- 1Section of Soil and Crop Sciences, School of Integrative Plant Science, Cornell University, Ithaca, NY, United States
- 2Department of Plant Protection, College of Agriculture and Biotechnology, Zhejiang University, Hangzhou, China
- 3Agroécologie, AgroSup Dijon, INRAE, Univ. Bourgogne, Univ. Bourgogne-Franche-Comté, Dijon, France
Agricultural impacts of climate change include direct effects on crop plants and indirect effects, such as changes to the distributions and competitiveness of weed species. In the northeastern United States, warming temperatures are likely to result in periods of soil moisture deficit and changes to weed communities. Ivyleaf morningglory (IMG, Ipomoea hederacea Jacq.) is a summer annual vine that competes with field crops and interferes with harvesting. Climate change may increase the competitive effects of IMG on northeastern U.S. field crops. We conducted a greenhouse study to evaluate the effects of IMG on corn (Zea mays L.) and soybean [Glycine max (L.) Merr.] under drought and non-drought conditions. The drought treatment was crossed against an IMG competition treatment with five levels: one crop plant without IMG plants, one crop plant with one, two, or three IMG plants, and one IMG plant without crop plants. Both drought and IMG (presence or biomass) reduced the biomass of corn and soybean (P < 0.05). Drought and IMG (presence) reduced soybean pod production (P < 0.001). IMG biomass was reduced by drought and the presence of corn (P < 0.001). Across all competition treatments, drought reduced IMG biomass by 71% in the corn experiment and 79% in the soybean experiment, compared with a corn biomass reduction of 50% and a soybean biomass reduction of 58%. Well-designed management programs should mitigate the risks associated with stressors such as IMG and drought, which may threaten northeastern U.S. field crop production under climate change.
Introduction
The impacts of climate change on agricultural weeds include shifts in weed species distributions and weed-crop competition dynamics (McDonald et al., 2009; Peters et al., 2014). The coexistence of these two response mechanisms can complicate efforts to predict future weed management challenges. Prediction efforts are important, especially given the likelihood that some agricultural weeds will thrive under novel conditions. As regional climates change, weeds may benefit from high plasticity (Baker, 1974) and the potential for rapid microevolution (Clements and DiTommaso, 2011; Ziska et al., 2019). The northeastern United States may be particularly vulnerable to worsening weed problems because this region is expected to experience a significant warming trend contributing to periods of soil moisture deficit (USGCRP, 2017). These climatic changes will have direct effects on major agricultural crops (Wolfe et al., 2018) and alter their associated weed communities (McDonald et al., 2009).
Ivyleaf morningglory (IMG, Ipomoea hederacea Jacq.) is a summer annual vine with a taproot, long running and climbing stems (up to 3 m), and funnel-shaped flowers (University of Missouri Division of Plant Sciences, 2020). The common name “ivy leaf morningglory” often distinguishes I. hederacea with three-lobed leaves (a distinctive ivy-like shape) from an otherwise similar form of the same species, “entire leaf morningglory” (Elmore, 1986). IMG is distributed across much of the continental United States (Kartesz, 2015; USDA NRCS, 2021), but its impacts vary by region. IMG and other morningglories (Ipomoea spp.) are consistently troublesome weeds of field crops in relatively warm areas, including mid-Atlantic and especially southern U.S. states (Webster and Coble, 1997; Riar et al., 2013; Teasdale et al., 2019). In southern states, IMG and other morningglories have been collectively identified as the most troublesome weed of corn (Zea mays L.) (Webster and Nichols, 2012). The use of glyphosate-tolerant crop cultivars may increase the prevalence of morningglories relative to weed species that are more easily controlled with glyphosate (Culpepper, 2006; Reddy and Norsworthy, 2010). When not adequately controlled, IMG plants often climb crop plants (Price and Wilcut, 2007) and reduce crop yield through interference with harvesting in addition to competition for resources (Wilson and Cole, 1966; Cordes and Bauman, 1984; Keeley et al., 1986). Morningglories including IMG are also present in the northeastern United States (USDA NRCS, 2021) but are not a major cause of yield losses in this region.
IMG might pose an increasing threat to northeastern U.S. field crops as the regional climate changes. The effects of climate factors on weed-crop competition vary with numerous weed and crop characteristics, such as photosynthetic pathway. IMG and soybean [Glycine max (L.) Merr.] both photosynthesize through the C3 pathway, whereas corn uses the C4 pathway. In general, C3 species are more likely to benefit from increased CO2 (Ziska et al., 2019). The C4 pathway promotes water use efficiency relative to the ancestral C3 pathway (Ehleringer and Monson, 1993), although its presence does not necessarily predict the bioclimatic or damage niches of individual species. Another relevant characteristic is the ability to adapt to changing conditions. Chaney and Baucom (2012) reported that tall morningglory [Ipomoea purpurea (L.) Roth] exhibited at least 9 of the 12 “ideal weed” traits proposed by Baker (1974), including tolerance and plasticity, rapid growth to flowering, and high seed output. Such traits are likely to promote fitness in novel or variable environments.
Among environmental factors sensitive to climate change, water availability exerts a particularly important influence on weed-crop competition (Korres et al., 2016; Kaur et al., 2018). Field studies have found that water availability can affect soybean yield losses due to morningglories. Some of these studies have suggested that yield losses due to morningglories are more likely or larger in drier years (Howe and Oliver, 1987; Holloway and Shaw, 1995). However, Mosier and Oliver (1995) found that soybean yield losses due to entire leaf morningglory did not decrease under irrigation. The authors also reported that soybean growing with entire leaf morningglory and common cocklebur (Xanthium strumarium L.) extracted water from greater soil depths than soybean growing alone. A greenhouse study showed that IMG plants used at least as much water as soybean plants when grown separately (Holloway and Shaw, 1996). Both IMG and soybean were inhibited by intraspecific or interspecific competition. Although these reports all provide useful insights, they do not fully explain how interspecific competition and water availability jointly affect the growth of field crops and morningglories. Understanding these dynamics might require identifying subtle main or interactive effects. If so, greenhouse experiments could reveal trends that are easily obscured by greater variability in field experiments.
We conducted a greenhouse study to test the effects of IMG and drought on corn and soybean. Because IMG causes significant yield losses in other regions, we hypothesized that competition from IMG would reduce crop height and biomass. We also expected crop growth to be reduced by drought, which might exacerbate the effects of IMG (i.e., interact synergistically). Lastly, we anticipated that IMG biomass would decrease under drought or interspecific competition, relative to an IMG monocrop in the non-drought treatment.
Materials and Methods
Growing Conditions
Corn, soybean, and IMG were grown in a greenhouse at Cornell University, Ithaca, New York, USA, from March 1st to May 9th, 2014. Natural light was supplemented with 400 W high pressure sodium lamps (15 h light: 9 h dark). Temperature and relative humidity averaged 25°C and 75%, respectively. Plants were grown in 11.4 L pots in standard Cornell Soil Mix (peat moss, perlite, vermiculite, lime, uni-mix). The corn variety was GROWMARK FS38R08SS, an early maturity silage hybrid. Soybean was a GROWMARK HiSOY Group I maturity variety. IMG seeds were collected in autumn 2013 from a corn field in Delaware, USA. Corn seeds were planted at 5 cm depth and soybean seeds at 3.8 cm depth. IMG seeds were germinated in petri dishes 2 days before planting and planted at 1.3 cm depth. The plants were irrigated with tap water except at 20 and 40 days after planting (DAP) when pots received 1.0 L of 200 ppm N-P-K 10-10-10 diluted at 1:100.
Experimental Procedure
We conducted an experiment on IMG-corn competition and an experiment on IMG-soybean competition. The two experiments were conducted simultaneously in one greenhouse. Each experiment followed a randomized complete block design with six blocks (replicates). Each block consisted of 10 pots representing all combinations of five competition treatments by two water treatments. The five competition treatments involved different combinations of crop (R) and weed (W) plants: one crop plant without IMG plants (1R0W), one crop plant with one IMG plant (1R1W), one crop plant with two IMG plants (1R2W), one crop plant with three IMG plants (1R3W), and one IMG plant without crop plants (0R1W).
Within each block and competition treatment, one pot was assigned to the non-drought treatment and the other pot was assigned to the drought treatment. Pots in the non-drought treatment were watered whenever the topsoil dried out. For the drought treatment in the corn experiment, water was suspended on April 14th, 2014, the 44th day after planting (DAP). Wilting was soon observed (April 16th, 2014). The weight of each pot was measured on the day of wilting. Plants were not provided with water until the 5th day after wilting. On the 5th day after wilting, we measured pot weights again and calculated the amount of water loss that had occurred since wilting. Enough water was added to pots with one corn plant and no IMG (1R0W) to restore the weights measured on the day of wilting. The same amount of water added to pots in the 1R0W treatment was added to pots in other competition treatments on a block-by-block basis. The process was repeated three times, after which the experiment was terminated (15th day after wilting). The drought treatment was imposed similarly in the soybean experiment. Water was suspended on April 14th, 2014 (44 DAP) and wilting of most plants was observed on April 20th, 2014. On the 5th day after wilting, enough water was added to the pots with one soybean plant and no IMG (1R0W) to restore pot weights to the weights measured on the day of wilting. The same amount of water added to pots in the 1R0W treatment was added to pots in other competition treatments on a block-by-block basis. The process was repeated twice in the soybean experiment, after which the experiment was terminated (10th day after wilting). In both experiments, plants were vegetative when drought was initiated and did not reach physiological maturity before the experiments were terminated.
The heights of corn and soybean plants were measured at the end of the experiments. The aboveground biomass of all plants was harvested for dry weight measurements (65°C for 48 h). When multiple IMG plants were grown in the same pot, they were harvested and weighed together. Seed pods on IMG and soybean plants were counted.
Data Analysis
Data analysis was performed in R (4.1.0, R Core Team, 2021). Data from the corn and soybean experiments were analyzed separately. We evaluated factors influencing crop height, crop dry biomass, and soybean pod production using analysis of variance (ANOVA) on linear mixed models with drought, IMG, and their interaction as fixed effects and replicate as a random effect (packages “lme4”, “lmerTest”). We created three sets of models of this form. In the first set of models, the fixed effect of IMG represented the presence or absence of one IMG plant (i.e., the difference between 1R0W and 1R1W). Competition treatments with two or three IMG plants were not included in these models. We used the same method to test the effects of drought, IMG presence, and their interaction on soybean pod number. In the second set of models, we tested the effect of IMG dry biomass on crop height and crop biomass. All competition treatments except 0R1W were included in these models. Lastly, we tested the effects of drought, IMG density (1R1W, 1R2W, or 1R3W), and their interaction on soybean pod number. The 0R1W and 1R0W competition treatments were excluded from this model.
We tested influences on IMG growth and pod production with two sets of models, continuing to separate the corn and soybean experiments. First, we tested the fixed effects of drought, crop, and their interaction, with replicate as a random effect, on IMG dry biomass and pod number. The fixed effect of crop represented the presence or absence of one crop plant (i.e., the difference between 0R1W and 1R1W). Competition treatments with two or three IMG plants were not included in these models. Second, we tested the effects of drought, IMG density (1R1W, 1R2W, or 1R3W), and their interaction on IMG biomass. The 0R1W and 1R0W competition treatments were excluded from these models.
Logarithmic transformations were applied to all response variables [ln(x) for height and biomass; log10(x + 1) for pod production].
Pairwise differences between treatments were evaluated with Tukey's Honest Significant Difference (HSD) test (α = 0.05, package “agricolae”). Biomass reductions due to drought were calculated for each drought/non-drought pair (competition treatment by replicate, untransformed data), then averaged across all pairs within species.
Results
Drought and IMG reduced crop growth. Effect sizes varied with crop species and growth parameter. Comparisons between individual crop plants (1R0W) and crop plants competing with one IMG plant (1R1W) showed that drought reduced corn height (P < 0.001, Figure 1A) and the presence of IMG reduced soybean height (P < 0.01, Figure 1B). Both corn and soybean produced more biomass in the absence of drought and IMG than in the presence of either stressor (Figures 1C,D). In addition to the significant negative effects of drought and IMG on crop biomass (P < 0.001), we observed an antagonistic drought by IMG interaction on crop biomass in both species (P < 0.05). Across all IMG densities, crop biomass was higher under non-drought conditions and negatively associated with IMG biomass (Figure 2). Both effects were significant (P < 0.05) but there was no drought by IMG interaction. Likewise, drought reduced crop height (P < 0.01) but did not interact with IMG biomass to affect crop height.
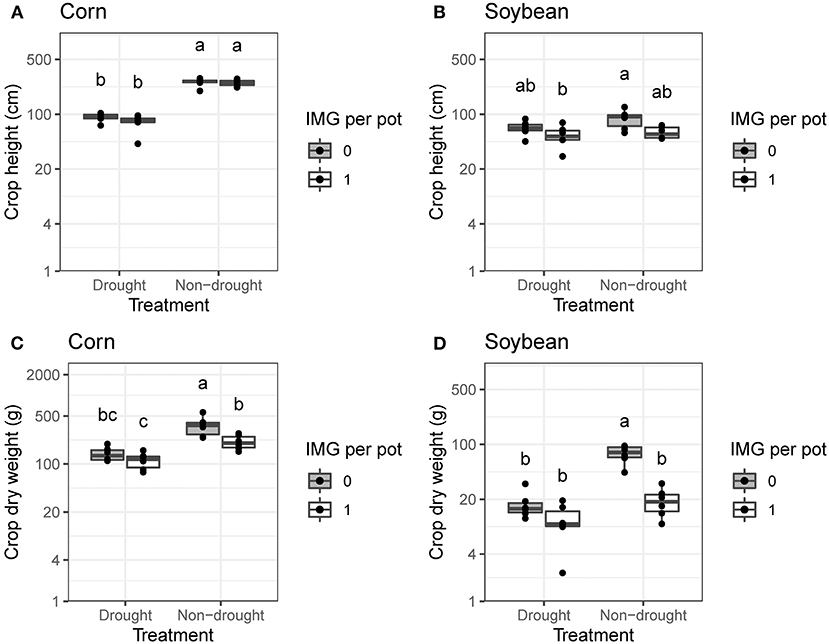
Figure 1. Effects of drought treatment and ivyleaf morningglory (IMG) on crop height and biomass under greenhouse conditions. Pots with a single crop plant (1R0W) are compared to pots with one crop plant and one IMG plant (1R1W). Data are presented for (A) corn height, (B) soybean height, (C) corn biomass, and (D) soybean biomass. Within crop species and growth parameter, treatments labeled with the same letter are not significantly different according to Tukey's HSD test (α = 0.05).
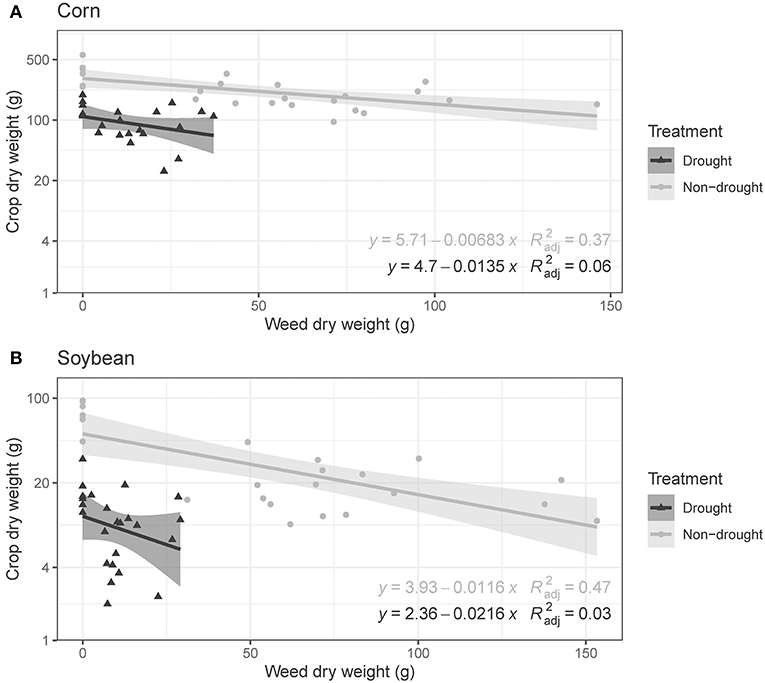
Figure 2. Effects of drought treatment and ivyleaf morningglory (IMG) biomass on crop biomass under greenhouse conditions. Data are presented for (A) corn and (B) soybean. Fitted lines represent linear regressions with 95% confidence intervals (drought in black and non-drought in gray). In regression equations, x is IMG biomass and y is the natural log of crop biomass.
In the corn experiment, we observed significant negative effects of drought and corn (1R1W vs. 0R1W) on IMG biomass (P < 0.001, Figure 3A). Drought also reduced IMG biomass in the soybean experiment (P < 0.001, Figure 3B). Under drought conditions, 0R1W and 1R1W treatments did not differ in either experiment (Figure 3). When IMG and a crop were both present (1R1W, 1R2W, and 1R3W), IMG biomass was reduced by drought and increasing IMG density (P < 0.05). Across all competition treatments, the mean IMG biomass reduction due to drought was 71% in the corn experiment and 79% in the soybean experiment, compared with a corn biomass reduction of 50% and a soybean biomass reduction of 58%.
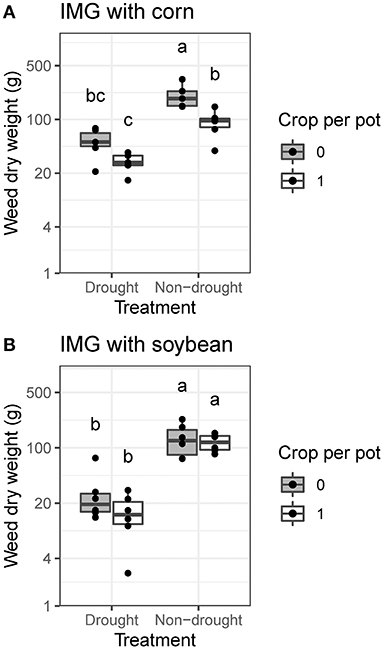
Figure 3. Effects of drought treatment and crop competition on ivyleaf morningglory (IMG) biomass under greenhouse conditions. Pots with a single IMG plant (0R1W) are compared to pots with one crop plant and one IMG plant (1R1W). Data are presented for IMG grown with (A) corn and (B) soybean. Within crop species, treatments labeled with the same letter are not significantly different according to Tukey's HSD test (α = 0.05).
Neither drought nor competition against corn (1R1W vs. 0R1W) affected IMG pod production (Figure 4A). Competition against soybean reduced IMG pod production (P < 0.01), but pairwise differences between competition treatments were not significant within drought treatments (Figure 4B). Conversely, competition against IMG (1R1W vs. 1R0W) had a strong negative effect on soybean pod production (P < 0.001), which was also reduced by drought (P < 0.001). Soybean pod production was highest in the non-drought monocrop (1R0W) treatment (Figure 4C). When IMG and soybean were both present (1R1W, 1R2W, and 1R2W), soybean pod production was reduced by drought (P < 0.01) but unaffected by increasing IMG density.
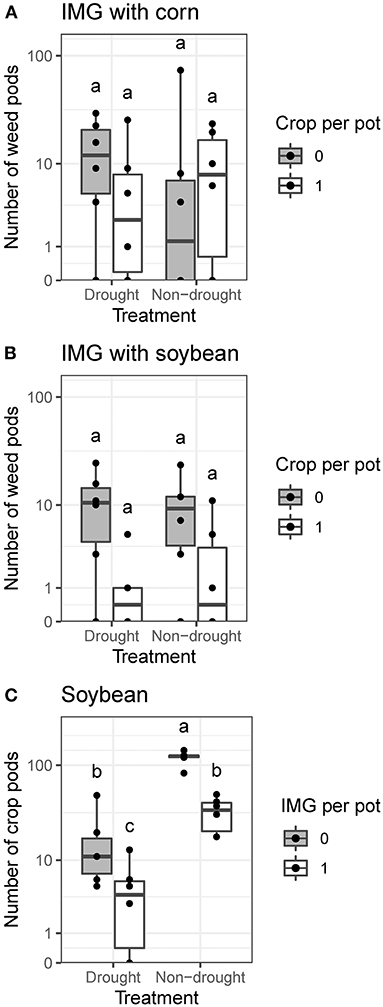
Figure 4. Effects of drought treatment and corn, soybean, or ivyleaf morningglory (IMG) on the number of IMG or soybean pods produced under greenhouse conditions. (A) For IMG pod production, pots with a single IMG plant (0R1W) are compared to pots with one corn plant and one IMG plant (1R1W). (B) For IMG pod production, pots with a single IMG plant (0R1W) are compared to pots with one soybean plant and one IMG plant (1R1W). (C) For soybean pod production, pots with a single soybean plant (1R0W) are compared to pots with one soybean plant and one IMG plant (1R1W). Within species combination, treatments labeled with the same letter are not significantly different according to Tukey's HSD test (α = 0.05).
Discussion
In agreement with our first and second hypotheses, IMG and drought reduced corn and soybean growth. The negative effects of IMG on crop growth probably reflected competition for resources. In a 2-year field experiment near Ithaca, New York, USA, IMG did not substantially reduce corn silage yield under either drought or non-drought conditions (Averill et al., unpublished data). Discrepancies between greenhouse and field results highlight the importance of using multiple experimental methods to investigate the mechanisms and outcomes of weed-crop competition. The negative effects of drought on crop growth are consistent with the fact that corn and soybean yields are sensitive to drought (Zipper et al., 2016; Matiu et al., 2017; Sweet et al., 2017). In this experiment, drought effects on crop biomass were generally similar between corn and soybean. However, an experiment that tracked crop water uptake might have revealed differences in these species' water use patterns. For example, corn can use water more efficiently than soybean (Dietzel et al., 2016). Crop water productivity (the ratio of crop yield to evapotranspiration) is likely to increase with increasing CO2, especially in C3 crops like soybean (Deryng et al., 2016).
Contrary to our second hypothesis, IMG and drought did not have synergistic effects on crop growth. This finding suggests that drought did not increase the competitive effects of IMG. IMG did not appear to be strongly drought resistant: drought reduced IMG biomass by >70% and crop biomass by <60%. However, it is worth noting that the outcomes of drought experiments can be highly sensitive to methodology (e.g., the timing, severity, and duration of drought periods). Some previous research indicated that vegetative growth of tall morningglory was not strongly sensitive to drought (Mason et al., 2015). The discrepancy between our study and this previous research could reflect a difference in experimental procedure. Mason et al. (2015) rewatered pots to field capacity when wilting was observed, whereas we imposed a waiting period and then rewatered pots only to wilting point. An interspecific difference is also possible, although the roots of IMG seedlings are morphologically similar to the roots of tall morningglory seedlings (Colom and Baucom, 2020).
Other research has demonstrated that morningglories may compete with crops for water. In a greenhouse study, Holloway and Shaw (1996) tested the effects of selective herbicides on IMG, soybean, or a mixture of both species. When applied to IMG or species mixtures, the herbicide treatments reduced water use by reducing or eliminating IMG water consumption. Similarly, chemical control of pitted morningglory (Ipomoea lacunosa L.) promoted soybean water consumption in the field (Norsworthy and Oliver, 2002a). Some fieldwork (Holloway and Shaw, 1995) has suggested an inverse association between water availability and the competitive effects of morningglories, although this trend is not universal (Mosier and Oliver, 1995). In the context of these various studies, our findings suggest that competition for water may have contributed to reductions in crop growth due to IMG.
Competition for nutrients might also have contributed to the competitive effects of IMG. However, one study of competition in an old field formerly used for corn suggested that IMG was a poor competitor for nitrogen (Whigham, 1984). A study of competition between tall morningglory and soybean showed continued expansion of the tall morningglory root system during the soybean reproductive phase (Scott and Oliver, 1976). This root growth could increase late-season competitiveness for water and nutrients.
In partial agreement with our third hypothesis, drought and the presence of corn reduced IMG biomass. In the presence of a crop, IMG biomass also decreased with increasing IMG density, suggesting that IMG suffered from resource limitation. These findings are consistent with numerous field studies demonstrating that crops can help suppress weeds, including morningglories (Jha et al., 2017). For example, Keeley et al. (1986) reported that April or May emergence of IMG could result in the loss of an entire cotton (Gossypium hirsutum L.) crop, but IMG planted after June was shaded by the cotton canopy and did not reduce yield. Shading could depend on crop planting pattern, although Murdock et al. (1986) found limited evidence that soybean row width (uniform density) affected competition between pitted morningglory and soybean. In the absence of herbicides, pitted morningglory growth could be reduced by an increase in soybean density (Norsworthy and Oliver, 2002a). Pitted morningglory seed production may vary with soybean density (Norsworthy and Oliver, 2002b) or remain constant (Norsworthy and Oliver, 2002a). Pitted morningglory seed production can also remain constant across irrigation treatments if water is not limiting or a tolerable moisture stress is offset by reduced competition from soybean (Norsworthy and Oliver, 2002a). Pitted morningglory seed production sometimes varies with soybean cultivar (e.g., height and maturity group, Bennett and Shaw, 2000). In our study, IMG pod production tended to decrease under competition against soybean, whereas soybean pod production was reduced by drought and competition against IMG.
These experiments demonstrate significant negative effects of drought and competition against IMG on corn and soybean. Drought and competition treatments did not consistently interact. However, we did observe some interactions and do not reject the possibility that others would emerge under field conditions. Our results also indicate that corn may help suppress IMG, suggesting that crop competitiveness could be an important component of IMG management. This hypothesis should be explored with field studies that update and build on older work. As agricultural practices and climate factors continue to change, continued research on weed-crop competition will guide the development of resilient cropping systems.
Data Availability Statement
The raw data supporting the conclusions of this article will be made available by the authors, without undue reservation.
Author Contributions
AD designed the study and funded the project. RH and JZ collected the data. SC and AW analyzed the data. AW, AD, RH, JZ, and SC were involved in interpreting the results, contributed to the writing of the original version of the manuscript, and improved subsequent versions. All authors contributed to the article and approved the submitted version.
Funding
This work was supported by the USDA National Institute of Food and Agriculture, Hatch project #1004532. Funding support from the Weed Science Society of America Undergraduate Research program to RH is also acknowledged. The collaboration with SC (INRAE) was initiated during his visiting stay at Cornell University, funded by INRAE and the INRAE department Environnement et Agronomie.
Conflict of Interest
The authors declare that the research was conducted in the absence of any commercial or financial relationships that could be construed as a potential conflict of interest.
Acknowledgments
Assistance provided by Scott Morris, Javaid Iqbal, and Zhoucen Feng with the experiment is gratefully acknowledged.
References
Baker, H. G. (1974). The evolution of weeds. Annu. Rev. Ecol. Evol. Syst. 5, 1–24. doi: 10.1146/annurev.es.05.110174.000245
Bennett, A. C., and Shaw, D. R. (2000). Effect of Glycine max cultivar and weed control on weed seed characteristics. Weed Sci. 48, 431–435. doi: 10.1614/0043-1745(2000)048<0431:EOGMCA>2.0.CO;2
Chaney, L., and Baucom, R. S. (2012). The evolutionary potential of Baker's weediness traits in the common morningglory, Ipomoea purpurea (Convolvulaceae). Am. J. Bot. 99, 1524–1530. doi: 10.3732/ajb.1200096
Clements, D. R., and DiTommaso, A. (2011). Climate change and weed adaptation: can evolution of invasive plants lead to greater range expansion than forecasted? Weed Res. 51, 227–240. doi: 10.1111/j.1365-3180.2011.00850.x
Colom, S. M., and Baucom, R. S. (2020). Belowground competition can influence the evolution of root traits. Am. Nat. 195, 577–590. doi: 10.1086/707597
Cordes, R. C., and Bauman, T. T. (1984). Field competition between ivyleaf morningglory (Ipomea hederacea) and soybeans (Glycine max). Weed Sci. 32, 364–370. doi: 10.1017/S0043174500059142
Culpepper, A. S. (2006). Glyphosate-induced weed shifts. Weed Technol. 20, 277–281. doi: 10.1614/WT-04-155R.1
Deryng, D., Elliott, J., Folberth, C., Müller, C., Pugh, T. A. M., Boote, K. J., et al. (2016). Regional disparities in the beneficial effects of rising CO2 concentrations on crop water productivity. Nat. Clim. Chang. 6, 786–790. doi: 10.1038/nclimate2995
Dietzel, R., Liebman, M., Ewing, R., Helmers, M., Horton, R., Jarchow, M., et al. (2016). How efficiently do corn- and soybean-based cropping systems use water? a systems modeling analysis. Glob. Chang. Biol. 22, 666–681. doi: 10.1111/gcb.13101
Ehleringer, J. R., and Monson, R. K. (1993). Evolutionary and ecological aspects of photosynthetic pathway variation. Ann. Rev. Ecol. Syst. 24, 411–439. doi: 10.1146/annurev.es.24.110193.002211
Elmore, C. D. (1986). Mode of reproduction and inheritance of leaf shape in Ipomoea hederacea. Weed Sci. 34, 391–395. doi: 10.1017/S0043174500067047
Holloway, J. C. Jr., and Shaw, D. R. (1995). Influence of soil-applied herbicides on ivyleaf morningglory (Ipomoea hederacea) growth and development in soybean (Glycine max). Weed Sci. 43, 655–659. doi: 10.1017/S0043174500081789
Holloway, J. C. Jr., and Shaw, D. R. (1996). Herbicide effects on ivyleaf morningglory (Ipomoea hederacea) and soybean (Glycine max) growth and water relations. Weed Sci. 44, 836–841. doi: 10.1017/S0043174500094790
Howe, O. W., and Oliver, L. R. (1987). Influence of soybean (Glycine max) row spacing on pitted morningglory (Ipomoea lacunosa) interference. Weed Sci. 35, 185–193. doi: 10.1017/S0043174500079030
Jha, P., Kumar, V., Godara, R. K., and Chauhan, B. S. (2017). Weed management using crop competition in the United States: a review. Crop Prot. 95, 31–37. doi: 10.1016/j.cropro.2016.06.021
Kartesz J. T. The Biota of North America Program. (2015). North American Plant Atlas. Available online at: http://bonap.net/napa (Accessed March 3, 2021).
Kaur, S., Kaur, R., and Chauhan, B. S. (2018). Understanding crop-weed-fertilizer-water interactions and their implications for weed management in agricultural systems. Crop Prot. 103, 65–72. doi: 10.1016/j.cropro.2017.09.011
Keeley, P. E., Thullen, R. J., and Carter, C. H. (1986). Influence of planting date on growth of ivyleaf morningglory (Ipomoea hederacea) in cotton (Gossypium hirsutum). Weed Sci. 34, 906–910. doi: 10.1017/S0043174500068089
Korres, N., Norsworthy, J., Tehranchian, P., Gitsopoulos, T., Loka, D., Oosterhuis, D., et al. (2016). Cultivars to face climate change effects on crops and weeds: a review. Agron. Sustain. Dev. 36:12. doi: 10.1007/s13593-016-0350-5
Mason, C. M., Christopher, D. A., Rea, A. M., Eserman, L. A., Pilote, A. J., Batora, N. L., et al. (2015). Low inbreeding depression and high plasticity under abiotic stress in the tall morningglory (Ipomoea purpurea). Weed Sci. 63, 864–876. doi: 10.1614/WS-D-15-00005.1
Matiu, M., Ankerst, D. P., and Menzel, A. (2017). Interactions between temperature and drought in global and regional crop yield variability during 1961-2014. PLoS ONE. 12:e0178339. doi: 10.1371/journal.pone.0178339
McDonald, A., Riha, S., DiTommaso, A., and DeGaetano, A. (2009). Climate change and the geography of weed damage: analysis of U.S. maize systems suggests the potential for significant range transformations. Agric. Ecosyst. Environ. 130, 131–140. doi: 10.1016/j.agee.2008.12.007
Mosier, D. G., and Oliver, L. R. (1995). Common cocklebur (Xanthium strumarium) and entireleaf morningglory (Ipomoea hederacea var. integriuscula) interference on soybeans (Glycine max). Weed Sci. 43, 239–246. doi: 10.1017/S0043174500081133
Murdock, E. C., Banks, P. A., and Toler, J. E. (1986). Shade development effects on pitted morningglory (Ipomoea lacunosa) interference with soybeans (Glycine max). Weed Sci. 34, 711–717. doi: 10.1017/S0043174500067734
Norsworthy, J. K., and Oliver, L. R. (2002a). Effect of irrigation, soybean (Glycine max) density, and glyphosate on hemp sesbania (Sesbania exaltata) and pitted morningglory (Ipomoea lacunosa) interference in soybean. Weed Technol. 16, 7–17. doi: 10.1614/0890-037X(2002)016<0007:EOISGM>2.0.CO;2
Norsworthy, J. K., and Oliver, L. R. (2002b). Pitted morningglory interference in drill-seeded glyphosate-resistant soybean. Weed Sci. 50, 26–33. doi: 10.1614/0043-1745(2002)050<0026:PMIIDS>2.0.CO;2
Peters, K., Breitsameter, L., and Gerowitt, B. (2014). Impact of climate change on weeds in agriculture: a review. Agron. Sustain. Dev. 34, 707–721. doi: 10.1007/s13593-014-0245-2
Price, A. J., and Wilcut, J. W. (2007). Response of ivyleaf morningglory (Ipomoea hederacea) to neighboring plants and objects. Weed Technol. 21, 922–927. doi: 10.1614/W.T.-06-146.1
R Core Team (2021). R: A Language and Environment for Statistical Computing. Vienna: R Foundation for Statistical Computing. Available online at: https://www.R-project.org/
Reddy, K. N., and Norsworthy, J. K. (2010). “Glyphosate-resistant crop production systems: impact on weed species shifts,” in Glyphosate Resistance in Crops and Weeds: History, Development, and Management, ed. V. K. Nandula (Hoboken, NJ: Wiley), 165–184. doi: 10.1002/9780470634394.ch9
Riar, D. S., Norsworthy, J. K., Steckel, L. E., Stephenson, D. O., Eubank, T. W., and Scott, R. C. (2013). Assessment of weed management practices and problem weeds in the midsouth United States—soybean: a consultant's perspective. Weed Technol. 27, 612–622. doi: 10.1614/WT-D-12-00167.1
Scott, H. D., and Oliver, L. R. (1976). Field competition between tall morningglory and soybean. II. Development and distribution of root systems. Weed Sci. 24, 454–460. doi: 10.1017/S0043174500066443
Sweet, S. K., Wolfe, D. W., DeGaetano, A., and Benner, R. (2017). Anatomy of the 2016 drought in the northeastern United States: implications for agriculture and water resources in humid climates. Agric. For. Meteorol. 247, 571–581. doi: 10.1016/j.agrformet.2017.08.024
Teasdale, J. R., Mirsky, S. B., and Cavigelli, M. A. (2019). Weed species and traits associated with organic grain crop rotations in the mid-Atlantic region. Weed Sci. 67, 595–604. doi: 10.1017/wsc.2019.38
University of Missouri Division of Plant Sciences (2020). Ivyleaf Morningglory. Weed ID Guide. Available online at: https://weedid.missouri.edu/weedinfo.cfm?weed_id=138 (accessed January 11, 2021).
USDA NRCS (2021). PLANTS Database. Available online at: https://plants.usda.gov/java (accessed March 2, 2021).
USGCRP (2017). Climate Science Special Report: Fourth National Climate Assessment. Washington, DC: U.S. Global Change Research Program.
Webster, T. M., and Coble, H. D. (1997). Changes in the weed species composition of the southern United States: 1974 to 1995. Weed Technol. 11, 308–317. doi: 10.1017/S0890037X00043001
Webster, T. M., and Nichols, R. L. (2012). Changes in the prevalence of weed species in the major agronomic crops of the southern United States: 1994/1995 to 2008/2009. Weed Sci. 60, 145–157. doi: 10.1614/WS-D-11-00092.1
Whigham, D. F. (1984). The effect of competition and nutrient availability on the growth and reproduction of Ipomoea hederacea in an abandoned old field. J. Ecol. 72, 721–730. doi: 10.2307/2259527
Wilson, H. P., and Cole, R. H. (1966). Morningglory competition in soybeans. Weeds 14, 49–51. doi: 10.2307/4041122
Wolfe, D. W., DeGaetano, A. T., Peck, G. M., Carey, M., Ziska, L. H., Lea-Cox, J., et al. (2018). Unique challenges and opportunities for northeastern US crop production in a changing climate. Clim. Chang. 146, 231–245. doi: 10.1007/s10584-017-2109-7
Zipper, S. C., Qiu, J., and Kucharik, C. J. (2016). Drought effects on US maize and soybean production: spatiotemporal patterns and historical changes. Environ. Res. Lett. 11:094021. doi: 10.1088/1748-9326/11/9/094021
Keywords: annual vine, climate change, Convolvulaceae, greenhouse, soil moisture deficit, weed-crop competition
Citation: Westbrook AS, Han R, Zhu J, Cordeau S and DiTommaso A (2021) Drought and Competition With Ivyleaf Morningglory (Ipomoea hederacea) Inhibit Corn and Soybean Growth. Front. Agron. 3:720287. doi: 10.3389/fagro.2021.720287
Received: 04 June 2021; Accepted: 28 June 2021;
Published: 20 July 2021.
Edited by:
Baruch Rubin, Hebrew University of Jerusalem, IsraelReviewed by:
Niv DeMalach, Hebrew University of Jerusalem, IsraelMartin Williams, United States Department of Agriculture, United States
Copyright © 2021 Westbrook, Han, Zhu, Cordeau and DiTommaso. This is an open-access article distributed under the terms of the Creative Commons Attribution License (CC BY). The use, distribution or reproduction in other forums is permitted, provided the original author(s) and the copyright owner(s) are credited and that the original publication in this journal is cited, in accordance with accepted academic practice. No use, distribution or reproduction is permitted which does not comply with these terms.
*Correspondence: Antonio DiTommaso, YWQ5N0Bjb3JuZWxsLmVkdQ==