- 1Department of Plant and Soil Sciences, Mississippi State University, Starkville, MS, United States
- 2Truck Crops Branch Experiment Station, Mississippi State University, Crystal Springs, MS, United States
One of the most significant yield losses in tomato (Solanum lycopersicum L.) is due to weeds. Yellow and purple nutsedge, large crabgrass, and Palmer amaranth are the most troublesome weed species in tomato production throughout the southeastern United States. This study aimed to determine the impact of soil steaming, plastic mulching, and cover crops on weed suppression, tomato height, and fruit yield. The cover crops used were hairy vetch (Vicia villosa), crimson clover (Trifolium incarnatum), and cereal rye (Secale cereale). The study was conducted at the Mississippi State University Truck Crops Experiment Station in Crystal Springs, Mississippi, USA. The experiment used a completely randomized block design with three fall cover crop treatments, including fallow, and each was replicated three times and repeated in two years. Each plot was broadcasted with a mixture of yellow nutsedge (Cyperus esculentus L.), large crabgrass (Digitaria sanguinalis L.), barnyardgrass (Echinochloa crus-galli), and Palmer amaranth [Amaranthus palmeri (S.) Watson] at a density of 20 plants m-2 for each weed species. Two days after sowing the weed seeds, the soil surface was steamed according to its assigned treatment until it reached 61°C for either 0, 5, or 20 min. After steaming, drip irrigation tubing was laid on each row, and covered by black, 0.0254-mm plastic mulch. Data were recorded in both years, including weed cover, plant height, and fruit yield. The lowest weed cover was observed at 5 min of soil steaming in mulched treatment, and the highest cover was noted at 0 min of soil steaming in the absence of mulching. Yellow nutsedge was the dominant weed species, even under steam and mulch treatments. The use of cover crops did not show a difference compared to fallow treatments. However, hairy vetch showed the lowest weed cover, followed by crimson clover. Tomato plants in steamed soil were up to 13 cm taller than those in unsteamed soils. Additionally, steaming at 5 or 20 min in combination with plastic mulch increased the marketable and cull yield. Soil steaming and mulching increased tomato plant height and yield while decreasing weed population and can, therefore, be effectively incorporated into an integrated weed management program in tomato.
Introduction
Tomato (Solanum lycopersicum L.) is one of the most produced and consumed vegetables worldwide, and it requires considerable care in its production to prevent yield loss (Sunday et al., 2022). One factor that reduces tomato yield considerably is weed competition, which can be described as the competition between crops and weeds for vital resources such as water, nutrients, light, soil, and space (Giles et al., 2004). Various methods prevent, control, and exterminate weeds in the field. Still, one of the most time-efficient and effective methods to manage different weeds is by using herbicides (Manisankar et al., 2022). However, chemical control should be used judiciously to protect its efficacy while also ensuring the safety of surface water and environmental pollution. Doing so slows the evolution of resistant weeds that threaten herbicide efficacy, ultimately protecting farmer profits while minimizing herbicide’s environmental impact. Rather than relying on chemical control alone, layering weed management strategies creates a more robust and sustainable approach (Naeem et al., 2022). Additionally, herbicide use is restricted in organic production and demands developing and optimizing alternative strategies.
Cover crops are primarily planted to improve soil structure, increase soil organic matter, enhance water viability, reduce erosion, control pests and diseases, and increase soil nitrogen rate, which is an essential component for different plants (Kenney et al., 2015), consequently increasing the yield (Finney et al., 2017). Cover crops control weeds by competing for resources and inhibiting weed development through allelochemical production, blocking stimuli for weed seed germination, or altering the soil microbial population to put weed species at a disadvantage (Teasdale et al., 2007). Some cover crop species release nitrogen faster than other species, such as hairy vetch (Vicia sativa L.) and cereal rye (Secale cereal L.). Hairy vetch is a legume with better nitrogen-fixing ability, deeper roots, and a lower C/N ratio that decays very rapidly in the soil. For these reasons, hairy vetch releases more nitrogen for immediate use and at a faster rate than cereal rye. Cereal rye is a grass species with fibrous roots extending to the soil and acting like a sponge soaking up water. The roots help anchor the plant to the ground and have greater C/N ratios resulting in a prolonged composting process (Brust, 2019). However, both species can decrease nitrogen fertilizer costs because they rapidly decompose and release nitrogen into the soil (Sievers and Cook, 2018). In Missouri, Cornelius and Bradley (2017) reported that cereal rye plus hairy vetch and cereal rye cover crops reduced the winter annual weed cover by 68 and 72%, respectively. Additionally, the same cover crop treatments substantially reduced (41%) early-season summer annual weeds. It has been reported that cover crops such as cereal rye exude phytotoxic allelopathic compounds that promote the suppression of different weeds (Schulz et al., 2013).
Another alternative to control weeds in a sustainable agricultural system is using synthetic materials or plant residues/waste on the soil, also known as mulching (Marıń-Guirao et al., 2022). One of the materials intensively used as mulch is plastic film. Mulch film improves soil temperature and moisture, providing a suitable environment for enzymes produced by the microorganism community and improving soil productivity. The additional advantage of mulching is improved weed management by preventing weed seed germination and blocking emerging seedlings’ growth. Also, mulching blocks photosynthetically active radiation while allowing the infrared transmission to maintain the soil warm (Akhtar et al., 2018; Monteiro and Santos, 2022; Zhang et al., 2022).
Soil steaming is another strategy that can be used to effectively control weed emergence and growth through exposure to high temperatures. It has traditionally been used as a fumigant replacement in ornamentals and horticultural crops where some chemicals are not permitted (Fennimore and Goodhue, 2016; Baldoin et al., 2010). Soil steaming is shown to reduce weed seed germination by 50% at 62-68°C and 90% at 76-86°C (Bitarafan et al., 2022). Also, high temperatures damage plant membranes, resulting in cellular damage (Monteiro and Santos, 2022; Samtani et al., 2011; Fennimore et al., 2016). According to Bitarafan et al. (2022), no germination occurred in soil steaming with a target temperature of 99°C at 90, 180, or 540 s. A study on strawberry (Fragaria ×ananassa) production reported that steaming soil at 70°C for 20 min provides similar weed control efficacy as methyl bromide and chloropicrin treatments (Samtani et al., 2011).
Combining cover crops, soil steaming, and mulching for weed suppression may improve weed control in tomato. Consequently, this study aimed to test the presence and absence of cover crops and the effects of soil steaming, with and without plastic mulch, on weed suppression, tomato plant growth, and tomato yield.
Material and methods
Studies were conducted in the 2021-2022 growing season at the MSU Truck Crops Experiment Station at Crystal Springs, Mississippi, United States (lat. 31°56′45.8’’N, 90°22′40.4’’ W). The experiment was conducted as a split-split plot design with year as the whole plot factor, the cover crops (crimson clover, cereal rye, hairy vetch, and fallow) as the split-plot factor, and the split-split plot factor being a factorial arrangement of steaming duration (0, 5, and 20 min), mulching (mulched, non-mulched), and weed species (barnyardgrass, large crabgrass, Palmer amaranth, and yellow nutsedge) in a randomized complete block, and each treatment combination had three replications. The cover crops were sowed in November 2020 and 2021 at a rate of 69, 84, and 84 kg/ha, respectively.
In the spring, the entire field was cut to a height of 13 cm and sprayed with glyphosate (Roundup Powermax®, 48.7% active ingredient) at a rate of 868 g/ha during the spring to control weeds. A week after herbicide application, the field was tilled to a depth of 10 cm. Twenty-four 0.6 m wide by 27 m long rows were formed to prepare the field for the tomato transplants. Each row was spaced 0.9 m apart. Twenty-four rows were formed per replication (block) and were broadcasted at a density of 20 plants m-2 for each weed species: yellow nutsedge (Cyperus esculentus L.), large crabgrass (Digitaria sanguinalis L.), Palmer amaranth [Amaranthus palmeri (S.) Watson] and barnyardgrass (Echinochloa crus-galli). These are the most problematic weed species in tomato (Webster, 2014). Weed seeds of large crabgrass, Palmer amaranth, and barnyardgrass and tubers (in the case of yellow nutsedge) were broadcasted on the soil surface. Immediately after broadcasting the seeds and tubers, a roller was used to endure good contact with the soil surface. Two days after sowing (DAS) the weed seeds, the soil surface in each plot was exposed to steaming until soil temperature reached 61°C at 10 cm deep, after which steaming was continued for 5 and 20 min based on the assigned steam treatment. Plots assigned for 0 min of steam were not steamed. The steam generator (Steam-Flo model SF-20, Sioux Corporation, Beresford, South Dakota, USA) used for this study is rated for 308 kg/hr steam output. To apply the steam, a permeable, woven, 5.30 cm nylon sleeve (Beresford, S. USA) was placed over the center of each plot assigned to be steamed and covered with a heat-resistant, three-ply, 0.1524-mm tarp with reinforced scrim (Beresford, S. USA) to capture the steam from the sleeve and allow it to penetrate the soil (Figure 1). Chains were placed around the perimeter of the tarp to hold the tarp in place during the steaming process and prevent steam loss through the sides of the tarp. The soil temperature was monitored at a depth of 10 cm by a temperature probe connected to a 4-channel temperature monitoring system (Signals 4-Channel, Thermoworks, American Fork, Utah, USA).
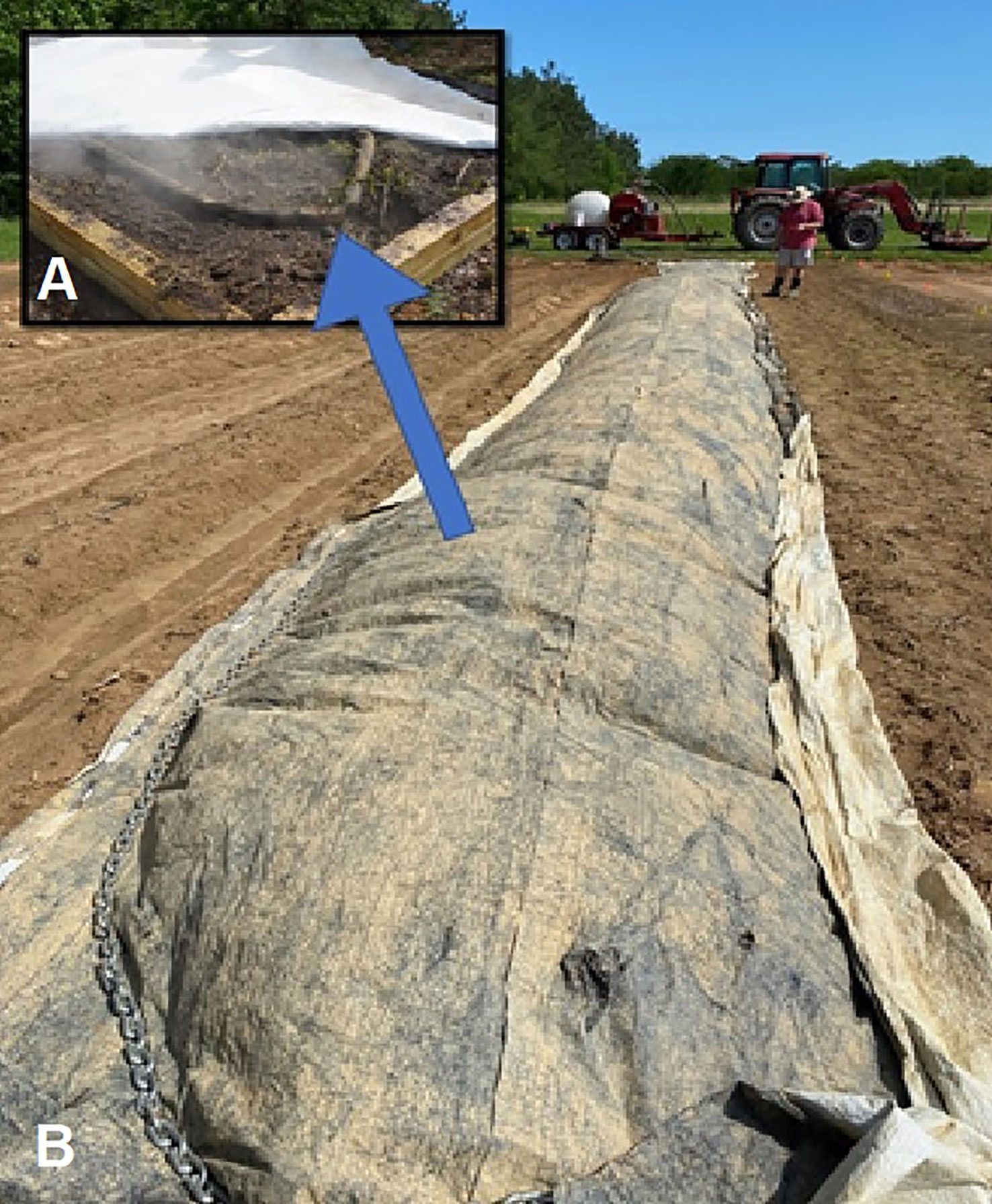
Figure 1 Steaming system used in the experiment with a permeable nylon sleeve placed over the center of each plot (A) and covered with a heat-resistant, three-ply, 0.1524-mm tarp with reinforced scrim with chains placed around the perimeter of the tarp to hold the tarp and prevent steam loss through the sides of the tarp (B).
After steaming, drip irrigation tubing was laid on each row. As per soil test recommendations, 3 kg of 0-20-20 (N-P-K; P2O5 and K2O; Bumper Crop, Schulenburg, TX) and 0.9 kg of 33-0-0 (50% CH4N2O and 50% (NH4)2SO4; Bumper Crop, Schulenburg, TX) were applied to each row. The clay-loam soil had a pH ranging from 5.9-6.2 within the field. Twelve of 24 rows in each replication (block) were randomly assigned to be covered by black, 0.0254-mm plastic mulch. Each treatment combination was replicated three times for a total of 72 0.9 × 6-m subplots. A 3-m gap was provided between subplots.
The tomato cultivar Roadster was used throughout the study. It is marketed as a determinate fresh market, slicing tomato with 227 to 340 g of fruits. The tomato transplants were generated by sowing seeds into 72-cell plug trays filled with soilless potting media (Pro-mix BX; Rivière-du-Loup, Quebec, Canada) and grown in a greenhouse. Tomato transplants developed at least four true leaves at the time of transplant. Six tomato plants were planted 61 cm apart in each subplot in May 2021 and 2022.
Weed cover per plot (0.9 × 6-m) were collected based on the visual ground cover (%) (“weed cover” hereafter) of each weed species 10 weeks after transplanting (WAT) and rated using the scale 0 to 100%, where 0% is no weed present, and 100% is complete ground cover within the plot by weed. The weed cover was measured once for the whole plot at 10 WAT. The height (cm) of the tomato plants was measured using a ruler from the base of the plant to the tip of the uppermost leaf at 10 WAT. Tomato height was based on average of three plants per plot. Tomato were harvested from all of the plants and combined from each subplot before they were weighted (i.e., we weighed the fruits as a group and not as individual plants) and graded as marketable or unmarketable, and the total yield (t/ha) based on fresh weight was determined.
All results were run through LS-means in JMP Pro 16.1 (SAS Institute Inc., Cary, NC, USA). For data that met the assumptions for ANOVA, treatment means were separated using the Tukey’s test at an alpha level of ≤ 0.05. Data from the two years of the experiment were pooled if there was no significant experimental effect.
Results
Weed cover
Cover crops on weed cover
Cover crop treatments did not significantly differ in weed emergence compared to fallow treatment in both years. Although not statistically significant, hairy vetch in 2021 and crimson clover in 2022 resulted in the lowest weed cover.
Mulching on weed cover
We found no significant interactions between mulching and year (Table 1). Nevertheless, the mean cumulative 2-year weed cover (%) in mulched treatment significantly affected weed emergence (P < 0.05) compared to non-mulched treatment (Figure 2). Plastic mulch reduced weed cover by almost 50% compared with no mulch (Figure 2). Although the use of plastic mulching was not significant for Palmer amaranth and barnyardgrass, a significant improvement in the control of yellow nutsedge and large crabgrass population was found under mulch application. However, among the weed species, the cover of yellow nutsedge was the highest, followed by large crabgrass under mulched and non-mulched treatments. In the presence of mulching, the cover of yellow nutsedge was reduced by 50%, while the cover of large crabgrass was decreased by 64% (Figure 3).
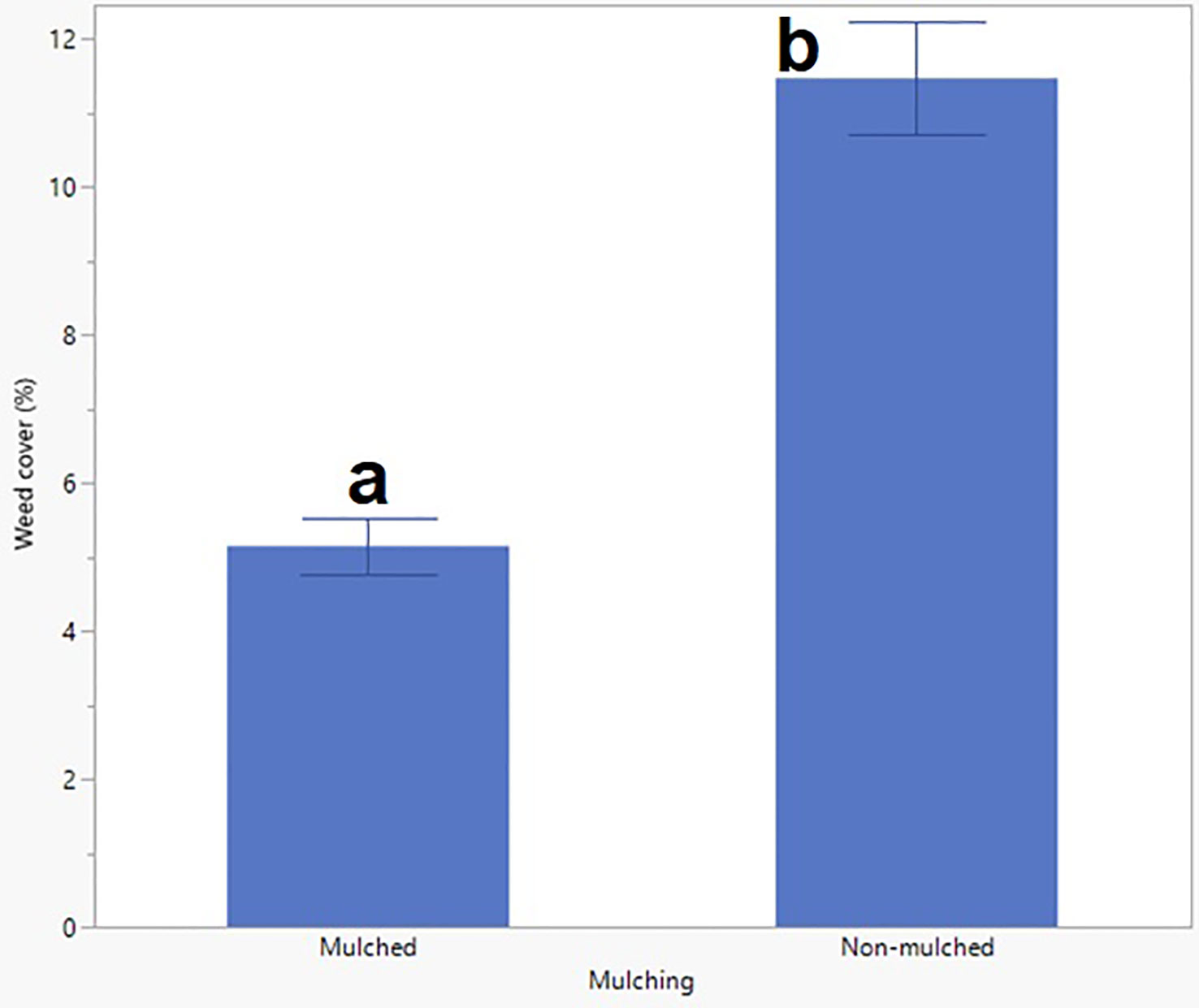
Figure 2 Mean cumulative 2-year weed cover (%) in mulched and non-mulched treatment collected based on the overall plot cover of each weed species 10 weeks after transplanting (WAT) and rated using the scale 0 to 100%, where 0% is no weed present, and 100% is complete coverage. The error bars indicate the standard error of three measurements. All results were run through LS-method in JMP Pro 16.1 (SAS Institute Inc., Cary, NC, USA). For data that met the assumptions for ANOVA, treatment means were separated using the Tukey’s test at an alpha level of ≤ 0.05. Means followed by the same letter are not significantly different (P < 0.05).
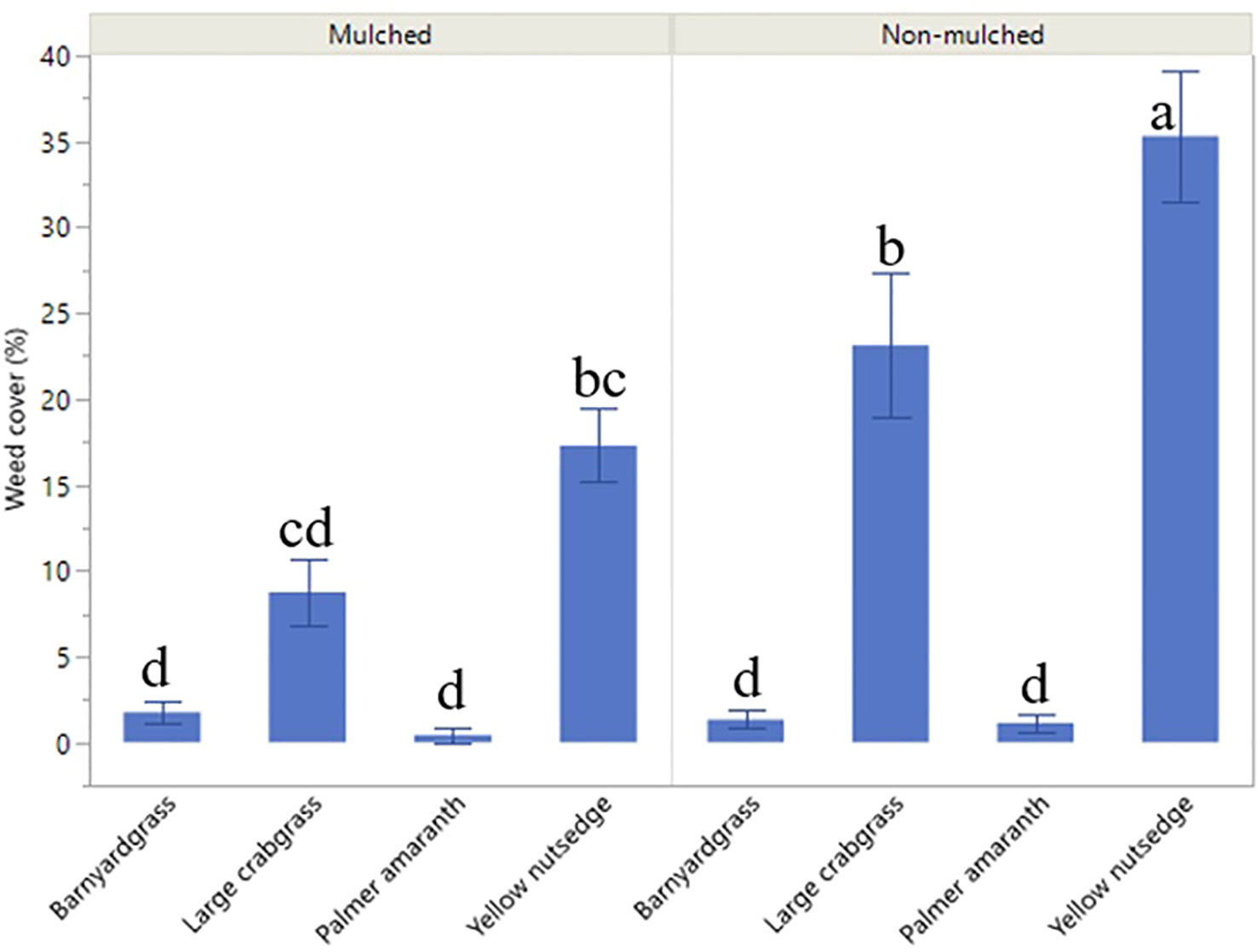
Figure 3 Mean cumulative 2-year weed cover (%) by weed species in mulched and non-mulched treatment collected based on the overall plot cover of each weed species 10 weeks after transplanting (WAT) and rated using the scale of 0 to 100%, where 0% is no weed present, and 100% is complete coverage. The error bars indicate the standard error of three measurements. All results were run through LS-method in JMP Pro 16.1 (SAS Institute Inc., Cary, NC, USA). For data that met the assumptions for ANOVA, treatment means were separated using the Tukey’s test at an alpha level of ≤ 0.05. Means followed by the same letter are not significantly different (P < 0.05).
Steaming on weed cover
The use of steaming significantly reduced the population of different weed species in both years (Table 1). In 2021 and 2022, the use of steaming at 5 and 20 minutes significantly reduced (P < 0.05) large crabgrass compared to 0 minutes. However, soil steaming did not significantly affect the population of barnyardgrass, and Palmer amaranth in either 5 or 20 minutes compared to 0 minutes. The major weed species in both years was yellow nutsedge. The 5- and 20-min steaming was less effective in controlling yellow nutsedge than unsteamed plots (Figure 4).
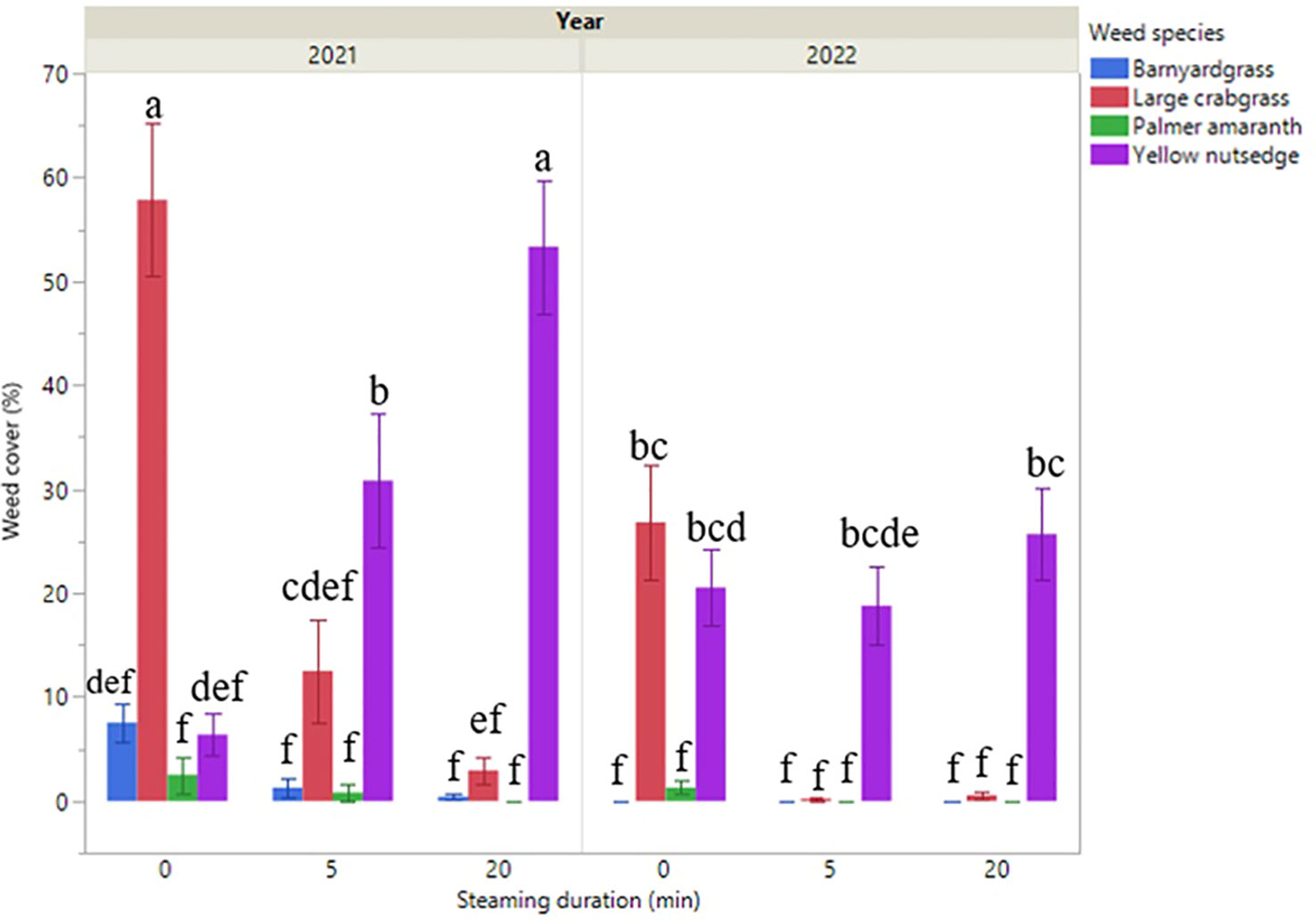
Figure 4 Mean weed cover (%) by weed species under soil steaming treatments of 0, 5, and 20 minutes among the year 2021 and 2022 collected based on the overall plot coverage by each weed species 10 weeks after transplanting (WAT) and rated using the scale 0 to 100%, where 0% is no weed present, and 100% is complete coverage. The error bars indicate the standard error of three measurements. All results were run through LS-method in JMP Pro 16.1 (SAS Institute Inc., Cary, NC, USA). For data that met the assumptions for ANOVA, treatment means were separated using the Tukey’s test at an alpha level of ≤ 0.05. Means followed by the same letter are not significantly different (P < 0.05).
Steaming and mulching on weed cover
Regardless of soil steaming, the non-mulched treatment resulted in the highest weed cover compared to the mulched treatment. The highest weed cover was that of large crabgrass (63%) and yellow nutsedge (52%) at 0 and 20 mins of steaming, respectively, in the absence of mulching. In the presence of mulching, these values were reduced to 23 and 25%, respectively (Figure 5).
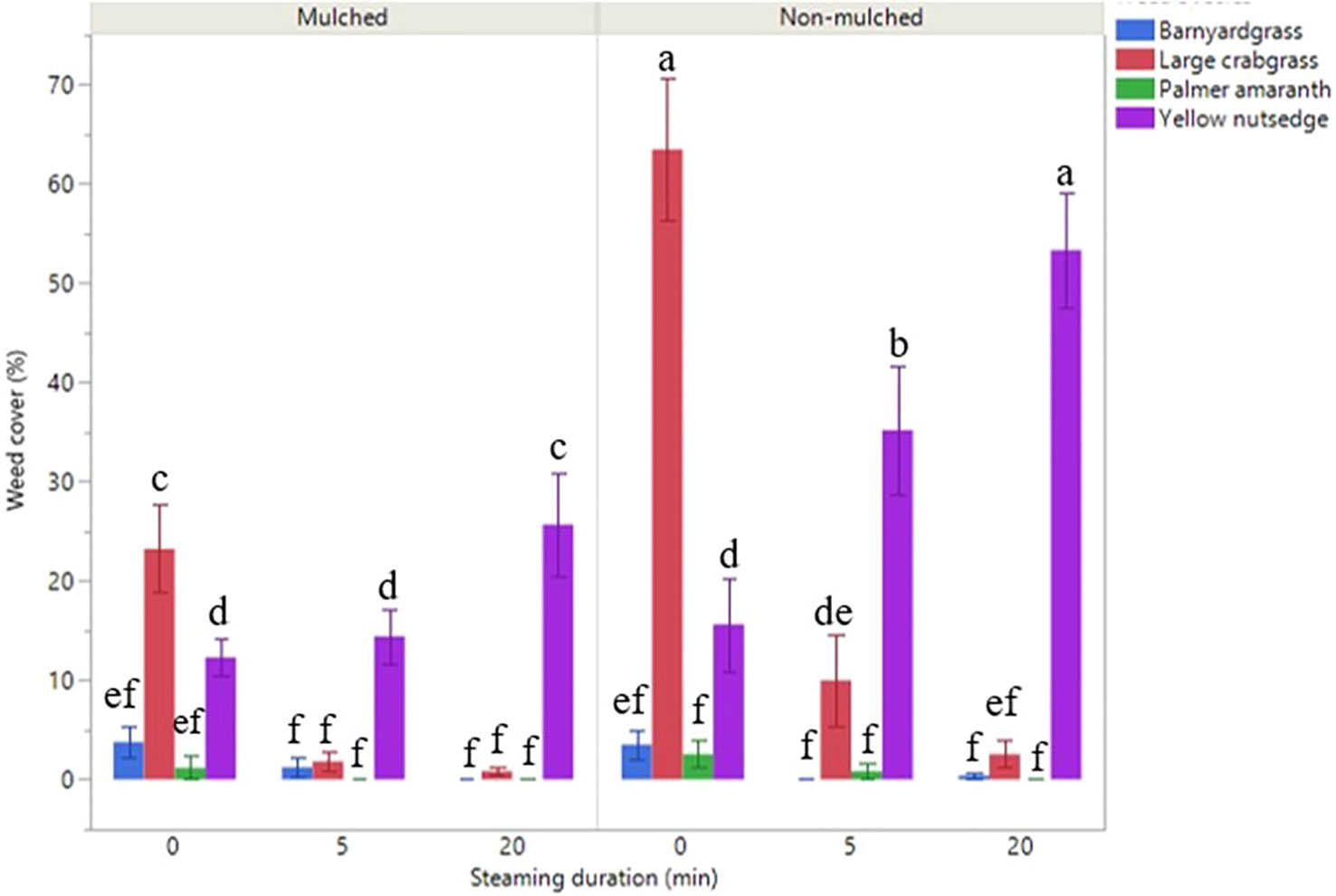
Figure 5 Mean cumulative 2-year weed cover (%) at different combinations of mulching and soil steaming treatment durations (0, 5, and 20 min) collected based on the overall plot coverage by each weed species 10 weeks after transplanting (WAT) and rated using the scale 0 to 100%, where 0% is no weed present, and 100% is complete coverage. The error bars indicate the standard error of three measurements. All results were run through LS-method in JMP Pro 16.1 (SAS Institute Inc., Cary, NC, USA). For data that met the assumptions for ANOVA, treatment means were separated using the Tukey’s test at an alpha level of ≤ 0.05. Means followed by the same letter are not significantly different (P < 0.05).
Yellow nutsedge cover was significantly higher at 5 and 20 minutes of steaming than 0 minutes, regardless of mulching. The presence and absence of mulching at 20 minutes increased the yellow nutsedge cover by 51 and 74%, respectively, compared to 0 minutes of soil steaming (Figure 5). An increase in the duration of soil steaming decreased large crabgrass cover and was lower in the presence of mulching. On the other hand, barnyardgrass and Palmer amaranth cover were not affected by steaming or mulching (Figure 5).
Tomato plant height
None of the parameters and their interactions were significantly different (P < 0.05) with respect to the heights of the tomato plants, except for steaming (Table 2). There was a considerable height increase (P < 0.05) in tomato plants under different steaming durations. At 10 WAT, plants were 12 and 13 cm taller in 5 and 20 min of steaming treatments, respectively, than plants that did not receive any soil steaming (Figure 6).
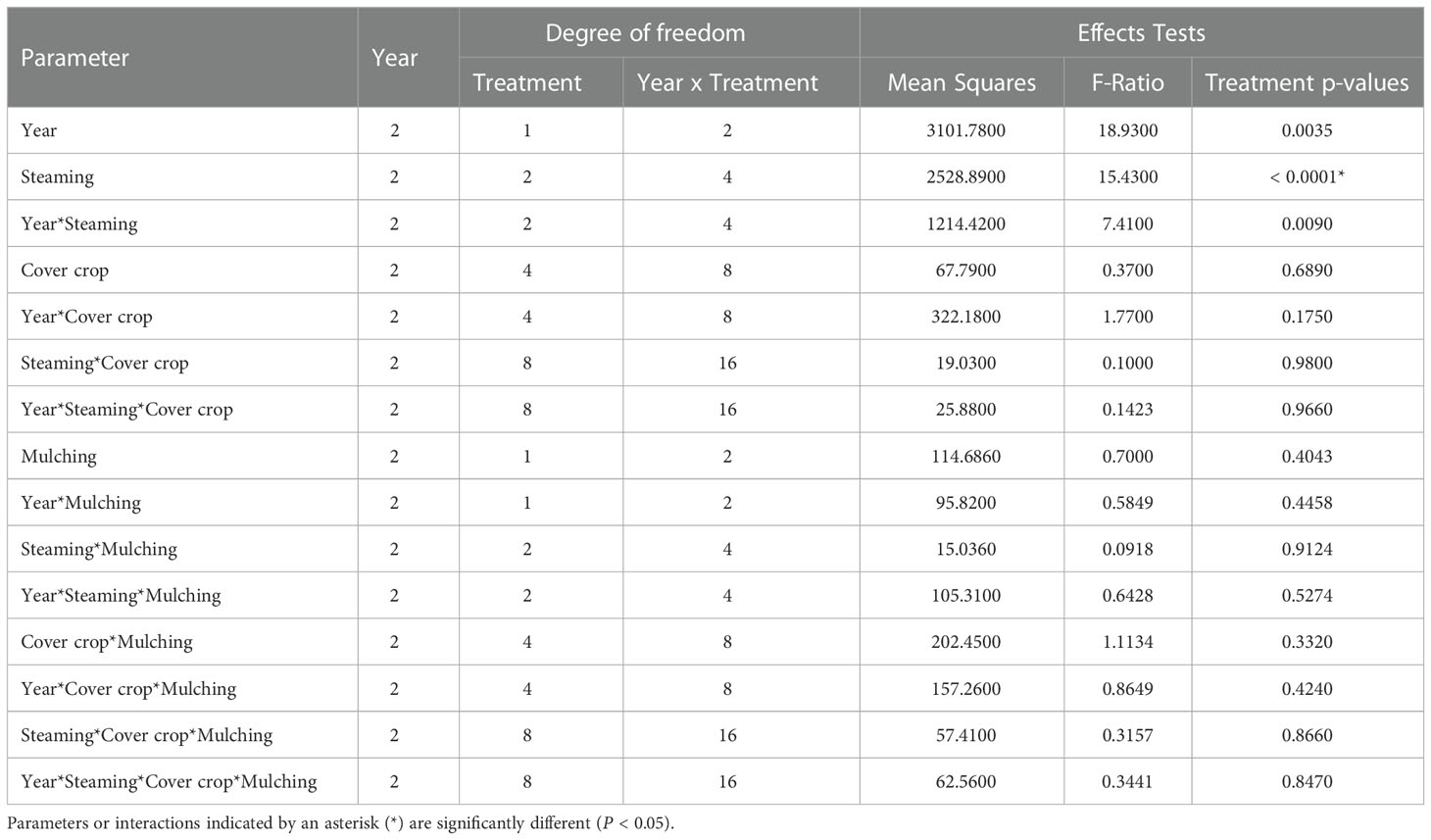
Table 2 Analysis of variance for all the study parameters with respect to tomato plant height (t/ha).
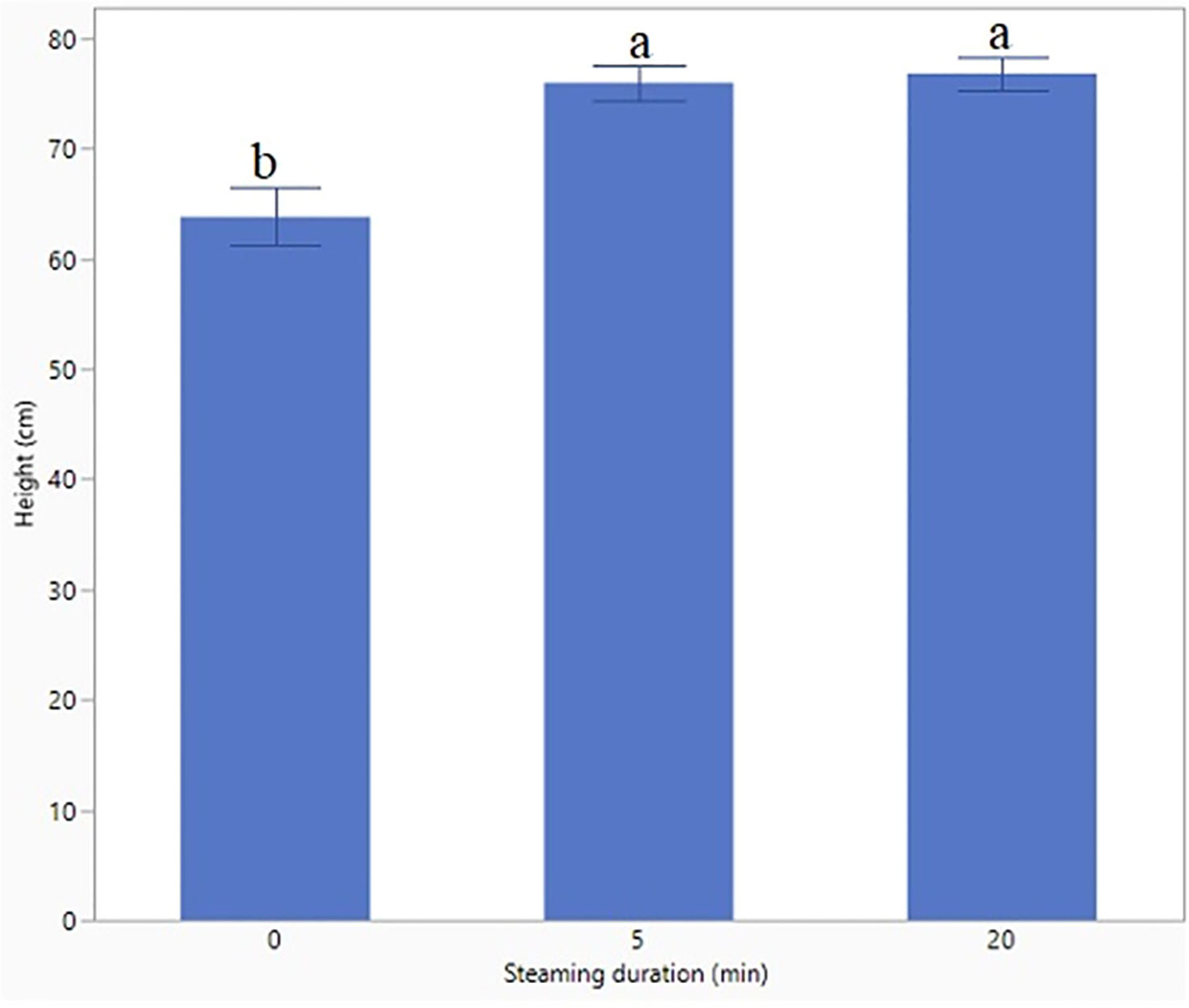
Figure 6 Mean cumulative 2-year height (cm) of tomato plants at 0, 5, and 20 min of soil steaming. The error bars indicate the standard error of three measurements. All results were run through LS-method in JMP Pro 16.1 (SAS Institute Inc., Cary, NC, USA). For data that met the assumptions for ANOVA, treatment means were separated using the Tukey’s test at an alpha level of ≤ 0.05. Means followed by the same letter are not significantly different (P < 0.05).
Tomato yield
The cover crop treatments did not result in yield differences from fallow treatment in both years. However, the interaction between steaming and year was significant (Table 3). The yield was similar regardless of mulching treatment in both years; however, on average, mulched plots produced 10 and 8 t/ha greater yield than non-mulched plots in 2021 and 2022 (Figure 7). Among the mulched and non-mulched treatments in 2021, longer soil steaming duration increased the total yield (P < 0.05). In mulched plots, 5 and 20 min of soil steaming produced 101 and 103 t/ha, respectively, while at 0 min, the yield was only 39 t/ha. Similar results were found in non-mulched plots where 5- and 20-min soil steaming produced 94 and 108 t/ha tomato fruits, which were higher than 0 min of soil steaming (4 t/ha; Figure 8). In 2022, plastic mulching resulted in a significantly higher yield when 5 and 20 min of soil steaming were applied; the yield increased by 58 and 64 t/ha, respectively, compared with 0 min of soil steaming. Additionally, yields in the absence of mulching were significantly higher at 5 and 20 min of soil steaming (54 and 60 t/ha, respectively) than at 0 min of steaming (7 t/ha; Figure 8).
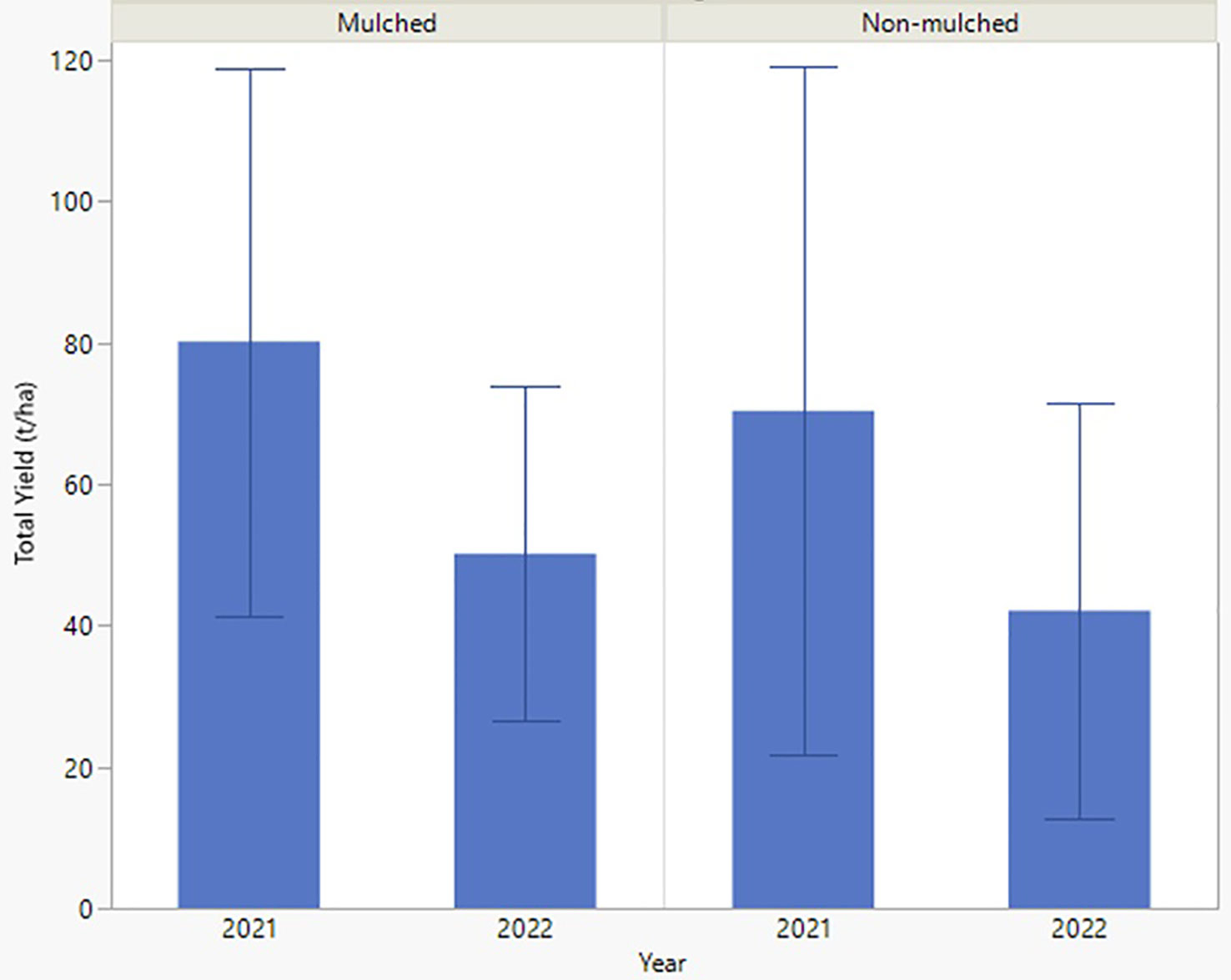
Figure 7 Total yield (t/ha) of marketable and cull fresh fruits in response to plastic mulching and absence of plastic mulch. The error bars indicate the standard error of three measurements. All results were run through LS-method in JMP Pro 16.1 (SAS Institute Inc., Cary, NC, USA). For data that met the assumptions for ANOVA, treatment means were separated using the Tukey’s test at an alpha level of ≤ 0.05. In 2021 and 2022, mean totals were not significantly different (P = 0.35 and 0.20, respectively).
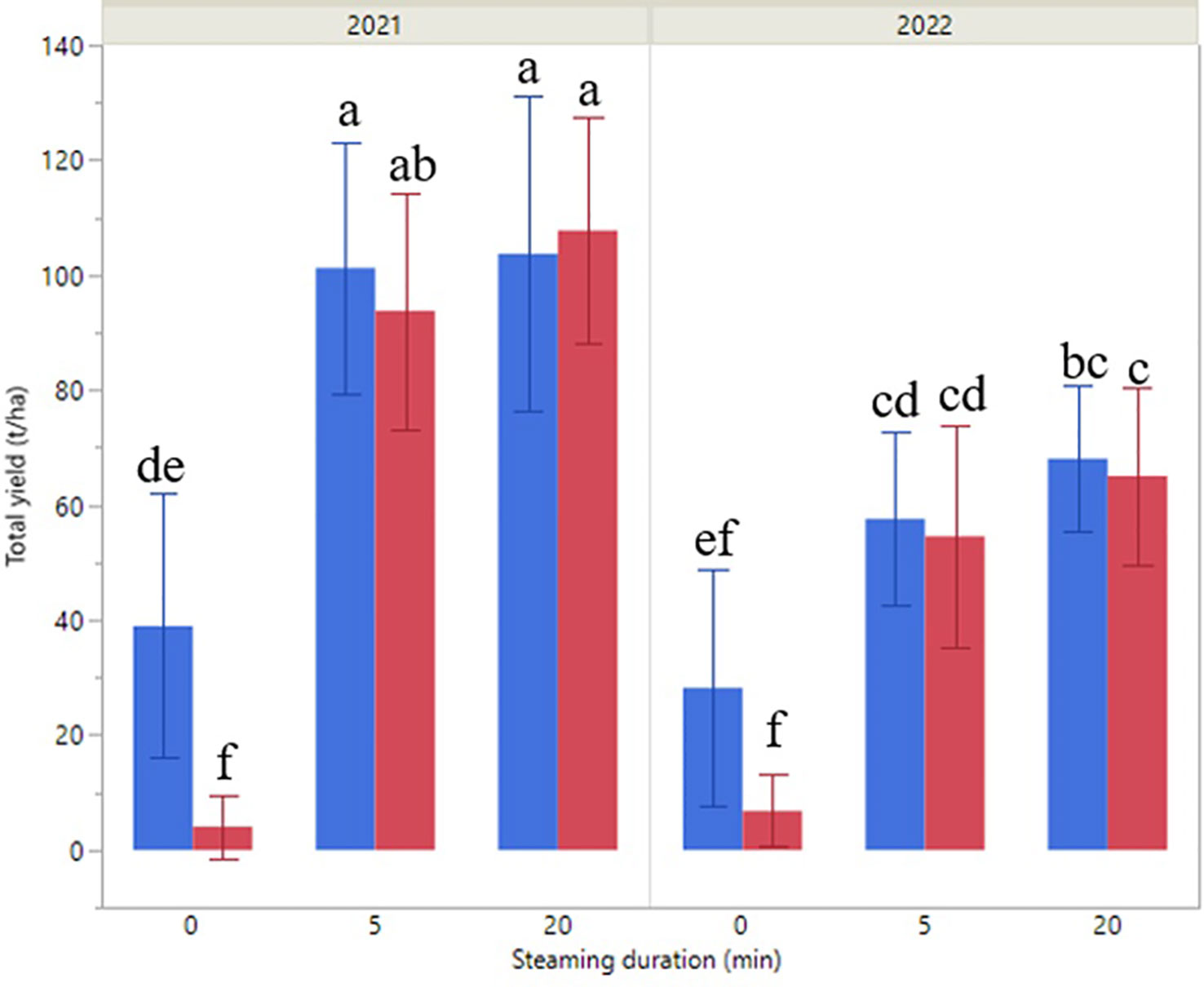
Figure 8 Total tomato yield (t/ha) in response to plastic mulching and different durations of soil steaming. The error bars indicate the standard error of three measurements. All results were run through LS-method in JMP Pro 16.1 (SAS Institute Inc., Cary, NC, USA). For data that met the assumptions for ANOVA, treatment means were separated using the Tukey’s test at an alpha level of ≤ 0.05. In 2021 and 2022, mean totals were significantly different (P < 0.05) compared to 0 min of soil steaming. Means followed by the same letter are not significantly different (P < 0.05).
Marketable fruit yields were significantly greater at 5 and 20 min (30 and 37 t/ha, respectively) than at 0 min (10 t/ha) of soil steaming in mulched treatment. The yield was higher in mulched treatments and was 0.37, 47, and 53 t/ha at 0, 5, and 20 min, respectively, compared to non-mulched (Figure 9). These results are consistent with the total number of cull fruits reported in this experiment. The total number of cull fruits at 5 and 20 min soil steaming was higher than 0 min for both mulched and non-mulched treatment.
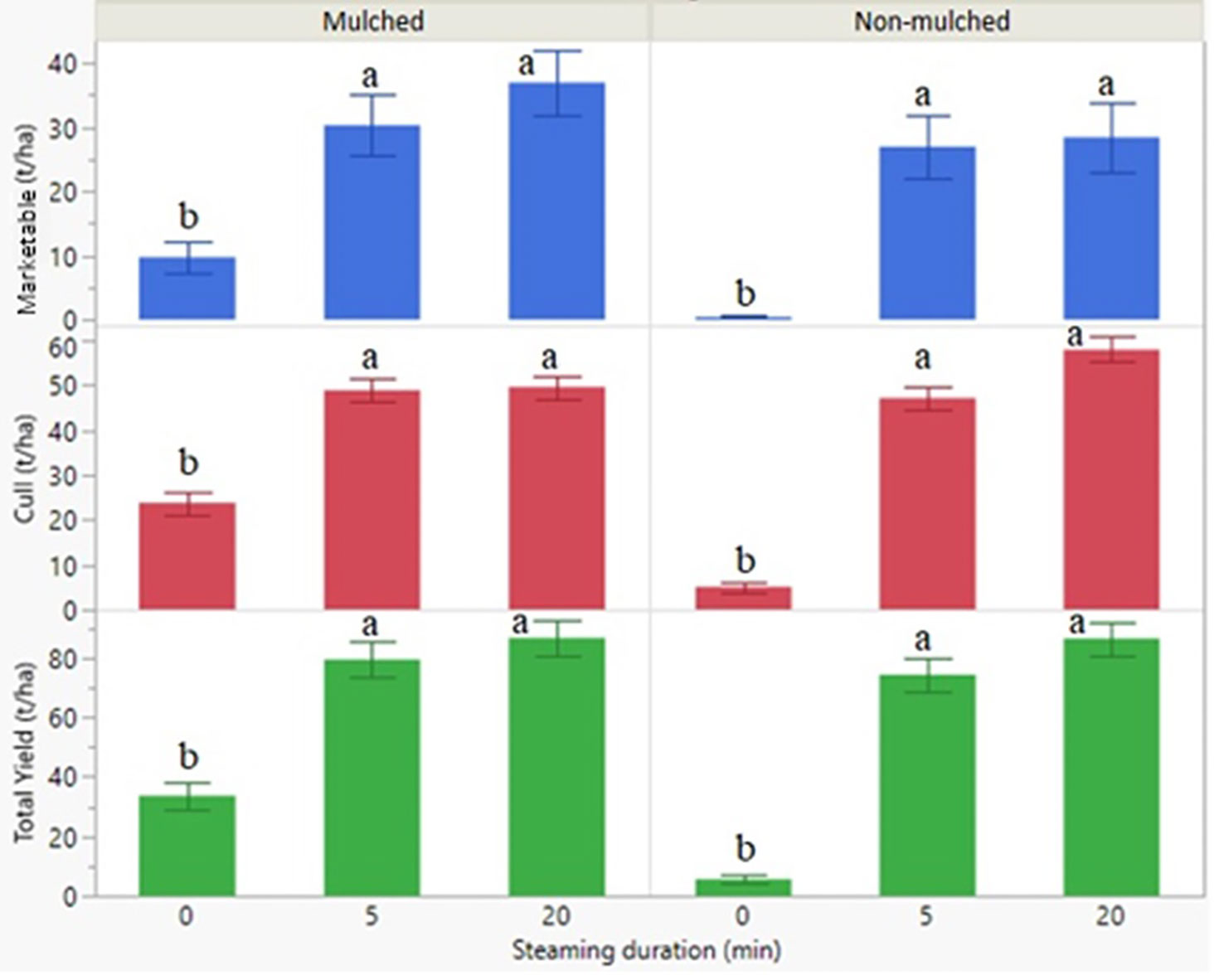
Figure 9 Cumulative marketable, cull, and total fruit yields (t/ha) in response to plastic mulching and different durations of soil steaming. The error bars indicate the standard error of three measurements. All results were run through LS-method in JMP Pro 16.1 (SAS Institute Inc., Cary, NC, USA). For data that met the assumptions for ANOVA, treatment means were separated using the Tukey’s test at an alpha level of ≤ 0.001. Means followed by the same letter are not significantly different (P < 0.05).
Statistical analysis identified significant differences in the marketable, cull, and total tomato yield (P < 0.05). For all treatments that included 5 or 20 min of soil steaming, the marketable, cull, and total yield were significantly greater than the non-steamed treatments (Figure 9).
Discussion
Some weed species are impacted more than others by cover crops. From the present research, the effect of the winter cover crop did not significantly reduce weed populations. Similar results were observed by Mennan et al. (2009), where winter cover crops did not considerably reduce weed cover over the years. Some cover crop species have a lower potential to control weeds due to properties such as seed size, vigor, time of establishment, and weed species characteristics (Lee, 1985; Mennan et al., 2009). On the contrary, some winter cover crops such as wheat and sorghum have been associated with weed suppression, especially in fallow season. Additionally, cover crop biomass is often correlated with weed biomass reduction (Mennan et al., 2009). The lack of weed control in our study may be attributed to cover crop biomass production being inadequate to affect the weeds adversely. The unavailability of cover crop and weed biomass data could be a possible limitation in our study. As such, we cannot confidently attribute insufficient weed control to cover crop biomass. Weed control is one indirect benefit within the many benefits to improve crop production, such as soil and water conservation and nutrient cycling that, in the long term, enhances the ecosystem dynamics (Ghimire et al., 2018).
Plastic mulch to manage weeds positively correlated with effective weed suppression. Mulching resulted in a more than 40% reduction in weed cover. Rajablariani et al. (2012) similarly reported that blue plastic mulch provides a 40% reduction in weed cover. Anzalone et al. (2010) found barley straw, considered a biodegradable mulch, reduced the cover of weeds by up to 40% in tomato. Mulch treatment promoted a significant reduction in yellow nutsedge and large crabgrass cover. Although not significant, mulching was able to reduce the cover of Palmer amaranth. Plastic mulching reduces the cover of most weed species. It is a common production practice in intensive vegetable production systems because it increases soil temperature, improves water management, decreases the growth of different weed species, and improves the use of nutrients in plants (Bond and Grundy, 2001; El-Beltagi et al., 2022).
In most cases, weed control in combination with soil steaming and mulching was better than in the absence of these treatments (Figure 5). Although not statistically different, barnyardgrass and Palmer amaranth showed lower weed cover in the presence of mulch at 5 and 20 minutes of steaming; however, steaming for 20 min resulted in complete weed suppression (100% for barnyardgrass and Palmer amaranth). Data presented in Figure 5 revealed that steaming the soil for 20 min significantly decreased the large crabgrass population for mulched and non-mulched treatment (100 and 96% weed suppression, respectively). Thi Melander and Jorgensen (2005) observed a 90% reduction in weed emergence when the soil temperature reached 61°C. Loenen et al., 2003 showed that steaming treatment at around 60°C for 3 min, followed by 8 min resulted in 100% control of common lambsquarters (Chenopodium album L.), an annual species. Our study also included three annual species: barnyardgrass, Palmer amaranth, and large crabgrass. The combination of mulching and steaming was ineffective against yellow nutsedge at 5 and 20 min. Since the yellow nutsedge plants can easily puncture plastic mulch, this control strategy is not useful in nutsedge control (Brandenberger et al., 2005). Nutsedge tubers cannot survive temperatures of 60°C (Rubin and Benjamin, 1984). Yet, nutsedge has been reported to survive soil temperatures of 80°C for 30 min. A likely explanation is that while shallower tubers cannot survive these temperatures, deeper rhizomes can escape fatal temperatures found in the upper layers of soils being steamed.
The success of the soil steaming usually depends on the temperature, duration of steaming, and the soil depth reached (Gelsomino et al., 2010). Here, the target temperature of 61°C was achieved and maintained for 5 and 20 min in separate treatments at a 4-in depth. Fennimore et al. (2014) found that the profile of weeds susceptible to steam corresponded to their seed coat permeability, morphology, and status of weed seeds (dormancy strategies) which would decrease the germination activity. According to Ascard (1995), the ability to produce new growth from vegetative and reproductive structures could explain the higher tolerance attributed to steaming. Gourd et al. (2002), in his research, found that soil steaming provided poor control (50%) of dandelion (Taraxacum officinale L.) species, which is also a perennial species like yellow nutsedge. In contrast, he showed that steaming effectively controlled (85%) downy brome (Bromus tectorum L.), an annual weed like barnyardgrass, large crabgrass, and Palmer amaranth. He also reported in his research that drought conditions could impact the steaming effectiveness since the steam was applied at three different times under different humidity conditions of 13, 28, and 62%, respectively. The low humidity may have decreased the benefit of steam applications. In general, steaming can effectively suppress numerous annual weed species; but perennials, some large weeds, and a few grass species may be tolerant to this method (Banks and Sandral, 2007). For some weed species, a higher soil temperature reduces seed viability more than the extended duration of steaming (Thompson et al., 1997). This is likely because the deeper roots can escape the hottest steam temperatures. For example, nutsedge tubers can grow several inches below the soil’s surface, likely making it resistant to this method of soil steaming. The results found in the second year of the present experiment showed a higher yellow nutsedge population even in plots that received 20 min of steaming.
The use of mulching had no significant effect on tomato height. However, a significant enhancement was noted at 5 and 20 min of steaming, which resulted in taller plants than plots that were not steamed. The increase in plant height under steaming may be due to a lower weed cover in the plots, reducing resource competition between the crop and weeds, and conserving soil moisture (Hussain et al., 2022). Additionally, soil treated with steaming enhanced nutrient supplementation and nutrient delivery to the crop. For instance, exchangeable and soluble manganese, nitrite, and ammonia were released in the strawberry experiment after treatment with steam above 65°C (Fennimore et al., 2014).
The highest yield of fresh tomato weight per unit area was obtained from 5 and 20 min of soil steaming in both non-mulched and mulched treatments, while the lowest was observed in control plots. Soil steaming, therefore, had the most substantial influence on tomato yield. Zangoueinejad et al., 2018, found that the highest yield per unit area was obtained from a plastic mulch (20.93 kg m-2), while the lowest (1.24 kg m-2) was observed in the control plots without plastic mulch. In onions, yield increased by up to 300% using plastic mulch (Barla and Upasani, 2019). Our results show that the combination of steaming and plastic mulching severely impaired weed competition and increased tomato yield by 136 and 158% at 5 and 20 min, respectively, than 0 min of steaming. The severity of weed competition depends on weed cover, growing conditions, and agricultural practices employed (Zimdahl, 1980; Qasem, 1992). The critical period for weed suppression begins at the flowering stage and goes through fruit ripening (Rahimian and Shahriay, 2002). The presence of weeds in these periods can affect the yield parameters and fruit quality of the crop. Steaming has been reported to increase yield in processing tomato and effectively control weeds such as common lambsquarters (Chenopodium album L.) (Loenen et al., 2003).
A major concern of soil steaming for weed control is the cost. According to Luvisi et al. (2008), applying steaming averages $1,975 per hectare, which can be expensive when the entire field is treated. Although steaming is costly because of its high fuel and labor requirement (Peruzzi et al., 2017), recent studies have explored ways to improve soil steaming methods to reduce costs, such as the band steaming prototype created at the University of Pisa, Italy (Raffaelli et al., 2016; Guerra, 2022). The maximum use of steam in this study resulted in reduced weed biomass (up to 74%) and increased carrot yield compared to untreated plots (Raffaelli et al., 2016). Chemicals to control weeds are generally more cost-effective than non-chemical control methods. Several herbicides are labeled in tomato, such as S-metolachlor, fomesafen, metribuzin (Met), and halosulfuron that are applied as pre-and post-transplant in tomato production. However tomato is sensitive to herbicides such as metribuzin, and may cause severe injury resulting in the fruits becoming unfit for consumption (Kemble 2014; Mohseni-Moghadam and Doohan, 2017). Additionally, improper herbicide applications may result in drift causing environmental concerns and human health risks or destroy the entire crop production with invaluable losses (Hart and Pimentel, 2002; Papadakis et al., 2015; Mac Loughlin et al., 2022).
Conclusion
The integrated use of mulch and steam effectively decreased the weed cover, which resulted in higher tomato yield in these treatments. In particular, the combination of 5 and 20 minutes with plastic mulching was more effective in increasing tomato yield than in the absence of mulching and soil steaming. The use of plastic mulching was effective in reducing most weed species in this study. Additionally, the use of mulch did not show any adverse effect on tomato yield components, while soil steaming improved the tomato yield by up to four times. On the other hand, cover crops did not reduce weed cover compared to the fallow system. Therefore, an integrated approach to mulching combined with soil steaming is recommended for effective weed control.
Although steaming may not be a cost-effective strategy for controlling weeds, it may be more sustainable in the long run. Incorporating soil steaming, cover crops, and mulching may result in the application of lower herbicide rates, which is good for the environment. Lower rates of herbicides will also discourage the selection of herbicide-resistant weed populations, thus decreasing the risk of herbicide-resistance evolution. For future research, it will be crucial to measure additional parameters such as weed biomass, cover crop biomass, weed and tomato photosynthetic efficiencies, and soil properties to further assess the association of weed cover reduction with these parameters, if any.
Data availability statement
The original contributions presented in the study are included in the article/supplementary material. Further inquiries can be directed to the corresponding author.
Author contributions
TT and SB designed the experiment. TO, BB, and AS conducted the experiment. AM, KB, VS, WS, JA, assisted with taking plant measurements; TO, SB, and TT conducted the data analysis; TO and TT wrote the original version of this paper. TT, SB, and TO provided technical expertise. All authors contributed to the article and approved the submitted version.
Funding
Funding for this project was provided by the Specialty Crop Block Grant sponsored by the Mississippi Department of Agriculture and Commerce/U.S. Department of Agriculture – Agriculture Marketing Service, award #CTD 10/8/19. This material is based upon work supported by the National Institute of Food and Agriculture, US Department of Agriculture, Hatch projects under accession numbers 230100 and 1023679.
Acknowledgments
The authors thank Clay Cheroni, Varsha Singh, Antonio Augusto Correa Tavares, Pawan Kumar, Ncomiwe Maphalala, Worlanyo Segbefia, Ziming Yue, Josiane Argenta, and Alyssa Miller for their assistance throughout the research.
Conflict of interest
The authors declare that the research was conducted in the absence of any commercial or financial relationships that could be construed as a potential conflict of interest.
Publisher’s note
All claims expressed in this article are solely those of the authors and do not necessarily represent those of their affiliated organizations, or those of the publisher, the editors and the reviewers. Any product that may be evaluated in this article, or claim that may be made by its manufacturer, is not guaranteed or endorsed by the publisher.
References
Anzalone A., Cirujeda A., Aibar J., Pardo G., Zaragoza C. (2010). Effect of biodegradable mulch materials on weed control in processing tomatoes. Weed Technology. 24 (3), 369–377. doi: 10.1614/WT-09-020.1
Akhtar K., Wang W., Ren G., Khan A., Feng Y., Yang G. (2018). Changes in soil enzymes, soil properties, and maize crop productivity under wheat straw mulching in guanzhong, China. Soil Tillage Res. 182, 94–102.
Ascard J. (1995). Effects of flame weeding on weed species at different developmental stages. Weed Res. 35 (5), 397–411. doi: 10.1111/j.1365-3180.1995.tb01636.x
Baldoin C., Sartorato I., Friso D., De Zanche C., De Paoli F., Bietresato M. (2010). Weed control by water steam using a self-propelled machine equipped with a condensation chamber. Proc. XVIIth World Congr. Intl. Commission Agr. Biosyst. Eng.(CIGR). Québec City QC Canada. 13, 17.
Banks J., Sandral G. (2007). Report on weed control using hot water/steam and herbicdes in the city of joondalup. Available at: http://api.joondalup.wa.gov.au/files/councilmeetings/2007/attach11brf210807.pdf.
Barla S., Upasani R. R. (2019). Study on different methods of weed management in onion (Allium cepa l.) 33, 1–7. doi: 10.9734/cjast/2019/v33i330079
Bitarafan Z., Kaczmarek-Derda W., Berge T. W., Tørresen K. S., Fløistad I. S. (2022). Soil steaming to disinfect barnyardgrass-infested soil masses. Weed Technology. 36 (1), 177–185. doi: 10.1017/wet.2021.107
Bond W., Grundy A. C. (2001). Nonchemical weed management in organic farming systems. Weed Res. 41, 383–405. doi: 10.1046/j.1365-3180.2001.00246.x
Brandenberger L. P., Talbert R. E., Wiedenfeld R. P., Shrefler J. W., Webber C. L., Malik M. S. (2005). Effects of halosulfuron on weed control in commercial honeydew crops. Weed Technology. 19 (2), 346–350.
Brust G. E. (2019). “Chapter 9 - management strategies for organic vegetable fertility,” in Safety and practice for organic food. Eds. Biswas D., Micallef S. A. (Academic Press), 193–212, ISBN: ISBN 9780128120606. doi: 10.1016/B978-0-12-812060-6.00009-X
Cornelius C. D., Bradley K. W. (2017). Influence of various cover crop species on winter and summer annual weed emergence in soybean. Weed Technology. 31 (4), 503–513. doi: 10.1017/wet.2017.23
El-Beltagi H. S., Basit A., Mohamed H. I., Ali I., Ullah S., Kamel E. A., et al. (2022). Mulching as a sustainable water and soil saving practice in agriculture: a review. Agronomy 12 (8), 1881. doi: 10.3390/agronomy12081881
Fennimore S. A., Martin F. N., Miller T. C., Broome J. C., Dorn N., Greene I. (2014). Evaluation of a mobile steam applicator for soil disinfestation in California strawberry. HortScience 49 (12), 1542–1549. doi: 10.21273/HORTSCI.49.12.1542
Fennimore S. A., Goodhue R. E. (2016). Soil disinfestation with steam: A review of economics, engineering, and soil pest control in California strawberry. Int. J. Fruit Science. 16 (sup1), 71–83.
Fennimore S. A., Slaughter D. C., Siemens M. C., Leon R. G., Saber M. N. (2016). Technology for automation of weed control in specialty crops. Weed Technology. 30 (4), 823–837. doi: 10.1614/WT-D-16-00070.1
Finney D. M., Murrell E. G., White C. M., Baraibar B., Barbercheck M. E., Bradley B. A., et al. (2017). Ecosystem services and disservices are bundled in simple and diverse cover cropping systems. Agric. Environ. Letters. 2 (1), 170033. doi: 10.2134/ael2017.09.0033
Garvey P. V., Meyers S. L., Monks D. W., Coble H. D. (2013). Influence of palmer amaranth (Amaranthus palmeri) on the critical period of weed control in plasticulture-grown tomato. Weed Technol. 27, 165–170. doi: 10.1614/WT-D-12-00028.1
Gelsomino A., Petrovičová B., Zaffina F., Peruzzi A. (2010). Chemical and microbial properties in a greenhouse loamy soil after steam disinfestation alone or combined with CaO addition. Soil Biol. Biochem. 42 (7), 1091–1100.
Ghimire R., Ghimire B., Mesbah A. O., Idowu O. J., O’Neill M. K., Angadi S. V., et al. (2018). Current status, opportunities, and challenges of cover cropping for sustainable dryland farming in the southern great plains. J. Crop Improvement. 32 (4), 579–598. doi: 10.1080/15427528.2018.1471432
Giles D. K., Downey D., Slaughter D. C., Brevis-Acuna J. C., Lanini W. T. (2004). Herbicide micro-dosing for weed control in field-grown processing tomatoes. Appl. Eng. Agriculture. 20 (6), 735.
Gordon G. G., Foshee III W.G., Reed S. T., Brown J. E., Vinson E., Woods F. M. (2008). Plastic mulches and row covers on growth and production of summer squash. Int. J. Veget. Sci. 14, 322–338. doi: 10.1080/19315260802215830
Gourd T., Ferrell T., Organic P. B., Market P. Y. (2002). Controlling weeds using propane generated flame and steam treatments in crop and non croplands (Santa Cruz, Calif, USA: Organic Farming Research Foundation).
Grubinger V. Winter rye: a reliable cover crop. university of Vermont extension. Available at: http://www.uvm.edu/vtvegandberry/factsheets/winterrye.Html (Accessed September 5, 2022).
Guerra N. (2022). Banded steaming for weed and disease control in California vegetables (University of California, Davis: Doctoral dissertation).
Hart K., Pimentel D. (2002). Public health and costs of pesticides. Encyclopedia Pest management. 1, 677–679. doi: 10.1201/NOE0824706326.ch313
Hussain M., Abbas Shah S. N., Naeem M., Farooq S., Jabran K., Alfarraj S. (2022). Impact of different mulching treatments on weed flora and productivity of maize (Zea mays l.) and sunflower (Helianthus annuus l.). PloS One 17 (4), e0266756. doi: 10.1371/journal.pone.0266756
Kemble J. M., Quesada-Ocampo L. M., Ivors K. L., Jennings K. M., Walgenbach J. F.. Southeastern US 2014 vegetable crop handbook.
Kenney I., Blanco-Canqui H., Presley D. R., Rice C. W., Janssen K., Olson B. (2015). Soil and crop response to stover removal from rainfed and irrigated corn. GCB Bioenergy. 7 (2), 219–230.
Lee W. O. (1985). Clover science and technology Vol. 25 (Madison, WI, USA: Agronomy Society of America), 295–308.
Loenen M. C. A., Turbett Y., Mullins C. E., Feilden N. E. H., Wilson M. J., Leifert C., et al. (2003). Low temperature–short duration steaming of soil kills soil-borne pathogens, nematode pests and weeds. Eur. J. @ Plant Pathol. 9, 993–1002. doi: 10.1023/b:ejpp.0000003830.49949.34
Luvisi A., Triolo E., Materazzi A. (2008). Control of soil-borne diseases in tomato by use of steam and an exothermic reaction. Control Soil-Borne Dis. Tomato by Use Steam an Exothermic Reaction., 1000–1008.
Mac Loughlin T. M., Peluso M. L., Marino D. J. (2022). Multiple pesticides occurrence, fate, and environmental risk assessment in a small horticultural stream of Argentina. Sci. Total Environment. 802, 149893. doi: 10.1016/j.scitotenv.2021.149893
Manisankar G., Gosh P., Malik G. C., Banerjee M. (2022) Recent trends in chemical weed management: A review The Pharma Innovation 11, 745–53.
Marín-Guirao J. I., Martín-Expósito E., García-García M. D., de Cara-García M. (2022). Alternative mulches for sustainable greenhouse tomato production. Agronomy 12 (6), 1333. doi: 10.3390/agronomy12061333
Melander B., Jorgensen M. H. (2005). Soil steaming to reduce intrarow weed seedling emergence. Weed rese 45, 202–211. doi: 10.1111/j.1365-3180.2005.00449.x
Mennan H., Ngouajio M., Isık D., Kaya E. (2009). Effects of alternative winter cover cropping systems on weed suppression in organically grown tomato (Solanum lycopersicum). Phytoparasitica 37 (4), 385–396. doi: 10.1007/s12600-009-0048-1
Mohseni-Moghadam M., Doohan D. (2017). Fomesafen crop tolerance and weed control in processing tomato. Weed technology. 31 (3), 441–446. doi: 10.1017/wet.2016.30
Monaco T. J., Grayson A. S., Sanders D. C. (1981). Influence of four weed species on the growth, yield, and quality of direct-seeded tomatoes (Lycopersicon esculentum). Weed Sci. 29, 394–397. doi: 10.1017/S0043174500039874
Monteiro A., Santos S. (2022). Sustainable approach to weed management: The role of precision weed management. Agronomy. 12 (1), 118.
Naeem M., Minhas W. A., Hussain S., Ul-Allah S., Farooq M., Farooq S., et al. (2022). Barley-based cropping systems and weed control strategies influence weed infestation, soil properties and barley productivity. Agriculture 12 (4), 487. doi: 10.3390/agriculture12040487
Papadakis E. N., Vryzas Z., Kotopoulou A., Kintzikoglou K., Makris K. C., Papadopoulou-Mourkidou E. (2015). A pesticide monitoring survey in rivers and lakes of northern Greece and its human and ecotoxicological risk assessment. Ecotoxicology Environ. Safety. 116, 1–9. doi: 10.1016/j.ecoenv.2015.02.033
Peruzzi A., Martelloni L., Frasconi C., Fontanelli M., Pirchio M., Raffaelli M. (2017). Machines for non-chemical intra-row weed control in narrow and wide-row crops: a review. J. Agric. Engineering. 48 (2), 57–70.
Qasem J. R. (1992). Pigweed (Amaranthus spp.) interference in transplanted tomato (Lycopersicon esculentum). J. Hortic. Sci. 67, 421–427. doi: 10.1080/00221589.1992.11516267
Raffaelli M., Martelloni L., Frasconi C., Fontanelli M., Carlesi S., Peruzzi A. (2016). A prototype band-steaming machine: design and field application. Biosyst. Engineering. 144, 61–71. doi: 10.1016/j.biosystemseng.2016.02.001
Rajablariani H. R., Hassankhan F., Rafezi R. (2012). Effect of colored plastic mulches on yield of tomato and weed biomass. Int. J. Environ. Sci. Dev. 3 (6), 590.
Rahimian S. H., Shahriay D. (2002). Determination of the critical period of weed control in tomato. Z. fur Pflanzenkrankheiten und Pflanenschutz 18, 511–518.
Rubin B., Benjamin A. (1984). Solar heating of the soil: involvement of environmental factors in the weed control process. Weed Science. 32 (1), 138–142. doi: 10.1017/S0043174500058653
Samtani J. B., Ajwa H. A., Weber J. B., Browne G. T., Klose S., Hunzie J., et al. (2011). Evaluation of non-fumigant alternatives to methyl bromide for weed control and crop yield in California strawberries (Fragaria ananassa l.). Crop Prot. 30, 45–51. doi: 10.1016/j.cropro.2010.08.023
Schulz M., Marocco A., Tabaglio V., Macias F. A., Molinillo J. M. (2013). Benzoxazinoids in rye allelopathy-from discovery to application in sustainable weed control and organic farming. J. Chem. ecology. 39 (2), 154–174. doi: 10.1007/s10886-013-0235-x
Sievers T., Cook R. L. (2018). Aboveground and root decomposition of cereal rye and hairy vetch cover crops. Soil Sci. Soc. America J. 82 (1), 147–155. doi: 10.2136/sssaj2017.05.0139
Sunday I. N., Olafimihan O. W. (2022). Marketing channels and marketing margin analysis of tomato retailing in two selected markets within kaduna metropolis, kaduna state, and Nigeria. RJOAS: Russian J. Agric. Socio-Economic Sci. 1 (121). doi: 10.18551/rjoas.2022-01.14
Teasdale J. R., Brandsaeter L. O., Calegari A. D., Neto F. S., Upadhyaya M. K., Blackshaw R. E. (2007). “Cover crops and weed management,” in Non chemical weed management principles. concepts and technology (Wallingford, UK: CABI), 49–64.
Thompson A. J., Jones N. E., Blair A. M. (1997). The effect of temperature on viability of imbibed weed seeds. Ann. Appl. Biol. 130 (1), 123–134.
United States Department of Agriculture (2019) Economics, statistics and market information systems. Available at: https://downloads.usda.library.cornell.edu/usdaesmis/files/02870v86p/gm80j322z/5138jn50j/vegean19.pdf.
Webster T. M. (2014). Weed survey–southern states: vegetable, fruit and nut crops subsection. Proc. South. Weed Sci. Soc 55, 237–258.
Zangoueinejad R., Kazemeini S. A., Ghadiri H., Javanmardi J. (2018). Effects of non-living mulches and metribuzin on yield and yield components of tomato (Lycopersicon escolentum cv. CH). Iran Agric. Res. 37, 43–48. doi: 10.22099/IAR.2018.4678
Zhang X. L., Zhao Y. Y., Zhang X. T., Shi X. P., Shi X. Y., Li F. M. (2022). Re-used mulching of plastic film is more profitable and environmentally friendly than new mulching. Soil Tillage Res. 216, 105256. doi: 10.1016/j.still.2021.105256
Keywords: sustainable agriculture, organic farming, weed control, pest management, integrated weed management, non-chemical
Citation: de Oliveira TR, Serafim AD, Breland B, Miller A, Beneton K, Singh V, Segbefia W, Argenta JC, Broderick SR and Tseng TM (2023) An integrated weed management approach in tomato using soil steaming, mulching, and winter cover crops. Front. Agron. 5:1075726. doi: 10.3389/fagro.2023.1075726
Received: 20 October 2022; Accepted: 14 April 2023;
Published: 05 June 2023.
Edited by:
Stéphane Cordeau, INRA UMR1347 Agroécologie, FranceReviewed by:
Doğan Işik, Erciyes University, TürkiyeStefano Carlesi, Sant’Anna School of Advanced Studies, Italy
Daniele Antichi, University of Pisa, Italy
Copyright © 2023 de Oliveira, Serafim, Breland, Miller, Beneton, Singh, Segbefia, Argenta, Broderick and Tseng. This is an open-access article distributed under the terms of the Creative Commons Attribution License (CC BY). The use, distribution or reproduction in other forums is permitted, provided the original author(s) and the copyright owner(s) are credited and that the original publication in this journal is cited, in accordance with accepted academic practice. No use, distribution or reproduction is permitted which does not comply with these terms.
*Correspondence: Te Ming Tseng, dC50c2VuZ0Btc3N0YXRlLmVkdQ==