- 1Environmental Science Program, Thompson Rivers University, Kamloops, BC, Canada
- 2Wildlife Research Division, Environment and Climate Change Canada, Delta, BC, Canada
- 3Department of Natural Resource Science, Thompson Rivers University, Kamloops, BC, Canada
Studies on habitat selection are central to our understanding of animal ecology and play an important role in the conservation and management of species. However, habitat selection is not necessarily consistent across sectors of populations and failing to understand both similarities and differences between age classes, for instance, may be problematic. Knowledge on habitat use by juvenile vertebrates in general is sorely lacking, particularly for species with precocial offspring, and here we address this gap by describing habitat use by juvenile Western Rattlesnakes (Crotalus oreganus). We measured habitat at two scales for radio-telemetered juvenile snakes along active-season movement paths at a site in southern British Columbia, Canada, where seasonal migrations and habitat associations of adult animals have been particularly well studied. Despite the relatively short distances these animals travelled and their diminutive size, we found there was selection for structurally stable cover (e.g., woody debris, shrub, and rock cover) similar to that documented for adult snakes in the same region. Additionally, we tested for differences in microhabitat features at sites used for short-duration (fewer than seven days) and long-duration (seven days or longer) stopovers: we detected negative selection for leaf litter at long-duration stopover sites, but otherwise identified no difference in the microhabitat features associated with these two categories of locations. Overall, this study contributes rare data to our growing understanding of the complexity of habitat requirements for migratory snake species, including northern crotalid vipers, while underscoring the crucial role of habitat selection research across all segments of populations. Comparative data on habitat selection by early-age classes within reptile populations informs conservation planning for the long-term survival of wild populations.
1 Introduction
In many species, patterns of movement and habitat use shift as animals age, presumably reflecting changes in resource needs, predator avoidance, life history strategies, or intraspecific competition (Werner and Gilliam, 1984; Blouin-Demers et al., 2007). Unfortunately, studies quantifying the ecology (including habitat use) of early age classes are sorely lacking in general across vertebrate taxa (e.g., aves – Cox et al., 2014; Dunn et al., 2017; marine megafauna – Hays et al., 2016; reptiles – Delaney and Warner, 2016; Vesy et al., 2021), and filling these gaps should be of paramount conservation concern (Roznik et al., 2009; Cox et al., 2014). Without an understanding of habitat use and selection by juvenile animals, our perception of a species’ habitat requirements becomes framed around knowledge of adult animals. In some cases, this may be acceptable, as in situations where there are long-term associations of different-aged individuals (e.g., colonial or herding species). However, in other species where gregarious behaviour is limited and offspring quickly become independent, resource use may differ notably between juveniles and adults (Delaney and Warner, 2016; Stillman et al., 2019). Understanding this level of variation in the ecology and habitat use of animals is important not only for developing a full appreciation of the natural history of species, but also for permitting effective planning and management of critical habitat (Roznik et al., 2009).
In snakes, juveniles become largely independent from adults early in life, setting conditions where divergent resource use may occur. Attaining adult size can take multiple years (Macartney et al., 1990), particularly for species living in highly seasonal environments (Fornasiero et al., 2016). This results in considerable differences in size between age-classes within many populations, raising the potential for younger, smaller animals to utilize different sets of resources for a significant portion of their life. An ontogenetic shift in diet is one such phenomenon that has received considerable attention: smaller prey, for example, may be required for juvenile animals that lack the capability to subdue and swallow larger prey (Hampton, 2011; Patterson et al., 2022); a notable example is neonate rattlesnakes in some populations that rely heavily on ectothermic prey (e.g., lizards) while adults use larger prey such as birds and mammals (Mackessy, 1988; Saviola et al., 2012). Of obvious importance is knowing whether ontogenetic patterns of habitat use exist, whether driven by diet, thermoregulatory opportunities, or other factors associated with body size (Shine et al., 2002; Blouin-Demers et al., 2007; Eskew et al., 2009). In situations where habitat partitioning between young and older snakes is not obvious and/or exists on a microhabitat scale, more subtle preferences in microhabitat selection may be revealed only through detailed study.
In Canadian populations of Western Rattlesnakes (Crotalus oreganus), migrations between hibernating sites and foraging grounds are conducted each year, with the animals typically associated with grassland and open forested habitats in the summer (Gomez et al., 2015; Harvey and Larsen, 2020). Within these landscapes, adult microhabitat selection as determined through radiotelemetry is largely described by proximity to cover (Macartney, 1985; Bertram et al., 2001; Gomez, 2007), with adults showing a particular preference for structurally stable cover objects (i.e., shrubs and rocks). Ectothermic prey is largely nonexistent at these northern latitudes, forcing young rattlesnakes to prey on smaller mammals within the habitat they share with adults, the latter also being able to take a more diverse array of prey species (Macartney, 1989; McAllister et al., 2016). Other than this difference in diet, scant details on the ecology of juvenile Western Rattlesnakes (and rattlesnakes in general) are known. Recently, however, we (Howarth et al., 2023) used externally-attached transmitters to show that smaller animals conduct shorter but similar movement patterns to adults over the active season, using ‘stopover’ points (Kaiser, 1999) by which to presumably conduct sit-and-wait bouts of hunting (Clark, 2016). This study also showed macro-habitat use (grasslands, open dry forest) was largely the same for both adults and juveniles.
In this paper we focus in on the meso- and micro-habitat selection of juvenile rattlesnakes at our Canadian study site during their summer migration. Specifically, we compare used stopover sites to nearby paired random sites to assess selection for various habitat variables. Further, we consider the overarching question of whether juvenile rattlesnakes select for similar habitat features on the landscape as have been recorded for adults in previous studies (Macartney, 1985; Bertram et al., 2001; Gomez, 2007). Following approaches taken in migration studies of birds, we also consider that stopover sites (Kaiser, 1999; Warnock, 2010) used for relatively longer periods of time may afford more desirable resources (Bastille-Rousseau et al., 2010). Although quantifying prey abundance at stopover sites is extremely difficult, we still improve on the resolution of our habitat analyses by dividing stopover points used by juveniles into ‘anchor’ (long-duration – Douglas et al., 2012) and ‘transient’ (short-duration) points.
2 Materials and methods
2.1 Study site
This study took place in the southeast corner of the Osoyoos Indian Reserve (OIR) near Osoyoos, British Columbia, Canada (49.05° N, 119.43° W);. This 450-ha study site is a dry arid shrub-steppe ecosystem dominated by Bluebunch Wheatgrass (Agropyron spicatum), Antelopebrush (Purshia tridentata), and Big Sagebrush (Artemisia tridentata). See Figure 1 for examples of typical habitat types on the OIR site, and see Howarth (2023) for a more detailed site description and site map. The study site contains drastic habitat quality contrasts (some sections are heavily altered from tourism development), though many snakes never encounter developed areas or leave developed areas completely during their active season (Lomas et al., 2019; Maida et al., 2020). We had two focal hibernacula on the OIR site that we targeted for capture of juvenile snakes; we hereafter refer to these as Den A and Den B (Indigenous designations in Nsyilxcən are iʔ x̌aʔx̌aʔulaʔxw iʔ q̓wc̓iʔs and iʔ x̌aʔx̌aʔulaʔxw iʔ snʔilitns ʔaslʔupnkst ʔɫ sisp̓lk, respectively). Both dens have among the highest relative populations on the OIR site (Howarth, unpubl.) and were selected in part to maximize the capture potential of suitable individuals during egress. Further, these dens were deliberately selected to minimize the likelihood of snakes venturing into developed areas, as observed by Lomas et al. (2019).
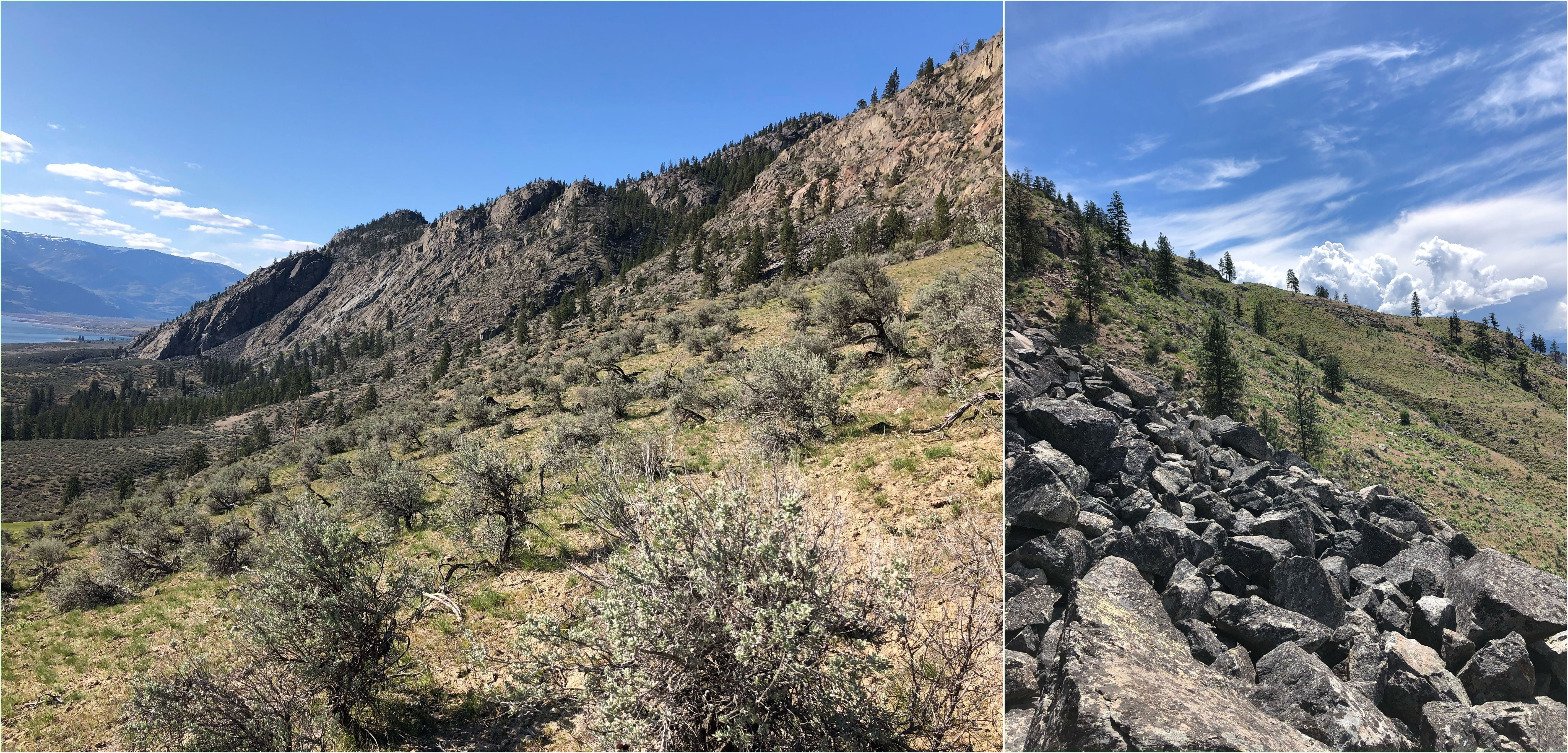
Figure 1 Examples of typical habitats found on the Osoyoos Indian Reserve (OIR) study site near Osoyoos, BC, Canada, where the habitat selection of juvenile Western Rattlesnakes was studied. Typical macro-habitats include open shrub-steppe (left), open forest stands (left), and rock piles or talus slopes (right).
2.2 Radio-telemetry
Juvenile snakes are relatively more difficult to encounter, particularly once the animals depart from communal hibernacula. Thus, most of our study animals were found by intensively searching the areas around Den A and Den B during egress (April–May). Individual snakes used in this study were involved in a larger concurrent study of juvenile Western Rattlesnake migratory movements (Howarth et al., 2023). We defined juvenile rattlesnakes as individuals in their second and third year of growth, which corresponds roughly to snakes with a snout–vent length (SVL) ranging from 35 to 45 cm, thus excluding all sexually mature individuals (Macartney et al., 1990; Petersen et al., unpublished). Transmitter size in relation to snake size was a major constraint in this study; hence we did not include individuals in their first year of growth (<35 cm SVL) as the appropriate transmitters would have had exceptionally short battery life spans (~two to four weeks).
Individuals were transported a short distance to a laboratory space and outfitted with radio-transmitters (BD-2, 1.0g, 5 week lifespan; Holohil Systems Ltd., Carp, Ontario). We followed the subdermal stitch attachment method described by Riley et al. (2017) (modified from Ciofi and Chelazzi, 1991) and since used in other studies (Hudson, 2019; Murphy et al., 2021). The subdermal stitch method involves running a subdermal catheter and thread under the subcaudal scales (under tail) and tie the transmitter to the dorsal surface (upper tail) of the snake. Likely less invasive than surgical implantation, the sub-dermal stitch method also allows for rapid non-invasive transmitter replacements as required by the lifespan of the batteries. The transmitters and associated attachment materials (catheter, suture) weighed no more than 5% of the total body mass of each snake. Snakes were administered a local anesthetic (1.5 mg/kg of bupicavaine) around the 20th subcaudal scale 15 minutes prior to the initial transmitter attachment (Riley et al., 2017), and immediately after were administered Baytril and Metacam (Brown et al., 2009). Snakes were monitored for 48 hours prior to release.
A handheld radio-telemetry receiver (TRX-3000; Wildlife Materials Inc., Murphysboro, Illinois) and a 3-element yagi antenna were used to track individuals, and the latitude/longitude coordinates of each snake were recorded using an SXBlue II+GPS device (Geneq Inc., Montreal, Quebec) that generally was accurate to ± 0.5 m. Radio-transmitter battery life was approximately five weeks; thus, snake recapture and transmitter replacement occurred on multiple occasions per individual over the course of the study. Telemetered snakes were located every two to three days over the course of the study until the point of transmitter removal, transmitter failure, accidental transmitter detachment, or natural mortality. Additionally, approximately every two to three weeks (coinciding with transmitter replacement, when possible), snakes were opportunistically re-captured and quickly inspected to monitor health; during these check-ups, the transmitter attachment and catheter protrusion sites were checked, and snakes were weighed (g) to ensure that no significant weight loss (>20% body mass) had occurred. If this was detected, the transmitter was removed.
2.3 Habitat data collection
Any shift in locations >5 m by a snake [half the distance used in Gomez’s (2007) study of adult habitat use, conservatively adjusted for juveniles] was considered a new stopover site and habitat data were collected. These data were not re-measured if a snake returned to a previously used stopover site or moved less than 5 m from their previous location. We also did not collect habitat data at locations that were within 10 m of the snake’s hibernaculum. Habitat data were collected using a paired use-availability design (Thomas and Taylor, 2006). At each rattlesnake stopover site, we set up one habitat plot and one paired plot at a randomly selected reference location. The paired plot was constrained within 30m from the habitat plot and was placed in a random direction at a random distance, selected using a number generator app (The Random Number Generator, Nicholas Dean, 2013). Following Gomez (2007), at each plot we directly measured three types of habitat features: (1) microhabitat (1 m2 plot); (2) mesohabitat (10 m2 plot), and (3) minimum distance to specific habitat features within the surrounding 30 m of plot centre (see Table 1).
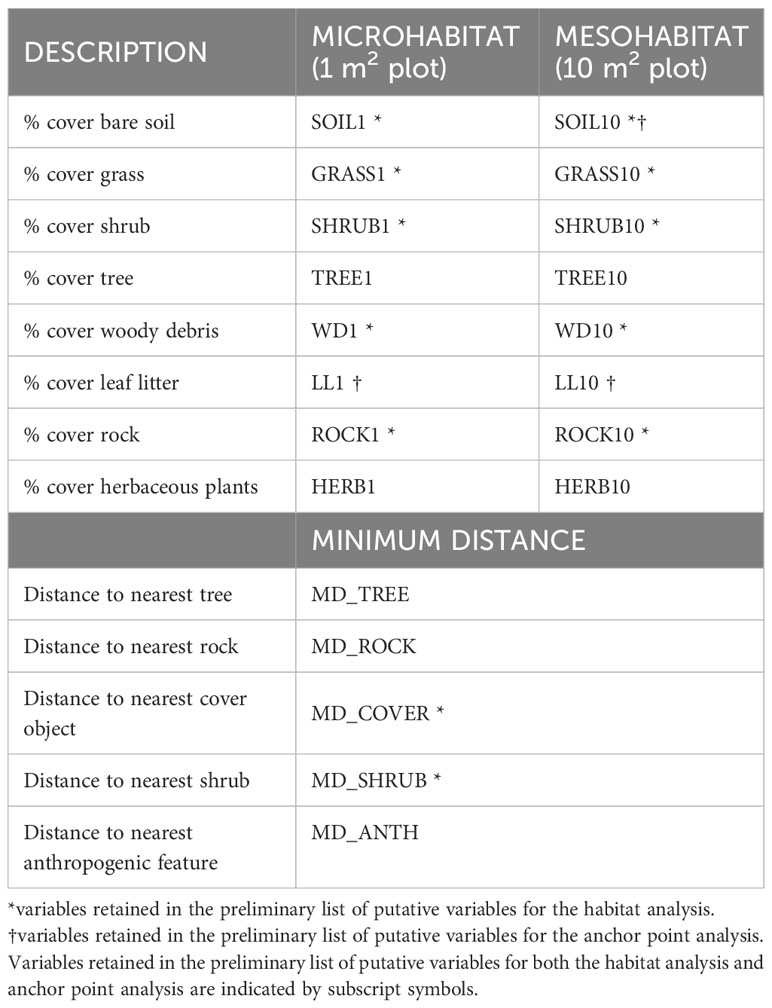
Table 1 Features measured in habitat plots centered at stopover sites used by juvenile snakes moving away from their hibernacula.
Microhabitat and mesohabitat features measured include the percentage cover of substrate, shrub, herbaceous plant, and rocks. For the microhabitat plot, percentage cover was visually estimated within a 1 m2 plot using the snake’s location, or the randomly selected reference location, as plot centre (Gardiner et al., 2015). For the mesohabitat plot, percentage cover was estimated within 10 m2 plot using the same method. Percentage cover estimates were performed by two observers (consistent throughout study) and were averaged in the case of inter-observer estimate discrepancy. The minimum distance to habitat features (e.g., tree, rock, shrub, anthropogenic features) within 30m of plot centre were measured to the nearest 1 m using a rangefinder (Bushnell Prime 1700, Bushnell Outdoor Products, Concord, Ontario); features were recorded as absent if not present within 30 m of plot centre.
2.4 Statistical analysis
All data were analyzed using R (R Version 4.2.0 – R Core Team, 2022) with α = 0.05 unless otherwise noted. We considered all habitat data as a single sample and did not consider the effect of season in our analysis. We used a series of univariable analyses to compare habitat features between habitat and paired plots. Normality was assessed using Shapiro-Wilk tests. We used arcsine- and log-transformations when variables were non-normal (for percentage cover and distance variables, respectively). If the resulting underlying distributions remained non-normal, we used untransformed values and non-parametric tests for all further analyses.
Following Hosmer and Lemeshow (2000), we established a list of putative explanatory variables: we used Wilcoxon signed-rank tests (paired sample) or paired t-tests and retained variables whose Wilcoxon signed-rank test or paired t-test had a P-value of <0.25 for use in subsequent analyses. We tested for autocorrelation between all retained habitat variables using Spearman rank correlation tests. When variables were highly correlated (ρ > 0.7), we retained only the variable that accounted for the most variation as indicated by the Wilcoxon signed-rank test or paired t-test (Mason and Perreault, 1991). We included all variables that were retained at this stage in the global multivariable conditional logistic regression models.
Habitat variables were modelled in two separate groups; microhabitat was modelled alone, while mesohabitat and minimum distance to habitat features were modelled together. We used conditional logistic regression with paired plots as strata, using the ‘clogit’ function in the ‘survival’ package (Therneau and Grambsch, 2000) in R (R Core Team, 2022). Top models were determined via Akaike Information Criterion (AIC) stepwise selection (Mazerolle, 2006) using the ‘stepAIC’ function in the ‘MASS’ package (Venables and Ripley, 2002) in R (R Core Team, 2022). Models with delta (∆) AIC values <2 were considered equally well-supported (Burnham and Anderson, 2002).
We performed more specific analysis on habitat points that received relatively greater use by the snakes. The duration of snake use was calculated for stays where a snake remained within a 5 m radius, confirmed through multiple tracking events. Locations where the duration of stay could not be confirmed, including locations where transmitters were removed, were excluded from this analysis. Locations in the upper 75th percentile, corresponding to stays of 7 or more days, were identified as ‘anchor points’. We then compared these anchor points, which received relatively long use, to ‘transient points’ that received less than seven days of consecutive use (lower 75% of locations). See Supplementary Figure 1 for distribution of duration of stays. All analysis was conducted in the same manner as described above; however, general logistic regression was used in place of conditional logistic regression as anchor and transient point plots were not paired.
3 Results
Twenty-one juvenile animals were captured for telemetry during this study, all at the target den sites, except four animals captured opportunistically in June (n = 3) and July (n = 1). Five of 17 denning-site animals remained <10 m from their hibernacula throughout their respective tracking periods (largely attributable to very short tracking periods as a result of transmitter removal) and thus were excluded from the habitat analysis. Among the remaining 16 animals, snakes were tracked for an average of 83 days (SD = 25; range = 45 to 140 days). Habitat data were collected between May 17 and September 29, 2021, though exact dates of tracking varied among individuals. The mean number of times that we tracked each snake to a new stopover location warranting a habitat plot was 8.6 (SD = 7.8; n = 137; range = 1 to 24 relocations). At the microhabitat scale (1 m2), the used stopover sites were dominated by rock (mean percentage cover: 41%), shrub (23%), grass (21%), soil (18%), and leaf litter (12%). At the mesohabitat scale (10 m2), the stopover sites were dominated by grass (mean percentage cover: 36%), rock (33%), shrub (19%), and soil (16%). All substrates not listed accounted for less than 10% cover each. Of 137 total snake locations, 122 locations were included in the anchor point analysis, and of those the top 75th percentile (n = 29) were identified as anchor points.
The underlying distributions of all habitat variables were non-normal even after transformations, so Wilcoxon signed-rank tests were used to establish the preliminary list of putative habitat variables. This resulted in retaining five microhabitat and seven mesohabitat variables (including two minimum distance features) for the habitat analysis (see Table 1). No pairs of retained variables showed autocorrelation. The preliminary multivariable conditional logistic regression models (hereafter, ‘global models’) for the microhabitat and microhabitat (including minimum distance to habitat features) were significant, as were the highest-ranked models at both scales (Table 2). Reduced models received stronger support at both microhabitat and mesohabitat scales. See Supplementary Table 1 for individual regression coefficients and P-values for the global models.
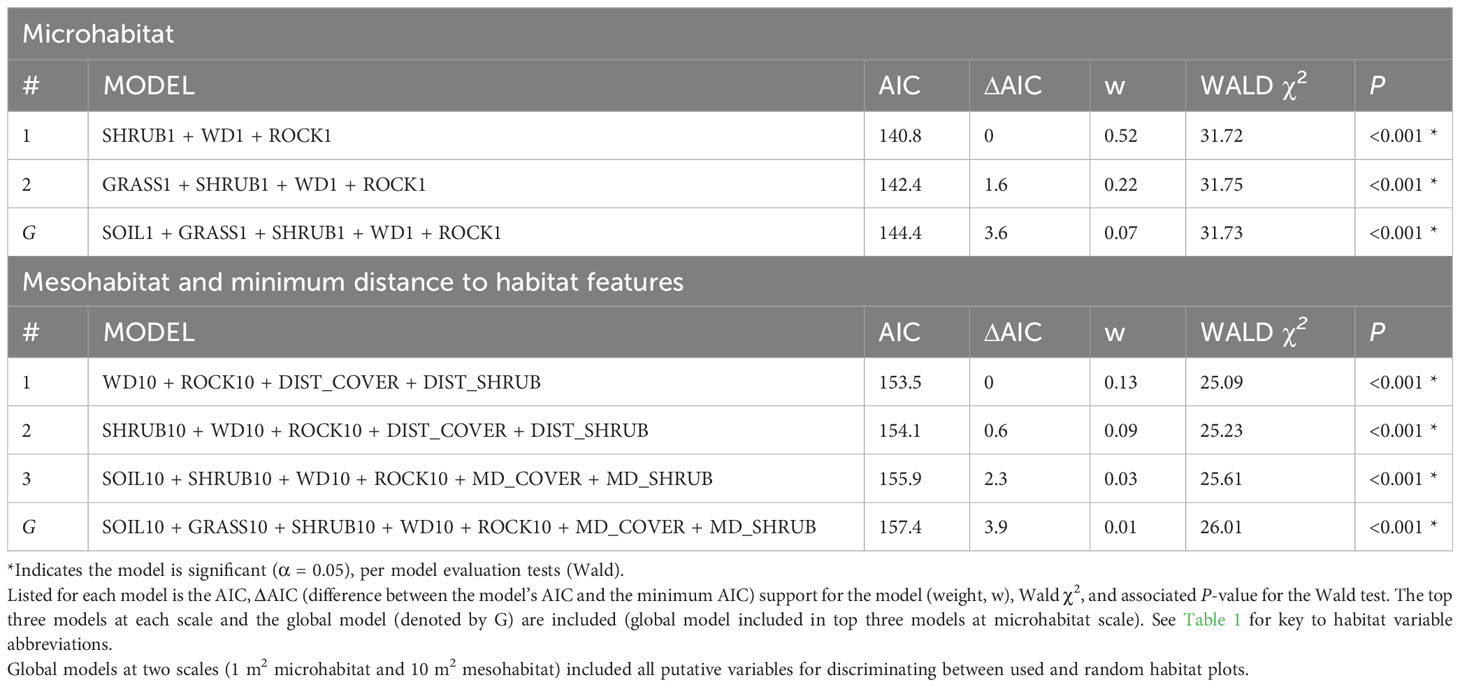
Table 2 Conditional logistic regression results for habitat assessments of stopover locations used by juvenile rattlesnakes.
At the microhabitat scale (1 m2) the top models suggested that the presence (positive association) of shrub, woody debris, and rock, and the absence (negative association) of grass predicted juvenile microhabitat selection (Figures 2, 3). At the mesohabitat scale (10 m2), the top models suggested that the presence (positive association) of shrub, woody debris, and rock, and smaller minimum distances to the nearest cover object and nearest shrub, together best predicted juvenile mesohabitat selection (Figures 4, 5). The preliminary list of putative explanatory variables for comparison of movement anchor points and transient points, using Wilcoxon-signed rank tests (retaining variables with a P-value of <0.25), resulted in only one variable (‘leaf litter’) being retained at the microhabitat scale, and at the mesohabitat level only two variables (‘leaf litter’ and ‘soil’) were entered into the analysis (see Table 1). No pairs of retained variables showed autocorrelation.
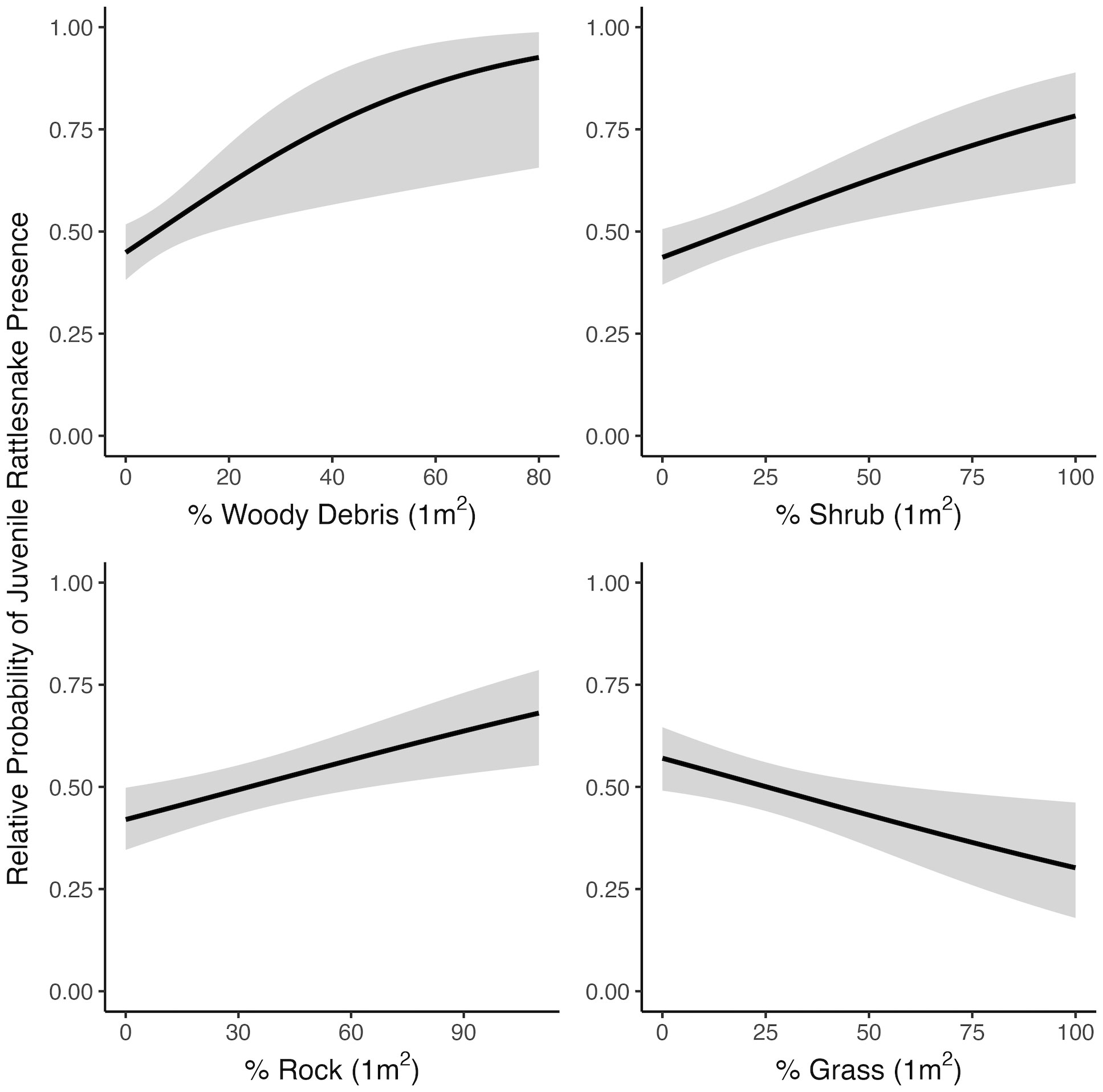
Figure 2 Relationships between juvenile rattlesnake habitat use and top-ranked habitat variables determined by selection models at the microhabitat (1 m2) scale, near Osoyoos, BC, Canada. Gray areas around regression lines represent 95% confidence intervals.
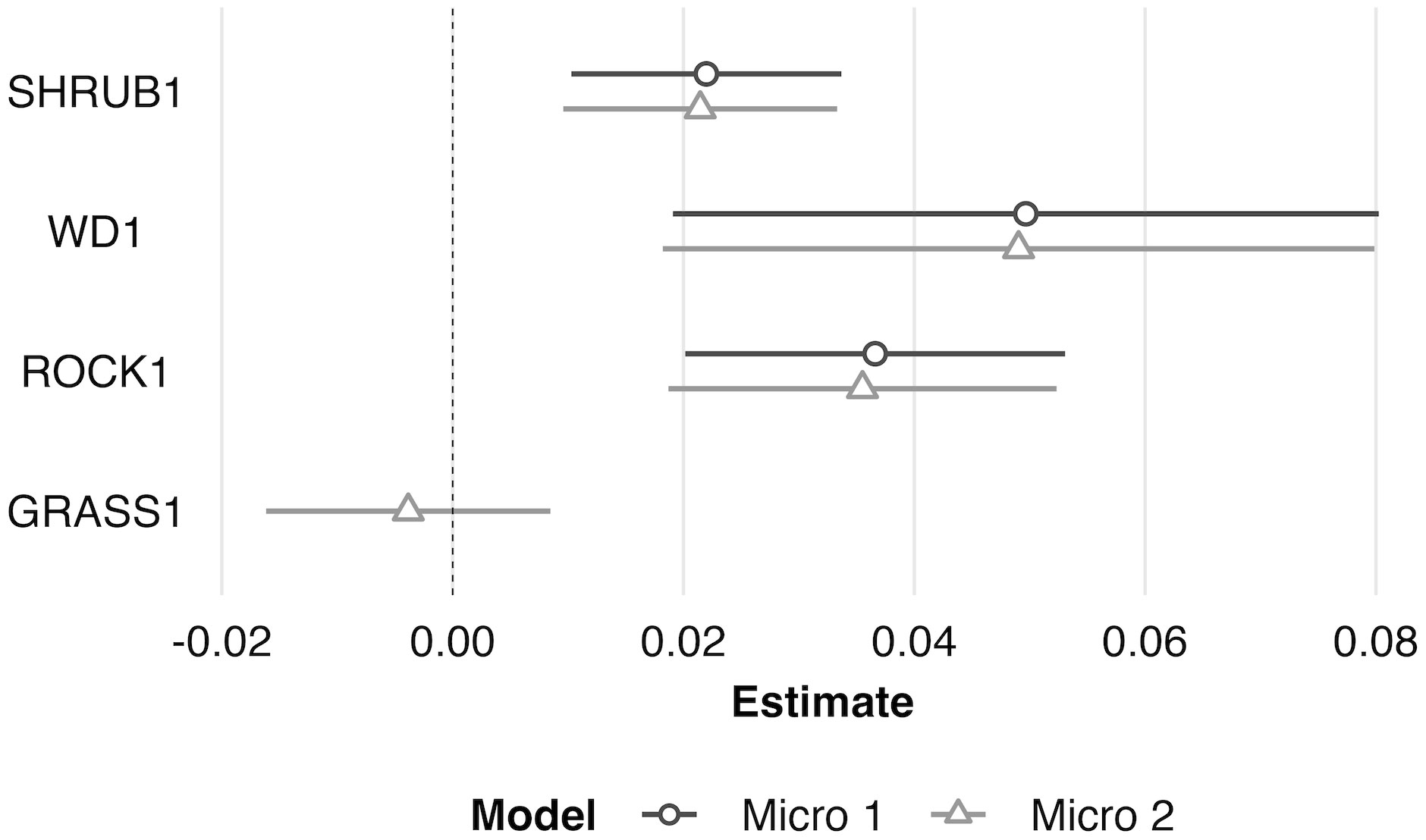
Figure 3 Scaled model coefficient estimates (logit scale with adjusted 95% CI) for the predicted microhabitat (1 m2) use by juvenile Western Rattlesnakes at the Osoyoos Indian Reserve (OIR) study site near Osoyoos, BC, Canada in 2021. Shown are the coefficient estimates for the top two models (Micro #1 and Micro #2, as indicated in Table 2). Positive coefficient estimates show active selection for habitat features, whereas negative estimates show avoidance. Scaled confidence intervals that do not overlap with the dashed line (i.e., 0) show significance. See Table 1 for key to habitat variable abbreviations.
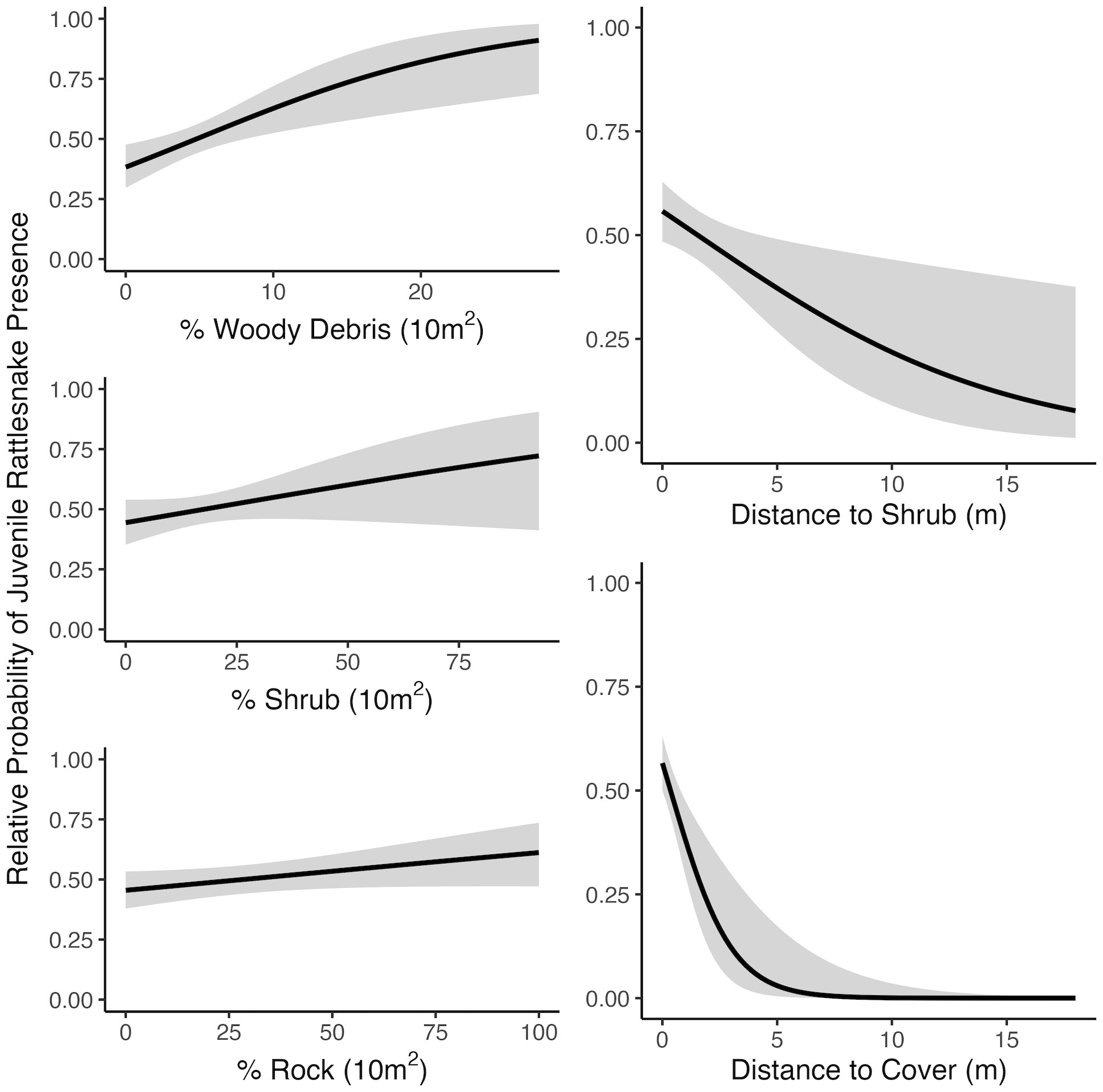
Figure 4 Relationships between juvenile rattlesnake habitat use and top-ranked habitat variables determined by selection models at the mesohabitat (10 m2) scale including minimum distance variables, near Osoyoos, BC, Canada. Gray areas around regression lines represent 95% confidence intervals.
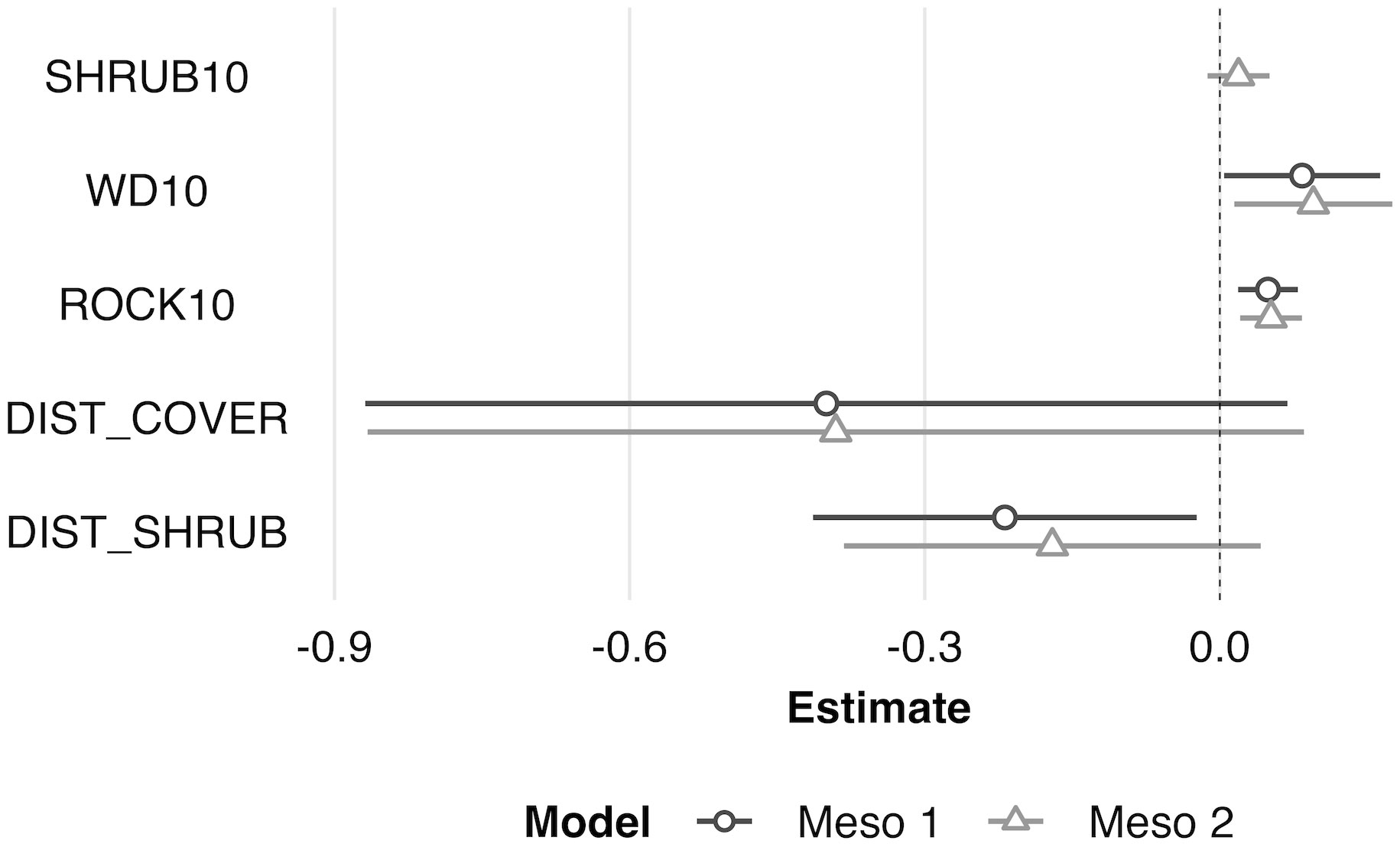
Figure 5 Scaled model coefficient estimates (logit scale with adjusted 95% CI) for the predicted mesohabitat (10 m2) use by juvenile Western Rattlesnakes at the Osoyoos Indian Reserve (OIR) study site near Osoyoos, BC, Canada in 2021. Shown are the coefficient estimates for the top two models (Meso #1 and Meso #2, as indicated in Table 2). Positive coeffi;cient estimates show active selection for habitat features, whereas negative estimates show avoidance. Scaled confidence intervals that do not overlap with the dashed line (i.e., 0) show significance. See Table 1 for key to habitat variable abbreviations.
The global multivariable conditional logistic regression models for comparison of anchor points and transient points (at both scales) are shown in Table 3 along with results of the stepwise AIC analysis. See Supplementary Table 2 for individual regression coefficients and P-values for the global models. At the microhabitat scale, the top model suggested that juvenile anchor point selection was negatively associated with the presence of leaf litter (Figure 6). At the mesohabitat scale, top models suggested that the absence (negative association) of leaf litter and soil predicted anchor point selection at the mesohabitat scale (Figure 6). However, the null model was also included within 2 delta AIC of the top model, and none of the models in the mesohabitat analysis were statistically significant.
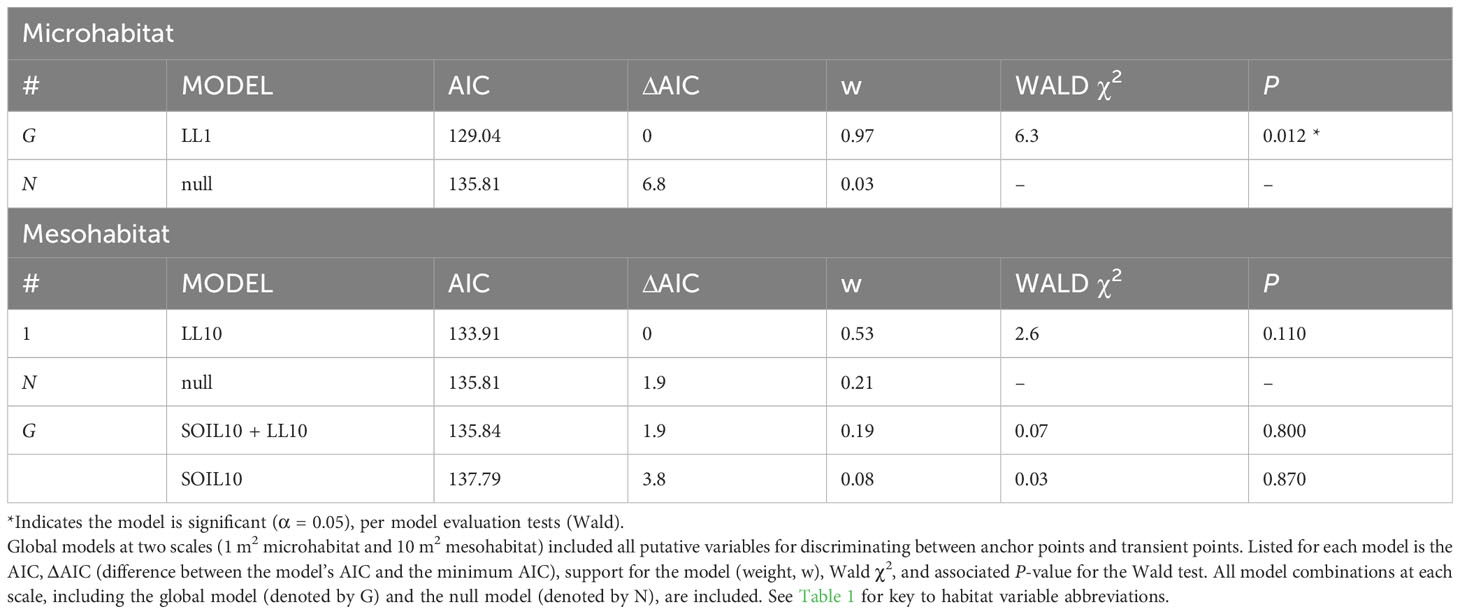
Table 3 Comparisons using logistic regression of habitat features at anchor points and transient points used by juvenile rattlesnakes over the active summer.
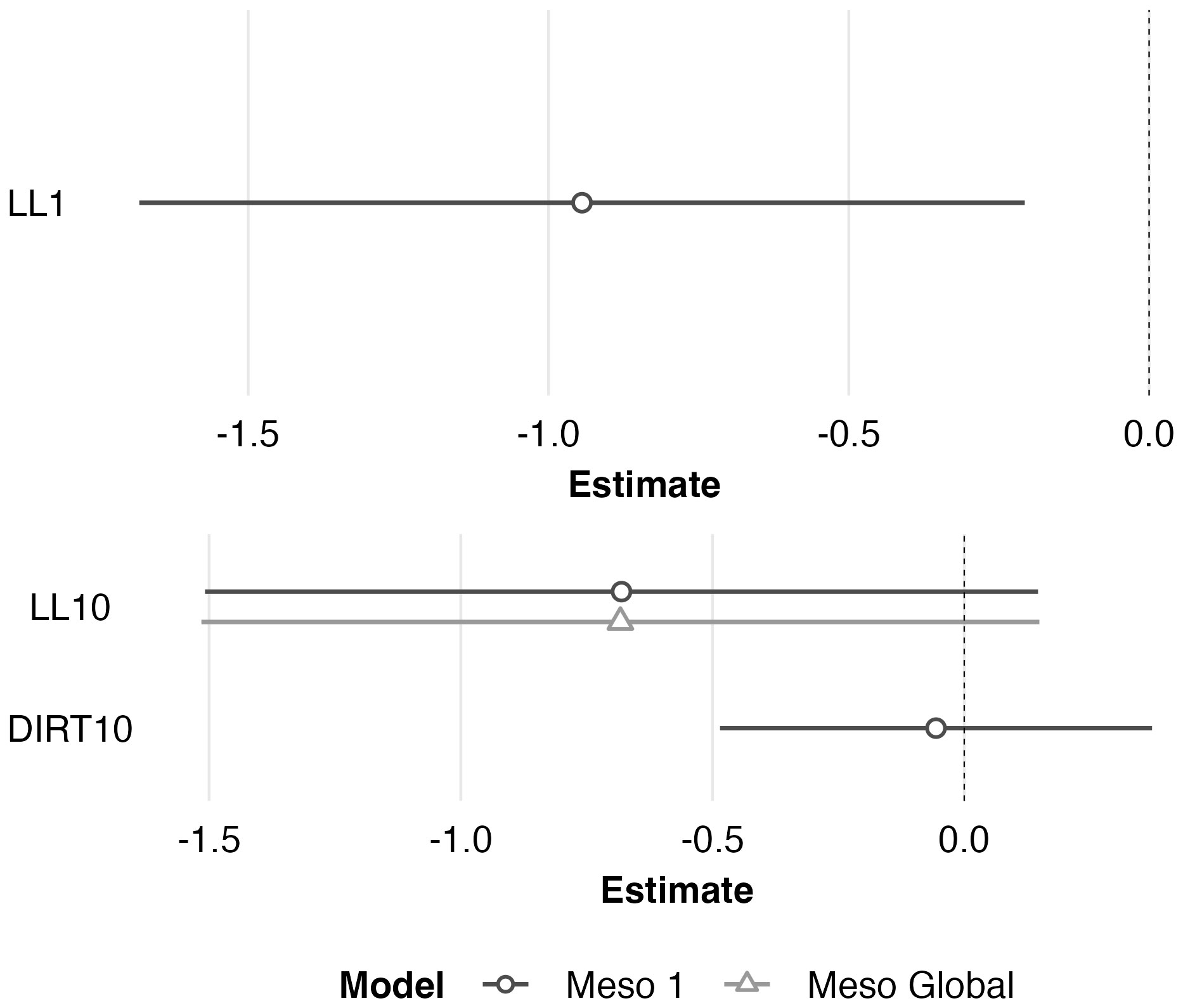
Figure 6 Scaled model coefficient estimates (logit scale with adjusted 95% CI) for the predicted microhabitat (1 m2; top) and mesohabitat (10 m2; bottom) use by juvenile Western Rattlesnakes at the Osoyoos Indian Reserve (OIR) study site near Osoyoos, BC, Canada in 2021. Shown are the coefficient estimates for the top microhabitat model, and the top two mesohabitat models (Meso #1 and Meso Global, as indicated in Table 2). Positive coefficient estimates show active selection for habitat features, whereas negative estimates show avoidance. Scaled confidence intervals that do not overlap with the dashed line (i.e., 0) show significance.
4 Discussion
The results of this study suggest that juvenile C. oreganus select for similar habitat features on the landscape as recorded for adults, despite their smaller size and smaller movement scale. Specifically, juvenile Western Rattlesnakes selected sites non-randomly at both scales for greater cover on the landscape in the form of rock, shrub, and woody debris, and selection was negatively associated with grass cover at the microhabitat scale. This suggests a preference for cover objects that are structurally stable, consistent with the preferred habitat of adult conspecifics elsewhere in British Columbia (Gomez, 2007). Habitat use was positively associated with the presence of cover not only at the microhabitat scale, but also at the mesohabitat scale, indicating that rather than finding a single isolated microhabitat site that fulfils their immediate needs (which in theory could be a very small cover object for these small-bodied individuals), juveniles may seek more heterogeneous patches of habitat that provide multiple opportunities for their ongoing hunting, crypsis, and thermoregulatory needs.
It is possible that the association of these small snakes to cover objects may be closely linked to the microhabitat selection (and therefore, availability) of prey on the landscape. For adult snakes, the selection of ambush sites is related to the presence of chemical cues left by prey (Theodoratus and Chiszar, 2000). With a paucity of small reptilian prey in the grasslands (e.g., lizards – a common feature in the ecology of the species further south; Mackessy, 1988), the diet of Western Rattlesnakes in British Columbia consists primarily of small mammals such as mice, voles, and shrews (McAllister et al., 2016). The primary prey species at our study site (Maida et al., 2020), the deer mouse (Peromyscus maniculatus) and Great Basin pocket mouse (Perognathus parvus), are also known to select for shrub cover, which can likely be attributed to food availability (Harris, 1986; Melaschenko and Hodges, 2020). Young and smaller-bodied rattlesnakes are largely limited to the smallest of these small mammals, presumably consuming neonatal and juvenile prey (Macartney, 1989). Thus, for these smaller snakes, it is likely important to base oneself in locations near small mammal nesting sites, though this remains unexplored.
We observed little difference in habitat features between sites used as anchor and transient stopover sites. Compared to transient points, juvenile snakes were using anchor point locations with lower leaf litter cover at both scales and with less bare soil cover at the mesohabitat scale, though neither the presence nor absence of these substrates provide any form of cover or opportunity for thermoregulation. The lack of association between anchor points and any of the measured habitat features may be explained by the ability of smaller snakes to make use of a wider variety of cover objects (Gregory, 2009) including small mammal nesting cavities, making suitable stopover sites for small snakes more prevalent on the landscape. Despite utilizing similar habitat types as adult rattlesnakes as suggested by the broader habitat use analysis, juvenile snakes may be able to find suitable locations to remain for extended periods of time more readily than adults, however, the specific habitat features used as anchor points by adults remains to be tested.
Although we obtained habitat data on 16 juvenile rattlesnakes, representing data over 137 stopover sites, we were still forced to pool data across individuals with variable tracking durations; this is a common issue in habitat selection studies that use animal relocations as experimental units (Marzluff et al., 2004; Thomas and Taylor, 2006). We attempted to average habitat features per snake and to then use the snakes as strata, but due to the variation and generally low number of locations for each individual, we were not able to fit individual models to each snake (Row and Blouin-Demers, 2006). As such, some caution should be taken in interpreting the results as they are based on the behaviour of a limited sample of snakes, and it is known that habitat use can differ markedly among individuals (Shine, 1987).
Further, we did not consider the effect of season in our analysis, and it is possible that habitat preferences may not be consistent throughout the active season. Other factors that may be at play, that we did not directly consider or account for here, include the effect of daily temperature and microclimate, the snakes’ physical condition, possible differences in personality and preference between individuals, nutritional state (hunting vs. digesting), and the presence of predators. We also did not incorporate cover object size into our analysis; in talus piles or other rock formations, it would in most cases have been impossible to deduce exactly what cover object an animal was under without flipping rocks, causing disruption and even danger to the animals. A finer-scale study across all age classes, incorporating cover object size, could expose ontogenetic shifts in cover object preferences should they exist. Regardless, this initial step in understanding the habitat preferences of immature snakes adds to our overall understanding of rattlesnake ecology. And, since selection for cover objects such as rocks and shrubs (or burrows, in prairie landscapes) appear relatively consistent for adults across North American rattlesnake species (e.g., Sistrurus catenatus – Harvey and Weatherhead, 2006; Crotalus mitchellii – Glaudas and Rodríguez-Robles, 2011; Crotalus viridis – Gardiner et al., 2015), the trends we observed in this study may also be consistent, or similar, for juveniles across rattlesnake taxa.
Understanding habitat preferences across all demographic groups within populations of wildlife is vital for effective management, as patterns of movement and habitat use can shift as animals age. Despite this, studies quantifying the ecology of early age classes are generally lacking across vertebrate taxa. Here, we address this considerable knowledge gap for an at-risk viper. By exploring the habitat preferences of young snakes and comparing them to what we know of adults, we shed light on the intricate dynamics of resource use within a species characterized by early independence. Ultimately, our study underscores the crucial role of habitat selection research and contributes to our broader understanding of the natural history and complexity of habitat requirements for rattlesnakes at northern latitudes. Recognizing the nuanced variations in habitat selection at different life stages can help to refine conservation efforts aimed at protecting critical habitats and ensuring the long-term viability of wildlife populations.
Data availability statement
The datasets presented in this study are filed with the BC Conservation Data Centre and are available through written request: https://www2.gov.bc.ca/gov/content/environment/plants-animals-ecosystems/conservation-data-centre.
Ethics statement
The animal study was approved by Thompson Rivers University Animal Care Committee. The study was conducted in accordance with the local legislation and institutional requirements.
Author contributions
CH, CB, and KL contributed to study conceptualization, methodology, project administration, and funding acquisition. Investigation, formal analysis, visualization, and writing (original draft) were performed by CH. CB and KL contributed to resources, supervision, and writing (review and editing). All authors contributed to the article and approved the submitted version.
Funding
This work was supported by Environment and Climate Change Canada, Aboriginal Fund for Species at Risk (#GCXE22C205), Natural Sciences and Engineering Research Council of Canada (NSERC), Habitat Conservation Trust Fund, Mitacs (#IT24608), Thompson Rivers University Undergraduate Research Experience Award Program, the Osoyoos Indian Band, Nk’Mip Desert Cultural Centre, and Spirit Ridge Resort (Hyatt Hotels Corporation).
Acknowledgments
We thank the Osoyoos Indian Band for providing access to the study site, laboratory facilities, and for their long-term commitment to rattlesnake research and conservation. We also thank the management and staff at the Nk’Mip Desert Cultural Centre, specifically C. Stringam and J. Bower for their logistical contributions and J. Herbert and B. Sabyan for their continued assistance and support. Data collection was aided by A. Wiebe and many other volunteers. Special thanks to D. Hill of Thompson Rivers University for the loan of the SX Blue GPS device. All rattlesnake handling, transportation, and surgical protocols were reviewed and approved by the Thompson Rivers University Animal Care Committee (File No. 101546). Data were collected under BC Wildlife Permit PE20-609553 and under federal Species-At-Risk Permit SARA-PYR-2021-0595.
Conflict of interest
The authors declare that the research was conducted in the absence of any commercial or financial relationships that could be construed as a potential conflict of interest.
This study received funding from Hyatt Hotels Corporation. The funder was not involved in the study design, collection, analysis, interpretation of data, the writing of this article or the decision to submit it for publication.
The author(s) KL declared that they were an editorial board member of Frontiers, at the time of submission. This had no impact on the peer review process and the final decision.
Publisher’s note
All claims expressed in this article are solely those of the authors and do not necessarily represent those of their affiliated organizations, or those of the publisher, the editors and the reviewers. Any product that may be evaluated in this article, or claim that may be made by its manufacturer, is not guaranteed or endorsed by the publisher.
Supplementary material
The Supplementary Material for this article can be found online at: https://www.frontiersin.org/articles/10.3389/famrs.2023.1251161/full#supplementary-material
References
Bastille-Rousseau G., Fortin D., Dussault C. (2010). Inference from habitat-selection analysis depends on foraging strategies. J. Anim. Ecology 79 (6), 1157–1163. doi: 10.1111/j.1365-2656.2010.01737.x
Bertram N., Larsen K. W., Surgenor J. (2001). Identification of critical habitats and conservation issues for the Western Rattlesnake and Great Basin Gopher Snake within the Thompson-Nicola region of British Columbia (Victoria, BC: British Columbia Ministry of Water, Land and Air Protection and the Habitat Conservation Trust Fund of British Columbia).
Blouin-Demers G., Bjorgan L. P. G., Weatherhead P. J. (2007). Changes in habitat use and movement patterns with body size in black ratsnakes (Elaphe obsoleta). Herpetologica 63 (4), 421–429. doi: 10.1655/0018-0831(2007)63[421:CIHUAM]2.0.CO;2
Brown J. R., Bishop C. A., Brooks R. J. (2009). Effectiveness of short-distance translocation and its effects on western rattlesnakes. J. Wildlife Management. 73 (3), 419–425. doi: 10.2193/2007-558
Burnham K. P., Anderson D. R. (2002). A practical information-theoretic approach. Model. selection multimodel inference. 2, 70–71. doi: 10.1007/b97636
Ciofi C., Chelazzi G. (1991). Radiotracking of Coluber viridiflavus using external transmitters. J. Herpetol. 25 (1), 37–40. doi: 10.2307/1564792
Clark R. W. (2016). “The hunting and feeding behavior of wild rattlesnakes,” in Rattlesnakes of arizona. Eds. Schuett G. W., Feldner M. J., Smith C. F., Reiserer R. S. (Rodeo, New Mexico, USA: Eco Publishing), 58–91.
Cox W. A., Thompson F. R., Cox A. S., Faaborg J. (2014). Post-fledging survival in passerine birds and the value of post-fledging studies to conservation. J. Wildlife Management. 78 (2), 183–193. doi: 10.1002/jwmg.670
Delaney D. M., Warner D. A. (2016). Age- and sex-specific variations in microhabitat and macrohabitat use in a territorial lizard. Behav. Ecol. Sociobiol. 70 (6), 981–991. doi: 10.1007/s00265-016-2121-3
Douglas D. C., Weinzierl R C., Davidson S., Kays R., Wikelski M., Bohrer G. (2012). Moderating Argos location errors in animal tracking data. Methods Ecol. Evolution. 3 (6), 999–1007. doi: 10.1111/j.2041-210X.2012.00245.x
Dunn J. C., Morris A. J., Grice P. V. (2017). Post-fledging habitat selection in a rapidly declining farmland bird, the European Turtle Dove Streptopelia turtur. Bird Conserv. Int. 27 (1), 45–57. doi: 10.1017/S0959270916000022
Eskew E. A., Willson J. D., Winne C. T. (2009). Ambush site selection and ontogenetic shifts in foraging strategy in a semi-aquatic pit viper, the eastern cottonmouth. J. Zoology. 277 (2), 179–186. doi: 10.1111/j.1469-7998.2008.00527.x
Fornasiero S., Bonnet X., Dendi F., Zuffi M. A. L. (2016). Growth, longevity and age at maturity in the European whip snakes, Hierophis viridiflavus and H. Carbonarius. Acta Herpetologica. 11 (2), 135–149. doi: 10.13128/Acta_Herpetol-18695
Gardiner L. E., Somers C. M., Parker D. L., Poulin R. G. (2015). Microhabitat selection by prairie rattlesnakes (Crotalus viridis) at the northern extreme of their geographic range. J. Herpetol. 49 (1), 131–137. doi: 10.1670/12-266
Glaudas X., Rodríguez-Robles J. A. (2011). A two-level problem: Habitat selection in relation to prey abundance in an ambush predator, the speckled rattlesnake (Crotalus mitchellii). Behaviour 148 (14), 1491–1524. doi: 10.1163/156853912x623739
Gomez L. M. (2007). Habitat use and movement patterns of the Northern Pacific Rattlesnake (Crotalus o. oreganus) in British Columbia (Victoria (BC: University of Victoria).
Gomez L., Larsen K. W., Gregory P. T. (2015). Contrasting patterns of migration and habitat use in neighboring rattlesnake populations. J. Herpetol. 49 (3), 371–376. doi: 10.1670/13-138
Gregory P. T. (2009). Variation in size of cover used by watersnakes (Nerodia): Do smaller snakes use smaller rocks? Acta Oecologica. 35 (2), 182–187. doi: 10.1016/j.actao.2008.09.008
Hampton P. M. (2011). Feeding performance in the Western Ribbon Snake (Thamnophis Proximus): ontogeny and the effects of prey type and size. Can. J. Zoology. 89 (10), 945–950. doi: 10.1139/z11-072
Harris J. H. (1986). Microhabitat segregation in two desert rodent species: the relation of prey availability to diet. Oecologia 68, 417–421. doi: 10.1007/BF01036749
Harvey J. A., Larsen K. W. (2020). Rattlesnake migrations and the implications of thermal landscapes. Movement Ecology 8 (1), 1–13. doi: 10.1186/s40462-020-00202-0
Harvey D. S., Weatherhead P. J. (2006). A test of the hierarchical model of habitat selection using eastern massasauga rattlesnakes (Sistrurus c. catenatus). Biol. Conserv. 130 (2), 206–216. doi: 10.1016/j.biocon.2005.12.015
Hays G. C., Ferreira L. C., Sequeira A. M. M., Meekan M. G., Duarte C. M., Bailey H., et al. (2016). Key questions in marineMegafauna movement ecology. Trends Ecol. Evolution. 31 (6), 463–475. doi: 10.1016/j.tree.2016.02.015
Howarth C. R. (2023). Western Rattlesnake (Crotalus oreganus) migration and habitat use in British Columbia, Canada (Kamloops, BC: Thompson Rivers University).
Howarth C. R., Bishop C. A., Larsen K. W. (2023). Western Rattlesnake (Crotalus oreganus) spring migration in British Columbia: A comparative study of juveniles and adults. Can. J. Zoology e-First, 0–11. doi: 10.1139/cjz-2022-0173
Hudson S. (2019). A multi-faceted approach to evaluating the detection probability of an elusive snake (Sistrurus catenatus) (Peterborough (ON: Trent University).
Kaiser A. (1999). Stopover strategies in birds: A review of methods for estimating stopover length. Bird Study. 46, S299–S308. doi: 10.1080/00063659909477257
Lomas E., Maida J. R., Bishop C. A., Larsen K. W. (2019). Movement ecology of northern pacific rattlesnakes (Crotalus o. oreganus) in response to disturbance. Herpetologica 75 (2), 153–161. doi: 10.1655/d-17-00060
Macartney J. M. (1985). The ecology of the northern pacific rattlesnake, Crotalus viridis oreganus, in British Columbia (Victoria (BC: University of Victoria).
Macartney J. M. (1989). Diet of the northern pacific rattlesnake, Crotalus viridis oreganus, in British Columbia. Herpetologica 45 (3), 299–304.
Macartney J. M., Gregory P. T., Charland M. B. (1990). Growth and sexual maturity of the western rattlesnake, Crotalus viridis, in British Columbia. Copeia 2, 528–542. doi: 10.2307/1446356
Mackessy S. P. (1988). Venom ontogeny in the pacific rattlesnakes Crotalus viridis helleri and C. v. oreganus. Copeia 1 (1), 92–101. doi: 10.2307/1445927
Maida J. R., Bishop C. A., Larsen K. W. (2020). Migration and disturbance: impact of fencing and development on western rattlesnake (Crotalus oreganus) spring movements in British Columbia. Can. J. Zoology. 98 (1), 1–12. doi: 10.1139/cjz-2019-0110
Marzluff J. M., Millspaugh J. J., Hurvitz P., Handcock M. S. (2004). Relating resources to a probabilistic measure of space use: Forest fragments and Steller’s Jays. Ecology 85 (5), 1411–1427. doi: 10.1890/03-0114
Mason C. H., Perreault W. D. (1991). Collinearity, power, and interpretation of multiple regression analysis. J. Marketing Res. 28 (3), 268–280. doi: 10.1177/002224379102800302
Mazerolle M. J. (2006). Improving data analysis in herpetology: Using Akaike’s information criterion (AIC) to assess the strength of biological hypotheses. Amphibia Reptilia. 27 (2), 169–180. doi: 10.1163/156853806777239922
McAllister J. M., Maida J. R., Dyer O., Larsen K. W. (2016). Diet of Roadkilled Western Rattlesnakes (Crotalus oreganus) and Gophersnakes (Pituophis catenifer) in Southern British Columbia. Northwestern Naturalist. 97 (3), 181–189. doi: 10.1898/NWN15-25.1
Melaschenko N. C., Hodges K. E. (2020). Density and habitat selection of mice on cheatgrass-invaded sites in British Columbia, Canada. J. Arid Environments. 181, 104242. doi: 10.1016/j.jaridenv.2020.104242
Murphy C. M., Smith L. L., O’Brien J. J., Castleberry S. B. (2021). Overwintering habitat selection of the eastern diamondback rattlesnake (Crotalus adamanteus) in the longleaf pine ecosystem. Herpetological Conserv. Biol. 16 (1), 203–210.
Patterson M., Wolfe A. K., Fleming P. A., Bateman P. W., Martin M. L., Sherrat E., et al. (2022). Ontogenetic shift in diet of a large elapid snake is facilitated by allometric change in skull morphology. Evolutionary Ecology 36, 489–509. doi: 10.1007/s10682-022-10164-x
R Core Team (2022). R: A language and environment for statistical computing (Vienna, Austria: R Foundation for Statistical Computing). Available at: https://www.R-project.org/.
Riley J. L., Baxter-Gilbert J. H., Litzgus J. D. (2017). A comparison of three external transmitter attachment methods for snakes. Wildlife Soc. Bulletin. 41 (1), 132–139. doi: 10.1002/wsb.748
Row J. R., Blouin-Demers G. (2006). Thermal quality influences habitat selection at multiple spatial scales in milksnakes. Ecoscience 13 (4), 443–450. doi: 10.2980/1195-6860(2006)13[443:TQIHSA]2.0.CO;2
Roznik E. A., Johnson S. A., Greenberg C. H., Tanner G. W. (2009). Terrestrial movements and habitat use of gopher frogs in longleaf pine forests: A comparative study of juveniles and adults. For. Ecol. Management. 259 (2), 187–194. doi: 10.1016/j.foreco.2009.10.007
Saviola A. J., Chiszar D., Mackessy S. P. (2012). Ontogenetic shirt in response to prey-derived chemical cues in prairie rattlesnakes Crotalus viridis viridis. Curr. Zoology 58 (4), 549–555. doi: 10.1093/czoolo/58.4.549
Shine R. (1987). Intraspecific variation in thermoregulation, movements and habitat use by Australian blacksnakes, Pseudechis porphyriacus (Elapidae). J. Herpetol. 21 (3), 165–177. doi: 10.2307/1564479
Shine R., Sun L.-X., Kearney M., Fitzgerald M. (2002). Why do Juvenile Chinese Pit-Vipers (Gloydius shedaoensis) Select Arboreal Ambush Sites? Ethology 108, 897–910. doi: 10.1046/j.1439-0310.2002.00824.x
Stillman A. N., Siegel R. B., Wilkerson R. L., Johnson M., Tingley M. W. (2019). Age-dependent habitat relationships of a burned forest specialist emphasise the role of pyrodiversity in fire management. J. Appl. Ecology 56 (4), 880–890. doi: 10.1111/1365-2664.13328
Theodoratus D. H., Chiszar D. (2000). Habitat selection and prey odor in the foraging behavior of western rattlesnakes (Crotalus viridis). Behaviour 137 (1), 119–135. doi: 10.1163/156853900501908
Therneau T. M., Grambsch P. M. (2000). Modeling survival data: Extending the cox model (New York: Springer).
Thomas D. L., Taylor E. J. (2006). Study designs and tests for comparing resource use and availability II. J. Wildlife Management. 70 (2), 324–336. doi: 10.2193/0022-541X(2006)70[324:SDATFC]2.0.CO;2
Venables W. N., Ripley B. D. (2002). Modern applied statistics with S. 4th ed. (New York: Springer).
Vesy M. N., Watters J. L., Moody R. W., Schauber E. M., Mook J. M., Siler C. D. (2021). Survivorship and spatial patterns of an urban population of texas horned lizards. J. Wildlife Management. 85 (6), 1267–1279. doi: 10.1002/jwmg.22064
Warnock N. (2010). Stopping vs. staging: The difference between a hop and a jump. J. Avian Biol. 41 (6), 621–626. doi: 10.1111/j.1600-048x.2010.05155.x
Keywords: Western Rattlesnake, Crotalus oreganus, juvenile, habitat selection, radiotelemetry, microhabitat
Citation: Howarth CR, Bishop CA and Larsen KW (2023) Microhabitat and stopover site selection by juvenile Western Rattlesnakes (Crotalus oreganus). Front. Amphib. Reptile Sci. 1:1251161. doi: 10.3389/famrs.2023.1251161
Received: 30 June 2023; Accepted: 08 November 2023;
Published: 22 November 2023.
Edited by:
Robert Jehle, University of Salford, United KingdomReviewed by:
David M. Green, McGill University, CanadaTrenton Garner, Zoological Society of London, United Kingdom
Ryan Hanscom, San Diego State University, in collaboration with reviewer TG
Copyright © 2023 Howarth, Bishop and Larsen. This is an open-access article distributed under the terms of the Creative Commons Attribution License (CC BY). The use, distribution or reproduction in other forums is permitted, provided the original author(s) and the copyright owner(s) are credited and that the original publication in this journal is cited, in accordance with accepted academic practice. No use, distribution or reproduction is permitted which does not comply with these terms.
*Correspondence: Chloe R. Howarth, aG93YXJ0aC5jckBnbWFpbC5jb20=