Soil Seed Bank Dynamics of Pastures Invaded by Navua Sedge (Cyperus aromaticus) in Tropical North Queensland
- 1Future Regions Research Centre, Federation University Australia, Mount Helen, VIC, Australia
- 2Queensland Department of Agriculture and Fisheries, Biosecurity Queensland, Dutton Park, QLD, Australia
Navua sedge (Cyperus aromaticus) is threatening crops, pasture species and natural ecosystems in Pacific Island countries and northern Queensland, Australia. To aid future management efforts of this exotic invasive weed, research was conducted to understand the soil seed bank dynamics of pastures invaded by Navua sedge. Six grazing properties were chosen across two landscapes, coastal and inland/upland, with areas that had Navua sedge infestations in Queensland, Australia. At each site, soil was collected from two soil depths, 0-5 and 5-10 cm, and from plots with high and low infestation levels of Navua sedge. It was observed that the soil seed bank dynamics varied significantly between these study sites. Navua sedge was the dominant species in the soil seed bank at all the sites contributing between 62% to 95% of the total seed bank, while pasture seeds occupied only 3% to 24%. Broadleaf seeds were even lower in abundance, showing between 2% to 13% of the total seed count. The abundance of Navua sedge seed present in the soil was significantly correlated with the aboveground biomass of Navua sedge (r=0.53, p=0.006), but no correlation was found with the infestation age of Navua sedge. Based on this work, it is clear that the seed bank of Navua sedge is very large and concentrated within the top 0-5 cm of the soil, with, 83% of total Navua sedge seeds being in this layer. It was also noted that, although, high infestation plots had significantly higher number of seeds than low infestation plots, more than 18,000 Navua sedge seeds/m2 were still found in the 0-5 cm soil layer of plots regarded as low infestation. We suggest that insights achieved from our soil seed bank study may be used to devise management strategies to minimise the impact of Navua sedge weed in critical grazing and agricultural areas.
Introduction
Weed management is an important aspect of crop productivity (Weisberger et al., 2019). Challenges faced in weed management are constantly evolving due to changes in weed traits, such as the challenge of herbicide resistance in some weeds, which necessitates constant adaptation of management practices (Llewellyn et al., 2016). Hence, understanding the biology and ecology of weeds is critical for developing any weed management strategy (Hosseini et al., 2014; Haring and Flessner, 2018). While the biological and ecological research of several established weed species have increased in recent years, there is still a lack of fundamental information on several important emerging weed species, including Navua sedge [Cyperus aromaticus (Ridley) Mattf. & Kukenth (syn. Kyllinga polyphylla; Kyllinga aromatica)].
Navua sedge is a C4 perennial sedge found in tropical climates. A native of equatorial Africa, this species has now been introduced into many tropical countries around the globe including Vanuatu, Samoa, Tahiti, Fiji, Sri Lanka, Malaysia, Solomon Islands, and tropical north Australia (Karan, 1975; Black, 1984). This species was introduced into the Cairns region of Queensland in Australia in the 1970s and it is reported that after a relatively short lag time of 23 years its population exploded (Osunkoya et al., 2021; Shi et al., 2021). Navua sedge is now a major biotic constraint to economic production in various agricultural systems in tropical north Queensland, Australia, including the dairy and beef industries and cropping systems such as banana (Musa acuminata Colla), sugarcane (Saccharum officinarum L.) and sweet potato (Ipomoea batatas L.) (Chadha et al., 2021). Crop and pasture production have been impacted by Navua sedge and it is also directly causing reductions in dairy and beef production in affected regions (Shi et al., 2021). Over 500 beef producers, dairy farmers and hay producers in the Atherton Tableland region in Queensland are affected by Navua sedge. It forms dense stands by strongly competing with other species for light, water, space and nutrients (Vitelli et al., 2010). Mechanical control options like crushing, slashing and rotary hoeing are time-consuming, impractical, and ineffective in large infestations (Vitelli et al., 2010). For chemical control, only one herbicide, halosulfuron-methyl, a group 2, acetolactate synthase inhibitor is registered for Navua sedge control in Australia. However, a single herbicide application is not enough to control this weed, and hence follow up treatments are required on the regrowth before seed set and to drive down the soil seed bank population (Vogler et al., 2015).
Soil seed banks are reserves of viable seeds found both in the soil profile and on the surface of the surrounding area (Liu et al., 2019). The seed bank is composed of both new and old seeds that have been shed and disseminated across landscapes and regions, and these serve as pools of genetic material for future generations (Gandía et al., 2022). The traits preserved in this way contribute to future weed populations by allowing seeds to bypass filters such as unfavourable environmental conditions and weed management measures, and hence the soil seed banks provide several opportunities for weeds to establish themselves in the plant populations (Booth and Swanton, 2002; Yang et al., 2021). Examination of seed banks can reveal important insights about what species may have been in previous standing vegetation in addition to their function as a reservoir for future populations (Ambrosio et al., 2004; Hopfensperger, 2007). Seed bank analysis can reveal historical seed inputs that may not be visible in aboveground vegetation due to environmental or competitive variables (Espeland et al., 2010). Soil seed banks are classically characterised by their lifetime, which is assessed by how long an individual seed may survive in a viable state within them. Transient seedbanks are those where the seeds only survive for a short time, and do not live past the second germination season post maturity, whereas persistent seedbanks have seeds that can survive beyond the second germination season and more (Thompson, 2000). Weed species that form persistent seedbanks, like Navua sedge are a concern for future weed management (Vitelli et al., 2010). The largest source of soil seed banks is from established plants within the vicinity. However, it is important to recognise that seeds, including those of many weeds, may be dispersed into an area by prevailing winds, moving waters, feral or domestic animals, agricultural equipment, or humans (Hussain et al., 2017; Mao et al., 2019). These types of seed dispersal can influence the seed bank diversity, and their horizontal and vertical distributions, and thus their resemblance to the current and previous aboveground vegetation patterns (Scott et al., 2010; Chauhan, 2012). Adding to this complexity is that the composition and density of the soil seed bank can vary both within and between sites (e.g., agricultural fields) depending on the land use and farming practices (Koocheki et al., 2009; Chejara et al., 2012).
Many weed species in a community are regulated by their seed bank dynamics. A better knowledge of their seed bank structure (e.g., abundance, distribution and longevity) could aid in the development of more effective weed management techniques (Maclaren et al., 2020). A crucial step is to determine whether removing aboveground materials and thus depleting the seed bank is a feasible approach, in addition to establishing the timeline for such management measures (Gioria and Osborne, 2010). A promising tactic of Navua sedge management will be to deplete the soil seed bank, and for such a tactic, a sound understanding of the species’ seed bank dynamics is required. Given the importance of this topic and the understanding that Navua sedge is currently recognised as an increasingly problematic species, particularly in the agricultural areas of tropical north Queensland (Osunkoya et al., 2019; Shi et al., 2021), the objectives of this study were to:
1. Determine the soil seed bank composition of pasture lands invaded by Navua sedge in varying sites and across different landscapes in the coastal and upland regions of Far North Queensland, Australia;
2. Establish the influence of time since infestation of Navua sedge and the density of aboveground vegetation on the soil seed bank dynamics of Navua sedge; and
3. Understand the spatial variation (in terms of both horizontal and vertical distribution) of the seed bank of Navua sedge.
Materials and Methods
Target Plant Description
Navua sedge is a clump-forming, creeping rhizomatous sedge growing up to 1 m in height (occasionally up to 2 m), with densely packed tillers. Lanceolate leaves are generally clustered at the base of the plant with each leaf about 15 cm in length and 3-5 mm in width (Shi et al., 2021). Five to eight leaf-like bracts subtend the flowers at the apex of the tiller (Shi et al., 2021). An irregular hemispheric to globose head with a central spike and occasionally several smaller lateral spikes make up the inflorescence (Mune, 1959; Shi et al., 2021). It is an aggressive weed species capable of spreading both via seeds and rhizomes (Vogler et al., 2015). It is a prolific seed producer, with each seed head producing approximately 250 seeds and the plants are capable of producing in excess of 450 million seeds/ha (Karan, 1975). The seeds are small and lightweight being easily dispersed to short distances by wind (Shi et al., 2021). Seeds are also dispersed by floodwaters, the hooves and digestive tract of cattle, humans, and harvesting machinery (Chadha et al., 2021). Vegetatively, it grows through the extension and fragmentation of the rhizome system (Karan, 1975). Secondary buds are responsible for producing new tillers, whereas the third bud is important for branching the rhizome system (Karan, 1975). A dense canopy is formed as new tillers keep on emerging from the rhizomes.
Sites Description
The study was conducted in the pastures and grazing lands of tropical north Queensland, Australia- a bioregion classified as the wet tropics. Sample collections of soil and aboveground vegetation were conducted in April 2021. Two landscapes (coastal vs inland/upland) were chosen for this study, with site selections being dependent on areas known to be invaded by Navua sedge. The first was a coastal region of high temperature and rainfall intensity, and the second area was an inland/upland region of the Atherton tablelands, which experience comparatively lower temperature and lower rainfall intensity. In addition, three cattle grazing sites were selected in each of the selected regions. In the coastal region, the sites were in Aloomba, Babinda and Cowley, while those of the inland/upland region were in Tarzali, Topaz and Atherton. Comprehensive information on the location, land use, climate, grazing intensity, probable time since Navua sedge infestation, probable method of arrival of Navua sedge at the study sites, aboveground major vegetation present and the soil physical traits, are provided in Table 1.
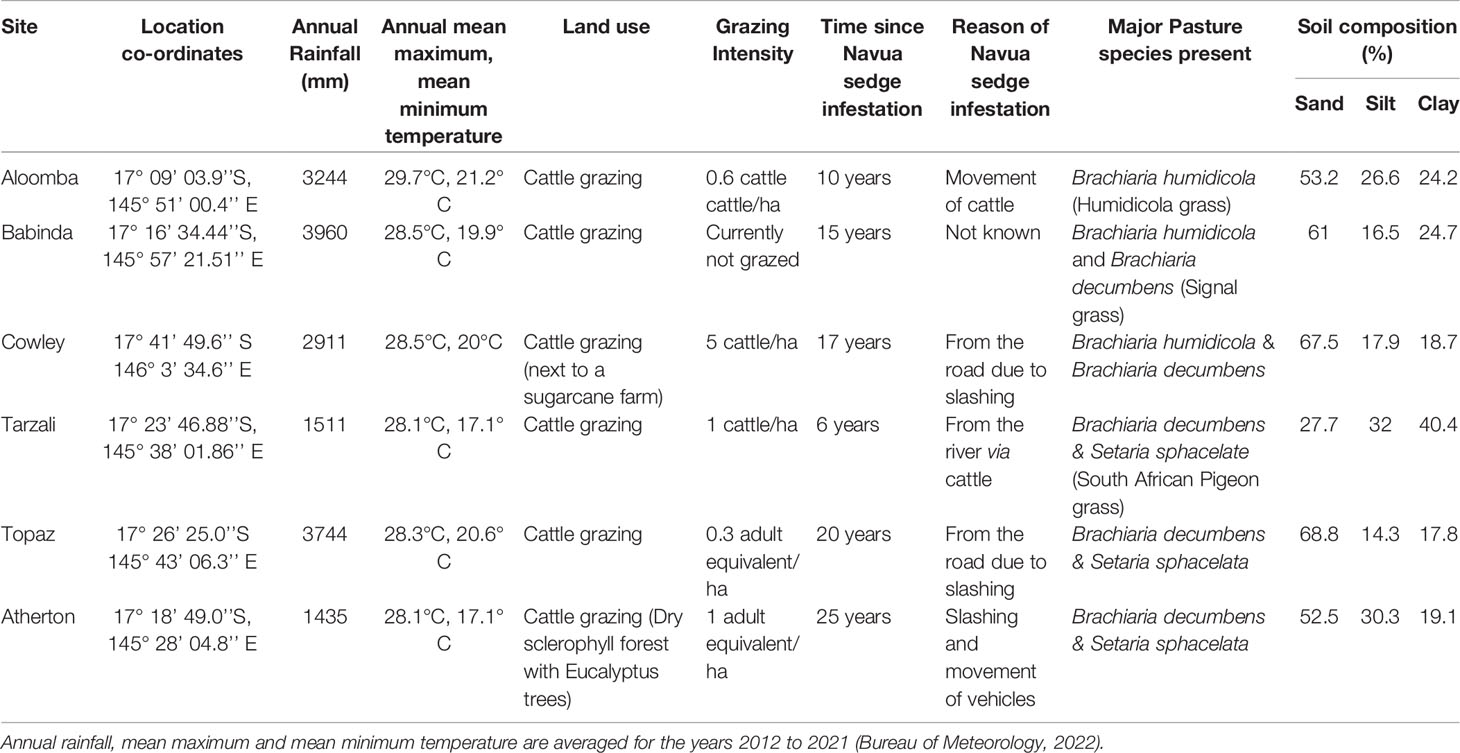
Table 1 Description of the study sites at Aloomba, Babinda, Cowley, Tarzali, Topaz and Atherton in far north Queensland.
Samples Collection
At each study site, topsoil within a randomly chosen area of 100 m x 100 m with visible Navua sedge infestations, were sampled. Each site had five soil collection areas (replicates), except for Aloomba and Tarzali sites which had six and four replicates, respectively. Each sample collection area contained a high infestation plot with an accompanying low to no infestation plot (referred to as low infestation hereafter), ensuring that the distance between the high and low infestation plots was no more than 5 m. High and low infestation plots were chosen on the basis of visual cover of Navua sedge in that area. In the high infestation plots, Navua sedge visual cover ranged from 50-100% in Aloomba, 50-85% in Babinda and Cowley, 60-80% in Malanda, 60-75% in Topaz, and 35-70% in Atherton. In the low infestation plots, Navua sedge visual cover ranged from 2-20% in Aloomba, 0-10% in Babinda, 1-5% in Cowley, 0-3% in Malanda and Topaz, and 0-5% in Atherton. Within each of the high and low infestation plots, 1 m x 1 m quadrats were placed on the ground for soil sampling; also, within each quadrat, another smaller quadrat area of 0.5 m x 0.5 m dimension was selected and marked for vegetation sampling and measurements. The plant species present and their % cover were recorded in the smaller quadrats. The entire mass of aboveground vegetation in each of the smaller plot was then clipped and placed in labelled paper bags for biomass measurement later in the laboratory. Thereafter, the soil samples were collected using a handheld soil corer (7 cm in diameter 5 cm deep). For each 1 m x 1 m quadrat plot, one sample was taken from each corner, and one was taken from the centre of the quadrat; the resulting five soil cores per plot were then pooled into a single bag to make a composite sample. To quantify the vertical distribution of seeds, soil samples were collected from two depths, 0-5 cm and 5-10 cm using the same sampling pattern described above.
For measurement of the soil particle composition, another five soil cores measuring 7 cm diameter and 5 cm depth were collected from each of the 1 m x 1 m quadrat (again one from each corner and one from the centre of the plot, but not where soils were sampled to study the soil seed bank) and pooled to make a composite sample. The soils were transported to the laboratory where they were sieved through a < 0.2 cm fine mesh screen to remove any fine roots and rhizomes prior to composition analysis. Hydrometer test was employed to determine the amount of sand, silt and clay in the soil. All analyses were conducted in a NATA (National Association of Testing Authorities) accredited soil laboratory, Australia. To ensure that the results were accurate and repeatable, a maximum of 10% variation within sample results was required before adding them to the data bank.
Biomass Measurement
The plants collected from the smaller inner plots (0.5 m x 0.5 m) were brought into the lab and separated into Navua sedge, pasture and broadleaf species. Navua sedge tillers were counted and recorded. The fresh weights of Navua sedge, pasture and broadleaf species were first recorded, then the samples were dried in an oven at 90°C for 72 hours to constant weight for estimation of dry biomass.
Seed Bank Measurement
The seed bank emergence study was conducted in the glasshouse at Federation University, Victoria Australia at the Mount Helen campus (37°37’41.4”S, 143°53’26.4”E) from April 2021 to December 2021. The glasshouse was maintained at day temperatures of 27°C to 32°C, and night temperatures of 18°C to 23°C, with the relative humidity > 80%.
An estimation of the magnitude of the seed bank was obtained using the ‘emergence’ method in the glasshouse. Each composite sample was thoroughly mixed and then spread to a depth of 1.5 to 2 cm over a 2 cm deep layer of sterilised river sand on a plastic seedling tray measuring 33 cm in length and 27 cm in width lined with paper towels. The trays containing soil were watered once daily for ten minutes using the automatic watering system to reduce any water stress. The trays were monitored weekly for seedling emergence. The seedlings that emerged at the end of each month were counted, identified either as Navua sedge, pasture or broadleaf species, and then removed thereafter. The plants that could not be identified at the seedling stage were removed and potted into separate pots to grow until they could be taxonomically identified. Two control trays were placed among the experimental trays, only filled with sterilised river sand to monitor any seedlings that might come from either the sterilised soil or from the glasshouse environment. After seven months of treatment, the soil was left to dry for a further two-week period without watering. Thereafter, the soil was then disturbed to make sure that any potential emergence of plant seedlings was not inhibited due to burial depth of the seeds. The trays were then re-watered daily, and seedling emergence counted at the end of the month. Finally, the total (cumulative) number of seedlings which emerged was converted to the number of seeds/m2 (i.e., density per unit area).
Statistical Analyses
The effect of landscape, site, infestation level and soil depth on the abundance of seeds of Navua sedge, pasture, and broadleaf species present in the soil were analysed by Multi-way ANOVA. Linear models were conducted to investigate the main effects of landscape type (coastal vs inland/upland), sites, infestation level (high infestation vs low infestation) and the depth of soil (0-5 cm vs 5-10 cm), in addition to their two- and three-way interactions. Where there were significant main and interaction effects, differences between levels within a given factor were further separated using Tukey’s post-hoc and/or Bonferroni adjustments procedures. All assumptions were checked by investigating the normality and spread of the residuals, and no violation of parametric analyses was observed in the abundance data collected. All statistical analyses were performed using SPSS Statistics, version 27 (IBM, New York, United States).
Results
Overall, across all the sites and over the eight months of seedling emergence from the soil, Navua sedge seeds dominated the soil seed bank, comprising 95%, 88%, 62%, 76%, 88% and 70% of the total soil seed bank at Aloomba, Babinda, Cowley, Tarzali, Topaz and Atherton, respectively. Significant effects of site, infestation level, soil depth and interaction effect of site * infestation level and site * soil depth were observed for abundance of Navua sedge seeds (Table 2). Averaged across both high and low infestation plots and 0-5 cm and 5-10 cm soil depths, the highest numbers of Navua sedge seeds were found at the Topaz (15,711 ± 3460 seeds/m2) and Babinda (14,907 ± 2227 seeds/m2) sites, and the lowest detected were at the Atherton (7,582 ± 1610 seeds/m2) and Tarzali (8,114 ± 2103 seeds/m2) sites (Figure 1). No seedlings emerged from the control trays.
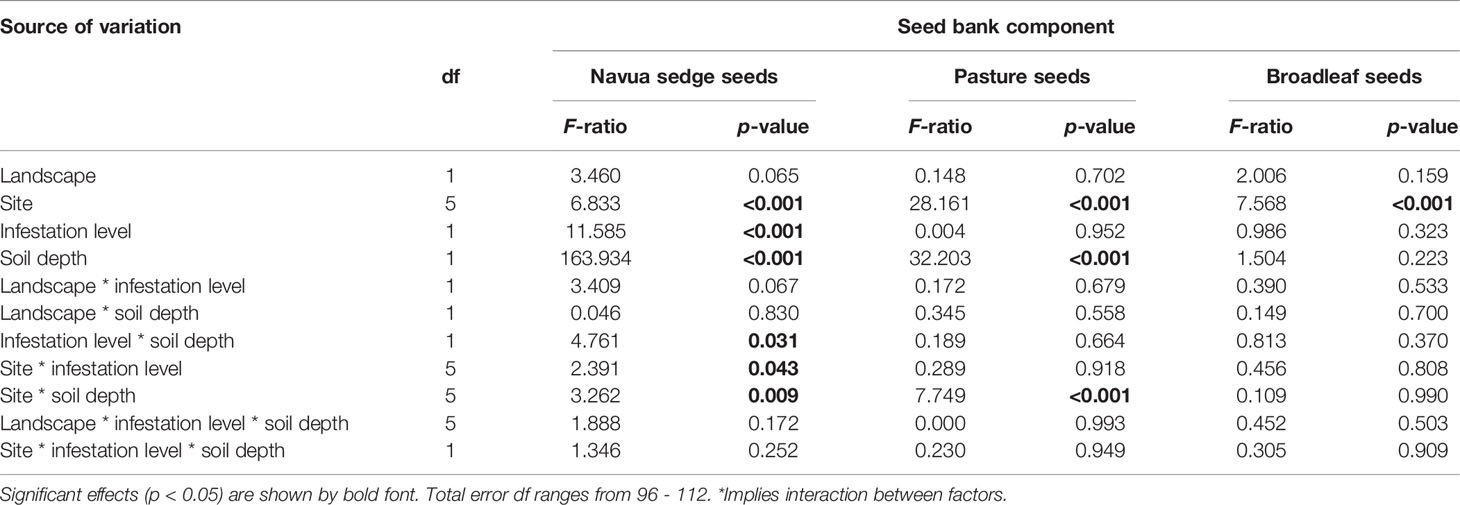
Table 2 Summary of ANOVA for all main effects and their interaction investigated from the mixed models for Navua sedge, pasture and broadleaf seeds present in the soil.
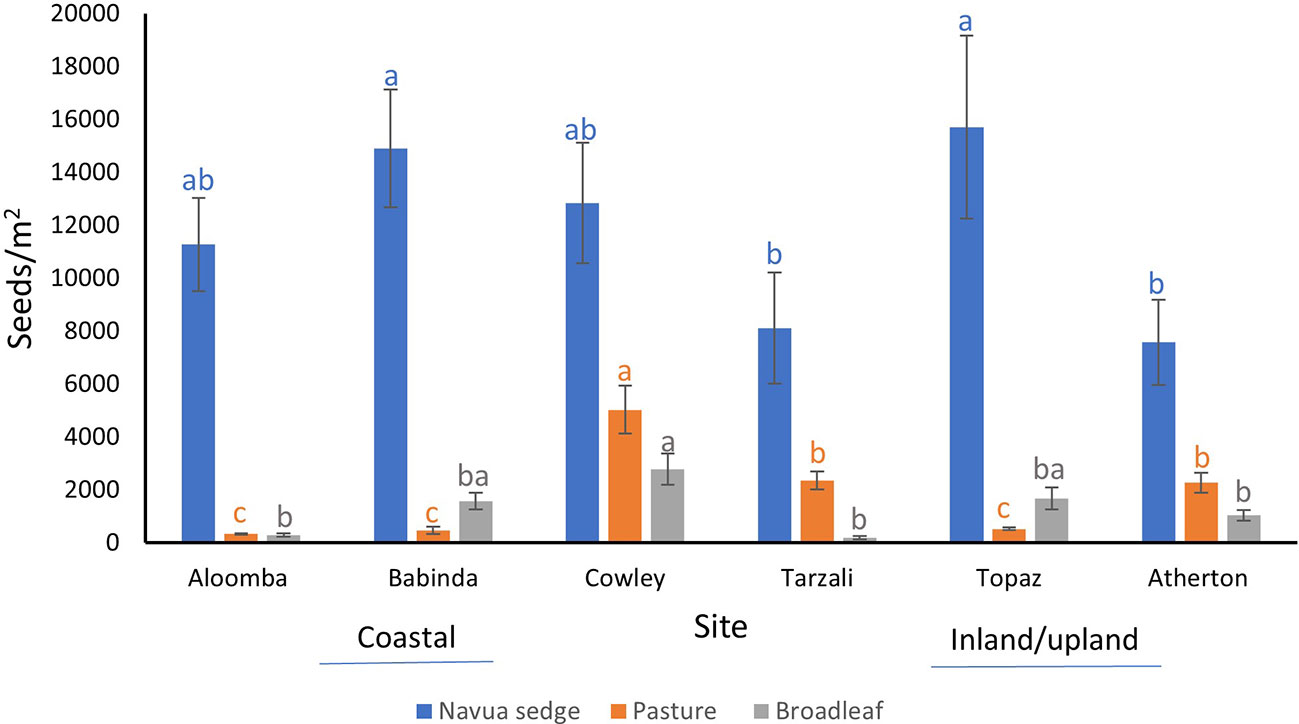
Figure 1 Abundance of Navua sedge (blue), pasture (orange) and broadleaf (grey) seeds/m2 in all the sites (mean ± standard error). Aloomba, Babinda and Cowley are coastal sites, while Tarzali, Topaz and Atherton are inland/upland sites. N= 4-6 plots per site; Abundance of seeds present is averaged across both the high and low infestation plots and the 0-5 cm and 5-10 cm soil profile. Same letters for each category of seeds indicate means are not statistically different at p < 0.05 when tested with Tukey’s HSD test.
Site, soil depth and their interaction had a significant effect on the abundance of pasture seeds present in the soil samples (Table 2). High variation was found between sites in the abundance of pasture seeds present (Figure 1). Out of all sites, Cowley (a coastal site) had a significantly higher number of pasture seeds (5,031 ± 910 seeds/m2) compared to all other sites (Figure 1). Of the three main factors, only ‘site’ had a significant effect on the abundance of broadleaf seeds present in the soil (Table 2). Although Cowley had the highest number of broadleaf seeds at 2,780 ± 593 seeds/m2, its abundance was similar to that of Topaz (an inland/upland site) and Babinda which had 1,674 ± 420 and 1,580 ± 312 seeds/m2, respectively (Figure 1). At any given time, a higher proportion of Navua sedge emerged from the soil compared to both pasture and broadleaf species in both 0-5 cm and 5-10 cm soil depth (Figure 2). Navua sedge and broadleaf species had the highest emergence in the first month, whereas pasture species had the highest emergence in the fifth month in both the soil depths (Figure 2).
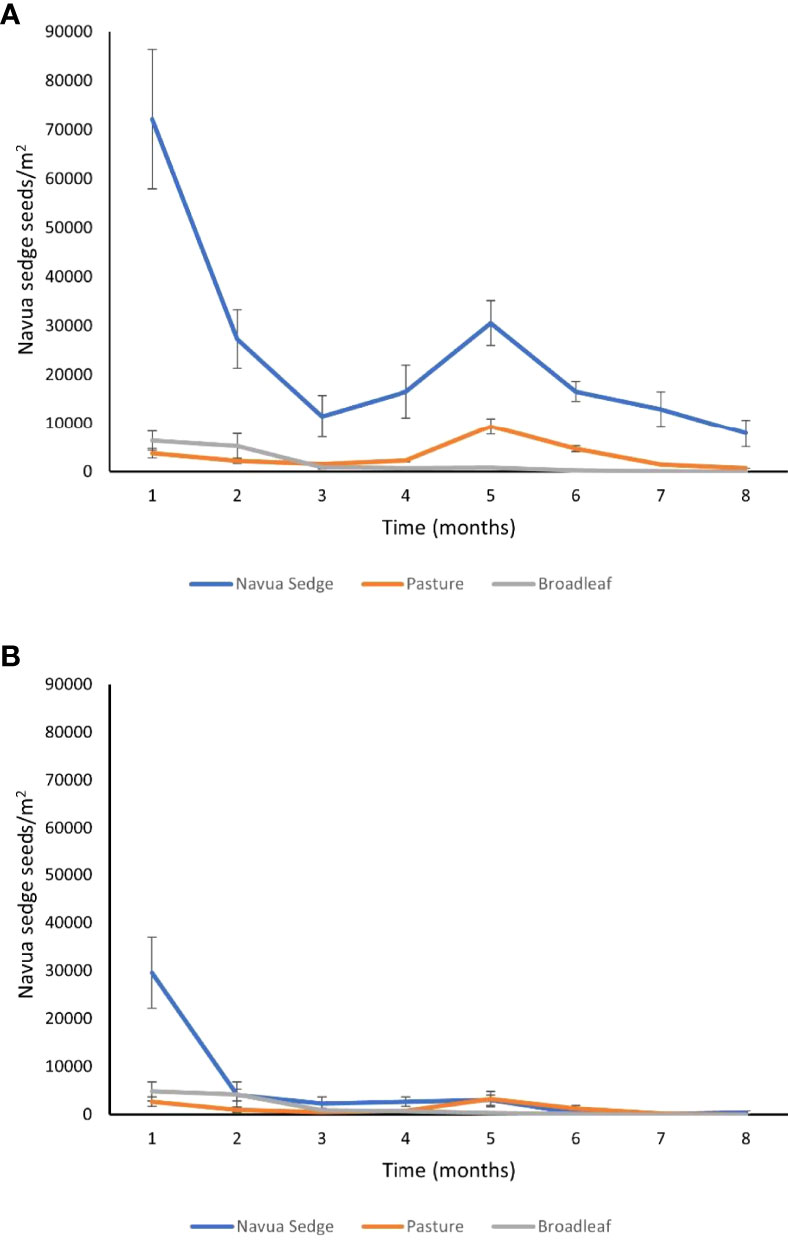
Figure 2 Monthly emergence of Navua sedge (blue), pasture (orange) and broadleaf (grey) seeds/m2 averaged across all the sites (mean ± standard error) in (A) 0-5 cm of the soil and (B) 5-10 cm of the soil.
Overall, only a marginal effect of landscape was observed on the abundance of Navua sedge seeds present in the soil (p = 0.065). However, infestation level and soil depth had highly significant effects on the abundance of Navua sedge seeds present in the soil (p < 0.001) (Table 2). No significant interaction was observed between landscape and infestation levels, nor between landscape and soil depth. However, a significant interaction (p = 0.03) was observed between the infestation level and soil depth, suggesting that soil depth effect on Navua sedge seed-soil abundance varied between the two infestation levels (Table 2). No significant interaction was found for the three-way interaction between landscape, infestation level and the soil depth (p = 0.172) (Table 2), implying consistency in the dynamics of seed abundance in the soil irrespective of landscape and soil depth. This suggests that, notwithstanding the landscape, infestation level or the type of site, Navua sedge seeds are consistently more abundant in topsoil (0-5 cm) compared to the lower depth (5-10 cm). As shown in Figure 3, the highest number of Navua sedge seeds were found in the top 0-5 cm of the soil in the high infestation plots with 22,883 ± 1,828 seeds/m2 averaged across all the sites. There were more Navua sedge seeds in the 0-5 cm (topsoil) of the high infestation plots compared to the same depth of the low infestation plots (Figure 3). In contrast, there was no significant difference in the amount of Navua sedge seeds found in the 5-10 cm soil layer between the high and low infestation levels (Figure 3). When compared between the two soil layers, significant differences in the amount of Navua sedge seeds present in the soil were detected between the 0-5 cm and 5-10 cm soil depths in both the high and low infestation plots (Figure 3).
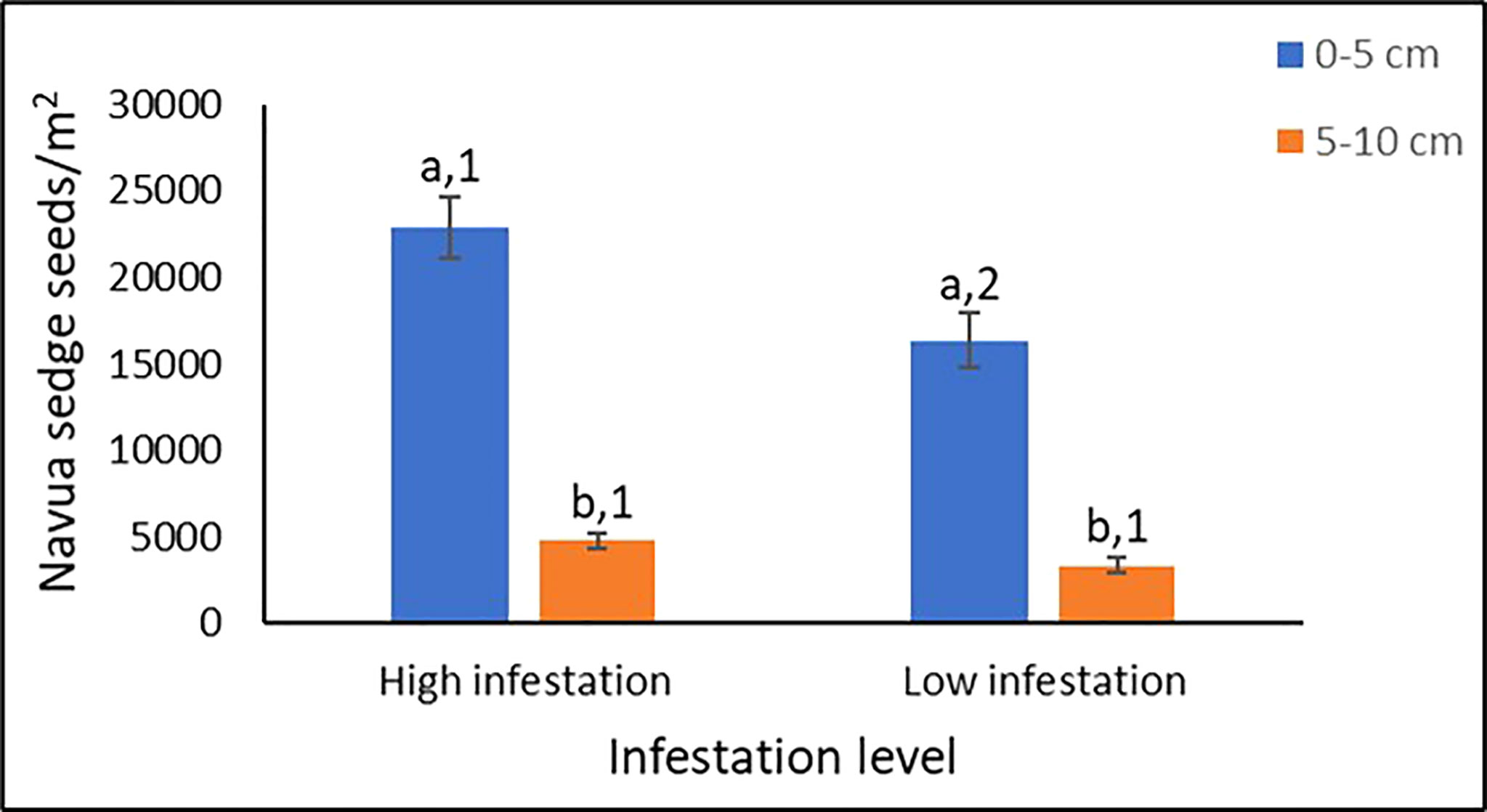
Figure 3 Navua sedge seeds/m2 present in the 0-5 cm and 5-10 cm soil profile in high and low infestation plots. N= 4-6 plots per site; Data has been combined across all six sites. The same letters within each infestation level indicates no significant difference at p < 0.05. The same numbers within each soil layer indicates no significant difference at p < 0.05.
A significant interaction was observed between site and infestation level (p = 0.043), and between site and soil depth (p = 0.009) (Table 2). Figure 4 provides a breakdown of the abundance of Navua sedge seeds present in the soil in both the 0-5 and 5-10 cm soil layers, and in both the high and low infestation plots, for each of the six study sites. The highest number of Navua sedge seeds was present in the 0-5 cm soil layer in the high infestation plots of the Topaz site, with 36,483 ± 4,537 seeds/m2. Compared with all other sites, Tarzali had the lowest number of Navua sedge seeds in the low infestation plots in both soil depths with 8,263 ± 1,916 seeds/m2 in the 0-5 cm soil layer and 1,221 ± 195 seeds/m2 in the 5-10 cm soil layer (Figure 4). When comparing sites, Tarzali and Topaz had significantly higher abundance of Navua sedge seeds present in the high infestation plots compared to the low infestation plots. In contrast, there was no significant difference in the abundance of Navua sedge seeds present in the soil in the high and low infestation plots in all the other sites (Figure 4).
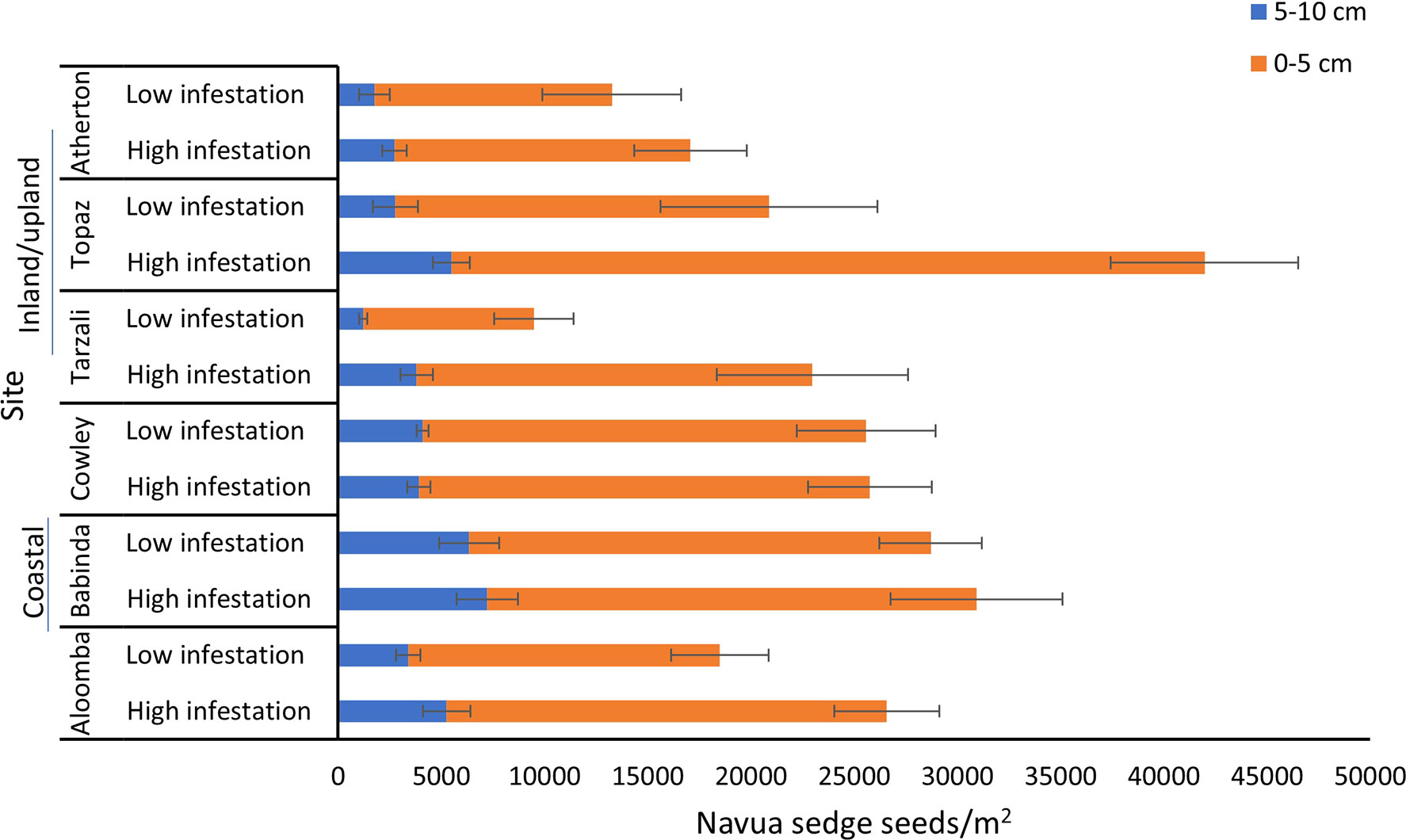
Figure 4 Navua sedge seeds/m2 present (mean ± standard error) in the upper (0-5 cm) and lower (5-10 cm) soil depths in high and low infestation plots in each of the study site. Aloomba, Babinda and Cowley are coastal sites and Tarzali, Topaz and Atherton are inland/upland sites. N= 4-6 plots per site per infestation level per soil depth.
The abundance of Navua sedge seeds in the soil was significantly positively correlated with (i) the abundance of broadleaf seeds in soil (r = 0.2; P = 0.03); (ii) aboveground biomass of Navua sedge (r = 0.53; P = 0.006; Figure 5); and (iii) the proportion of sand in the soil (r = 0.34; P = 0.009); and was negatively correlated with silt proportion (r = -0.39; P = 0.002) of the soil (Table 3). The positive correlation between the abundance of Navua sedge seeds in soil with that of aboveground biomass of Navua sedge is mainly driven by two sites in the inland/upland region (Topaz: r = 0.78, p = 0.007; Tarzali: r = 0.84, p = 0.009) (Figure 5). No significant correlation was observed between the abundance of Navua sedge seeds present in the soil and the time since infestation by the Navua sedge weed (p = 0.799) (Table 3).
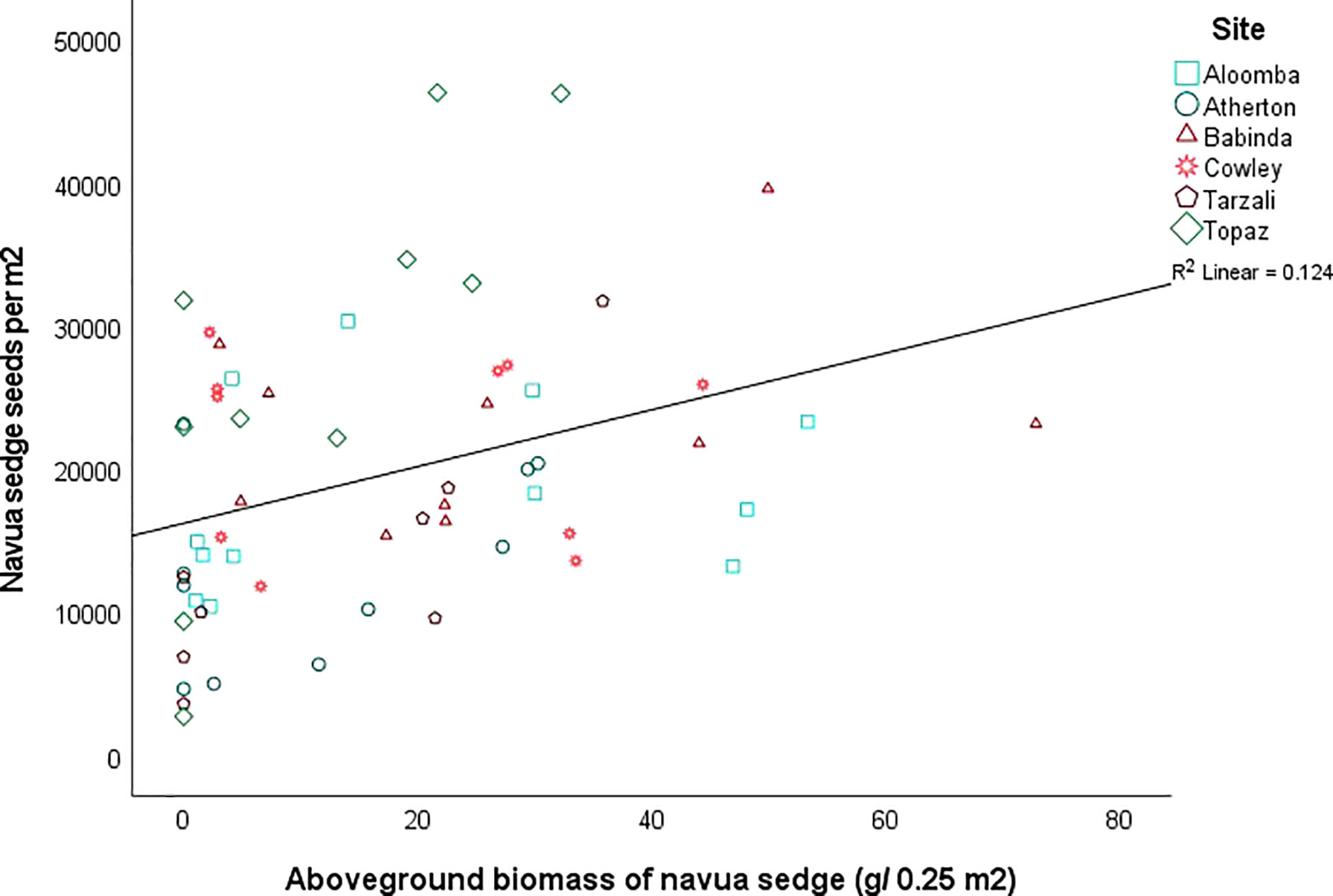
Figure 5 Relationship between number of Navua sedge seeds present in the soil and aboveground biomass of the weed (y=1.62E4+1.98E2*x). N= 4-6 plots per site; For each of the site, data has been pooled across both high and low infestation plots and 0-5 and 5-10 cm soil depths.
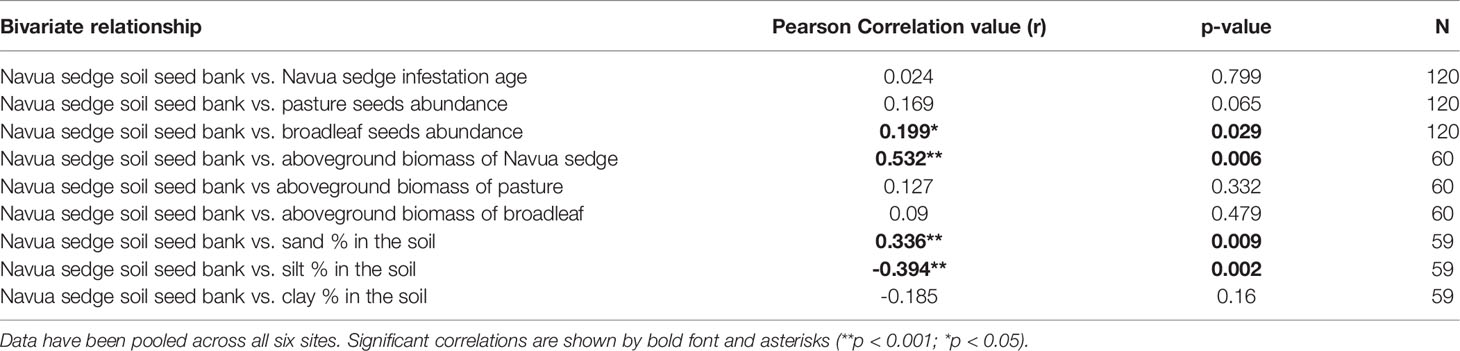
Table 3 Pearson’s correlation coefficients (r) for correlation of the amount of Navua sedge seeds present in the soil.
Discussion
While a diverse number of species typically constitute the soil seed bank in any landscape, a larger proportion (70-90%) of the assemblage is usually composed of a few dominant species, and hence these are often the primary cause of concern where such species are invasive (Wilson, 1988; Roham et al., 2014). We observed that Navua sedge has a large and persistent reserve of seeds in the soil in the sites examined in this study. These seeds, like many other small-seeded species measure ̴ 1 mm in length by 0.75 mm in width, with an average weight of 100 seeds being 25 ± 0.1 mg. As a consequence, they are easy to incorporate into the spaces (pores) between soil particles like many other small-seeded species (Rejmánek, 2000).
The relatively low number of pasture seeds in the soil seed bank of all sites could be due to the fact that these were all grazing sites where cattle preferred pasture over Navua sedge. The pasture species are therefore often given minimal chance to grow to reproductive maturity and produce seeds. This is consistent with other studies, where in most cases, weed-infested plots often have lower assemblage of pasture and broad leaf species (Gioria and Osborne, 2009; Nigatu et al., 2010; Nguyen et al., 2017). The short life span of pasture seeds may also contribute to their low numbers in the soil seed bank (Dantas-Junior et al., 2018). In addition, most pasture grasses are usually perennial which could prioritise vegetative growth over seed production. It is noteworthy that the soil was collected only once, during the month of April which is the time when the pasture plants start to produce seeds in tropical north Queensland. Hence, to improve the accuracy of soil seedbank estimations in pastures infested by Navua sedge, more frequent sampling of these soils across seasons may be required (Osunkoya et al., 2013).
Although the high infestation plots have significantly higher numbers of seeds in the 0-5 cm of soil layer compared with low infestation plots, a large number of Navua sedge seeds (> 16,000 seeds/m2) were present in the low infestation plots (Figure 3). This observation suggests that the absence of Navua sedge plants in a particular standing vegetation may not necessarily reflect its absence in the soil seed bank as there may still be a significant possibility of recruitment of Navua sedge seeds into the soil in such habitats if conditions are conducive (Gioria et al., 2014; García et al., 2021). Lateral soil movement and/or dispersal of seeds may contribute to the presence of seeds of Navua sedge in the soils in weed free plots or low-weed areas. As our investigated sites are pasture lands open to continuous cattle movement while being grazed, it is likely that Navua sedge seeds may have been dispersed through cattle movement and faecal excretion of ingested seeds into weed free areas, some of which may easily get incorporated into the soil (Chadha et al., 2021).
The levels of Navua sedge seed density in the two soil depths, 0-5 cm and 5-10 cm, were significantly different with 83% of the total Navua sedge seeds present in the top 0-5 cm soil depth (Figure 2). This difference in the amount of Navua sedge seeds present was found across both the high and low infestation plots. Similar to our findings, Vitelli et al. (2010) reported similar declines in seed density with soil depth, with 55% of the total Navua sedge seeds found in the top 2 cm of the soil. Other studies have also found highest densities of seeds of Cyperus species in the upper (0-10 cm) soil depth (Roham et al., 2014; Srivastava and Singh, 2014; Khanghahi et al., 2019). Small seeds are typical for the genera Cyperus, which are more likely to be buried and thus contribute to a persistent seed bank (Leck and Schütz, 2005). The maximum densities of weed seeds are also found in the 0-5 cm layer of the soil in cropping systems (Thompson et al., 1997; Hosseini et al., 2014; Singh et al., 2015). The spatial distribution of Navua sedge seeds in the soil seed bank could inform specific management strategies required (Bhowmik, 1997; Mall and Singh, 2014). As Navua sedge seeds require light for germination and do not emerge when buried deeper than 2 cm, the soil seed bank can potentially be manipulated through tillage at certain intensities (Chadha et al., 2021). The weed seed bank can reduce in abundance by encouraging germination of buried seeds via surface tillage that tend to bring Navua sedge seeds to the surface, or by using more intense tillage that can bury the seeds deep enough to prevent them from emerging. However, the persistent nature of the seeds should be considered when burying the seeds deeper than 2 cm as more than 30% seeds remain viable after 5 years (Vitelli et al., 2010).
Although a positive correlation was observed between the aboveground biomass of Navua sedge and the number of Navua sedge seeds present in the soil (Figure 5), disturbances and environmental conditions can affect the relationship between below and above ground vegetation (Scott et al., 2010; García et al., 2021). The highest amount of aboveground Navua sedge biomass was present in the high infestation plot at Babinda, but the site did not have the highest number of Navua sedge seeds in the soil (Figure 4). In contrast, Topaz site had the least number of aboveground biomass of the weed but the amount of Navua sedge seeds present in the soil was the highest. High aboveground biomass of Navua sedge present at the Babinda site could be due to the site not being grazed around the time of survey (Table 1), whereas all the other sites were currently grazed pastures. Although, Navua sedge is not readily palatable and is not usually grazed due to its low nutritional value, it can be consumed by cattle if there is a lack of other palatable species (Kerr et al., 1995). This could be an important reason for the lack of relationship found between the aboveground biomass of Navua sedge and the amount of Navua sedge seeds present in the soil at some of the sites. Also, increased competition from grasses may reduce the biomass of Navua sedge (Soni, 2019).
No correlation was observed between seed bank abundance of Navua sedge and the time since the weed’s infestation. Incorrect estimation of infestation age provided by the farmers may be a contributing factor to the lack of relationship observed, as small infestations in the farms could have gone unnoticed for years. However, we would expect that as an invasion progresses, changes in the aboveground vegetation’s composition will have a greater impact on the seed bank, with overall effects on the vegetation being additive or even multiplicative over time (Gioria and Osborne, 2010). The size of a species’ seed bank is typically proportional to the period of time it has been present in the soil seed bank. Thus, in the long run, in the absence of (or where there is lower) recruitment of seeds from the vegetation, considerable changes in the seed bank will occur, even for persistent weed species due to natural seed senescence, predation and probable changes in the viability of seeds present in the seed bank (Marchante et al., 2011; González-Muñoz et al., 2012). Ideally, fine soil particles like clay impede the availability of oxygen and the elimination of toxic fermentative metabolites evoked by hypoxia, thus providing a more favourable environment to extend seed longevity of buried seeds (Bailly, 2004; Wiebach et al., 2020; Benvenuti and Mazzoncini, 2021). However, we have found contrary results in our study, with a significant positive correlation between the abundance of Navua sedge seeds and sand content in the soil (Table 3). This could be due to reduced water holding capacity of the soil with increasing sand content (Libohova et al., 2018), and hence affording greater opportunity for trapped seeds to remain viable for a longer time.
In theory, soil seed banks of weed species can be depleted by preventing or severely reducing the addition of seeds to the seed bank, increasing seed mortality, and by management strategies that give favourable environment to germinate the existing seeds in the soil (Buhler et al., 1997; Gulden and Shirtliffe, 2009). In this regard, weed seed production needs to fall below the seedbank replacement value to ensure overall reduction in the soil seed bank (Schwartz-Lazaro and Copes, 2019). In practice, however, managing the soil seed bank of Navua sedge is complex due to multiple reasons. It is difficult to prevent the addition of new seeds as Navua sedge, being a rhizomatous plant, has new growth continuously occurring from its rhizome which can subsequently add to the seed bank (Karan, 1975). Another reason is the remarkable persistency of seed banks formed by Navua sedge; it has been found in depletion studies, that nearly 30% of its seeds are still viable after five years (Vitelli et al., 2010). Also, the extraordinary high potential of seed production aids in the difficulty of reducing its seed bank. Ecological study conducted by Vitelli et al. (2010) has shown that a dense stand of Navua sedge (200 plants/m2) may contain seed bank densities of around 56,700 viable seeds/m2. Hence, it may be more practical to accept the soil seed bank of Navua sedge as part of the environment and devise management strategies to reduce germination from or maintain dormancy of its soil seed bank, rather than attempt to eliminate it. It will also be beneficial to develop models of the weed’s aboveground threshold level (abundance or biomass) below which its impact on natural ecosystem or crop/pasture yield is either zero to minimal (Panetta et al., 2019; Schwartz-Lazaro and Copes, 2019).
It is now widely recognised that Navua sedge exhibits intrinsic reproductive traits that aid its invasive potential, including: (i) comparatively rapid growth (A. Chadha, unpublished data), (ii) prolonged and often extended flowering period, provided growth conditions are favourable, (iii) the ability to form vegetative buds on the rhizome (Karan, 1975), (iv) significantly high fecundity (Vitelli et al., 2010), high germination rates (Chadha et al., 2021), (vi) a lack of primary dormancy in seed (Chadha et al., 2021) and (vii) long seed life (Vitelli et al., 2010). It is recommended that these attributes should be the targets of management strategies for Navua sedge. Plants like Navua sedge which produces a high number of seeds with long term viability may undergo rapid range expansion and create problems for seedbank management long after the aboveground biomass has been removed (D’Antonio and Meyerson, 2002; King, 2011). As a consequence, future studies should strive to understand the soil seed bank dynamics of Navua sedge in other cropping systems such as sugar cane and banana farms and across more varying landscapes, such as riparian zones, roadsides, etc in order to create site focussed management practices.
The seedling emergence method as used in this study provides a reliable indication of the size of the rapidly germinable seed bank, but it does not provide a comprehensive assessment of the overall seed bank flora unless the soil sample is kept at the simulated habitat environments for extended periods of time (Thompson and Grime, 1979). Although the seed extraction method often provides a better estimate than the seedling emergence method (Bernhardt et al., 2008; Hussain et al., 2017), it is very laborious and ineffective at finding small seeds such as that of Navua sedge. It could also overestimate seed abundance due to the inclusion of non-viable seeds (Thompson et al., 1997; Baskin and Baskin, 1998). Hence, the approach in the present study of observing seedling emergence for eight months during which germination asymptotes (in terms of cumulative seedling emerging) were reached indicates that the methodology gives a good estimate of comparative (rather than absolute) abundance of seeds buried in the soil.
Other barriers to this study included not having adequate or verified information from local farmers on the actual time of the weed’s invasion, which was a research shortcoming that could not be avoided. Also, it can be assumed that dormancy of all seed of all plant species in the soil would not have been broken as only two factors, temperature and moisture, were used to stimulate germination in the glasshouse.
Conclusion
The influence of time of collection (season or year) cannot be discounted not to have a major influence on plant dynamics, including the soil seed bank. Thus, more studies of differing seasons should be encouraged. Nonetheless, there is a strong signal that our findings are robust and can be generalised as data collected in this present work came from multiple sites (six), and seed bank patterns obtained per site and/or landscape were reasonably consistent in terms of major factors investigated (infestation level, soil depth and species group identity).
The study has demonstrated significant variations in the seed bank flora in six grazing lands of tropical north Queensland that are experiencing Navua sedge infestations. However, it was shown that landscape effects (coastal vs inland/upland) were minimal despite differences in their long-term climate scenarios, implying that there are similar dynamics operating for the weed across differing landscapes, and, by implication, similar management tactics are likely to be successful for this weed. Navua sedge was the dominant species in the soil seed bank at all the sites contributing between 62% to 95% of the total seed bank. In most cases, within a given site, a greater abundance of seeds of the weed occurred in densely infested plots relative to low/weed-free areas, and was mostly found in the topsoil layer of 0-5 cm. The abundance of Navua sedge seed present in the soil was significantly correlated with the aboveground biomass of Navua sedge. However, no correlation was observed between the amount of Navua sedge seeds present in the soil and the infestation age of Navua sedge.
The high number of Navua sedge seeds in the soil suggests that continuous management is required even without further input of seeds to deplete the soil seed bank. The results obtained from this study will serve as important baseline work necessary for improving our understanding of the soil seed bank of Navua sedge. Understanding the changes in the soil seed bank community of invaded pastures is essential in designing effective management programs for Navua sedge. Management strategies for Navua sedge should take into account not only the control of above ground foliage but also the soil stored seed and bud banks.
Data Availability Statement
The raw data supporting the conclusions of this article will be made available by the authors, without undue reservation.
Author Contributions
SF and KD, conceptualisation. AC, OO, BS, SF, and KD, methodology and writing. AC, OO, BS, and KD, data collection. AC and OO, analysis. All authors contributed to the article and approved the submitted version.
Funding
The Ph.D scholarship for AC was funded by Federation University Australia, and the funding for the project was provided by the Department of Agriculture and Fisheries, Biosecurity Queensland, Australia.
Conflict of Interest
The authors declare that the research was conducted in the absence of any commercial or financial relationships that could be construed as a potential conflict of interest.
Publisher’s Note
All claims expressed in this article are solely those of the authors and do not necessarily represent those of their affiliated organizations, or those of the publisher, the editors and the reviewers. Any product that may be evaluated in this article, or claim that may be made by its manufacturer, is not guaranteed or endorsed by the publisher.
Acknowledgments
The authors would like to thank Bernie English, Melissa Setter and Stephen Setter from the Department of Agriculture and Fisheries, Biosecurity Queensland, for their assistance with the collection of samples.
References
Ambrosio L., Iglesias L., Marín C., Del Monte J. P. (2004). Evaluation of Sampling Methods and Assessment of the Sample Size to Estimate the Weed Seedbank in Soil, Taking Into Account Spatial Variability. Weed. Res. 44, 224–236. doi: 10.1111/j.1365-3180.2004.00394.x
Bailly C. (2004). Active Oxygen Species and Antioxidants in Seed Biology. Seed. Sci. Res. 14, 93–107. doi: 10.1079/SSR2004159
Baskin C. C., Baskin J. M. (1998). Seeds: Ecology, Biogeography, and, Evolution of Dormancy and Germination (New York: Elsevier).
Benvenuti S., Mazzoncini M. (2021). “Active” Weed Seed Bank: Soil Texture and Seed Weight as Key Factors of Burial-Depth Inhibition. Agronomy 11, 210. doi: 10.3390/agronomy11020210
Bernhardt K. G., Koch M., Kropf M., Ulbel E., Webhofer J. (2008). Comparison of Two Methods Characterising the Seed Bank of Amphibious Plants in Submerged Sediments. Aquat. Bot. 88, 171–177. doi: 10.1016/j.aquabot.2007.10.004
Bhowmik P. C. (1997). Weed Biology: Importance to Weed Management. Weed. Sci. 45, 349–356. doi: 10.1017/S0043174500092973
Booth B. D., Swanton C. J. (2002). Assembly Theory Applied to Weed Communities. Weed. Sci. 50, 2–13. doi: 10.1614/0043-1745(2002)050[0002:AIATAT]2.0.CO;2
Buhler D. D., Hartzler R. G., Forcella F. (1997). Weed Seed Bank Dynamics. J. Crop Prod. 1, 145–168. doi: 10.1300/J144v01n01_07
Bureau Of Meteorology (2022)Climate Statistics for Australian Locations. Available at: http://www.bom.gov.au/climate/data/ (Accessed February 2, 2022).
Chadha A., Florentine S. K., Dhileepan K., Dowling K., Turville C. (2021). Germination Biology of Three Populations of Navua Sedge (Cyperus Aromaticus). Weed. Sci. 69, 69–81. doi: 10.1017/wsc.2020.82
Chauhan B. S. (2012). Weed Ecology and Weed Management Strategies for Dry-Seeded Rice in Asia. Weed. Technol. 26, 1–13. doi: 10.1614/WT-D-11-00105.1
Chejara V. K., Kristiansen P., Sindel B. M., Whalley R. D. B., Nadolny C. (2012). Seed-Bank and Seedling Dynamics in Hyparrhenia Hirta Are Influenced by Herbicide Application and Mowing Management. Rangeland. J. 34, 199–210. doi: 10.1071/RJ11057
Dantas-Junior A. B., Musso C., Miranda H. S. (2018). Seed Longevity and Seedling Emergence Rate of Urochloa Decumbens as Influenced by Sowing Depth in a Cerrado Soil. Grass. Forage. Sci. 73, 811–814. doi: 10.1111/gfs.12347
D’Antonio C., Meyerson L. A. (2002). Exotic Plant Species as Problems and Solutions in Ecological Restoration: A Synthesis. Restor. Ecol. 10, 703–713. doi: 10.1046/j.1526-100X.2002.01051.x
Espeland E. K., Perkins L. B., Leger E. A. (2010). Comparison of Seed Bank Estimation Techniques Using Six Weed Species in Two Soil Types. Rangeland. Ecol. Manage. 63, 243–247. doi: 10.2111/REM-D-09-00109.1
Gandía M., Del Monte J., Santín-Montanyá M. (2022). Efficiency of Methodologies Used in the Evaluation of the Weed Seed Bank Under Mediterranean Conditions. Agronomy 12, 138. doi: 10.3390/agronomy12010138
García A., Loydi A., Distel R. A. (2021). Temporal and Spatial Variation in the Soil Seed Bank of Nassella Trichotoma (Serrated Tussock) in Its Native Range. Aust. J. Bot. 69, 45–51. doi: 10.1071/BT20046
Gioria M., Jarošík V., Pyšek P. (2014). Impact of Invasions by Alien Plants on Soil Seed Bank Communities: Emerging Patterns. Perspect. Plant Ecol. Evol. Syst. 16, 132–142. doi: 10.1016/j.ppees.2014.03.003
Gioria M., Osborne B. (2009). The Impact of Gunnera Tinctoria (Molina) Mirbel Invasions on Soil Seed Bank Communities. J. Plant Ecol. 2, 153–167. doi: 10.1093/jpe/rtp013
Gioria M., Osborne B. (2010). Similarities in the Impact of Three Large Invasive Plant Species on Soil Seed Bank Communities. Biol. Invasions. 12, 1671–1683. doi: 10.1007/s10530-009-9580-7
González-Muñoz N., Costa-Tenorio M., Espigares T. (2012). Invasion of Alien Acacia Dealbata on Spanish Quercus Robur Forests: Impact on Soils and Vegetation. For. Ecol. Manage. 269, 214–221. doi: 10.1016/j.foreco.2011.12.026
Gulden R. H., Shirtliffe S. J. (2009). Weed Seed Banks: Biology and Management. Prairie. Soils. Crops J. 2, 46–52.
Haring S. C., Flessner M. L. (2018). Improving Soil Seed Bank Management. Pest Manage. Sci. 74, 2412–2418. doi: 10.1002/ps.5068
Hopfensperger K. N. (2007). A Review of Similarity Between Seed Bank and Standing Vegetation Across Ecosystems. Oikos 116, 1438–1448. doi: 10.1111/j.0030-1299.2007.15818.x
Hosseini P., Karimi H., Babaei S., Mashhadi H. R., Oveisi M. (2014). Weed Seed Bank as Affected by Crop Rotation and Disturbance. Crop Prot. 64, 1–6. doi: 10.1016/j.cropro.2014.05.022
Hussain M., Safdar A., Tahir M. N., Shah G. A., Ijaz A., Sarwar M. A., et al. (2017). A Comparative Study of Soil Weed Seed Bank Determination in Pothwar Region by Using Different Methodologies. Pak. J. Agric. Res. 30, 310–315. doi: 10.17582/journal.pjar/2017/30.4.310.315
Karan B. (1975). Studies of Navua Sedge (Cyperus Aromaticus). 1. Review of the Problem and Study of Morphology, Seed Output and Germination. Fiji. Agric. J. 37, 59–67.
Kerr D. V., Fell R. F., Murray A. J., Chaseling J. (1995). An Assessment of Factors Associated With Increased Productivity of Dairy Farms in Fiji. Asian-Australas. J. Anim. Sci. 8, 481–487. doi: 10.5713/ajas.1995.481
Khanghahi M. Y., Pirdashti H., Mohseni−Moghadam M., Roham R. (2019). Vertical Distribution of Nutsedge (Cyperus Spp. L.) and Bahiagrass (Paspalum Notatum L.) Seed Bank in Rice Growth Cycle. Acta Universitatis. Agricult. Silvicult. Mendelianae. Brunensis. 67, 787–795. doi: 10.11118/actaun201967030787
King J. R. (2011). Total Nonstructural Carbohydrate Trends and Seed Ecophysiology of the Exotic Invasive Deeprooted Sedge (Cyperus Entrerianus) and its Response to Herbicide and Prescribed Fire Treatments on the Texas Coast.(Master’s Thesis). Ed. Stephen F. (USA: Austin State University).
Koocheki A., Nassiri M., Alimoradi L., Ghorbani R. (2009). Effect of Cropping Systems and Crop Rotations on Weeds. Agron. Sustain. Dev. 29, 401–408. doi: 10.1051/agro/2008061
Leck M. A., Schütz W. (2005). Regeneration of Cyperaceae, With Particular Reference to Seed Ecology and Seed Banks. Perspect. Plant Ecol. Evol. Syst. 7, 95–133. doi: 10.1016/j.ppees.2005.05.001
Libohova Z., Seybold C., Wysocki D., Wills S., Schoeneberger P., Williams C., et al. (2018). And Owens, PReevaluating the Effects of Soil Organic Matter and Other Properties on Available Water-Holding Capacity Using the National Cooperative Soil Survey Characterization Database. R J. Soil. Water Conserv. 73, 411–421. doi: 10.2489/jswc.73.4.411
Liu X., He Y., Xiao Y., Wang Y., Jiang Y., Jiang Y. (2019). Soil Seed Burial and Competition With Surrounding Plants Determine the Emergence and Development of Seedling of an Endangered Species Horsfieldia Hainanensis Merr. In China. Sci. Rep. 9, 17970. doi: 10.1038/s41598-019-54644-7
Llewellyn R., Ronning D., Clarke M., Walker S., Ouzman J. (2016). Impact of Weeds on Australian Grain Production: The Cost of Weeds to Australian Grain Growers and the Adoption of Weed Management and Tillage Practices (Australia: Grains Research & Development Corporation).
Maclaren C., Storkey J., Menegat A., Metcalfe H., Dehnen-Schmutz K. (2020). An Ecological Future for Weed Science to Sustain Crop Production and the Environment. A Review. Agron. Sustain. Dev. 40, 24. doi: 10.1007/s13593-020-00631-6
Mall U., Singh G. S. (2014). “Soil Seed Bank Dynamics: History and Ecological Significance in Sustainability of Different Ecosystems,” in Environment and Sustainable Development. Eds. Fulekar M. H., Pathak B., Kale R. K. (New Delhi: Springer India).
Mao R., Nguyen T. L. T., Osunkoya O. O., Adkins S. W. (2019). Spread Pathways of the Invasive Weed Parthenium Hysterophorus L.: The Potential for Water Dispersal. Austral Ecol. 44, 1111–1122. doi: 10.1111/aec.12774
Marchante H., Freitas H., Hoffmann J. H. (2011). The Potential Role of Seed Banks in the Recovery of Dune Ecosystems After Removal of Invasive Plant Species. Appl. Veg. Sci. 14, 107–119. doi: 10.1111/j.1654-109X.2010.01099.x
Mune T. (1959). Navua Sedge (Cyperus Melanospermus (Nees) Valck. Suring.), a Common Pasture Weed. Fiji. Agric. J. 29, 23–25.
Nguyen T., Bajwa A. A., Belgeri A., Navie S., O’donnell C., Adkins S. (2017). Impact of an Invasive Weed, Parthenium Hysterophorus, on a Pasture Community in South East Queensland, Australia. Environ. Sci. Pollut. Res. 24, 27188–27200. doi: 10.1007/s11356-017-0327-1
Nigatu L., Hassen A., Sharma J., Adkins S. W. (2010). Impact of Parthenium Hysterophorus on Grazing Land Communities in North-Eastern Ethiopia. Weed. Biol. Manage. 10, 143–152. doi: 10.1111/j.1445-6664.2010.00378.x
Osunkoya O. O., Froese J. G., Nicol S., Perrett C., Moore K., Callander J., et al. (2019). A Risk-Based Inventory of Invasive Plant Species of Queensland, Australia: Regional, Ecological and Floristic Insights. Austral Ecol. 44, 1123–1138. doi: 10.1111/aec.12776
Osunkoya O. O., Lock C. B., Dhileepan K., Buru J. C. (2021). Lag Times and Invasion Dynamics of Established and Emerging Weeds: Insights From Herbarium Records of Queensland, Australia. Biol. Invasions. 23, 1–26. doi: 10.1007/s10530-021-02581-w
Osunkoya O. O., Perrett C., Fernando C., Clark C. (2013). Patterns of Seed Bank and Size Asymmetry of Plant Growth Across Varying Sites in the Invasive Lantana Camara L. (Verbenaceae). Plant Ecol. 214, 725–736. doi: 10.1007/s11258-013-0202-1
Panetta F. D., O’loughlin L. S., Gooden B. (2019). Identifying Thresholds and Ceilings in Plant Community Recovery for Optimal Management of Widespread Weeds. NeoBiota 42, 1–18. doi: 10.3897/neobiota.42.30797
Rejmánek M. (2000). Invasive Plants: Approaches and Predictions. Austral Ecol. 25, 497–506. doi: 10.1046/j.1442-9993.2000.01080.x
Roham R., Pirdashti H., Yaghubi M., Nematzadeh G. (2014). Spatial Distribution of Nutsedge (Cyperus Spp. L.) Seed Bank in Rice Growth Cycle Using Geostatistics. Crop Prot. 55, 133–141. doi: 10.1016/j.cropro.2013.09.006
Schwartz-Lazaro L. M., Copes J. T. (2019). A Review of the Soil Seedbank From a Weed Scientists Perspective. Agronomy 9, 369. doi: 10.3390/agronomy9070369
Scott K., Setterfield S., Douglas M., Andersen A. (2010). Soil Seed Banks Confer Resilience to Savanna Grass-Layer Plants During Seasonal Disturbance. Acta Oecol. 36, 202–210. doi: 10.1016/j.actao.2009.12.007
Shi B., Osunkoya O. O., Chadha A., Florentine S. K., Dhileepan K. (2021). Biology, Ecology and Management of the Invasive Navua Sedge (Cyperus Aromaticus)—A Global Review. Plants 10, 1–16. doi: 10.3390/plants10091851
Singh M., Bhullar M. S., Chauhan B. S. (2015). Seed Bank Dynamics and Emergence Pattern of Weeds as Affected by Tillage Systems in Dry Direct-Seeded Rice. Crop Prot. 67, 168–177. doi: 10.1016/j.cropro.2014.10.015
Soni A. (2019). Management of Navua Sedge (Cyperus Aromaticus) Using Competition and Simulated Herbivory Interactions of Humidicola Grass (Brachiaria Humidicola) and Rhodes Grass (Chloris Gayana). (Master’s Thesis) (Australia: The University of Queensland).
Srivastava R., Singh K. P. (2014). Diversity in Weed Seed Production and the Soil Seed Bank: Contrasting Responses Between Two Agroecosystems. Weed. Biol. Manage. 14, 21–30. doi: 10.1111/wbm.12029
Thompson K. (2000). “The Functional Ecology of Soil Seed Banks,” in Seeds: The Ecology of Regeneration in Plant Communities. Ed. Fenner M. (Wallingford, UK: CAB International).
Thompson K., Bakker J. P., Bekker R. M. (1997). “Methods of Seed Bank Analysis,” in The Soil Seed Banks of North West Europe: Methodology, Density and Longevity (Cambridge: Cambridge University Press).
Thompson K., Grime J. P. (1979). Seasonal Variation in the Seed Banks of Herbaceous Species in Ten Contrasting Habitats. J. Ecol. 893-921. doi: 10.2307/2259220
Vitelli J. S., Madigan B. A., Van Haaren P. E. (2010). Control Techniques and Management Strategies for the Problematic Navua Sedge (Cyperus Aromaticus). Invasive. Plant Sci. Manage. 3, 315–326. doi: 10.1614/IPSM-D-09-00036.1
Vogler W. D., Carlos E. H., Setter S. D., Roden L., Setter M. J. (2015). Halosulfuron-Methyl: A Selective Herbicide Option for the Control of the Invasive Cyperus Aromaticus (Ridley) Mattf. And Kukenth (Navua Sedge). Plant Prot. Q. 30, 61–66. doi: 10.3316/informit.441829144890169
Weisberger D., Nichols V., Liebman M. (2019). Does Diversifying Crop Rotations Suppress Weeds? A Meta-Analysis. PloS One 14, e0219847. doi: 10.1371/journal.pone.0219847
Wiebach J., Nagel M., Börner A., Altmann T., Riewe D. (2020). Age-Dependent Loss of Seed Viability is Associated With Increased Lipid Oxidation and Hydrolysis. Plant Cell. Environ. 43, 303–314. doi: 10.1111/pce.13651
Wilson R. G. (1988). “Biology of Weed Seeds in the Soil,” in Weed Management in Agroecosystems: Ecological Approaches. Eds. Altieri M. A., Liebman M. (Boca Raton: CRC Press).
Keywords: weed, wet-tropics, invasion time, seed distribution, seed density, population dynamics
Citation: Chadha A, Osunkoya OO, Shi B, Florentine SK and Dhileepan K (2022) Soil Seed Bank Dynamics of Pastures Invaded by Navua Sedge (Cyperus aromaticus) in Tropical North Queensland. Front. Agron. 4:897417. doi: 10.3389/fagro.2022.897417
Received: 16 March 2022; Accepted: 02 May 2022;
Published: 27 May 2022.
Edited by:
Iraj Nosratti, Razi University, IranReviewed by:
Agnieszka Synowiec, University of Agriculture in Krakow, PolandMd Asaduzzaman, New South Wales Department of Primary Industries, Australia
Copyright © 2022 Chadha, Osunkoya, Shi, Florentine and Dhileepan. This is an open-access article distributed under the terms of the Creative Commons Attribution License (CC BY). The use, distribution or reproduction in other forums is permitted, provided the original author(s) and the copyright owner(s) are credited and that the original publication in this journal is cited, in accordance with accepted academic practice. No use, distribution or reproduction is permitted which does not comply with these terms.
*Correspondence: Aakansha Chadha, aakanshachadha@students.federation.edu.au