- 1Department of Agronomy, University of Wisconsin-Madison, Madison, WI, United States
- 2Department of Soil Science, University of Wisconsin-Madison, Madison, WI, United States
Waterhemp is a troublesome weed species in cropping systems throughout Wisconsin and much of the US Midwest. Coupled with extended emergence window, rapid growth, high genetic diversity, and herbicide resistance to multiple sites of action, waterhemp represents a major challenge for chemical-based weed control. The objective of this experiment was to evaluate the impact of soybean and corn canopy on growth and development of multiple waterhemp cohorts in 2019 and 2020. Treatments consisted of narrow- (38 cm row spacing) and wide-row (76 cm row spacing) soybeans, corn (76 cm row spacing), and fallow (no crop), with 6 waterhemp transplant timings (cohorts) in 2019 and 2020, respectively. Waterhemp seedlings grown in the greenhouse were transplanted at the 2-3 true leaves growth stage to the field at 10-day increments throughout the typical waterhemp emergence season for Wisconsin (June-July). Waterhemp plants were measured for height and harvested for biomass when they reached the flowering stage. The number of days from the first transplant timing to result in 50% and 90% biomass and height reduction (ED50 and ED90) of subsequent waterhemp cohorts were estimated and used as indicators of weed suppression ability by the respective crop. Narrow-row soybeans required fewer days to suppress 50% growth of new waterhemp cohorts followed by corn, wide row soybeans, and fallow, respectively (4, 6, 9, and 14 d for 50% biomass reduction, and 10, 14, 18, and 42 d for 50% height reduction, respectively). Similarly, narrow-row soybeans required fewer days to suppress 90% biomass of waterhemp, followed by corn, wide-row soybeans, and fallow (11, 15, 18, and 78 d, respectively). Conversely, narrow and wide-row soybeans required fewer days to suppress 90% height of new waterhemp cohorts followed by corn and fallow (20, 26, 43, and 85 d, respectively). Rapid soybean canopy closure (e.g., planting soybeans earlier and in narrow row-spacing) combined with other integrated weed management practices have the potential to reduce reliance on POST herbicide application and mitigate herbicide resistance evolution. In corn, mid- to late-season monitoring and management of later emerging waterhemp cohorts is recommended due to longer time required to reach 90% waterhemp growth suppression.
Introduction
In most developed regions of the world, herbicides are already used as the primary mean of weed control in cropping systems, while in developing regions, population migration from rural to urban areas is reducing labor availability for hand-weeding and other labor-intensive activities as means of weed control, leading to a higher reliance on chemical weed control (Gianessi, 2013). Herbicide resistance is the consequence of high reliance on chemical weed control whereas 513 unique cases of herbicide resistance have been documented globally (Heap, 2022). In the United States, the introduction of glyphosate-resistant (GR) soybean, cotton, and corn in 1996, 1997, and 1998, respectively, revolutionized POST-emergence weed control in these crops reducing overall chemical costs, reliance on tillage, and labor requirements given glyphosate’s broad-spectrum efficacy (Johnson et al., 2000; Reddy and Whiting, 2000; Bradley et al., 2004). The high reliance on glyphosate has however resulted in widespread selection and occurrence of glyphosate-resistant weeds (Powles, 2008; Givens et al., 2009; Duke, 2015), with 55 glyphosate-resistant species reported globally, 17 in the United States (Heap, 2022).
Waterhemp (Amaranthus tuberculatus Moq.) is a C4 summer annual species in the pigweed (Amaranthaceae) family highly adapted to current corn and soybean cropping systems due to extended emergence window (mid-May to mid-July), fast growth, acclimation to adverse conditions such as shading and drought, and high seed production (Keeley et al., 1987; Horak and Loughin, 2000; Sellers et al., 2003; Jha and Norsworthy, 2009; Jha et al., 2009; Werle et al., 2014). Waterhemp is ranked as one of the most troublesome weeds in corn and soybean production in the Midwest (Van Wychen, 2019). In Wisconsin, waterhemp has been documented in 61 of 72 counties (Zimbric et al., 2018) whereas the first glyphosate resistance case was confirmed in 2013 by Butts and Davis (2015). To confront herbicide resistance, integrated weed management (IWM) with the emphasis on systematically applied weed control measures including cultural, genetic, mechanical, biological, and chemical tactics need to be restored, with the aim to reduce weed-crop interference and weed fecundity, while favoring crop yield potential and profit (Shaw, 1982; Walker and Buchanan, 1982; Regnier and Janke, 1990; Swanton and Weise, 1991; Swanton and Murphy, 1996; Liebman and Staver, 2001; Blackshaw et al., 2008; Butts et al., 2016).
Soybean [(Glycine max (L.) Merr] and corn (Zea mays L.) are globally important crops and key components of annual cropping system rotations in the United States, accounting for 33.9 and 37.2 million planted crop ha in 2020, respectively (USDA-NASS, 2020). Approximately 830 thousand and 1.6 million ha of soybean and corn, respectively, were planted in Wisconsin in 2020 (USDA-NASS, 2020) and are commonly rotated every growing season (Stanger and Lauer, 2008). Soybean is not a strong competitor during the early developmental stages (Jannink et al., 2000), and unlike corn, has limited effective POST-emergence chemical weed control options (Lingenfelter and Wallace, 2021). In response to the occurrence of herbicide-resistant weeds, PRE-emergence herbicides with soil residual activity are once again being commonly used due to limited options of POST chemical control, and their integration is an effective strategy for the management of herbicide-resistant weeds (Norsworthy et al., 2012; Ribeiro et al., 2021). However, herbicides with soil residual activity applied PRE (e.g., at crop planting) may not have sufficient residual efficacy to control later-emerging cohorts of weeds such as waterhemp throughout the growing season (Hager et al., 2002), which in many cases requires subsequent applications of POST herbicides and/or other means for season-long weed control. Earlier canopy closure can limit the amount of light reaching the soil surface, thus, impacting weed seed germination, establishment, and growth (Norsworthy and Oliveira, 2007; Sanyal et al., 2008; Oliveira et al., 2022). In general, soybean is a poor competitor during earlier stages of development, however, earlier planting and narrow row spacing can contribute to its competitiveness with weeds (Legere and Schreiber, 1989; Klingaman and Oliver, 1994). Narrow-row spacing in the soybean cropping system can increase the crop competitiveness against late-emerging weeds. Soybean canopy closure is faster in 19- to 38 cm soybean in comparison to wide-spaced (76 cm) soybean, resulting in lower radiation transmitted through the canopy and partially contributing to greater competitiveness over weeds in narrow- versus wide-spaced soybeans (Mulugeta and Boerboom, 2000; Puricelli et al., 2003; Steckel and Sprague, 2004; Arsenijevic et al., 2022). The advantage of narrow-spaced soybean was demonstrated by Butts et al. (2016), indicating a reduction in waterhemp growth and fecundity under such crop management practice. Furthermore, previous research has reported the impact of corn on waterhemp growth and fecundity, where waterhemp biomass, survival, and fecundity was significantly reduced with delayed emergence relative to corn, but weeds emerging at or before the V5 corn growth stage could still contribute significantly to the seed bank (Nordby and Hartzler, 2004).
The weed suppression potential of the cultivated crops themselves is often overlooked. The alarming rate of herbicide resistance evolution in the past decade and shortage of new chemistry (Heap, 2022) warrant investigation of other weed management practices, including cultural weed control through rapid crop canopy closure. Such results can be beneficial in developing and enhancing the IWM recommendations for controlling and/or suppressing waterhemp and other troublesome weed species by creating an environment unsuitable for their growth and reproduction. Therefore, the objective of this experiment was to determine the impact of narrow-spaced (38 cm row spacing) soybean, wide-spaced (76 cm) soybean, and corn (76 cm) on the growth and development of multiple waterhemp cohorts throughout the growing season. We hypothesized that narrow-spaced soybeans and corn would be more effective at suppressing waterhemp biomass and height than wide-spaced soybean.
Materials and methods
Experimental site description
A field experiment was conducted in 2019 and 2020 at the University of Wisconsin-Madison Arlington Agricultural Research Station (AARS) near Arlington, WI (43.3097° N, 89.3458° W). The experiment was conducted as a 4x5 factorial in 2019 and as a 4x6 factorial in 2020 with treatments consisting of 4 crop treatments (38 cm row spacing soybean [“narrow rows”], 76 cm row spacing soybean [“wide rows”], 76 cm row spacing corn, and no crop [“fallow”]) and 5 (2019) and 6 (2020) waterhemp transplant timings (simulated cohorts). The experiment was established in a split-plot design employed in a randomized complete block design (RCBD) with four replications (Figure 1). Crops were considered as the whole-plot level, whereas the waterhemp transplant timings (simulated cohorts) were considered as the split-plot level (defined as “segment” later). Each whole-plot was 6 m wide and 12.2 m long. The experiment area was chisel plowed before the crops were planted and sprayed with glyphosate (glyphosate 863 g ae ha-1, Roundup® PowerMAX, Bayer AG, Leverkusen, Germany; + ammonium-sulfate 1,430 g ha-1) 14 days later to eliminate emerged weeds before the first waterhemp transplant timing. Complete soil and crop establishment information within each year is available in Table 1. Weather information is presented in Table 2.
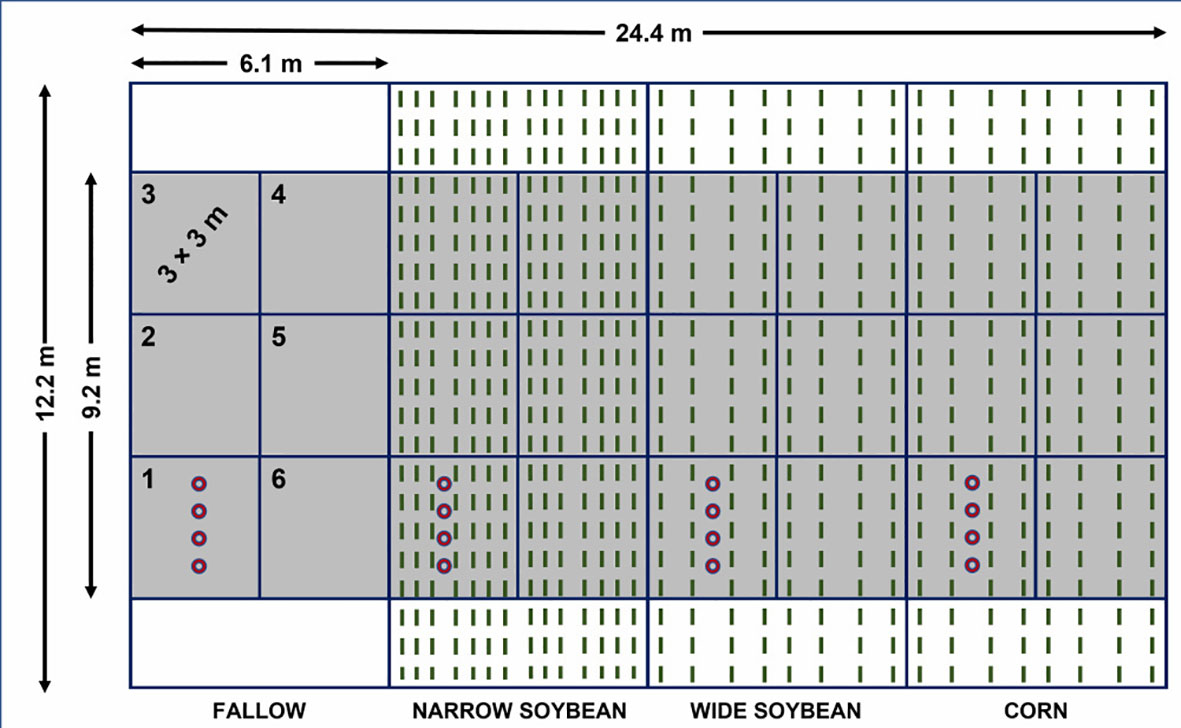
Figure 1 Example illustrating placement of transplanted waterhemp seedlings in fallow, narrow and wide soybean, and corn in the randomized experimental block. Numbers starting from 1 to 6 represent respective transplant timings. Transplanted waterhemp seedlings and their placement inside the crop rows are presented as red circles. Green dashed lines illustrate soybean rows (narrow [38 cm] and wide [76 cm]) and corn (76 cm).
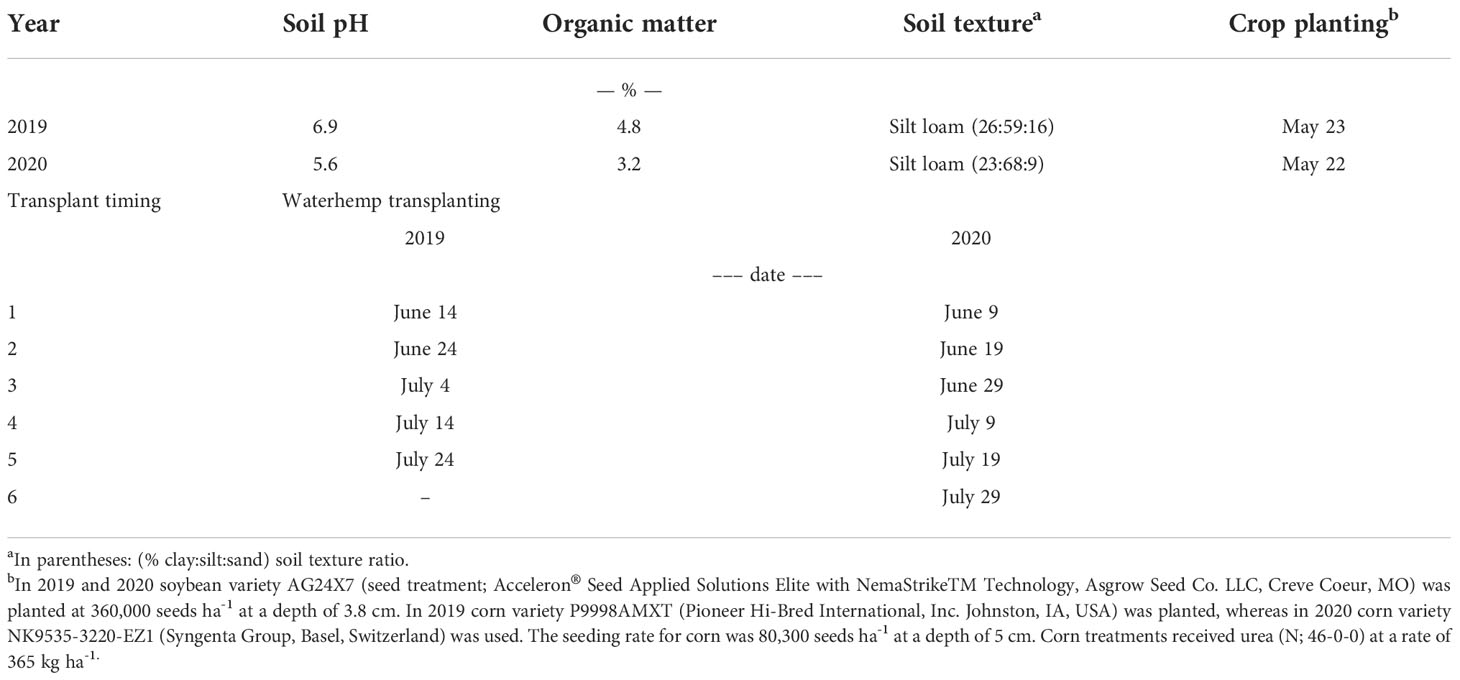
Table 1 Soil characteristics, crop management, and waterhemp transplant timings for the field experiments in 2019 and 2020 at Arlington, Wisconsin.
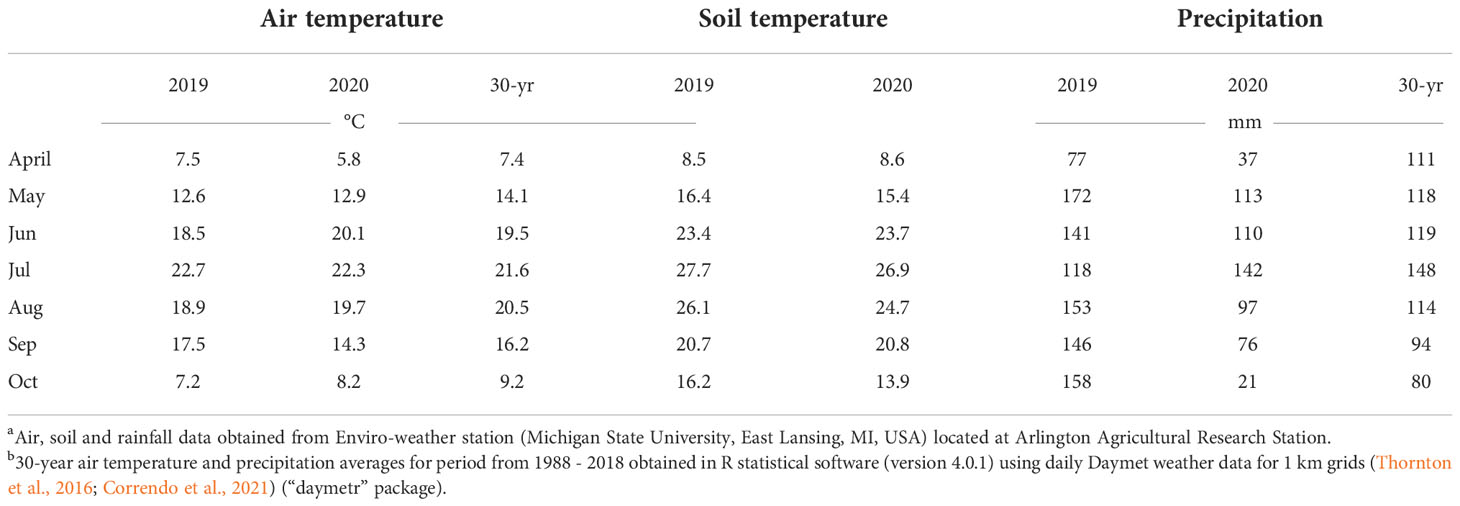
Table 2 Monthly average air and soil temperature, and precipitation for the experimental site at Arlington, Wisconsin, and greenhouse air temperatures during the 2019 and 2020 growing seasons.ab.
Waterhemp cohorts
Waterhemp seedlings were grown in the greenhouse before each transplant timing. Transplanted waterhemp seedlings were used in this experiment for two main reasons: i) waterhemp seeds were not present in the soil seedbank at AARS where this experiment was conducted, and ii) by transplanting we were able to successfully establish and monitor the growth and development of multiple waterhemp cohorts. The waterhemp accession used in this experiment was collected near Fond du Lac, WI (43.7146° N, 88.5170° W) in the fall of 2018. Seed samples were threshed, cleaned through an air column, and cold stratified to improve seed germination. For the cold stratification procedure, all seeds were placed in a glass container with a thin layer of water, allowing seeds to float, and stored in a refrigerator (dark conditions) at 5 C for 2 weeks. After stratification, waterhemp seeds were washed with distilled water using a soil sieve and dried on paper towels at room temperature for 24 h (adapted from Kohlhase et al., 2018). Waterhemp seeds were placed in plastic bags and kept in storage at 5 C until use. Waterhemp seeds were planted in 30 × 60 cm plastic trays, filled with potting mix (Promix® HP Mycorrhizae, Premier Tech Horticulture, Delson, QC, Canada). Waterhemp seedlings were supplied with adequate water and kept under greenhouse conditions at 28/20 C day/night temperature. Artificial lighting was provided using metal halide lamps (600 mmol m-2 s-1) to ensure a 15-hour photoperiod. Waterhemp seedlings at the 2-3 leaf growth stage were transplanted to cell insert containers (6 × 6 cm, 1 cell; 196 cm-3) which were then transplanted in the field the next day. Transplanting of waterhemp seedlings in the field started mid-June (typical period of active waterhemp emergence in Wisconsin in fields treated with a PRE-emergence herbicide; Striegel et al., 2021) when soybeans reached V2-V3 and corn was in a V3-V4 growth stage (Table 1). Transplant timings that followed were 10-days apart, with a total of 5 (2019) and 6 transplant timings (2020) (Table 1). Whole-plots for each crop (6 m wide x 12.2 m long) were divided into six segments (3 × 3 m), each segment designated for a different transplant timing. Four waterhemp seedlings spaced 40 cm apart in a row were transplanted in each segment. For wide rows soybean and corn (76 cm row spacing), seedlings were transplanted between the 2nd and 3rd rows of each segment, while for narrow rows soybean, seedlings were placed between 3rd and 4th rows (Figure 1). Seedlings transplanted in the fallow treatment were placed in the center of the respective segment. The study area was kept free of other weeds throughout the season by weekly hoeing. Newly transplanted waterhemp seedlings were watered for 3 days to ensure acclimation to field conditions. A total of 64 waterhemp seedlings were transplanted in the field per transplant timing (4 crops x 4 whole plots x 4 seedlings/segment/whole plot) similar to the methodology by Uscanga-Mortera et al. (2007).
Data collection
The field experiment was visited at least 3 times per week during the growing season to monitor the growth and development of waterhemp plants; individual waterhemp plants that reached the reproductive stage were immediately harvested. Waterhemp harvest occurred at flowering to avoid seed set at AARS; moreover, maximum height and biomass accumulation was expected at this developmental stage. Similar methodology was adopted by Oliveira et al., 2022. At harvest time of each waterhemp plant, the day of year, treatment (segment [transplant timing] and whole-plot [crop]), plant sex, and height (from ground level to the top of the inflorescence) were recorded. Harvested waterhemp plants were dried in the oven at 45 ° C until constant weight for biomass estimation.
Statistical analyses
All statistical analyses were conducted in R statistical software 4.0.1 (R Foundation for Statistical Computing, Vienna, Austria). Waterhemp biomass and height reduction were used to estimate the impact of crops on waterhemp growth and development as:
where biomass or height reduction of each waterhemp plant (R) growing within a specific crop was estimated by comparing its biomass or height (T) to the average biomass or height of waterhemp plants from the first transplant timing within that crop (F). A 3-parameter Weibull 2 model was fit to waterhemp biomass (Figure 2) and height (Figure 3) reduction data (%; response variable) pooled across years (2019-2020) regressed on the day from the first waterhemp transplant timing (explanatory variable) for each crop using the “drc” package in R:
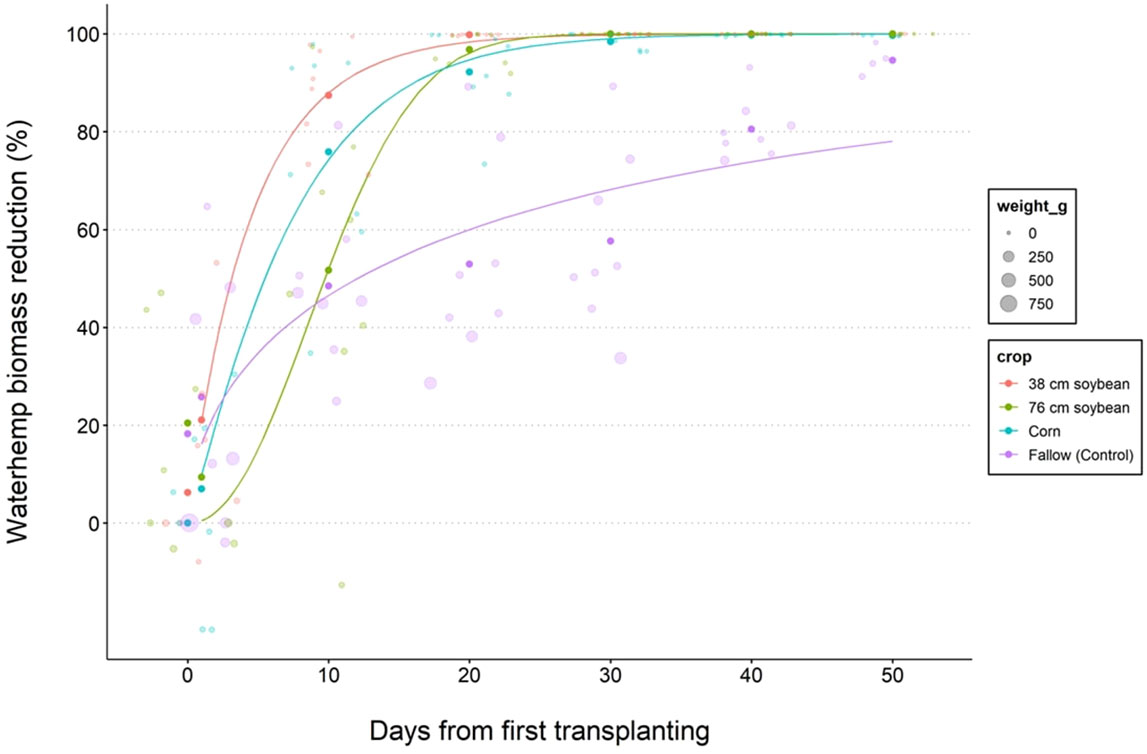
Figure 2 Nonlinear regression 3-parameter Weibull 2 models depicting reduction of waterhemp biomass for 38 (narrow) and 76 (wide) cm row spacing soybeans, corn (76 cm row spacing), and fallow treatment. Data lines represent the best model fit for cumulative waterhemp biomass regressed on the days from the first transplant timing. Waterhemp transplanting started on June 14 in 2019, and June 9 in 2020.
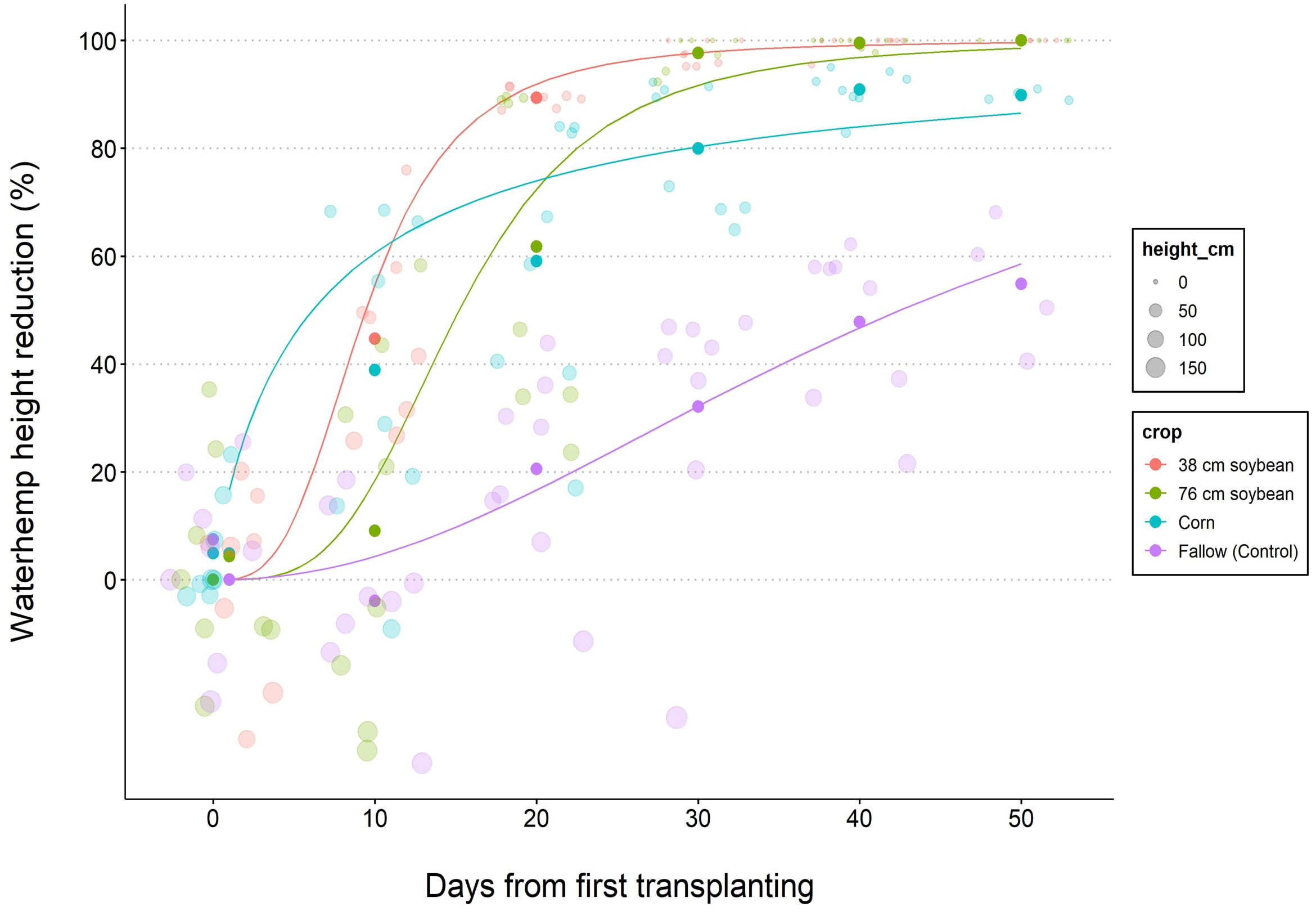
Figure 3 Nonlinear regression 3-parameter Weibull 2 models depicting reduction of waterhemp height for 38 (narrow) and 76 (wide) cm row spacing soybeans, corn (76 cm row spacing), and fallow treatment. Data lines represent the best model fit for cumulative waterhemp height regressed on the days from the first transplant timing. Waterhemp transplanting started on June 14 in 2019, and June 9 in 2020.
where y is the waterhemp height or biomass reduction (%), c is the lower limit (fixed at 0%), d is the upper limit (fixed at 100%), b is the slope, x is the number of days from the first waterhemp transplant timing, and e is the inflection point (Ritz and Strebig, 2016). D50 and D90 were estimated using the ED function in R and represent the number of days from the first transplant timing for the crop to reduce the biomass or height of a newly waterhemp cohort by 50% and 90%, respectively.
Results
Average waterhemp biomass (g plant-1) and height (cm plant-1) used to estimate biomass and height reduction over time are presented in Tables 3, 4. The average data are reported herein to support researchers conducting future modeling work. Overall, waterhemp biomass and height reduced with later establishment time. As anticipated, the crops (corn and soybean) had a major impact on waterhemp growth whereas the fallow treatment had the heaviest and tallest plants within each transplant timing. Although similar in height, waterhemp plants in the fallow treatment were >6X heavier than plants growing in the corn and soybean treatments in the earliest transplant timing (transplant timing 1). Regarding the last transplant timings (transplant timings 5 and 6), waterhemp plants in the fallow treatment were >500X heavier and >6X taller than plants growing in the corn. No waterhemp plant survived and produced biomass in the last transplant timing in both soybean treatments.
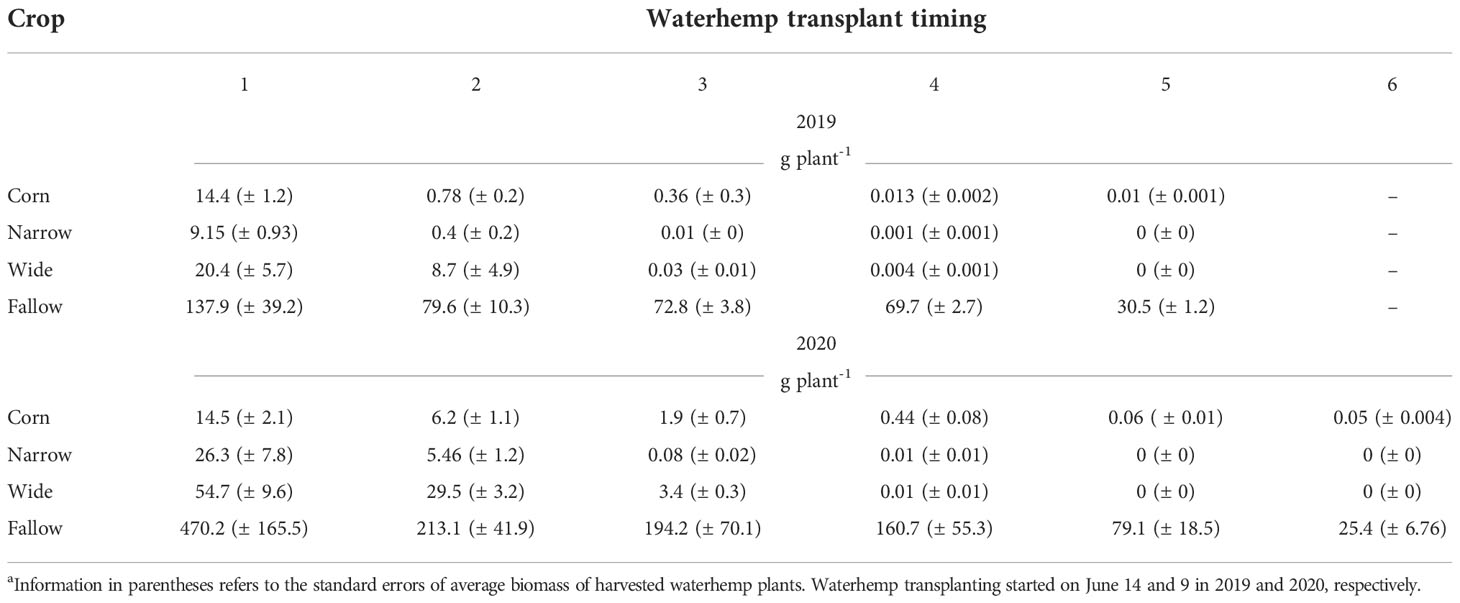
Table 3 The average biomass (g plant-1) of harvested waterhemp plants per each crop and transplant timing in 2019 and 2020, respectively.a.
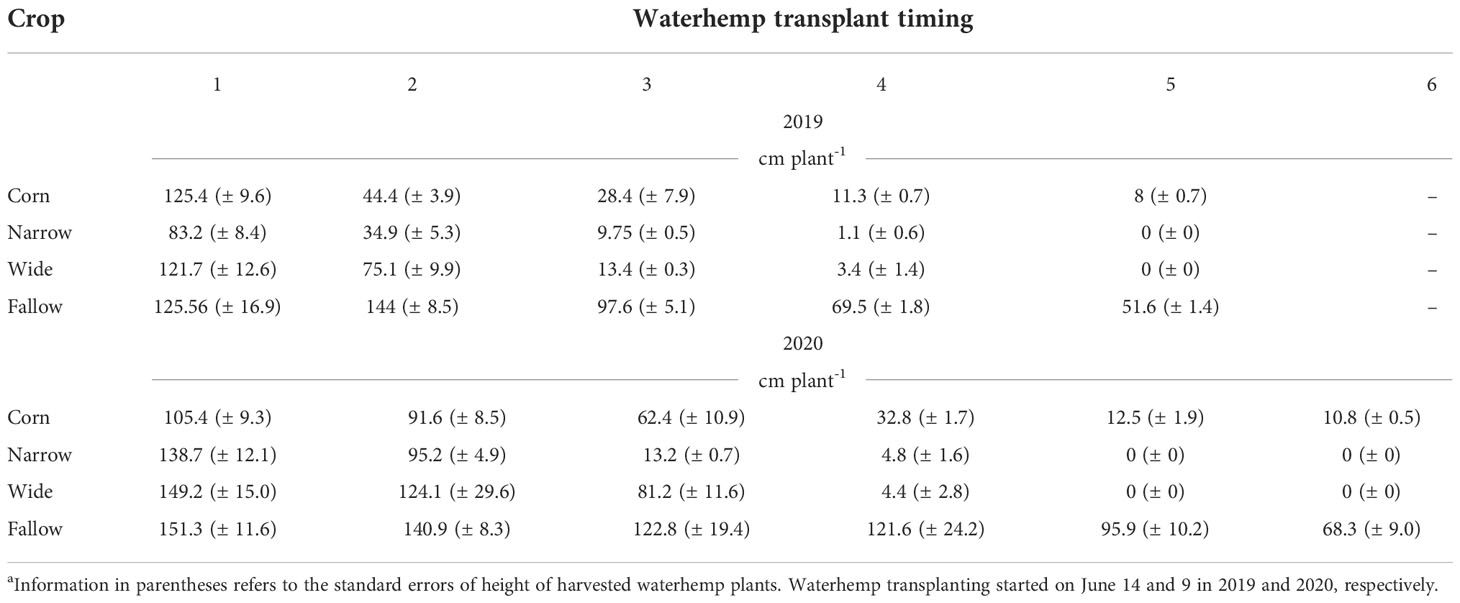
Table 4 The average height (cm plant-1) of harvested waterhemp plants per each crop and transplant timing in 2019 and 2020, respectively.a.
According to the biomass reduction model (Table 5), narrow-row soybeans required fewer days to suppress 50% biomass of new waterhemp cohorts followed by corn, wide row soybeans, and fallow, respectively (4, 6, 9, and 14 d, respectively), and 10, 14, 18, and 42 d for 50% height reduction of waterhemp (Table 6). Similarly, narrow-row soybeans required fewer days to suppress 90% biomass of waterhemp, followed by corn, wide-row soybeans, and fallow (11, 15, 18, and 78 d, respectively). Conversely, narrow and wide-row soybeans required fewer days to suppress 90% height of new waterhemp cohorts followed by corn and fallow (20, 26, 43, and 85 d, respectively).
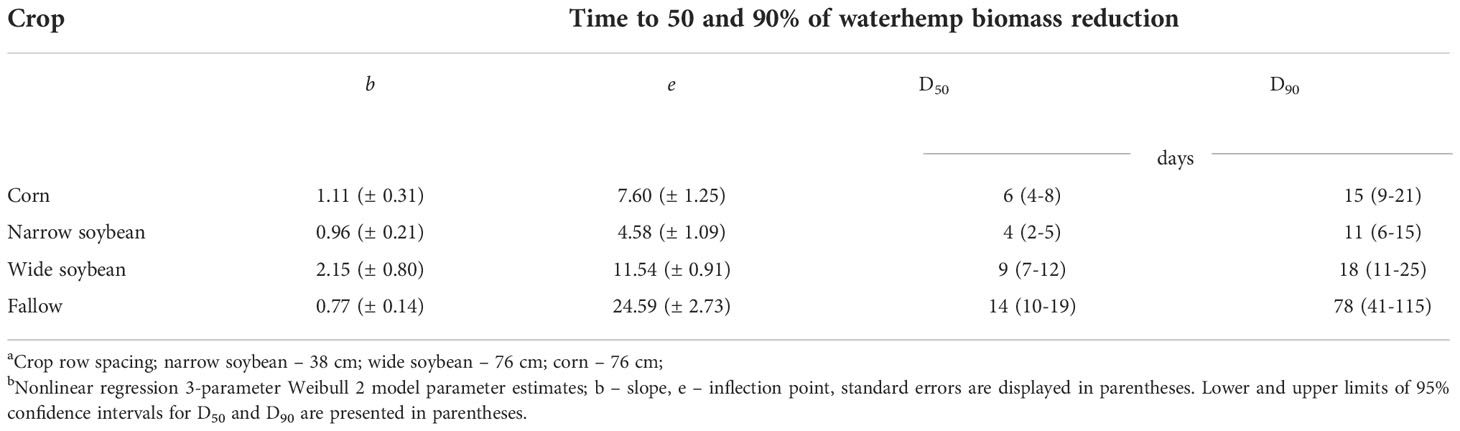
Table 5 Predicted days (D50; D90) after the first waterhemp transplant timing required to reach 50 and 90% biomass reduction of waterhemp for each crop, respectively.ab.
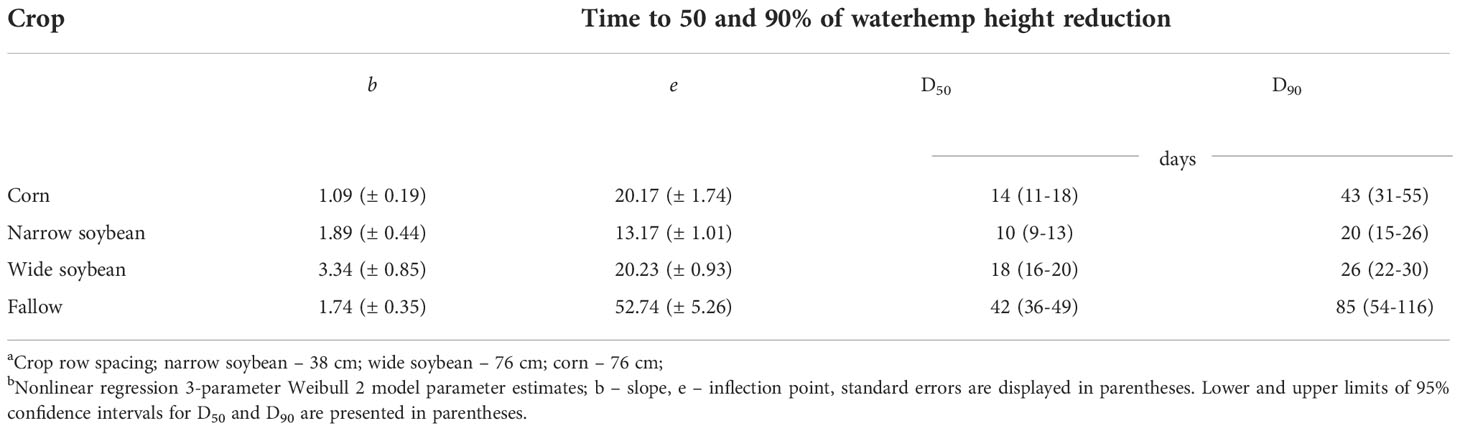
Table 6 Predicted days (D50; D90) after the first waterhemp transplant timing required to reach 50 and 90% height reduction of waterhemp for each crop, respectively.ab.
Discussion
Light is an essential component of photosynthesis and plays a major role in the growth and competitive ability of plants (Holt, 1995). Waterhemp is a light-sensitive C4 plant, and under an environment with high irradiation, it is extremely photosynthetically efficient (Pearcy and Ehleringer, 1984). Waterhemp cohorts that emerge later in the season will be naturally smaller and produce lower biomass due to shorter daylight (Khan et al., 2021). Similarly, Oliveira et al. (2022) reported that later emerging cohorts of another troublesome Amaranthus species, Palmer amaranth (Amaranthus palmeri), accumulated lower biomass and flowered faster. Combining this knowledge in tandem with the competitive ability of the cultivated crop can hinder the growth of waterhemp. The predicted days required for biomass and height reduction of waterhemp were the highest in the fallow treatment due to transplanted waterhemp seedlings being able to grow in a non-competitive environment, where daylength was the contributing factor for plant growth and the initiation of flowering. This is further supported by the higher mean dry waterhemp biomass and height for the fallow treatment, when compared to treatments where a crop was established (Tables 3, 4). Knezevic et al. (2003) indicated that reduction in the soybean row spacing (< 76 cm) improves early-season crop tolerance to weeds, delays the critical time for weed removal, and requires a less intensive weed management program than wide-spaced soybean. Furthermore, the early impact of corn on waterhemp growth and development has been reported by Nordby and Hartzler (2004); however, they indicated that later emerging waterhemp plants in the corn were able to produce seed and could contribute significantly to the weed seed bank.
Narrow-row soybeans required fewer days (e.g., were more effective at suppressing) to reduce biomass and height of new waterhemp cohorts by 50% followed by corn and wide-row soybeans, respectively (Tables 5 6). In contrast, fewer days to reduce growth of new waterhemp cohorts by 90% were required in both soybean row spacing treatments compared to corn (Table 6). There was a 17- and 23-day difference in the predicted time in days to 90% height reduction of waterhemp cohorts established in wide- and narrow-spaced soybeans, respectively, when compared to corn treatment (Table 6). This indicates that corn reaches its maximum weed suppressive effect later in the growing season compared to soybean. Corn was unable to completely suppress waterhemp growth in this study, like the soybean treatments. The difference in suppression of later established cohorts between soybean and corn can also be reflected by corn treatment having consistently taller and less branched waterhemp plants across all transplant timings likely due to increased allocation of dry matter in the main stem and less in branches (Arsenijevic, in-field observation; McLachlan et al., 1993). This difference in suppression potential between soybean and corn can be significant when it comes to late season waterhemp management and seed production of late emerging cohorts. Gaps in the canopy allow for light penetration, which results in a greater growth and seed production potential (Steckel et al., 2003). According to the results by Uscanga-Mortera et al. (2007), although waterhemp transplanted in the corn was always shaded by the crop canopy, it was able to produce high amounts of seeds even if it was transplanted after July 26, which they did not observe in the soybean (76 cm row spacing) crop. Planting corn earlier in the season and in narrower row space could amplify its suppressive effect on later emerging waterhemp cohorts, however that deserves further investigation. 76 cm row spacing is the standard for corn planting across the US Midwest (Porter et al., 2013).
It is important to mention that waterhemp seedling mortality in this experiment was only observed in the soybean treatments. Waterhemp mortality in both soybean treatments occurred for transplant timings 5 and 6 (after July 19; Tables 3, 4). Even though the late-season transplanted waterhemp seedlings adapted well after they were placed in the soil, they exhibited etiolation on the leaf tissue due to lack of light, and none of the seedlings were able to survive under such conditions (Arsenijevic, in-field observation). Our observation corroborates with results from Steckel and Sprague (2004), who reported mortality close to 100% after the R1 soybean growth stage. This indicates that once soybeans are reaching the point of their full canopy closure, they will effectively suppress the late-emerging waterhemp cohorts under its canopy, causing other means of weed control unnecessary. Moreover, under low-irradiance environments, waterhemp requires more time to accumulate biomass and will partition more biomass to leaves at the expense of reproductive structures (Steckel et al., 2003), reducing their seed production potential. Schwartz et al. (2017) reported a strong correlation between pigweed biomass and total seed production. This indicates that suppression of waterhemp exerted by soybean canopy in the late-season greatly contributes to the reduced seed production of waterhemp cohorts, protects the yield potential, and eases the harvestability of the crop.
From an ecological perspective, reducing the seed production potential of waterhemp is paramount, given this troublesome weed species has a high fecundity and adaptation potential (Keeley et al., 1987; Horak and Loughin, 2000; Sellers et al., 2003; Jha et al., 2009; Jha and Norsworthy, 2009; Werle et al., 2014). Moreover, understanding the biology of waterhemp and conditions that suit this weed species can support best management decisions. As observed in this experiment, the healthiest, tallest, and highest biomass plants were in fallow, non-competitive treatments. Such plants have the highest seed production potential. Introducing waterhemp to more stressful environment, such as a competitive crop canopy, could mitigate waterhemp growth (biomass and height) thus seed production.
The results from this experiment showcase the impact of soybean and corn canopy on the growth and development of multiple waterhemp cohorts. The predicted estimates in this experiment can also be used as rough indicators of the length of effective chemical and other non-cultural weed control options needed until the crop can effectively suppress waterhemp. Reducing soybean row spacing from 76 cm to 38 cm significantly enhanced the crops’ ability to suppress waterhemp. In addition, narrow-spaced soybean can reduce the need for multiple POST herbicide applications due to the earlier canopy closure and can provide more flexibility for a well-timed POST application due to overall lower biomass and height of waterhemp. It is highly recommended that growers scout corn in the mid-season to evaluate the need to manage later established waterhemp cohorts, which if present, will likely thrive and produce seeds. Thus, we partially rejected our initial hypothesis that narrow-spaced soybeans and corn would be more effective at suppressing waterhemp growth and development than wide-spaced soybean. A fully closed soybean canopy, regardless or row spacing, completely suppressed late emerging waterhemp cohorts in this study, whereas corn didn’t provide complete suppression of late-established waterhemp plants, and such plants were able to reach flowering stages. It is important to mitigate seed production potential of prolific weed species, such as waterhemp, in order to effectively deplete the soil seedbank. Utilizing the suppressive ability of the crop in synergism with other IWM practices can assist growers manage troublesome weeds and mitigate further herbicide-resistance evolution, while increasing sustainability and profitability of farmers.
Data availability statement
The datasets presented in this study can be found in online repositories. Repositories can be found below: https://github.com/Nikola704/Waterhemp-Adaptation.
Author contributions
RW and NA designed the experiment. NA and RD conducted the experiment. NA and RW analyzed the data and wrote the manuscript. All authors reviewed and revised the manuscript. All authors contributed to the article and approved the submitted version.
Funding
This research was partially funded by the Wisconsin Soybean Marketing Board and the United Soybean Board.
Acknowledgments
We thank the members of the Cropping Systems Weed Science lab at UW-Madison for their invaluable assistance in conducting this research.
Conflict of interest
The authors declare that the research was conducted in the absence of any commercial or financial relationships that could be construed as a potential conflict of interest.
Publisher’s note
All claims expressed in this article are solely those of the authors and do not necessarily represent those of their affiliated organizations, or those of the publisher, the editors and the reviewers. Any product that may be evaluated in this article, or claim that may be made by its manufacturer, is not guaranteed or endorsed by the publisher.
References
Arsenijevic N., DeWerff R., Conley S., Ruark M., Werle R. (2022). Influence of integrated agronomic and weed management practices on soybean canopy development and yield. Weed Technol. 36 (1), 73–78. doi: 10.1017/wet.2021.92
Blackshaw R. E., Harker K. N., O’Donovan J. T., Beckie H. J., Smith E. G. (2008). Ongoing development of integrated weed management systems on Canadian prairies. Weed Sci. 56, 146–150. doi: 10.1614/WS-07-038.1
Bradley K. W., Hagood E. S., Davis P. H. (2004). Trumpetcreeper (Campsis radicans) control in double-crop glyphosate-resistant soybean with glyphosate and conventional herbicide systems. Weed Technol. 18, 298–303. doi: 10.1614/WT-03-050R1
Butts T. R., Davis V. M. (2015) Glyphosate resistance confirmed in two Wisconsin common waterhemp (Amaranthus rudis) populations. In: WisCropManager, (2015). Available at: https://wcws.webhosting.cals.wisc.edu/wp-content/uploads/sites/96/2013/03/WCWS_207_waterhemp_resistance_WEB.pdf (Accessed March 13, 2021).
Butts T. R., Norsworthy J. K., Kruger G. R., Sandell L. D., Young B. G., Steckel L. E., et al. (2016). Management of pigweed (Amaranthus spp.) in glufosinate- resistant soybean in the Midwest and mid-south. Weed Technol. 30 (2), 355–365. doi: 10.1614/WT-D-15-00076.1
Correndo A. A., MoroRosso L. H., Ciampitti I. A. (2021). Agrometeorological data using r-software (Harvard Dataverse). doi: 10.7910/DVN/J9EUZU
Duke S. O. (2015). Perspectives on transgenic, herbicide-resistant crops in the United States almost 20 years after introduction. Pest Manage. Sci. 71 (5), 652–657. doi: 10.1002/ps.3863
Gianessi L. P. (2013). The increasing importance of herbicides in worldwide crop production. Pest Manag Sci. 69, 1099–1105. doi: 10.1002/ps.3598
Givens W. A., Shaw D. R., Johnson W. G., Weller S. C., Young B. G., Wilson R. G., et al. (2009). A grower survey of herbicide use patterns in glyphosate-resistant cropping systems. Weed Technol. 23 (1), 156–161. doi: 10.1614/WT-08-039.1
Hager A., Wax L., Stoller E., Bollero G. (2002). Common waterhemp (Amaranthus rudis) interference in soybean. Weed Sci. 50 (5), 607–610. doi: 10.1614/0043-1745(2002)050[0607:CWARII]2.0.CO;2
Heap I. (2022) The international herbicide-resistant weed database. Available at: https://www.weedscience.org (Accessed August 12, 2022).
Holt J. S. (1995). Plant responses to light: a potential tool for weed management. WeedSci 43, 474–482. doi: 10.1017/S0043174500081509
Horak M. J., Loughin T. M. (2000). Growth analysis of four amaranthus species. WeedSci 48, 347 355. doi: 10.1614/0043-1745(2000)048[0347:GAOFAS]2.0.CO;2
Jannink J. L., Orf J. H., Jordan N. R., Shaw R. G. (2000). Index selection for weed suppressive ability in soybean. CropSci 40, 1087–1094. doi: 10.2135/cropsci2000.4041087x
Jha P., Norsworthy J. K. (2009). Soybean canopy and tillage effects on emergence of palmer amaranth (Amaranthus palmeri) from a natural seed bank. Weed Sci. 57, 644–651. doi: 10.1614/WS-09-074.1
Jha P., Norsworthy J. K., Riley M. B., Bielenberg D. G., Bridges W. Jr. (2009). Acclimation of palmer amaranth (Amaranthus palmeri) to shading. Weed Sci. 56, 729–734. doi: 10.1614/WS-07-203.1
Johnson W. G., Bradley P. R., Hart S. E., Buesinger M. L., Massey R. E. (2000). Efficacy and economics of weed management in glyphosate-resistant corn (Zea mays). Weed Technol. 14, 57–65. doi: 10.1614/0890-037X(2000)014[0057:EAEOWM]2.0.CO;2
Keeley P. E., Carter C. H., Thullen R. J. (1987). Influence of planting date on growth of palmer amaranth (Amaranthus palmeri). Weed Sci. 35 (2), 199–204. doi: 10.1017/S0043174500079054
Khan A., Mobli A., Werth J., Chauhan B. (2021). Effect of emergence time on growth and fecundity of redroot pigweed (Amaranthus retroflexus) and slender amaranth (Amaranthus viridis): Emerging problem weeds in Australian summer crops. Weed Sci. 69 (3), 333–340. doi: 10.1017/wsc.2021.9
Klingaman T. W., Oliver L. R. (1994). Influence of cotton (Gossypium hirsutum) and soybean (Glycine max) planting date on weed interference. Weed Sci. 42, 61–65. doi: 10.1017/S0043174500084162
Knezevic S. Z., Evans S., Mainz M. (2003). Row spacing influences critical time of weed removal in soybean. Weed Technol. 17, 666–673. doi: 10.1614/WT02-49
Kohlhase D. R., Edwards J. W., Owen M. D. K. (2018). Inheritance of 4- hydroxyphenylpyruvate dioxygenase inhibitor herbicide resistance in an amaranthus tuberculatus population from Iowa, USA. Plant Sci. 274, 360–368. doi: 10.1016/j.plantsci.2018.06.004
Korres N., Norsworthy J., Bagavathiannan M., Mauromoustakos A. (2015). Distribution of arable weed populations along eastern Arkansas–Mississippi delta roadsides: Factors affecting weed occurrence. Weed Technol 29 (3), 596–604. doi: 10.1614/WT-D-14-00152.1
Legere A., Schreiber M. M. (1989). Competition and canopy architecture as affected by soybean (Glycine max) row width and density of redroot pigweed (Amaranthus retroflexus). Weed Sci. 37, 84–92. doi: 10.1017/S0043174500055909
Liebman M., Staver C. P. (2001). “Crop diversification for weed management”. in Liebman M., Mohler C.L., Staver C.P. eds. Ecological Management of Agricultural Weeds (Cambridge, UK: Cambridge University Press), 322–374.
Lingenfelter D., Wallace J. (2021). Problem weed control in midseason corn and soybean. Available at: https://extension.psu.edu/problem-weed-control-in-midseason-corn-and-soybean (Accessed April 27, 2021).
McLachlan S. M., Tollenaar M., Swanton C. J., Weise S. F. (1993). Effect of corn induced shading on dry matter accumulation, distribution, and architecture of redroot pigweed (Amaranthus retroflexus). Weed Sci. 41, 568–573. doi: 10.1017/S0043174500076335
Mulugeta D., Boerboom C. M. (2000). Critical time of weed removal in glyphosate- resistant glycine max. Weed Sci. 48, 35–42. doi: 10.1614/0043-1745(2000)048[0035:CTOWRI]2.0.CO;2
Nordby D. E., Hartzler R. G. (2004). Influence of corn on common waterhemp (Amaranthus rudis) growth and fecundity. Weed Sci. 52 (2), 255–259. doi: 10.1614/WS-03-060R
Norsworthy J. K., Oliveira M. J. (2007). Tillage and soybean canopy effects on common cocklebur (Xanthium strumarium) emergence. Weed Sci. 55, 474–480. doi: 10.1614/WS-07-003.1
Norsworthy J. K., Ward S. M., Shaw D. R., Llewellyn R. S., Nichols R. L., Webster T. M., et al. (2012). Reducing the risks of herbicide resistance: best management practices and recommendations. Weed Sci. 60, 31–62. doi: 10.1614/WS-D-11-00155.1
Oliveira M. C., Jhala A. J., Bernards M. L., Proctor C. A., Stepanovic S., Werle R. (2022). Palmer amaranth (Amaranthus palmeri) adaptation to US Midwest agroecosystems. Front. Agron. 4, 887629. doi: 10.3389/fagro.2022.887629
Pearcy R. W., Ehleringer J. (1984). Comparative ecophysiology of C3 and C4 plants. Plant Cell Environ. 7 (1), 1–3. doi: 10.1111/j.1365-3040.1984.tb01194.x
Porter P. M., Hicks D. R., Lueschen W. E., Ford J. H., Warnes D. D., Hoverstad T. R. (2013). Corn response to row width and plant population in the northern corn belt. J. Production Agric. 10 (2), 293–300. doi: 10.2134/jpa1997.0293
Powles S. B. (2008). Evolved glyphosate-resistant weeds around the world: lessons to be learnt. Pest Manag. Sci. 64 (4), 360–365. doi: 10.1002/ps.1525
Puricelli E. C., Faccini D. E., Orioli G. A., Sabbatini M. R. (2003). Spurred anoda (Anoda cristata) competition in narrow- and wide-row soybean (Glycine max). Weed Technol. 17, 446–451. doi: 10.1614/0890-037X(2003)017[0446:SAACCI]2.0.CO;2
Reddy K. N., Whiting K. (2000). Weed control and economic comparisons of glyphosate-resistant, sulfonylurea-tolerant, and conventional soybean (Glycine max) systems. Weed Technol. 14 (1), 204–211. doi: 10.1614/0890-037X(2000)014[0204:WCAECO]2.0.CO;2
Regnier E. E., Janke R. R. (1990). “Evolving strategies for managing weeds,” in Sustainable agricultural systems. Eds. Edwards R., Lal R., Madden R., Miller R. H., House G. (Ankeny, IA: Soil Water Conservation Society), 174–202.
Ribeiro V. H. V., Oliveira M. C., Smith D. H., Santos J. B., Werle R. (2021). Evaluating efficacy of preemergence soybean herbicides using field treated soil in greenhouse bioassays. Weed Technol. 35, 830–837. doi: 10.1017/wet.2021.22
Ritz C., Strebig J. (2016) Package “drc.” Available at: https://cran.r-project.org/web/packages/drc/drc.pdf (Accessed March 12, 2021).
Sanyal D., Bhowmik P. C., Anderson R. L., Shrestha A. (2008). Revisiting the perspective and progress of integrated weed management. Weed Sci. 56, 161–167. doi: 10.1614/WS-07-108.1
Schwartz L. M., Norsworthy J. K., Young B. G., Bradley K. W., Kruger G. R., Davis V. M., et al. (2017). Tall waterhemp (Amaranthus tuberculatus) and palmer amaranth (Amaranthus palmeri) seed production and retention at soybean maturity. Weed Technol. 30 (1), 284–290. doi: 10.1614/WT-D-15-00130.1
Sellers B. A., Smeda R. J., Johnson W. G., Kendig J. A., Ellersieck M. R. (2003). Comparative growth of six amaranthus species in Missouri. Weed Sci. 51 (3), 329–333. doi: 10.1614/0043-1745(2003)051[0329:CGOSAS]2.0.CO;2
Shaw W. C. (1982). Integrated weed management systems technology for pest management. Weed Sci. 30 (Suppl. 1), 2–12. doi: 10.1017/S0043174500060252
Stanger T. F., Lauer J. G. (2008). Corn grain yield response to crop rotation and nitrogen over 35 years. Agron. J. 100 (3), 643–650. doi: 10.2134/agronj2007.0280
Steckel L. E., Sprague C. L. (2004). Late-season common waterhemp (Amaranthus rudis) interference in narrow- and wide-row soybean. Weed Technol. 18, 947–952. doi: 10.1614/WT-03-131R
Steckel L. E., Sprague C. L., Hager A. G., Simmons W., Bollero G. A. (2003). Effects of shading on common waterhemp (Amaranthus rudis) growth and development. Weed Sci. 51 (6), 898–903. doi: 10.1614/P2002-139
Striegel S., Oliveira M. C., DeWerff R. P., Stoltenberg D. E., Conley S. P., Werle R. (2021). Influence of postemergence Dicamba/Glyphosate timing and inclusion of acetochlor as a layered residual on weed control and soybean yield. Front. Agron. 100. doi: 10.3389/fagro.2021.788251
Swanton C. J., Murphy S. D. (1996). Weed science beyond the weeds: the role of integrated weed management (IWM) in agroecosystem health. Weed Sci. 44, 437–445. doi: 10.1017/S0043174500094145
Swanton C. J., Weise S. F. (1991). Integrated weed management: the rationale and approach. Weed Technol. 5, 657–663. doi: 10.1017/S0890037X00027512
Thornton P. E., Thornton M. M., Mayer B. W., Wei Y., Devarakonda R., Vose R. S., et al. (2016). Daymet: Daily surface weather data on a 1-km grid for North America, version 3 (Oak Ridge, Tennessee, USA: ORNL DAAC). doi: 10.3334/ORNLDAAC/1328
Uscanga-Mortera E., Clay S. A., Forcella F. (2007). Common waterhemp growth and fecundity as influenced by emergence date and competing crop. Agron. J. 99, 1265–1270. doi: 10.2134/agronj2007.0029
USDA-NASS (2020). Available at: https://www.nass.usda.gov/Statistics_by_State/Wisconsin/Publications/Crops/2020/WI-Acreage-06-20.pdf (Accessed April 27, 2021).
Van Wychen L. (2019)2019 survey of the most common and troublesome weeds in broadleaf crops, fruits & vegetables in the united states and Canada. In: Weed science society of America national weed survey dataset. Available at: http://wssa.net/wp-content/uploads/2019-Weed-Survey_Broadleaf-crops.xlsx (Accessed April 24, 2021).
Walker R. H., Buchanan G. A. (1982). Crop manipulation in integrated weed management systems. Weed Sci. 30 (Suppl.), 17–24. doi: 10.1017/S0043174500060276
Werle R., Sandel L., Buhler D., Hartzler R., Lindquist J. (2014). Predicting emergence of 23 summer annual weed species. Weed Sci. 62 (2), 267–279. doi: 10.1614/WS-D-13-00116.1
Zimbric J., Stoltenberg D. E., Renz M., Werle R. (2018) Herbicide resistance in Wisconsin: An overview. Available at: http://www.wiscweeds.info/post/herbicide-resistance-in-wisconsin-an-overview/ (Accessed March 11, 2021).
Keywords: integrated weed management, row spacing, cultural weed control, weed suppression, weed biology
Citation: Arsenijevic N, DeWerff R, Conley S, Ruark MD and Werle R (2022) Growth and development of multiple waterhemp (Amaranthus tuberculatus) cohorts in corn and soybeans. Front. Agron. 4:1037483. doi: 10.3389/fagro.2022.1037483
Received: 05 September 2022; Accepted: 15 November 2022;
Published: 07 December 2022.
Edited by:
Caleb Squires, Corteva Agriscience, United StatesReviewed by:
Aritz Royo-Esnal, Universitat de Lleida, SpainAlessandra Carrubba, Università di Palermo, Italy
Panagiotis Kanatas, University of Patras, Greece
Copyright © 2022 Arsenijevic, DeWerff, Conley, Ruark and Werle. This is an open-access article distributed under the terms of the Creative Commons Attribution License (CC BY). The use, distribution or reproduction in other forums is permitted, provided the original author(s) and the copyright owner(s) are credited and that the original publication in this journal is cited, in accordance with accepted academic practice. No use, distribution or reproduction is permitted which does not comply with these terms.
*Correspondence: Rodrigo Werle, cndlcmxlQHdpc2MuZWR1