- 1Department of Chemistry and Physics, Warren Wilson College, Asheville, NC, United States
- 2Asheville Sustainability Research LLC, Asheville, NC, United States
- 3Forage-Animal Production Research Unit, United States Department of Agriculture, Agricultural Research Service, Lexington, KY, United States
Methane and ammonia are byproducts of rumen fermentation that do not promote animal growth, and methane is a key contributor to anthropogenic climate disruption. Cows eructate every few breaths and typically emit 250–500 L of methane gas daily. Significant research is focused on finding diets and additives that lower the production of methane and ammonia. Emerging research has shown that humulones and lupulones, molecules that are found in the cones of hops (Humulus lupulus), have potential in this regard. These molecules, which are also key flavor components in beer, are biologically active: they are known inhibitors of Gram-positive bacteria. Ruminants' sophisticated digestive systems host billions of microorganisms, and these systems' outputs will likely be affected in the presence of brewer's yeast (Saccharomyces cerevisiae). So-called spent yeast is produced during the beer-brewing process and contains humulones and lupulones in concentrations that vary by beer style, but it is generally discarded as waste. Our research suggests that adding spent craft brewer's yeast to rumen microbes by single time-point 24-h in vitro incubations suppresses production of methane and ammonia. This project examines the correlation between the quantities of hop acids in spent yeast and the production of methane and ammonia by bovine rumen microbes in vitro. We determined, by HPLC, the hop acid concentrations in spent yeast obtained from six beer styles produced at a local brewery. We performed anaerobic incubation studies on bovine rumen microbes, comparing the effects of these materials to a baker's yeast control and to the industry-standard antibiotic monensin. Results include promising decreases in both methane (measured by GC–FID) and ammonia (measured by colorimetric assay) in the presence of craft brewer's yeast, and a strong correlation between the quantities of hop acids in the spent yeast and the reduction of methane and ammonia. Notably, two of the yeast samples inhibited methane production to a greater degree than the industry-standard antibiotic monensin. Our results suggest that spent brewer's yeast has potential to improve ruminant growth while reducing anthropogenic methane emission.
Introduction
In ruminants such as cattle, the rumen is the first “stomach” in the gastrointestinal tract (Hungate, 1966). The biology of the rumen is rich and complex: this anaerobic environment is host to a variety of microorganisms that break down the cellulosic biomass that the animal eats, processing it into a form that can be absorbed by the mammalian host (Kim et al., 2011; Global Rumen Census Collaborators et al., 2015). However, not all of these microbes produce compounds of nutritional value to the host animal (Hungate, 1966). Two key waste products are methane (CH4) and ammonia (NH3), in processes that can be detrimental to the animal, the farmer, and the environment (Newbold and Rode, 2006; Flythe et al., 2017b). Methane and ammonia byproducts represent losses to the host animal of carbon and nitrogen, respectively, from which carbohydrates and proteins form (Newbold and Rode, 2006; Flythe et al., 2017b). For the farmer, this means that proportionally more feed is required for each unit of weight gain.
An individual cow emits 250–500 L of methane daily, and enteric fermentation constitutes more than half of agricultural methane emissions (Johnson and Johnson, 1995; Karakurt et al., 2012). There is a significant environmental cost represented by the methane byproduct, for methane is a powerful greenhouse gas that contributes to global warming: Methane has roughly 30 times the global warming potential of CO2 over 100 years (US EPA, 2016). Furthermore, methane has a 20-year global warming potential of 84 times that of CO2, meaning there would be a strong effect of cutting off its production on global temperature rise (Shindell et al., 2021).
The methane is produced by ruminal archaea; ammonia is produced by ruminal bacteria (Krause and Russell, 1996; Moss et al., 2000; Janssen and Kirs, 2008; Flythe, 2009; Knapp et al., 2014). Ionophore antibiotics such as monensin (marketed as Rumensin®) are fed in many large-scale agricultural operations to improve feed efficiency and to prevent diseases (Russell and Strobel, 1989; Ogunade et al., 2018). However, there are drawbacks to long-term usage of antibiotics. They can negatively impact rumen function by depressing fiber digestion, and there is strong selection pressure toward resistant organisms (Chen and Wolin, 1979).
Hops (Humulus lupulus) have potential as an alternative source of natural products for tuning the rumen microbial community (Flythe, 2009; Flythe and Aiken, 2010; Wang et al., 2010; Narvaez et al., 2013; Axman et al., 2015; Pszczolkowski et al., 2016; Flythe et al., 2017a,b). These molecules, best known as flavoring and bittering agents in beer, can be classified in three main categories: α-acids, β-acids, and iso-α-acids (Intelmann et al., 2011; Urban et al., 2013). These compounds are quite hydrophobic (Figures 1, 2), and thus tend to have low solubility in beer, and instead partition significantly into the yeast during brewing (Bryant and Cohen, 2015). At the end of the brewing process most of this yeast (called “spent yeast”) is disposed of, while only a small amount is saved for the next batch (Bryant and Cohen, 2015).
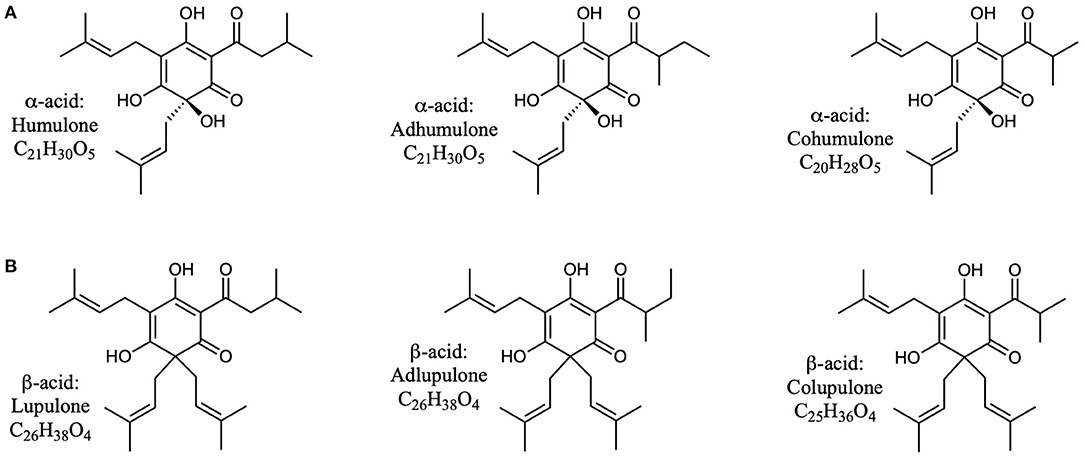
Figure 1. Structures of α- and β-acids found in hops (Humulus lupulus). (A) The three common α-acids found in hops. (B) The three common β-acids found in hops.
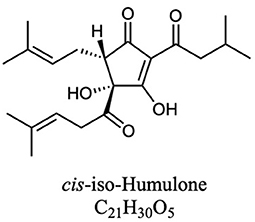
Figure 2. One representative structure of the iso-α-acids that form when the α-acids isomerize during the brewing process (Each humulone molecule in Figure 1A can produce four iso-humulone isomers. Lupulones do not isomerize in this way).
When used as a feed supplement, spent brewer's yeast is a source of protein and vitamins, potentially lowering feed costs for agricultural operations (Ferreira et al., 2010). When fed to ruminants, spent yeast can have interesting effects distinct from its nutritional properties. Brewer's yeast, particularly from craft breweries that emphasize highly hopped styles such as India Pale Ales, contains significant quantities of the α-, β-, and iso-α-acids (Bryant and Cohen, 2015). These molecules have the privileged ability to suppress the activity of methane- and ammonia-producing microbes that reside in the anaerobic environment of the rumen (Pszczolkowski et al., 2016; Flythe et al., 2017b).
Repurposing spent craft brewer's yeast as a feed additive for ruminants could increase feed efficiency and decrease the need for antibiotics. Spent brewer's grain is well-established as a beneficial protein supplement for cattle and other livestock, as is spent yeast from multi-national breweries which has been characterized as having low levels of hop metabolites (Grieve, 1979; Mussatto et al., 2006; Bryant and Cohen, 2015).
There is little data quantifying the effects of craft brewer's yeast as a ruminant feed supplement. The methane- and ammonia-tuning effects of using this material may be heavily dependent on the amount of the various hop acids in a beer, which depends on a range of factors. For example, there are dozens of hop varietals with intrinsic differences in hop acid concentrations. When the hops are harvested and how they were processed also plays a factor, as well as the hopping procedures of each brewery (Matsui et al., 2016).
In order to further study the viability of craft brewery waste as a potential feed supplement, the correlation between hop acid levels and methane-suppressing properties in these materials needs to be better understood. We obtained spent yeast, comprising a variety of different beer styles and hopping levels, from a brewery in Asheville, NC. Using HPLC, we quantified the different hop acids found in these samples. We then examined the in vitro ability of these samples to suppress methane and ammonia produced by bovine rumen fluid in the presence of a substrate of silage and ground corn using single time-point 24-h experiments. Comparisons were made to the levels of hops found in each spent yeast sample, using baker's yeast as a nutritionally similar, hop-free control, along with comparisons to monensin using the same microbial base.
Materials and Methods
Chemicals
Solvents and chemicals were purchased from Fisher Scientific unless otherwise noted. HPLC-grade solvents were used whenever possible; 99%+ purity otherwise. High-purity argon and carbon dioxide compressed gases were obtained from Andy Oxy Co. Inc, Asheville, NC and had <10 ppm oxygen.
Spent Yeast Sampling
Spent craft yeast samples were collected from a single brewery in Asheville, NC. Yeasts (Saccharomyces cerevisiae) were collected at the end of the fermentation stage from beer styles comprising low- to high-hopped ales such as India Pale Ales (IPA). Data including beer variety, brew date, and batch number were recorded. Yeast samples were collected in 1-quart plastic containers and kept refrigerated. Upon return to Warren Wilson College the samples were pasteurized for 3 min at 63–65°C in order to prevent live yeast from potentially interfering with rumen microbial growth. After pasteurization the yeast samples were chilled to 4°C, centrifuged, for 10 min at 4000 × g and pellets stored in a −80°C freezer until extraction. Moisture content of the pastes, typically 60% to 70%, were determined by taking samples to constant weight over 3 h in a 105°C oven.
Incubation Media
Before rumen fluid collection (generally on the day prior), a basal suspension media was prepared to contain KH2PO4 (240 mg/L), K2HPO4 (240 mg/L), (NH4)2SO4 (480 mg/L), NaCl (480 mg/L), CaCl2•2H2O (64 mg/L), MgSO4•7H2O (100 mg/L), and NaHCO3 (4 g/L) (Flythe, 2009). The media was autoclaved for 45 min and sparged with CO2 during cooling. After cooling, cysteine•HCl (0.6 g/L) was added while sparging with CO2 and then the jar was sealed with a rubber stopper. The pH of the final media was adjusted to 6.5–6.7.
Feed substrate was a 2:1 (by mass) mixture of ground dried silage (provided by Dr. Deidre Harmon, North Carolina State Univ.) and stone-ground cornmeal. Glass jars for incubation were charged with 300 mg of this substrate and (as necessary) 50 mg of dried spent craft yeast or Red Star baker's yeast. Jars were then sparged with CO2 and capped with a rubber stopper. Jars for each treatment were prepared in triplicate.
A 7 mg/mL solution of monensin sodium (Alfa Aeser, 90+%) was prepared in 95% ethanol and stored between 0 and −20°C until use.
Rumen Fluid Isolation and Incubations
Rumen fluid samples were collected at slaughter from beef cattle at the Wells Jenkins and Wells abattoir in Forest City, NC. When possible, Warren Wilson College pastured steers were used. This study comprises experiments from the rumen fluid of four animals Immediately after the animal was processed, the rumen was cut open with a box cutter. Rumen fluid was collected with a ladle and filtered through four layers of cheesecloth into a 750 mL thermos with a tight-fitting lid containing 1 g of inulin and sealed. During transport to the lab, the rumen fluid digested the inulin, forming an anaerobic atmosphere; the bottle was invariably under pressure when opened in the laboratory.
In the lab, rumen fluid was added to an equal volume of cell suspension buffer and stirred under a gentle stream of CO2. To each glass 125 mL media bottle (Wheaton) was added 10 mL of the 1:1 rumen fluid–suspension buffer mixture under a gentle stream of CO2. To each was added 10 μL of 95% ethanol, either with or without dissolved monensin, as appropriate. Bottles were then capped with tight-fitting fold-over rubber stoppers. Bottles were incubated at 39°C with occasional agitation in an incubator. After 24 h, samples for all measured variables were removed. Headspace methane analysis and pH were performed immediately; solutions were frozen at −80°C until the ammonia analyses were performed.
Methane Analysis
Methane was analyzed from headspace gas samples taken from each flask using a gas-tight syringe at the post-incubation sample time after bottles had cooled to room temperature. Headspace gas (50 μL) was removed from each flask using a gastight syringe and then injected into a GC–FID for analysis (Eun et al., 2004; Pszczolkowski et al., 2016). The instrument used was an HP 6890 GC fitted with a 30 m × 0.53 mm I.D. column packed with HayeSep-D porous polymer coated on 20 μm particles (Valco Instruments, Houston, TX). The back inlet temperature was maintained at 100°C, the oven at 50°C, and the flame ionization detector at 250°C. A standard curve for methane was obtained using 94% methane utility gas (PSNC Energy, Asheville, NC). Analysis was generally done in triplicate and an average taken. Methane production after 24 h was expressed as parts per million (ppm) in the headspace gas which occupied a volume of 141 mL. A value of 10,000 ppm of methane translates to 1.66 mL methane at STP for measurement conditions at Warren Wilson College, Asheville, NC, T = 23°C, P = 94 mPa.
Ammonia Analysis
Ammonia was analyzed with a Plate Reader (Infinite M200 Pro, TECAN Instruments). Two stock reagent solutions were prepared: one containing 25 g of phenol and 0.125 g of sodium nitroferricyanide; and one containing 12.5 g of sodium hydroxide and 8.4 mL of 6% sodium hypochlorite (from a commercial bleach solution); both were diluted with distilled water to 500 mL (Chaney and Marbach, 1962).
Standards of 0, 0.27, 0.54, 1.09, 2.17, and 4.35 mM NH3 were made using ammonium monobasic phosphate in distilled water. For samples, 100 μL of fluid was diluted with 900 μL of water and the material was centrifuged for 3 min at 12,000 rpm.
For analysis, 100 μL of the phenol/sodium nitroferricyanide solution and 100 μL of the sodium hypochlorite solution were added to 10 μL of standard or sample in a 96 well microtiter plate and mixed by gentle agitation of the plate. The plate was covered and incubated at 39°C for 30 min and read at 630 nm. The initial concentration of ammonia in the buffer solution was 7.3 mM ammonium [from 480 mg/L (NH4)2SO4], and this was subtracted from the ammonia concentration found by the assay in order to determine the amount of ammonia produced during fermentation.
Statistical Analysis
Methane and ammonia production were each analyzed by comparing baker's yeast, craft yeast, or baker's yeast + monensin additives using two-tailed t-tests.
Agar Diffusion Assay
A Petri plate bioassay was modified from Kagan and Flythe (2014). The growth medium was a carbonate-based semi-defined broth (Chen and Russell, 1989). The indicator culture was Acetoanaerobium sticklandii strain SR. Chain of custody: Isolated by J. Russell (Chen and Russell, 1989), transferred to M. Flythe in 2006. The growth medium was thermally degassed in an autoclave and cooled under CO2, as described for the incubation buffer. The medium was anaerobically dispensed into serum bottles containing agar (15 mg/mL final conc.). The bottles were stoppered with butyl rubber stoppers, sealed with aluminum crimps and autoclaved for sterility (121°C, 15 min). The growth media bottles were amended with a sterile, anaerobic stock solution of a pancreatic digest of casein (Trypticase; Fisher BioReagents, Fair Lawn, NJ; 15 mg/mL final conc.) as a growth substrate. When the bottles reached pouring temperature (~50°C) the agar was inoculated with an overnight culture of A. sticklandii SR (2% v/v). The bottles were rapidly brought into an anaerobic chamber (Coy, Grass Lake, MI; 95% CO2, 5% H2) and the agar was poured into sterile Petri plates. The agar was permitted to solidify (RT, 1 h).
Wells were made in the center of agar plates with reverse aperture of a sterile Pasteur pipette. Spent craft yeast, control yeast or a hops extract was loaded into the wells. The hops extract was donated by the manufacturer (S.S. Steiner, Inc., Yakima, WA) and contained <1% alpha-acid and 30.7% beta-acid per the label. The extract was diluted in growth media (30 μg/mL final conc. beta-acid) before loading. The plates were incubated (39°C, 24 h) in the anaerobic chamber to permit growth. They were stained with tetrazolium red to visualize living A. sticklandii SR cell growth. The dye was composed of 2,3,5-triphenyltetrazolium chloride (Sigma Aldrich, MO) 1% w/v in water. The dye was allowed to develop for ~20 min, then the plates were removed from the anaerobic chamber for photography.
Extraction of Hop Acids
Craft yeast samples were removed from the freezer and put into a warm water bath. Yeast paste (1 g) of yeast paste was weighed into a 15 mL polypropylene centrifuge tube followed by 3 mL of a methanol:water:phosphoric acid (100:10:1) mixture. The tubes were vortexed vigorously and put in a sonic bath for 10 min, followed by addition of 6 mL of methanol and 10 additional minutes of sonication. Samples were typically centrifuged at 12,000 rpm in a microfuge prior to injection.
HPLC Analysis of Hop Acids
Hop Acid Standards
A supercritical CO2 extract paste containing 56.3% α-acids and 18.6% β-acids was kindly provided by Hopsteiner (New York, NY). A sample of trans-iso-α-acids as DCHA salts was obtained from the American Society of Brewing Chemists (St. Paul, MN), and xanthohumol was kindly provided by Prof. Fred Stevens, OSU, Corvallis, OR. Stock solutions were prepared in DMSO and stored at −20°C; stocks were diluted in methanol for analysis.
HPLC Instrument
All HPLC work was done with a Shimadzu system with a SPD-20A/20AV UV/Vis dual wavelength detector, LC-10ATVP pump, FCV-10ATVP gradient mixer, and a Restek, Pinnacle II, 5 μ, C18, 150 X 4.6 mm column. Samples were injected with a Rheodyne 7125I Injector Valve fitted with a 20 μl loop.
HPLC Elution and Detection
Mobile phase A was water:methanol:phosphoric acid, 50:50:0.5 by volume and mobile phase B was methanol. The run began at 50% B for 4 min, ramping via linear gradient to 100% B at 8 min, and held there until 10 min. The gradient was then reset to 50% B at 11 min. The flow rate was maintained at 1 mL/min. UV absorbances were measured at 270 nm (iso-α-acids, α-acids and β-acids) and 340 nm (xanthohumol, α-acids and β-acids) using a Vernier instrument amplifier, recorded and analyzed using Logger Pro software, Vernier Software and Technology, Beaverton, OR.
Results
Our previous research had established that hop-rich spent yeast inhibited methane production by bovine and caprine rumen microbes in the presence of a fructose substrate (Pszczolkowski et al., 2016). We wanted to follow up these results to better understand their potential applicability. For this study, we switched to a 2:1 mixture of ground silage and cornmeal—a more representative carbohydrate source in a ruminant's diet—as a fermentation substrate. Furthermore, hop β-acids have been shown to inhibit ammonia production by ruminal bacteria (Flythe, 2009; Flythe et al., 2017b). We therefore examined the production of both methane and ammonia under these conditions.
Inhibition of Production of Methane and Ammonia by Hop Acids
We compared the effects of pasteurized spent craft yeast to the industry-standard ionophore antibiotic, monensin (Rumensin®) on methane and ammonia production in vitro. Rumen fluid was obtained immediately after slaughter from an 18-month-old steer. This was mixed with buffer and substrate and incubated for 24 h in an atmosphere of CO2, which produced measurable amounts of methane under all conditions examined. Headspace methane concentrations were analyzed by GC–FID, and ammonia concentrations were analyzed using a colorimetric assay. Substrate was supplemented with baker's yeast only, craft brewer's yeast only, or baker's yeast and monensin; all supplements contained comparable amounts of protein. Results are shown in Figure 3.
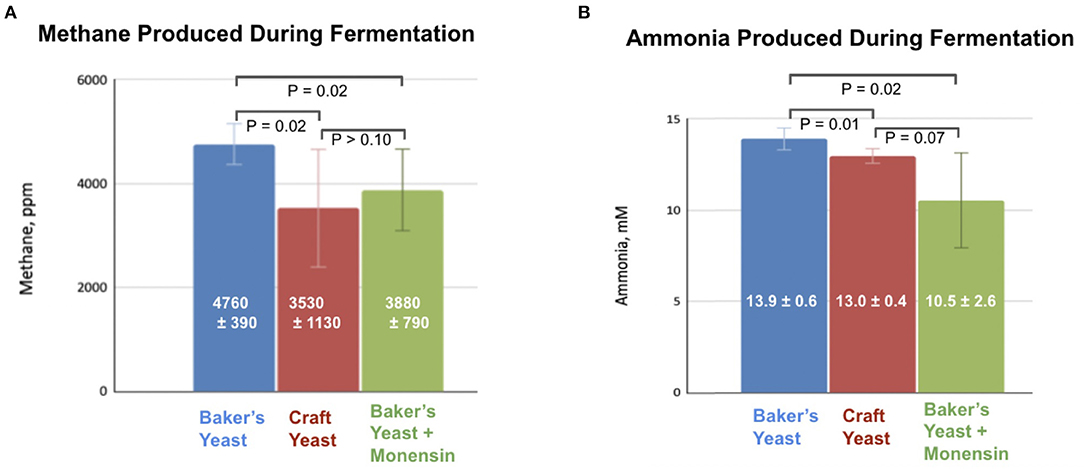
Figure 3. Addition of monensin or switching to hop-rich craft brewer's yeast inhibits production of (A) methane and (B) ammonia during anaerobic fermentation of a silage/corn substrate and a yeast supplement.
There was a higher methane concentration produced by the samples treated with baker's yeast samples than in either the craft brewer's yeast or monensin samples; these latter treatments produced statistically indistinguishable concentrations of methane (Figure 3A). Likewise, ammonia produced by the craft-yeast and monensin-treated microbes was lower than for baker's yeast-treated microbes, but not statistically different from each other (Figure 3B).
Inhibition of Growth of Hyper Ammonia-Producing Bacteria
The previous data show that craft brewer's yeast decreased rumen fermentation end products, which could be due to a number of factors. Figure 4 represents a bioassay showing that craft brewer's yeast inhibits growth of the anaerobe Acetoanaerobium sticklandii SR. This bacterium is a well-known amino acid-fermenter and ammonia-producer that was originally used to explain the mechanism of action of monensin on rumen ammonia (Chen and Russell, 1989). This indicates that reduction of the ammonia end product could be due to growth inhibition of specific rumen microorganisms.
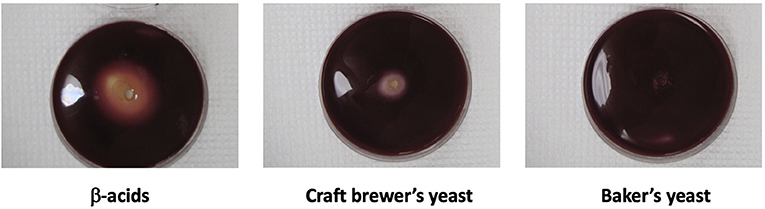
Figure 4. Acetoanaerobium sticklandii SR, a hyper ammonia-producing bacterium isolated from the bovine rumen, was inoculated into molten agar and grown anaerobically within the agar. Pasteurized yeast slurry, equivalent to 5–10 mg dry yeast, was placed in center wells. Bacteria were stained with the vital dye, Tetrazolium Red. β-Acids, 30 mg/ml, were a positive control.
HPLC Analysis of Hop Acids
We assayed spent craft yeast harvested from different ale-style beers to determine the contents of the biologically active hop acids. A representative HPLC trace of an extraction of spent craft brewer's yeast is shown in Figure 5. Two bands are seen for the α-acids (360–470 s): the first is the co-humulone isomer, and the second is the coeluting humulone and adhumulone isomers, which have an additional methylene group and are therefore more hydrophobic (Figure 1A). A similar pattern is seen for the β-acids (520–570 s) with a band for colupulone and then a second band for the coeluting lupulone and adlupulone. The more polar iso-α-acids elute first (170–230 s). These show up as two broad bands; a total of six pairs of cis and trans isomers are present following the conversion of α-acids to iso-α-acids (Figure 2). The bands were integrated, and the α-acids, β-acids and iso-α-acids clusters were each aggregated. Table 1 shows the concentration of hop acids found in the spent yeasts from six different styles of beer.
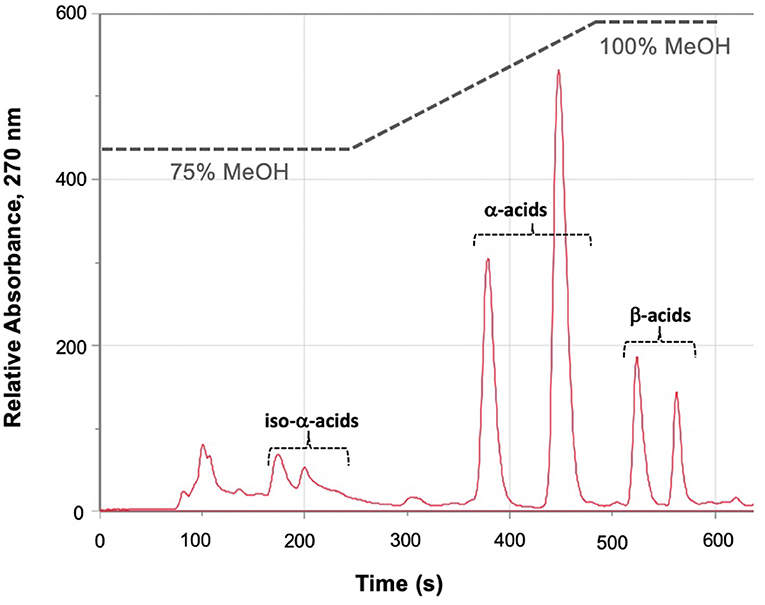
Figure 5. Representative HPLC trace, A270, of the extract of spent yeast from a hoppy ale-style beer. Iso-alpha acids elute as a cluster between 180 and 210 s; cohumulone elutes at ~390 s; humulone and adhumulone coelute at ~450 s; colupulone elutes at ~520 s; lupulone and adlupulone coelute at ~560 s.
Correlation Between Hop Acid Concentration and Inhibition of Methane and Ammonia Production
Next, we aimed to determine whether there was correlation between hop acid content in spent yeast and the rate of inhibition of methanogenesis. Hop acids (Figures 1, 2) are known antimicrobial agents that are likely responsible for the inhibition of methanogenesis; the β-acids (Figure 1B) are particularly potent (Axman et al., 2015; Flythe et al., 2017b). The amount of these small molecules found in spent yeast varies widely between spent yeasts produced during the production of different beer styles with different hopping rates—to say nothing of the variations between breweries and hop sources.
We set up fermentations with the six spent yeast samples shown in Table 1, and again included a comparison to monensin. Baker's yeast was used in control and monensin-containing fermentations as a nutritionally similar (but hop-free) additive. Results of these experiments are shown in Table 2, Figure 6. These results are similar to two other (unpublished) experiments showing a correlation of hop acid concentration and inhibition of methanogenesis. Each experiment utilized rumen fluid from a different animal.
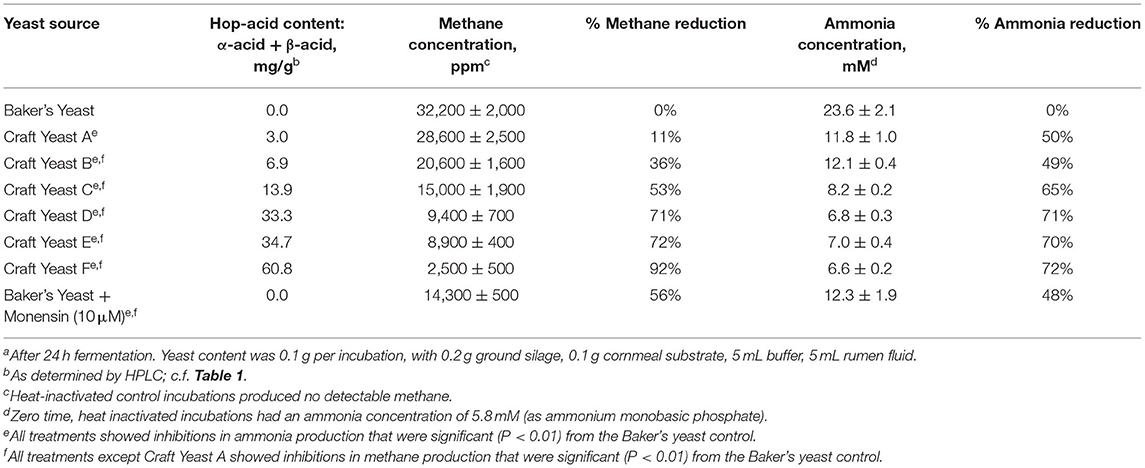
Table 2. Hop acid content in spent craft yeast correlates with inhibition of methane and ammonia by in vitro rumen fluid fermentationsa.
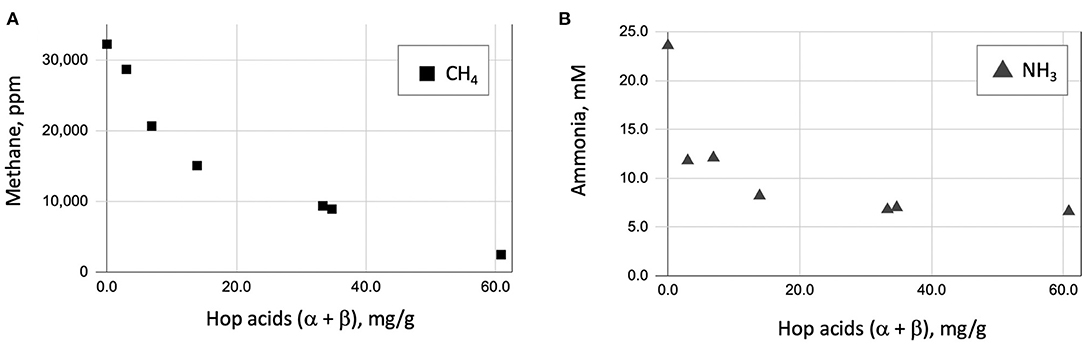
Figure 6. Scatter plot of methane (A) and ammonia (B) produced in rumen fluid fermentation vs. Hop acid content (α-acid + β-acid).
We also looked at the production of ammonia under these conditions. Hop acids are known inhibitors of ammonia-producing bacteria. While not a greenhouse concern, ammonia is an important ruminal byproduct that can adversely affect animal health at high concentrations. Results of ammonia production are also shown in Table 2, Figure 6. There is a strong correlation between the inhibition of methane (Figure 6A) and ammonia (Figure 6B) with the non-isomerized hop acid (α-acid + β-acid) content in the spent craft yeast additive.
Discussion
Our results support the extensive literature regarding the antimicrobial activity of hop acids and show that hop-rich spent yeasts may be a promising target as a future additive to the feed of ruminants. Spent yeast is a protein-rich material produced by breweries worldwide, and it is treated as waste by most small- and mid-sized breweries, meaning this material has potential for upcycling.
Our experimental design is limited by our reliance on rumen fluid from a different animal for each individual experiment. Nevertheless, the rates of methane production in each experiment is reasonable. In Table 2, the Baker's Yeast-treated rumen fluid produced 32,200 ppm of methane, which converts to 5mL of methane from 5 mL of rumen fluid. Thus, our in vitro incubations mimic the normal metabolic range of 250–500 L per day from a 200 L rumen.
Comparison of Moderately-Hopped Spent Yeast to Monensin
Hops and hop acids are known inhibitors of microbial growth (Teuber and Schmalreck, 1973; Axman et al., 2015). Our previous research demonstrated that hoppy yeast inhibited methane production by uncultivated bovine rumen microorganisms in the presence of an easily-metabolized fructose substrate (Pszczolkowski et al., 2016). The first experiment of this research established that the inhibitory effects are present when a more natural substrate is used, namely a 2:1 mixture of ground silage and ground corn. When craft yeast, obtained from a moderately-hopped ale-style beer, was used as an additive, headspace methane production was reduced from an average of 4760 ppm to 3530 ppm after a 24-h incubation (Figure 3A), representing a roughly 25% inhibition. In fact, this inhibition was comparable to the reduction in methane caused by adding 10 μM of the ionophore antibiotic monensin (Figure 3A).
Ammonia (NH3) is another byproduct of ruminal fermentation. Rumen ammonia is the result of amino acid fermentation (Russell and Strobel, 1989). Decreased availability of essential amino acids to the animal can result in decreased growth rate or milk production. Although ammonia represents less of an acute environmental concern than methane, it likewise represents a loss: these nitrogen atoms are unavailable to the animal for milk or meat production. In addition to the headspace methane analysis, we assayed for ammonia after the 24-h fermentation (Figure 3B). The hop-rich craft yeast causes a slight (0.9 mM, or 6%) but significant (P = 0.01) inhibition of ammonia production compared to a baker's yeast control, although craft yeast is not as potent an inhibitor of ammonia as is monensin (Figure 3B).
Hop acids likewise act as ionophores in rumen fluid (Flythe et al., 2017b). However, the precise mechanism by which hop acids inhibit archaeal, protozoal, or bacterial activity is unknown, as with other promising natural products leads in this field such as seaweed-derived additives. In contrast, the molecule 3-nitropropanol, a synthetic feed additive candidate, is known to inactivate the N-methyl reductase enzyme in methanogens (Duin et al., 2016). Future studies would be necessary to understand what enzymatic processes are being affect by hop acids, or whether there might be a synergistic effect with other feed additives.
Hop acids are known inhibitors of bovine hyper ammonia-producing bacteria (Flythe, 2009; Flythe et al., 2017a). Supporting the role of hop acids in suppressing ammonia formation by rumen microbes, craft yeast was previously found to generate less ammonia than baker's yeast even in the presence of exogenous amino acids by inhibition of hyper ammonia-producing bacteria (Harlow et al., 2016). The lowered ammonia production appears to result from an inhibition of the growth of the hyper ammonia-producing bacteria by the molecules extracted from hops into the spent yeast during the beer-brewing process: Yeast alone does not inhibit growth of hyper ammonia-producing bacteria, whereas yeast containing hop acids do (Figure 4).
The results shown in Figures 3, 4 were obtained using spent yeast from a moderately-hopped ale-style beer. This led us to wonder whether the hopping-level of the spent yeast would correlate with the inhibitory effects. We therefore obtained spent yeasts from a variety of ale-style beers representing different hopping rates and protocols, in order to examine spent yeast samples of different hop-acid content.
Correlating Hop-Contents of Spent Yeasts With Inhibition of Methane and Ammonia
We had previously found that spent yeast from craft brewing contain much higher amounts of hops acids, e.g., 12 times more α-acids (Bryant and Cohen, 2015). Furthermore, the concentrations of iso-α-acids, α-acids and β-acids in the yeast phase were >100 times that found in the beer. Yeast that accumulates during beer fermentation is apparently a sink for hop acids. Several of the six spent yeast samples analyzed here were from craft beer styles brewed with large amounts of hops. Consequently, they contained larger amounts of hop acids, particularly α- and β-acids, as shown in Table 1, and provided a set of yeasts with different levels of hop acids. These yeasts were used in growth experiments as before, using rumen fluid from steer harvested immediately after slaughter. There was a strong correlation between the amounts of hop acid present and the inhibition of methane and ammonia production (Table 2; Figure 6). In fact, the three yeasts with the highest concentrations of hop acids were more potent than the antibiotic monensin at inhibiting both methane and ammonia production in this experiment (Table 2). The inhibition of ammonia-producing Acetoanaerobium sticklandii SR by hop-rich craft yeast (Figure 4) further corroborates this result. The inhibitions of methanogenesis were significant (P < 0.01) for all treatments except Craft Yeast A, and inhibition of ammonia production was significant for all treatments (Table 2). However, modulation of other microbial populations by hop acids and other hop metabolites are possible particularly at higher amounts of hop metabolites. Given the poor solubility of α- and β-acids, the delivery by yeast may enable higher concentrations to be achieved.
We suspect that the α- and β-hop acids are primarily responsible for the observed effects, because these have generally shown higher antimicrobial activity than the iso-α-acids (Teuber and Schmalreck, 1973; Axman et al., 2015). Also, they represent on average <20% of the total hop acids present in our spent yeast analysis (Table 1); however, the role of iso-α-acids in these effects cannot be ruled out. Likewise, our spent craft yeast samples contained small amounts (0.2 to 3.0 mg/g) of the hop metabolite xanthohumol (data not shown). Xanthohumol has potent anaerobic antimicrobial activity and could also contribute to the overall metabolic effects of spent yeast on rumen microbes (Cermak et al., 2017; Sleha et al., 2021). Based on our in vitro results, spent yeasts from the most heavily-hopped beer styles have the strongest potential to inhibit methane and ammonia production (Figure 6). Future studies will need to establish how the hopping level of spent yeast affects other metabolic parameters such as overall rate of fermentation, substrate consumption, and acetate/propionate ratios. Overall, these results suggest that spent yeast, a problematic waste product for some brewers, may have future potential as a feed supplement with benefits to farmers and with a positive environmental effect.
Data Availability Statement
The raw data supporting the conclusions of this article will be made available by the authors, without undue reservation.
Author Contributions
RB, EB, CF-C, MF, and LM designed research and wrote the paper. RB, EB, CF-C, DC, and MF conducted research. RB, MF, and LM analyzed data. All authors read and approved the final manuscript.
Funding
RB, DC, and LM were supported by USDA–SBIR grant #2018-33610-28499. EB was supported by a Sutherland Family grant. MF was supported by USDA–ARS. LM was supported by the Warren Wilson College Center for Faculty Innovation and Excellence and the Western Office of the North Carolina Biotechnology Center for equipment.
Conflict of Interest
RB works for Asheville Sustainability Research LLC and consults for a brewing company in Asheville, NC.
The remaining authors declare that the research was conducted in the absence of any commercial or financial relationships that could be construed as a potential conflict of interest.
Publisher's Note
All claims expressed in this article are solely those of the authors and do not necessarily represent those of their affiliated organizations, or those of the publisher, the editors and the reviewers. Any product that may be evaluated in this article, or claim that may be made by its manufacturer, is not guaranteed or endorsed by the publisher.
Acknowledgments
We are grateful for valuable conversations and input from Josie Abramczyk, Broxton Ashburn, Kim Borges, Matthew Burleson, Stephen Cartier, Deidre Harmon, Jeffrey Holmes, Thomas Robacker, Jonathan Snover, and Joseph Young.
References
Axman, J. E., Van Bibber, C. L., Alvarado, C., Thieszen, J., and Drouillard, J. S. (2015). Hops βeta-acid extract yields feedlot performance similar to rumensin. Kans. Agric. Exp. Stn. Res. Rep. 1–4. doi: 10.4148/2378-5977.1026
Bryant, R., and Cohen, S. D. (2015). Characterization of hop acids in spent brewer's yeast from craft and multinational sources. J. Am. Soc. Brew. Chem. 73, 159–164. doi: 10.1094/ASBCJ-2015-0315-01
Cermak, P., Olsovska, J., Mikyska, A., Dusek, M., Kadleckova, Z., Vanicek, J., et al. (2017). Strong antimicrobial activity of xanthohumol and other derivatives from hops (Humulus lupulus L.) on gut anaerobic bacteria. APMIS 125, 1033–1038. doi: 10.1111/apm.12747
Chaney, A. L., and Marbach, E. P. (1962). Modified reagents for determination of urea and ammonia. Clin. Chem. 8, 130–132. doi: 10.1093/clinchem/8.2.130
Chen, G., and Russell, J. B. (1989). More monensin-sensitive, ammonia-producing bacteria from the rumen. Appl. Environ. Microbiol. 55, 1052–1057. doi: 10.1128/aem.55.5.1052-1057.1989
Chen, M., and Wolin, M. J. (1979). Effect of monensin and lasalocid-sodium on the growth of methanogenic and rumen saccharolytic bacteria. Appl. Environ. Microbiol. 38, 72–77. doi: 10.1128/aem.38.1.72-77.1979
Duin, E. C., Wagner, T., Shima, S., Prakash, D., Cronin, B., Yáñez-Ruiz, D. R., et al. (2016). Mode of action uncovered for the specific reduction of methane emissions from ruminants by the small molecule 3-nitrooxypropanol. Proc. Natl. Acad. Sci. U.S.A. 113, 6172–6177. doi: 10.1073/pnas.1600298113
Eun, J. S., Fellner, V., and Gumpertz, M. L. (2004). Methane production by mixed ruminal cultures incubated in dual-flow fermentors. J. Dairy Sci. 87, 12–21. doi: 10.3168/jds.S0022-0302(04)73148-3
Ferreira, I. M. P. L. V. O., Pinho, O., Vieira, E., and Tavarela, J. G. (2010). Brewer's Saccharomyces yeast biomass: characteristics and potential applications. Trends Food Sci. Technol. 21, 77–84. doi: 10.1016/j.tifs.2009.10.008
Flythe, M. D. (2009). The antimicrobial effects of hops (Humulus lupulus L.) on ruminal hyper ammonia-producing bacteria. Lett. Appl. Microbiol. 48, 712–717. doi: 10.1111/j.1472-765X.2009.02600.x
Flythe, M. D., and Aiken, G. E. (2010). Effects of hops (Humulus lupulus L.) extract on volatile fatty acid production by rumen bacteria. J. Appl. Microbiol. 109, 1169–1176. doi: 10.1111/j.1365-2672.2010.04739.x
Flythe, M. D., Harlow, B. E., Aiken, G. E., Gellin, G. L., Kagan, I. A., and Pappas, J. (2017a). Inhibition of growth and ammonia production of ruminal hyper ammonia-producing bacteria by chinook or galena hops after long-term storage. Fermentation 3:68. doi: 10.3390/fermentation3040068
Flythe, M. D., Kagan, I. A., Wang, Y., and Narvaez, N. (2017b). Hops (Humulus lupulus L.) bitter acids: modulation of rumen fermentation and potential as an alternative growth promoter. Front. Vet. Sci. 4:131. doi: 10.3389/fvets.2017.00131
Global Rumen Census Collaborators, Henderson, G., Cox, F., Ganesh, S., Jonker, A., Young, W., et al. (2015). Rumen microbial community composition varies with diet and host, but a core microbiome is found across a wide geographical range. Sci. Rep. 5:14567. doi: 10.1038/srep14567
Grieve, D. G. (1979). Feed intake and growth of cattle fed liquid brewer's yeast. Can. J. Anim. Sci. 59, 89–94. doi: 10.4141/cjas79-011
Harlow, B. E., Bryant, R. W., Cohen, S. D., O'Connell, S. P., and Flythe, M. D. (2016). Degradation of spent craft brewer's yeast by caprine rumen hyper ammonia-producing bacteria. Lett. Appl. Microbiol. 63, 307–312. doi: 10.1111/lam.12623
Intelmann, D., Haseleu, G., Dunkel, A., Lagemann, A., Stephan, A., and Hofmann, T. (2011). Comprehensive sensomics analysis of hop-derived bitter compounds during storage of beer. J. Agric. Food Chem. 59, 1939–1953. doi: 10.1021/jf104392y
Janssen, P. H., and Kirs, M. (2008). Structure of the archaeal community of the rumen. Appl. Environ. Microbiol. 74, 3619–3625. doi: 10.1128/AEM.02812-07
Johnson, K. A., and Johnson, D. E. (1995). Methane emissions from cattle. J. Anim. Sci. 73, 2483–2492. doi: 10.2527/1995.7382483x
Kagan, I. A., and Flythe, M. D. (2014). Thin-layer chromatographic (TLC) separations and bioassays of plant extracts to identify antimicrobial compounds. J. Vis. Exp. e51411. doi: 10.3791/51411
Karakurt, I., Aydin, G., and Aydiner, K. (2012). Sources and mitigation of methane emissions by sectors: a critical review. Renew. Energy 39, 40–48. doi: 10.1016/j.renene.2011.09.006
Kim, M., Morrison, M., and Yu, Z. (2011). Status of the phylogenetic diversity census of ruminal microbiomes: status of microbial census in rumen. FEMS Microbiol. Ecol. 76, 49–63. doi: 10.1111/j.1574-6941.2010.01029.x
Knapp, J. R., Laur, G. L., Vadas, P. A., Weiss, W. P., and Tricarico, J. M. (2014). Invited review: enteric methane in dairy cattle production: Quantifying the opportunities and impact of reducing emissions. J. Dairy Sci. 97, 3231–3261. doi: 10.3168/jds.2013-7234
Krause, D. O., and Russell, J. B. (1996). An rRNA approach for assessing the role of obligate amino acid-fermenting bacteria in ruminal amino acid deamination. Appl. Environ. Microbiol. 62, 815–821. doi: 10.1128/aem.62.3.815-821.1996
Matsui, H., Inui, T., Oka, K., and Fukui, N. (2016). The influence of pruning and harvest timing on hop aroma, cone appearance, and yield. Food Chem. 202, 15–22. doi: 10.1016/j.foodchem.2016.01.058
Moss, A. R., Jouany, J.-P., and Newbold, J. (2000). Methane production by ruminants: its contribution to global warming. Ann. Zootech. 49, 231–253. doi: 10.1051/animres:2000119
Mussatto, S. I., Dragone, G., and Roberto, I. C. (2006). Brewers' spent grain: generation, characteristics, and potential applications. J. Cereal Sci. 43, 1–14. doi: 10.1016/j.jcs.2005.06.001
Narvaez, N., Wang, Y., Xu, Z., Alexander, T., Garden, S., and McAllister, T. (2013). Effects of hop varieties on ruminal fermentation and bacterial community in an artificial rumen (rusitec). J. Sci. Food Agric. 93, 45–52. doi: 10.1002/jsfa.5725
Newbold, C. J., and Rode, L. M. (2006). Dietary additives to control methanogenesis in the rumen. Int. Congr. Ser. 1293, 138–147. doi: 10.1016/j.ics.2006.03.047
Ogunade, I., Schweickart, H., Andries, K., Lay, J., and Adeyemi, J. (2018). Monensin alters the functional and metabolomic profile of rumen microbiota in beef cattle. Animals 8:211. doi: 10.3390/ani8110211
Pszczolkowski, V. L., Bryant, R. W., Harlow, B. E., Aiken, G. E., Martin, L. J., and Flythe, M. D. (2016). Effects of spent craft brewers' yeast on fermentation and methane production by rumen microorganisms. Adv. Microbiol. 06, 716–723. doi: 10.4236/aim.2016.69070
Russell, J. B., and Strobel, H. J. (1989). Effect of ionophores on ruminal fermentation. Appl. Environ. Microbiol. 55, 1–6. doi: 10.1128/aem.55.1.1-6.1989
Shindell, D., Ravishankara, R. V., Kuylenstierna, J. C. I., Michalopoulou, E., HöglundIsaksson, L., Zhang, Y., et al. (2021). United Nations Environment Programme and Climate and Clean Air Coalition (2021). Global Methane Assessment: Benefits and Costs of Mitigating Methane Emissions. Nairobi: United Nations Environment Programme.
Sleha, R., Radochova, V., Mikyska, A., Houska, M., Bolehovska, R., Janovska, S., et al. (2021). Strong antimicrobial effects of xanthohumol and beta-acids from hops against clostridioides difficile infection in vivo. Antibiotics 10:392. doi: 10.3390/antibiotics10040392
Teuber, M., and Schmalreck, A. F. (1973). Membrane leakage in Bacillus subtilis 168 induced by the hop constituents lupulone, humulone, isohumulone and humulinic acid. Arch. Für Mikrobiol. 94, 159–171. doi: 10.1007/BF00416690
Urban, J., Dahlberg, C. J., Carroll, B. J., and Kaminsky, W. (2013). Absolute configuration of beer′s bitter compounds. Angew. Chem. Int. Ed. 52, 1553–1555. doi: 10.1002/anie.201208450
US EPA (2016). Understanding Global Warming Potentials. Washington, DC: US EPA. Available online at: https://www.epa.gov/ghgemissions/understanding-global-warming-potentials (accessed June 3, 2021).
Keywords: methane, ammonia, brewer's yeast, rumen biology, hops, feed supplements
Citation: Bryant RW, Burns EER, Feidler-Cree C, Carlton D, Flythe MD and Martin LJ (2021) Spent Craft Brewer's Yeast Reduces Production of Methane and Ammonia by Bovine Rumen Microbes. Front. Anim. Sci. 2:720646. doi: 10.3389/fanim.2021.720646
Received: 04 June 2021; Accepted: 10 September 2021;
Published: 01 October 2021.
Edited by:
Sarita Bonagurio Gallo, University of São Paulo, BrazilReviewed by:
Suban Foiklang, Maejo University, ThailandAhmed Elolimy, University of Arkansas for Medical Sciences, United States
Copyright © 2021 Bryant, Burns, Feidler-Cree, Carlton, Flythe and Martin. This is an open-access article distributed under the terms of the Creative Commons Attribution License (CC BY). The use, distribution or reproduction in other forums is permitted, provided the original author(s) and the copyright owner(s) are credited and that the original publication in this journal is cited, in accordance with accepted academic practice. No use, distribution or reproduction is permitted which does not comply with these terms.
*Correspondence: Langdon J. Martin, bG1hcnRpbkB3YXJyZW4td2lsc29uLmVkdQ==