- 1Department of Crop and Soil Sciences, Lilongwe University of Agriculture and Natural Resources, Lilongwe, Malawi
- 2Department of Trait Diversity and Function, Royal Botanic Gardens, Kew Green, Richmond, United Kingdom
- 3Natural Resources Institute, University of Greenwich, Chatham Maritime, United Kingdom
Introduction: Fall armyworm continues to disrupt smallholder farming systems across sub-Saharan Africa, with sporadic outbreaks and chronic cereal crop losses. Smallholders have been adapting to the pest by increasing crop surveillance for targeted control measures and developing low-cost solutions. For example, some report placing soil or ash in maize whorls where the mechanism of pest control may be suffocation, abrasion leading to desiccation, or through the introduction of soil-borne entomopathogens.
Methods: To verify the efficacy of this approach we evaluated different soil types on maize infested with fall armyworm to assess their efficacy. We also evaluated the efficacy of pesticidal plant species, powdered and placed in leaf whorls to control fall armyworm.
Results and discussion: Different United States Department of Agriculture-characterised soil types (sand, loam, clay) and wood ash were effective in reducing the number of larvae and maize leaf damage by approximately 50%. Maize yield with the synthetic control (chlorpyriphos) was 13,700 kg/ha, which was 42% higher than the untreated control (7,900 kg/ha). Soil and ash treatments yields between 10,400 to 12,400 kg/ha were 24-36% higher than the untreated control. Dry soil applied after watering was most effective regardless of soil type. However, wet soil treatments applied before watering were also highly effective in reducing the number of fall armyworm larvae and reducing insect damage to maize leaves. Botanical powders from Azadirachta indica, Nicotiana tabacum, Cymbopogon citratus and Lippia javanica were also effective when applied to maize leaf whorls. Plant powder treatments and water extracts were significantly effective in reducing the number of larvae and leaf damage. The highest yield obtained with botanicals was observed with A. indica powder (5,600 kg/ha), C. citratus extract (5,800 kg/ha) and N. tabacum extract (5,800 kg/ha), where the synthetic treatment yield was 6,900 kg/ha and the untreated yield was 1,700 kg/ha. We conclude that smallholder farmer innovations in managing fall armyworm are effective low-cost options. Scientific validation of soil treatments and botanicals should help increase the confidence of policy makers and allow knowledge extension services to recommend their use to smallholder farmers, which in turn may reduce reliance on imported synthetic pesticides and improve farmer resilience, circular economies and human and environmental health.
Introduction
Fall armyworm (Spodoptera frugiperda J E Smith, Lepidoptera: Noctuidae) (FAW) arrived in West Africa from its native New World range in 2016 and is now widely established across the African continent (Goergen et al., 2016). Due to decades of synthetic pesticide mis-use and over-use in the Americas, FAW is resistant to many common commercial insecticides (Yu, 1991). FAW pest impacts have been compounded through its disruption to African ecosystems while taking advantage of often degraded agricultural landscapes where low-input smallholder farming systems have reduced resilience to FAW invasion (Hruska, 2019). Agronomic practices to manage FAW on cereal crops, such as the use of push-pull systems (Midega et al., 2018; Cheruiyot et al., 2021) and strengthening ecosystem services such as soil fertility and landscape biodiversity have been studied (Harrison et al., 2019). Agroecological practices including the use of local pesticidal plants (Sisay et al., 2019; Phambala et al., 2020; Rioba and Stevenson, 2020), commercial botanical pesticides (Bateman et al., 2021; Ahissou et al., 2022) and microbial biocontrol products (Guo et al., 2020) have also received considerable attention for FAW management. These practices are perceived as sustainable alternatives to synthetic pesticides that are known to have a high cost to the environment and human health (Sharma et al., 2020; Andersson and Isgren, 2021; Rani et al., 2021). These agroecological approaches require different levels of farmer investment in terms of labour and finance and are often knowledge intense. Improving soil organic matter and fertility is a longer term endeavour, whereas using a botanical pesticide may provide locally available and potentially immediate benefit. As FAW is often perceived in the context of crisis management, smallholder farmers are often looking for quick and economic solutions to deal with an immediate threat to their livelihoods (Lunt et al., 2018; Constantine et al., 2020).
While there are reports of smallholder farmers adapting to FAW including low-cost and sustainable options to manage the pest (Kumela et al., 2019; Tambo et al., 2020; Asare-Nuamah, 2022), the use of high-cost synthetic pesticides remains the main method of control (Abro et al., 2021; Yang et al., 2021). This is particularly concerning within a context of widespread FAW insecticide resistance, driving smallholder farmers to use some of the more toxic or restricted pesticides (Donald et al., 2016; Nyamutukwa et al., 2022). One low-cost option that has been reported anecdotally in some reviews (Hruska, 2019; Kumela et al., 2019) is the use of sand or ash placed in the leaf whorl of maize plants, but evidence for its efficacy is limited and inconclusive (Babendreier et al., 2020). The mechanism by which soil could control FAW could be similar to the well-established practice of using diatomaceous earths (Aniwanou et al., 2020; Zeni et al., 2021), acting as an abrasive and desiccant to FAW larvae. As soils can contain many micro-organisms, it has been postulated (FAO, 2018) that using soil for FAW management may also distribute entomopathogens such as Bacillus thuringiensis, Beauveria bassiana or polyhedral viruses known to be present in soils (Valicente and Barreto, 2003; Ramirez-Rodriguez and Sánchez-Peña, 2016). Considering the use of this indigenous practice for FAW control, our research was designed to address this knowledge gap, systematically evaluating different soil types for efficacy as well as assessing methodological issues such as whether the soil is dry or wet, where efficacy could be potentially impacted by the timing of application and rainfall patterns. Furthermore, we wanted to investigate whether similar principles to adding ash or soil to leaf whorls could be applied to the use of botanical powders, potentially acting as a physical abrasive similar to soils as well as a toxin or deterrent. Using a botanical powder could reduce the amount of plant material required, and potentially have dual physical and chemical properties that improve efficacy against FAW.
Materials and methods
Study site
The field sites used for the botanical and soil application maize cropping trials were located on the Bunda campus farm of Lilongwe University of Agriculture and Natural Resources in Mitundu, Lilongwe District, Malawi (Latitude 14°11′S Longitude 33°46′E). The location is at an elevation of 1,100 m above sea level with a mean annual rainfall of 700mm, mean maximum temperature of 29°C and mean minimum temperature of 17°C. Field trials were carried out during the 2020-2021 rainy season (Dec 2020 to May 2021). A further enclosed screenhouse trial on the Bunda campus was carried out to assess the impact of the moisture content of different soil types and the timing of soil treatment applications on FAW survival and crop damage to maize.
Botanical pesticides
Four plant species (Azadirachta indica, Nicotiana tabacum, Cymbopogon citratus, Lippia javanica) with well-known pesticidal properties against various crop pests (Mkindi et al., 2017; Sarker and Lim, 2018; Phambala et al., 2020; Kilani-Morakchi et al., 2021) were selected for evaluation against FAW. Fresh leaves were collected from several locations around Mitundu, Lilongwe District where these species are commonly available. Harvested leaves were shade dried and ground to a fine powder using a pestle and mortar, and the powders were kept in a cool dark place until required. Application of the plant treatments was either through applying the ground plant leaf powder directly to maize leaf whorls or extracting the plant powder in water. Extracts were made by adding 100 g of plant species powder to 1 l of water containing 0.1% liquid soap (Tembo et al., 2018). The plant material was left to extract for 24 h in a cool dark place, followed by sieving to filter out the plant material; thereby producing a 10% w/v extract to immediately apply to maize plants using a knapsack sprayer. Extracts were applied using a knapsack sprayer to leaf whorls because this is the location of FAW larval infestations in maize. Plant powders were also applied to each leaf whorl but using a small teaspoon (approx. 3 g/whorl).
We used ten treatments: 4 botanical extracts, 4 botanical powders, a positive control using the synthetic chlorpyriphos and negative untreated control. Chlorpyriphos was applied following recommended application rates (480 g/l EC, 200 ml/ha). A Completely Randomized Block Design was used with four replicates. Replicated plot sizes were 5 m by 5 m with a 2 m space between replicates and plots. The maize variety MH34 was used with a spacing of 75 cm between rows and 25 cm between plants within a row, with one seed planted per hill. The plots were fertilized with NPK (23:10:5 + 6S + 1Zn) for basal dressing, and urea was used for top dressing at four weeks after planting at a rate of 100 kg/ha following standard agricultural practices in the area. When FAW infestation levels reached 20% of plants infested, botanical extracts and powders were applied weekly in the maize whorl using a small spoon for the plant powders, and a knapsack sprayer for the extracts and positive control. After each treatment, the sprayer was washed with soapy water and rinsed twice with plain water. The day before each weekly treatment semi-destructive and non-destructive sampling of maize plants was done to assess the presence of FAW larvae and damage, with data collected over nine consecutive weeks. In each plot ten maize plants were randomly selected. The selected plants were first non-destructively assessed for leaf damage caused by feeding larvae, where damage was scored using the Williams’ 0–9 whole plant damage scale (Toepfer et al., 2021). The plants were then semi-destructively sampled by pulling apart damaged leaf whorls to count the number of larvae per plant. At harvest, the maize grain yield per plot was collected and converted to the standard kg/ha.
Soil applications
Ash from wood fires was collected from households around the village of Mitundu. The wood ash was from a variety of Brachystegia species which was mixed, dried, sieved and kept in dark, dry conditions until use. Different soil textural classes (sand, loam, clay) were collected from locations around Bunda college campus, Lilongwe District. The soils were dried and classified using the United States Department of Agriculture soil classification system (USDA Soil Science Staff, 2017). Each soil type was kept in dark, dry conditions until use.
Trial design involved four experimental treatments (ash, sand, loam, clay) as well as positive (chlorpyriphos) and negative (untreated) control treatments. A Completely Randomized Block Design was used with four replicates. Replicated plot sizes were 5 m by 5 m with a 2 m space between replicates and plots. The maize variety and agronomic practices were the same as in the botanical treatment trial. When FAW infestation levels reached 20% of plants infested, the ash and soil treatments were applied weekly using a small spoon to add ash or soil type (approx. 3 g) to each leaf whorl on the maize plant. Chlorpyriphos was applied following recommended application rates (480 g/l EC, 200 ml/ha) using a knapsack sprayer. Data collection was over nine consecutive weeks for maize leaf damage and FAW numbers, with maize crop yield data collected at harvest using the same protocols as described in the botanical treatment trial.
Assessment of soil moisture and timing of application
The impact of moisture and the timing of application on the efficacy of soil was determined in a screenhouse trial to manage environmental variability and FAW infestation levels. A split plot design was used to incorporate three parameters where the first parameter was soil type (sand, loam, clay), with further parameters of soil moisture status (dry soil or wet soil) when the soil is applied to the whorl and the timing of when the soil is applied (before or after watering the maize plants), with positive (chlorpyriphos) and negative (untreated) controls, four replicates per treatment. Maize variety MH34 was planted in plastic pots (10 l) in a screen house located at the Bunda campus farm. Pots were filled with loamy soil, and five maize seeds were planted per pot. Thinning was done to three seedlings per pot two weeks after emergence. The maize pots were fertilized with NPK (23:10:5 + 6S + 1Zn) for basal dressing, while urea was used for top dressing at four weeks after planting at a rate of 100 kg/ha. Hand weeding was done as weeds emerged and pots were watered equally by hand with a watering can. Twenty days after seedling emergence, each plant was infested with five third instars (Sisay et al., 2019). The larvae were spaced at different leaf nodes to prevent cannibalism (Phambala et al., 2020). The larvae were obtained from a university maintained culture of FAW established in 2019 within the entomology laboratory. To ensure larval survival, the infestation was done early in the morning before temperatures increased to assist their establishment (Prasanna et al., 2018). Plant pots were caged separately (cage size: 1.8 m × 0.6 m × 0.6 m) to prevent cross movement of larvae from one pot to another. The trial treatments commenced one week after larval establishment where soil treatments were applied weekly using a small spoon (approx. 3 g) to each leaf whorl on the maize plant. Chlorpyriphos was applied following recommended application rates (480 g/l EC, 200 ml/ha) using a knapsack sprayer. Data collection on maize leaf damage and FAW numbers was collected over 5 weeks and used the same protocols as described in the botanical treatment trial. As this was a potted plant screenhouse trial with limited numbers of maize plants, yield data were not collected.
Data analysis
Data on mean percentage leaf damage score, the mean number of FAW larvae present and mean maize crop yield were subjected to an analysis of variance (ANOVA) at P < 0.05. Mean values were separated using the Fisher’s Least Significant Difference test at the 95% confidence interval. All statistical analyses were performed using Xlstat version 17.01 (Addinsoft, Paris France).
Results
Botanical pesticides
All four botanical species, A. indica, N. tabacum, C. citratus and L. javanica reduced maize leaf damage and the number of FAW larvae while increasing maize crop yield in comparison to the untreated control (Figure 1; Table 1). The botanical treatments were less effective at reducing leaf damage and larval numbers than the synthetic pesticide treatment. However, the yield achieved across the botanical and synthetic treatments was statistically similar (Figure 1). The yield obtained from botanical treatments was highest with A. indica powder (5,600 kg/ha), C. citratus extract (5,800 kg/ha) and N. tabacum extract (5,800 kg/ha) with the synthetic treatment yield at 6,900 kg/ha and the untreated yield at 1,700 kg/ha. Maize yields obtained with all botanical treatments were at least double that recorded in the untreated control. Applying botanicals as water extracts or as powders directly in the maize leaf whorls did not make much difference in terms of damage, larval number, or yield. Using the powder treatment for A. indica and C. citratus led to marginally lower damage and larval numbers than their respective extract treatment, but this trend was not observed with respect to maize yield where all botanical species applied either as extracts or as powders did not produce significantly different yields. Yields were considerably lower in this trial than those achieved in the soil application trial described below. As the season progressed, the field location where the botanical trial was located developed a problem with termites in the soil, which together with other potential differences in baseline soil fertility could possibly explain the lower crop yields achieved.
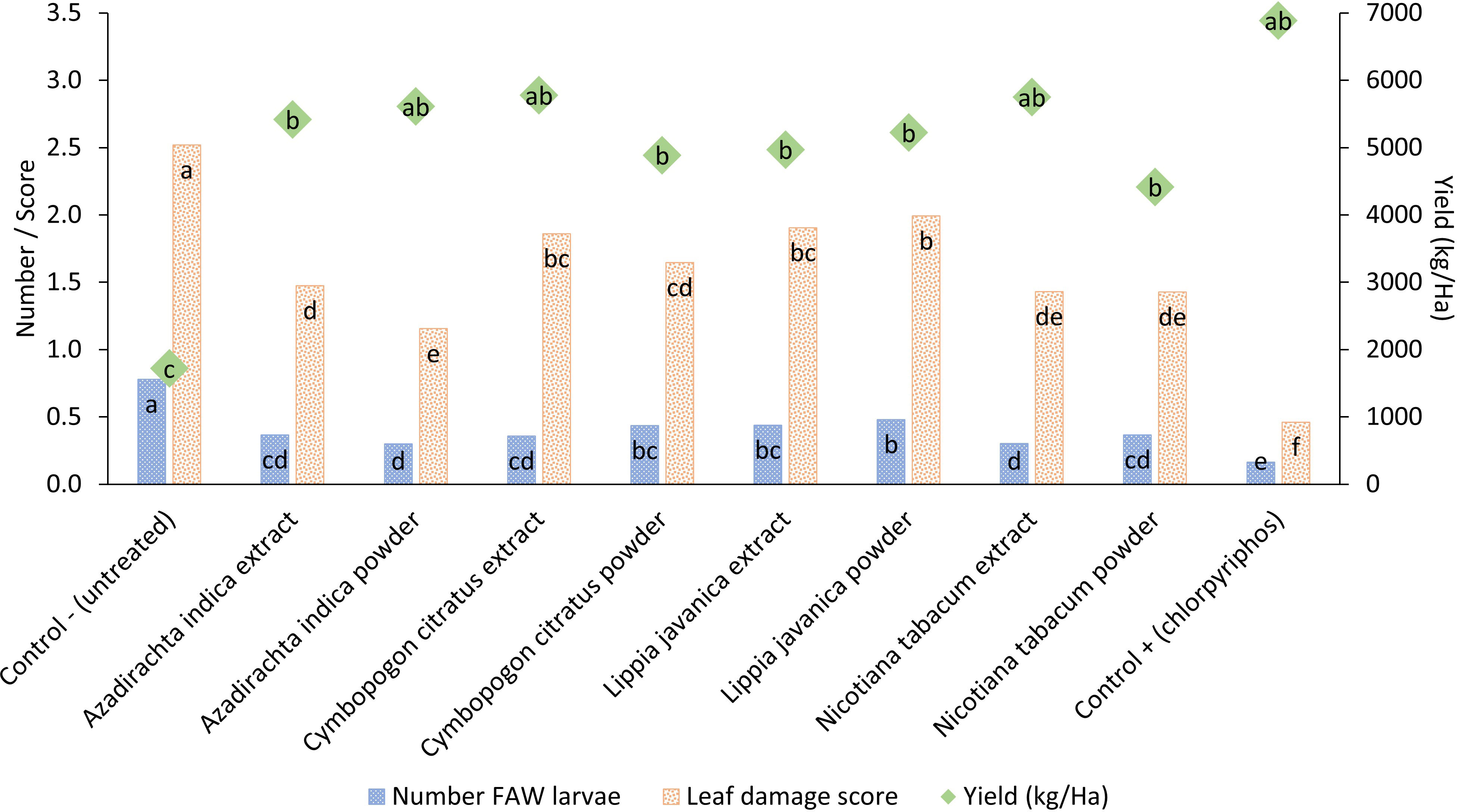
Figure 1 Pesticidal plant treatments applied either as a water extract (10% w/v) or as dry powdered leaf material (~ 3 g) deposited directly in the maize leaf whorl. Treatments were scored for their impact on the mean number of fall armyworm larvae present per plant, mean larval feeding damage to maize leaves and the mean yield of maize obtained at the end of the cropping season. Treatments across each parameter with different letters are significantly different using Fisher’s LSD test at the 95% confidence interval.
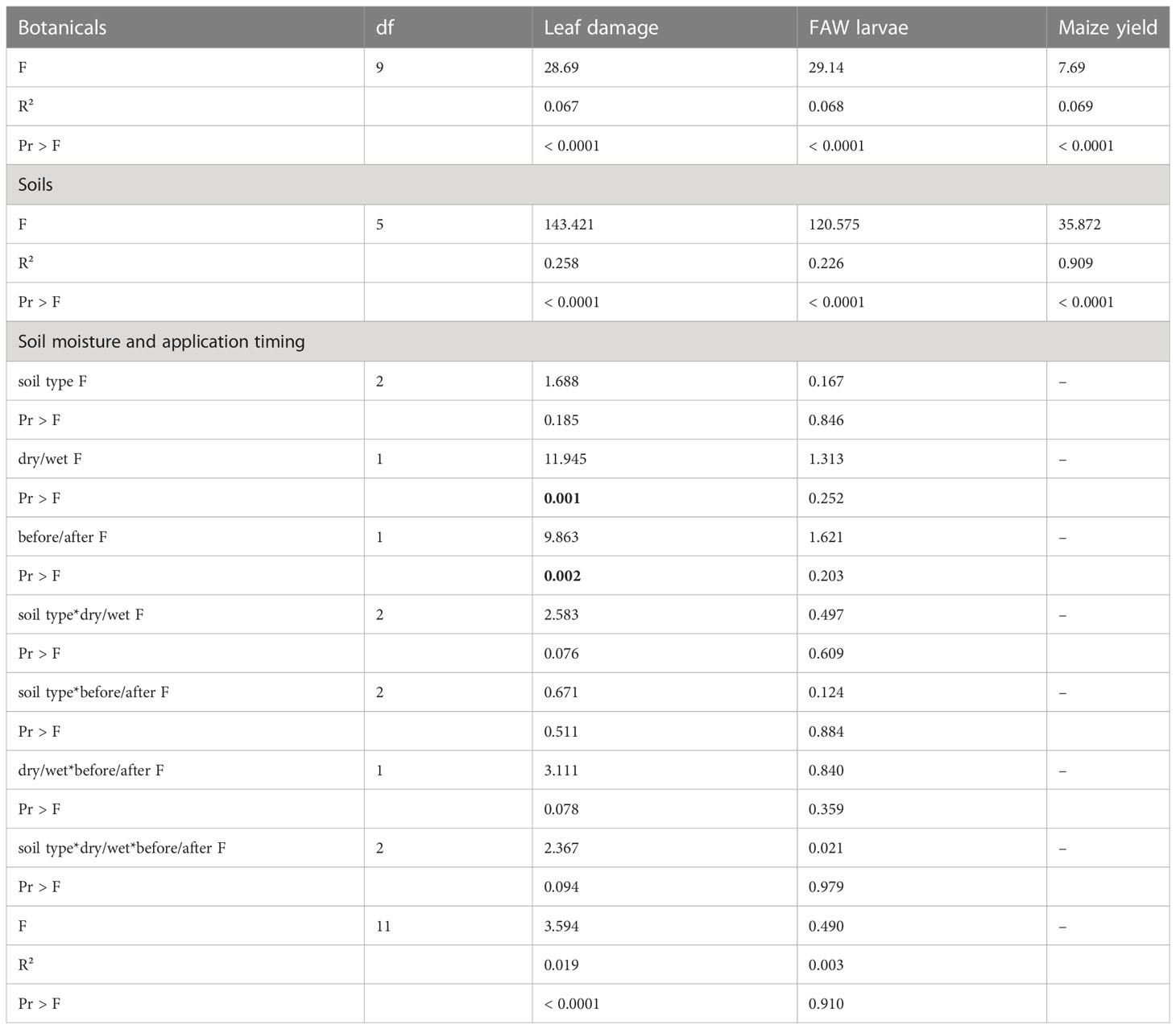
Table 1 Analysis of Variance (ANOVA) for maize leaf damage scoring, the number of fall armyworm (FAW) found in maize leaf whorls and the maize crop yield obtained across separate cropping trials: 1) evaluating the use of botanical treatments applied either as extracts or powders; 2) evaluating different soil types and wood ash treatments applied to maize leaf whorls; 3) the impact of soil moisture content and timing of application for different soil type treatments applied to maize leaf whorls.
Soil applications
Wood ash and the three soil types were all able to reduce the number of FAW larvae and leaf damage scores leading to significantly higher maize yields than the untreated control (Figure 2; Table 1). Ash, clay and sand treatments provided similar levels of protection against FAW, whilst the treatment with loam was less effective. The synthetic treatment outperformed all other treatments in terms of reducing damage and larvae and increasing yield. Yield with the synthetic control (13,700 kg/ha) was almost double that of the untreated control (7,900 kg/ha) while soil and ash treatments had significantly higher yields (10,400 to 12,400 kg/ha) closer to that recorded for the synthetic treatment.
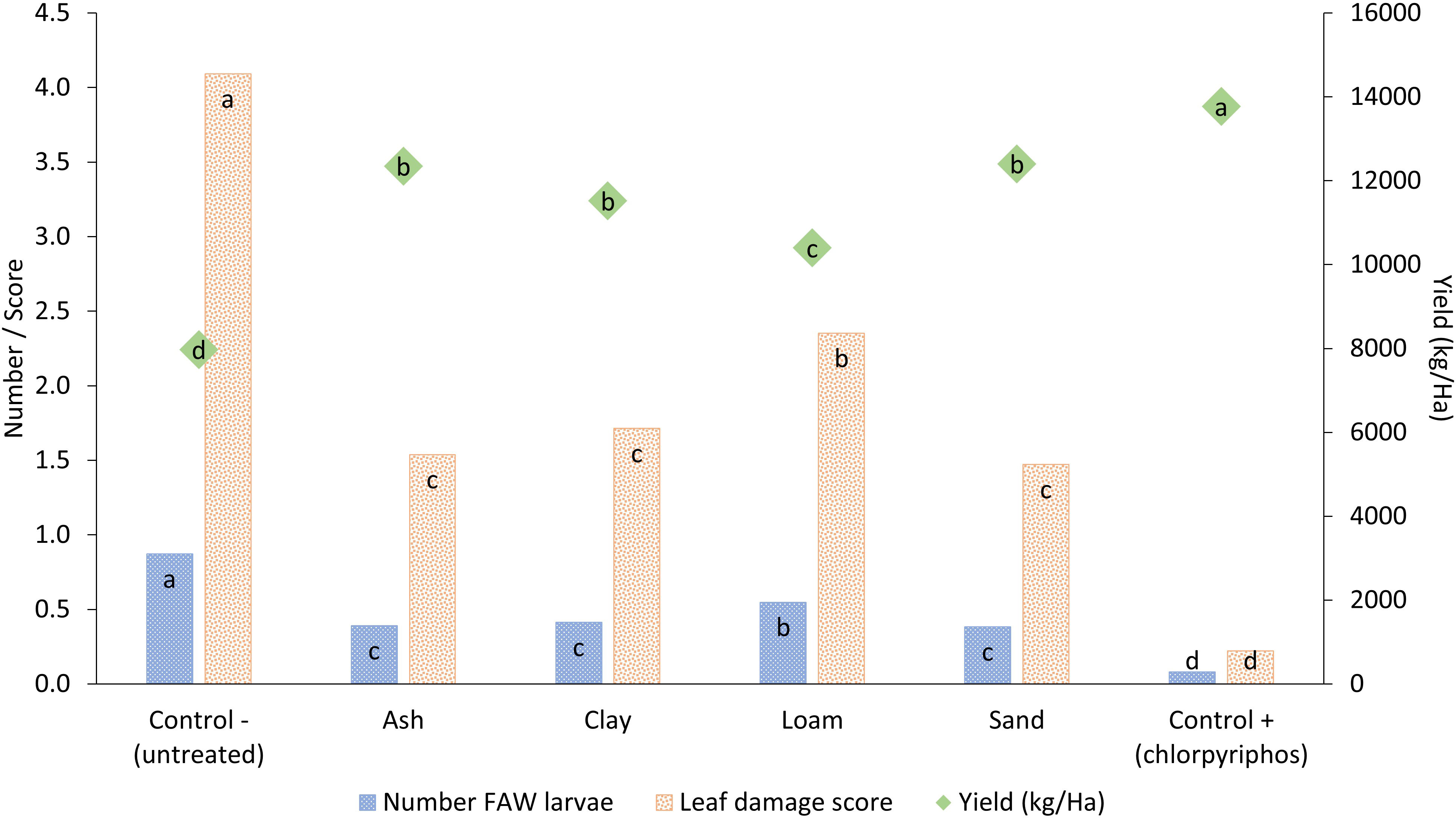
Figure 2 Impact of different soil types and wood ash applied to maize leaf whorls to control fall armyworm larval numbers, maize leaf damage and crop yield. Treatments across each parameter with different letters are significantly different using Fisher’s LSD test at the 95% confidence interval.
Assessment of soil moisture and timing of application
There was no significant difference in FAW numbers and maize leaf damage among the sand, clay and loam treatments as shown above although a multi-factor analysis of variance with parameters of soil type, wet or dry soil and time of application before or after watering showed that timing and moisture content of the soil was important (Figure 3; Table 1). In general, using dry soil was better than using wet soil and applying the soil after watering was better than applying it before watering. This indicated that moisture of the soil does influence efficacy; however, a post-hoc Fisher’s LSD test did not show significant differences across the treatments suggesting any impact of moisture availability is minimal (Figure 3). The synthetic control treatment reduced the number of FAW larvae by approximately 50% compared to the untreated control, a reduction that was also observed in the soil treatments.
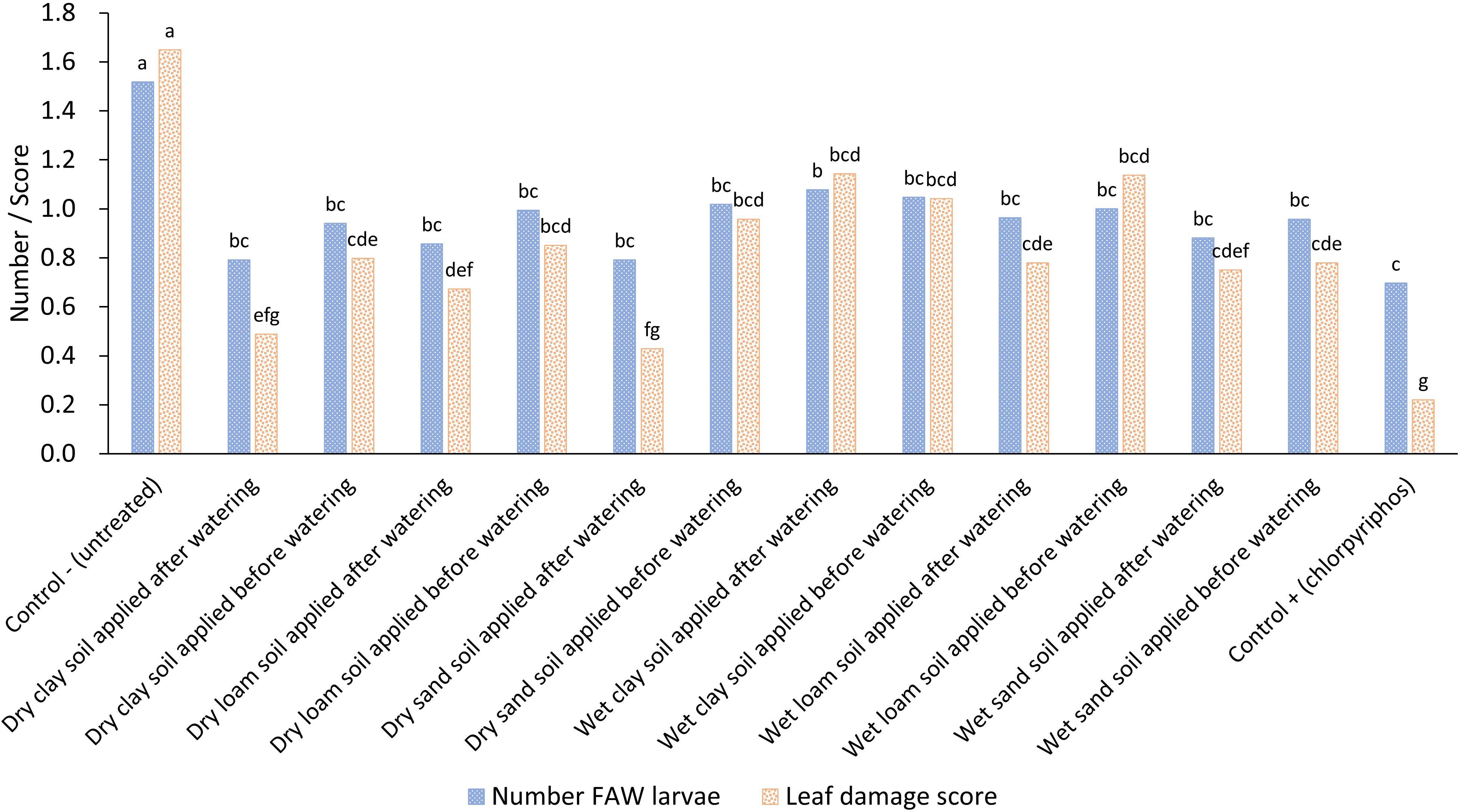
Figure 3 Impact of different soil type treatments applied to maize leaf whorls either dry or wet and either before or after crop irrigation on the average fall armyworm larval numbers and average maize leaf damage. Treatments across each parameter with different letters are significantly different using Fisher’s LSD test at the 95% confidence interval.
Discussion
Botanical pesticides have been extensively demonstrated to control a number of important crop pests (Stevenson et al., 2017; Isman, 2020; Uyi et al., 2021; Ngegba et al., 2022) including FAW (Phambala et al., 2020; Rioba and Stevenson, 2020). Research on the efficacy of botanicals (Mkindi et al., 2017), their environmental safety (Tembo et al., 2018), cost-benefits (Amoabeng et al., 2014; Mkenda et al., 2015) and farmer-perceived benefits to health (Mkindi et al., 2021) highlight their agroecological importance, particularly within smallholder farming systems (Isman, 2008; Belmain et al., 2022). Botanical pesticides are typically formulated as liquids to spray on to crops. However, traditional uses of locally available pesticidal plants in Africa often involves different application approaches. For example, in post-harvest protection of stored legumes and cereals, plant materials are often ground to a powder and admixed with stored grain to prevent infestation (Kamanula et al., 2011). Some stored product protection practices also involve using whole plant materials, such as leaves, being layered in stored grain bins (Jembere et al., 1995). The use of pesticidal plants against livestock ectoparasites may involve making pastes which are applied to areas infested with ticks or mites (Güler et al., 2021), and botanical pastes have also been used to protect germinating seeds (Borges et al., 2018). The practice of using pesticidal plant powders in field crop protection is not widely reported in the scientific literature; although some of the earliest use of botanicals such as Derris spp. involved crop dusting (Webb, 1946). Reports of smallholder farmers using botanical powders to control stem borer pests has received some evaluation (Dejen et al., 2011), but using botanical powders has not been explicitly evaluated in the context of FAW. Our research indicated that botanical powders are effective for FAW management and comparable to alternative application approaches such as preparing water extracts to spray on crops. Using plant powders requires less labour than making extracts and alongside specifically targeting the whorls would mean less plant material was required making their use more cost effective. Although the botanical treatments were not as effective as the synthetic pesticide treatment in reducing FAW larval numbers and leaf damage, the yield achieved using botanical treatments was statistically similar to the synthetic treatment. This phenomenon has been noted in other crop trials evaluating botanical pesticide treatments (Mkindi et al., 2017; Tembo et al., 2018), indicating that most crop plants are able to compensate for some pest damage and maintain yield as long as pest damage can be minimised (Bardner and Fletcher, 1974; Rubia et al., 1996).
Applying soil and wood ash to leaf whorls has been reported in farmer surveys to control stem borers and armyworm (Lawani, 1982; Kumela et al., 2019), but with limited evidence of systematic evaluation (Babendreier et al., 2020). Our results indicated that all soil types and ash generated from household wood fires were effective treatments to manage FAW larval numbers and damage rates. We did not investigate the mode of action but would argue that our results suggest the main mode of action is most likely to be physical and involve suffocation and/or abrasion of the insect cuticle which leads to death through desiccation, because similar results were observed between wood ash and the soil treatments. Wood ash would not contain entomopathogens, as has been argued for soil treatments (Hruska, 2019), where ash would most closely resemble the action of diatomaceous earth applications (Aniwanou et al., 2020). Furthermore, in our trial the loamy soil treatment was statistically less effective than sand, clay and ash, which could be explained through this soil type most likely being less abrasive as it contains more organic matter than the other types. With its higher organic matter content, loamy soil may be more likely to contain entomopathogenic organisms. However, our research did not attempt to verify the presence of entomopathogens in any of the soil treatments we used, and we cannot exclude the role of soil-borne entomopathogens contributing to the observed efficacy. Further research to clarify the mode of action of using soil treatments to control FAW is recommended.
The practical conditions under which soil is applied to leaf whorls does not seem to be important with respect to whether soil is wet when applied or becomes wet later on through rainfall. Our research does show that soil moisture can be important, and it is best to use dry soil and to apply it after it rains, but this does not have a large effect on efficacy overall. This makes the method much more practical and flexible for smallholder farmer use but where our results on moisture and timing facilitates some standardisation of optimised practices. Our trials were implemented based on crop surveillance indicators of 20% plant infestation rates. Simple surveillance methods for FAW are crucial to detect its presence when infestation is still early and when the larvae are in their early instars (Harrison et al., 2019). Appropriate surveillance methods for smallholder farmers have been developed (Prasanna et al., 2018; Nyamutukwa et al., 2022) and are widely recommended to help farmers implement pest management activities before damage becomes widespread and severe as the larvae increase in size and start rapidly consuming the crop (Hruska, 2019). Our implementation of soil and botanical treatments at 20% crop infestation rate could be argued to be ideal timing as it means the larvae are still small and thus more vulnerable to control methods (Overton et al., 2021). It is likely that the efficacy of soil and botanical treatments will decline as the larvae mature, a phenomenon that has been observed where synthetic pesticides have been used to control FAW (FAO, 2018). An optimised protocol for using soil and botanical powders must therefore consider timing of application as an important factor, ideally along with applying materials under dry conditions where rain is not imminent.
In conclusion our research confirms some of the smallholder farmer innovations traditionally used by farmers to manage FAW. All soil types are likely to be effective, but sand and clay soils are most likely to be effective, particularly if considering the main role of action is through physical abrasion of the insect cuticle or suffocation. Loam type soils generally contain more organic matter, where its presence is likely to reduce abrasive properties, but loam soils may be more likely to introduce soil-borne entomopathogens that can attack FAW larvae. Further investigation on the mode of action (physical and/or biocontrol) of soil to control FAW using a wider range of soil types is recommended. Similarly, our work did not determine whether using pesticidal plants used as a powder acted physically on the insect cuticle through abrasion or absorbing cuticular waxes compared to their known biochemical activities as antifeedants, repellents and toxins. Powdered plant materials are unlikely to be highly abrasive, but plant powders may stick to the insect cuticle, potentially absorbing cuticular waxes that could interfere with the insect’s internal water regulation. Considering our data showed no clear difference between using pesticidal plants as an extract or powder we would argue that the mode of action is most likely related to bioactivity of phytochemicals. Further research could help determine modes of action of botanicals related to differences in application protocols. Considering smallholder needs to manage highly damaging pests such as FAW, but doing so in low-cost agroecologically sustainable ways, we would argue the use of soils and botanicals are effective options that are safe and cost-beneficial.
Data availability statement
The raw data supporting the conclusions of this article will be made available by the authors, without undue reservation.
Author contributions
Conceptualization: SB, PS, YT. Methodology: GC, SB, PS, YB, TK, VK. Field work: GC. Analysis: GC, SB. Data curation: SB. Writing original draft preparation: GC, SB. Reviewing: GC, SB, PS, YT, TK, VK. Project administration: YT, SB. Funding acquisition: SB. All authors contributed to the article and approved the submitted version.
Funding
This research was supported by a grant (20-034) from the McKnight Foundation Collaborative Crop Research Programme.
Acknowledgments
Special thanks to LUANAR technical support staff Maxwell Katulu, Faida Kennedy, Frank Chibwana, Febby Kaluwa, Highten Francis and Herbert Folias.
Conflict of interest
The authors declare that the research was conducted in the absence of any commercial or financial relationships that could be construed as a potential conflict of interest.
Publisher’s note
All claims expressed in this article are solely those of the authors and do not necessarily represent those of their affiliated organizations, or those of the publisher, the editors and the reviewers. Any product that may be evaluated in this article, or claim that may be made by its manufacturer, is not guaranteed or endorsed by the publisher.
References
Abro Z., Kimathi E., De Groote H., Tefera T., Sevgan S., Niassy S., et al. (2021). Socioeconomic and health impacts of fall armyworm in Ethiopia. PloS One 16, e0257736. doi: 10.1371/journal.pone.0257736
Ahissou B. R., Sawadogo W. M., Dabiré G. T., Kambiré F. C., Bokonon-Ganta A. H., Somda I., et al. (2022). Susceptibility of fall armyworm Spodoptera frugiperda (JE smith) to microbial and botanical bioinsecticides and control failure likelihood estimation. Biotechnol. Agron. Société Environ. 26, 136–143. doi: 10.25518/1780-4507.19793
Amoabeng B. W., Gurr G. M., Gitau C. W., Stevenson P. C. (2014). Cost:benefit analysis of botanical insecticide use in cabbage: implications for smallholder farmers in developing countries. Crop Prot. 57, 71–76. doi: 10.1016/j.cropro.2013.11.019
Andersson E., Isgren E. (2021). Gambling in the garden: pesticide use and risk exposure in Ugandan smallholder farming. J. Rural Stud. 82, 76–86. doi: 10.1016/j.jrurstud.2021.01.013
Aniwanou C. T. S. S., Sinzogan A. A. C. C., Deguenon J. M., Sikirou R., Stewart D. A., Ahanchede A. (2020). Bio-efficacy of diatomaceous earth, household soaps, and neem oil against Spodoptera frugiperda (Lepidoptera: noctuidae) larvae in Benin. Insects 12, 18. doi: 10.3390/insects12010018
Asare-Nuamah P. (2022). Smallholder farmers’ adaptation strategies for the management of fall armyworm (Spodoptera frugiperda) in rural Ghana. Int. J. Pest Manag. 68, 8–18. doi: 10.1080/09670874.2020.1787552
Babendreier D., Koku Agboyi L., Beseh P., Osae M., Nboyine J., Ofori S. E. K., et al. (2020). The efficacy of alternative, environmentally friendly plant protection measures for control of fall armyworm, Spodoptera frugiperda, in maize. Insects 11, 240. doi: 10.3390/insects11040240
Bardner R., Fletcher K. E. (1974). Insect infestations and their effects on the growth and yield of field crops: a review. Bull. Entomol. Res. 64, 141–160. doi: 10.1017/S0007485300027061
Bateman M. L., Day R. K., Rwomushana I., Subramanian S., Wilson K., Babendreier D., et al. (2021). Updated assessment of potential biopesticide options for managing fall armyworm (Spodoptera frugiperda) in Africa. J. Appl. Entomol. 145, 384–393. doi: 10.1111/jen.12856
Belmain S. R., Tembo Y., Mkindi A. G., Arnold S. E. J., Stevenson P. C. (2022). Elements of agroecological pest and disease management. Elem. Sci. Anthr. 10, 1–14. doi: 10.1525/elementa.2021.00099
Borges D. F., Lopes E. A., Fialho Moraes A. R., Soares M. S., Visôtto L. E., Oliveira C. R., et al. (2018). Formulation of botanicals for the control of plant-pathogens: a review. Crop Prot. 110, 135–140. doi: 10.1016/j.cropro.2018.04.003
Cheruiyot D., Chidawanyika F., Midega C. A. O., Pittchar J. O., Pickett J. A., Khan Z. R. (2021). Field evaluation of a new third generation push-pull technology for control of striga weed, stemborers, and fall armyworm in western Kenya. Exp. Agric. 57, 301–315. doi: 10.1017/S0014479721000260
Constantine K. L., Murphy S. T., Pratt C. F. (2020). The interaction between pests, mixed-maize crop production and food security: a case study of smallholder farmers in mwea West, Kenya. Cogent. Food Agric. 6, 1857099. doi: 10.1080/23311932.2020.1857099
Dejen A., Getu E., Azerefegne F., Ayalew A. (2011). Efficacy of some botanicals on stem borers, Busseola fusca (Fuller) and Chilo partellus (Swinhoe) on sorghum in Ethiopia under field conditions. Biopestic. Int. 7, 24–34.
Donald C. E., Scott R. P., Blaustein K. L., Halbleib M. L., Sarr M., Jepson P. C., et al. (2016). Silicone wristbands detect individuals’ pesticide exposures in West Africa. R. Soc. Open Sci. 3, 160433. doi: 10.1098/rsos.160433
FAO (2018). Integrated management of the fall armyworm on maize: a guide for farmer field schools in Africa (Rome, Italy: FAO).
Goergen G., Kumar P. L., Sankung S. B., Togola A., Tamò M. (2016). First report of outbreaks of the fall armyworm Spodoptera frugiperda (J e smith) (Lepidoptera, noctuidae), a new alien invasive pest in West and central Africa. PloS One 11, e0165632. doi: 10.1371/journal.pone.0165632
Güler O., Polat R., Karaköse M., Çakılcıoğlu U., Akbulut S. (2021). An ethnoveterinary study on plants used for the treatment of livestock diseases in the province of giresun (Turkey). South Afr. J. Bot. 142, 53–62. doi: 10.1016/j.sajb.2021.06.003
Guo J., Wu S., Zhang F., Huang C., He K., Babendreier D., et al. (2020). Prospects for microbial control of the fall armyworm Spodoptera frugiperda: a review. BioControl 65, 647–662. doi: 10.1007/s10526-020-10031-0
Harrison R. D., Thierfelder C., Baudron F., Chinwada P., Midega C., Schaffner U., et al. (2019). Agro-ecological options for fall armyworm (Spodoptera frugiperda JE smith)management: providing low-cost, smallholder friendly solutions to an invasive pest. J. Environ. Manage 243, 318–330. doi: 10.1016/j.jenvman.2019.05.011
Hruska A. J. (2019). Fall armyworm (Spodoptera frugiperda) management by smallholders. CABI Rev. 2019, 1–11. doi: 10.1079/PAVSNNR201914043
Isman M. B. (2008). Botanical insecticides: for richer, for poorer. Pest Manag. Sci. 64, 8–11. doi: 10.1002/ps.1470
Isman M. B. (2020). Botanical insecticides in the twenty-first century–fulfilling their promise? Annu. Rev. Entomol. 65, 233–249. doi: 10.1146/annurev-ento-011019-025010
Jembere B., Obeng-Ofori D., Hassanali A., Nyamasyo G. N. (1995). Products derived from the leaves of Ocimum kilimandscharicum (Labiatae) as post-harvest grain protectants against the infestation of three major stored product insect pests. Bull. Entomol. Res. 85, 361–367. doi: 10.1017/S0007485300036099
Kamanula J., Sileshi G. W., Belmain S. R., Sola P., Mvumi B. M., Nyirenda G. K. C., et al. (2011). Farmers ‘ insect pest management practices and pesticidal plant use in the protection of stored maize and beans in southern Africa. Int. J. Pest Manag. 57, 41–49. doi: 10.1080/09670874.2010.522264
Kilani-Morakchi S., Morakchi-Goudjil H., Sifi K. (2021). Azadirachtin-based insecticide: overview, risk assessments, and future directions. Front. Agron., 32 (3). doi: 10.3389/fagro.2021.676208
Kumela T., Simiyu J., Sisay B., Likhayo P., Mendesil E., Gohole L., et al. (2019). Farmers’ knowledge, perceptions, and management practices of the new invasive pest, fall armyworm (Spodoptera frugiperda) in Ethiopia and Kenya. Int. J. Pest Manag. 65, 1–9. doi: 10.1080/09670874.2017.1423129
Lawani S. M. (1982). A review of the effects of various agronomic practices on cereal stem borer populations. Trop. Pest Manag. 28, 266–276. doi: 10.1080/09670878209370720
Lunt T., Ellis-Jones J., Mekonnen K., Schulz S., Thorne P., Schulte-Geldermann E., et al. (2018). Participatory community analysis: identifying and addressing challenges to Ethiopian smallholder livelihoods. Dev. Pract. 28, 208–226. doi: 10.1080/09614524.2018.1417354
Midega C. A. O. O., Pittchar J. O., Pickett J. A., Hailu G. W., Khan Z. R. (2018). A climate-adapted push-pull system effectively controls fall armyworm, Spodoptera frugiperda (J.E. smith), in maize in East Africa. Crop Prot. 105, 10–15. doi: 10.1016/j.cropro.2017.11.003
Mkenda P., Mwanauta R., Stevenson P. C., Ndakidemi P., Mtei K., Belmain S. R. (2015). Extracts from field margin weeds provide economically viable and environmentally benign pest control compared to synthetic pesticides. PloS One 10, e0143530. doi: 10.1371/journal.pone.0143530
Mkindi A. G., Coe R., Stevenson P. C., Ndakidemi P. A., Belmain S. R. (2021). Qualitative cost-benefit analysis of using pesticidal plants in smallholder crop protection. Agriculture 11, 1007. doi: 10.3390/agriculture11101007
Mkindi A., Mpumi N., Tembo Y., Stevenson P. C., Ndakidemi P. A., Mtei K., et al. (2017). Invasive weeds with pesticidal properties as potential new crops. Ind. Crops Prod. 110, 0–1. doi: 10.1016/j.indcrop.2017.06.002
Ngegba P. M., Cui G., Khalid M. Z., Zhong G. (2022). Use of botanical pesticides in agriculture as an alternative to synthetic pesticides. Agriculture 12, 600. doi: 10.3390/agriculture12050600
Nyamutukwa S., Mvumi B. M., Chinwada P. (2022). Sustainable management of fall armyworm, Spodoptera frugiperda (J.E. smith): challenges and proposed solutions from an African perspective. Int. J. Pest Manag., 1–19.
Overton K., Maino J. L., Day R., Umina P. A., Bett B., Carnovale D., et al. (2021). Global crop impacts, yield losses and action thresholds for fall armyworm (Spodoptera frugiperda): a review. Crop Prot. 145, 105641. doi: 10.1016/j.cropro.2021.105641
Phambala K., Tembo Y., Kasambala T., Kabambe V. H., Stevenson P. C., Belmain S. R. (2020). Bioactivity of common pesticidal plants on fall armyworm larvae (Spodoptera frugiperda). Plants 9, 112. doi: 10.3390/plants9010112
Prasanna B. M., Huesing J. E., Eddy R., Peschke V. M. (2018). Fall armyworm in Africa: a guide for integrated pest management. Ciudad de Mexico, Mexico: International Maize and Wheat Improvement Center (CIMMYT).
Ramirez-Rodriguez D., Sánchez-Peña S. R. (2016). Endophytic Beauveria bassiana in Zea mays: Pathogenicity against larvae of fall armyworm, Spodoptera frugiperda. Southwest Entomol. 41, 875–878. doi: 10.3958/059.041.0330
Rani L., Thapa K., Kanojia N., Sharma N., Singh S., Grewal A. S., et al. (2021). An extensive review on the consequences of chemical pesticides on human health and environment. J. Clean Prod. 283, 124657. doi: 10.1016/j.jclepro.2020.124657
Rioba N. B., Stevenson P. C. (2020). Opportunities and scope for botanical extracts and products for the management of fall armyworm (Spodoptera frugiperda) for smallholders in Africa. Plants 9, 207. doi: 10.3390/plants9020207
Rubia E. G., Heong K. L., Zalucki M., Gonzales B., Norton G. A. (1996). Mechanisms of compensation of rice plants to yellow stem borer Scirpophaga incertulas (Walker) injury. Crop Prot. 15, 335–340. doi: 10.1016/0261-2194(95)00102-6
Sarker S., Lim U. T. (2018). Extract of Nicotiana tabacum as a potential control agent of Grapholita molesta (Lepidoptera: tortricidae). PloS One 13, e0198302. doi: 10.1371/journal.pone.0198302
Sharma A., Shukla A., Attri K., Kumar M., Kumar P., Suttee A., et al. (2020). Global trends in pesticides: a looming threat and viable alternatives. Ecotoxicol. Environ. Saf. 201, 110812. doi: 10.1016/j.ecoenv.2020.110812
Sisay B., Tefera T., Wakgari M., Ayalew G., Mendesil E. (2019). The efficacy of selected synthetic insecticides and botanicals against fall armyworm, Spodoptera frugiperda, in maize. Insects 10, 45. doi: 10.3390/insects10020045
Stevenson P. C., Isman M. B., Belmain S. R. (2017). Pesticidal plants in Africa: a global vision of new biological control products from local uses. Ind. Crops Prod. 110, 2–9. doi: 10.1016/j.indcrop.2017.08.034
Tambo J. A., Kansiime M. K., Mugambi I., Rwomushana I., Kenis M., Day R. K., et al. (2020). Understanding smallholders’ responses to fall armyworm (Spodoptera frugiperda) invasion: evidence from five African countries. Sci. Total Environ. 740, 140015. doi: 10.1016/j.scitotenv.2020.140015
Tembo Y., Mkindi A. G., Mkenda P. A., Mpumi N., Mwanauta R., Stevenson P. C., et al. (2018). Pesticidal plant extracts improve yield and reduce insect pests on legume crops without harming beneficial arthropods. Front. Plant Sci. 9, 1425. doi: 10.3389/fpls.2018.01425
Toepfer S., Fallet P., Kajuga J., Bazagwira D., Mukundwa I. P., Szalai M., et al. (2021). Streamlining leaf damage rating scales for the fall armyworm on maize. J. Pest Sci. 2004) 94, 1075–1089. doi: 10.1007/s10340-021-01359-2
USDA Soil Science Staff (2017). Soil survey manual, USDA handbook 18, 4th ed. Washington D.C., USA: United States Department of Agriculture.
Uyi O., Mukwevho L., Ejomah A. J., Toews M. (2021). Invasive alien plants in Sub-Saharan Africa: a review and synthesis of their insecticidal activities. Front. Agron. 3, 76. doi: 10.3389/fagro.2021.725895
Valicente F. H., Barreto M. R. (2003). Bacillus thuringiensis survey in Brazil: geographical distribution and insecticidal activity against Spodoptera frugiperda (J.E. smith) (Lepidoptera: noctuidae). Neotrop. Entomol. 32, 639–644. doi: 10.1590/S1519-566X2003000400014
Webb J. E. (1946). The penetration of derris through the spiracles and cuticle of Melophagus ovinus, l. Bull. Entomol. Res. 36, 15–22. doi: 10.1017/S0007485300023889
Yang X., Wyckhuys K. A. G., Jia X., Nie F., Wu K. (2021). Fall armyworm invasion heightens pesticide expenditure among Chinese smallholder farmers. J. Environ. Manage 282, 111949. doi: 10.1016/j.jenvman.2021.111949
Yu S. J. (1991). Insecticide resistance in the fall armyworm, Spodoptera frugiperda (J. e. smith). Pestic. Biochem. Physiol. 39, 84–91. doi: 10.1016/0048-3575(91)90216-9
Keywords: Spodoptera frugiperda, Zea mays, pesticidal plant, Azadirachta indica, Cymbopogon citratus, Lippia javanica, Nicotiana tabacum, fall armyworm
Citation: Chawanda G, Tembo YLB, Donga TK, Kabambe VH, Stevenson PC and Belmain SR (2023) Agroecological management of fall armyworm using soil and botanical treatments reduces crop damage and increases maize yield. Front. Agron. 5:1114496. doi: 10.3389/fagro.2023.1114496
Received: 02 December 2022; Accepted: 24 April 2023;
Published: 25 May 2023.
Edited by:
Julio S. Bernal, Texas A&M University, United StatesReviewed by:
Osariyekemwen Uyi, University of Benin, NigeriaMurray B. Isman, University of British Columbia, Canada
Copyright © 2023 Chawanda, Tembo, Donga, Kabambe, Stevenson and Belmain. This is an open-access article distributed under the terms of the Creative Commons Attribution License (CC BY). The use, distribution or reproduction in other forums is permitted, provided the original author(s) and the copyright owner(s) are credited and that the original publication in this journal is cited, in accordance with accepted academic practice. No use, distribution or reproduction is permitted which does not comply with these terms.
*Correspondence: Steven R. Belmain, cy5yLmJlbG1haW5AZ3JlLmFjLnVr