- Departamento de Microbioloxía e Parasitoloxía, Instituto de Acuicultura, Universidade de Santiago de Compostela, Santiago de Compostela, Spain
Photobacterium damselae subsp. damselae (Pdd), an important pathogen for marine animals, is also an opportunistic human pathogen that can cause fatal necrotizing fasciitis. The regulatory changes triggered by the temperature shift experienced by this marine pathogen upon entering the human body, are completely unknown. Here we report an RNA-seq approach combined with phenotypical assays to study the response of Pdd to cultivation at 37°C in comparison to 25°C. We found that cultivation of a Pdd highly virulent strain for fish and mice, RM-71, at 37°C, initially enhanced bacterial growth in comparison to 25°C as evidenced by the increase in optical density. However, cells were found to undergo a progressive loss of viability after 6 h cultivation at 37°C, and no viable cells could be detected from 30 h cultures at 37°C. In contrast, at 25°C, viable cell counts achieved the highest values at 30 h cultivation. Cells grown at 25°C showed normal rod morphology by scanning electron microscopy analysis whereas cells grown at 37°C exhibited chain-like structures and aberrant long shapes suggesting a defect in daughter cell separation and in septum formation. Cells grown at 37°C also exhibited reduced tolerance to benzylpenicillin. Using a RNA-seq approach we discovered that growth at 37°C triggered a heat-shock response, whereas genes involved in motility and virulence were repressed including iron acquisition systems, the type two secretion system, and damselysin toxin, a major virulence factor of Pdd. Human isolates did not exhibit advantage growing at 37°C compared to fish isolates, and comparative genomics did not reveal gene markers specific of human isolates, suggesting that any Pdd genotype existing in the marine environment might potentially cause disease in humans. Altogether, these data indicate that the potential of Pdd to cause disease in humans is an accidental condition rather than a selected trait, and that human body temperature constitutes a stressful condition for Pdd. This study provides the first transcriptome profile of Pdd exposed at human body temperature, and unveils a number of candidate molecular targets for prevention and control of human infections caused by this pathogen.
Introduction
Photobacterium damselae subsp. damselae (hereafter Pdd) is a bacterium of the family Vibrionaceae pathogenic for a broad range of hosts including marine animals and humans (Osorio et al., 2018). It represents a major threat for marine fish aquaculture worldwide, and disease outbreaks in fish farms are typically preceded by increases in sea water temperature during summer months (Fouz et al., 1992; Pedersen et al., 2009; Eissa et al., 2018; Yu et al., 2019). In humans, Pdd can cause severe wound infections and necrotizing fasciitis that may evolve into a fatal outcome despite prompt antibiotic administration (Morris et al., 1982; Clarridge and Zighelboim-Daum, 1985; Yuen et al., 1993; Asato and Kanaya, 2004; Yamane et al., 2004; Hundenborn et al., 2013; Akram et al., 2015; Alhemairi et al., 2015). Notably, some authors recommend to surgically debride and to amputate at an early stage of the infection to save lives of patients (Goodell et al., 2004). Underlying diseases (as diabetes, liver disorders and immunodeficiency among others) may accompany infection (Knight-Madden et al., 2005; Nakamura et al., 2008; Kim et al., 2009), but they are not a prerequisite for the development of disease since apparently healthy individuals are also susceptible (Morris et al., 1982; Barber and Swygert, 2000; Yamane et al., 2004). Human infections by Pdd originate through exposure of subcutaneous tissue to the marine environment or through wounds inflicted during fish handling. Pdd is considered one of the main zoonotic pathogens acquired topically from fish (Lehane and Rawlin, 2000; Austin, 2010), and the majority of human cases concentrate in coastal areas of the United States of America, Japan and Australia, during the warm season.
The major reported virulence factors of Pdd are cytotoxins with hemolytic activity (Osorio et al., 2018). The virulence plasmid pPHDD1 encodes the cytotoxins damselysin (Dly) and phobalysin P (PhlyP) (Rivas et al., 2011), and two additional cytotoxins, phobalysin C (PhlyC) and phospholipase PlpV are encoded within chromosome I (Rivas et al., 2013; Vences et al., 2017). These virulence factors are secreted via the type II secretion system (Rivas et al., 2015a; Vences et al., 2017). Recently, it was reported that the two-component regulatory system RstAB regulates transcription of the genes encoding Dly, PhlyP, and PhlyC toxins, and consequently its inactivation strongly impairs virulence (Terceti et al., 2017, 2019).
Despite the aggressive outcome of Pdd infections in humans, nothing is known about how this bacterium responds to the temperature shift that it encounters in its transition from warm sea waters to invading a human host. In order to shed light on this, in the current work we compared the transcriptomes of Pdd at 25 and 37°C, mimicking the environmental vs. the host temperature conditions. In addition, the genome sequences of two human isolates of Pdd are reported for the first time, and a comparative genomics analysis between human and fish strains is presented. Growth assays, viability tests and cell morphology studies, together with the transcriptomics data, all suggest that growth at 37°C represents a stressful condition and not an evolutionarily selected trait for Pdd. Overall, these data are expected to contribute to the investigation of novel approaches for prevention and for less invasive control of human infections caused by Pdd in a global warming scenario.
Materials and Methods
Culture Conditions, Viability Assays, and Fatty Acid Methyl Ester (FAME) Analysis
Pdd strains were grown at 25 or 37°C on tryptic soy agar (TSA) or in tryptic soy broth (TSB) supplemented with NaCl up to 1% (TSA-1 and TSB-1, respectively). For growth curves, three replicates for each temperature of the assay (25 and 37°C) were grown in TSB-1 until obtaining exponentially growing precultures (OD600: 0.3). Then, 1:100 dilutions of each preculture were grown in 100 μl of TSB-1 in 96 well plates and the optical density (OD600) was measured using the spectrophotometer Epoch2 microplate reader (Biotek). For drop-plating viability assays, three independent cultures were grown at each temperature using the spectrophotometer Epoch2 microplate reader under the same conditions used for growth curve analysis, and 5 μl aliquots of 10-fold dilutions were drop-plated in TSA-1 plates after 6, 12, 24, 30, 36, and 48 h of incubation, and plates were incubated at 25°C for 24 h. FAME analysis was performed by extracting and preparing fatty acid methyl esters from 24 h Pdd RM-71 cultures on TSA-1 at 25 or 37°C and using the MIDI Sherlock® Microbial Identification System (MIDI, Inc., United States), following manufacturer’s recommendations.
Genome Sequencing and Comparative Genomics
Draft genome sequences of human isolates CDC-2227-81 (Kreger, 1984) and 80077637 (Hundenborn et al., 2013) were obtained using an Illumina platform as previously described (Abushattal et al., 2019) and annotated using RAST tool (Aziz et al., 2008). Sequence similarity analysis by comparison of orthologous fragments between pairs of genomes was conducted with OrthoAni (Lee et al., 2016). Core genome and unique genes were calculated using RAST (Aziz et al., 2008). Genomic BLAST file of Photobacterium damselae subsp. damselae strains 80077637, CDC-2227-81, RM-71, CIP102761, A-162, and LD-07 was downloaded from NCBI1, and the dendrogram was visualized by Interactive Tree of Life (iTOL v5) (Letunic and Bork, 2019). The draft genome sequences obtained in this study have been deposited at GenBank/EMBL/DDBJ under accession number VZUQ00000000 (CDC-2227-81) and WAEO00000000 (80077637).
Scanning Electron Microscopy
Exponentially growing cultures of Pdd RM-71 in TSB-1 at each temperature were stopped when they reached an OD600 of 0.55, cells were carefully pelleted down by centrifugation (4,000 g) and fixed for 3 h at 4°C in 4% paraformaldehyde and 2% glutaraldehyde in 0.1 M phosphate buffer, pH 7.4, and postfixed for 1.5 h in 1% osmium tetroxide in the same buffer. Samples were washed three times in dH2O, dehydrated using a series of graded ethyl alcohols, chemically dried using HMDS (hexamethyldisilazane) (Sigma), sputter-coated with iridium, and viewed and photographed in an Ultra Plus ZEISS scanning electron microscope.
E-test Assay
To determine the susceptibility to benzylpenicillin at the two assayed temperatures, Pdd strain RM-71 was grown at a temperature of 25°C in 10 ml TSB-1 in 100 ml flasks, and collected when cultures reached a sharp optical density at 600 nm (OD600) of 0.5. Aliquots of 100 μl were spread onto TSA-1 plates in the presence of E-test gradient antibiotic strips (bioMérieux), and incubated for 24 h at 25°C and 37°C.
RNA-Seq
For RNA-seq, three independent precultures of RM-71 strain for each temperature condition were started and grown until an OD600 of 0.3. Then, 1:100 dilutions of each preculture were grown in 10 ml of TSB-1 in 100 ml flasks until they reached a sharp OD600 of 0.55. Cells were immediately treated with RNAprotect Bacteria Reagent (Qiagen) for stabilization of RNA following manufacturer’s instructions. Pelleted cells were then carefully resuspended in 100 μl TE buffer (30 mM Tris⋅Cl, 1 mM EDTA, pH 8.0), adding 10 μl lysozyme (15 mg/ml) (Sigma Aldrich) and 15 μl Proteinase K (20 mg/ml) (Qiagen). RNA was extracted with RNeasy Mini Kit (Qiagen), and DNase I treatment was performed using the on-column kit RNase-free DNase (Qiagen). The quality and the quantity of the total RNA was determined using a Bioanalyzer 2100 (RNA 6000 Nano chip assay) and a Qubit 3.0 (Quant-It dsRNA BR Assay).
Total RNA was rRNA-depleted using the Ribo-Zero rRNA Removal Kit (Gram Negative Bacteria) (Illumina) and cDNA libraries were obtained using the TruSeq RNA kit following Illumina’s recommendations. Briefly, rRNA-depleted RNA was chemically fragmented prior to reverse transcription and cDNA generation. The cDNA fragments then went through an end repair process, the addition of a single “A” base to the 3′ end and then ligation of the adapters. Finally, the products were purified and enriched by PCR to create the indexed final double stranded cDNA library. The pool of libraries was sequenced on an Illumina HiSeq 2500 sequencer. The quality control of the raw reads was performed using FastQC2 program as previously described (Matanza and Osorio, 2018). The raw pair-end reads were mapped against the reference genome of the Pdd type strain CIP102761 (GenBank accession number NZ_ADBS00000000.1), using Bowtie2 (Langmead and Salzberg, 2012) v2.2.6 algorithm. Several quality control steps were performed. Reads displaying a very low quality were removed by using Samtools (Li et al., 2009) and Picard Tools software3. Furthermore, one of the key factors that can condition the sequencing process is the GC content of samples which was checked as normal (distribution between 40 and 60%) in our experiment. Likewise, distribution of duplicates was evaluated to confirm the normal small proportion. The process of genetic quantification was carried out by the HTSeq (Anders et al., 2015) software (0.6.1 version).
Concordance between samples of the same condition (replicates of each of the two assayed temperatures) was carried out by a study of correlation and distance considering the whole transcriptome normalized by the size of the library. This process was made using the statistics program R. Differential expression analysis was assessed using DESeq2 (Love et al., 2014) method (1.18.1 version). The analysis of Differentially Expressed Genes (DEG) was done by using statistical packages designed by Python and R, using the DESseq2 (Love et al., 2014) algorithm applying a differential negative binomial distribution for the statistics significance. Comparison between the two different conditions (37°C vs. 25°C) was set as fixed effect in DEseq2. A Python script developed at Sistemas Genómicos (Valencia, Spain) was employed to generate a data matrix for each group condition with the counts obtained from HTSeq count for each sample (each of the three replicates at each of the two temperatures). Genes with Fold Change (FC) value lower than -1.5 or higher than 1.5 and a P-value adjusted by False Discovery Rate (FDR) ≤ 0.05 were considered as differentially expressed. FPKM (Fragments per kilobase per million fragments mapped) values calculated with Cufflinks v2.2.1 (Langmead and Salzberg, 2012) were used to calculate the expression of each individual gene. FPKM is used for normalization of the data since it indicates the number of lectures of a given gene per kilobase (independently of the length of the gene), and per million reads (independently of the size of the library).
Construction of Insertional Mutants in Heat-Shock Related Genes
For insertional mutation of clpB, groEl, and htpG genes encoding heat-shock proteins, an internal fragment of each gene was PCR amplified and cloned into the suicide vector pNidKan (Mouriño et al., 2004). The mutant allele constructions were mobilized from Escherichia coli S17-1-λpir into the Pdd parental strain RM-71. Insertions of the suicide vectors into the chromosome by a single crossover would result in a Kmr phenotype and disruption of the coding sequence. The insertional mutants were selected on thiosulfate-citrate-bile-sucrose (TCBS) agar plates containing kanamycin (50 mg L–1), and the presence of the plasmid inserted into the target gene was confirmed by PCR. Mutants were grown in parallel with the parental strain RM-71 at 25 and 37°C to assess differences in growth, using the spectrophotometer Epoch2 microplate reader (Biotek).
Screening of a Mini-Tn10 Transposon Insertional Library
A mini-Tn10 transposon mutant library of approximately 2,000 clones was generated in Pdd parental strain RM-71 in a previous study (Terceti et al., 2017), and clones were always grown at a temperature of 25°C. The library, which consisted of clones maintained frozen at -80°C in 96-well plates, was used in the present study for the screening of putative mutant clones exhibiting impaired growth at 37°C. To this aim, each 96-well plate was carefully thawed, and clones were replicated into 96-well plates containing fresh TSB-1 medium. Two replicas per plate were incubated at 25 and 37°C respectively, and bacterial growth was monitored for 24 h using the spectrophotometer Epoch2 microplate reader (Biotek).
β-Galactosidase Assays
RM-71 strain derivatives carrying the dly, hlyApl, and hlyAch hemolysin gene promoters fused to a promotorless lacZ gene in reporter plasmid pHRP309 were grown in TSB-1 at 25 and 37°C, and β-galactosidase activities measured as previously described (Rivas et al., 2013). Three independent experiments with 3 replicates each were conducted. The statistical analysis of the expression data was carried out with the Student’s t-test (adjusted P < 0.05). Mann–Whitney test was used for non-parametric comparison of the mean values.
Results
Growth at 37°C Causes Impairment in Viability, Changes in Cell Morphology, Reduced Tolerance to Benzylpenicillin, and Modification of Membrane Lipid Composition
In order to cause disease in a human host, a marine bacterium has to be able to replicate at temperatures near 37°C. The ability to grow at temperatures > 30°C is indeed a differential phenotypic trait of P. damselae subsp. damselae in comparison to its sibling subspecies, the subsp. piscicida (Pdp). This difference is supposed to contribute to the ability of Pdd to colonize and establish an infection in homeothermic animals, whereas Pdp is pathogenic only for fish (Romalde, 2002).
To assess the effect of temperature in Pdd growth, the highly virulent strain RM-71, isolated from a disease outbreak in a turbot farm (Fouz et al., 1992), was cultured at 25 and 37°C for 48 h. Cultivation at 37°C triggered an earlier entry into exponential phase than at 25°C, but OD600 values dropped after 24 h suggesting a cell death scenario (Figure 1A). In order to test this hypothesis, viable cells were quantified by drop-counting on agar plates. As a result, there was a > 3.5-fold reduction in the number of colony-forming units (CFU) at 12 h incubation in cultures at 37°C in comparison to 25°C. Notably, CFU were no longer detected from cultures at 37°C after 30 h (Figures 1B,C). The observation of cell viability reduction at 37°C prompted us to study cell morphology by scanning electron microscopy, in exponentially-growing (OD600 of 0.55) cultures at 25 and 37°C. Remarkably, cells grown at 25°C showed normal rod morphology by scanning electron microscopy analysis whereas cells grown at 37°C exhibited chain-like structures and aberrant long shapes suggesting a defect in daughter cell separation and in septum formation (Figure 2A). Quantitatively, mean cell length values at 37°C were significantly higher than at 25°C (Figure 2B). In addition, growth at 37°C caused a reduction in the intrinsic tolerance of Pdd RM-71 to benzylpenicillin in comparison to 25°C (Figure 2C).
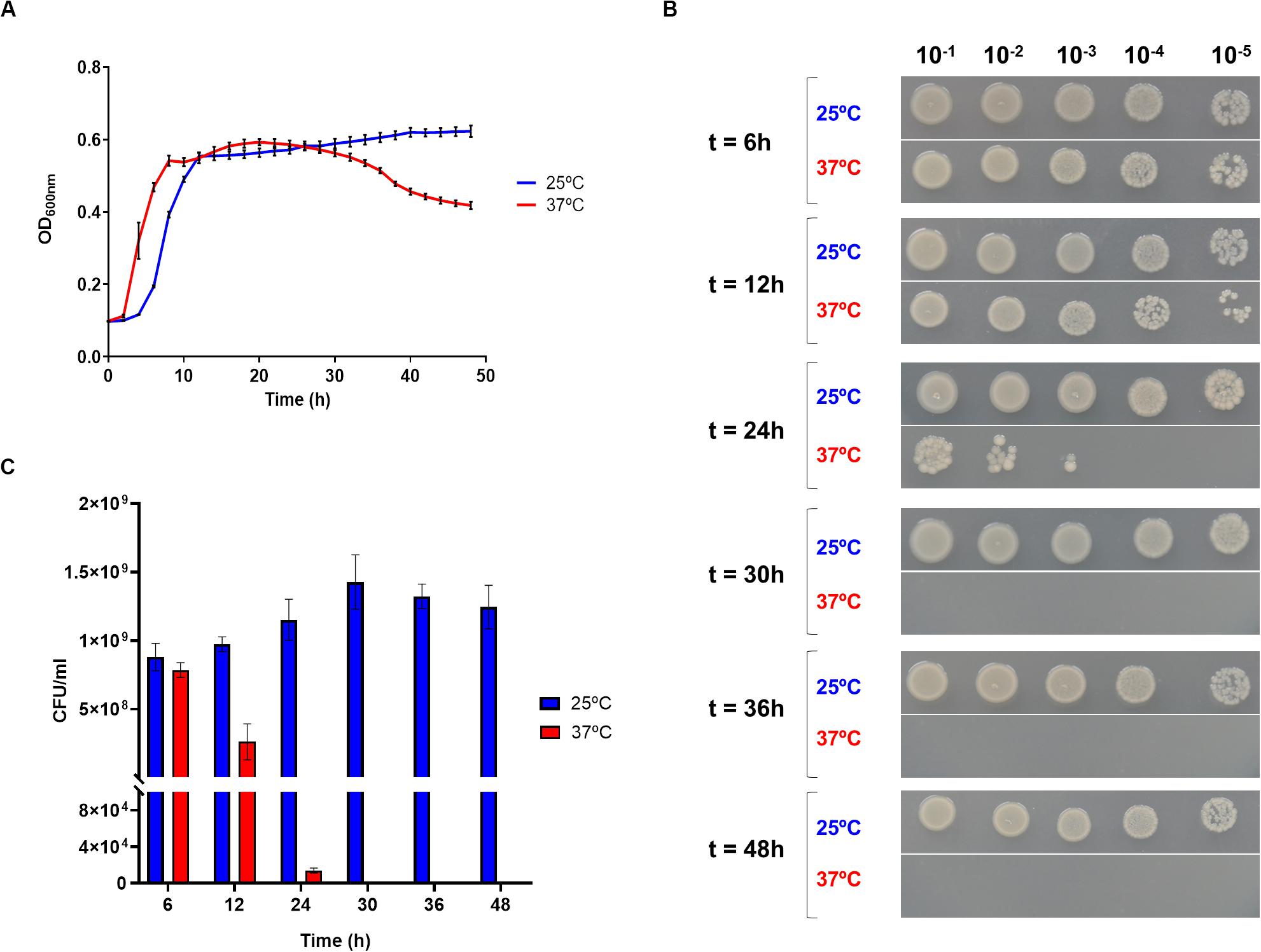
Figure 1. Growth at 37°C impairs cell survival in Pdd. (A) Growth curves of Pdd RM-71 at 25 and 37°C in TSB-1, showing the brief stationary phase and the start of the death phase at 37°C, contrasting with the prolonged stationary phase at 25°C. Mean data of three independent experiments are shown. (B) Growth at 37°C causes a drastic reduction in CFU numbers as detected by drop-plate assays. Serial decimal dilutions of cultures at 25 and 37°C were prepared from samples taken at 6, 12, 24, 30, 36, and 48 h incubation, and 5 μl aliquots were applied as drops onto TSA-1 plates and incubated at 25°C for 24 h. (C) Quantitative representation of the drop-plate assay data. Viable counts are zero after 30 h cultivation at 37°C whereas at 25°C the number of CFUs is still increasing.
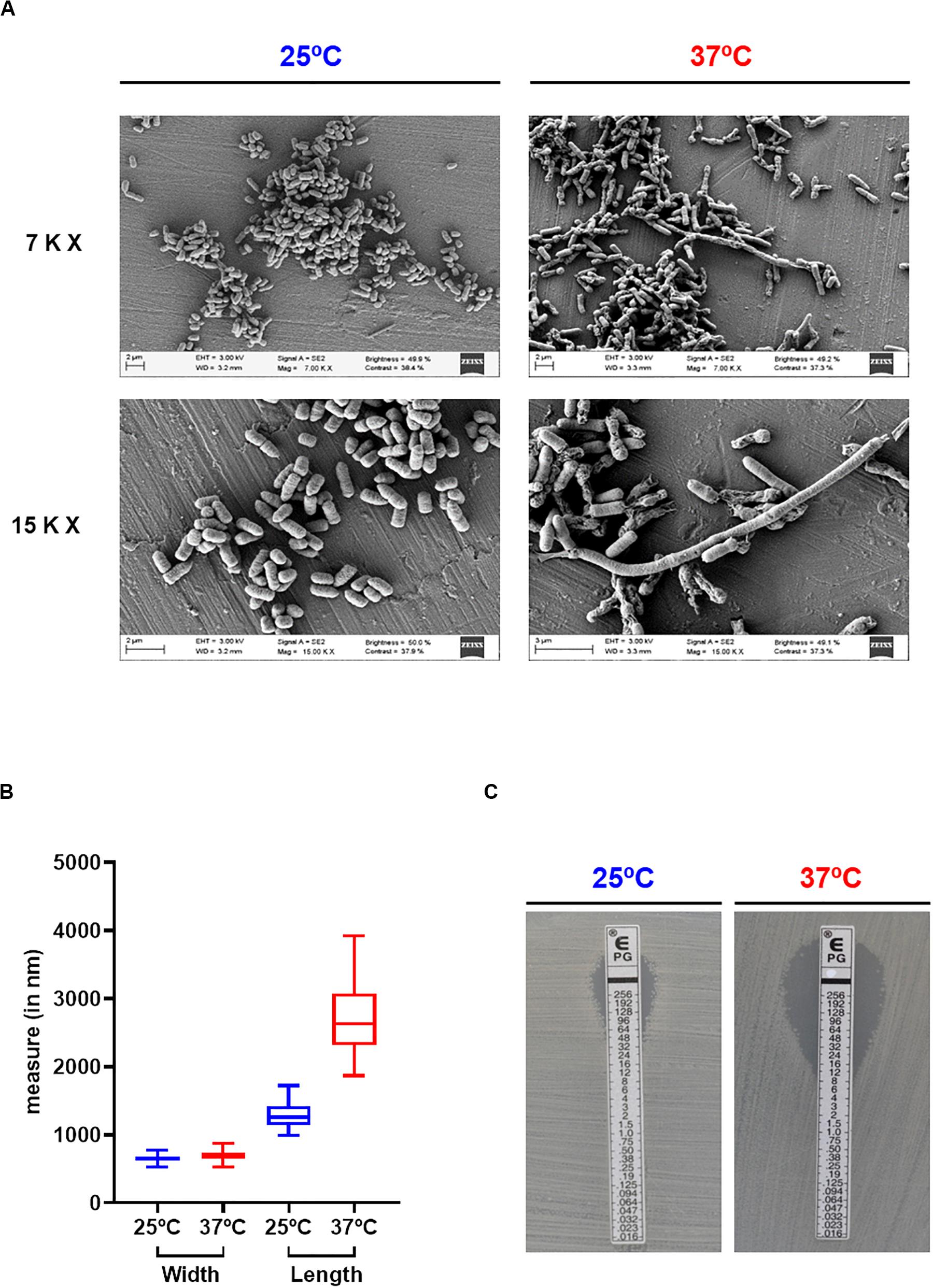
Figure 2. Growth at 37°C impairs cell morphology and benzylpenicillin tolerance in Pdd. (A) Scanning electron microscopy images showing the increase in cell length and the impairment in daughter cell separation in Pdd cells grown at 37°C with respect to the normal cell size at 25°C. Pictures from two magnifications, 7,000× (7 K X) and 15,000× (15 K X) are shown. (B) Box plot graphs showing cell width and cell length values at 25 and at 37°C. Whiskers indicate min and max values. Data correspond to the measurements of 50 independent cells per temperature assayed. (C) E-tests for benzylpenicillin of Pdd RM-71 cultivated at 25 and 37°C, showing the decreased tolerance to this antimicrobial compound at 37°C.
Bacteria can adapt to variations in environmental temperature by altering the ratio between saturated (SFA) and unsaturated (UFA) fatty acids in membranes (Marr and Ingraham, 1962). However, the effects of temperature in membrane lipids composition in Pdd have not been studied so far. We here carried out an analysis of fatty acid methyl esters (FAME) from Pdd RM-71 cultures at 25 and 37°C. We observed that the percentage of saturated fatty acids in membranes increased at 37°C with respect to 25°C, and the three saturated fatty acids 16:0 N alcohol, 16:0 iso and 19:0 cyclo w8c were exclusively detected at 37°C (Supplementary Table S1).
Human Isolates Are Not Better Adapted for Growth at 37°C Than Fish Isolates, and Comparative Genomics Does Not Reveal Human-Specific Gene Markers
We selected two strains from human clinical cases (CDC 2227-81 and 80077637), and three strains from diseased fish (RM-71, LD-07, and A-162), and growth of all the isolates was monitored at 25 and 37°C. The ability to grow at 37°C was not a differential trait of human isolates since all the strains showed similar dynamics: early exponential growth and a drop in the OD600 after approximately 24 h (Figures 3A,B). Of note, human strain 80077637 achieved the least OD600 values at the two temperatures. These data, together with the above described survival assays, suggest that 37°C, rather than being a normal condition, represents a stress condition for Pdd.
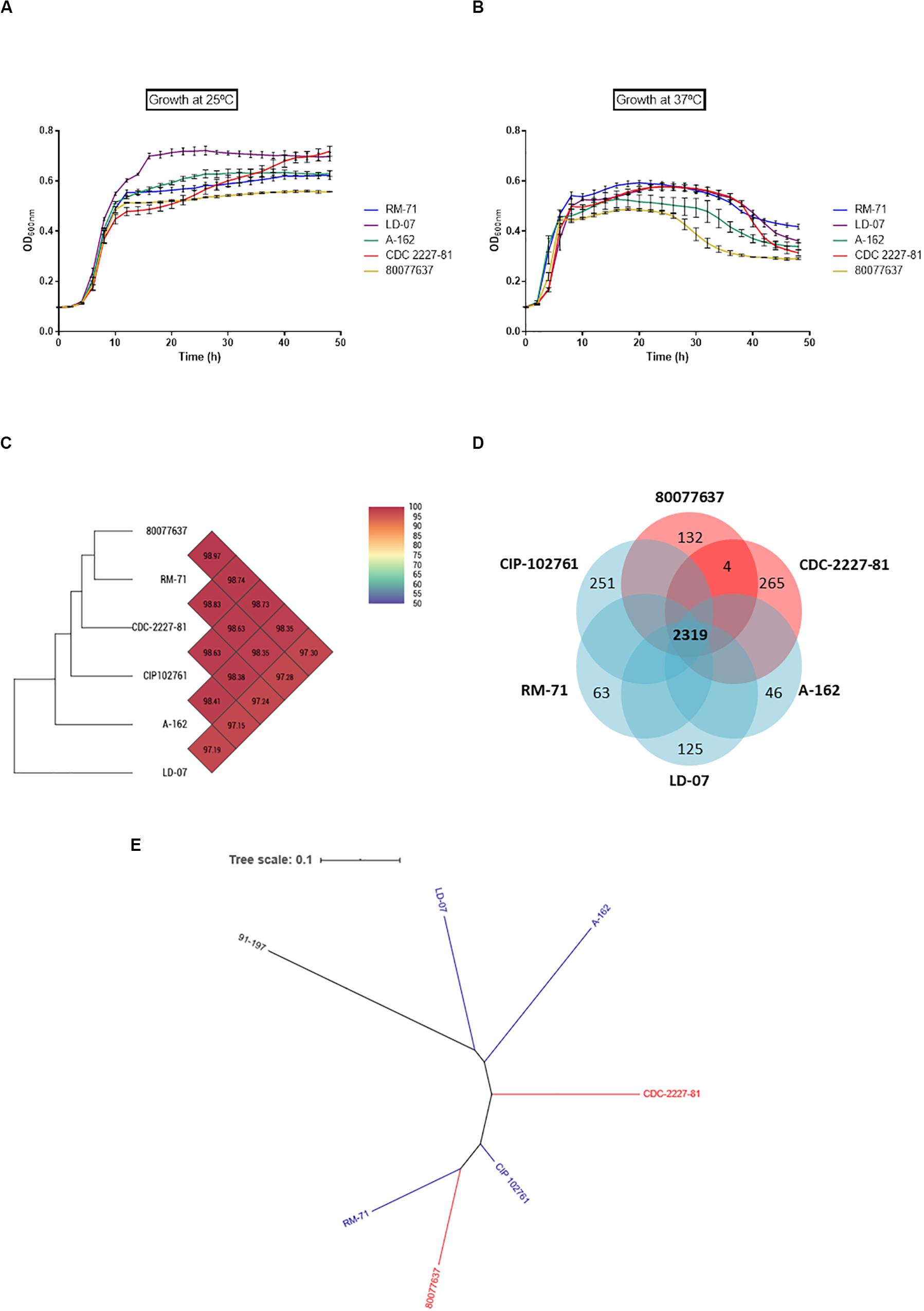
Figure 3. Pdd isolates from human origin do not exhibit an advantage for growth at 37°C compared to fish isolates, and do not contain specific gene markers unique to human isolates. Growth curves of Pdd strains from human (CDC 2227-81; 80077637) and fish (RM-71; LD-07; A-162) origin, cultivated at 25°C (A) and at 37°C (B). Note that human clinical strain 80077637 achieves the least OD600 values of all strains at the two temperatures. (C) Heatmap generated by comparison of core genes with OrthoANI values calculated from the OAT software shows the absence of close phylogenetic association of human isolates vs. fish isolates. (D) Venn diagram depicting the comparative genomics of six Pdd strains. The core genome was estimated in 2319 genes, and each isolate has a varying number of strain-unique genes, ranging from 46 unique genes to strain A-162 to the 265 unique genes to strain CDC-2227-81. Of note, the two human-isolated strains only share 4 specific genes which are absent from the fish strains. (E) Dendrogram of Pdd strains based on genomic BLAST, showing that human clinical strains do not form an independent clade with respect to fish isolates. The genome of the sibling subspecies P. damselae subsp. piscicida strain 91–197 was included for comparative purposes. The genomic BLAST file was downloaded from the NCBI database and the tree was visualized by Interactive Tree Of Life (iTOL v5). The genome of the Pdd type strain CIP 102761 was included in the genomic analyses depicted in (C–E).
To assess the existence of gene markers characteristic of human isolates, we here obtained for the first time the draft genome sequences of two Pdd strains isolated from humans, CDC-2227-81 (Kreger, 1984) and 80077637 (Hundenborn et al., 2013), and a comparative genomics analysis was conducted including the genomes of four fish isolated strains, RM-71, LD-07, A-162, (Vences et al., 2017) and CIP102761. Ortho Average nucleotide identity (OrthoANI) values, which are derived from the pairwise comparison of strains taking into consideration the core genes shared by all the strains, ranged from 97.19 to 98.97 between strains (Figure 3C). Two genomes are considered the same species when the ANI value is higher than 95–96% (Lee et al., 2016). The 4 strains harboring the virulence plasmid pPHDD1 clustered together independently of their source of isolation, and human isolates were not more similar between them than to the other genomes, when core genes where compared. In fact, the highest similarity value was found between RM-71 (fish isolate) and 80077637 (human isolate). Notably, genomics analysis unveiled a large number of strain-specific genes, with 265 genes unique to strain CDC-2227-81 and 132 genes unique to strain 80077637 (Figure 3D). Four genes were common to the human isolates and absent in the fish isolates. These genes encoded a racemase (locus F6450_06750), an HD domain-containing protein (F6450_11305), a MFS transporter (F6450_16290), and a multidrug transporter (F6450_07685) (GenBank loci tags correspond to CDC 2227-81). However, BLAST searches in GenBank database unveiled that three of these genes were also present in other Pdd strains isolated from fish, and the multidrug transporter F6450_07685 was found in a Pdd isolate from the porpoise Neophocaena asiaeorientalis and in non-pathogenic Photobacterium species (data not shown). A phylogenetic tree constructed based on the genome alignments (including core and accessory genome) of Pdd strains revealed that human-isolated strains do not represent an independent evolutionary line with respect to the fish isolates, and human clinical strain 80077637 fell into the same clade with fish-isolated RM-71 (Figure 3E).
RNA Sequencing Reveals Major Changes in the Pdd Transcriptome in Response to Growth at 37°C
The RNAseq analysis of RM-71 resulted in 1607 differentially expressed genes (DEGs): 804 upregulated (Foldchange (FC) higher than 1.5) and 803 downregulated (FC lower than −1.5) at 37°C with respect to 25°C (Supplementary Table S2). The principal component analysis (PCA) showed that samples grown at each different temperature were distinguishable and replicates clustered together (Figure 4A). A heat map with gene expression plotted as z-score normalized FPKM (Fragments Per Kilobase of transcript per Million mapped reads) value for differentially expressed genes (DEGs) was performed. Hierarchical clustering showed the different expression profiles of RM-71 at the two temperatures and the grouping of the biological replicates (Figure 4B).
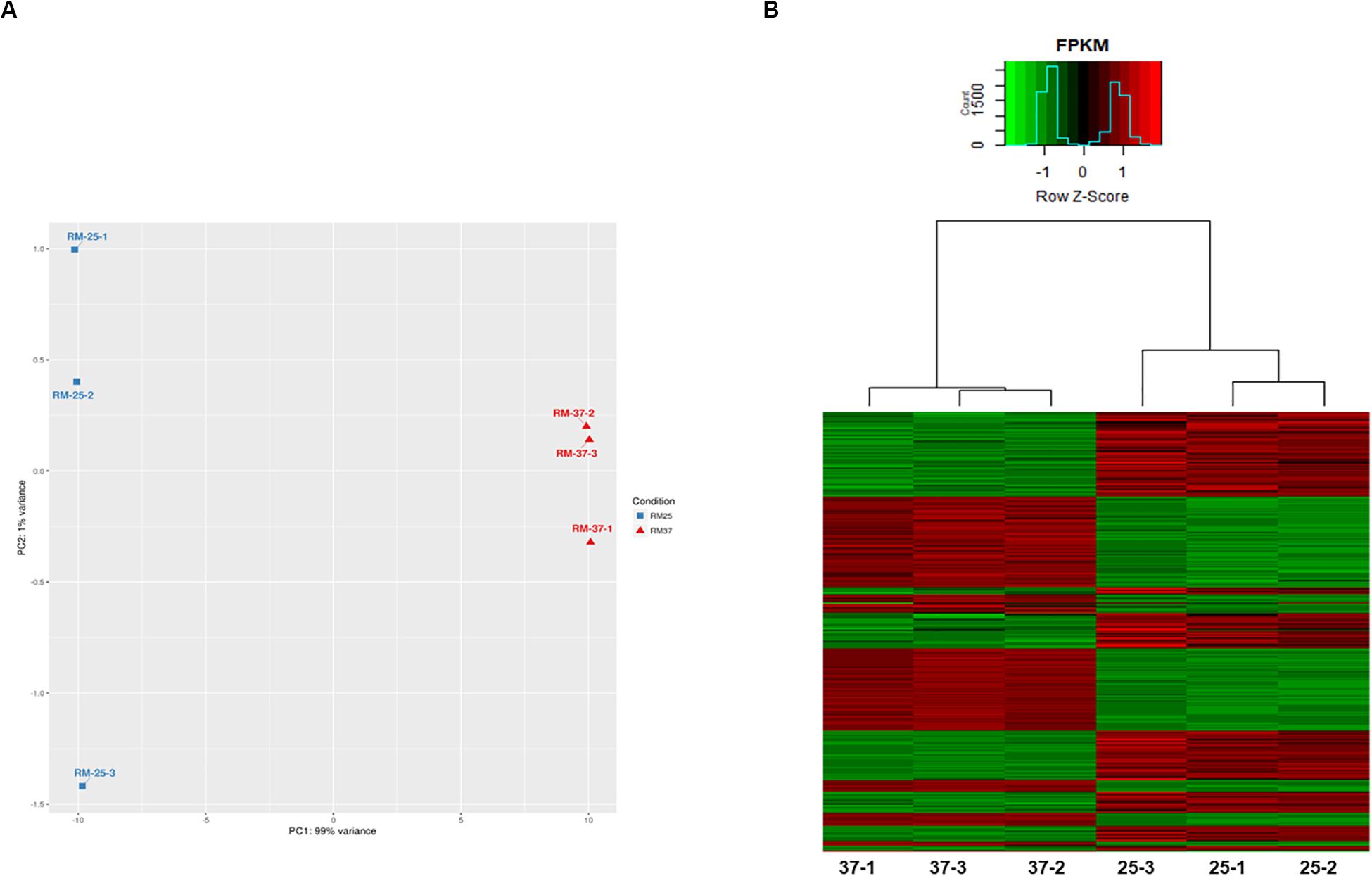
Figure 4. RNA sequencing reveals changes in the Pdd transcriptome in response to growth at 37°C vs. 25°C. (A) Hierarchical clustering of all genes by Principal Component Analysis (PCA) demonstrates clear separation of the 2 sample groups (25°C vs. 37°C). (B) Heat map with gene expression plotted as z-score normalized FPKM (Fragments Per Kilobase of transcript per Million mapped reads) value for differentially expressed genes (DEGs). The hierarchical clustering shows the different expression profiles of RM-71 at the two temperatures and provides strong evidence of the grouping of the biological replicates.
Growth at 37°C Triggers a Strong Stress Response: Heat Shock Proteins and Defense-Related Mechanisms Are Upregulated at 37°C
To better illustrate the impact of human body temperature on gene expression, a heat map was generated with 32 selected DEGs that showed remarkable FC values (Figure 5). Notably, heat-shock proteins and molecular chaperones were among the most upregulated genes at 37°C (Table 1 and Figure 5). The genes encoding ClpB, GroEl and HtpG heat-shock proteins were selected for construction of insertional mutants, using the suicide plasmid pNidKan (Mouriño et al., 2004) containing an internal fragment of the target gene. A htpG insertional mutant was successfully generated although it did not show any impairment in growth at 37°C compared to 25°C (data not shown). Notably, we were unable to obtain insertional mutants for clpB and groEL despite numerous attempts, suggesting that these genes are essential, even for growth at 25°C (the temperature at which strains were incubated during the mutant construction process). We thus aimed to gain a further insight into the existence of gene markers essential for growth at 37°C but dispensable at 25°C. Identification of such markers would be of interest for the design of control strategies of human infections caused by Pdd. For this, we screened a > 2000-clone Tn10 transposon mutant library of Pdd RM-71 constructed in a previous study (Terceti et al., 2017), and each clone was grown in TSB-1 in 96-well plates per duplicate at 25 and at 37°C. However, all the mutant clones were able to grow at 37°C without any evident signs of impairment (data not shown), which suggests that any potential transposon-insertional mutation that would prevent growth at 37°C, would also be deleterious for growth at 25°C (the temperature at which the transposon library was originally obtained).
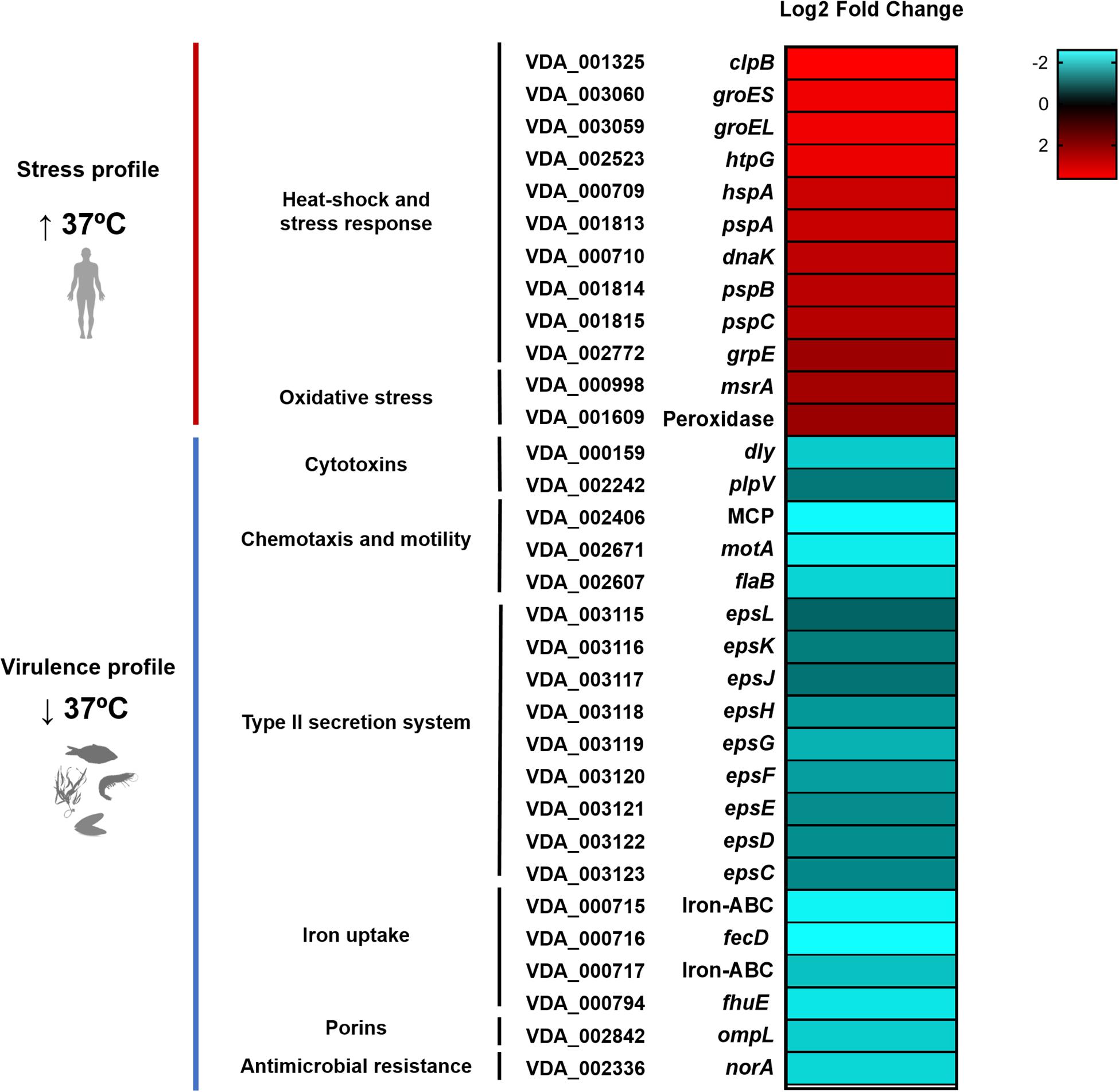
Figure 5. Heatmap of 32 selected DEGs of the Pdd transcriptome, including the main upregulated genes at 37°C in the categories of heat-shock and stress response mechanisms, and the main downregulated genes related to virulence properties. Cultivation of Pdd at 37°C triggers a strong stress response, indicating that human body temperature represents a stressful condition for this marine pathogen. Conversely, growth at 37°C downregulates the virulence gene expression profile with respect to 25°C, corroborating that 25°C is the optimal temperature for the pathogenic lifestyle of Pdd in the marine environment. VDA gene tags correspond to the complete genome sequence of the Pdd type strain CIP 102761.
Genes involved in prevention of oxidative damage were found among the most up-regulated at 37°C (Table 1 and Figure 5), including the peptide methionine sulfoxide reductase MsrA, alkyl hydroperoxide reductase subunit C-like protein and the thioredoxin peroxidase, among others. These enzymes are produced by bacteria as defensive strategies against host defenses. Together with the viability assays, this association between high temperatures and upregulation of stress and defense mechanisms supports the idea that 37°C constitutes a stressful condition for Pdd.
Metabolic Pathways, Transporters, ATP Generation, and Nutrient Acquisition Are Upregulated at 37°C
Cultivation at 37°C causes a rapid initial growth of Pdd as shown above. In the RNA seq analysis, the expression of genes encoding enzymes of catabolic and anabolic pathways were strongly up-regulated at 37°C (Table 1), being the glycolytic enzyme NAD-dependent glyceraldehyde-3-phosphate dehydrogenase one of the most strongly upregulated genes at 37°C. Leucine, isoleucine, threonine and thiamine biosynthetic genes, enzymes of the phosphate pentose pathway and the purine/pyrimidine metabolism were upregulated at 37°C, likely to supply precursors for proteins, nucleotides and coenzymes to sustain the rapid initial growth of Pdd observed at 37°C. Accordingly, genes encoding the ATP-synthase subunits were upregulated. A number of pH-dependent and independent transporters were among the most upregulated genes at 37°C, including a Na+/H+ antiporter of NhaA-type. In V. cholerae this gene contributes to resistance to Na+ at alkaline pH leading to improved fitness in the environment (Herz et al., 2003). Also upregulated were several sulfate transporters, and the potassium-efflux system protein KefB. Of relevance, kefB belongs to the locus of heat resistance in E. coli (Mercer et al., 2017) and it has been studied for its ability to acidify intracellular pH in response to electrophilic stress (Ferguson et al., 1995).
Pdd is one of the few urease-producer species within the Vibrionaceae family, and genes encoding urease subunits were upregulated at human body temperature. Urease is a well-studied enzyme in gastric pathogens for its role in maintaining periplasmic pH near neutrality in highly acidic environments (Sachs et al., 2003) and it also constitutes a key virulence factor of numerous urinary pathogens (Nielubowicz and Mobley, 2010; de Paiva-Santos et al., 2018). To date no functional studies of ure genes have been carried out in Pdd. This up-regulation of ure genes might play a role in some Pdd infections as this pathogen has been isolated as causative agent of a urinary tract infection in a pregnant woman in warm coastal areas of the Caribbean Sea (Alvarez et al., 2006).
Genes encoding degradative functions were upregulated, including several putative lipases (VDA_000412 and VDA_002140) and an operon for utilization and transport of galactosamine and glycosaminoglycans (VDA_001072-VDA_001083). Glycosaminoglycans are major components in extracellular matrix of animals (Gandhi and Mancera, 2008). Pdd is considered a generalist pathogen of a wide range of animals (Osorio et al., 2018) so genetic networks involved in the utilization of different carbon sources allows colonization of different niches and hosts. At 37°C, Pdd also upregulated genes of two pili structures encoded within the virulence plasmid pPHDD1, including the tra genes for conjugative pilus biogenesis, and the genes of the Flp/Tad pilus, with a potential role in adhesion/colonization.
A Virulence Plasmid-Encoded H-NS Repressor Is Upregulated at 37°C
How Pdd modulates genetic expression in response to external conditions remains unknown. Several LysR family transcriptional regulators were upregulated at 37°C (VDA_001014, VDA_001042, VDA_000547, and VDA_001035). Of special relevance is the observation that the DNA-binding protein H-NS (VDA_000143), encoded within the virulence plasmid pPHDD1 showed a > fivefold upregulation at 37°C. In Vibrio cholerae, this protein functions as a virulence repressor controlling motility and biofilm formation (Wang et al., 2015). As detailed below, growth at 37°C was found to downregulate virulence and motility functions in Pdd.
Growth at 37°C Causes Downregulation of Virulence Factors
In a previous work, we demonstrated that many Pdd virulence factors were up-regulated at 25°C in comparison with 15°C, thus contributing to partially explain the onset of disease outbreaks in marine fish farms when seawater temperature is close to 25°C (Matanza and Osorio, 2018). We here investigated how human body temperature affects virulence gene expression in Pdd. Of note, well-known virulence factors of Pdd were downregulated at 37°C, suggesting that infection of a human host is not a condition that has been favored during evolution of this marine pathogen (Table 2 and Figure 5). Damselysin (Dly) toxin, a major virulence factor of Pdd, showed a fourfold down-regulation at 37°C. The two pore-forming toxins PhlyP and PhlyC did not show a differential expression at 37°C, and the phospholipase PlpV showed downregulation at 37°C. β-galactosidase assays of transcriptional fusions of the hemolysin gene promoters to lacZ gene validated the RNA-seq results (Figure 6). Despite the absence of upregulation at 37°C, the three major cytotoxins Dly, PhlyP, and PhlyC exhibited high FPKM values (Supplementary Table S3), in accordance with previous studies that demonstrated that the three cytotoxin genes are highly expressed and the toxins represent a major fraction of the Pdd secretome (Matanza and Osorio, 2018; Terceti et al., 2019). Genes of the type II secretion system (eps genes, VDA_003115-VDA_003123) that play a major role in the secretion of the cytotoxins and therefore in virulence (Rivas et al., 2015a; Vences et al., 2017), were downregulated at 37°C (Figure 5). The two-component RstAB system, a major positive regulator of virulence in Pdd (Terceti et al., 2017, 2019), was not significantly affected by temperature.
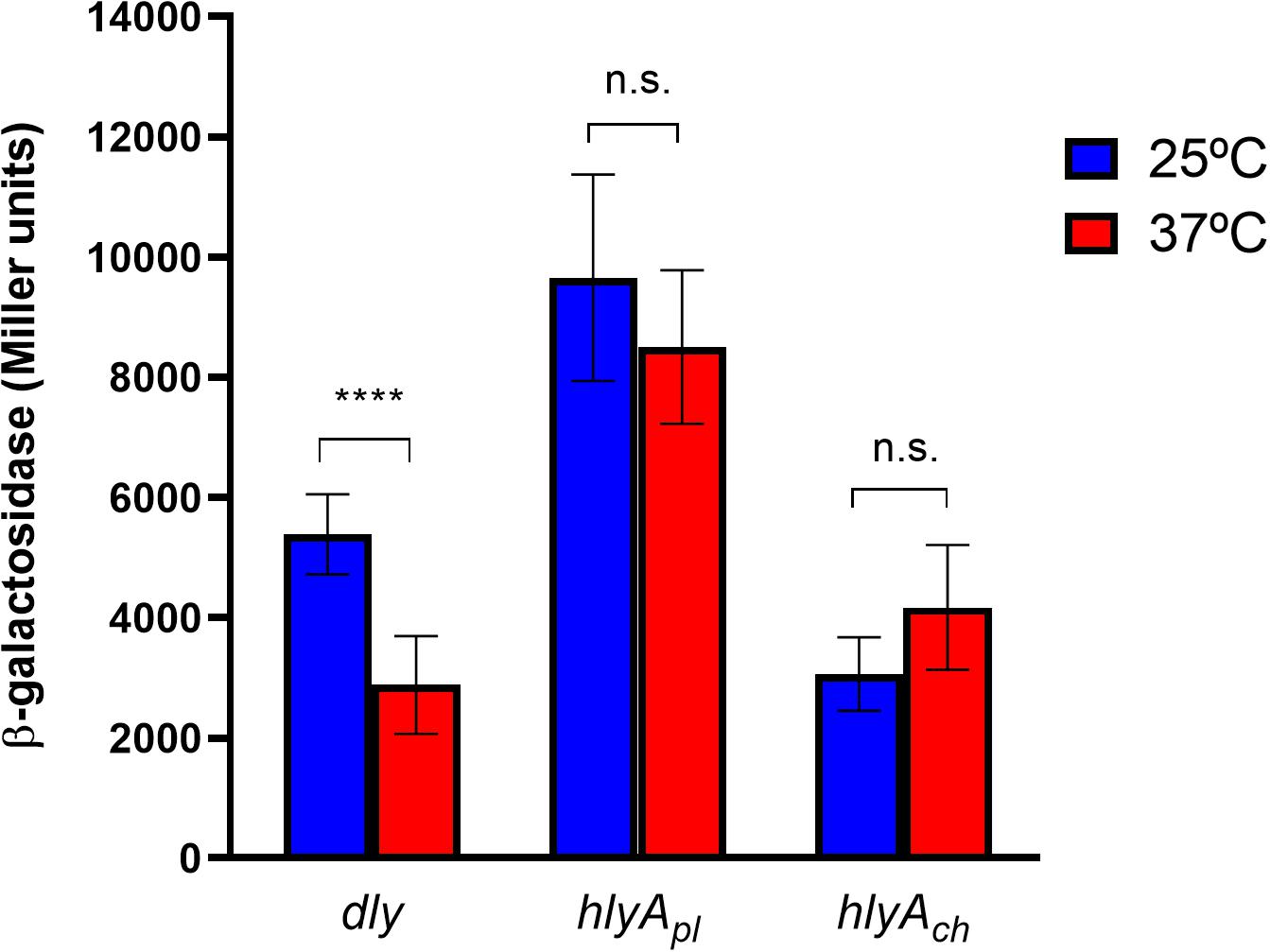
Figure 6. Transcription activities of the three major cytotoxin genes of Pdd, damselysin (dly gene), phobalysin P (hlyApl gene), and phobalysin C (hlyAch gene) under the temperature conditions of a human host (37°C) in comparison to the temperature in warm seawaters (25°C), as determined by transcriptional fusions of dly, hlyApl, and hlyAch promoters to lacZ reporter gene. The β-galactosidase values (expressed in Miller units) show that dly is significantly downregulated at 37°C whereas expression of hlyApl and hlyAch is not significantly affected at 37°C vs. 25°C, thus validating the results of the transcriptomics analysis. ****denotes the degree of significance in the Mann-Whitney test (p value was <0.0001).
Other virulence-related functions were downregulated at 37°C (Table 2 and Figure 5): an iron ABC-transporter (VDA_00715-VDA_000717), a TonB-dependent siderophore receptor (VDA_000794), and the potABCD operon (VDA_002114-VDA_002117, VDA_000341) encoding a polyamine (spermidine and putrescine) transport system. Polyamines are essential for normal growth and proliferation in bacteria (Shah and Swiatlo, 2008). In Vibrio vulnificus expression of pot genes is up-regulated in presence of human serum (Williams et al., 2014) and in V. cholerae they regulate biofilm formation (McGinnis et al., 2009).
Porins are outer membrane proteins that modulate pathogenicity of many Vibrio species. VDA_002842 is downregulated at 37°C and shows similarity to Vibrionaceae porins, including OmpU. OmpU has been pointed for its role in adhesion, host recognition and pathogenesis in several Vibrio species (Goo et al., 2006; Duperthuy et al., 2011; Sakharwade and Mukhopadhaya, 2015). Multidrug efflux protein VDA_002336 showed a down-regulation at 37°C. It belongs to the multidrug and toxic-compound extrusion (MATE) proteins group, involved in protecting cells against antibiotics and other substances in human pathogenic Vibrios as Vibrio parahaemolyticus and Vibrio cholerae (Begum et al., 2005; Otsuka et al., 2005).
Motility and adhesion to hosts are considered of special relevance in Pdd pathogenicity (Fouz et al., 2000; Rivas et al., 2015b). A recent study demonstrated that disruption of chemotaxis regulator cheA reduced motility and production of PhlyP, and subsequently impaired bacterial adhesion to human cell lines (von Hoven et al., 2018). The current study revealed a large set of genes with a role in chemotaxis and flagellar motility which were downregulated at 37°C (Table 2 and Figure 5). All these data indicate that exposure of Pdd at human body temperature triggers a transcriptomic pattern of defense against stress rather than a high virulence profile.
Genes Encoding Cold Shock Response, Membrane Transporters, Metabolic Functions, and Adjustment of Membrane Composition Are Downregulated at 37°C
There was a downregulation of cold shock functions at 37°C (Table 2), including two proteins that might play a role in cold tolerance in Pdd: VDA_002460 and VDA_002316. Phosphate, nucleoside and vitamin transporters, and permeases for inorganic ions were downregulated at 37°C (Table 2). DNA can be utilized as a source of phosphate, carbon and nitrogen. Phosphate limitation is encountered by marine pathogens in either the environment or inside the host. We have found that a phosphate transport regulator (VDA_002943) and a low-affinity inorganic phosphate transporter (VDA_002944), which shares homology with E. coli pitA (identity 33%), are down-regulated at 37°C. As well, nucleoside permeases NupC (VDA_001789 and VDA_002313) were also down-regulated at 37°C. A previous study (Matanza and Osorio, 2018) showed that NupC VDA_001789 presented a high up-regulation at 25°C compared to 15°C. These results suggest that Pdd takes advantage of the utilization of DNA as a source of biomolecules especially at 25°C (temperature at which outbreaks in fish farms are favored). An operon of type ECF (energy coupling factor) ABC transporters (VDA_002275-VDA_002277) was down-regulated at 37°C, as well as the putative vitamin transporter VDA_003148. The myo-inositol-1 (or 4) – monophosphatase (VDA_002716), which participates in myo-inositol biosynthesis, showed a remarkable down-regulation at 37°C. This function has been associated with a role in correct rRNA biosynthesis (Singh et al., 2016).
During anaerobic respiration, bacteria use alternative electron acceptors such as trimethylamine N-oxide (TMAO) to oxidize organic compounds. The cytochrome c-type protein TorC (VDA_001717) that takes part in this electronic transfer (Gon et al., 2001) shows a down-regulation at 37°C. The association between anaerobic growth and temperature in Pdd has not been explored to date. Functions related to nucleotide and pyrimidine biosynthesis, and of carbohydrate metabolism were also downregulated. Functions for the maintenance of lipid membranes, phospholipid biosynthesis, fatty acid biosynthesis and lipid IVA biosynthesis were markedly downregulated at 37°C. These observations might correlate with the observed changes in membrane lipid composition in Pdd cultured at 37°C vs. 25°C as reported above (Supplementary Table S1).
Discussion
Different to other human pathogenic Vibrios (as V. cholerae and V. parahaemolyticus) that cause diarrhea in humans and therefore gain exit from the host, infection of a human by Pdd can be seen as an end-point for the bacterium. Most clinical cases evolve as wound infections that may or may not complicate into fatal necrotizing fasciitis, and there is no evident exit strategy for the successful Pdd genotypes to go back to the marine environment. The lines of evidence presented in this study clearly suggest that the ability of Pdd to infect humans is more an anecdotic circumstance than an evolutionarily selected trait. Pdd cells growing at human body temperature undergo stressful conditions that impair viability, control of cell shape, and size. This evidence is in agreement with earlier studies that reported the difficulties to isolate Pdd from bullae fluid and blood in a human clinical case, whereas streaking the specimens on blood agar plates caused hemolysis in absence of growth (Clarridge and Zighelboim-Daum, 1985). In a rapidly fatal infection case, wound specimens yielded Pdd in pure culture but blood cultures were negative, and it was suggested that a systemic toxin released by Pdd contributed to the rapid fatal outcome and that septicemia did not (Fraser et al., 1997). Other studies were unable to detect Pdd cells upon Gram staining of specimens from tissues in infected individuals (Coffey et al., 1986; Goodell et al., 2004). All these observations can be explained, on the one hand, by the low viability of Pdd at 37°C as demonstrated in the present study and, on the other hand, by the high amounts of hemolysins produced, that would accumulate in host fluids during the initial phases of infection. Accumulated hemolysins might cause further tissue damage in absence of bacterial replication. Recent studies have demonstrated that under laboratory growth conditions at 25°C, the hemolysin genes are among the most highly expressed genes in Pdd (Matanza and Osorio, 2018; Terceti et al., 2019), and the FPKM values obtained in the present study (Supplementary Table S3) also confirmed that the three major hemolysins, Dly, PhlyP, and PhlyC exhibit high transcription levels at 37°C. Our findings on affected cell morphology and size in Pdd upon growth at 37°C are in agreement with previous observations of bacilli with large dimensions in Gram-stained sections from Pdd-infected tissues (Coffey et al., 1986) and with aberrant cell morphologies (Clarridge and Zighelboim-Daum, 1985; Goodell et al., 2004).
Similar to other species of the genera Vibrio and Photobacterium, Pdd exhibits high levels of intrinsic tolerance to bactericidal inhibitors of cell wall biosynthesis, as benzylpenicillin. Recent studies reported that Pdd RM-71 regulatory mutants in the RstAB two-component system exhibited aberrant cell morphologies, increased cell size and length, and reduced tolerance to benzylpenicillin (Terceti et al., 2019). In the present study, growth at 37°C was also found to cause increased sensitivity to benzylpenicillin with respect to 25°C. Thus, conditions that impair cell size and shape also impair penicillin tolerance in Pdd. A recent study reported that tolerance to beta lactams in Vibrio cholerae has a pleiotropic nature, and genes involved in cell envelope biogenesis and modification also play a major role in tolerance to beta lactams (Weaver et al., 2018).
A conserved strategy used by bacteria to face variations in environmental temperature is the modulation of membrane fluidity by adjustment of lipid composition (Sinensky, 1974; Rock and Cronan, 1996; Mansilla et al., 2004). The predominant strategy consists of altering the ratio between saturated (SFA) and unsaturated (UFA) fatty acids in membranes (Marr and Ingraham, 1962; Russell, 1983; Zhang and Rock, 2008). The analysis of FAME in Pdd RM-71 at 25 and 37°C in the present study has demonstrated that the percentage of saturated fatty acids in membranes increases at 37°C with respect to 25°C, and three saturated fatty acids are exclusively detected at 37°C. It is conceivable that the ability of this marine pathogen to inhabit different environments may rely on its skills to reversibly modify its membranes, assuring the survival outside and inside the diversity of hosts it can successfully colonize.
On the light of the current study, it is not possible to propose gene markers specific for human-adapted strains vs. fish-adapted strains in Pdd. The pPHDD1 virulence plasmid shared by the two human isolates analyzed here, also occurs in fish isolates (Rivas et al., 2011). A close look at the hemolytic activities of human isolates reported in an early study (Kreger, 1984) suggests that some human isolates fall within the category of low hemolytic activity (isolates that lack pPHDD1). These lines of evidence suggest that any Pdd genotype thriving in the marine environment might potentially cause an opportunistic infection in humans.
Recent transcriptomic studies have revealed the connection between temperature and virulence in marine pathogenic bacteria (Williams et al., 2014; Kong et al., 2017; Huang et al., 2018). In the current work, we demonstrate that Pdd undergoes a stress response at 37°C, with heat-shock proteins accounting for the most upregulated functions at this temperature. On the contrary, Vibrio species pathogenic for humans and marine animals as Vibrio parahaemolyticus, V. vulnificus and V. cholerae are routinely cultured at 37°C because such temperature does not represent a limiting factor. Indeed, V. parahaemolyticus shows the highest percentage of genes with stable expression at 37°C (Urmersbach et al., 2015).
Growth at 37°C caused the downregulation of most of the virulence-related functions known in Pdd. This not only pertains to the Pdd cytotoxins, but also to iron acquisition systems, motility and chemotaxis. Previous studies have reported the importance of iron acquisition in Pdd virulence for fish and mammals (Fouz et al., 1994, 1997). In the present study, it was demonstrated that growth at 37°C caused downregulation of iron ABC transporters and siderophore receptors. Downregulated genes included VDA_000794 encoding a TonB-dependent siderophore receptor. Of note, this gene was found to be induced when Pdd is cultured under iron-limitation conditions (Puentes et al., 2017).
A previous study that compared the Pdd transcriptomes at 15 and 25°C, revealed that warm seawater temperatures (25°C) upregulated genes for chemotaxis, flagellar motility and virulence with respect to 15°C (Matanza and Osorio, 2018). This clearly suggests that the optimal temperature for Pdd to trigger a virulence expression profile is close to 25°C. Proteins for prevention of oxidative damage, found among the most up-regulated genes at 37°C, are produced by bacteria as defensive strategies against host defenses, and constitute interesting targets for the design of antimicrobial compounds (Ezraty et al., 2017). In this line, another gene upregulated at 37°C was an outer membrane protein (VDA_000104) whose homologs in V. cholerae (VC1835) and V. parahaemolyticus (VP1390) are related to virulence and antimicrobial activities (Bina et al., 2003; Ronholm et al., 2016). Some of the most DEGs genes unveiled in this study still await further investigation. It is the case of VDA_000412, a protein of the patatin-like phospholipase superfamily that exhibits a 10-fold upregulation at 37°C. The patatin-like ExoU plays an important role in pathogenesis in Pseudomonas aeruginosa (Sato and Frank, 2014). Overall, the transcriptomics data reported here are expected to contribute to the investigation of novel approaches for prevention and for less invasive control of human infections caused by Pdd. On balance, these results integrate transcriptomics, genomics and phenotypic data providing strong evidence that human infections caused by Pdd are opportunistic rather than originated by specific clones adapted to the human host. Transcriptomics analysis reveals that, under human host temperature conditions, Pdd triggers a defensive strategy by upregulating heat-shock proteins and defense mechanisms, while downregulating a virulence expression profile.
Data Availability Statement
The datasets generated for this study can be found in the GenBank: VZUQ00000000 and WAEO00000000.
Author Contributions
XM and CO conceived the study, designed the experiments, analyzed the data, and wrote the manuscript. XM performed the experiments. All authors contributed to the article and approved the submitted version.
Funding
This work was supported by the Agencia Estatal de Investigación (AEI) of Spain co-funded by the FEDER Programme from the European Union (grant no. AGL2016-79738-R) and by Xunta de Galicia (Spain) (grant no. ED431C 2018/18). XM thanks Xunta de Galicia for a predoctoral fellowship.
Conflict of Interest
The authors declare that the research was conducted in the absence of any commercial or financial relationships that could be construed as a potential conflict of interest.
Acknowledgments
We thank Dr. Oliver Nolte, from Zentrum für Labormedizin, Sankt Gallen, Switzerland, for providing Pdd strain 80077637.
Supplementary Material
The Supplementary Material for this article can be found online at: https://www.frontiersin.org/articles/10.3389/fmicb.2020.01771/full#supplementary-material
Footnotes
- ^ https://www.ncbi.nlm.nih.gov/genome/675
- ^ http://www.bioinformatics.babraham.ac.uk/projects/fastqc/
- ^ http://broadinstitute.github.io/picard/
References
Abushattal, S., Vences, A., dos Santos, N. M. S., do Vale, A., and Osorio, C. R. (2019). Draft genome sequences of Photobacterium damselae subsp. piscicida SNW-8.1 and PP3, two fish-isolated strains containing a type III secretion system. Microbiol. Resour. Announc. 8:e00426-19. doi: 10.1128/mra.00426-19
Akram, A., Stevens, R. P., and Konecny, P. (2015). Photobacterium damselae and Vibrio harveyi hand infection from marine exposure. Med. J. 203, 224–225. doi: 10.5694/mja15.00179
Alhemairi, M., Alghanmi, F., and And Alshamrani, A. S. (2015). Child death due to infection with Photobacterium damselae subsp. damselae, a new case. J. Med. Sci. 23, 176–178.
Alvarez, J. R., Lamba, S., Dyer, K. Y., and Apuzzio, J. J. (2006). An unusual case of urinary tract infection in a pregnant woman with Photobacterium damsela. Infect. Dis. Obstet. Gynecol. 2006:80682. doi: 10.1155/IDOG/2006/80682
Anders, S., Pyl, P. T., and Huber, W. (2015). HTSeq–a Python framework to work with high-throughput sequencing data. Bioinformatics 31, 166–169. doi: 10.1093/bioinformatics/btu638
Asato, J., and Kanaya, F. (2004). Fatal infection of the hand due to Photobacterium damsela: a case report. Clin. Infect. Dis. 38, e100–e101. doi: 10.1086/383468
Austin, B. (2010). Vibrios as causal agents of zoonoses. Vet. Microbiol. 140, 310–317. doi: 10.1016/j.vetmic.2009.03.015
Aziz, R. K., Bartels, D., Best, A. A., DeJongh, M., Disz, T., Edwards, R. A., et al. (2008). The RAST server: rapid annotations using subsystems technology. BMC Genomics 9:75. doi: 10.1186/1471-2164-9-75
Barber, G. R., and Swygert, J. S. (2000). Necrotizing fasciitis due to Photobacterium damsela in a man lashed by a stingray. N. Engl. J. Med. 342:824. doi: 10.1056/nejm200003163421118
Begum, A., Rahman, M. M., Ogawa, W., Mizushima, T., Kuroda, T., and Tsuchiya, T. (2005). Gene cloning and characterization of four MATE family multidrug efflux pumps from Vibrio cholerae non-O1. Microbiol. Immunol. 49, 949–957. doi: 10.1111/j.1348-0421.2005.tb03690.x
Bina, J., Zhu, J., Dziejman, M., Faruque, S., Calderwood, S., and Mekalanos, J. (2003). ToxR regulon of Vibrio cholerae and its expression in vibrios shed by cholera patients. Proc. Natl. Acad. Sci. U.S.A. 100, 2801–2806. doi: 10.1073/pnas.2628026100
Clarridge, J. E., and Zighelboim-Daum, S. (1985). Isolation and characterization of two hemolytic phenotypes of Vibrio damsela associated with a fatal wound infection. J. Clin. Microbiol. 21, 302–306. doi: 10.1128/jcm.21.3.302-306.1985
Coffey, J. A. J., Harris, R. L., Rutledge, M. L., Bradshaw, M. W., and Williams, T. W. J. (1986). Vibrio damsela: another potentially virulent marine vibrio. J. Infect. Dis. 153, 800–802. doi: 10.1093/infdis/153.4.800-a
de Paiva-Santos, W., de Sousa, V. S., and Giambiagi-deMarval, M. (2018). Occurrence of virulence-associated genes among Staphylococcus saprophyticus isolated from different sources. Microb. Pathog. 119, 9–11. doi: 10.1016/j.micpath.2018.03.054
Duperthuy, M., Schmitt, P., Garzón, E., Caro, A., Rosa, R. D., Le Roux, F., et al. (2011). Use of OmpU porins for attachment and invasion of Crassostrea gigas immune cells by the oyster pathogen Vibrio splendidus. Proc. Natl. Acad. Sci. U.S.A. 108, 2993–2998. doi: 10.1073/pnas.1015326108
Eissa, I. A. M., Derwa, H. I., Ismail, M., El-Lamie, M., Dessouki, A. A., Elsheshtawy, H., et al. (2018). Molecular and phenotypic characterization of Photobacterium damselae among some marine fishes in Lake Temsah. Microb. Pathog. 114, 315–322. doi: 10.1016/j.micpath.2017.12.006
Ezraty, B., Gennaris, A., Barras, F., and Collet, J.-F. (2017). Oxidative stress, protein damage and repair in bacteria. Nat. Rev. Microbiol. 15, 385–396. doi: 10.1038/nrmicro.2017.26
Ferguson, G. P., McLaggan, D., and Booth, I. R. (1995). Potassium channel activation by glutathione-S-conjugates in Escherichia coli: protection against methylglyoxal is mediated by cytoplasmic acidification. Mol. Microbiol. 17, 1025–1033. doi: 10.1111/j.1365-2958.1995.mmi_17061025.x
Fouz, B., Biosca, E. G., and Amaro, C. (1997). High affinity iron-uptake systems in Vibrio damsela: role in the acquisition of iron from transferrin. J. Appl. Microbiol. 82, 157–167. doi: 10.1111/j.1365-2672.1997.tb02846.x
Fouz, B., Larsen, J., Nielsen, B., Barja, J., and Toranzo, A. (1992). Characterization of Vibrio damsela strains isolated from turbot Scophthalmus maximus in Spain. Dis. Aquat. Organ. 12, 155–166. doi: 10.3354/dao012155
Fouz, B., Toranzo, A. E., Biosca, E. G., Mazoy, R., and Amaro, C. (1994). Role of iron in the pathogenicity of Vibrio damsela for fish and mammals. FEMS Microbiol. Lett. 121, 181–188. doi: 10.1111/j.1574-6968.1994.tb07097.x
Fouz, B., Toranzo, A. E., Milán, M., and Amaro, C. (2000). Evidence that water transmits the disease caused by the fish pathogen Photobacterium damselae subsp. damselae. J. Appl. Microbiol. 88, 531–535. doi: 10.1046/j.1365-2672.2000.00992.x
Fraser, S. L., Purcell, B. K., Delgado, B. J., Baker, A. E., and Whelen, A. C. (1997). Rapidly fatal infection due to Photobacterium (Vibrio) damsela. Clin. Infect. Dis. 25, 935–936. doi: 10.1086/597647
Gandhi, N. S., and Mancera, R. L. (2008). The structure of glycosaminoglycans and their interactions with proteins. Chem. Biol. Drug Des. 72, 455–482. doi: 10.1111/j.1747-0285.2008.00741.x
Gon, S., Giudici-Orticoni, M. T., Méjean, V., and Iobbi-Nivol, C. (2001). Electron transfer and binding of the c-Type cytochrome TorC to the trimethylamine N-oxide reductase in Escherichia coli. J. Biol. Chem. 276, 11545–11551. doi: 10.1074/jbc.M008875200
Goo, S. Y., Lee, H.-J., Kim, W. H., Han, K.-L., Park, D.-K., Lee, H.-J., et al. (2006). Identification of OmpU of Vibrio vulnificus as a fibronectin-binding protein and its role in bacterial pathogenesis. Infect. Immun. 74, 5586–5594. doi: 10.1128/IAI.00171-06
Goodell, K. H., Jordan, M. R., Graham, R., Cassidy, C., and Nasraway, S. A. (2004). Rapidly advancing necrotizing fasciitis caused by Photobacterium (Vibrio) damsela: a hyperaggressive variant. Crit. Care Med. 32, 278–281. doi: 10.1097/01.ccm.0000104920.01254.82
Herz, K., Vimont, S., Padan, E., and Berche, P. (2003). Roles of NhaA, NhaB, and NhaD Na+/H+ antiporters in survival of Vibrio cholerae in a saline environment. J. Bacteriol. 185, 1236–1244. doi: 10.1128/JB.185.4.1236-1244.2003
Huang, L., Liu, W., Jiang, Q., Zuo, Y., Su, Y., Zhao, L., et al. (2018). Integration of transcriptomic and proteomic approaches reveals the temperature-dependent virulence of Pseudomonas plecoglossicida. Front. Cell. Infect. Microbiol. 8:207. doi: 10.3389/fcimb.2018.00207
Hundenborn, J., Thurig, S., Kommerell, M., Haag, H., and Nolte, O. (2013). Severe wound Infection with Photobacterium damselae ssp. damselae and Vibrio harveyi, following a laceration injury in marine environment: a case report and review of the literature. Case Rep. Med. 2013:610632. doi: 10.1155/2013/610632
Kim, H. R., Kim, J. W., Lee, M. K., and Kim, J. G. (2009). Septicemia progressing to fatal hepatic dysfunction in a cirrhotic patient after oral ingestion of Photobacterium damsela: a case report. Infection 37, 555–556. doi: 10.1007/s15010-009-9049-8
Knight-Madden, J. M., Barton, M., Gandretti, N., and Nicholson, A. M. (2005). Photobacterium damsela bacteremia in a child with sickle-cell disease. Pediatr. Infect. Dis. J. 24, 654–655. doi: 10.1097/01.inf.0000168845.26758.e3
Kong, H.-K., Law, H.-W., Liu, X., Law, C. O. K., Pan, Q., Gao, L., et al. (2017). Transcriptomic analysis of Laribacter hongkongensis reveals adaptive response coupled with temperature. PLoS One 12:e0169998. doi: 10.1371/journal.pone.0169998
Kreger, A. S. (1984). Cytolytic activity and virulence of Vibrio damsela. Infect. Immun. 44, 326–331. doi: 10.1128/iai.44.2.326-331.1984
Langmead, B., and Salzberg, S. L. (2012). Fast gapped-read alignment with Bowtie 2. Nat. Methods 9, 357–359. doi: 10.1038/nmeth.1923
Lee, I., Ouk Kim, Y., Park, S.-C., and Chun, J. (2016). OrthoANI: an improved algorithm and software for calculating average nucleotide identity. Int. J. Syst. Evol. Microbiol. 66, 1100–1103. doi: 10.1099/ijsem.0.000760
Lehane, L., and Rawlin, G. T. (2000). Topically acquired bacterial zoonoses from fish: a review. Med. J. 173, 256–259. doi: 10.5694/j.1326-5377.2000.tb125632.x
Letunic, I., and Bork, P. (2019). Interactive tree of life (iTOL) v4: recent updates and new developments. Nucl. Acids Res. 47, 256–259. doi: 10.1093/nar/gkz239
Li, H., Handsaker, B., Wysoker, A., Fennell, T., Ruan, J., Homer, N., et al. (2009). The sequence alignment/map format and SAMtools. Bioinformatics 25, 2078–2079. doi: 10.1093/bioinformatics/btp352
Love, M. I., Huber, W., and Anders, S. (2014). Moderated estimation of fold change and dispersion for RNA-seq data with DESeq2. Genome Biol. 15:550. doi: 10.1186/s13059-014-0550-8
Mansilla, M. C., Cybulski, L. E., Albanesi, D., and De Mendoza, D. (2004). Control of membrane lipid fluidity by molecular thermosensors. J. Bacteriol. 186, 6681–6688. doi: 10.1128/JB.186.20.6681-6688.2004
Marr, A. G., and Ingraham, J. L. (1962). Effect of temperature on the composition of fatty acids in Escherichia coli. J. Bacteriol. 84, 1260–1267. doi: 10.1128/jb.84.6.1260-1267.1962
Matanza, X. M., and Osorio, C. R. (2018). Transcriptome changes in response to temperature in the fish pathogen Photobacterium damselae subsp. damselae: clues to understand the emergence of disease outbreaks at increased seawater temperatures. PLoS One 13:e0210118. doi: 10.1371/journal.pone.0210118
McGinnis, M. W., Parker, Z. M., Walter, N. E., Rutkovsky, A. C., Cartaya-Marin, C., and Karatan, E. (2009). Spermidine regulates Vibrio cholerae biofilm formation via transport and signaling pathways. FEMS Microbiol. Lett. 299, 166–174. doi: 10.1111/j.1574-6968.2009.01744.x
Mercer, R., Nguyen, O., Ou, Q., McMullen, L., and Gänzle, M. G. (2017). Functional analysis of genes comprising the locus of heat resistance in Escherichia coli. Appl. Environ. Microbiol. 83, e1400–e1417. doi: 10.1128/AEM.01400-17
Morris, J. G., Wilson, R., Hollis, D. G., Weaver, R. E., Miller, H. G., Tacket, C. O., et al. (1982). Illness caused by Vibrio damsela and Vibrio hollisae. Lancet 319, 1294–1297. doi: 10.1016/S0140-6736(82)92853-7
Mouriño, S., Osorio, C. R., and Lemos, M. L. (2004). Characterization of heme uptake cluster genes in the fish pathogen Vibrio anguillarum. J. Bacteriol. 186, 6159–6167. doi: 10.1128/JB.186.18.6159-6167.2004
Nakamura, Y., Uchihira, M., Ichimiya, M., Morita, K., and Muto, M. (2008). Necrotizing fasciitis of the leg due to Photobacterium damsela. J. Dermatol. 35, 44–45. doi: 10.1111/j.1346-8138.2007.00412.x
Nielubowicz, G. R., and Mobley, H. L. T. (2010). Host-pathogen interactions in urinary tract infection. Nat. Rev. Urol. 7, 430–441. doi: 10.1038/nrurol.2010.101
Osorio, C. R., Vences, A., Matanza, X. M., and Terceti, M. S. (2018). Photobacterium damselae subsp. damselae, a generalist pathogen with unique virulence factors and high genetic diversity. J. Bacteriol. 200:e00002-18. doi: 10.1128/JB.00002-18
Otsuka, M., Yasuda, M., Morita, Y., Otsuka, C., Tsuchiya, T., Omote, H., et al. (2005). Identification of essential amino acid residues of the NorM Na+/multidrug antiporter in Vibrio parahaemolyticus. J. Bacteriol. 187, 1552–1558. doi: 10.1128/JB.187.5.1552-1558.2005
Pedersen, K., Skall, H. F., Lassen-Nielsen, A. M., Bjerrum, L., and Olesen, N. J. (2009). Photobacterium damselae subsp. damselae, an emerging pathogen in Danish rainbow trout, Oncorhynchus mykiss (Walbaum), mariculture. J. Fish Dis. 32, 465–472. doi: 10.1111/j.1365-2761.2009.01041.x
Puentes, B., Balado, M., Bermudez-Crespo, J., Osorio, C. R., and Lemos, M. L. (2017). A proteomic analysis of the iron response of Photobacterium damselae subsp. damselae reveals metabolic adaptations to iron levels changes and novel potential virulence factors. Vet. Microbiol. 201, 257–264. doi: 10.1016/j.vetmic.2017.01.040
Rivas, A. J., Balado, M., Lemos, M. L., and Osorio, C. R. (2011). The Photobacterium damselae subsp. damselae hemolysins damselysin and HlyA are encoded within a new virulence plasmid. Infect. Immun. 79, 4617–4627. doi: 10.1128/IAI.05436-11
Rivas, A. J., Balado, M., Lemos, M. L., and Osorio, C. R. (2013). Synergistic and additive effects of chromosomal and plasmid-encoded hemolysins contribute to hemolysis and virulence in Photobacterium damselae subsp. damselae. Infect. Immun. 81, 3287–3299. doi: 10.1128/IAI.00155-13
Rivas, A. J., Vences, A., Husmann, M., Lemos, M. L., and Osorio, C. R. (2015a). Photobacterium damselae subsp. damselae major virulence factors Dly, plasmid-encoded HlyA, and chromosome-encoded HlyA are secreted via the type II secretion system. Infect. Immun. 83, 1246–1256. doi: 10.1128/IAI.02608-14
Rivas, A. J., von Hoven, G., Neukirch, C., Meyenburg, M., Qin, Q., Fuser, S., et al. (2015b). Phobalysin, a small beta-pore-forming toxin of Photobacterium damselae subsp. damselae. Infect. Immun. 83, 4335–4348. doi: 10.1128/IAI.00277-15
Rock, C. O., and Cronan, J. E. (1996). Escherichia coli as a model for the regulation of dissociable (type II) fatty acid biosynthesis. Biochim. Biophys. Acta Lipids Lipid Metab. 1302, 1–16. doi: 10.1016/0005-2760(96)00056-2
Romalde, J. L. (2002). Photobacterium damselae subsp. piscicida: an integrated view of a bacterial fish pathogen. Int. Microbiol. 5, 3–9. doi: 10.1007/s10123-002-0051-6
Ronholm, J., Petronella, N., Chew Leung, C., Pightling, A. W., and Banerjee, S. K. (2016). Genomic features of environmental and clinical Vibrio parahaemolyticus isolates lacking recognized virulence factors are dissimilar. Appl. Environ. Microbiol. 82, 1102–1113. doi: 10.1128/AEM.03465-15
Russell, N. J. (1983). Adaptation to temperature in bacterial membranes. Biochem. Soc. Trans. 11, 333–335. doi: 10.1042/bst0110333
Sachs, G., Weeks, D. L., Melchers, K., and Scott, D. R. (2003). The gastric biology of Helicobacter pylori. Annu. Rev. Physiol. 65, 349–369. doi: 10.1146/annurev.physiol.65.092101.142156
Sakharwade, S. C., and Mukhopadhaya, A. (2015). Vibrio cholerae porin OmpU induces LPS tolerance by attenuating TLR-mediated signaling. Mol. Immunol. 68, 312–324. doi: 10.1016/j.molimm.2015.09.021
Sato, H., and Frank, D. W. (2014). Intoxication of host cells by the T3SS phospholipase ExoU: PI(4,5)P2-associated, cytoskeletal collapse and late phase membrane blebbing. PLoS One 9:e103127. doi: 10.1371/journal.pone.0103127
Shah, P., and Swiatlo, E. (2008). A multifaceted role for polyamines in bacterial pathogens. Mol. Microbiol. 68, 4–16. doi: 10.1111/j.1365-2958.2008.06126.x
Sinensky, M. (1974). Homeoviscous adaptation: a homeostatic process that regulates the viscosity of membrane lipids in Escherichia coli. Proc. Natl. Acad. Sci. U.S.A. 71, 522–525. doi: 10.1073/pnas.71.2.522
Singh, N., Bubunenko, M., Smith, C., Abbott, D. M., Stringer, A. M., Shi, R., et al. (2016). Suhb associates with nus factors to facilitate 30S ribosome biogenesis in Escherichia coli. mBio 7:e0114-16. doi: 10.1128/mBio.00114-16
Terceti, M. S., Rivas, A. J., Alvarez, L., Noia, M., Cava, F., and Osorio, C. R. (2017). rstB regulates expression of the Photobacterium damselae subsp. damselae major virulence factors Damselysin, Phobalysin P and Phobalysin C. Front. Microbiol. 8:582. doi: 10.3389/fmicb.2017.00582
Terceti, M. S., Vences, A., Matanza, X. M., Barca, A. V., Noia, M., Lisboa, J., et al. (2019). The RstAB system impacts virulence, motility, cell morphology, penicillin tolerance and production of Type II Secretion System-dependent factors in the fish and human pathogen Photobacterium damselae subsp. damselae. Front. Microbiol. 10:897. doi: 10.3389/fmicb.2019.00897
Urmersbach, S., Aho, T., Alter, T., Hassan, S. S., Autio, R., and Huehn, S. (2015). Changes in global gene expression of Vibrio parahaemolyticus induced by cold- and heat-stress. BMC Microbiol. 15:229. doi: 10.1186/s12866-015-0565-7
Vences, A., Rivas, A. J., Lemos, M. L., Husmann, M., and Osorio, C. R. (2017). Chromosome-encoded hemolysin, phospholipase, and collagenase in plasmidless isolates of Photobacterium damselae subsp. damselae contribute to virulence for fish. Appl. Environ. Microbiol. 83, e401–e417. doi: 10.1128/AEM.00401-17
von Hoven, G., Neukirch, C., Meyenburg, M., Schmidt, S., Vences, A., Osorio, C. R., et al. (2018). Cytotoxin- and chemotaxis-genes cooperate to promote adhesion of Photobacterium damselae subsp. damselae. Front. Microbiol. 9:2996. doi: 10.3389/fmicb.2018.02996
Wang, H., Ayala, J. C., Benitez, J. A., and Silva, A. J. (2015). RNA-seq analysis identifies new genes regulated by the histone-like nucleoid structuring protein (H-NS) affecting Vibrio cholerae virulence, stress response and chemotaxis. PLoS One 10:e0118295. doi: 10.1371/journal.pone.0118295
Weaver, A. I., Murphy, S. G., Umans, B. D., Tallavajhala, S., Onyekwere, I., Wittels, S., et al. (2018). Genetic determinants of penicillin tolerance in Vibrio cholerae. Antimicrob. Agents Chemother. 62:e01326-18. doi: 10.1128/AAC.01326-18
Williams, T. C., Blackman, E. R., Morrison, S. S., Gibas, C. J., and Oliver, J. D. (2014). Transcriptome sequencing reveals the virulence and environmental genetic programs of Vibrio vulnificus exposed to host and estuarine conditions. PLoS One 9:e114376. doi: 10.1371/journal.pone.0114376
Yamane, K., Asato, J., Kawade, N., Takahashi, H., Kimura, B., and Arakawa, Y. (2004). Two cases of fatal necrotizing fasciitis caused by Photobacterium damsela in Japan. J. Clin. Microbiol. 42, 1370–1372. doi: 10.1128/jcm.42.3.1370-1372.2004
Yu, Y., Zhang, Z., Wang, Y., Liao, M., Rong, X., Li, B., et al. (2019). Complete Genome sequence of Photobacterium damselae subsp. damselae strain SSPD1601 isolated from deep-sea cage-cultured Sebastes schlegelii with septic skin ulcer. Int. J. Genom. 2019:4242653. doi: 10.1155/2019/4242653
Yuen, K.-Y., Ma, L., Wong, S. S. Y., and Ng, W.-F. (1993). Fatal necrotizing fasciitis due to Vibrio damsela. Scand. J. Infect. Dis. 25, 659–661. doi: 10.3109/00365549309008557
Keywords: Photobacterium damselae, zoonosis, temperature, transcriptome, hemolysin, heat-shock response
Citation: Matanza XM and Osorio CR (2020) Exposure of the Opportunistic Marine Pathogen Photobacterium damselae subsp. damselae to Human Body Temperature Is a Stressful Condition That Shapes the Transcriptome, Viability, Cell Morphology, and Virulence. Front. Microbiol. 11:1771. doi: 10.3389/fmicb.2020.01771
Received: 10 February 2020; Accepted: 06 July 2020;
Published: 24 July 2020.
Edited by:
Jennifer M. Ritchie, University of Surrey, United KingdomReviewed by:
Yunzeng Zhang, Yangzhou University, ChinaJens Andre Hammerl, Federal Institute for Risk Assessment (BfR), Germany
Copyright © 2020 Matanza and Osorio. This is an open-access article distributed under the terms of the Creative Commons Attribution License (CC BY). The use, distribution or reproduction in other forums is permitted, provided the original author(s) and the copyright owner(s) are credited and that the original publication in this journal is cited, in accordance with accepted academic practice. No use, distribution or reproduction is permitted which does not comply with these terms.
*Correspondence: Carlos R. Osorio, cr.osorio@usc.es