- 1Energy Institute, The University of Texas at Austin, Austin, TX, United States
- 2Department of Computer Engineering, Cummins College of Engineering for Women, Pune, India
1 Introduction
Agriculture is critical for the survival of human civilization. We obtain food, such as millets, lentils, fruits, etc., as well as non-food products like cotton, jute, rubber, etc., from agriculture. In the Indian context, agriculture contributed to about 20.2 percent of the Gross Value Added (GVA) of India for the year 2020-21 (PIB Delhi, 2021), and 1,48,078 thousand hectares (75%) and 49,242 thousand hectares (25%) of land are used for growing food crops and non-food crops, respectively (Department of Agriculture and Farmers Welfare, 2021). These products, in their final form, constitute only a fraction of the total biomass produced in the process. For example, Dhanraj et al. (2021) summarized the ratio of residue to crop for cotton, sorghum, sugarcane, wheat, and rice. The ratio ranges from 0.25 for sugarcane to 3.8 for cotton. In other words, for 1 kg of cotton produced, 3.8 kg of residual biomass needs to be disposed of. Thus, there is a significant untapped potential for residual biomass.
One of the main utilities of this biomass is the production of biofuels. According to one estimate by International Energy Agency (2023) (IEA), 6% of the global energy is supplied using biomass-based resources. Studies have been conducted to explore the use of these resources; for example, Hiloidhari et al. (2014) analyzed the potential of bioenergy from crop residue in India. They considered 26 main crops, which produce about 686 MT of crop residue. Their analysis indicated that if this agro-biomass is utilized effectively to produce bioenergy, it could compensate 17% of India’s total primary energy consumption (bioenergy equivalent to about 4.15 EJ). As discussed by Samer (2019); Otoni et al. (2021), another route of utilizing the agro-biomass is the production of bioplastics and other advanced materials However, these routes are relatively less explored than biofuel production routes and are yet to be established. This overall process of value retention of agro-biomass is termed agro-biomass valorization.
If this residual biomass is not used for any of the uses discussed above, then typically, it is treated as waste. There is a cost associated with the disposal of this material. Further, the decomposition of waste biomass releases methane, which is a greenhouse gas, thus, contributing to climate change. Moreover, it can also cause health hazards. Owing to the short crop cycle, in-situ burning is considered one of the ways of disposal of agro-biomass, which again leads to all the above problems. Annual air pollution spike in the northern part of India is caused by such practices. Venkatramanan et al. (2021); Baghel et al. (2023) have studied these aspects in more details. Hence, we can say that, apart from being a great source of high-value products, agro-biomass valorization can also help in reducing the intensity of several problems.
2 Agro-biomass valorization and SDGS
The scale of most of the scientific problems involving humans can be identified as micro, meso or macro. This classification is standardized across different disciplines. For example, the works of Serpa and Ferreira (2019) in sociology, Dopfer et al. (2004) in economics and Gilberthorpe and Papyrakis (2015) in the field of industrial ecology. The micro level focusses on individual level, meso on a group or regional level and macro on an even larger level could be a province, state, country and even the world. Methods and analysis approaches change with the level. Selection of appropriate level for analysis depends upon the problem at hand. Since, the sustainable development is a global issue and we are looking at a propagation of influence of a systemic change, we carry out an assessment at a macro level.
Studies so far have indicated that agro-biomass valorization can help in reducing dependence on fossil fuels via the production of biofuels. One can directly interpret that this is a step towards progress in Sustainable Development Goal (SDG) 7, which is “Ensure access to affordable, reliable, sustainable and modern energy for all” (United Nations, 2021). However, we ponder a larger question: can the agro-biomass valorization’s potential be leveraged to accelerate overall sustainable development? In other words, can it positively and significantly impact all the SDGs? In this article, we deliberate on this question using a systems thinking approach as discussed by (Arnold and Wade, 2015). Dörgő et al. (2018); Zelinka and Amadei (2019) ˝have carried out studies exploring connections between various SDGs. These examine a detailed picture of the interaction between various objectives of each of the goals. In contrast, we take a macro view to develop a holistic understanding to identify future directions of research.
Figure 1 shows the relationship between various SDGs when analyzed to propagate the influence of agro-biomass valorization. Different levels of SDGs are formed proportional to the influence they receive. First, we examine the SDGs whose progress has direct contributions to agro-biomass valorization. These are SDGs 9, 7, and 17. SDG 9 aims to “Build resilient infrastructure, promote sustainable industrialization and foster innovation”. A resilient infrastructure that can accelerate the innovation process is essential to utilize the full potential of agro-biomass valorization. Currently, except for the industrialized countries, the agricultural sector is used mainly for food production. Opportunities and incentives for the valorization of waste biomass to produce valuable products are scarce owing to limited infrastructure. Thus, infrastructure and innovation become driving forces for extracting benefits from agro-biomass valorization. Another essential factor is the energy supply. There has been extensive research examining the role of energy in agriculture. Interested readers may refer to the works of Markussen et al. (2015); Pimentel (2019); Krupnik et al. (2022); Walston et al. (2022) for better insights. Agro-biomass valorization would require additional energy, and hence, by extension, a sustainable energy supply becomes a necessity. Thus, SDG 7 is also critical for extracting the benefits of agro-biomass valorization. Apart from these technically influential SDGs, monetary and institutional support is also necessary for the successful implementation of any policy, idea, or project. SDG 17 deals with these aspects and hence, would be indispensable for agro-biomass valorization.
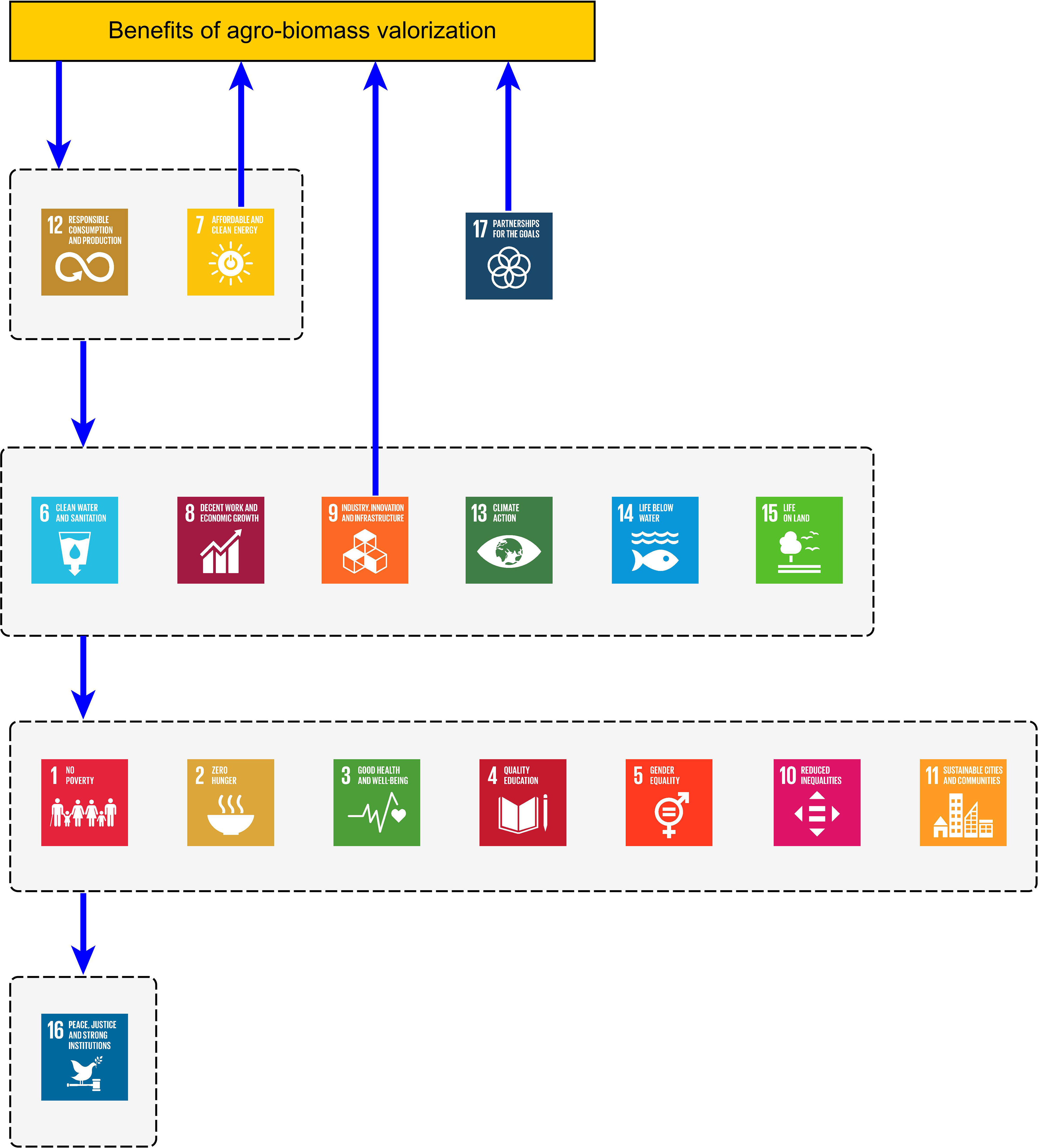
Figure 1 Causal relationship between Agro-Biomass Valorization and SDGs is shown in the figure. There are multiple levels, and the distance of each level indicates the direct effect of Agro-Biomass valorization on SDGs aggregated in it. SDG 9 is expected to govern the technological advancements and accessibility of agro-biomass valorization. All causalities are considered to be positive, and hence, no polarization signs are shown on the arrowheads. Further, to avoid cluttering, only the significant influences are shown, whereas other intra-level influences between the SDGs are discussed in Section 2.
Now, let us look at the propagation of benefits of agro-biomass valorization. SDG 7 and 12 are influenced directly. The importance of SDG 7, which highlights the need to ensure the supply of sustainable energy, is already discussed earlier. SDG 12 refers to sustainable production and consumption. It is an umbrella of objectives that intend to overhaul the entire production and consumption systems of the present global economy. One of the tenets of this SDG is the circular economy. Agro-biomass valorization is a shining example of the circular economy, which retains the value of materials that are already produced rather than disposing of them. It is clear that SDG 7 and 12 would get a significant boost because of agro-biomass valorization.
Progress in SDGs 7 and 12 have can have significant benefits in the SDGs pertaining to two domains, the environmental and the industry related. Industry-related SDGs include SDG 8 and SDG 9. SDG 8.4 targets to improve resource efficiency, which is indirectly benefitted by the agro-biomass valorization via progress in SDG 12. Processes of building, operating, maintaining, and updating the infrastructure are energy-intensive in nature. Hence, SDG 9, which focuses on infrastructure, is expected to be benefitted from the availability of sustainable energy (SDG 7). Other SDGs, namely, 6 (water quality), 13 (climate action), 14 (ocean acidification), and 15 (water ecosystem), benefit because of reduced consumption of fossil-based energy sources and avoided emissions and pollution.
Development in the environmental and industry SDGs propagates into the social SDGs. Better economic opportunities lead to poverty reduction and, subsequently, hunger reduction, better education, and expenses towards a healthy life. Other aspects related to equality also get positively affected by higher affluence. Lower pollution and a better environment mean better health. Lastly, better infrastructure and innovation can contribute towards sustainable communities. Thus, SDGs 1, 2, 3, 4, 5, 10, and 11 can be benefitted. Lastly, progress in all other SDGs is expected to be a stepping stone toward a peaceful and just world (SDG 16).
One example of impact propagation is discussed here. Implementation of agro-biomass valorization will reduce the material footprint of fuel production (Indicator 12.2.1). Lower material footprint means lower greenhouse gas (GHG) emissions (Indicator 13.2.2). Climate change is expected to affect agricultural productivity, thus, lower GHG emissions mean lower intensity of climate change and hence, relatively higher productivity (Indicator 2.3.1). In a similar way, influencing linkages to other indicators, whether direct or indirect, can be determined. However, development of the complete causal loop diagram and its calibration with real world data is part of a larger project and is beyond the scope of the present work.
3 Impact evaluation and techno-economical-environmental aspects
We propose that the direct impact of agro-biomass valorization to be quantified using an impact detection index defined as the ratio of fractional change in a particular indicator ΔX for a country to the weighted average of increment in valorized farm area normalized with total agricultural area in that country, as shown in Equation 1.
Impact propagation through the SDG targets can be similarly quantified by Equation 2
Where, i and j are the SDGs closer and farther from the agro-biomass valorization influence and is the indirect influence of agro-biomass valorization on SDG j via SDG i. This method can be used for impact propagation of any policy decisions on the SDGs.
The inputs to such evaluations are sought from national level data. For example, the progress in nationally determined contributions (NDC) can be used to assess the unit of overall progress towards SDG 13 (Climate action). Progress towards achievements of NDCs is reported by various countries. Other such similar external data can be used to evaluate SDG progress. However, Voluntary National Reviews, which is a mechanism to follow-up and track the SDG progress provides unobstructed view of the local realities. Thus, assessment of progress towards SDGs and hence, impact evaluation has minuscule scope of under or overestimation.
This work forms is the first step of a larger project of development of a complete system dynamics model to analyze impact of agro-biomass valorization on SDGs. In future, the model will be refined by calibrating it with SDG performance indicators. Then validation of the proposed view will be carried out through ex-post analysis. However, in context of the present work, verification of causal relationships can be carried out through examination of the SDG performance indicators.
As discussed by Shastri (2017), agro-biomass valorization intersects with second generation biofuels which can also be produced from energy crops, forest residues and waste from wood industry. Hence, infrastructural, techno-economic feasibility and environmental issues of second generation biofuels map to agro-biomass valorization. In order to fully utilize the potential of the agro-biomass, integrated biorefineries are needed. Several research groups, for example, Arranz-Piera et al. (2018); Ali et al. (2019); Gojiya et al. (2019), have analyzed feasibility of these systems. The valorization process requires use of chemicals like sulfuric acid, hydrochloric acid, sodium and ammonium hydroxide, ethanol, acetone, and butanol. Hence, valorization process invokes chemical safety concerns. From pollution perspective, solid byproducts like cellulose residues, liquid byproducts like waste water containing organic compounds and alcohols may be a matter of concern. Suitable emission control technologies and waste management practices become essential to this issue. Lastly, works of several researchers such as Guerrero and Muñoz (2018); Munagala and Shastri (2020); Prasad et al. (2020); Kumar and Verma (2021) have brought out that agro-biomass valorization has benefits whether from energy security, economic as well as environmental perspective. Hence, it can be considered as an investment towards long term benefits.
4 Final considerations
“Think global act local” has become the philosophy of today’s globalized world. Sustainability in agriculture is no exception which is largely focused on progressive measures to be taken towards making agriculture more productive. These measures fall under the ambit of under Voluntary National Reviews of SDGs which help us understand the local realities. However, macro-level assessment of agricultural sustainability becomes critical in developing a holistic understanding of the agricultural sustainability. This assessment calls for “agro-biomass valorization”. The impact of agro-biomass valorization on different sustainable development goals (SDGs) can be considered in four levels. These levels have differed influence of agro-biomass valorization. The energy and circular bioeconomy are the direct beneficiaries in the first level. Second level consists of key factors governing agriculture, that is, climate, water, soil, and technology. Overall development of a society can be benchmarked by social indicators such as poverty, education, gender equality and so on. These aspects are aggregated in level three. The overall objective of peace and just society is far away from this fulcrum on level four. The performance of agro-biomass valorization can be catalyzed through the infrastructure development, innovation in technology, access to renewable energy and support of global institutions. This inter-SDG impact can be quantified using the impact detection index proposed here. For the developed and developing countries, on a macro scale, while the causal relationship between agro-biomass valorization and SDGs remains the same, the quantum of impact propagated would differ based on local realities.
We conclude with a quote, by Thomas Jefferson, modified to suit the present work:
Agro-biomass valorization is our wisest pursuit because it will in the end contribute most to real wealth, good morals and happiness.
Author contributions
NH and NM drafted, critically revised the manuscript, and agree to be accountable for the content of the work. All authors contributed to the article and approved the submitted version.
Conflict of interest
The authors declare that the research was conducted in the absence of any commercial or financial relationships that could be construed as a potential conflict of interest.
Publisher’s note
All claims expressed in this article are solely those of the authors and do not necessarily represent those of their affiliated organizations, or those of the publisher, the editors and the reviewers. Any product that may be evaluated in this article, or claim that may be made by its manufacturer, is not guaranteed or endorsed by the publisher.
References
Ali M., Saleem M., Khan Z., Watson I. A. (2019). “The use of crop residues for biofuel production,” in Biomass, biopolymer-based materials, and bioenergy ((Woodhead Publishing), Woodhead Publishing Series in Composites Science and Engineering.), 369–395. doi: 10.1016/B978-0-08-102426-3.00016-3
Arnold R. D., Wade J. P. (2015). A definition of systems thinking: a systems approach. Proc. Comput. Sci. 44, 669–678. doi: 10.1016/j.procs.2015.03.050
Arranz-Piera P., Kemausuor F., Darkwah L., Edjekumhene I., Cortés J., Velo E. (2018). Mini-grid electricity service based on local agricultural residues: feasibility study in rural ghana. Energy 153, 443–454. doi: 10.1016/j.energy.2018.04.058
Baghel N., Singh K., Lakhani A., Kumari K. M., Satsangi A. (2023). A study of real-time and satellite data of atmospheric pollutants during agricultural crop residue burning at a downwind site in the indo-gangetic plain. Pollutants 3, 166–180. doi: 10.3390/pollutants3010013
Department of Agriculture and Farmers Welfare, Ministry of Agriculture and FarmersWelfare, Government of India (2021). Annual report 2021-22 (Ministry of Agriculture and Farmers Welfare, Government of India).
Dhanraj R., Punnathanam V., Shastri Y. (2021). Multi objective optimization of ethanol production based on regional resource availability. Sustain. Product. Consumpt. 27, 1124–1137. doi: 10.1016/j.spc.2021.02.021
Dopfer K., Foster J., Potts J. (2004). Micro-meso-macro. J. evolutionary econom. 14, 263–279. doi: 10.1007/s00191-004-0193-0
Dörgő G., Sebestyén V., Abonyi J. (2018). Evaluating the interconnectedness of the sustainable development goals based on the causality analysis of sustainability indicators. Sustainability 10, 3766. doi: 10.3390/su10103766
Gilberthorpe E., Papyrakis E. (2015). The extractive industries and development: the resource curse at the micro, meso and macro levels. extractive industries Soc. 2, 381–390. doi: 10.1016/j.exis.2015.02.008
Gojiya A., Deb D., Iyer K. K. (2019). Feasibility study of power generation from agricultural residue in comparison with soil incorporation of residue. Renewable Energy 134, 416–425. doi: 10.1016/j.renene.2018.11.003
Guerrero A. B., Muñoz E. (2018). Life cycle assessment of second generation ethanol derived from banana agricultural waste: environmental impacts and energy balance. J. Cleaner Product. 174, 710–717. doi: 10.1016/j.jclepro.2017.10.298
Hiloidhari M., Das D., Baruah D. (2014). Bioenergy potential from crop residue biomass in india. Renewable Sustain. Energy Rev. 32, 504–512. doi: 10.1016/j.rser.2014.01.025
International Energy Agency (2023) Bioenergy. Available at: https://www.iea.org/fuels-and-technologies/bioenergy (Accessed 26 Mar. 2023).
Krupnik T. J., Hossain M. K., Timsina J., Gathala M., Sapkota T., Yasmin S., et al. (2022). Adapted conservation agriculture practices can increase energy productivity and lower yield-scaled greenhouse gas emissions in coastal bangladesh. 4. doi: 10.3389/fagro.2022.829737
Kumar B., Verma P. (2021). Life cycle assessment: blazing a trail for bioresources management. Energy Conversion Manag.: X 10, 100063. doi: 10.1016/j.ecmx.2020.100063
Markussen M. V., Pugesgaard S., Oleskowicz-Popiel P., Schmidt J. E., Østergård H. (2015). Net-energy analysis of integrated food and bioenergy systems exemplified by a model of a self-sufficient system of dairy farms. Front. Energy Res. 3, 49. doi: 10.3389/fenrg.2015.00049
Munagala M., Shastri Y. (2020). Sustainable valorization of sugar industry waste: status, opportunities, and challenges. Bioresource Technol. 303, 122929. doi: 10.1016/j.biortech.2020.122929
Otoni C. G., Azeredo H. M., Mattos B. D., Beaumont M., Correa D. S., Rojas O. J. (2021). The food–materials nexus: next generation bioplastics and advanced materials from agri-food residues. Advanced Materials 33, 2102520. doi: 10.1002/adma.202102520
PIB Delhi (2021) Contribution of agriculture sector towards gdp. Available at: https://www.pib.gov.in/PressReleasePage.aspx?PRID=1741942 (Accessed 2 Apr. 2023).
Prasad S., Singh A., Korres N. E., Rathore D., Sevda S., Pant D. (2020). Sustainable utilization of crop residues for energy generation: a life cycle assessment (lca) perspective. Bioresource Technol. 303, 122964. doi: 10.1016/j.biortech.2020.122964
Samer M. (2019). Bioplastics production from agricultural crop residues. Agric. Eng. International: CIGR J. 21, 190–194.
Serpa S., Ferreira C. M. (2019). Micro, meso and macro levels of social analysis. Int’l J. Soc Sci. Stud. 7, 120. doi: 10.11114/ijsss.v7i3.4223
Shastri Y. (2017). Renewable energy, bioenergy. Curr. Opin. Chem. Eng. 17, 42–47. doi: 10.1016/j.coche.2017.06.003
Venkatramanan V., Shah S., Rai A. K., Prasad R. (2021). Nexus between crop residue burning, bioeconomy and sustainable development goals over north-western india. Front. Energy Res. 8, 614212. doi: 10.3389/fenrg.2020.614212
Walston L. J., Barley T., Bhandari I., Campbell B., McCall J., Hartmann H. M., et al. (2022). Opportunities for agrivoltaic systems to achieve synergistic food-energy-environmental needs and address sustainability goals. Front. Sustain. Food Syst. 6, 374. doi: 10.3389/fsufs.2022.932018
Keywords: circular bioeconomy, policy, bioplastics, biofuels, systems thinking
Citation: Hanumante N and Maitre N (2023) Can agro-biomass valorization be the fulcrum for sustainable development? Front. Agron. 5:1203543. doi: 10.3389/fagro.2023.1203543
Received: 10 April 2023; Accepted: 09 May 2023;
Published: 24 May 2023.
Edited by:
Arnab Bhowmik, North Carolina Agricultural and Technical State University, United StatesReviewed by:
Dipti Rai, North Carolina Agricultural and Technical State University, United StatesCopyright © 2023 Hanumante and Maitre. This is an open-access article distributed under the terms of the Creative Commons Attribution License (CC BY). The use, distribution or reproduction in other forums is permitted, provided the original author(s) and the copyright owner(s) are credited and that the original publication in this journal is cited, in accordance with accepted academic practice. No use, distribution or reproduction is permitted which does not comply with these terms.
*Correspondence: Neeraj Hanumante, TmVlcmFqLkhhbnVtYW50ZUBnbWFpbC5jb20=
†These authors have contributed equally to this work and share first authorship