- 1University of Maryland Extension, Queenstown, MD, United States
- 2Department of Plant and Soil Sciences, University of Delaware, Georgetown, DE, United States
Cereal rye as a cover crop is often used to improve soil health and as part of integrated weed management programs. Despite this, cereal rye biomass is often not managed for optimal weed suppression. This study evaluated the effects of managing cereal rye as part of an integrated weed management strategy in soybean. Factors consisted of levels of cereal rye management (no cereal rye, no nitrogen, or 20 kg/ha of nitrogen); cereal rye termination timing (20 or 10 d before soybean planting); and residual herbicide treatment applied at cereal rye termination (with or without). Winter annual weed control with cereal rye was generally greater compared to no cereal rye. Winter annual weed control was consistently better when cereal rye was terminated at 20 d before soybean planting compared to 10 d; while summer annual weed control was improved if termination was delayed. Effect of cereal rye management on summer annual weed control varied by weed species. In the absence of residual herbicides, Palmer amaranth control responded to the different levels of cereal rye management. However, morningglory spp. only responded to rye with supplemental N applications. Large crabgrass control was similar for treatments containing cereal rye, regardless of nitrogen input. Our results demonstrate the importance of cover crop management when incorporating cereal rye into an integrated weed management program for soybean.
Introduction
Cover crops provide a number of ecological services, or benefits. These benefits include reducing erosion, preventing nutrient loss, providing pollinator habitat, fixing atmospheric nitrogen, and increasing soil microbial densities. In the Chesapeake Bay Watershed, adoption of cover crops for nutrient management has been very successful. Most cover crops are planted under cost-share programs that specify deadlines for fall planting dates, but allow termination in early spring. As a result, many cover crop fields receive a non-selective herbicide application in the spring prior to the rapid growth phase of most winter-hardy cover crops, which leads to very little cover crop residue present at planting.
Non-selective herbicide applications to terminate cover crops often provide excellent control of emerged weed seedlings. However, early spring termination often occurs before there is sufficient cover crop biomass to provide weed suppression of later-emerging weeds. Cover crops allowed to advance to the late-vegetative or early-reproductive stages produce significantly more biomass. In addition, the cover crop tissue contains a higher C:N ratio that resists decomposition and allows the dead biomass to persist longer (Wagger et al., 1998; Pittman et al., 2020).
Cover crops terminated later in the spring, provide weed suppression through direct plant-to-plant competition and/or as a physical barrier. Prior to cover crop termination, cover crops compete with weeds for resources required for growth (nutrients, moisture, sunlight) and typically cover crop species that gain a height advantage or rapidly shade the soil surface are the most competitive. These interactions occur between fall-seeded cover crops and winter annual weeds and weed species that germinate early spring before the cover crop is terminated.
After termination, desiccated cover crops tissue suppress weeds by altering light exposure at the soil surface, lowering night/day temperature fluctuations, lowering soil temperatures, and serving as a physical barrier that limits weed seedling growth (Teasdale and Mohler, 1993; Mirsky et al., 2011; DeVore et al., 2013). The higher the level of biomass the better weed suppression (Mohler and Teasdale, 1993).
Studies have documented lower weed densities and shorter weeds in the presence of cover crops (Montgomery et al., 2018; Wallace et al., 2019; DeSimini et al., in press). As a result, emerged weeds are susceptible to postemergence herbicides over a longer time period due to delayed emergence and slower growth.
Studies examining termination timing have shown that allowing an additional 2 wk of cover crop growth during the rapid stem elongation phase can result in a substantial increase in cover crop biomass (Mirsky et al., 2011; Cornelius and Bradley, 2017a; Whalen et al., 2020). However, there are few published studies examining late-spring termination on both winter annual and summer annual weeds.
Cover crops have been researched as an integrated weed management tool, particularly, to manage herbicide-resistant biotypes. Multiple-herbicide resistance has been documented in numerous weed species in the Mid-Atlantic region, including acetolactate synthase-inhibiting (ALS)- and glyphosate-resistant Palmer amaranth (Amaranthus palmeri [S. Wats.]), common ragweed (Ambrosia artemisiifolia [L.]), and horseweed (Erigeron canadensis L. [Cronq.]) (Heap, 2020). Although not widespread, common ragweed resistant to protoporphyrinogen oxidase inhibiting (PPO)-herbicides has also been documented in several Mid-Atlantic States (Heap, 2020). Preliminary studies have shown cover crops can play an important role in mitigating resistance and improving overall weed control. Cover crops, as a part of an integrated approach, requires additional research to better understand the contribution of the various tactics for overall weed control. This experiment was initiated to evaluate cereal rye (Secale cereale L.) management in combination with herbicides for integrated management of winter and summer annual weeds.
Materials and Methods
This study was conducted over three soybean (Glycine max [L.] Merr.) growing seasons beginning in 2015 at the University of Delaware's Carvel Research and Education Center located near Georgetown, DE (38.64°N, 75.46°W). In 2015 and 2017, the soil was a Pepperbox loamy sand (loamy, mixed, mesic Aquic Arenic Paleudults) and in 2016 the soil was a Rosedale loamy sand (loamy, siliceous, mesic Arenic Hapludults). Soil texture was either loamy sand or sandy loam with 1.5–2.5% organic matter and pH ranging from 4.8 to 6.5 (Table 1).
The study included three factors, cereal rye management, timing of cereal rye termination, and use of residual herbicides. Cereal rye management was no rye, rye without additional N, or rye with 20 kg ha−1 of nitrogen applied before jointing. Rye was terminated 20 or 10 d prior to soybean planting. The residual herbicide treatment was included at the time of cover crop termination or no residual herbicide was used.
The entire site was drilled (rows 18 cm spacing) with 135 kg ha−1 of cereal rye in the fall (mid-October–mid-November). The no rye treatments were sprayed in the fall with glyphosate (2015) or clethodim (2016 and 2017).
There were two cereal rye termination timings, which were intended to be 20 or 10 days prior to soybean planting. Early-termination timings ranged from 18 to 29 days early preplant (EPP) while late terminations ranged from 10 to 16 days EPP (Table 1). Cereal rye was terminated with glyphosate (Roundup PowerMax®, Monsanto Company, St. Louis, MO) at 1.3 kg ae ha−1 plus 2,4-D at 0.5 kg ae ha−1 (Weedone® LV4, Nufarm, Inc., Alsip, IL). 2,4-D was included to control glyphosate-resistant weeds. Treatments with residual herbicides were treated with a pre-packaged mixture of chlorimuron plus thifensulfuron plus flumioxazin (19 + 61 + 6 g ai ha−1, respectively) (Envive®, E.I. du Pont de Nemours and Company, Wilmington, DE) tankmixed with the glyphosate plus 2,4-D application.
Comparison treatments included no rye and no spring herbicide application (weedy check for winter annual weeds) and no rye but glyphosate applied early spring (non-treated check for summer annual weeds) to provide reference plots for visual ratings. Weed control ratings for the comparison treatments were not included in statistical analysis. The entire experimental site was treated 5 wk after soybean planting (WAP) with glyphosate plus fomesafen (Reflex®, Syngenta Crop Protection, Greensboro, NC) at 1.3 kg ae ha−1 plus 420 g ai ha−1, respectively, plus non-ionic surfactant at 0.25 % v v−1 (Scanner®, Loveland Products, Greeley, CO).
Soybeans were planted no-till in 38 cm rows at 60,700–72,800 seeds ha−1 between May 20 and June 1 (Table 1). Individual plots were 7.6 m long and 3 m wide (7 rows per plot). All herbicide applications were made with a tractor mounted sprayer, using compressed air, traveling 4.8 km h−1. The spray volume was 187 L ha−1 and nozzles were 11,002 (Greenleaf AirMix®, Greenleaf Technologies, Covington, LA) with a pressure of 276 kPa. Treatments were replicated three times in 2015 and four times in 2016 and 2017. The plots were arranged in a randomized complete block design.
Cereal rye biomass was collected 1 wk before planting in 2015 and 2017, and 8 WAP in 2016. Rye biomass was collected in 1 m−2 quadrats in all plots without residual herbicide treatments to obtain a representative sample of biomass production. Rye biomass was dried to a constant weight and recorded.
Weed control was evaluated visually on a scale of 0–100, with 0 = no plant response and 100 = complete plant death. Winter annual weeds were rated at soybean planting and summer annual weeds were rated 1, 4, and 8 WAP. The middle five rows of each plot were harvested with a combine at physiological maturity. Yields were adjusted to 13% moisture content.
Statistical Analysis
Weed control data were arcsine square-root transformed prior to analysis to address variance in homogeneity and normality, and untransformed data are presented in the tables. Statistical analyses were conducted with PROC Mixed in SAS version 9.4 (SAS Institute Inc., Cary, NC), using year and replications within year as random effect. Fisher's protected LSD was used for mean separation at P = 0.05.
Results
Cereal Rye Biomass
Differences in cereal rye biomass were achieved through supplemental spring nitrogen and termination timing each year. Early-spring nitrogen applications resulted in a 39% increase in biomass in 2015 and 2017 (p = 0.026), and a 124% increase in 2016 (p = 0.0004) (Table 2). In 2016, rye biomass was sampled 8 WAP, which allowed for a significant amount of biomass decomposition, but it is difficult to say how much decomposition had occurred (Poffenbarger et al., 2015; Sievers and Cook, 2018).
In 2015 and 2017, delaying rye termination resulted in a 60% increase in cereal rye biomass (p = 0.0031, Table 2). No differences were detected in 2016 when rye sampling was done 8 WAP. Early termination corresponded to stem elongation stage (Zadoks 35–37), while later termination occurred during boot stage (Zadoks 41–47). Levels of cereal rye biomass achieved in our trial were similar to other trials investigating weed suppression in the region (Mischler et al., 2010; Mirsky et al., 2011, 2017; Ryan et al., 2011a,b).
Winter Annual Weed Control
Glyphosate was used to remove cereal rye in the no rye treatment in 2015. This resulted in confounding between winter annual weed control and no rye treatments. So analysis did not include 2015 data. Winter annual weeds were rated within 3 d after planting and the main effects were significant but there were no interactions.
Horseweed and cutleaf eveningprimrose (Oenothera laciniata [Hill]) were the predominant species in 2016 and 2017, respectively. A multiple-resistant horseweed biotype (glyphosate- and ALS-resistant) was the dominant biotype at this site.
Horseweed control in 2016, rated at soybean planting was greatest for rye with N and least for rye without N (p = 0.001, Table 3). However, by 4 WAP, horseweed control was better for rye without N compared to rye with N or no rye (p = 0.002). Horseweed density was extremely high (over 100 plants m−2) and intra-specific competition accounted for some inconsistency among rye management treatments. Cereal rye terminated 20 d EPP provided better horseweed control than cereal rye terminated 10 d EPP at both rating dates (p = 0.0001). At the time of early application, horseweed height was up to 13 cm and up to 23 cm at late termination. Residual herbicide did not have a significant influence on horseweed control since the biotype was predominately glyphosate- and ALS-resistant. 2,4-D was the herbicide active ingredient providing horseweed control and its effectiveness is reduced on larger horseweed plants.
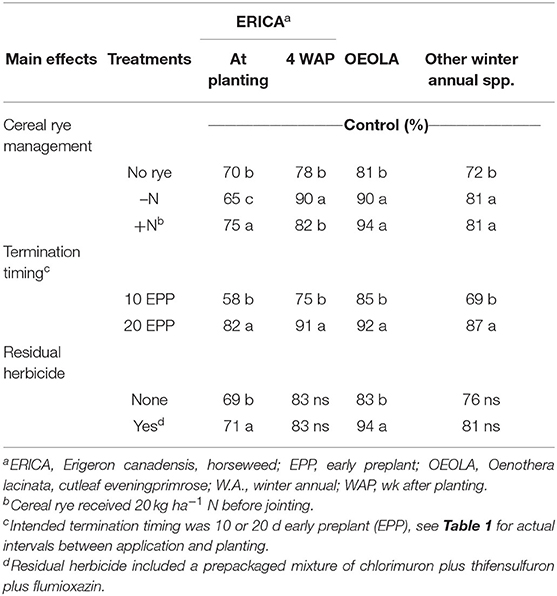
Table 3. Influence of cereal rye management, termination timing, and residual herbicide on horseweed in 2016, cutleaf eveninggprimrose in 2017, winter annual weed control in 2016 and 2017 when rated at soybean planting.
Cutleaf eveningprimrose control was also greatest if cereal rye was present (p = 0.0002) and with earlier termination (p = 0.004) (Table 3) in 2017. In addition, control was better when chlorimuron plus thifensulfuron plus flumioxazin was included (p = 0.0001). Chlorimuron plus thifensulfuron plus flumioxazin is labeled for postemergence control of cutleaf eveningprimrose. In this trial, the rate of 2,4-D was not adequate to control cutleaf eveningprimrose, for instance glyphosate plus 2,4-D applied without cover crop or residual herbicide provided only 72% control.
Densities of the remaining winter annual weeds were low, so they were rated together. Remaining winter annual weeds included common chickweed (Stellaria media [L.] Vill.), henbit (Lamium amplexicaule L.), jagged chickweed (Holosteum umbellatum L.), knawel (Scleranthus annuus L.) and redstem filaree (Erodium cicutarium [L.] L'Her.). Cereal rye management (p = 0.01) and termination timing were significant (p = 0.0001) (Table 3). Remaining winter annual weed control was best when cereal rye was present, regardless of the amount, and when cereal rye was terminated 20 d EPP compared to applications closer to planting.
Summer Annual Weed Control
Palmer amaranth, morningglory species (predominantly Ipomoea hederacea Jacq. and secondarily Ipomoea lacunosa L.) and large crabgrass (Digitaria sanguinalis L. [Scop.]) were the dominant summer annual species and were present in each site year. Main effects were significant for all species rated. Cereal rye management by residual herbicide for Palmer amaranth control was the only interaction observed. Cereal rye terminated 10 d EPP provided better summer annual weed control than rye terminated several weeks before planting. At 1 WAP, cereal rye terminated 10 d EPP improved Palmer amaranth control by 6% compared to rye terminated 20 d EPP (p = 0.017, Table 4). Palmer amaranth control with residual herbicides was 67–71% regardless of cereal rye management, while treatments without a residual herbicide provided only 35–57% control (p = 0.0151). When residual herbicides were omitted, additional cereal rye management improved Palmer amaranth control. Rye with N improved Palmer amaranth control by 11% compared to rye without N and 22% greater than no rye. Loux et al. (2017) reported similar results, with benefits of cereal rye for Amaranthus spp. control only observed in the absence of preemergence herbicides.
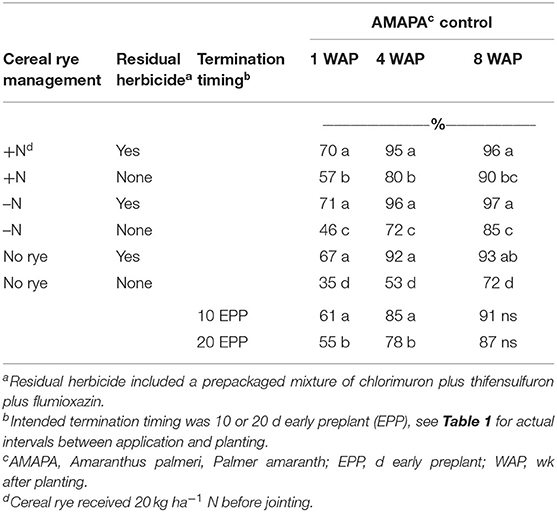
Table 4. Influence of cereal rye management, termination, timing, and residual herbicides on Palmer amaranth control.
At 4 WAP, trends were similar to those observed 1 WAP. Rye terminated 10 d EPP provided better Palmer amaranth control than rye terminated 20 d EPP (p = 0.0016, Table 4). Treatments that included residual herbicides provided at least 92% Palmer amaranth control, regardless of rye management (p = 0.0001). When residual herbicides were omitted, rye with N controlled Palmer amaranth 8% more than rye without N, and 27% greater than no rye.
The entire study was treated with glyphosate plus fomesafen 5 WAP to assess cereal rye management, termination timing, and residual herbicides for full-season weed control. At 8 WAP, data shows similar trends to what was observed prior to the glyphosate plus fomesafen applications (Table 4). Residual herbicides treatments provided at least 93% control of Palmer amaranth, regardless of rye management (p = 0.0029). When residual herbicides were omitted, cereal rye provided 85–90% Palmer amaranth control. Furthermore, rye with N alone provided similar control as the residual herbicide treatment with no rye. This is likely due to the increased rye biomass that suppressed growth of Palmer amaranth seedlings and thus were more susceptible to the glyphosate plus fomesafen treatment applied 5 WAP. Fomesafen is a contact herbicide that works best when weeds are small and in low densities. Cereal rye resulted in fewer, smaller amaranth plants, which improved control with the postemergence herbicide application.
At 1 WAP, morningglory spp. control was greater for rye with N compared to rye without N or no cereal rye p = 0.003, Table 5). Terminating cereal rye 10 d EPP provided 62% control compared to 54% when terminating 20 d EPP (p = 0.002). Including a residual herbicide provided 67% compared to 49% control with no residual herbicide (0.0001).
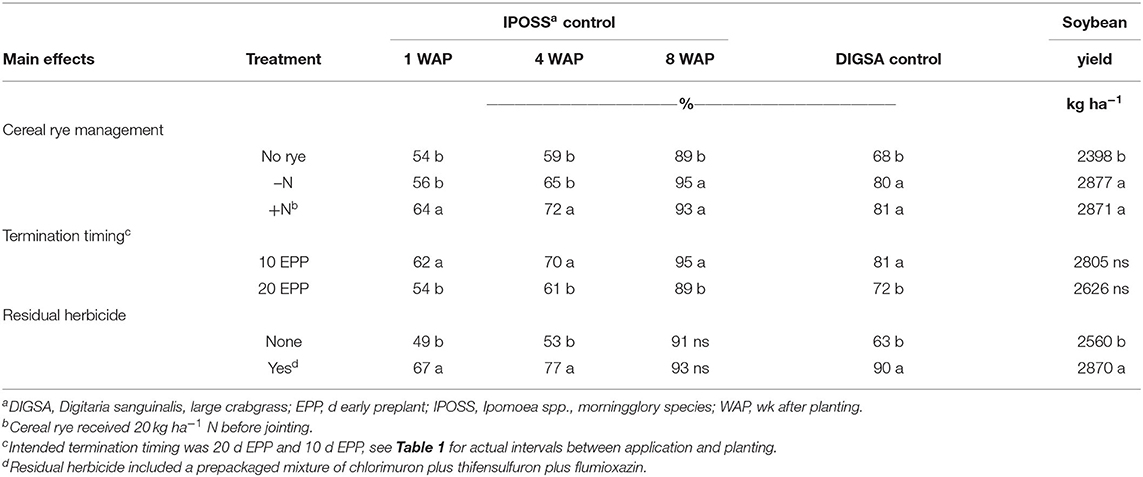
Table 5. Influence of cereal rye management, termination timing, and residual herbicide on control of morningglory spp. and large crabgrass and soybean yield.
Trends for morningglory spp. control were similar at 4 WAP (Table 5). Control improved when N was applied to rye (p = 0.005), when rye was terminated 10 d EPP (p = 0.0014), and when a residual herbicide was included (p = 0.0001). Morningglory spp. control improved with glyphosate plus fomesafen application when rated 8 WAP, but only the main effects of cereal rye management (p = 0.002) and termination timing (p = 0.0001) were significant (Table 5). The presence of cereal rye, regardless of management improved morningglory spp. control compared to no cereal rye (Table 5).
At 4 WAP, large crabgrass control was greater when cereal rye was present (p = 0.0003), when rye was terminated 10 d EPP (p = 0.0008), and when a residual herbicide was included (p = 0.0001) (Table 5). The greatest improvement in control was the result of including a residual herbicide at termination timing, improving control from 63 to 90%. At 8 WAP, all plots had >97% large crabgrass control due to the glyphosate plus fomesafen application.
Soybean Yield
Soybean yield was greater when cereal rye was present (p = 0.0001) and when a residual herbicide was included (p = 0.001) (Table 5). A yield increase of 20% was observed with cereal rye, regardless of management (Table 5). Including a residual herbicide resulted in a 12% increase in yield compared to no residual herbicide.
Discussion
Altering cereal rye management resulted in different levels of biomass, with cereal rye responding to nitrogen applications. Our results show that while residual herbicide treatments were very effective for summer annual weed control, cereal rye with spring N was a consistently successful weed management tactic. While residual herbicides alone provided effective control in this trial, their effectiveness is dependent on environmental factors such as rainfall and soil type. For example, a lack of rainfall or too much rainfall can reduce the efficacy of residual herbicides resulting in lower levels of weed control. In addition, chlorimuron plus thifensulfuron plus flumioxazin will provide 3–4 wk of residual control. In our study, these residual herbicides were applied at least a week before soybean planting. As a result, additional tactics of cereal rye or residual applications close to planting were needed for the highest level of control. Since the Palmer amaranth biotype in this study was glyphosate- and ALS-resistant, there was only one active effective ingredient for residual control, which is not an effective resistance-management strategy (Norsworthy et al., 2012). Including cereal rye helped reduce the selection pressure on the herbicides used in this study.
Our research supports other studies that have shown cereal rye effectiveness in managing both winter (Hayden et al., 2012; Cornelius and Bradley, 2017b; Pittman et al., 2019; Sherman et al., 2020) and summer annual weeds (DeVore et al., 2013; Wiggins et al., 2015, 2016; Loux et al., 2017). Suppression of summer annual weeds is often positively correlated to higher cover crop biomass (Teasdale and Mohler, 2000; Ryan et al., 2011a,b). Cereal rye contains allelochemicals that can influence weed growth, although these effects often are reduced as rye reaches maturity and dissipate within a few weeks of rye termination (Reberg-Horton et al., 2005; Teasdale et al., 2012). Our study was not designed to separate the effects of allelopathy from plant to plant competition or the physical barrier from rye residues.
Additional N inputs or delaying cereal rye termination did improve weed control. These two approaches allowed cover crops to produce more biomass, resulting in additional mulch that hinders weed emergence and is more resistant to decay (Wagger, 1989; Mirsky et al., 2011). However, not all weed species responded consistently to the two levels of cereal rye management. No differences in winter annual weed control were observed among cereal rye treatments with and without N. Cereal rye affects winter annual weeds through inter-species competition, rather than physical suppression. The nitrogen applications were applied in mid-March and so there would have been no difference in ground cover or cereal rye biomass in the fall or early spring presumably when many of the winter annuals emerged. Furthermore, this study did not detect differences between the two levels of rye on the plant competition between winter annuals and cereal rye.
For summer annual weeds, we observed a rye management response only for Palmer amaranth control, and it occurred at all rating dates in the absence of residual herbicides. After the postemergence application, the rye with N, rye without N, and no rye biomass provided 90, 85, and 72% control, respectively. Morningglory spp. control was similar for rye without N and no rye. Only when rye received an application of N was there enough biomass production to improve morningglory spp. control at 1 and 4 WAP. Large crabgrass response was similar in the presence of rye, regardless of N application, and this control was greater than no rye. Our results imply that small-seeded species like Palmer amaranth and large crabgrass will respond to modest levels of rye biomass, but morningglory spp. need biomass levels >538 g m−2 to influence seedling growth.
The effect of termination timing differed by weed life cycles. Winter annual weed control was better when cereal rye was terminated 20 d EPP, while summer annual weed control was improved when cereal rye was terminated 10 d EPP. In this trial, we did not explore why these differences were observed. However, this provides a challenge for farmers trying to manage winter and summer annual weeds efficiently. Additional research needs to focus on minimizing the number of herbicide applications, while maintaining high levels of weed control.
Increased soybean yield in the presence of cereal rye biomass cannot be attributed solely to improved weed control. Cereal rye mulch conserves soil moisture, which improved soybean yield.
Furthermore, this research is consistent with research showing that cereal rye does not negatively influence herbicide efficacy (Perkins et al., in press). While some research has demonstrated delayed cover crop termination can result in a reduction of herbicide reaching the soil (Whalen et al., 2020), this reduction in herbicide efficacy may be offset by increased cover crop biomass. Future research needs to investigate the relationship between cover crop biomass and soil-applied herbicide performance.
While cereal rye did not eliminate the need for soil-applied herbicides, it consistently improved weed control. This research contributes to our growing knowledge of weed control with cover crops and demonstrates the effects cereal rye management can have on ecological services.
Data Availability Statement
The raw data supporting the conclusions of this article will be made available by the authors, without undue reservation.
Author Contributions
MV, BS, and QJ conceptualized, designed the study, and participated in its execution and data collection. MV and KV did the statistical analysis. KV wrote the first draft. MV, BS, and QJ edited the draft. All authors contributed to the article and approved the submitted version.
Funding
Financial support for this study was provided by the Delaware Soybean Board.
Conflict of Interest
The authors declare that the research was conducted in the absence of any commercial or financial relationships that could be construed as a potential conflict of interest.
References
Cornelius, C. D., and Bradley, K. W. (2017a). Herbicide programs for the termination of various cover crop species. Weed Tech. 31, 514–522. doi: 10.1017/wet.2017.20
Cornelius, C. D., and Bradley, K. W. (2017b). Influence of various cover crop species on winter and summer annual weed emergence in soybean. Weed Tech. 31, 503–513. doi: 10.1017/wet.2017.23
DeSimini, S. A., Gibson, K. D., Armstrong, S. D., Zimmer, M., Maia, L. O. R., and Johnson, W. G. (in press). Effects of cereal rye canola on winter summer annual weed emergence in corn. Weed Tech. 1–7. doi: 10.1017/wet.2020.51
DeVore, J. D., Norsworthy, J. K., and Brye, K. R. (2013). Influence of deep tillage, a rye cover crop, and various soybean production systems on Palmer amaranth emergence in soybean. Weed Tech. 27, 263–270. doi: 10.1614/WT-D-12-00125.1
Hayden, Z. D., Brainard, D. C., Henshaw, B., and Ngoajio, M. (2012). Winter annual weed suppression in rye-vetch cover crop mixtures. Weed Tech. 26, 818–825. doi: 10.1614/WT-D-12-00084.1
Heap, I. (2020). The International Herbicide-Resistant Weed Database. Available online at: http://weedscience.org (accessed: August 19, 2020).
Loux, M. M., Dobbels, A. F., Bradley, K. W., Johnson, W. G., Young, B. G., Spaunhorst, D. J., et al. (2017). Influence of cover crops on management of amaranthus species in glyphosate- and glufosinate-resistant soybean. Weed Tech. 31, 487–495. doi: 10.1017/wet.2017.30
Mirsky, S. B., Curran, W. S., Mortensen, D. M., Ryan, M. R., and Shumway, D. L. (2011). Timing of cover-crop management effects on weed suppression in no-till planted soybean using a roller-crimper. Weed Sci. 59, 380–389. doi: 10.1614/WS-D-10-00101.1
Mirsky, S. B., Spargo, J. T., Curran, W. S., Reberg-Horton, S. C., Ryan, M. R., Schomberg, H. H., et al. (2017). Characterizing cereal rye biomass and allometric relationships across a range of fall available nitrogen rates in the eastern United States. Agron. J. 109, 1520–1531. doi: 10.2134/agronj2016.09.0557
Mischler, R. A., Curran, W. S., Duiker, S. W., and Hyde, J. A. (2010). Use of a rolled-rye cover crop for weed suppression in no-till soybeans. Weed Tech. 24, 253–261. doi: 10.1614/WT-D-09-00004.1
Mohler, C. L., and Teasdale, J. R. (1993). Response of weed emergence to rate of Vicia villosa Roth and Secale cereale L. residue. Weed Res. 33, 487–499.
Montgomery, G. B., McClure, A. T., Hayes, R. M., Walker, F. R., Senseman, S. A., and Steckel, L. E. (2018). Dicamba-tolerant soybean combined cover crop to control Palmer amaranth. Weed Tech. 32, 109–115. doi: 10.1017/wet.2017.96
Norsworthy, J. K., Ward, S. M., Shaw, D. R., Llewellyn, R. S., Nichols, R. L., Webster, T. M., et al. (2012). Reducing the risks of herbicide resistance: best management practices and recommendations. Weed Sci. 60, 31–62. doi: 10.1614/WS-D-11-00155.1
Perkins, C. M., Gage, K. A., Norsworthy, J. K., Young, B. D., Bradley, K. W., and Bish, M. D. (in press). Efficacy of residual herbicides influenced by cover crop residue for control of Amaranthus spp. in soybean. Weed Tech. 1–20. doi: 10.1017/wet.2020.77.
Pittman, K., Barney, J., and Flessner, M. (2019). Horseweed (Conyza canadensis) suppression from cover crop mixtures and fall-applied residual herbicides. Weed Tech. 33, 303–311. doi: 10.1017/wet.2018.111
Pittman, K. B., Barney, J. N., and Flessner, M. L. (2020). Cover crop residue components and their effect on summer annual weed suppression in corn and soybean. Weed Sci. 68, 301–310. doi: 10.1017/wsc.2020.16
Poffenbarger, H. J., Mirsky, S. B., Weil, R. R., Kramer, M., Spargo, J. T., and Cavigelli, M. J. (2015). Legume proportion, poultry litter, and tillage effects on cover crop decomposition. Agron. J. 107, 2083–2096. doi: 10.2134/agronj15.0065
Reberg-Horton, S. C., Burton, J. D., Danehower, D. A., Ma, G., Monks, D. W., Murphy, J. P., et al. (2005). Changes over time in the allelochemical content of ten cultivars or rye (Secale cereal L.). J. Chem Ecol. 31, 179–193 doi: 10.1007/s10886-005-0983-3
Ryan, M. R., Curran, W. S., Grantham, A. M., Hunsberger, L. K., Mirsky, S. B., Mortensen, D. A., et al. (2011a). Effects of seeding rate and poultry litter on weed suppression from a rolled cereal rye cover crop. Weed Sci. 59, 438–444. doi: 10.1614/WS-D-10-00180
Ryan, M. R., Mirsky, S. B., Mortensen, D. A., Teasdale, J. R., and Curran, W. S. (2011b). Potential synergistic effects on cereal rye biomass and soybean planting density on weed suppression. Weed Sci. 59, 238–246. doi: 10.1614/WS-D-10-00110.1
Sherman, A. D., Haramoto, E. R., and Green, J. D. (2020). Integrating fall and spring herbicides with a cereal rye cover crop for horseweed (Conyza canadensis) management prior to soybean. Weed Tech. 34, 64–72. doi: 10.1017/wet.2019.116
Sievers, T., and Cook, R. L. (2018). Aboveground and root decomposition of cereal rye and hairy vetch cover crops. Soil. Sci. Soc. Am. J. 82, 14–155. doi: 10.2136/sssaj2017.05.0139
Teasdale, J. R., and Mohler, C. L. (1993). Light transmittance, soil temperature, and soil moisture under residue of hairy vetch and rye. Agron. J. 85, 673–680. doi: 10.2134/agronj1993.00021962008500030029x
Teasdale, J. R., and Mohler, C. L. (2000). The quantitative relationship between weed emergence and the physical properties of mulches. Weed Sci. 48, 385–392. doi: 10.1614/0043-1745(2000)048[0385:TQRBWE]2.0.CO;2
Teasdale, J. R., Rice, C. P., Cai, G., and Mangum, R. W. (2012). Expression of allelopathy in the soil environment: soil concetration and activity of benzoxazinoid compounds released by rye cover crop residue. Plant. Ecol. 213, 1893–1905 doi: 10.1007/s11258-012-0057-x
Wagger, M. G. (1989). Time of desiccation effects on plant composition and subsequent nitrogen release from several winter annual cover crops. Agron. J. 8, 236–241. doi: 10.2134/agronj1989.00021962008100020020x
Wagger, M. G., Cabrera, M. L., and Ranells, N. N. (1998). Nitrogen and carbon cycling in relation to cover crop residue quality. J. Soil Water Conserv. 53, 214–218
Wallace, J., Curran, W., and Mortensen, D. (2019). Cover crop effects on horseweed (Erigeron canadensis) density and size inequality at the time of herbicide exposure. Weed Sci. 67, 327–328. doi: 10.1017/wsc.2019.3
Whalen, D. M., Shergill, L. S., Kinne, L. P., Bish, M. D., and Bradley, K. W. (2020). Integration of residual herbicides with cover crop termination in soybean. Weed Tech. 34, 11–18. doi: 10.1017/wet.2019.111
Wiggins, M. S., Hayes, R. M., and Steckel, L. E. (2016). Evaluating cover crops and herbicides for glyphosate-resistant Palmer amaranth (Amaranthus palmeri) control in cotton. Weed Tech. 30, 415–422. doi: 10.1614/WT-D-15-00113.1
Keywords: Amaranthus palmeri, Erigeron canadensis, herbicide resistance, integrated weed management, Ipomoea spp. weed suppression, winter annual weeds
Citation: Vollmer KM, VanGessel MJ, Johnson QR and Scott BA (2020) Influence of Cereal Rye Management on Weed Control in Soybean. Front. Agron. 2:600568. doi: 10.3389/fagro.2020.600568
Received: 30 August 2020; Accepted: 24 November 2020;
Published: 17 December 2020.
Edited by:
Karla Leigh Gage, Southern Illinois University, United StatesReviewed by:
Jatinder Aulakh, Connecticut Agricultural Experiment Station, United StatesIlias Travlos, Agricultural University of Athens, Greece
Copyright © 2020 Vollmer, VanGessel, Johnson and Scott. This is an open-access article distributed under the terms of the Creative Commons Attribution License (CC BY). The use, distribution or reproduction in other forums is permitted, provided the original author(s) and the copyright owner(s) are credited and that the original publication in this journal is cited, in accordance with accepted academic practice. No use, distribution or reproduction is permitted which does not comply with these terms.
*Correspondence: Mark J. VanGessel, bWp2QHVkZWwuZWR1