- 1College of Agriculture and Biotechnology, Yunnan Agricultural University, Kunming, China
- 2National-Local Joint Engineering Research Center on Germplasm Innovation & Utilization of Chinese Medicinal Materials in Southwest China, Yunnan Agricultural University, Kunming, Yunnan, China
- 3The Key Laboratory of Medicinal Plant Biology of Yunnan Province, Yunnan Agricultural University, Kunming, Yunnan, China
Pairs polyphylla var. yunnanensis is a perennial herb, and its rhizomes are used as medicine to invigorate blood circulation, dissipate blood stasis, relieve swelling and pain, clear heat and detoxify the body. However, due to its slow growth, most of the saponin content is low, while the content of saponins is higher in Paris fargesii Franch of the same genus. The isolation and screening of strains from P. fargesii Franch that promote the accumulation of saponin content in P. polyphylla var. yunnanensis is important to improve the growth rate of artificially cultivated P. polyphylla var. yunnanensis and increase its medicinal activity. Plant symbiotic strains are biologically active microorganisms that can promote the growth and development of host plants through direct probiotic effects and indirect disease resistance mechanisms. Here, we isolated four strains of symbiotes, including two bacteria, Bacillus cereus (LgD2) and Bacillus thuringiensis (LgD10), and two fungi, Aspergillus tamari (TPD11) and Fusarium oxysporum (TPB). All of them promoted the biomass, chlorophyll content and photosynthetic efficiency of P. polyphylla var. yunnanensis seedlings to different degrees and effectively stimulated the accumulation of saponin content in P. polyphylla var. yunnanensis seedlings to improve the expression of saponin synthesis pathway genes. The symbiotes also promoted the enzymatic activities of the enzymes superoxide dismutase (SOD), peroxidase (POD), polyphenol oxidase (PPO) and phenylalanine aminolase (PAL), improved the physiological indicators of tobacco plants (plant height, crown width, leaf length and leaf width), reduced the incidence of tobacco black shank, and improved the growth and resistance-related genes of tobacco. In conclusion, the four symbiotic strains TPD11, TPB, LgD10 and LgD2 play an important role in regulating the growth, promoting saponin accumulation, and disease resistance of P. polyphylla var. yunnanensis.
1 Introduction
Pairs polyphylla var. yunnanensis is a perennial herb of the genus Paris in the family Trillaceae and is used as a medicine with rhizomes to activate blood circulation and disperse blood stasis, reduce swelling and pain, cool the liver and calm the panic, clear heat and detoxify the body. P. polyphylla var. yunnanensis has anticancer and antibacterial effects (Li et al., 2014; Wen et al., 2015) and is currently the main ingredient of some important Chinese patent medicines, such as Yunnan Baiyao, Gonghening capsules, and Sichuan Baiyao (Wu et al., 2004). Steroidal saponins are the main active medicinal ingredients of bryophyllum, including polyphyllin I, polyphyllin II, polyphyllin VI, polyphyllin VII, polyphyllin D, and polyphyllin H (Zhao et al., 2009; Zhao et al., 2017). Successive generations of the Chinese Pharmacopoeia have included P. polyphylla var. yunnanensis as a traditional Chinese medicine. With the deep development of the medicinal value of P. polyphylla var. yunnanensis, market demand is rising year by year. However, due to years of slow growth and overharvesting, the regeneration of wild resources of P. polyphylla var. yunnanensis has been seriously damaged, and the current cultivation of P. polyphylla var. yunnanensis, because its long growth cycle (8-10 years) is unsatisfactory, and its saponin content is low, is often unable to meet the needs of pharmaceutical companies. However, Paris fargesii Franch is a sibling species of P. polyphylla var. yunnanensis and has a high saponin content (Wen et al., 2019). Therefore, it is important to isolate and identify symbiotic strains from P. fargesii Franch and screen them for the growth and accumulation of saponins to improve the growth rate of P. fargesii Franch. It is important to improve the growth rate and increase the accumulation of medicinal active ingredients in artificial cultivation.
Symbiotic strains are a group of microorganisms that can achieve a symbiotic relationship with plants during plant growth and in the environment, mainly including endophytes and rhizospheres. Symbiotic strains can promote plant growth through direct and indirect mechanisms (Glick, 1995): direct mechanisms are manifested in the ability of the strain to promote nutrient uptake by the host plant through its own nitrogen fixation (Pérez-Montaño et al., 2014), phosphate solubilization (Alori et al., 2017), potassium solubilization (Zhang et al., 2020) and other probiotic characteristics, providing vitamins needed for plant growth, and through the production of growth hormone-like phytohormones (Ahmed and Hasnain, 2010; Wagi and Ahmed, 2019) similar to the phytohhormones of the host plant. A symbiotic strain also promotes the accumulation of active substances in the host plant by producing some physiologically active metabolites that are the same as or similar to the metabolites of the host plant, such as paclitaxel (Stierle et al., 1993), podophyllotoxin (Eyberger et al., 2006), camptothecin (Kusari et al., 2009), huperzine (Li et al., 2007) and quercetin (Min et al., 2010); the indirect mechanism is manifested mainly in the ability of symbiotic strains to directly inhibit the growth of pathogenic bacteria (Zhang et al., 2017) and to enhance host plant biocontrol enzymes such as polyphenol oxidase (PPO), phenylalanine aminolase (PAL), superoxide dismutase (SOD) and peroxidase (POD) active enzymes (Sang et al., 2019) and the expression of related resistance genes (Sherameti et al., 2005), thus indirectly promoting plant growth.
Tobacco black shank is a serious tobacco disease caused by the soil-borne fungus Phytophthora nicotianae. The severity of tobacco black shank disease is increasing every year, causing significant economic losses. Chemical fungicides such as methomyl, carbendazim and daidzein are commonly used in the field for disease control, relying on chemicals and leaving fungicide residues causing environmental pollution. Biological control provides a safe alternative to control tobacco black shank disease (Edlayne et al., 2009; Ji et al., 2014; Guo et al., 2020; Ding et al., 2021). Currently, some plant symbiotic strains, such as Bacillus spp., Trichoderma spp. and Pseudomonas spp., have been demonstrated to be useful as biocontrol agents for the suppression of tobacco black shank disease (Cui et al., 2014; Ma et al., 2018; Guo et al., 2020). Therefore, the aim of this study was to isolate and identify symbiotic strains of P. fargesii Franch, to evaluate their growth-promoting properties and pathogenic antagonistic activity, and to identify strains that could promote the growth and saponin content accumulation of P. fargesii Franch and antagonize the tobacco black shank disease.
2 Materials and methods
2.1 Plant materials
Four-year-old P. fargesii Franch and two-year-old P. polyphylla var. yunnanensis seedlings were purchased from Wuding County, Yunnan Province, China, and samples attached to the roots were collected from the top five centimeters of soil around healthy P. fargesii Franch plants. The collected soil samples (20) were sealed in sterile polyethylene bags and transferred to ice boxes for transport. Plant and soil samples were preserved at the Yunnan Agricultural University Laboratory, with follow-up experiments. For endophyte isolation, healthy P. fargesii Franch rhizomes (20) collected from the samples described above were packed in polyethylene bags, labeled and stored in the laboratory in a 4°C refrigerator and processed within 48 h of collection. The seeds of the disease-susceptible variety of tobacco Hongda were purchased from the Yunnan Provincial Tobacco Monopoly Bureau, and the tobacco seeds were grown in seedling trays until the seedling stage and then transplanted to the field for the trial. The experimental site was in the greenhouse of Yunnan Agricultural University.
2.2 Isolation of endophytic and interrhizosphere bacteria
2.2.1 Isolation of endophytic bacteria
The harvested healthy P. fargesii Franch was rinsed with running tap water, selected healthy tissues were cut into small sections, and the surface water was blotted with filter paper. The samples were soaked in 75% ethanol for 1 min, rinsed in sterile water 3 times, soaked in effective chlorine 5% sodium hypochlorite solution for 3 min, and finally washed in sterile water 3-4 times until the disinfection solution was washed off thoroughly. The different tissues of Rhizoma Paridis were ground in a sterilized mortar, mixed with sterile water and transferred to an Eppendorf (EP) tube containing 5 ml of Luria-Bertani (LB) liquid medium; the shaker was set at 37°C and 180 rpm and shaken for 2-3 h. The suspension was then diluted to three different concentration gradients of 10-3, 10-4 and 10-5; 100 μl of the above suspension was applied to LB plate medium, and 100 μl of sterile water from the last wash was used as a control. Three replicates of each gradient were set up and incubated in an inverted incubator at 37°C for 24-48 h. When bacterial colonies grew, single colonies with different characteristics of morphology and color were selected, scraped onto LB solid culture plates with an inoculation loop for delineation, and numbered. After several rounds of delineation and purification, the isolated bacterial strains were stored at -80°C at a ratio of 1:1 bacterial solution and 50% sterilized glycerol.
2.2.2 Isolation of endophytic fungi
The freshly isolated rhizomes of P. fargesii Franch were rinsed with tap water. The samples were rinsed with 70% ethanol for 1 min, followed by 5 repeated rinses with sterile water and then disinfected with 5% NaClO for 5 min, followed by 5 repeated rinses with sterile water and blotting with sterilized filter paper to remove excess water. Finally, the rootstock was cut into small pieces with a sterilized blade. Potato dextrose agar (PDA) medium (with streptomycin) was prepared, and small segments of the roots were placed in the medium with 4 pieces of tissue in each Petri dish and incubated at a constant temperature of 30°C. Then, we inoculated 100 μl from the last wash of sterile water onto PDA plates for surface sterilization examination of the plant tissue samples. Promptly after 5-10 d of incubation, we used the tip mycelium picking method to pick morphologically different mycelia or colonies to transfer to new plates. After 5-10 d of incubation, we picked mycelia or colonies with different morphologies, transferred them to fresh PDA medium for purification, and then transferred them to agar slants for storage.
2.2.3 Isolation of interroot bacteria
The collected soil samples were sieved to remove the large hard particles and nonsoil materials. The soil suspension was made as follows: 10 g of soil was weighed and placed in a culture bottle with 90 ml sterile water, and the bottle was sealed with film. The bottle was placed in a shaker at 37°C and 180 rpm for 2 h to make a soil suspension. The bottle was removed and left for 5 min to precipitate the large particles, and 2 ml of soil suspension was pipetted into a 2.5-ml sterilized centrifuge tube with a 1000-ml pipette. After a short centrifugation, 1 ml of supernatant was taken and added to a test tube with 9 ml of sterile water, shaken back and forth 5 times, mixed well, marked as 10-2, and diluted into 10-4, 10-5, and 10-6 gradient dilutions. For the coating plate method culture, we used a 100-µl pipette, aspirated 80 µl dilution from the 4 dilution tubes, added it to LB medium, used an applicator to coat the dilution evenly on LB medium, sealed the tube, and placed it in a 30°C incubator inverted culture for 1-2 d. We repeated each gradient three times, set a blank control at the same time; waited for the bacterial colonies to grow out, selected the different morphology and color different characteristics of the single colonies, scraped the single colonies onto LB solid culture plates with an inoculation ring for scribing and numbering, respectively; after purification of the bacterium, it was placed in shaking culture with 1.5 ml of liquid LB into bacterial solution. We aspirated 1 ml of bacterial solution, 50% sterilized glycerol was added a ratio of 1:1, and the sample was stored at -80°C.
2.2.4 Isolation of interroot fungi
One gram of the raw interroot soil material was weighed for the experiment, and 90 ml of sterile water was added to a 100-ml conical flask. We prepared PDA medium (plus penicillin) in 10 Petri dishes and took 100 μl of bacterial suspension coated on PDA plate medium, set 5 gradients, each gradient with 2 replicates, placed the samples in a 25°C incubator for incubation for 24~48 h. We observed different colors and shapes to screen the colonies, distinguished different colors and sizes of the single colonies, separated and grew them until there was only one kind of bacteria in the medium after purification.
2.3 Amplification and sequencing of bacterial 16S rRNA and fungal internal transcribed spacer
The bacterial 16S rRNA gene and fungal ITS rDNA were amplified and sequenced for molecular identification. Endophyte genomic DNA was extracted using the cetyltrimethylammonium bromide (CTAB) method (Kosecka-Strojek et al., 2019). The primer sequences used for PCR identification were 27F (5’-GAGTTTGA TCACTGGCTCAG-3’), 1492R (5’-TACGGCTACCTTGTTACGA-3’) ITS1 (5′-TCCGTGGACTGACTCGG-3′) and ITS4 (5′-TCCTCGG-CTTA TGA TGC-3′) (Hassan, 2017). The polymerase chain reaction (PCR) system contained 22.5 μl of Gold MIX, 1 μl of each primer, and 0.5 μl of genomic template. The PCR amplification system was as follows: 98°C predenaturation for 2 min, 98°C denaturation for 10 s, 55°C annealing for 30 s, 72°C extension for 10 s for 30 cycles followed by 5 min extension at 72°C. After the reaction, the products were loaded on a 1% agarose gel and electrophoresced at 150 V for approximately 20 min. The amplification effect was detected by electrophoresis, and the quality was assessed according to the test results and sent to Kunming Shuokeng Biotechnology Co. The sequencing results were compared with basic local alignment search tool (BLAST) in the National Center for Biotechnology Information (NCBI) database, and homologous sequences were searched to find the strains with the highest similarity and determine their biological classification status.
2.4 Growth-promoting biological activity of the strains
The nitrogen fixation capacity of the symbiotic strains was tested on a modified low nitrogen medium (Ogura-Tsujita and Okubo, 2006), the potassium solubilization capacity of the symbiotic strains was tested by the method of Zheng et al. (Li et al., 2019), the phosphorus solubilization capacity of the symbiotic strains was tested by the method of Jasim et al. (2013), and the indole acetic acid (IAA) production capacity was determined by the Salkowski color development method, which was developed at room temperature in the dark for 20 min. The appearance of a pink color was positive, indicating the presence of IAA production, and the darker the color, the stronger the ability to produce IAA (Chen et al., 2019). The absorbance of the mixture was calibrated with a spectrophotometer at 530 nm using the same solvent calibration (control), and the concentration of IAA production by the strain was determined using the calculated standard curve y=40.063x-1.9228 (R2 = 0.9469).
All the isolated symbiotic strains were antagonistic with Phytophthora nicotianae to screen for antagonistic strains of the symbiotic strains. The pathogen was inoculated in the middle of a Petri dish containing PDA medium, and the isolated and purified symbiotic strains placed on the side of the pathogen were recorded as the treatment group. A control of Phytophthora nicotianae alone was set up and incubated in an incubator at 28°C for more than 7 d. Each treatment was repeated three times. After standoff culture for more than 14 d, the growth of the pathogenic fungus was observed, and pathogenic fungi covering the strain growth were not inhibited. The radius of the control pathogenic fungus with inhibitory effects and treatment group pathogenic fungus size were measured, and the inhibition rate was calculated according to the size of the inhibition rate to determine the antagonistic ability of the fungus. Inhibition rate (100%) = (control colony radius - treatment colony radius)/control colony radius x 100%.
2.5 Promotional effect of symbiotic strains on the seedlings of P. polyphylla var. yunnanensis
2.5.1 Inoculation of P. polyphylla var. yunnanensis seedlings with symbiotic strains
Preparation of symbiotic strain suspension: On an ultraclean bench, we aspirated 1 ml of purified symbiotic strains into a triangular flask containing 500 ml of autoclaved LB liquid medium, sealed the flask, incubated it at 200 rpm at 37°C until the OD600 was 0.5-0.6, centrifuged it at 5000 rpm for 5 min in a 500 ml sterile centrifuge tube, and resuspended the bacteria in 500 ml of sterile water. Preparation of the spore suspension of symbiotic fungi for watering: Five hundred milliliters of PDB liquid medium was poured into a culture bottle sterilized by autoclaving at 121°C in an aseptic environment. Then, fresh mycelium was picked, placed in a culture bottle and sealed. After 200 rpm-min-1 and 30°C shock incubation for 6 d, the effective live count of the liquid was determined to reach 1×108 CFU/ml, and then it was used for watering. In September 2020, two-year-old seedlings of P. polyphylla var. yunnanensis. were transplanted, and five months after transplanting, four strains were used to irrigate the P. polyphylla var. yunnanensis. in the form of a root suspension, and sterile water was used as the control, with a total of five treatments. Each treatment was planted in six pots, and each pot was planted with 10 two-year-old seedlings of P. polyphylla var. yunnanensis., and each pot was irrigated with 50 ml of bacterial suspension. After a total of 60 d of incubation, the rhizomes were removed from the roots, washed with water, and blotted with wiping paper. The following data were statistically analyzed.
2.5.2 Analysis of agronomic traits and chlorophyll and photosynthesis parameters
The natural plant height, root length, leaf length and leaf width of the seedlings were measured with a straight edge (accuracy 0.1 cm); the fresh weight root weight and aboveground fresh weight of the seedlings were weighed accurately with an electronic balance (accuracy 0.001 g). Fresh leaves were taken, the midvein was removed, and 0.1 g of sample was weighed and placed in a mortar. We added 1 ml of distilled water, a small amount (10 mg) of reagent one, ground it thoroughly under low light conditions, and transferred it into a 10-ml test tube wrapped with aluminum foil. We rinsed the mortar with the extraction solution, transferred all the rinsing solution into a 10-ml test tube, adjusted the volume to 10 ml with the extraction solution, and placed it under dark conditions for 3 h. When we observed that the tissue residue at the bottom was close to white, we considered the extraction complete. The absorbance values at 663 nm and 645 nm were measured by taking 1 ml of the upper layer of the extract in a glass cuvette and recorded as A663 and A645, respectively. The gas exchange parameters of the functional leaves of the heavy plant were measured using a Li-6400 XT photosynthesis-fluorescence recorder (Li-Cor, Lincoln, NE, USA) with a red and blue light source leaf chamber, setting a light quantum flux density of 300 µmol/(m2·s), a temperature of 25°C, and a CO2 concentration of 400 µmol/mol. The measurement time was chosen from 9:00 to 11:00 in clear weather, and seven representative Dianthus seedlings were selected for measurement in each treatment, with one leaf per plant randomly selected. The four indices of the net photosynthetic rate (Pn), stomatal conductance (Gs), intercellular CO2 concentration (Ci), and transpiration rate (Tr) were measured.
2.5.3 Saponin content determination
The treated rhizomes were collected, washed, dried at 45°C to a constant weight, and crushed. We weighed 0.5 g of the crushed sample (sieve No. 3, 50 mesh) into a 50 ml volumetric flask, added methanol and fixed the volume to 20 ml, weighed the sample, soaked it for 30 min, extracted it with ultrasound for 30 min, cooled it at room temperature, made up the lost weight with methanol, shook it well, and filtered the extract with a 0.45-μm microporous membrane. A total of 700 µl was placed into a brown injection vial, and the sample was injected into the machine. The content of steroidal saponins in the rhizomes of the seedlings was determined by high-performance liquid chromatography (HPLC) on an Agilent (Santa Clara, CA, USA) 4.6×250 mm column with the following chromatographic conditions: mobile phase acetonitrile (A): water (B) (gradient elution, 0→40 min (A:B=30:70→60:40), 40→41 min (A:B=60:40→80:20), 41→44 min (A:B=80:20→80:20), (A:B=80:20→80:20); detection wavelength of 203 nm, column temperature of 30°C, flow rate of 1.0 ml/min, injection volume of 20 μl.
2.5.4 Fluorescence quantification of steroid saponin synthesis pathway-related genes
Total RNA was extracted from the rhizomes of the Paris Rhizome using the Polysaccharide Polyphenol Plant Total RNA Kit according to the instructions, and the extracted total RNA of the qualified sample was used for reverse transcription synthesis of first-strand cDNA. cDNA synthesis was performed using Abm’s 5× All-in-One RT MasterMix kit. The EvaGreen 2X qPCR MasterMix (abm) kit was used to validate the expression levels of seven genes related to the steroid saponin synthesis pathway: hydroxymethylglutaryl coenzyme A synthase (HMGS), geranyl diphosphate synthase (GPPS), farnesyl pyrophosphate synthase (FPPS), squalene synthase (SS), squalene epoxidase (SE), and cycloartenol synthase (CAS). The primers for the Actin, SE1, SE2 gene were designed by the method of Xu Yan et al. (Xu et al., 2017) and the primers for the FPPS, SS, CAS, GPPS, HMGS gene were designed by the method of Xu Yongyan (Xu, 2017) because the P. polyphylla var.yunnanensis genes have not been published. All primers were ordered from Tsingke Biotechnology Co., Ltd. (Beijing, China). (Table 1). The PCR conditions were as follows: enzyme activation at 95°C for 10 min, denaturation at 95°C for 15 s, annealing at 60°C for 1 min, and storage at 4°C after 40 cycles. The relative quantification of gene expression was performed using the 2-△△Ct method, and the gene expression in the control group was set as the standard in the analysis (Table 1).
2.6 Promotional and antagonistic effects of symbiotic strains on tobacco
2.6.1 Tobacco inoculation with growth-promoting and antagonistic bacteria
The test treatments were divided into four groups (Table 2): control group: only 20 ml of sterile water was applied; pathogenic group: the black shank pathogen was inoculated by stem inoculation to the susceptible tobacco variety Hongda as a pathogen infestation test group; probiotic group: the four probiotic strains were singly applied to the tobacco by root irrigation as a bacterial suspension; antagonistic group: the four probiotic strains were coinoculated with the pathogenic fungus as an antagonistic test.
2.6.2 Determination of agronomic traits and enzyme activity of tobacco
The natural plant height, crown width, leaf length and leaf width of the seedlings were measured with a straightedge (accuracy 0.1 cm); SOD, POD, PPO, PAL and enzyme activities were determined by a visible spectrophotometric method using a Solarbio kit (Purchased from Beijing Solarbio Science & Technology Co., Ltd., Beijing, China).
2.6.3 Tobacco growth-related resistance gene expression
Following the above steps, RNA was extracted from tobacco plants and reverse-transcribed into cDNA, and finally, the expression of tobacco growth resistance-related genes was determined using the NtActin gene as an internal reference gene, as shown in Table 3. The ChamQ Universal SYBR qPCR Master Mix kit (Purchased fromVazyme Biotech Co.,Ltd Nanjing, China). was selected for the validation of related enzymes and resistance-related gene expression levels. The PCR conditions were as follows: predenaturation phase 95°C for 30 s, cycling phase 95°C for 10 s, 60°C for 30 s, 95°C for 15 s, 60°C for 1 min, and 95°C for 15 s, for a total of 40 cycles. After the reaction, the relative quantification of gene expression was performed using the 2-△△Ct method, and the gene expression in the control group was set as the standard in the analysis.
2.7 Data processing
The experimental data obtained were statistically analyzed by Excel 2013 software; SPSS 19.0 (SPSS, Chicago, IL, USA) analysis software was used for statistical analysis, and one-way analysis of variance (ANOVA) was used for the significance of differences. Comparison of means between treatments was performed using Duncan’s multiple comparison method for significance of differences, and Sigmaplot 10.0 software was used for graphing.
3 Results
3.1 Evaluation of the probiotic function of symbiotic strains
Symbiotic strains were isolated, 73 symbiotic strains were obtained from P. fargesii Franch, and the growth-promoting ability of the symbiotic strains was measured. The results showed that among the 43 symbiotic bacteria (Table 4), 25 strains had the ability to fix nitrogen, 5 strains had the ability to dissolve phosphorus, 22 strains had the ability to dissolve potassium, and 17 strains could produce IAA; among the 31 symbiotic fungi (Table 5), 4 strains had the ability to dissolve phosphorus, 31 strains had the ability to dissolve potassium, and 9 strains could produce IAA.
The 72 isolated symbiotic strains were cultured with Phytophthora nicotianae in plate standoffs, and the growth of the strains was observed after 7 d. Nine strains were found to have inhibitory effects on pathogenic fungus. Among these strains, LgD2, LgD10, TPB and TPD11 had the strongest inhibitory effect; therefore, these four strains were cultured in a secondary standoff with the pathogen, and the diameter of the control and treated pathogens was measured after 7 d. As shown in Table 6, the two symbiotic bacterial strains LgD2 and LgD10 showed 90 and 88% inhibition against Phytophthora nicotianae, while the two symbiotic fungal strains TPB and TPD11 showed 92 and 81% inhibition against Phytophthora nicotianae.
3.2 Phylogenetic analysis of antagonistic probiotic strains
Four strains (LgD2, LgD10, TPB and TPD11) were selected for subsequent growth promotion tests based on their growth promotion characteristics and their ability to antagonize pathogenic fungi. The 16S rDNA and ITS sequences of the four selected strains of P. fargesii Franch. were compared with multiple sequences using the software ClustalX2, and then the software MEGA4 was used to construct a phylogenetic tree according to the neighbor-joining method, as shown in Figures 1 and 2. Two strains of the interrhizosphere fungus TPD11 clustered with Aspergillus tamarii (OP405014), and TPB clustered with Fusarium oxysporum (OP405007); two strains of the endophytic bacterium LgD10 clustered with Bacillus thuringiensis (OP404360), and strain LgD2 clustered with Bacillus cereus (OP404241). The datasets presented in this study can be found in online repositories. The names of the repository/repositories and accession number(s) can be found below: GenBank (https://www.ncbi.nlm.nih.gov/)
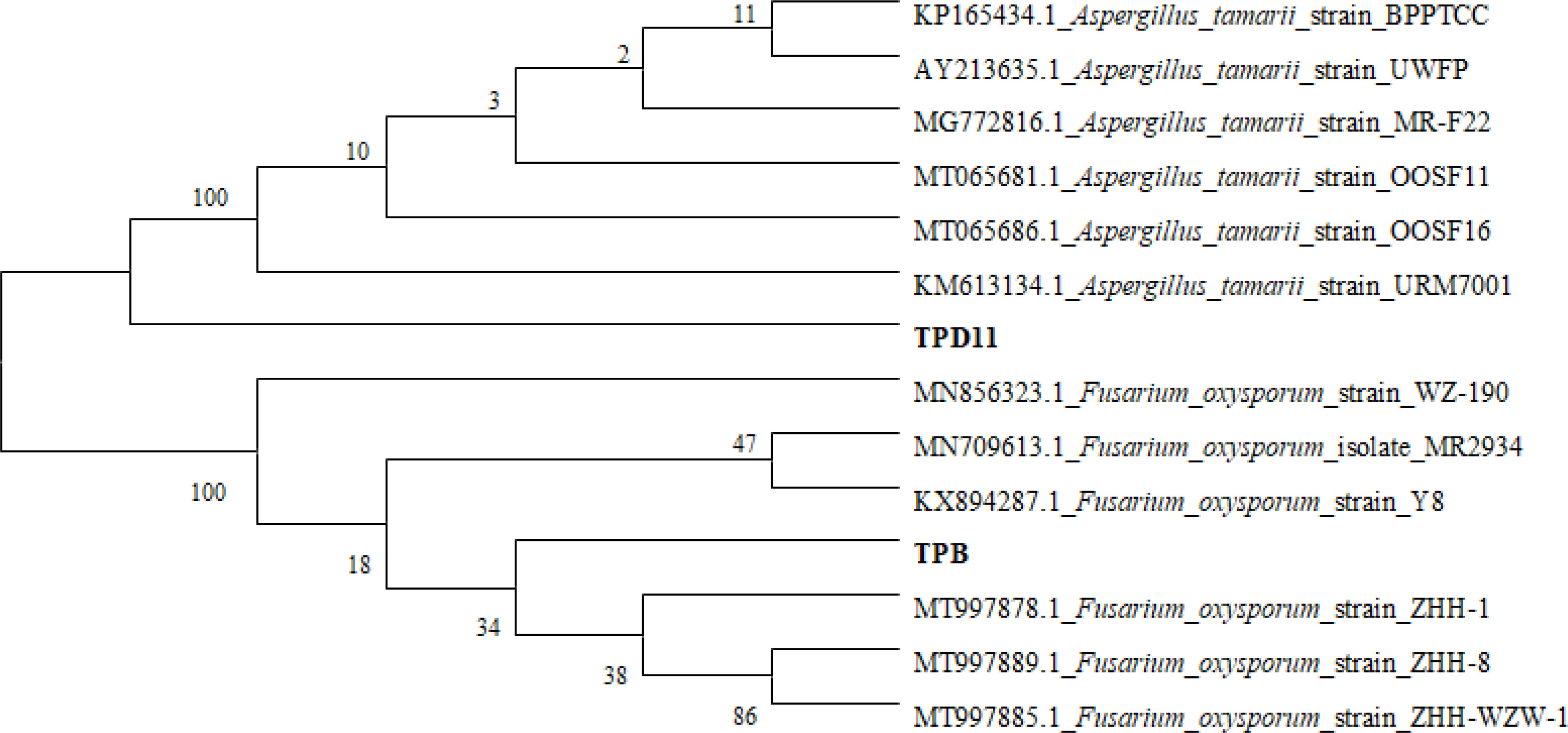
Figure 1 Phylogenetic analysis of the TPB and TPD11 strains. ITS regions of fungal isolates, with the sequences from NCBI TPD11 and TPB being the sequences obtained from fungi. Phylogenetic trees were constructed by the neighbor-joining method using the software MEGA4.
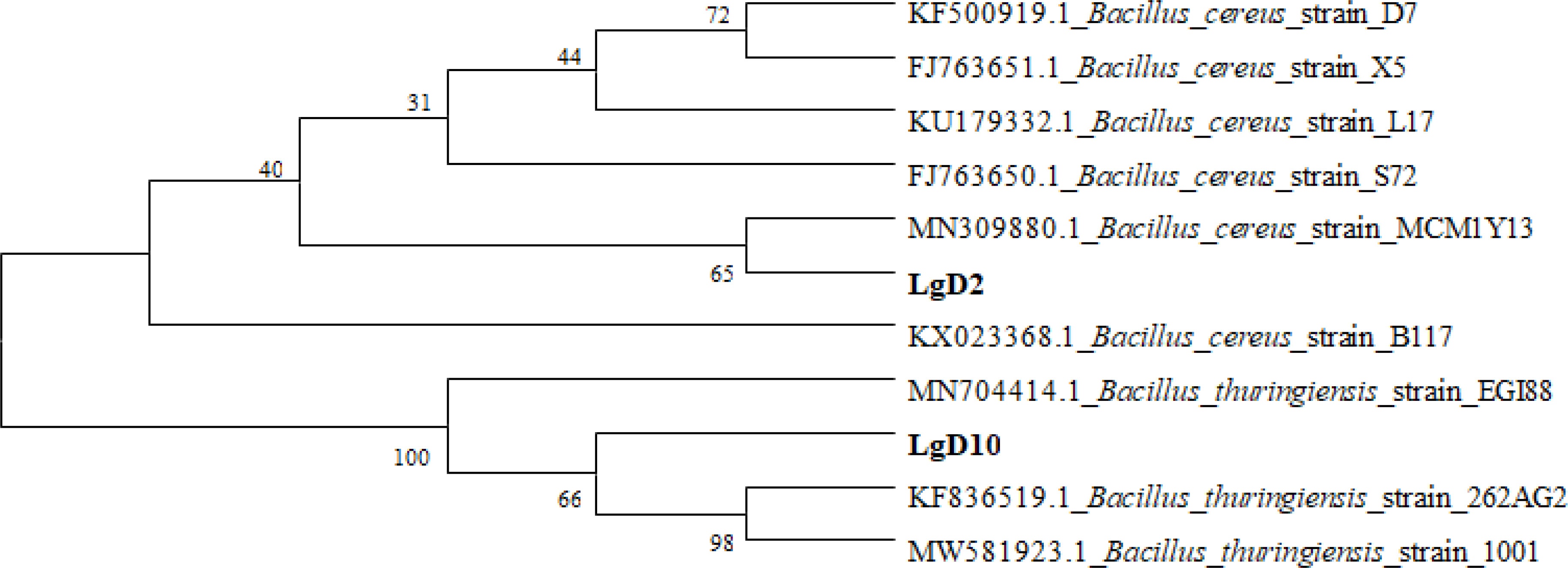
Figure 2 Phylogenetic analysis of the LgD2 and LgD10 strains. 16S rRNA sequences of the bacterial isolates, with the sequences from NCBI. LgD2 and LgD10 refer to 16S rRNA sequences retrieved from bacteria. Phylogenetic trees were constructed by the neighbor-joining method using the software MEGA4.
3.3 Symbiotic culture of growth-promoting strains and Pairs polyphylla var.yunnanensis seedlings
3.3.1 The effect of probiotic strains on the biomass of P. polyphylla var. yunnanensis
After the above screening, four symbiotic strains (LgD2, LgD10, TPB and TPD11) were cultured in symbiosis with P. polyphylla var. yunnanensis seedlings, the external morphology of the seedlings after coculture was observed, and the seedlings grew well between treatments without disease infection traits (Figure 3). Some of the agronomic traits of P. polyphylla var. yunnanensis were evaluated (Table 7). The four inoculated symbiotic strains of TPB, LgD10, TPD11 and LgD2 treatments were all significantly taller than the control CK, at 55, 47, 44 and 43% higher than the control, respectively. The five treatments in descending order of growth in plant height were TPB > LgD10 > TPD11 > LgD2 > CK. The greatest effect on plant height was found in the TPB strain, followed by LgD10, while the difference between TPD11 and LgD2 was not significant. The treatments inoculated with the TPB strain had significantly higher fresh weight of the aboveground parts, leaf length and leaf width than the control by 65, 46 and 42%, respectively; treatments inoculated with the TPD11 strain by 53, 46 and 41%, respectively; the treatments inoculated with the LgD10 strain were 52.38, 42.19 and 38% higher, respectively; and the treatments inoculated with the LgD2 strain were 48, 43 and 37% higher, respectively. The main medicinal part of P. polyphylla var. yunnanensis is the rhizome, so the root weight and root length of its underground part were also measured separately. The results showed that the root weight and root length of the underground parts of the treatments inoculated with the growth-promoting bacteria and fungi were significantly higher than the root weight and root length of the underground parts of the control. The ground weight of the treatment inoculated with the TPB strain was 46% higher than the ground weight of the control, while TPD11, LgD2 and LgD10 were not significantly different. The longest roots were found in the treatment inoculated with the TPB strain, which was 50% longer than the control, followed by the LgD2 strain treatment, which was 42% longer than the control, while the difference between TPD11 and LgD10 was not significant. In summary, all four symbiotic strains increased the plant height, leaf length and leaf width of P. polyphylla var. yunnanensis promoted the fresh weight of the above- and belowground parts of P. polyphylla var. yunnanensis and stimulate root elongation and promote the development of the root system of P. polyphylla var. yunnanensis.
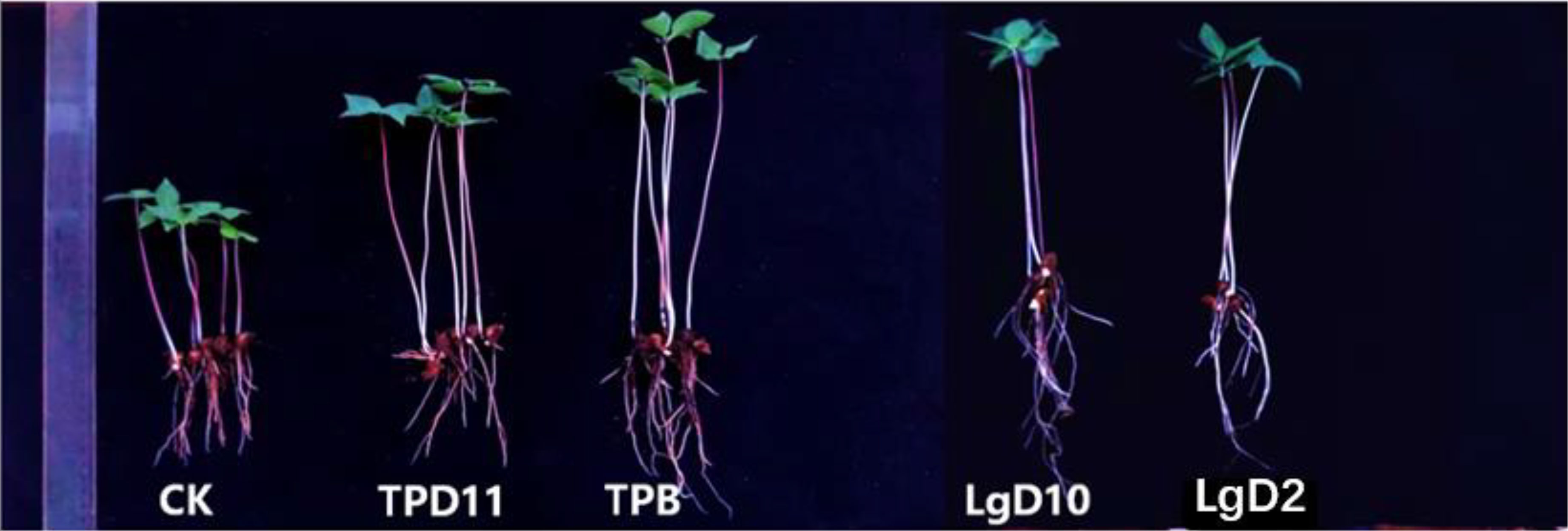
Figure 3 Two-year-old P. polyphylla var. yunnanensis seedling morphology after symbiotic strain treatment. CK is distilled water treatment; TPD11 and TPB were two symbiotic fungal treatments, and LgD2 and LgD10 were two symbiotic bacterial treatments.
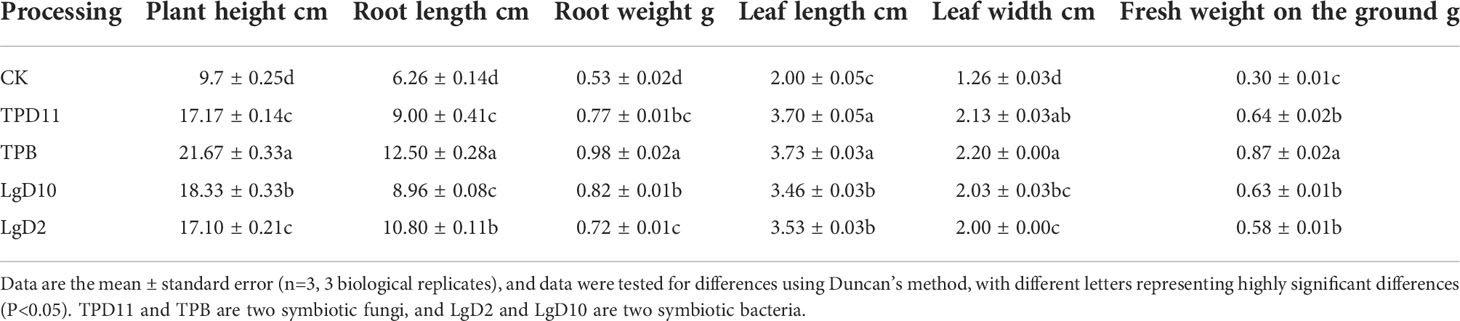
Table 7 Effects of symbiosis on the biomass of two-year-old P. polyphylla var. yunnanensis seedlings.
3.3.2 Effect of probiotic strains on the chloroplast pigment content of P. polyphylla var. yunnanensis
As shown in Figure 4, the chlorophyll a, chlorophyll b and total chlorophyll content of P. polyphylla var. yunnanensis seedlings inoculated with symbiotic strains were significantly higher than the chlorophyll a, chlorophyll b and total chlorophyll content of the control. With a peak at the LgD10 strain treatment, the values were 0.31, 0.57 and 0.89 (mg g-1 fresh weight (FW)), respectively, followed by the LgD2 strain treatments at 0.31, 0.56 and 0.86 (mg g-1 FW), respectively. The TPB strain treatments contained 0.26, 0.55 and 0.82 (mg g-1 FW), respectively. TPD11 strain treatments contained 0.24, 0.54 and 0.79 (mg g-1 FW), respectively. The chlorophyll a, chlorophyll b and total chlorophyll content among the five treatments decreased in the order LgD10> LgD2> TPB> TPD11> CK. The chlorophyll a/b was significantly higher in the treatment CK without inoculation of probiotic bacteria and fungi than in the other treatments, while the differences between the treatments of LgD10 and LgD2 strains were not significant.
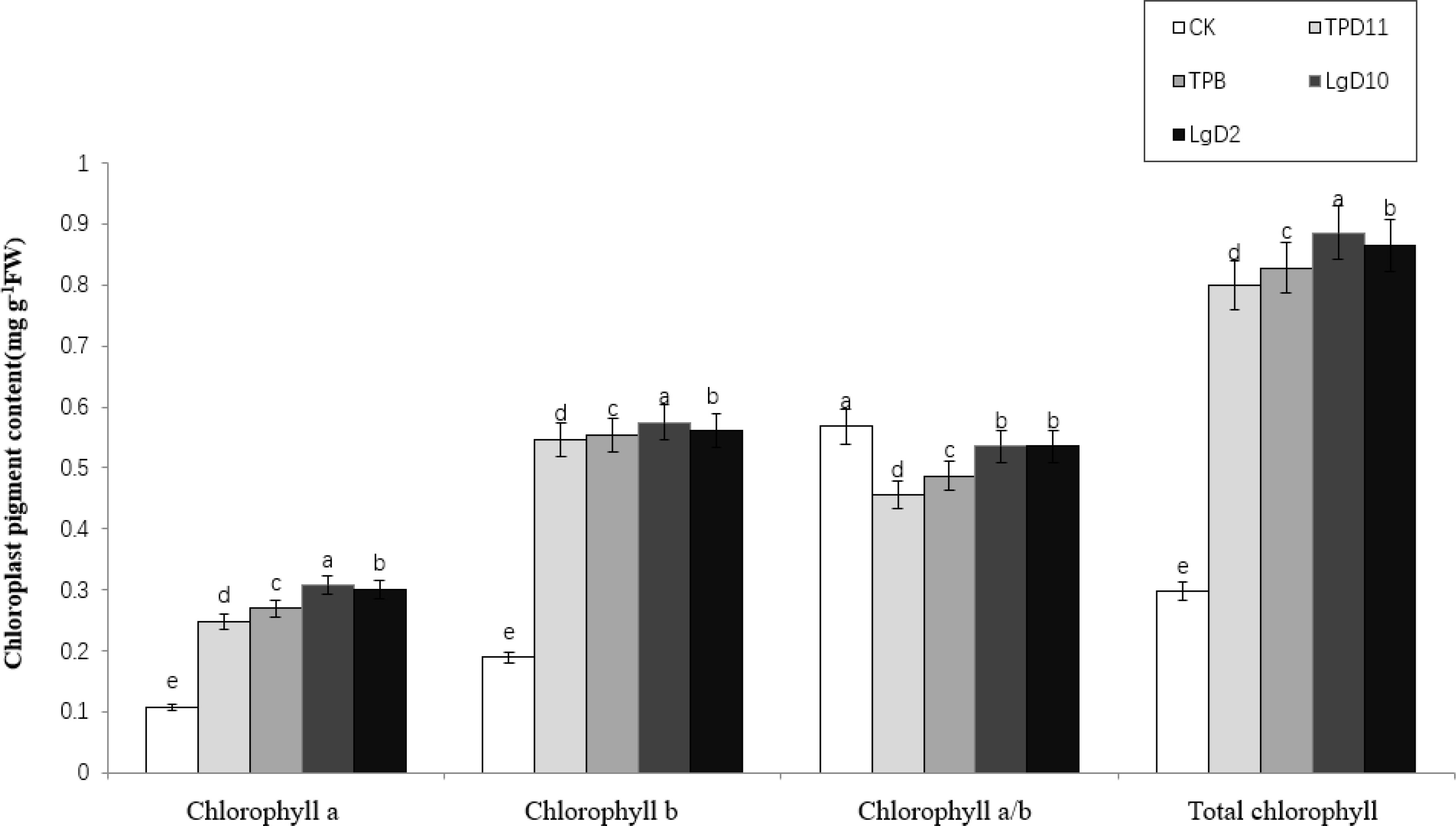
Figure 4 Effect of symbiotic strains on chloroplast pigment content in leaves of two-year-old P. polyphylla var. yunnanensis seedlings. SOD activity of tobacco under different strain treatments. Data are the means of three replicates (mean ± SE). Values followed by different letters indicate significant differences at P < 0.05 levels by Duncan’s multiple range tests.
3.3.3 Effect of probiotic strains on the gas exchange parameters of P. polyphylla var. yunnanensis
After the coculture of four strains of symbiotic strains with two-year-old P. polyphylla var. yunnanensis seedlings, the gas exchange parameters of the plants were measured for each treatment and statistically analyzed, and the results are shown in Table 8. Inoculation with four strains of probiotic bacteria and fungi significantly increased the net photosynthetic rate (Pn), stomatal conductance (Cond), transpiration rate (Tr) and intercellular CO2 concentration (Ci) of P. polyphylla var. yunnanensis leaves compared with CK. Statistically, the treatment of the TPD11 strain had the highest net photosynthetic rate, stomatal conductance, transpiration rate and intercellular CO2 concentration among all treatments, followed by the TPB, LgD10 and LgD2 strain treatments. Overall, inoculation with probiotic bacteria enhanced photosynthesis in the leaves of P. polyphylla var. yunnanensis.
3.3.4 Effect of probiotic bacteria and fungi on the saponin content of P. polyphylla var. yunnanensis
After single back inoculation with four strains of symbiotic strains, the contents of Parissaponin I, Parissaponin II, Parissaponin VI, Parissaponin VII, Parissaponin D, Parissaponin H and Rhizoma Paridis total saponins were determined in the treated rhizomes of P. polyphylla var. yunnanensis. The contents were calculated against Parissaponin standards, and the statistical results are shown in Figure 5. There was a significant effect on the yield of Parissaponin after a single back-injection of the symbiotic strains.
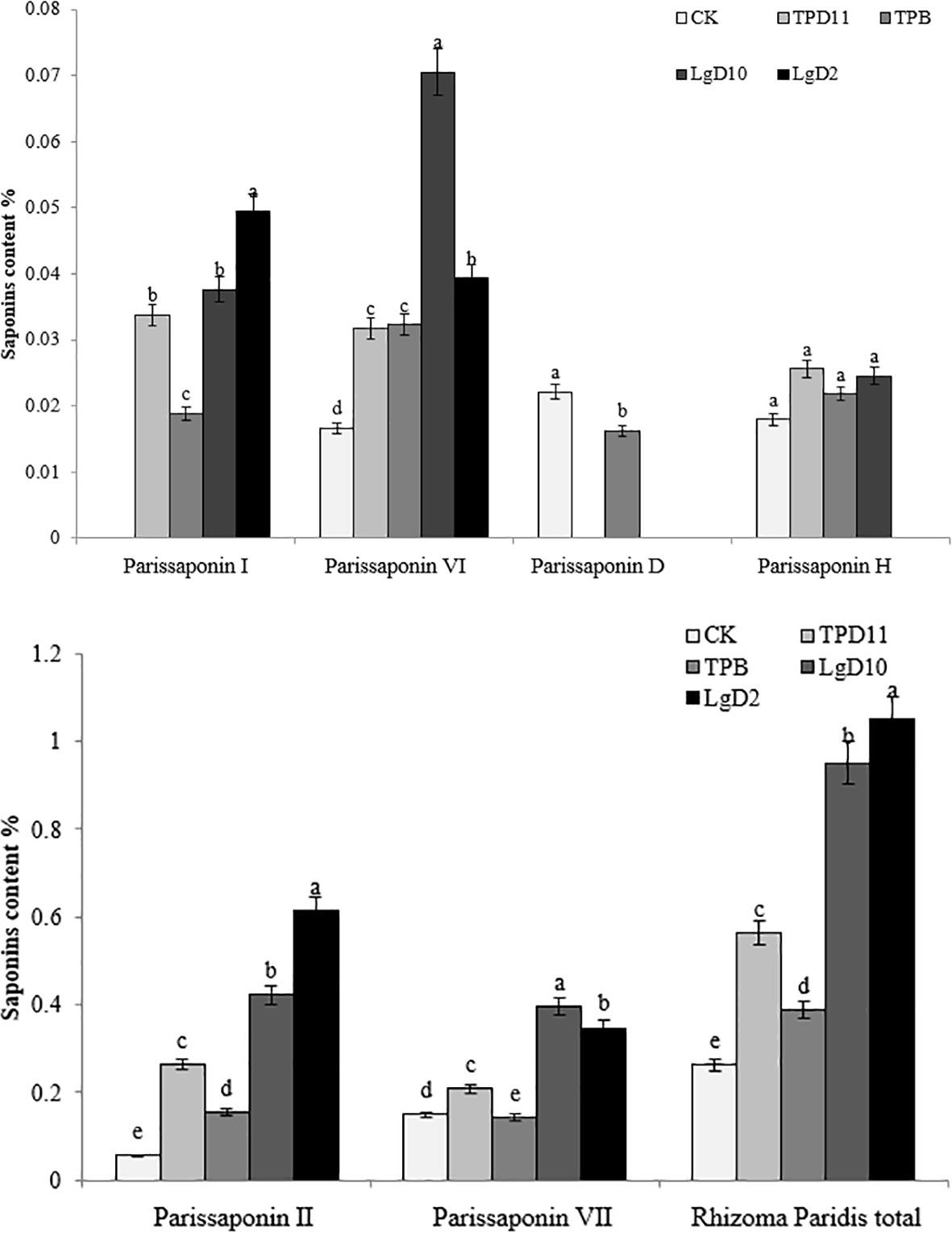
Figure 5 Effect of symbiotic strains on the content of saponins in the rhizome of two-year-old seedlings. Data are the means of three replicates (mean ± SE). Values followed by different letters indicate significant differences at P < 0.05 levels by Duncan’s multiple range tests.
Parissaponin I had the highest content after treatment with the LgD2 strain at 0.049%, followed by LgD10, TPD11 and TPB at 0.034, 0.038 and 0.019%, respectively. The Parissaponin I content in the LgD2 strain treatment was significantly higher than the Parissaponin I content in the LgD10, TPD11 and TPB strains; there was no significant difference between the LgD10 and TPD11 strain treatments, and Parissaponin I was not detected in the CK treatment. The inoculation of four strains of LgD2, LgD10, TPD11 and TPB treated with Parissaponin II decreased in the order of LgD2> LgD10> TPD11 > TPB > CK, with values of 0.613, 0.422, 0.264, 0.155 and 0.056%, respectively. The content of Parissaponin VI was highest under the LgD10 strain, and the content after inoculation treatment was LgD10> LgD2> TPB> TPD11> CK in descending order, 0.071, 0.039, 0.032, 0.031 and 0.016%, respectively. The content of LgD2 was significantly higher than the content of the TPD11 and TPB strain treatments, while the TPD11 and TPB strain treatments were not significantly different from each other. The content of Parissaponin VII in the inoculated probiotic treatment was significantly higher than the Parissaponin VII content in the control, from high to low, LGD10 > LGD2 > TPD11 > CK > TPB, with values of 0.396, 0.347, 0.208, 0.149 and 0.142%, respectively. Parissaponin D was detected only under the CK and TPB strain treatments at 0.022 and 0.016%, respectively, while not being detected in the rest of the treatments. Parissaponin D was detected only under the CK and TPB strain treatments at 0.022 and 0.016%, respectively, while Parissaponin D was not detected in the rest of the treatments. The P. polyphylla var. yunnanensis total saponin treatments inoculated with probiotic bacteria and fungi alone were significantly higher than the control, from high to low, LgD2 > LgD10 > TPD11 > TPB > CK, 1.049, 0.951, 0.563, 0.387 and 0.262%, respectively. In brief, all four strain treatments increased the rhizome Parissaponin content of seedlings to varying degrees, with particularly pronounced changes in the total saponin content of P. polyphylla var. yunnanensis.
3.3.5 Expression analysis of genes of the Parissaponin synthesis pathway induced by probiotic bacteria and fungi
To investigate the effect of symbiotic strains on the expression of genes related to the saponin synthesis pathway of P. polyphylla var. yunnanensis, quantitative fluorescence PCR analysis of key genes of the steroidal saponin synthesis pathway (HMGS, GGPS, FPPS, SS, SE1, CAS, SE2), which were previously reported by previous authors, was performed, and Actin was used as an internal reference gene to calculate the expression results, as shown in Figure 6. All treatments differentially increased the expression of Parissaponin synthesis pathway genes, with the highest expression of HMGS, SE2, and CAS under the LgD10 strain treatment, the highest expression of GGPS and FPPS under the TPD11 strain treatment, and the highest expression of SE1 under the LgD2 treatment. The expression of HMGS was upregulated in the treatments with four strains of probiotic bacteria and fungi TPB, LgD10, TPD11 and LgD2, which were 0.3, 1.9, 2.5 and 2.2 times higher than the control, respectively, and the difference between TPD11 and the control was not significant; the expression of the GGPS gene was significantly upregulated in the four strains of probiotic bacteria and fungi inoculated separately, which were 7.8, 1.6, 4.6 and 3.1 times higher than the control, respectively. The expression of the FPPS gene was upregulated in all four strains, 10.1, 1.4, 8.3 and 9.8 times that of the control, respectively, and the difference was not significant among TPD11, LgD10 and LgD2. TPB was upregulated but not significantly different from the control. The expression of the SS gene was significantly upregulated in all four strains, 3.0, 3.1, 2.3 and 3.1 times that of the control, respectively, and the difference was not significant among the four strains. The expression of the SE1 gene was significantly upregulated in all four strains, TPD11, LgD10 and LgD2. The expression of the SE1 gene was significantly upregulated by inoculation with the TPD11, LgD10 and LgD2 strains, which was 4.0, 6.8 and 8.4 times higher than the expression of the SE1 gene of the control, respectively, and treatment with the TPB strain suppressed the expression of the SE1 gene. The expression of the SE2 gene was upregulated by inoculation of the four strains of probiotic treatment, 3.0, 2.9, 9.2 and 7.3 times more than the control, respectively, and the difference between TPB and TPD11 was not significant; the expression of the CAS gene was upregulated by inoculation of the four strains of probiotic treatment, 1.8, 0.5, 12.1 and 4.7 times more than the control, respectively, and the difference between TPB and TPD11 was not significant compared with the control.
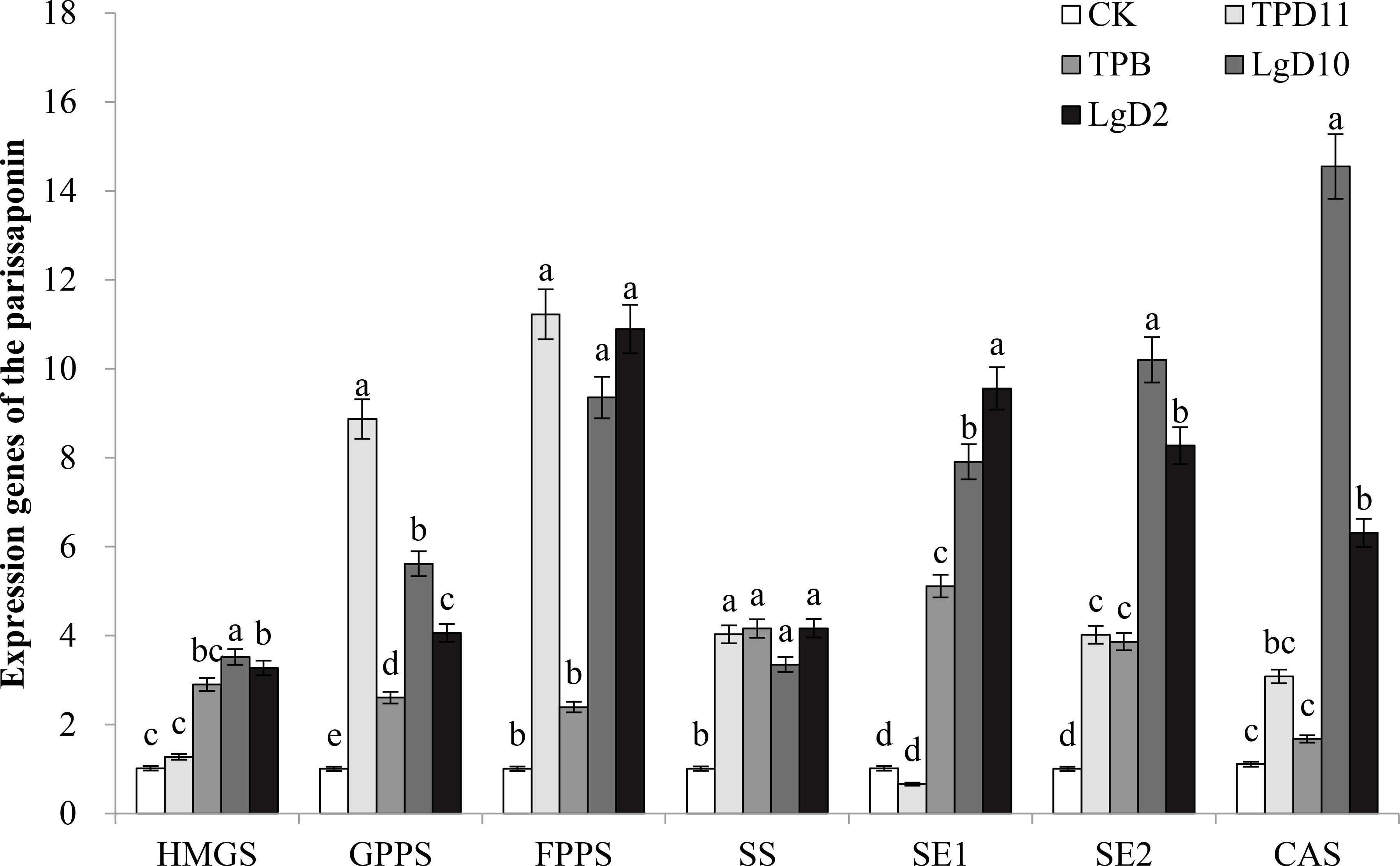
Figure 6 Effect of symbiotic strains on the expression of related genes in the rhizome of two-year-old seedlings. Data are the means of three replicates (mean ± SE). Values followed by different letters indicate significant differences at P < 0.05 levels by Duncan’s multiple range tests.
3.4 Effect of inoculation of tobacco with probiotic and pathogenic fungi on tobacco physiology
3.4.1 Effect of growth-promoting bacteria and fungi on tobacco biomass
Tobacco biomass was significantly higher in the promotion group treatment as well as in the antagonist group treatment than in the control and pathogenic groups (Table 9). In the growth-promoting group, plant height, crown width, leaf length and leaf width increased by 20, 8, 24 and 21% in the LgD2 strain treatment; 19, 10, 27 and 24% in the LgD10 strain treatment; and 16, 6, 20 and 17% in the TPD11 strain treatment, respectively, compared to the control. TPB strain treatments increased by 8, 8, 23 and 21%, respectively. Compared to CK, the LgD2 and LgD10 strain treatments had the greatest effect on tobacco plant height, crown width and leaf length, and the differences between them were not significant. The height, crown width, leaf length and leaf width of tobacco plants treated with Phytophthora nicotianae alone were significantly lower than the height, crown width, leaf length and leaf width of the antagonistic group treated with the four probiotic strains and Phytophthora nicotianae together, where LgD2+Y increased by 11, 17, 13 and 12%, respectively, compared to YM inoculation alone; LgD10+Y increased 12, 17, 19, and 19%, respectively, over YM inoculation alone; TPB+Y improved 11, 18, 15, and 12%, respectively, compared with YM inoculation alone; and TPD11+Y improved 7, 19, 13, and 16%, respectively, compared with YM inoculation alone. The differences in plant height and leaf width in the pathogenic group treatments were not significant compared to the control.
3.4.2 Effect of probiotic bacteria and fungi on the enzyme activity of tobacco
3.4.2.1 Effect of probiotic bacteria and fungi on SOD enzyme activity of tobacco
The SOD enzyme activities of the probiotic antagonist strains, pathogenic fungus and tobacco were measured at the end of coculture, and the results are shown in Figure 7. The SOD enzyme activity of the treatments in the promotion group inoculated with three strains of the probiotic antagonist strains, TPD11, LgD10 and LgD2, alone were higher than the control CK by 5, 24 and 4%, respectively, but the difference between LgD2 and the control was not significant, while the SOD enzyme activity of the TPB inoculation treatment was 18% lower than the control. All three treatments, TPD11+Y, LgD10+Y and LgD2+Y, in the antagonistic group had significantly higher SOD enzyme activity than the pathogenic group treatment YM, 8, 6 and 3% higher than YM, respectively, and the differences among the three probiotic group treatments were not significant. The enzyme activity of the TPB+Y inoculation treatment was 18% lower than the enzyme activity of the control. The SOD enzyme activity of YM inoculated with Phytophthora nicotianae alone was significantly lower than the SOD enzyme activity of the control CK.
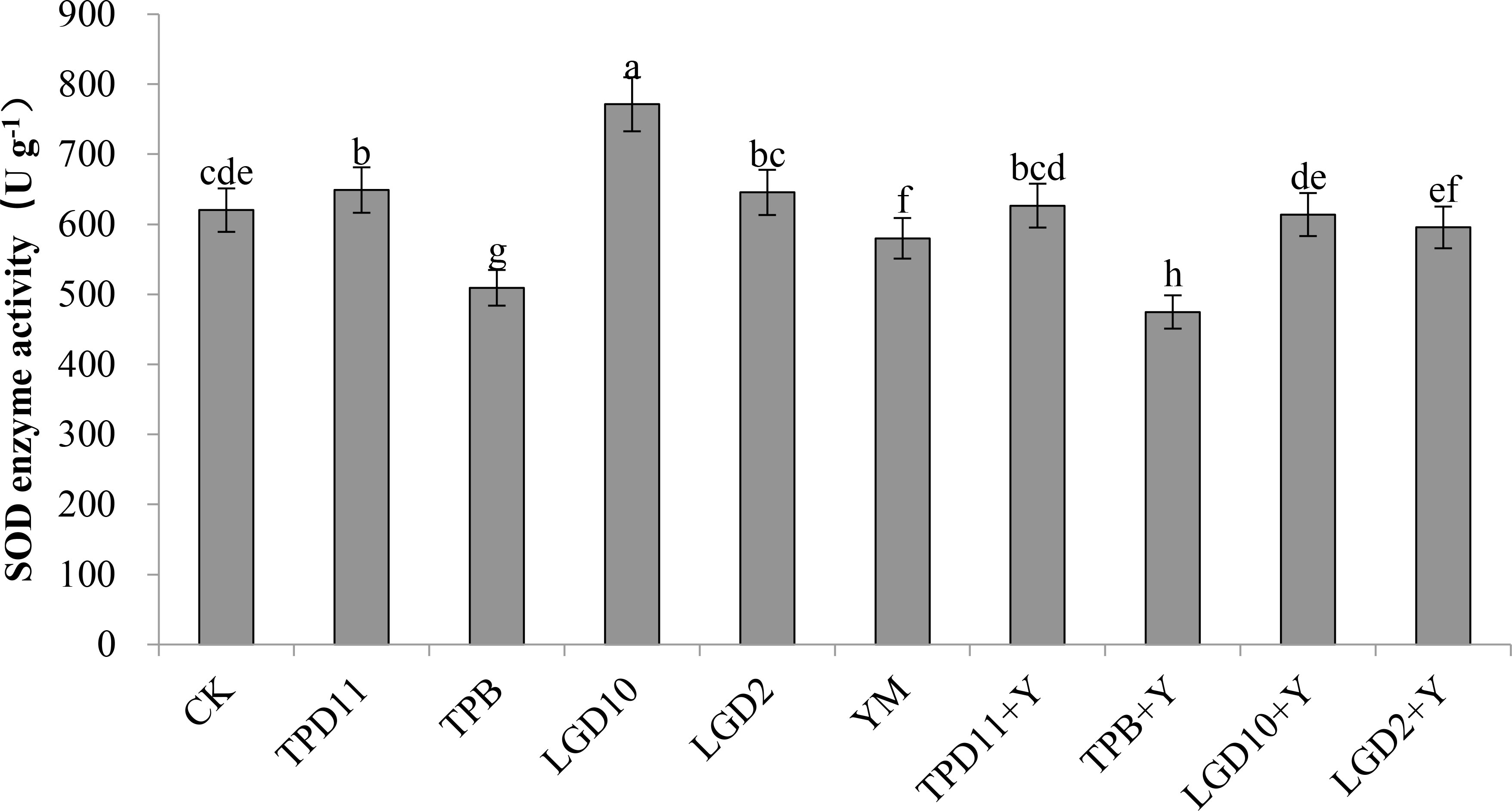
Figure 7 SOD activity of tobacco under different strain treatments. Data are the means of three replicates (mean ± SE). Values followed by different letters indicate significant differences at P < 0.05 levels by Duncan’s multiple range tests.
3.4.2.2 Effect of probiotic bacteria and fungi on POD enzyme activity of tobacco
The POD enzyme activities of the probiotic antagonist strain, pathogenic fungus and tobacco were measured at the end of coculture, and the results are shown in Figure 8. The POD enzyme activities of TPD11, TPB, LgD10 and LgD2 were significantly higher than the control CK in the promotion group treatment and 18, 7, 32 and 42% higher than the control, respectively, and the POD enzyme activities of the four strains of promotion antagonists decreased in the order LgD2> LgD10> TPD11> TPB. The POD enzyme activity of TPD11+Y, TPB+Y, LgD10+Y and LgD2+Y in the antagonist group treatment was significantly higher than the POD enzyme activity of the pathogenic group treatment YM, 13, 8, 26 and 21% higher than the POD enzyme activity of YM, respectively, and the enzyme activities were ranked as LgD10+Y>LgD2+Y>TPD11+Y>TPB+Y, respectively. The POD enzyme activity of YM treated with Phytophthora nicotianae alone was significantly higher than the POD enzyme activity of the control CK.
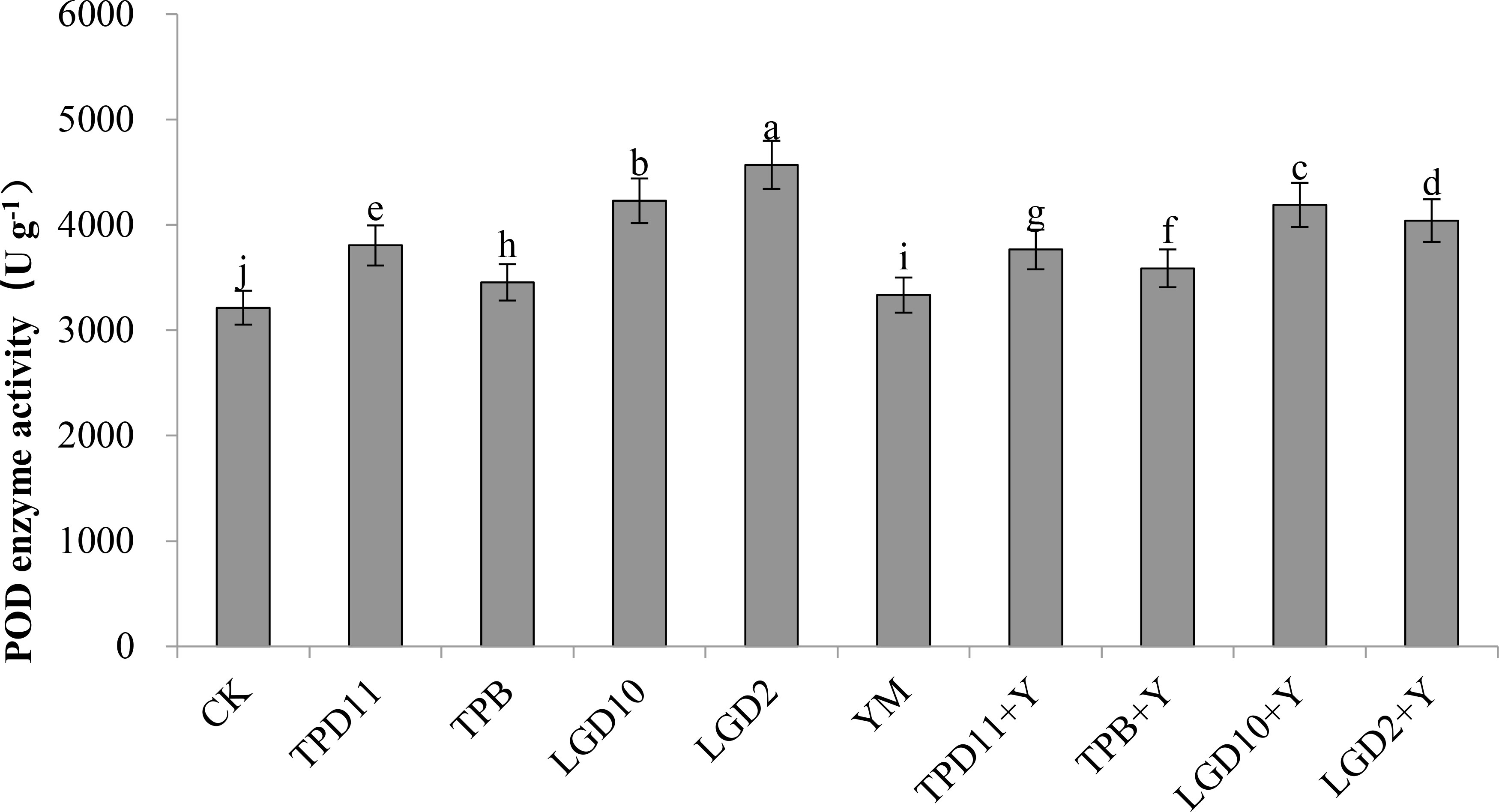
Figure 8 POD activity of tobacco under different strain treatments. SOD activity of tobacco under different strain treatments. Data are the means of three replicates (mean ± SE). Values followed by different letters indicate significant differences at P < 0.05 levels by Duncan’s multiple range tests.
3.4.2.3 Effect of probiotic bacteria and fungi on PPO enzyme activity in tobacco
The PPO enzyme activity of the probiotic antagonist strain, pathogenic fungus and tobacco was measured at the end of coculture, and the results are shown in Figure 9. The PPO activity of TPD11, LgD10 and LgD2 was significantly higher than the control CK in the promotion group treatment and 30, 34 and 11% higher than the control, respectively, and the PPO activity of the three strains of promotion antagonists was LgD10> TPD11> LgD2 in descending order, while the PPO activity of the TPB inoculation treatment were 11% lower than the control. Among the antagonist group treatments, only inoculation with LgD10+Y treatment PPO activity was significantly higher than YM treatment by 9%, while the TPD11+Y and LgD2+Y treatments were not significantly different from YM treatment, and inoculation with TPB+Y treatment PPO activity was significantly lower than YM treatment by 13%. The PPO enzyme activity of YM treated with Phytophthora nicotianae alone was significantly lower than the PPO enzyme activity of the control CK.
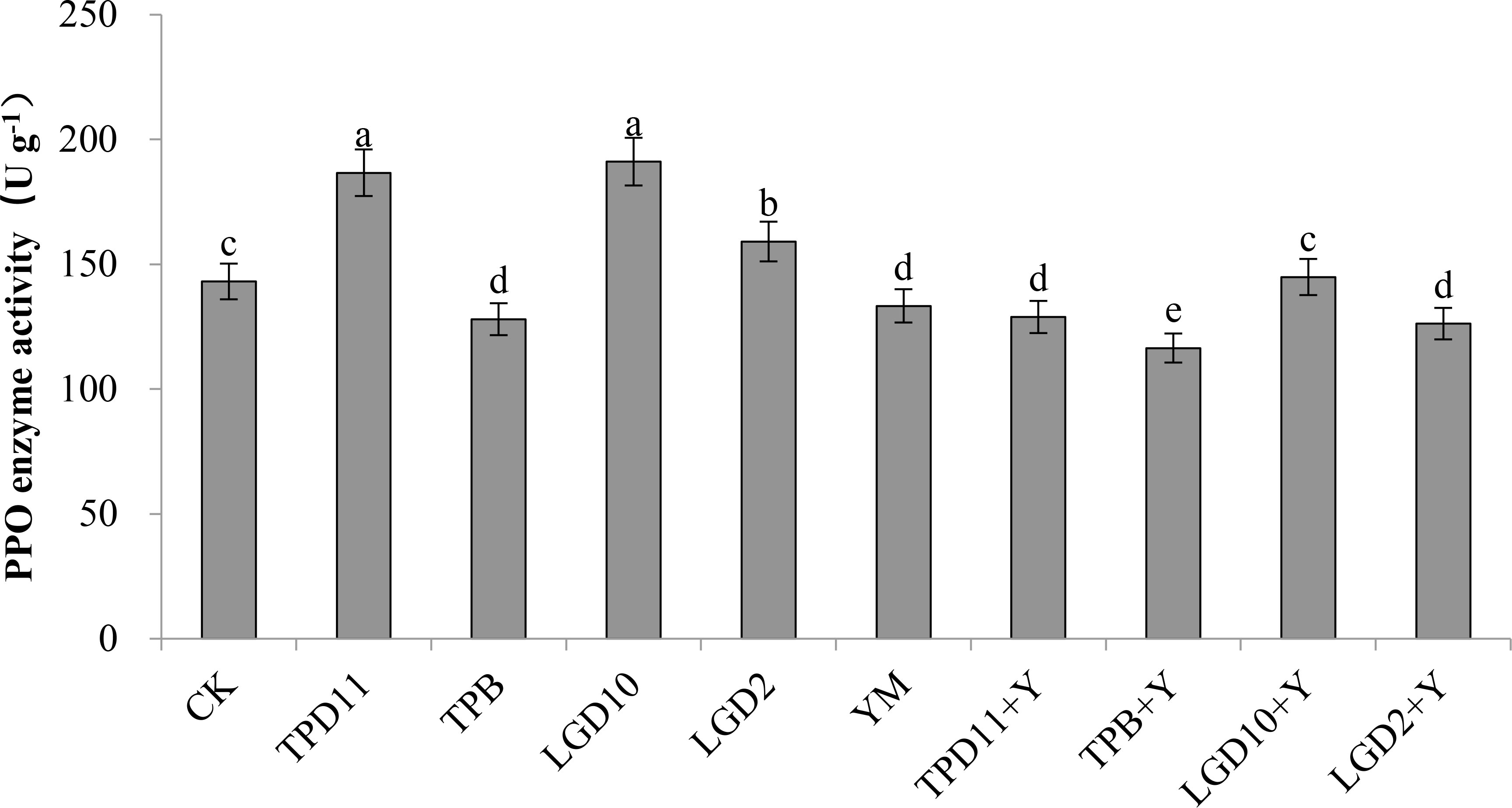
Figure 9 PPO activity of tobacco under different strain treatments. SOD activity of tobacco under different strain treatments. Data are the means of three replicates (mean ± SE). Values followed by different letters indicate significant differences at P < 0.05 levels by Duncan’s multiple range tests.
3.4.2.4 Effect of probiotic bacteria and fungi on PAL enzyme activity in tobacco
The PAL enzyme activity of the probiotic antagonist strains, pathogenic fungus and tobacco were measured at the end of coculture, and the results are shown in Figure 10. The PAL activities of TPD11 and LgD10 were significantly higher than the control CK by 12 and 13% in the promotion group treatment, while the difference between the PAL activity of the LgD2 inoculation treatment and the control was not significant, and the PAL activity of the TPB inoculation treatment were significantly lower than the control CK by 9%. The PAL activity of the four strains of promotion antagonists were, in descending order, LgD10> TPD11> LgD2> TPB. The PAL enzyme activity of TPD11+Y, TPB+Y, LgD10+Y and LgD2+Y was higher in the antagonist group treatment than in the pathogenic group treatment YM, 18, 7, 10 and 2% higher than the PAL enzyme activity of YM, respectively, and only TPD11+Y enzyme activity was significantly higher than the PAL enzyme activity of the Phytophthora nicotianae treatment YM, while TPB+Y, LgD10+Y and LgD2+ Y treatments were not significantly different compared to the YM treatment. The PAL activity of YM treated with Phytophthora nicotianae alone was not significantly different compared to the control CK.
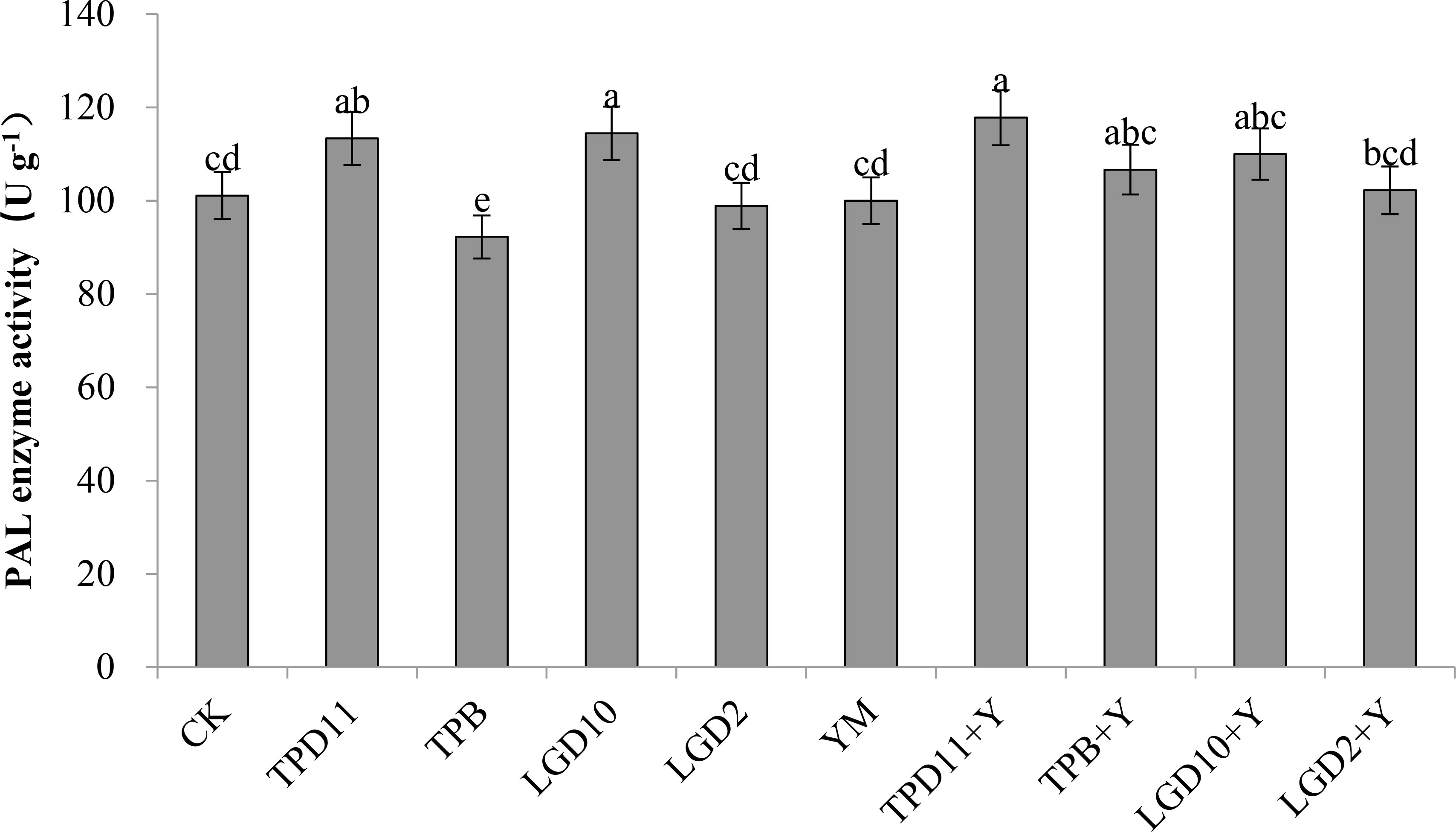
Figure 10 PAL activity of tobacco under different strain treatments. SOD activity of tobacco under different strain treatments. Data are the means of three replicates (mean ± SE). Values followed by different letters indicate significant differences at P < 0.05 levels by Duncan’s multiple range tests.
3.5 Inhibition of tobacco pathogens by probiotic bacteria and fungi
After cocultivation of the antagonistic strain with tobacco, the number of diseased strains and disease levels were measured separately, and according to the formula for tobacco disease, incidence (%) = number of diseased strains/total number of plants investigated x 100%, disease index (%) = ∑ (number of diseased strains at each level x corresponding level)/total number of plants investigated x highest level value x 100%, relative suppression (%) = (1 - disease index in treatment area/disease index in control area) x 100%, the incidence, disease index and relative suppression rate were calculated for each treatment, and the results are shown in Figure 11. The incidence of YM treatment alone reached 100%, and compared to YM alone, the incidence of TPD11+Y, TPB+Y, LgD10+Y, and LgD2+Y in the antagonist group treatment was reduced by 33, 14, 31, and 17%, respectively, and the incidence of TPD11+Y and LgD10+Y inoculation was significantly lower than that of the TPB+Y and LgD2+Y treatments. The disease indices of the TPD11+Y, TPB+Y, LgD10+Y and LgD2+Y treatments were significantly reduced, 48, 35, 46 and 40%, respectively, compared with the disease indices of the control, and the disease indices of the TPD11+Y inoculation treatments were significantly lower than the disease indices of the other treatments. Compared with the control, the relative inhibition rates of inoculation with TPD11+Y, TPB+Y, LgD10+Y and LgD2+Y treatments were significantly lower, 48, 35, 46 and 40%, respectively, and the lowest relative inhibition rate was reached under inoculation with TPD11+Y treatment.
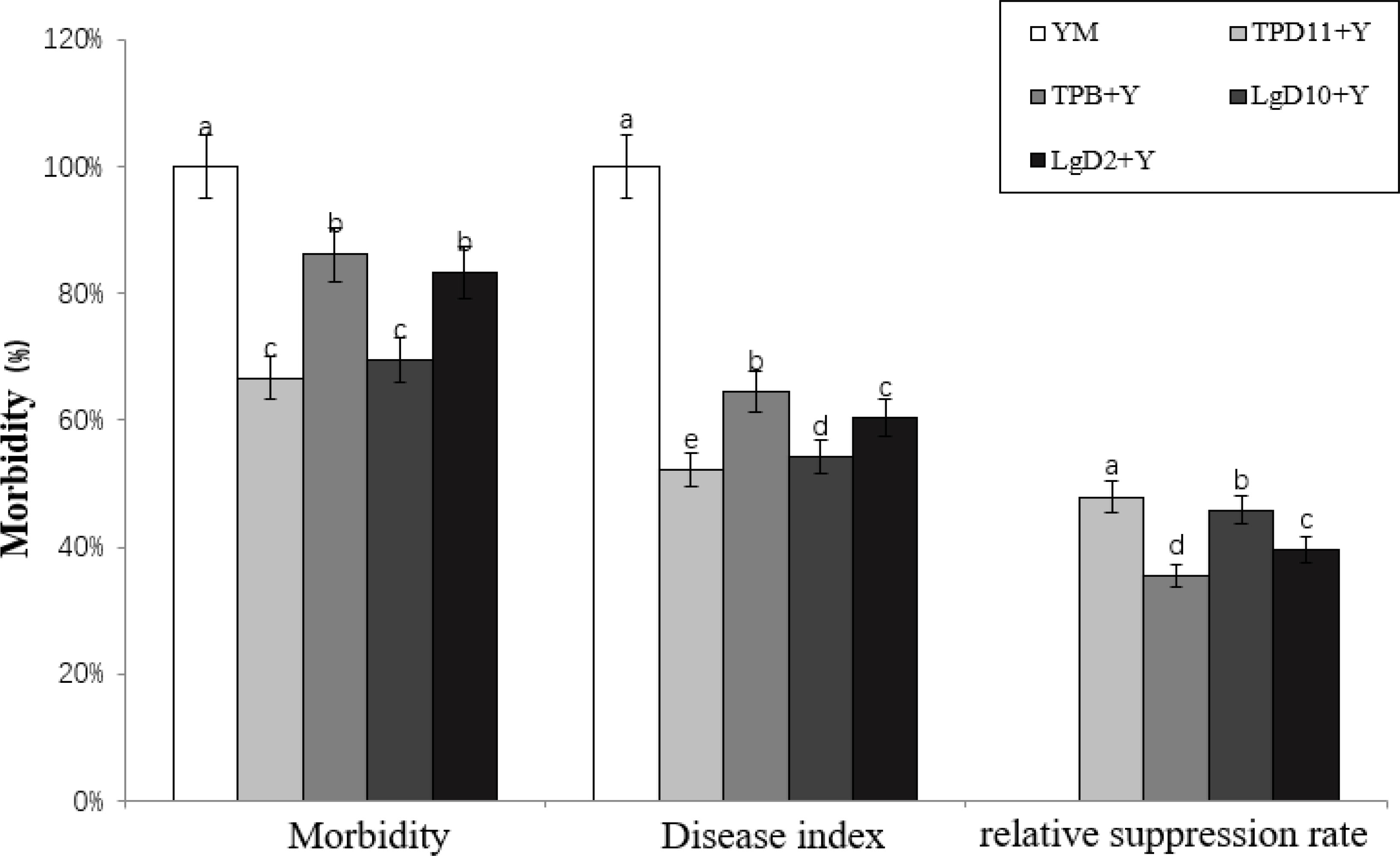
Figure 11 Incidence of tobacco after inoculation with symbiotic strains. SOD activity of tobacco under different strain treatments. Data are the means of three replicates (mean ± SE). Values followed by different letters indicate significant differences at P < 0.05 levels by Duncan’s multiple range tests.
3.6 Expression of defense enzymes and resistance-related genes by probiotic bacteria and fungi
3.6.1 Effect of probiotic antagonistic bacteria and fungi on defense enzyme-related genes
PPO, PAL, SOD and POD are all enzymes that play a role in disease resistance in tobacco, and the NtPPO, PAL, NtSOD and NbDADIR genes control the synthesis of these four enzymes. As shown in Figure 12, NtPPO gene expression was significantly upregulated in the TPD11 and TPB+Y inoculations compared to the control, 92% and 73% higher than the control, followed by both LgD10 and LgD2+Y treatments, 73% and 69% higher than the control, respectively. Compared to the control, PAL genes were upregulated in TPD11- and LgD10-treated plants, 94 and 58% higher than the control, respectively, while the remaining strains treated with PAL genes were not significantly different compared to the control. Plants inoculated with TPD11, LgD10 and LgD10+Y treatments had significantly upregulated NtSOD genes compared to the control, 99% and 65% higher than the control, respectively, while the NtSOD genes were not expressed in the plants of the remaining treatments. The expression of NbDADIR genes was upregulated in the inoculated TPD11, LgD10 and TPB+Y treatments, which were 99, 93 and 3% higher than the control, respectively, while the NbDADIR genes were not expressed in the plants of the remaining treatments. The expression of four enzyme genes, NtPPO, PAL, NtSOD and NbDADIR, peaked in tobacco leaves after inoculation with the TPD11 strain.
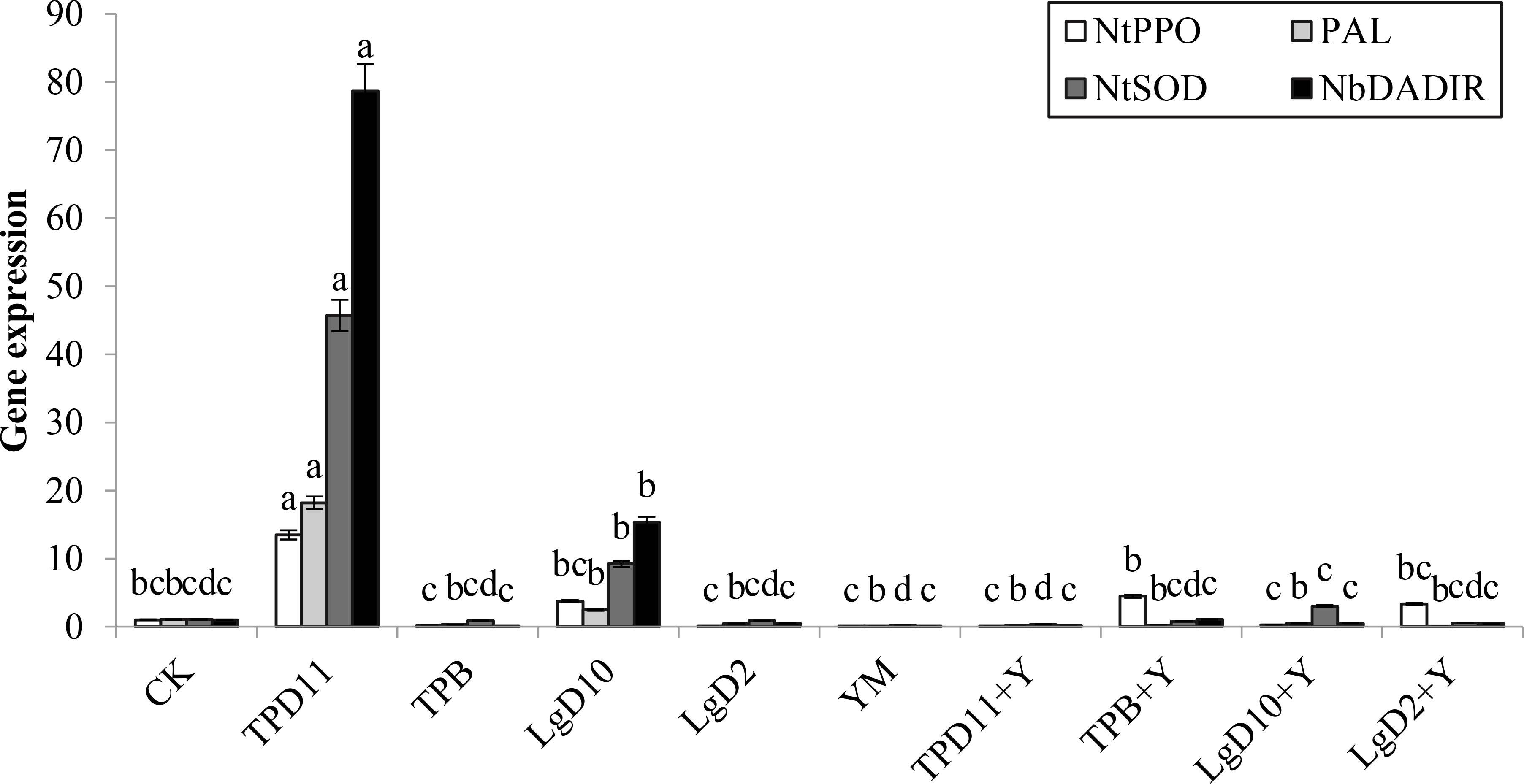
Figure 12 Gene expression under different strain treatments. SOD activity of tobacco under different strain treatments. Data are the means of three replicates (mean ± SE). Values followed by different letters indicate significant differences at P < 0.05 levels by Duncan’s multiple range tests.
3.6.2 The expression of the tobacco growth-related resistance genes
NtHSR203J, NtTTG2 and NtPR1a are related to the growth and disease resistance of tobacco. As shown in Figure 13, the expression of the NtHSR203J gene was significantly upregulated by the TPD11 and LgD10 treatments and was 95 and 85% higher than the expression of the NtHSR203J gene of the control, respectively, and the expression level of TPD11 was the highest. None of the other strains expressed these genes. The expression of the NtTTG2 gene was upregulated in the inoculated TPD11 and LgD10 treatments, which was 90 and 38% higher than the expression of the NtTTG2 gene in the control, respectively, and the NtTTG2 gene was not expressed in the remaining strain treatments, which is consistent with the results of NtHSR203J gene expression. The NtPR1a gene was not expressed in all strain inoculation treatments.
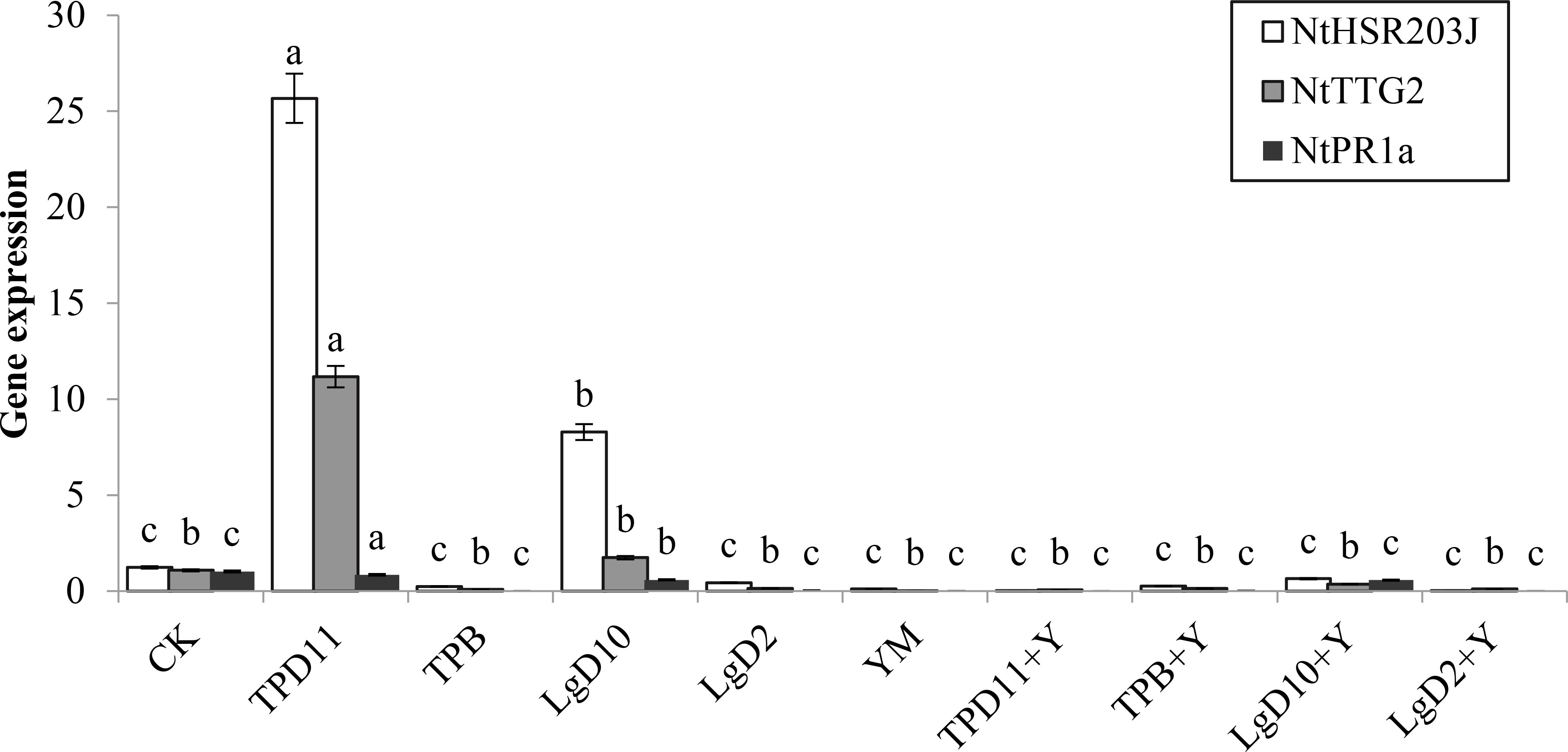
Figure 13 Gene expression under different strain treatments. SOD activity of tobacco under different strain treatments. Data are the means of three replicates (mean ± SE). Values followed by different letters indicate significant differences at P < 0.05 levels by Duncan’s multiple range tests.
3.6.3 Expression of resistance genes associated with black shank disease in tobacco
Tobacco NbRbohA and NbRbohB genes have important roles in tobacco resistance to Phytophthora infestans. As shown in Figure 14, the expression of the NbRbohA gene was upregulated in both the TPD11 and LgD10 treatments compared to the control, 80 and 89% higher than the control, respectively, and the expression of the NbRbohA gene in the LgD10+ Y treatment also showed upregulation of the NbRbohA gene, but the difference was not significant, and the NbRbohA gene was not expressed in the rest of the treated tobacco plants. The expression of the NbRbohB gene was significantly upregulated in both the TPD11 and LgD10 treatments and was 92 and 92% higher than the expression of the NbRbohB gene in the control, respectively. The NbRbohB gene was upregulated in both the LgD2 and LgD10+Y treatments, but the difference was not significant, and the NbRbohA gene was not expressed in the rest of the treated tobacco plants.
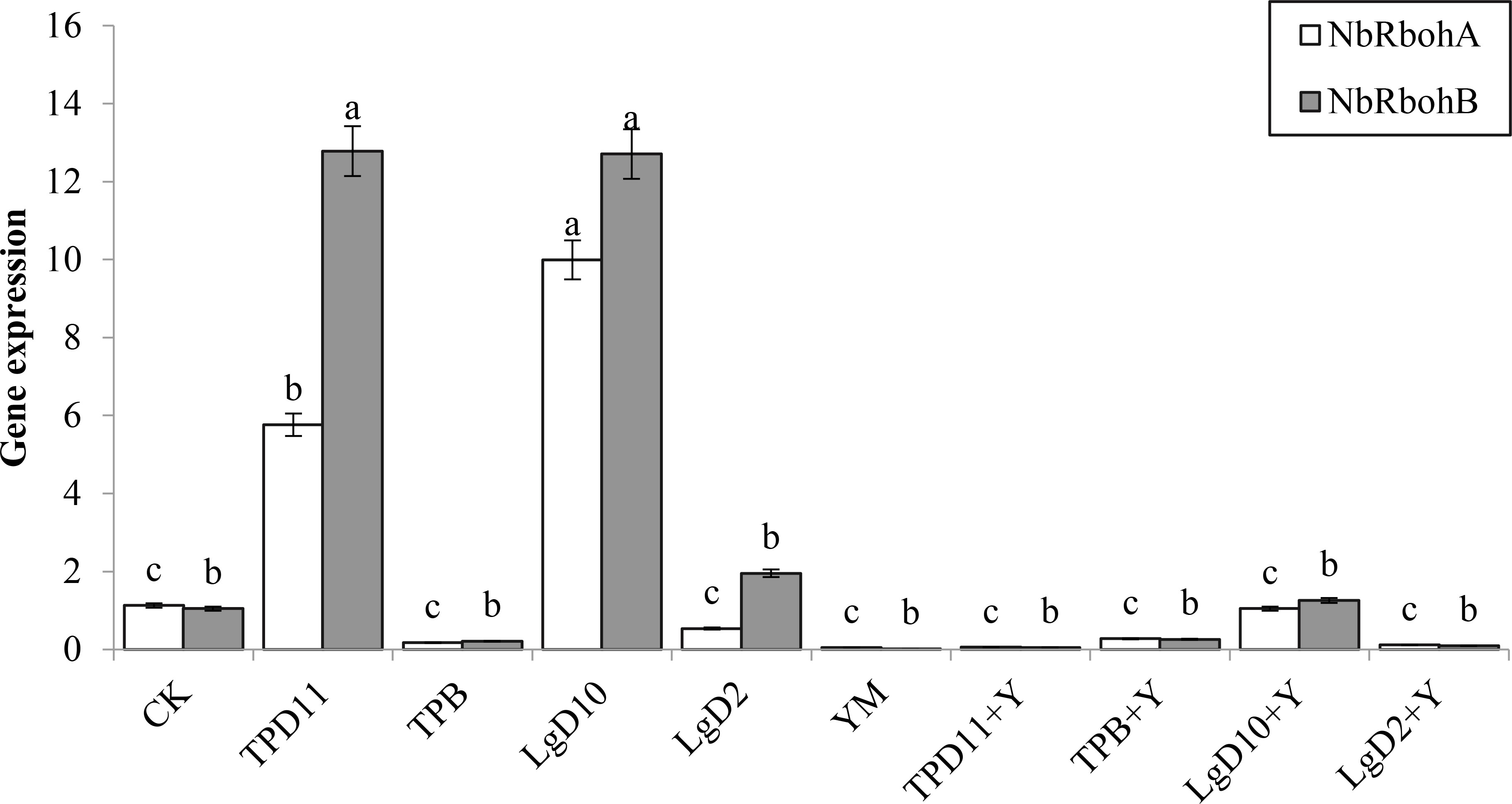
Figure 14 Gene expression under different strain treatments. SOD activity of tobacco under different strain treatments. Data are the means of three replicates (mean ± SE). Values followed by different letters indicate significant differences at P < 0.05 levels by Duncan’s multiple range tests.
4 Discussion
4.1 Functional studies on the probiotic antagonism of symbiotic strains
In this study, we isolated 73 symbiotic strains from the rhizomes and interrhizosphere soil of bacteria isolated from P. fargesii Franch. By screening all the symbiotic strains for their pro- and antagonistic effects, we aimed to screen the strains that showed the most promising applications in promoting the growth of P. polyphylla var. yunnanensis and the accumulation of the medicinal active ingredient saponin and controlled tobacco black shank. Symbiotic strains provide nitrogen, phosphorus and potassium nutrients to plants through biological nitrogen fixation, dissolved phosphate and solid potassium (Banerjee, 2005; Zhao et al., 2009; Nag et al., 2020), promote the absorption of nutrients by the host plant and provide the vitamins needed for plant growth. Symbiotic strains can increase the root surface area and length by producing IAA to stimulate plant cell elongation or affect cell division, thereby increasing plant access to more soil nutrients and directly promoting plant growth (Woodward and Bartel, 2005). The most powerful phosphate solubilizers are from the genera Bacillus, Rhizobium and Pseudomonas and nonsymbiotic nitrogen-fixing bacteria such as Azotobacter and the nitrogen-fixing spirochete Azospirillum (Saharan and Nehra, 2011). Symbiotic strains also have the ability to directly inhibit the growth of pathogenic bacteria (Ni et al., 2019), which helps to protect plants from certain pathogens. Most of the symbiotic strains obtained in this study had more than one growth-promoting function characteristic. By screening the symbiotic strains for in vitro growth-promoting and plate antagonistic functions, four strains of symbiotic strains with strong growth-promoting and antagonistic functions were obtained, two from the interrhizosphere and two from endophytes. Phylogenetic analysis showed that the four strains were TPD11 (Aspergillus tamarii), TPB (Fusarium oxysporum), LgD10 (Bacillus thuringiensis) and LgD2 (Bacillus cereus), and their growth-promoting effects on the content of parissaponin and their effects on the control of tobacco black shank were investigated.
4.2 The effect of probiotic strains on host plants
The growth of P. polyphylla var. yunnanensis was promoted by the growth-promoting antagonistic strains obtained in this study, mainly in terms of biomass, photosynthesis and chlorophyll. According to previous reports, inoculation with probiotic bacteria can promote the growth of host plants and increase biomass, photosynthetic efficiency and chlorophyll content (Chen et al., 2005; Nautiyal et al., 2008; Naveed et al., 2014). Two symbiotic strains of bacteria isolated from cucumber roots by Waqas et al. (2012) significantly increased the branch length, chlorophyll content, fresh weight and dry weight of rice. In this study, inoculation with four strains of probiotic bacteria increased the plant height, root weight, aboveground fresh weight, chlorophyll content, net photosynthetic rate, stomatal conductance, and transpiration rate of P. polyphylla var. yunnanensis to different degrees.
Some symbiotic strains have the potential to produce biosynthesis of the same or similar metabolites present in the host plant, in addition to promoting plant growth. Bashyal et al. (1999) isolated the endophytic bacterium Seimatoantlerium nepalense from redbud to produce paclitaxel, and Chithra et al. (2014) isolated Colletotrichum gloeosporioides from Piperaceae that had the ability to form piperine. In the present study, the symbiotic strains TPD11, TPB, LgD10 and LgD2 promoted the accumulation of total saponins of P. polyphylla var. yunnanensis to different degrees.
Previous studies have suggested that upregulation of genes related to the secondary metabolite synthesis pathway may contribute to the increased secondary metabolite content (Alam et al., 2021). Cui et al. (2017) isolated the endophytic fungus Trimmatostroma sp. from red buttercup that could induce the accumulation of rhodopsin and tyrosol by regulating the expression activity of key enzyme genes. The endophytic fungus Paraconiothyrium SSM001 (Soliman et al., 2013) enhances paclitaxel content in host plants by upregulating the genes TS, DXR, and HMGR, which are related to paclitaxel synthesis. Mevalonate (MVA) is the main pathway for the synthesis of steroidal saponins, and HMGS, GPPS, FPPS, SS, CAS, and SE are key genes in the MVA pathway (Mishra et al., 2016; Yang et al., 2017), so it is important to study the expression of related genes for saponin synthesis. We showed that the expression levels of HMGS, GPPS, FPPS, SS, CAS and SE genes were significantly upregulated after inoculation with probiotic bacteria, with the highest expression of HMGS, SE2 and CAS under the LgD10 strain treatment, the highest expression of GGPS and FPPS under the TPD11 strain treatment, and the highest expression of SE1 under the LgD2 treatment.
4.3 Effect of inoculation with growth-promoting strains on tobacco
Symbiotic strains can indirectly improve plant growth by resisting harmful pathogens or inhibiting the growth of pathogenic bacteria in a variety of ways, reducing the impact of pests and diseases on plant growth and development. Gang et al. (Gang, 1992) inoculated cucumber seeds with probiotic antagonistic bacteria, which significantly reduced the anthracnose of cucumber leaves compared to the uninoculated control. Silosuh et al. (Silo-Suh et al., 1994) isolated a strain of Bacillus cereus UW85 that controlled alfalfa damping-off by inhibiting oomycete pathogens and producing two antimicrobial substances, aminopolyols and aminoglycosides. Baskar et al. (2020) isolated and identified an endophytic fungus from the cactus Aspergillus tamarii based on the fact that its mycelial metabolite ethyl acetate has a good insecticidal effect. An efficient system for the bioconversion of sucrose to oligofructose (FOS) was obtained using Aspergillus tamarii NKRC 1229 mycelial fructosyltransferase (Choukade and Kango, 2019). Akhtar (Akhtar et al., 2021) isolated 15 strains from heavy metal-contaminated soil and screened them for heavy metal tolerance and plant promotion potential to identify the most efficient strain, Bacillus cereus. Significantly improved plant growth and reduced chromium toxicity after inoculation with Bacillus cereus also improved photosynthetic pigments, i.e., chlorophyll a, chlorophyll b, chlorophyll a+b and carotenoids also increased plant superoxide dismutase activity SOD. Fravel et al. (2003) showed that the nonpathogenic Fusarium oxysporum shows several modes of action that contribute to its biocontrol capacity. They can compete for nutrients in the soil and thus affect the germination rate of thick-walled spores of the pathogen. They can also compete for infection sites in the roots and can trigger plant defense responses and induce systemic resistance. Arabidopsis thuringiensis reduced the levels of cytokinin, gibberellin, jasmonic acid and abscisic acid and increased the levels of indole-3-acetic acid IAA and salicylic acid SA after 24 h of treatment with Bacillus thuringiensis (Subramanian, 2019). Jung et al. (2008) treated Bacillus thuringiensis to increase phenolic production, phenylalanine deaminase activity (a lignification-related enzyme) and levels of peroxidase and superoxide dismutase (antioxidant enzymes) in 2-week-old soybean plants, indicating that it stimulated defense system responses. In this study, the biocontrol ability of four strains of probiotic bacteria and fungi was determined, and pathogenic fungal antagonism experiments and pot tests showed that all four strains of probiotic antagonistic bacteria inhibited the growth of tobacco black shank, significantly reduced the incidence and disease index of tobacco black shank and promoted plant growth. The most significant effects of the TPD11+Y and LgD10+Y treatments were observed in the antagonistic group.
Biotrophic bacteria were shown to be able to promote host plant growth and disease resistance by inducing defense enzyme activities, similar to the mechanism of innate plant disease resistance. Polyphenol oxidase (PPO) and phenylalanine aminolase (PAL) are secondary metabolism-related enzymes, and the higher the PPO and PAL activities of the plant are, the greater its disease resistance (Schneider et al., 1994). The synergistic action of superoxide dismutase (SOD) and peroxidase (POD) can scavenge superoxide radicals and prevent peroxide-induced cell membrane damage (Benliang et al., 2019). Wang et al. (2020) found that inoculation with Pseudomonas aeruginosa strain CQ-4 significantly promoted tomato seed germination and seedling growth and induced upregulation of the activities of four disease-resistant defense enzymes (PPO, POD, SOD and PAL) in plants, thereby effectively controlling Botrytis cinerea. Chowdappa (Chowdappa et al., 2013) reported increased host enzyme activities induced by Bacillus subtilis, including POD, PPO and SOD, and various hormone synthesizing enzymes to induce ISR against early and late blight of tomato seedlings.
4.3 Effect of probiotic strains on the expression of related genes
In this study, inoculation with four strains of probiotic antagonist bacteria promoted the activity of tobacco PPO, POD, SOD and PAL defense enzymes to different degrees, and the probiotic group of TPD11 and LGD10 strains had the most significant effect on defense enzyme activity. Previous studies have shown that the NtPPO, PAL, NtSOD and NbDADIR genes are genes that control the synthesis of four enzymes, PPO, PAL, SOD and POD, respectively (Li et al., 2020); the NbRbohA and NbRbohB genes play an important role in tobacco resistance to Phytophthora nicotianae infection (Yoshioka et al., 2003); and the NtHSR203J, NtTTG2 and NtPR1a genes are associated with growth and disease resistance in tobacco (Li et al., 2012; Xu et al., 2013). In the present study, these nine genes were analyzed in terms of disease resistance performance and growth promotion, and only the expression of these nine genes was upregulated in the inoculated TPD11 and LgD10 strain treatments compared to the control, while the rest of the genes were not expressed in the strain treatments.
In summary, in this study, a total of 73 strains of symbiotic bacteria were isolated from the rhizomes and interrhizosphere soil of P. fargesii Franch. A comprehensive analysis of the disease-promoting effects of symbiotic strains shows that four strains, TPD11, TPB, LgD10 and LgD2, were found to have significant effects on regulating the growth of P. polyphylla var. yunnanensis, promoting the accumulation of saponin VI and the disease resistance of tobacco.
Data availability statement
The datasets presented in this study can be found in online repositories. The names of the repository/repositories and accession number(s) can be found below: GenBank, accession numbers OP404241, OP404360, OP405007 and OP405014.
Author contributions
LT conceived and designed the experiments and wrote this paper. DY and XWanalyzed the data. CX and SZ prepared the plant materials. All authors contributed to the article and approved the submitted version.
Funding
This work was supported by the Key R & D program of Yunnan Province, China (grant no. 202103AC100003) and the National Natural Science Foundation of China (Grant No. 31860075).
Conflict of interest
The authors declare that the research was conducted in the absence of any commercial or financial relationships that could be construed as a potential conflict of interest.
Publisher’s note
All claims expressed in this article are solely those of the authors and do not necessarily represent those of their affiliated organizations, or those of the publisher, the editors and the reviewers. Any product that may be evaluated in this article, or claim that may be made by its manufacturer, is not guaranteed or endorsed by the publisher.
Abbreviations
PPO, Polyphenol oxidase; PAL, Phenylalanine aminolase; SOD, Superoxide dismutase; POD, Peroxidase; PDA, Potato Dextrose Agar (Medium); LB, Luria-Bertani (Medium); Pn, Net photosynthetic rate; Gs, Stomatal conductance; Ci, Intercellular CO2 concentration; Tr, Transpiration rate; HPLC, High-performance liquid chromatography; HMGS, Hydroxymethylglutaryl coenzyme A synthase; GPPS, Geranyl diphosphate synthase; FPPS, Farnesyl pyrophosphate synthase; SS, Squalene synthase; SE, Squalene epoxidase; CAS, Cycloartenol synthase; IAA, Indole acetic acid.
References
Ahmed A., Hasnain S. (2010). Auxin producing Bacillus sp.: Auxin quantification and effect on the growth of solanum tuberosum. Pure Appl. Chem. 82, 313–319. doi: 10.1351/PAC-CON-09-02-06
Akhtar N., Ilyas N., Yasmin H., Sayyed R. Z., Hasnain Z., Elsayed E. A., et al. (2021). Role of bacillus cereus in improving the growth and phytoextractability of brassica nigra (L.) K. Koch Chromium Contaminated Soil. Molecules. 26, 1569. doi: 10.3390/OLECULES26061569
Alam B., Lï J., Gě Q., Khan M. A., Gōng J., Mehmood S., et al. (2021). Endophytic fungi: From symbiosis to secondary metabolite communications or vice versa? Front. Plant Sci. 12, 791033. doi: 10.3389/fpls.2021.791033
Alori E. T., Glick B. R., Babalola O. O. (2017). Microbial phosphorus solubilization and its potential for use in sustainable agriculture. Front. Microbiol. 8, 971. doi: 10.3389/fmicb.2017.00971
Banerjee M. R. (2005). “Plant-growth-promoting rhizobacteria as biofertilizers and biopesticides,” in Handbook of microbial biofertilizers. Ed. Rai M. K. (New York: Food Products Press Inc), 137–181.
Bashyal B., Li J. Y., Strobel G., Hess W. M., Sidhu R. (1999). Seimatoantlerium nepalense, an endophytic taxol producing coelomycete from himalayan yew (taxus wallachiana). Mycotaxon 72, 33–42.
Baskar K., Chinnasamy R., Pandy K., Venkatesan M., Sebastian P. J., Subban M., et al. (2020). Larvicidal and histopathology effect of endophytic fungal extracts of Aspergillus tamarii against aedes aegypti and Culex quinquefasciatus. Heliyon 6, e05331. doi: 10.1016/j.heliyon.2020.e05331
Benliang D., Mingxin G., Hongxia L., Shan T., Xusheng Z. (2019). Inhibition of autophagy by hydroxychloroquine enhances antioxidant nutrients and delays postharvest fruit senescence of ziziphus jujuba. Food Chem. 296, 56–62. doi: 10.1016/j.foodchem.2019.05.189
Chen X. M., Guo S. X., Wang C. L. (2005). Effects of four endophytic fungi on the growth and polysaccharide content of anoectochilus roxburghii(wall.) lindl. J. Chin. Pharm. ences 40, 13–16. doi: 10.3321/j.issn:1001-2494.2005.01.006
Chen L., Shi H., Heng J., Wang D., Bian K. (2019). Antimicrobial, plant growth-promoting and genomic properties of the peanut endophyte bacillus velezensis LDO2. Microbiol. Res. 218, 41–48. doi: 10.1016/j.micres.2018.10.002
Chithra S., Jasim B., Sachidanandan P., Jyothis M., Radhakrishnan E. K. (2014). Piperine production by endophytic fungus colletotrichum gloeosporioides isolated from piper nigrum. Phytomedicine 21, 534–540. doi: 10.1016/j.phymed.2013.10.020
Choukade R., Kango N. (2019). Characterization of a mycelial fructosyltransferase from aspergillus tamarii NKRC 1229 for efficient synthesis of fructooligosaccharides. Food Chem. 286, 434–440. doi: 10.1016/j.foodchem.2019.02.025
Chowdappa P., Kumar S., Lakshmi M. J., Upreti K. K. (2013). Growth stimulation and induction of systemic resistance in tomato against early and late blight by bacillus subtilis otpb1 or trichoderma harzianum otpb3. Biol. Control 65, 109–117. doi: 10.1016/j.biocontrol.2012.11.009
Cui X. L., Shi-Gui L. I., Yang J., Fan X. C., Jin-Gang G. U. (2014). Screening and identification of saline-alkali tolerant and tobacco black shank resistant trichoderma. J. Agr Sci. Tech-Iran 16, 81–89. doi: 10.13304/j.nykjdb.2013.450
Cui J. L., Wang Y. N., Jiao J., Gong Y., Wang J. H., Wang M. L. (2017). Fungal endophyte-induced salidroside and tyrosol biosynthesis combined with signal cross-talk and the mechanism of enzyme gene expression in rhodiola crenulata. Sci. Rep. 7, 12540. doi: 10.1038/s41598-017-12895-2
Ding H., Mo W., Yu S., Cheng H., Peng L., Liu Z. (2021). Whole genome sequence of Bacillus velezensis strain GUMT319: A potential biocontrol agent against tobacco black shank disease. Front. Microbiol. 12, 658113. doi: 10.3389/fmicb.2021.658113
Edlayne G., Simone A., Felicio J. D. (2009). Chemical and biological approaches for mycotoxin control: a review. Recent Pat. Food Nutr. Agric. 1, 155–161. doi: 10.2174/2212798410901020155
Eyberger A. L., Dondapati R., Porter J. R. (2006). Endophyte fungal isolates from podophyllum peltatum produce podophyllotoxin. J. Nat. Prod 69, 1121–1124. doi: 10.1021/np060174f
Fravel D., Olivain C., Alabouvette C. (2003). Fusarium oxysporum its biocontrol. New Phytol. 157, 493–502. doi: 10.1046/j.1469-8137.2003.00700.x
Gang W. (1992). Induction of systemic resistance of cucumber to colletotrichum orbiculare by select strains of plant growth-promoting rhizobacteria. Phytopathology 81, 1508–1512.
Glick B. R. (1995). The enhancement of plant growth by free-living bacteria. Can. J. Microbiol. 41, 109–117. doi: 10.1139/m95-015
Guo D., Yuan C., Luo Y., Chen Y., Lu M., Chen G., et al. (2020). Biocontrol of tobacco black shank disease (Phytophthora nicotianae) by Bacillus velezensis Ba168. Pestic Biochem. Physiol. 165, 104523. doi: 10.1016/j.pestbp.2020.01.004
Hassan S. E. (2017). Plant growth-promoting activities for bacterial and fungal endophytes isolated from medicinal plant of Teucrium polium l. J. Adv. Res. 8, 687–695. doi: 10.1016/j.jare.2017.09.001
Jasim B., Jimtha J., Jyothis M., Radhakrishnan K. (2013). Plant growth promoting potential of endophytic bacteria isolated from piper nigrum. Plant Growth Regul. 71, 1–11. doi: 10.1007/s10725-013-9802-y
Ji P., Csinos A. S., Hickman L. L., Hargett U. (2014). Efficacy and application methods of oxathiapiprolin for management of black shank on tobacco. Plant Dis. 98, 1551–1554. doi: 10.1094/PDIS-02-14-0172-RE
Jung W. J., Mabood F., Souleimanov A., Smith D.L. (2008). Effect of chitin hexamer and thuricin 17 on lignification-related and antioxidative enzymes in soybean plants. J. Plant Biol. 51, 145–149. doi: 10.1007/BF03030724
Kosecka-Strojek M., Sabat A. J., Akkerboom V., Kooistra-Smid A. M. D. M., Miedzobrodzki J., Friedrich A. W. (2019). Development of a reference data set for assigning streptococcus and enterococcus species based on next generation sequencing of the 16S-23S rRNA region. Antimicrob. Resist. Infect. Control 8, 178. doi: 10.1186/s13756-019-0622-3
Kusari S., Zühlke S., Spiteller M. (2009). An endophytic fungus from camptotheca acuminata that produces camptothecin and analogues. J. Nat. Prod 72, 2–7. doi: 10.1021/np800455b
Li W., Dang C., Ye Y., Wang Z., Hu B., Zhang F., et al. (2020). Overexpression of grapevine VvIAA18 gene enhanced salt tolerance in tobacco. Int. J. Mol. Sci. 21, 1323. doi: 10.3390/ijms21041323
Li B., Gao R., Cui R., Lü B., Li X., Zhao Y., et al. (2012). Tobacco TTG2 suppresses resistance to pathogens by sequestering NPR1 from the nucleus. J. Cell Sci. 125, 4913–4922. doi: 10.1242/jcs.111922
Li Y., Man S., Li J., Chai H., Fan W., Liu Z., et al. (2014). The antitumor effect of formosanin c on HepG2 cell as revealed by 1H-NMR based metabolic profiling. Chem. Biol. Interact. 220, 193–199. doi: 10.1016/j.cbi.2014.06.023
Li J., Zheng B., Hu R., Liu Y., Jing Y., Xiao Y., et al. (2019). Pseudomonas species isolated from tobacco seed promote root growth and reduce lead contents in nicotiana tobacum K326. Can. J. Microbiol. 65, 214–223. doi: 10.1139/cjm-2018-0434
Li W., Zhou J., Lin Z., Hu Z. (2007). Study on fermentation condition for production of huperzine a from endophytic fungus 2F09P03B of huperzia serrata. Chin. Med. Biotechnol. 2, 254–259. doi: 10.3969/j.issn.1673-713X.2007.04.004
Ma L., Zhang H. Y., Zhou X. K., Yang C. G., Zheng S. C., Duo J. L., et al. (2018). Biological control tobacco bacterial wilt and black shank and root colonization by bio-organic fertilizer containing bacterium pseudomonas aeruginosa NXHG29. Appl. Soil Ecol. 129, 136–144. doi: 10.1016/j.apsoil.2018.05.011
Min Q., Xie R. S., Shi Y., Zhang H., Chen H. M. (2010). Isolation and identification of two flavonoid-producing endophytic fungi from Ginkgo biloba l. Ann. Microbiol. 60, 143–150. doi: 10.1007/s13213-010-0016-5
Mishra S., Bansal S., Mishra B., Sangwan R. S., Asha, Jadaun J. S., et al. (2016). RNAi and homologous over-expression based functional approaches reveal triterpenoid synthase gene-cycloartenol synthase is involved in downstream withanolide biosynthesis in withania somnifera. PloS One 11, e0149691. doi: 10.1371/journal.pone.0149691
Nag P., Shriti S., Das S. (2020). Microbiological strategies for enhancing biological nitrogen fixation in nonlegumes. J. Appl. Microbiol. 129, 186–198. doi: 10.1111/jam.14557
Nautiyal C. S., Govindarajan R., Lavania M., Pushpangadan P. (2008). Novel mechanism of modulating natural antioxidants in functional foods: involvement of plant growth promoting rhizobacteria NRRL b-30488. J. Agric. Food Chem. 56, 4474–4481. doi: 10.1021/jf073258i
Naveed M., Hussain M. B., Zahir Z. A., Mitter B., Sessitsch A. (2014). Drought stress amelioration in wheat through inoculation with burkholderia phytofirmans strain psjn. Plant Growth Regul. 73, 121–131. doi: 10.1007/s10725-013-9874-8
Ni M., Wu Q., Wang H. L., Liu W. C., Hu B., Zhang D. P., et al. (2019). Identification of a novel strain, streptomyces blastmyceticus JZB130180, and evaluation of its biocontrol efficacy against monilinia fructicola. J. Zhejiang Univ Sci. B 20, 84–94. doi: 10.1631/jzus.B1700609
Ogura-Tsujita Y., Okubo H. (2006). Effects of low nitrogen medium on endogenous changes in ethylene, auxins, and cytokinins in in vitro shoot formation from rhizomes of cymbidium kanran. In Vitro Cell Dev-Pl 42, 614–616. doi: 10.1079/IVP2006823
Pérez-Montaño F., Alías-Villegas C., Bellogín R. A., del Cerro P., Espuny M. R., Jiménez-Guerrero I., et al. (2014). Plant growth promotion in cereal and leguminous agricultural important plants: from microorganism capacities to crop production. Microbiol.Res 169, 325–336. doi: 10.1016/j.micres.2013.09.011
Saharan B. S., Nehra V. (2011). Plant growth promoting rhizobacteria: A critical review. Life Sci. Med. Res. 7, 52–63. doi: 10.1016/S1002-0160(19)60839-8
Sang S., Li S., Fan W., Wang N., Gao M., Wang Z. (2019). Zinc thiazole enhances defense enzyme activities and increases pathogen resistance to ralstonia solanacearum in peanut (Arachis hypogaea) under salt stress. PloS One 14, e0226951. doi: 10.1371/journal.pone.0226951
Schneider S., Ullrich W. R., Pathology M. P. (1994). Differential induction of resistance and enhanced enzyme activities in cucumber and tobacco caused by treatment with various abiotic and biotic inducers. Physiological. 45, 291–304. doi: 10.1016/S0885-5765(05)80060-8
Sherameti I., Shahollari B., Venus Y., Altschmied L., Varma A., Oelmüller R. (2005). The endophytic fungus piriformospora indica stimulates the expression of nitrate reductase and the starch-degrading enzyme glucan-water dikinase in tobacco and arabidopsis roots through a homeodomain transcription factor that binds to a conserved motif in their promoters. J. Biol. Chem. 280, 26241–26247. doi: 10.1074/jbc.M500447200
Silo-Suh L. A., Lethbridge B. J., Raffel S. J., He H., Clardy J., Handelsman J. (1994). Biological activities of two fungistatic antibiotics produced by bacillus cereus UW85. Appl. Environ. Microbiol. 60, 2023–2030. doi: 10.1128/aem.60.6.2023-2030.1994
Soliman S. S., Trobacher C. P., Tsao R., Greenwood J. S., Raizada M. N. (2013). A fungal endophyte induces transcription of genes encoding a redundant fungicide pathway in its host plant. BMC Plant Biol. 13, 93. doi: 10.1186/1471-2229-13-93
Stierle A., Strobel G., Stierle D. (1993). Taxol and taxane production by taxomyces andreanae, an endophytic fungus of pacific yew. Science 260, 214–216. doi: 10.1126/science.8097061
Subramanian S. (2019). Mass spectrometry based proteome profiling to understand the effects of lipo-chito-oligosaccharide and thuricin 17 in arabidopsis thaliana and glycine max under salt stress. J. Exp. Med. 51, 1–10.
Wagi S., Ahmed A. (2019). Bacillus spp.: potent microfactories of bacterial IAA. PeerJ 7, e7258. doi: 10.7717/peerj.7258
Wang X., Zhou X., Cai Z., Guo L., Chen X., Chen X., et al. (2020). A biocontrol strain of pseudomonas aeruginosa CQ-40 promote growth and control botrytis cinerea in tomato. Pathogens 10, 22. doi: 10.3390/pathogens10010022
Waqas M., Khan A. L., Kamran M., Hamayun M., Kang S. M., Kim Y. H., et al. (2012). Endophytic fungi produce gibberellins and indoleacetic acid and promotes host-plant growth during stress. Molecules 17, 10754–10773. doi: 10.3390/molecules170910754
Wen F., Chen T., Yin H., Lin J., Zhang H. (2019). In vitro effects on thrombin of Paris saponins and In vivo hemostatic activity evaluation of Paris fargesii var. brevipetala. Molecules 24, 1420. doi: 10.3390/molecules24071420
Wen Y. S., Ni W., Qin X. J., Yan H., Chen C. X., Hua Y., et al. (2015). Steroidal saponins with cytotoxic activity from the rhizomes of Paris polyphylla var. yunnanensis. Phytochem. Lett. 12, 31–34. doi: 10.1016/j.phytol.2015.02.011
Woodward A. W., Bartel B. (2005). Auxin: regulation, action, and interaction. Ann. Bot. 95, 707–735. doi: 10.1093/aob/mci083
Wu S. S., Gao W. Y., Duan H. Q., Jia W. (2004). Advances in studies on chemical constituents and pharmacological activities of Rhizoma paridis. Chin. Tradit Herb Drugs 35, 344–347. doi: 10.3321/j.issn:0253-2670.2004.03.049
Xu Y. Y. (2017). Screening provenances of Paris polyphylla var. yunnanensis(Franch.) hand.-mazz. and identification of genes related to saponin synthesis. Chin. Acad. Forestry. doi: 10.27625/d.cnki.gzlky.2019.000053
Xu H., Xu W., Xi H., Ma W., He Z., Ma M. (2013). The ER luminal binding protein (BiP) alleviates Cd(2+)-induced programmed cell death through endoplasmic reticulum stress-cell death signaling pathway in tobacco cells. J. Plant Physiol. 170, 1434–1441. doi: 10.1016/j.jplph.2013.05.017
Xu Y., Zhao S., Dong X., Ye X. (2017). Cloning and expression of squalene epoxidase from Paris polyphylla var. yunnanensis. Chin. Traditional Herbal Drugs 48 (9), 6. doi: 10.1016/j.jplph.2013.05.017
Yang Y., Ge F., Sun Y., Liu D., Chen C. (2017). Strengthening triterpene saponins biosynthesis by over-expression of farnesyl pyrophosphate synthase gene and RNA interference of cycloartenol synthase gene in panax notoginseng cells. Molecules 22, 581. doi: 10.3390/molecules22040581
Yoshioka H., Numata N., Nakajima K., Katou S., Kawakita K., Rowland O., et al. (2003). Nicotiana benthamiana gp91phox homologs NbrbohA and NbrbohB participate in H2O2 accumulation and resistance to phytophthora infestans. Plant Cell 15, 706–718. doi: 10.1105/tpc.008680
Zhang M., Riaz M., Liu B. (2020). Two-year study of biochar: Achieving excellent capability of potassium supply via alter clay mineral composition and potassium-dissolving bacteria activity. Sci. Total Environ. 717, 137286. doi: 10.1016/j.scitotenv.2020.137286
Zhang X., Zhou Y., Li Y., Fu X., Wang Q. (2017). Screening and characterization of endophytic Bacillus for biocontrol of grapevine downy mildew. Crop Prot 96, 173–179. doi: 10.1016/j.cropro.2017.02.018
Zhao Y., Kang L. P., Liu Y. X., Liang Y. G., Tan D. W., Yu Z. Y., et al. (2009). Steroidal saponins from the rhizome of Paris polyphylla and their cytotoxic activities. Planta Med. 75, 356–363. doi: 10.1055/s-0028-1088380
Keywords: Paris fargesii Franch, tobacco, steroid saponin, symbiotic strains, tobacco black shank
Citation: Yan D, Wang X, Xi C, Zi S and Liu T (2022) Isolation and identification of symbiotic strains in Paris fargesii Franch that promote disease resistance. Front. Agron. 4:1021028. doi: 10.3389/fagro.2022.1021028
Received: 16 August 2022; Accepted: 19 October 2022;
Published: 01 November 2022.
Edited by:
Basharat Ali, Khwaja Fareed University of Engineering and Information Technology (KFUEIT), PakistanReviewed by:
Ling Xu, Zhejiang Sci-Tech University, ChinaShahbaz Atta Tung, Pir Mehr Ali Shah Arid Agriculture University, Pakistan
Copyright © 2022 Yan, Wang, Xi, Zi and Liu. This is an open-access article distributed under the terms of the Creative Commons Attribution License (CC BY). The use, distribution or reproduction in other forums is permitted, provided the original author(s) and the copyright owner(s) are credited and that the original publication in this journal is cited, in accordance with accepted academic practice. No use, distribution or reproduction is permitted which does not comply with these terms.
*Correspondence: Tao Liu, NTIxMzM0OTBAcXEuY29t
†These authors have contributed equally to this work