 0.11 mm TL and 2-year-old specimens 21.0 ± 0.13 mm TL. The older individuals showed increasingly narrow annual growth rings (Table 2). Moreover, edge analysis showed that the largest margin increment was laid down in summer and the smallest was deposited in autumn-winter (Figure 4). This was confirmed by the margin extent of a specimen caught in June 2018, which measured 2.4 mm (Figure 2A).
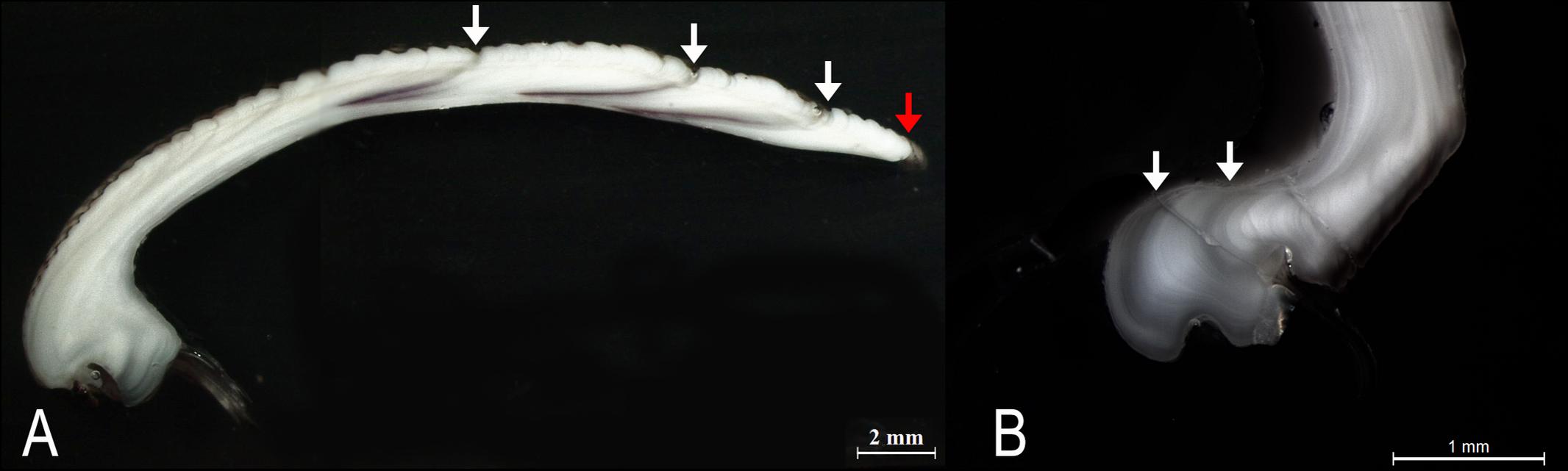
Figure 2. (A) Thin section of a C. gallina shell from a specimen harvested in June 2018. White arrows: annual growth rings; red arrow: marginal increment. (B) The annual growth rings were occasionally also observed in the umbo region (white arrows).
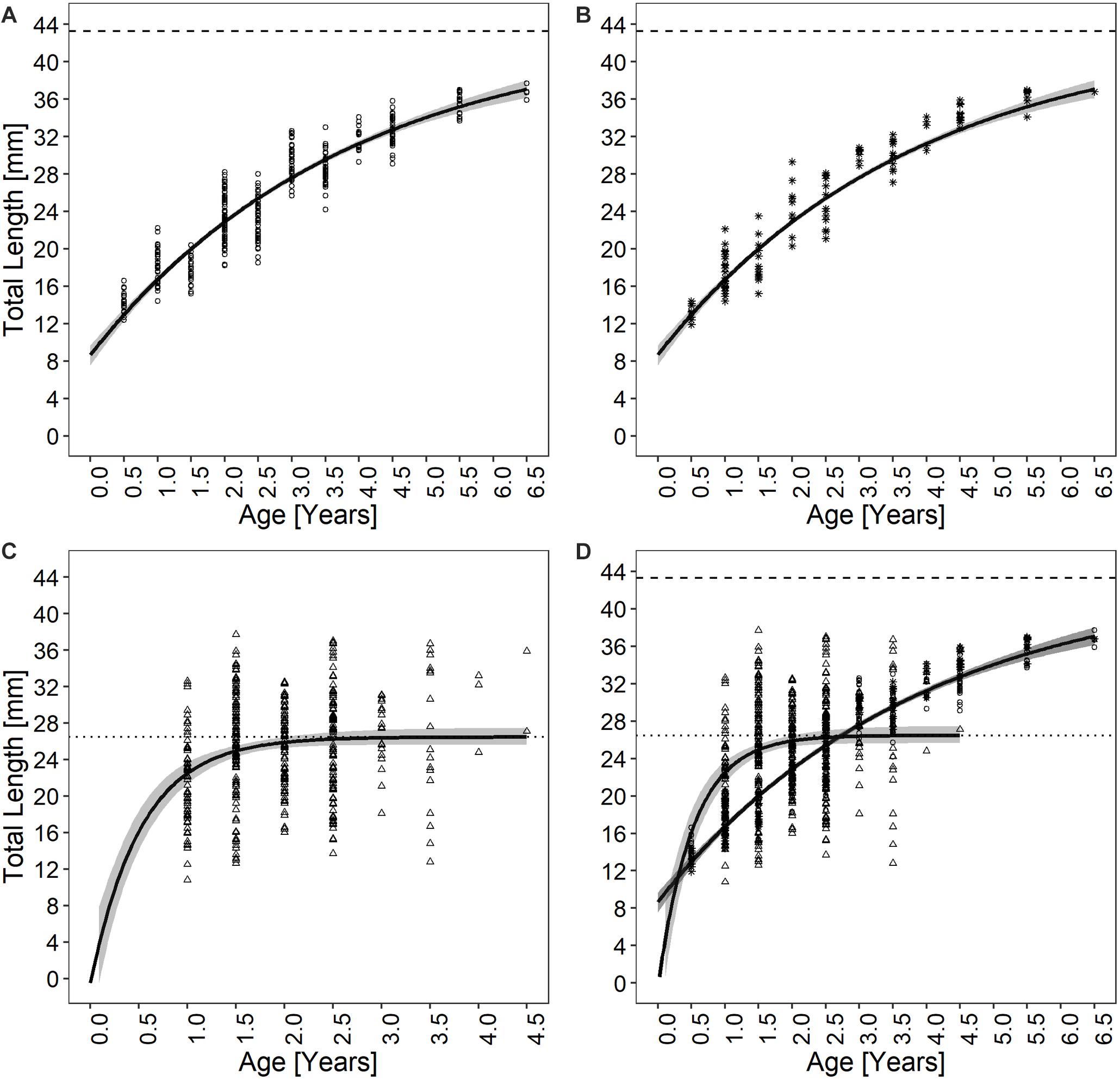
Figure 3. Chamelea gallina growth curves determined using (A) thin sections, (B) acetate peel replicas, (C) surface growth rings, and (D) by fitting all the von Bertalanffy (VBF) growth curves together. The shaded areas around the growth curves are the 95% CIs. Different dot types represent the observed individuals, being respectively 353 for both thin sections and surface growth rings techniques and 118 for the acetate peels.
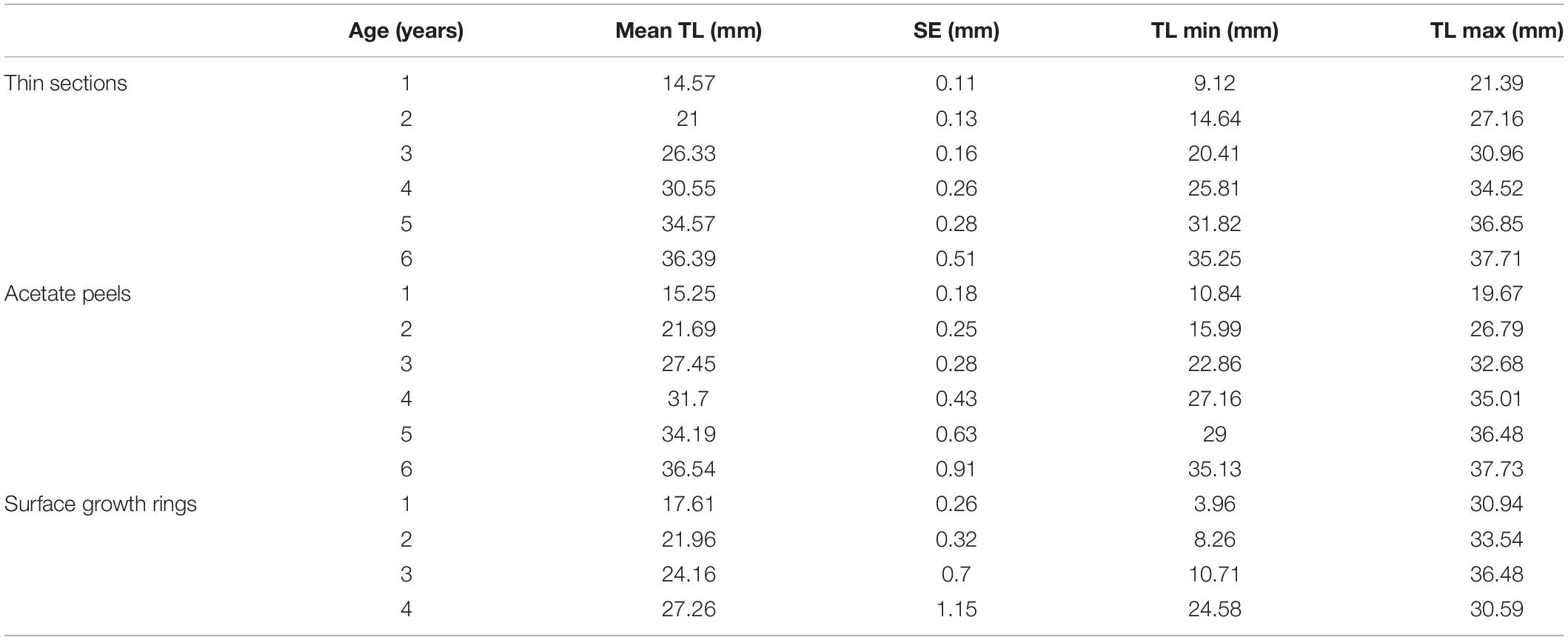
Table 1. Maximum, minimum and mean length-at-age and respective SE of C. gallina specimens from the mid-western Adriatic Sea calculated with the three aging techniques.
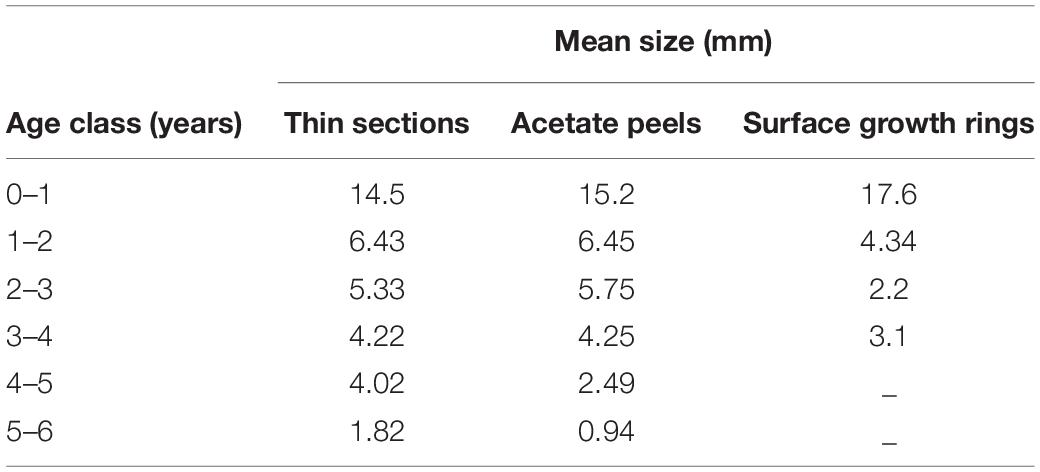
Table 2. Mean annual growth rate calculated with the three aging techniques in the different age classes.
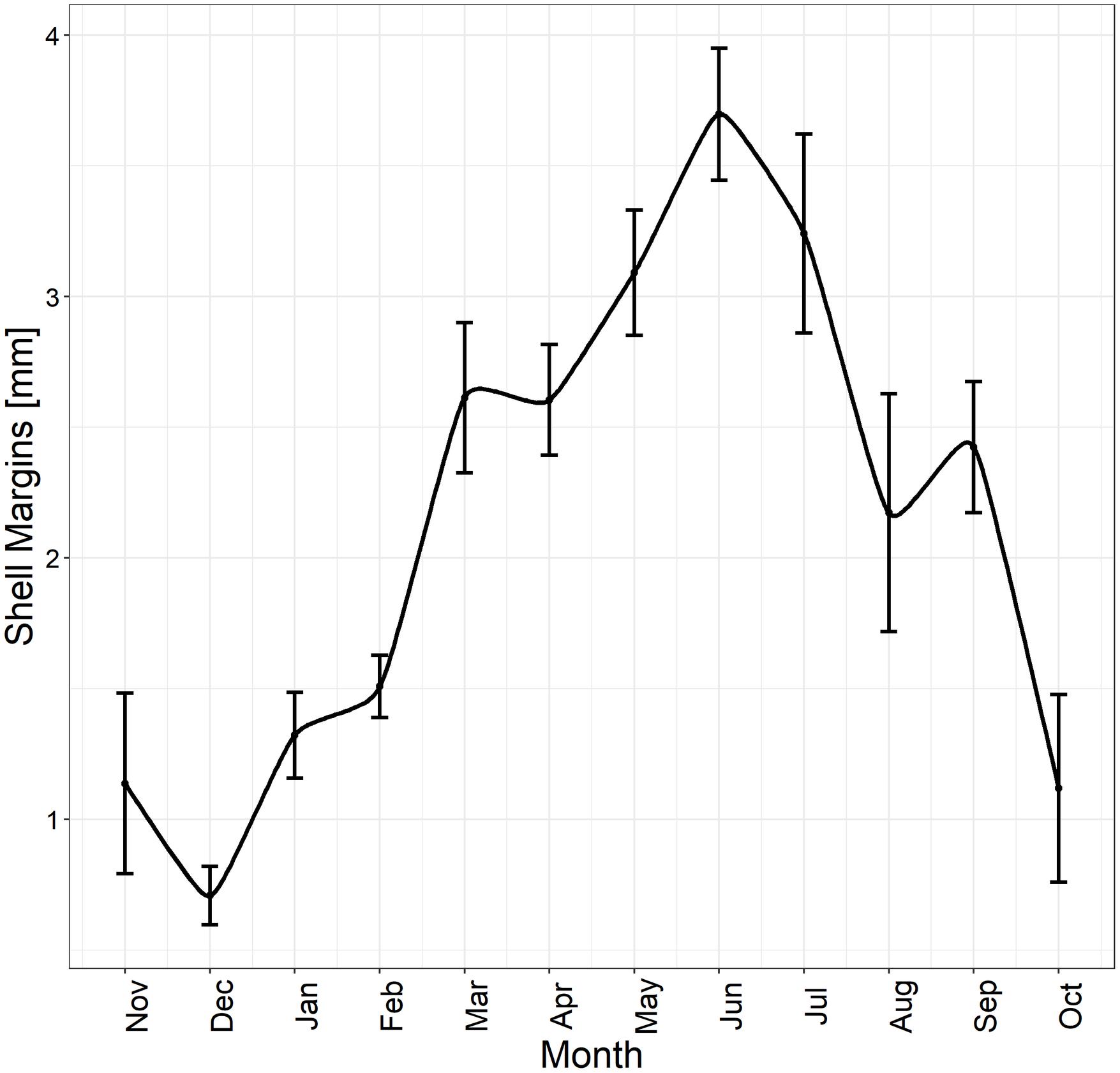
Figure 4. Edge analysis. Different mean shell margin extents across months and associated SE (black bars) of 353 specimens analyzed throughout the year, highlighting the maximum margin extent occurring during the summer season.
The acetate peel replica technique allowed identifying seasonal growth bands deposited parallel to the ventral margin on the outer (prismatic) shell layer. Annual growth rings were identified as alternating clusters of wider and narrower bands. Under the light microscope the growth bands were visible as more (Figure 5A) or less distinct (Figure 5B) dark lines. The annual growth rings were formed by clusters of wide and narrow bands, which often ended with a cleft (Figure 5C,D). Occasionally, a ring showed two clefts (Figure 5E). This regular pattern allowed distinguishing real rings from false ones, which though characterized by a cleft, appeared as a sudden interruption of the regular pattern and did not show a regular narrowing of the band, but a mixture of different growth increments (Figure 5F).
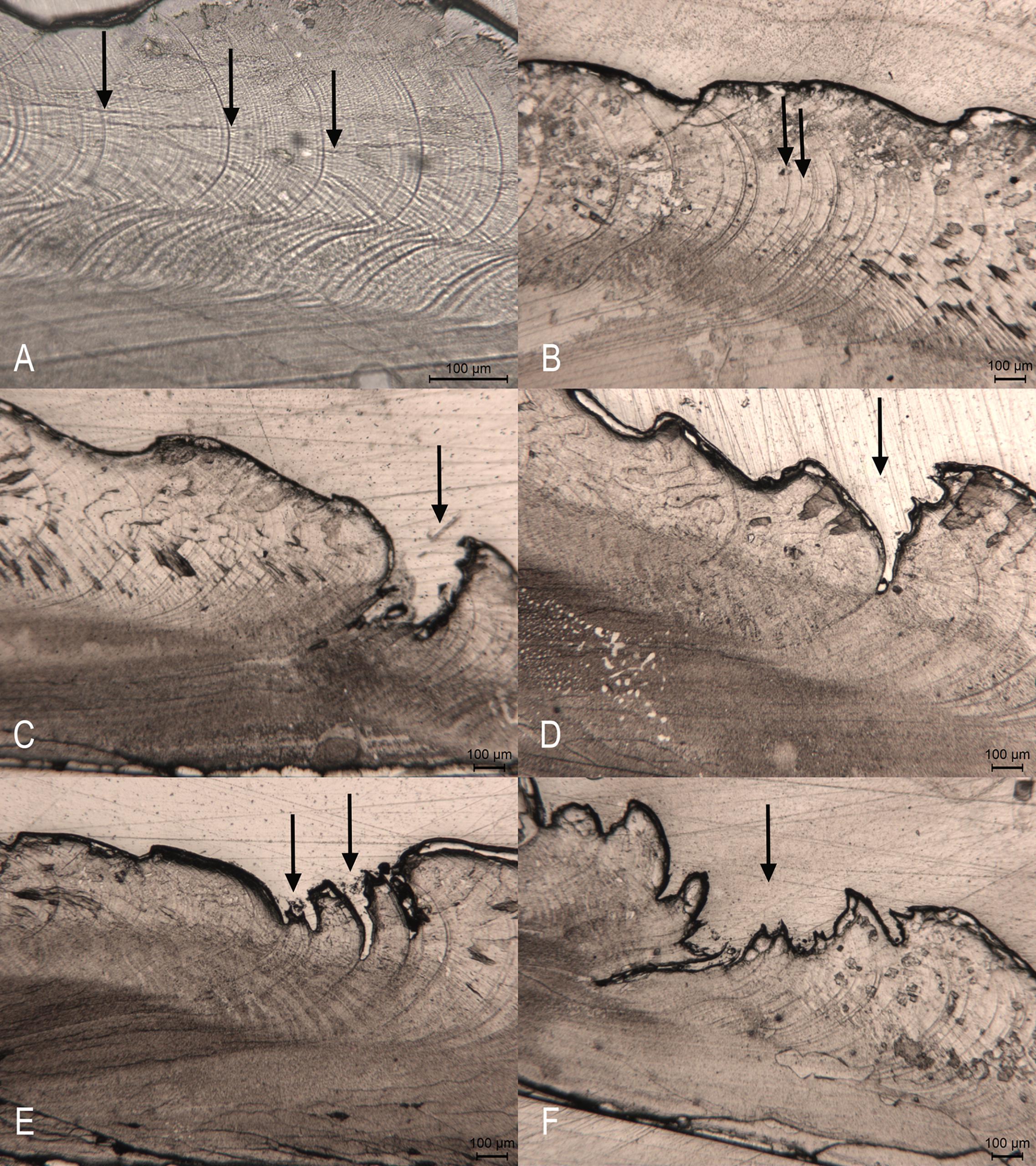
Figure 5. Photomicrographs of acetate peel replicas of C. gallina shell sections. (A) Widely spaced and marked bands deposited during the fast growth period (arrows). (B) Narrower and less defined bands deposited during the slow growth period (arrows). (C,D) Annual growth ring with an associated cleft (arrow). (E) Annual growth ring with two occasional associated clefts (arrows). (F) A stress ring (arrow).
The age of the specimens analyzed, which measured 11.9 to 37.7 mm, was estimated to range from 0.5 to 6.5 years (Figure 3B). The values of the indices of age precision were very low (APE = 1.18%; ACV = 2.92%), reflecting good method consistency and reproducibility. The mean length-at-age and SE were comparable to those obtained with the thin section method (Table 1). In particular, 1-year-old clams measured 15.25 ± 0.18 mm TL and 2-year-old specimens 21.69 ± 2.10 mm. The annual growth rate was faster in the first year of life (ca. 14–15 mm) and slower in the following years, as also noted in the thin sections (Table 2). The absence of significant differences between the two shell sectioning approaches on the LogLikelihood ratio test (χ2 = 4.66, df = 3, p = 0.198) indicated that both were described by the same VBF growth curve parameters. Their VBF growth curve parameters (L8 = 43.9, k = 0.26, t0 = −0.84) and their 95% confidence intervals (CIs) are compared with those of the shell surface growth rings in Table 1. Moreover, the ACV and APE between techniques were low, respectively 4.61 and 3.26%. Age bias plots showed that the mean age points lie almost perfectly on the agreement line, without differences in the age estimates yielded by the two techniques (Figure 6).
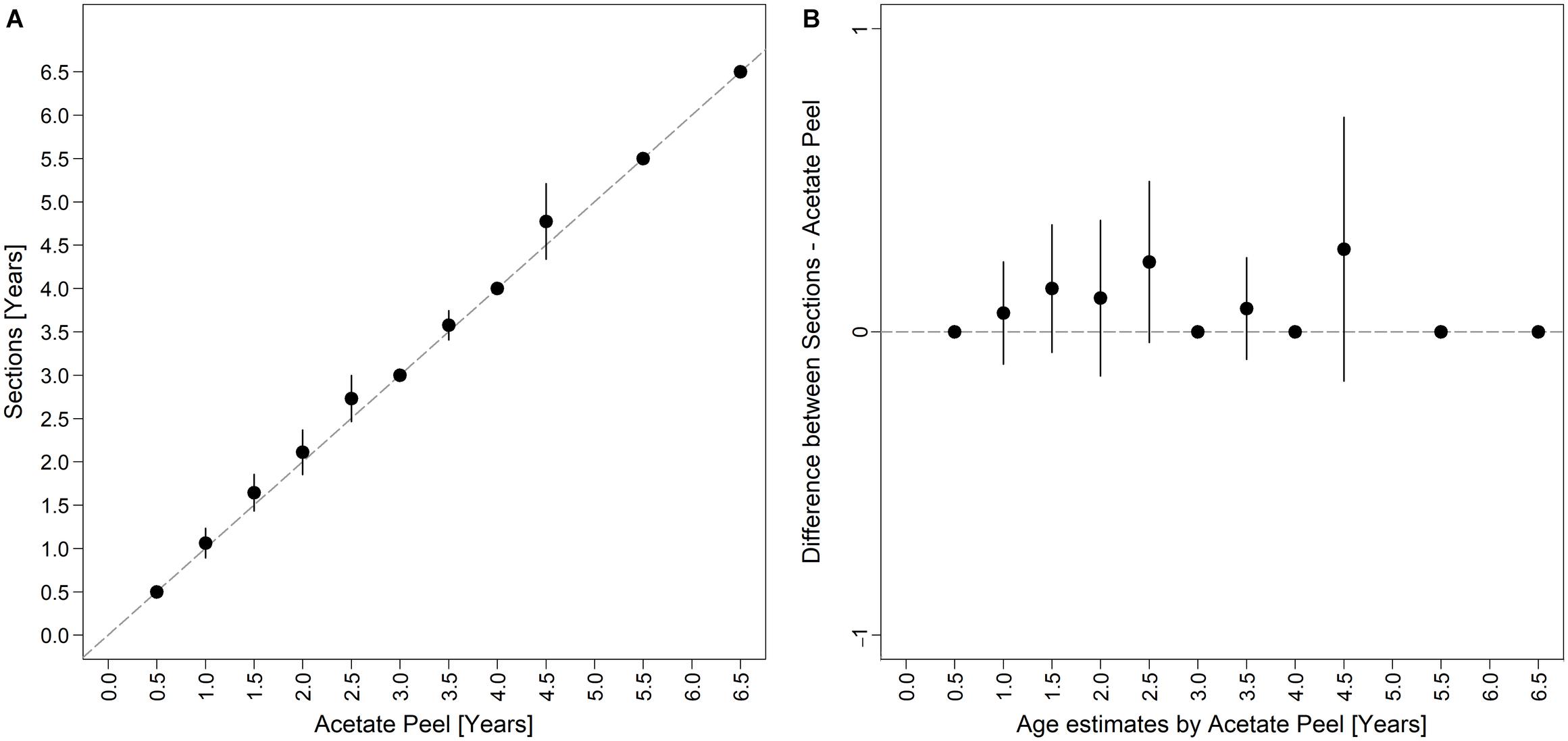
Figure 6. Bias plot comparing (A) differences in age readings between the two shell sectioning techniques as described by Campana et al. (1995), and (B) the differences between thin sections and acetate peels readings versus the acetate peels age estimates as described by Muir et al. (2008).
Surface Growth Rings
All the specimens used to asses age with the thin sections (length range, 12.4 – 37.7 mm) were also analyzed by the surface growth ring technique (Figure 7). The age estimates provided by this method ranged from 1.0 to 4.5 years (Figure 3C), thus missing the smallest and the largest age classes compared to the other two techniques (Figure 3D). Moreover, according to the VBF growth curve most specimens were aged 1.0–2.5 years, irrespective of their size, despite the relative low values of the precision indices (APE = 5.55%; ACV = 7.85%) reflected good estimates of the age. The VBF growth curve parameters (L8 = 26.4, k = 1.91, t0 = −0.11) and their 95% CIs (Table 3) differed widely from those of the other two techniques. Indeed, the LogLikelihood ratio test highlighted significantly different L8 and k (L8: χ2 = 13.62, df = 1, p < 0.001; k: χ2 = 9.18, df = 1, p < 0.002) between acetate peels/thin sections and surface growth rings, whereas the t0 values did not show any significant differences (χ2 = 0.93, df = 1, p = 0.335). The very high value of the instantaneous growth rate (k = 1.95) indicated a very fast growth, while the other methods yielded values ranging from 0.24 to 0.36, indicating a slow growth. The asymptotic length (26.3 mm) assumed by the model was also much lower than the maximum shell length of 37.7 mm recorded at sea. The mean length-at-age underestimated age size classes 3 and 4 compared with the other two techniques (Table 2), even without considering the absence of age classes 5 and 6. The minimum TL measured in 1-year-old specimens was an unrealistic 3.96 mm, indicating that false rings may occur throughout the shell height. Unlike the shell sectioning techniques, this method highlighted an alternate annual growth rate pattern (Table 2). Due to the very different age estimates, the age bias plot of the surface growth rings and one of the two shell sectioning methods is not reported.
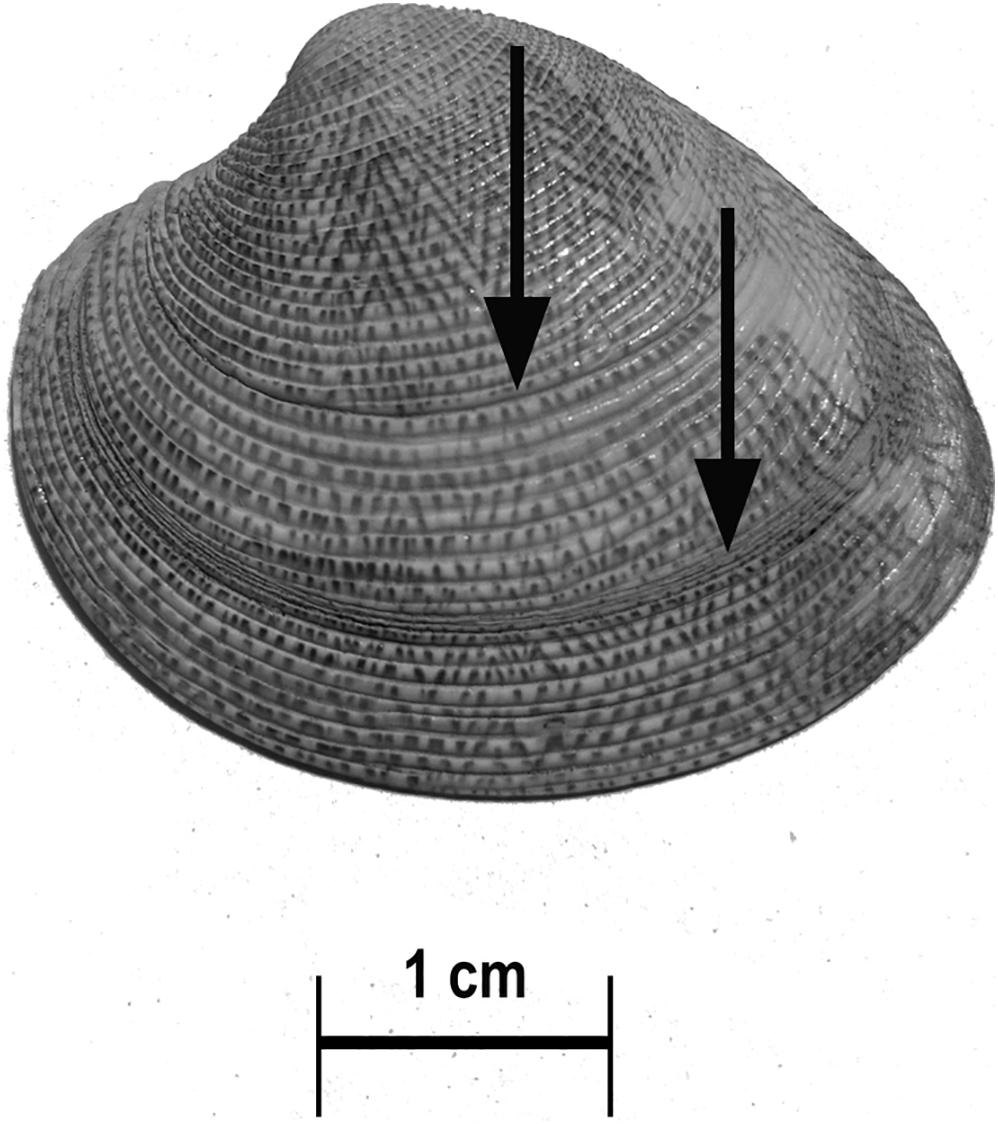
Figure 7. Surface growth rings (arrows) on the shell of C. gallina aging 2 years old following this approach. The specimen measured 37.7 mm total length and was aged 6 years according to both the thin section and the acetate peel replica techniques.
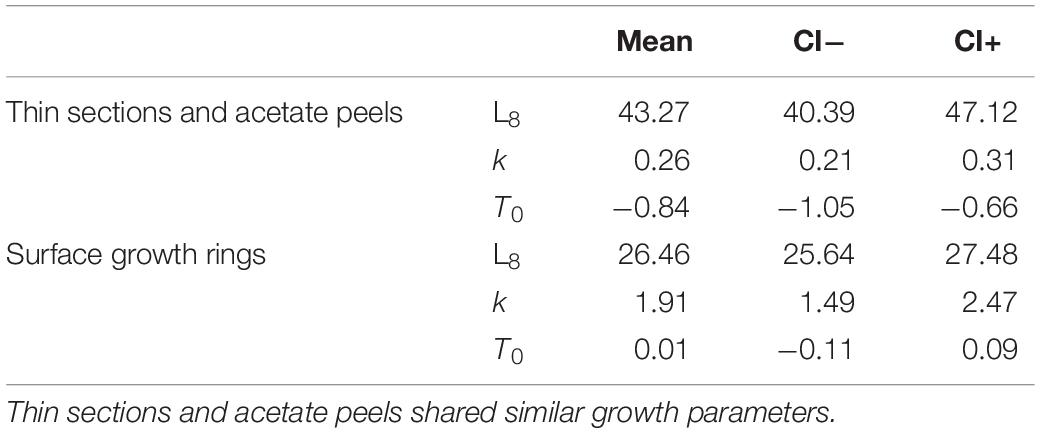
Table 3. Mean VBF growth curves parameters and respective CIs assessed by the model for Chamelea gallina from the mid-western Adriatic Sea using the three aging techniques.
Discussion
Age and growth studies provide crucial clam fishery management data. Although these parameters are generally considered together, each provides unique and useful information on specimens and populations. A variety of aging methods have been applied to assess C. gallina growth and population structure (Table 4). In this work, three techniques (thin sections, acetate peel replicas and surface growth rings) were compared to identify the most reliable and least time-consuming approach. Acetate peel replicas had never been employed to estimate the growth of C. gallina harvested in the Adriatic, despite its successful use in other geographical areas (e.g., Ramón and Richardson, 1992; Deval, 2001; Gaspar et al., 2004). According to our findings, the two shell sectioning techniques were equally reliable, whereas the shell surface growth ring approach underestimated age. Indeed, the approach has yielded contrasting results in various bivalve species, either underestimating (Gaspar et al., 2004; Hernández-Otero et al., 2014) or overestimating age (Gaspar et al., 1995; Peharda et al., 2002), despite the occasional success (Mancuso et al., 2019). In our study, underestimation was due to the large amount of shells presenting a smooth surface with no clefts, independently from the size, which led to a mismatch with the length-at-age data derived from the internal readings. The L8 value of 26.46 mm estimated by the model was lower than the maximum specimen length (37.7 mm) measured at sea; this result, combined with age underestimation (which in turn led to overestimation of the k value), made the approach unreliable. Moreover, the wholly unrealistic age attributed to the smallest specimens, aged 1, 2, and 3 years (measuring respectively 3.96, 8.26, and 10.71 mm TL) demonstrated that false rings may occur throughout the shell surface, whereas the age of 1 year, attributed to a specimen measuring 30.94 mm TL, was the result of the absence of visible annual growth rings, a feature that was shared by the majority of our samples. The method was therefore unreliable and error-prone, due to inherent difficulties related to the absence/misinterpretation of rings on the shell surface. Misinterpretation problems can partly be overcome by investigating the growth lines in the shell sections, which unlike the outer shell layer cannot be affected by environmental disturbances, thus ensuring greater accuracy in age determination (Anwar et al., 1990; Henderson and Richardson, 1994).
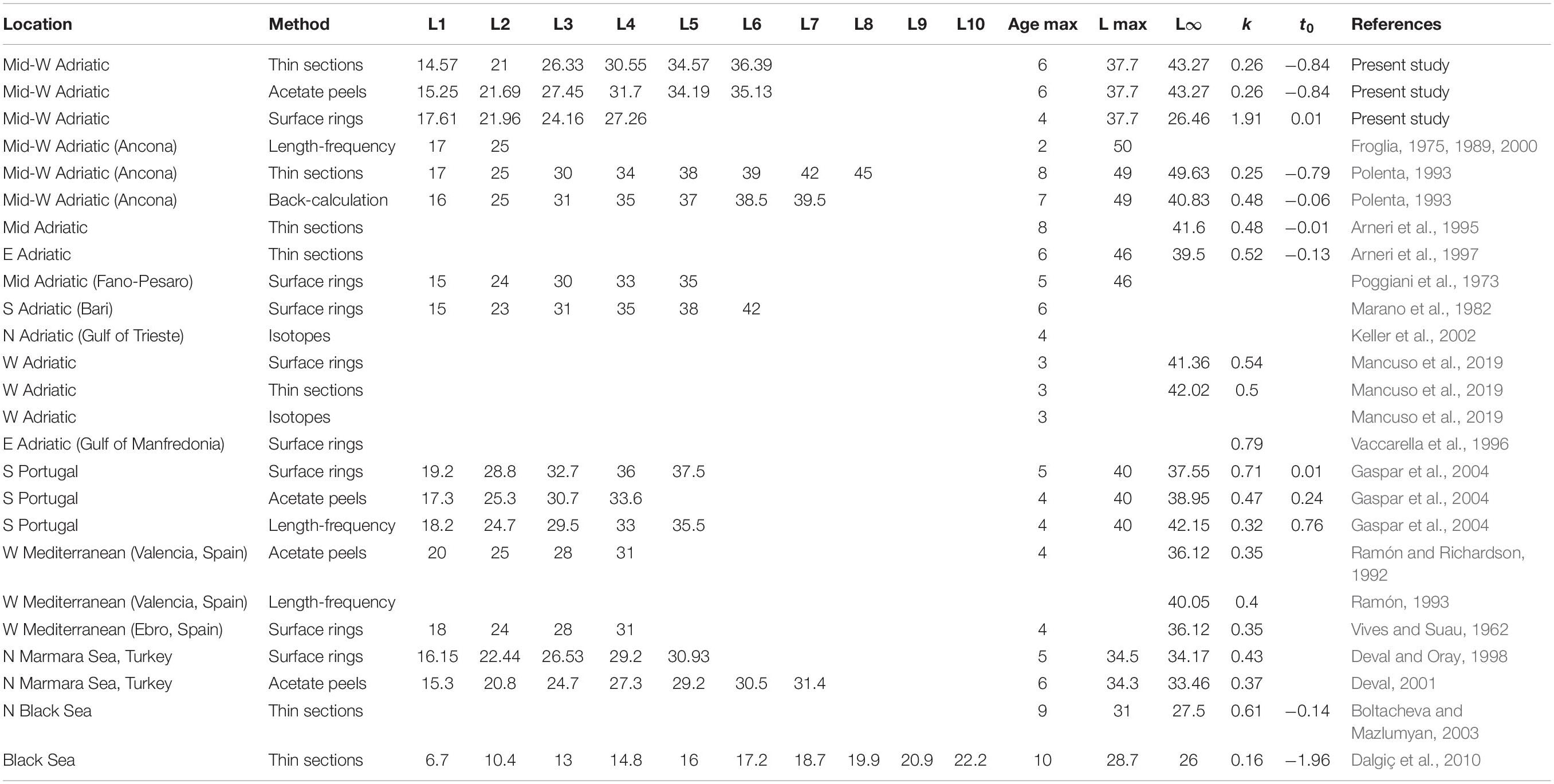
Table 4. Length at age, k, L8, t0 and maximum estimated age values obtained with different aging techniques in C. gallina specimens harvested inside and outside the Adriatic Sea (W, western; E, eastern; N, northern; S, southern).
The age range estimates provided by the two sectioning techniques was 0.5–6.5 years, although specimens aged 5 and 6 years were few; this entailed that length-at-age estimates were influenced by interindividual growth variability. Since the acetate peel replica technique was more time consuming than the thin section method, the latter approach was more suitable for age estimation in C. gallina. In the exceptional cases when the growth patterns are unclear both along the shell section and in the region of the umbo, which at times help counting (Dalgiç et al., 2010), then acetate peel replicas can be used. The annual periodicity of the growth bands on C. gallina thin sections has been validated in the western Adriatic Sea by Arneri et al. (1995), who determined that the translucent (dark zones) bands are laid down once a year, approximately between October and February, whereas the opaque bands (light zones) are deposited from March to September. Indeed, our edge analysis results, indicating that the largest margin increments were recorded in summer and the lowest in autumn-winter, were also confirmed by the observation of a specimen caught in June 2018, where the shell margin extent was 2.4 mm.
The two shell sectioning techniques demonstrated that the growth rate decreases as specimens become older, highlighting a very fast growth in the first year of life (of 14–15 mm TL). In the second year the growth rate had more than halved already. Several factors, including spawning, food availability, type of substratum, depth, light, temperature, salinity and population density may affect shell growth rate (Gaspar et al., 2004; Dalgiç et al., 2010). Growth is the result of linear extension along the umbonal-ventral axis per unit of time, and slows down with increasing age or size (Lorrain et al., 2004), as also confirmed by isotope analysis (Keller et al., 2002; Mancuso et al., 2019).
A comparison of our length-at-age results with data from works conducted in the same area highlighted a reduction in the maximum shell length ever recorded at sea on fishing grounds, and as well as in the k value, indicating a decline in shell growth rate over time (see Table 4). The differences in length-at-age data reported outside the Adriatic might be explained by different ecological conditions (Polenta, 1993; Gaspar et al., 2004; Dalgiç et al., 2010). In the Adriatic the estimated length at 1 year was very similar with all techniques, whereas differences emerged from the second year. In the past, 2-year-old clams have been reported to have a mean length of about 25 mm TL (the previous MCRS), whereas in this study it was just under 22 mm (the current MCRS), reflecting a reduction in shell growth rate over time that has already been reported by Biondi and Del Piero (2012) in the Gulf of Trieste. Froglia (1987) described a limited proportion of 3-year-old clams (TL > 35 mm) in the areas examined in the present study, indicating a faster growth rate at the time. We can assume that the fishing pressure may have induced a reduction in shell growth in the Adriatic, as in the Black Sea the growth rate has declined in areas subject to high fishing pressure compared to non-dredged areas, where clams grew faster (Dalgiç et al., 2010).
However, fishing pressure cannot be computed as the only cause of shell size reduction. Surveys conducted in the Adriatic by the National Research Council - Institute for Biological Resources and Marine Biotechnologies (CNR – IRBIM) of Ancona (Italy) in 2017, 2018, and 2019, when the MCRS was already 22 mm TL, found tens of thousands specimens under the MCRS per 100 m2 (DGPEMAC, 2019), reflecting not only a strong recruitment of the species supporting the commercial fractions of the stock for the following years, but also a very high density of specimens per unit area. In areas characterized by such density the strong competition for food limits growth and in extreme cases leads to mass mortality events (Liu et al., 2006). Fluctuations in salinity and rising chlorophyll concentration negatively affect C. gallina growth in the western Adriatic Sea (Mancuso et al., 2019), as well as temperatures below 10°C and above 27°C slow or inhibit shell linear extension rates (Ramón and Richardson, 1992; Moschino and Marin, 2006; Romanelli et al., 2009). Even a study carried out at the coastal station of the LTER (Long Term Ecosystem Research) Senigallia-Susak transect, ca. 40 km to the north of our study area, showed significantly increased phytoplankton abundance and biomass and inorganic nutrient concentrations in the period 2007–2016 compared with 1988–2002 due to increased Po River flows, which were observed since 2007; moreover, abnormally abundant rainfall combined with greater freshwater inputs in 2007–2016, especially in winter, explained the decreasing salinity trend recorded in the same years (Totti et al., 2019). Water acidification also affects clam shell growth (Fabry et al., 2008) and reduces shell thickness (Bressan et al., 2014). Even though increasing water acidification has been documented in north Adriatic dense deep waters (Luchetta et al., 2010), the change is too limited to reduce the saturation state of carbonates to an extent that would significantly affect clam calcification processes (Totti et al., 2019).
Therefore, shell growth decline may be the result of the synergistic action of multiple factors. Studies should also be carried out in non-dredged areas, to understand and quantify the role of fishing activity. Detailed studies of the population age structure and growth of C. gallina are essential, since uncertainties in age estimation undermine the effectiveness of management actions. A responsible management plan for the striped venus clam fishery should take into account the biological aspects of the species and the effects of the gear on the populations, and provide guidelines to ensure the persistence and conservation of the species over time. A management plan capable to return larger individuals as in the past should not to be intended as an appropriate measure, not possible condition due to the intrinsic characteristics of the gear, but a suitable plan is the one able to allow the exploitation of the resource not exceeding the maximum sustainable yield. The present study found that clams of 22 mm TL were, on average, individuals of two years old while in the past decades the achievement of the second year was on average reported at about 25 mm TL. Therefore, to allow the maintenance in Italian territorial waters of the present MCRS set at 22 mm TL [Delegated Regulation (UE) No. 2016/2376, 2016; Commission Delegated Regulation (EU) 2020/3, 2020] by way of derogation to the previous 25 mm TL [Council Regulation (CE) No. 1967/2006, 2006] further studies including investigations into the reproductive potential of C. gallina at different sizes should be carried out based on the knowledge that the species reaches the size of maturity during the first year of life.
Data Availability Statement
The raw data supporting the conclusions of this article will be made available by the authors, without undue reservation.
Ethics Statement
All applicable international, national, and/or institutional guidelines for the care and use of animals were followed.
Author Contributions
GB wrote the manuscript with the contribution of CV, MV, and AL. GB, MV, and AP collected the samples. GB, FD, and AP conducted the different lab activities. CV performed the statistical analysis. AL was the scientific responsible of the study. All the authors contributed to the article and approved the submitted version.
Funding
The research was made within the European Data Collection Framework and was financially supported by the Italian Ministry for Agricultural, Food and Forestry Policies (MiPAAF).
Conflict of Interest
The authors declare that the research was conducted in the absence of any commercial or financial relationships that could be construed as a potential conflict of interest.
Acknowledgments
The research that led to these results was carried out in the framework of the Research Doctorate in Innovative Technologies and Sustainable Use of Mediterranean fishing and biological resources (FishMed-Ph.D.). We are indebted to the crews of the fishing vessels for their contribution to the field work and to the graduate student Vittoria Ghiglione who collaborated in some activities. Word Designs is acknowledged for the language revision.
References
Adjei-Boateng, D., and Wilson, G. J. (2013). Age determination and growth rate of the freshwater clam Galatea paradoxa (Born 1778) from the Volta river estuary, Ghana. J. Aquat. Sci. 1, 31–38.
Anwar, N. A., Richardson, C. A., and Seed, R. (1990). Age determination, growth rate and population structure of the horse mussel Modiolus modiolus. J. Mar. Biol. Assoc. U. K. 70, 441–457. doi: 10.1017/S0025315400035529
Arneri, E., Froglia, C., Polenta, R., and Antolini, B. (1997). Growth of Chamelea gallina (Bivalvia: Veneridae) in the eastern Adriatic (Neretva river estuary). Tisuću God. Prv. Spomena Ribar. Hrvata 597, 669–676.
Arneri, E., Giannetti, G., Polenta, R., and Antolini, B. (1995). Age and growth of Chamelea gallina (Bivalvia: Veneridae) in the Central Adriatic Sea obtained by thin sections. Rapp. Commun. Int. Mer. Médit. 34:17.
Biondi, S., and Del Piero, D. (2012). Survey on Chamelea gallina beds in the Lignano area (Gulf of Trieste, Adriatic Sea). Annales: Series Historia Naturalis 22, 35–44.
Boltacheva, N. A., and Mazlumyan, S. A. (2003). The growth and longevity of Chamelea gallina (Mollusca, Veneridae) in the Black Sea. Vestn. Zool. 37, 71–74.
Bressan, M., Chinellato, A., Munari, M., Matozzo, V., Manci, A., Marčeta, T., et al. (2014). Does seawater acidification affect survival, growth and shell integrity in bivalve juveniles? Mar. Environ. Res. 99, 136–148. doi: 10.1016/j.marenvres.2014.04.009
Campana, S. E., Annand, M. C., and McMillan, J. I. (1995). Graphical and statistical methods for determining the consistency of age determinations. Trans. Am. Fish. Soc. 124, 131–138. doi: 10.1577/1548-8659(1995)124<0131:gasmfd>2.3.co;2
Carlucci, R., Piccinetti, C., Scardi, M., Del Piero, D., and Mariani, A. (2015). Evaluation of the effects on the clam resource in the light of a new minimum landing size and a better biological and commercial management of the product. Final Rep. 76, 1–76.
Christian, A. D., Davidson, C. L., Posey, I. I., William, R., Rust, P. J., Farris, J. L., et al. (2000). Growth curves of four species of commercially valuable freshwater mussels (Bivalva: Unionidae). J. Ark. Acad. Sci. 54, 41–50.
Commission Delegated Regulation (EU) 2020/3 (2020). Commission delegated regulation (EU) 2020/3 of 28 August 2019 establishing a discard plan for Venus shells (Venus spp.) in. (certain)Italian territorial waters. Off. J. Eur. Union 2020, 1–4. doi: 10.1007/978-3-319-23534-9_1
Cordisco, C. A., Romanelli, M., and Trotta, P. (2003). Annual determination and description of the larval stages of Chamelea gallina (L.) and Mytilus galloprovincialis (Lamark) in the central and southern Adriatic. Atti. Assoc. Ital. Ocean. Limnol. 16, 93–103.
Council Regulation (CE) No. 1967/2006 (2006). Council Regulation (EC) No 1967/2006 of 21 December 2006 Concerning Management Measures for the Sustainable Exploitation of Fishery Resources in the Mediterranean Sea, AMENDING REGULation (EEC) No 2847/93 and Repealing Regulation (EC) No 1626/94. Off. J. Eur. Union, L 409/11, 75. Available online at: https://eur-lex.europa.eu/legal-content/en/ALL/?uri=CELEX%3A32006R1967 (accessed June 10, 2020).
Dalgiç, G., Okumuş, İ, and Karayücel, S. (2010). The effect of fishing on growth of the clam Chamelea gallina (Bivalvia: Veneridae) from the Turkish Black Sea coast. J. Mar. Biol. Assoc. U. K. 90, 261–265. doi: 10.1017/s0025315409000939
Delegated Regulation (UE) No. 2016/2376 (2016). Commission delegated regulation (EU) 2016/2376 of 13 October 2016 establishing a rejection plan for bivalve molluscs Venus spp. in Italian territorial waters. Off. J. Eur. Union 2016, 48–49.
Deval, M. C. (2001). Shell growth and biometry of the striped venus Chamelea gallina (L) in the Marmara Sea, Turkey. J. Shellf. Res. 20, 155–159.
Deval, M. C., and Oray, I. K. (1998). The annual shell increments of bivalvia Chamelea gallina L. 1758 in the northern Sea of Marmara. Oebalia 24, 93–109.
DGPEMAC (2019). National Management Plan for Fishing Activities with the Hydraulic Dredger System and Rakes for Boats as Identified in the Designation of Fishing Gear in Mechanical Dredgers Including Hydraulic Mechanized Dredger (HMD) and Mechanized Dredger. Public Law No. 9913. Ministry of Agricultural, Food and Forestry Policies: Rome
Ezgeta-Balić, D., Peharda, M., Richardson, C. A., Kuzmanić, M., Vrgoč, N., and Isajlović, I. (2011). Age, growth, and population structure of the smooth clam Callista chione in the eastern Adriatic Sea. Helgol. Mar. Res. 65:457. doi: 10.1007/s10152-010-0235-y
Fabry, V. J., Seibel, B. A., Feely, R. A., and Orr, J. C. (2008). Impacts of ocean acidification on marine fauna and ecosystem processes. ICES J. Mar. Sci. 65, 414–432. doi: 10.1093/icesjms/fsn048
Fiori, S. M., and Morsán, E. M. (2004). Age and individual growth of Mesodesma mactroides (Bivalvia) in the southernmost range of its distribution. ICES J. Mar. Sci. 61, 1253–1259. doi: 10.1016/j.icesjms.2004.07.025
Froglia, C. (1975). Observations on the growth of Chamelea gallina (L.) and Ensis minor (Chenu) in the middle Adriatic. Quad. Lab. Tecnol. Pesca 2, 37–48.
Froglia, C. (1987). Evaluation of the consistency of clams in the maritime compartments of Ancona and San Benedetto del Tronto. Final Rep. 28, 1–28.
Froglia, C. (1989). “Clam fisheries with hydraulic dredges in the Adriatic Sea,” in Management of Fisheries for Marine Invertebrates, ed. J. F. Caddy (Springer: Berlin), 507–524.
Froglia, C. (2000). The contribution of scientific research to the management of bivalve mollusc fishing with hydraulic dredgers. Biol. Mar. Mediterr. 7, 71–82.
Gaspar, M. B., Castro, M., and Monteiro, C. C. (1995). Age and growth rate of the clam, Spisula solida L., from a site off Vilamoura, south Portugal, determined from acetate replicas of shell sections. Sci. Mar. 59, 87–93.
Gaspar, M. B., Pereira, A. M., Vasconcelos, P., and Monteiro, C. C. (2004). Age and growth of Chamelea gallina from the Algarve coast (southern Portugal): influence of seawater temperature and gametogenic cycle on growth rate. J. Molluscan Stud. 70, 371–377. doi: 10.1093/mollus/70.4.371
Gibson, R., Barnes, M., and Atkinson, R. (2001). Molluscs as archives of environmental change. Ocean. Mar. Biol. Annu. Rev. 39, 103–164.
Henderson, S. M., and Richardson, C. A. (1994). A comparison of the age, growth rate and burrowing behaviour of the razor clams, Ensis siliqua and E. ensis. J. Mar. Biol. Assoc. U. K. 74, 939–954. doi: 10.1017/S0025315400090160
Hernández-Otero, A., Gaspar, M. B., Macho, G., and Vázquez, E. (2014). Age and growth of the sword razor clam Ensis arcuatus in the Ría de Pontevedra (NW Spain): influence of environmental parameters. J. Sea Res. 85, 59–72. doi: 10.1016/j.seares.2013.09.006
Herrmann, M., Lepore, M. L., Laudien, J., Arntz, W. E., and Penchaszadeh, P. E. (2009). Growth estimations of the Argentinean wedge clam Donax hanleyanus: a comparison between length-frequency distribution and size-increment analysis. J. Exp. Mar. Bio. Ecol. 379, 8–15. doi: 10.1016/j.jembe.2009.07.031
Jones, D. S., Quitmyer, I. R., Arnold, W. S., and Marelli, D. C. (1990). Annual shell banding, age, and growth rate of hard clams (Mercenaria spp.) from Florida. J. Shellf. Res. 9, 215–255.
Jones, D. S., Thompson, I., and Ambrose, W. (1978). Age and growth rate determinations for the Atlantic surf clam Spisula solidissima (Bivalvia: Mactracea), based on internal growth lines in shell cross-sections. Mar. Biol. 47, 63–70. doi: 10.1007/BF00397019
Keller, N., Del Piero, D., and Longinelli, A. (2002). Isotopic composition, growth rates and biological behaviour of Chameleagallina and Callistachione from the Bay of Trieste (Italy). Mar. Biol. 140, 9–15. doi: 10.1007/s002270100660
Liu, B., Dong, B., Tang, B., Zhang, T., and Xiang, J. (2006). Effect of stocking density on growth, settlement and survival of clam larvae, Meretrix meretrix. Aquaculture 258, 344–349. doi: 10.1016/j.aquaculture.2006.03.047
Lorrain, A., Paulet, Y.-M., Chauvaud, L., Dunbar, R., Mucciarone, D., and Fontugne, M. (2004). δ13C variation in scallop shells: increasing metabolic carbon contribution with body size? Geochim. Cosmochim. Acta 68, 3509–3519. doi: 10.1016/j.gca.2004.01.025
Luchetta, A., Cantoni, C., and Catalano, G. (2010). New observations of CO2-induced acidification in the northern Adriatic Sea over the last quarter century. Chem. Ecol. 26, 1–17. doi: 10.1080/02757541003627688
Mancuso, A., Stagioni, M., Prada, F., Scarponi, D., Piccinetti, C., and Goffredo, S. (2019). Environmental influence on calcification of the bivalve Chamelea gallina along a latitudinal gradient in the Adriatic Sea. Sci. Rep. 9, 1–11.
Marano, G., Casavola, N., Saracino, C., and Rizzi, E. (1982). Reproduction and growth of Chamelea gallina (L.) and Venus verrucosa (L.) (Bivalvia: Veneridae) in the southern Adriatic. Mem. Biol. Mar. Ocean. 12, 97–114.
Masu, T., Watanabe, S., Aoki, S., Katayama, S., Fukuda, M., and Hino, A. (2008). Establishment of shell growth analysis technique of juvenile Manila clam Ruditapes philippinarum: semidiurnal shell increment formation. Fish. Sci. 74, 41–47. doi: 10.1111/j.1444-2906.2007.01493.x
Morello, E. B., Froglia, C., Atkinson, R. J. A., and Moore, P. G. (2006). The effects of hydraulic dredging on the reburial of several molluscan species. Biol. Mar. Mediterr. 13, 610–613.
Moschino, V., and Marin, M. G. (2006). Seasonal changes in physiological responses and evaluation of “well-being” in the Venus clam Chamelea gallina from the Northern Adriatic Sea. Comp. Biochem. Physiol. Part A Mol. Integr. Physiol. 145, 433–440. doi: 10.1016/j.cbpa.2006.07.021
Moura, P., Gaspar, M. B., and Monteiro, C. C. (2009). Age determination and growth rate of a Callista chione population from the southwestern coast of Portugal. Aquat. Biol. 5, 97–106. doi: 10.3354/ab00119
Muir, A. M., Ebener, M. P., He, J. X., and Johnson, J. E. (2008). A comparison of the scale and otolith methods of age estimation for lake whitefish in Lake Huron. North Am. J. Fish. Manag. 28, 625–635. doi: 10.1577/m06-160.1
Ogle, D., Wheeler, P., and Dinno, A. (2020). FSA: Fisheries Stock Analysis. R Package Version 0.8.30. Available online at: https://github.com/droglenc/FSA (accessed June 15, 2020).
Orban, E., Di Lena, G., Nevigato, T., Casini, I., Caproni, R., Santaroni, G., et al. (2007). Nutritional and commercial quality of the striped venus clam, Chamelea gallina, from the Adriatic sea. Food Chem. 101, 1063–1070. doi: 10.1016/j.foodchem.2006.03.005
Peharda, M., Richardson, C. A., Onofri, V., Bratos, A., and Crnčević, M. (2002). Age and growth of the bivalve Arca noae L. in the Croatian Adriatic sea. J. Molluscan Stud. 68, 307–310. doi: 10.1093/mollus/68.4.307
Péres, J. M., and Picard, J. (1964). New manual for benthic bionomics in the Mediterranean Sea. Trav. Stn. Marittime Endoume 31:137.
Poggiani, L., Piccinetti, C., and Piccinetti Manfrin, G. (1973). Observations on the biology of bivalve molluscs Venus gallina L. and Tapes aureus Gmelin in the northern Adriatic. Note Lab. Biol. Mar. Pesca Fano 4, 189–212.
Polenta, R. (1993). Observations on Growth of the Striped Venus Clam Chamelea gallina L. in the Middle Adriatic. Bologna, IT: Bachelor of Science degree, University of Bologna, 1–61.
R Core Team (2013). R: A Language and Environment for Statistical Computing. Vienna: R Foundation for Statistical Computing.
Ramón, M. (1993). Study of the Populations of Chamelea gallina (Linnaeus, 1758) and Donax trunculus Linnaeus, 1758 (Mollusca: Bivalvia) in the Gulf of Valencia (Western Mediterranean). PhD dissertation, University of Barcelona, Barcelona, 1–412.
Ramón, M., and Richardson, C. A. (1992). Age determination and shell growth of Chamelea gallina (Bivalvia: Veneridae) in the western Mediterranean. Mar. Ecol. Prog. Ser. 89, 15–23. doi: 10.3354/meps089015
Rhoads, D. C., and Lutz, R. A. (1980). Skeletal growth of aquatic organisms: biological records of environmental change. Top. Geobiol. 1:763.
Rhoads, D. C., and Pannella, G. (1970). The use of molluscan shell growth patterns in ecology and paleoecology. Lethaia 3, 143–161. doi: 10.1111/j.1502-3931.1970.tb01854.x
Richardson, C., Crisp, D., and Runham, N. (1979). Tidally deposited growth bands in the shell of the common cockle, Cerastoderma edule (L.). Malacology 18, 277–290.
Rizzo, G., Cernigai, F., Marceta, T., Bressan, M., and Marin, M. G. (2011). Physiological and reproductive features in Chamelea gallina as a contribution to stock management in the northern Adriatic Sea. Biol. Mar. Mediterr. 18:324.
Rizzo, G., Marceta, T., MaTozzo, V., BRessan, M., and MaRin, M. G. (2010). New insight on clam population (Chmelea gallina) in the Chioggia District: morphometric and physiological data. Biol. Mar. Mediterr. 17:330.
Romanelli, M., Cordisco, C. A., and Giovanardi, O. (2009). The long-term decline of the Chamelea gallina L.(Bivalvia: Veneridae) clam fishery in the Adriatic Sea: is a synthesis possible. Acta Adriat. 50, 171–205.
Ropes, J. W., Jones, D. S., Murawski, S. A., Serchuk, F. M., and Jerald, A. (1984). Documentation of annual growth lines in ocean quahogs Arctica islandica Linné. Fish. Bull. 82, 1–19.
Scopa, M., Nerone, E., Recchi, S., and Barile, N. B. (2014). Trends in the Chamelea gallina production from molise region (Adriatic Sea, Italy): a ten-year survey. Glob. J. Sci. Front. Res. D Agric. Vet. 14, 1–17.
Tirado, C., and Salas, C. (1998). Reproduction and fecundity of Donax trunculus L., 1758 (Bivalvia: Donacidae) in the littoral of Málaga (southern Spain). J. Shellf. Res. 17, 169–176.
Totti, C., Romagnoli, T., Accoroni, S., Coluccelli, A., Pellegrini, M., Campanelli, A., et al. (2019). Phytoplankton communities in the northwestern Adriatic Sea: interdecadal variability over a 30-years period (1988-2016) and relationships with meteoclimatic drivers. J. Mar. Syst. 193, 137–153. doi: 10.1016/j.jmarsys.2019.01.007
Vaccarella, R., Pastorelli, A. M., De Zio, V., Rositani, L., and Paparella, P. (1996). Evaluation of the biomass of marketable bivalve molluscs in the Gulf of manfredonia. Biol. Mar. Medit. 3, 237–241.
Versteegh, E. A. A., Vonhof, H. B., Troelstra, S. R., Kaandorp, R. J. G., and Kroon, D. (2010). Seasonally resolved growth of freshwater bivalves determined by oxygen and carbon isotope shell chemistry. Geochem. Geophys. Geosyst. 11, 1–16. doi: 10.1029/2009GC002961
Keywords: striped venus clam, age, growth, thin sections, acetate peels, surface growth rings
Citation: Bargione G, Vasapollo C, Donato F, Virgili M, Petetta A and Lucchetti A (2020) Age and Growth of Striped Venus Clam Chamelea gallina (Linnaeus, 1758) in the Mid-Western Adriatic Sea: A Comparison of Three Laboratory Techniques. Front. Mar. Sci. 7:582703. doi: 10.3389/fmars.2020.582703
Received: 13 July 2020; Accepted: 03 September 2020;
Published: 24 September 2020.
Edited by:
Tomaso Fortibuoni, Higher Institute for Environmental Protection and Research (ISPRA), ItalyReviewed by:
Marco Munari, University of Naples Federico II, ItalyAna Bratoš Cetinić, University of Dubrovnik, Croatia
Copyright © 2020 Bargione, Vasapollo, Donato, Virgili, Petetta and Lucchetti. This is an open-access article distributed under the terms of the Creative Commons Attribution License (CC BY). The use, distribution or reproduction in other forums is permitted, provided the original author(s) and the copyright owner(s) are credited and that the original publication in this journal is cited, in accordance with accepted academic practice. No use, distribution or reproduction is permitted which does not comply with these terms.
*Correspondence: Giada Bargione, giada.bargione@irbim.cnr.it