- 1Department of Animal and Rangeland Sciences, Oregon State University, Corvallis, OR, United States
- 2Department of Animal Sciences, Food and Nutrition, Università Cattolica del Sacro Cuore, Piacenza, Italy
- 3Department of Pharmaceutical Sciences, College of Pharmacy, Linus Pauling Institute, Oregon State University, Corvallis, OR, United States
Some pasture species are rich in phytochemicals, able to improve milk yield and quality and to reduce the environmental impacts of livestock farming. The phytochemicals interact with the different gene networks within the animal, such as nuclear factor erythroid 2-related factor 2 (NRF2), but their overall impact on animal health remains to be fully understood. The objective of this study was to identify the effects of pasture Legumes and non-leguminous Forbs containing high bioactive compounds on metabolism and activity of the liver, antioxidant response, kidney function, and inflammation of dairy cows using a large array of blood parameters associated with metabolism and the innate immune system. For this purpose, 26 parameters and the concentration of certain bioactive compounds were assessed in blood plasma, collected from the Jersey cows grazing either Grass, Legume, or Forb-based pastures. In addition, serum collected from all the cows was utilized to detect the changes in NRF2 activation in bovine mammary alveolar cells (MACT) and hepatocytes. Compared with Grass, the cows that grazed both Forb and Legume pastures had lower β-hydroxybutyric acid (BHB) and creatinine and larger vitamin E and the ferric reducing ability of the plasma, supporting an improved antioxidative status for these animals. Compared with both Grass and Legume, the cows that graze Forb pasture had lower urea and urea to creatinine ratio, and lower creatinine, indicating a better kidney function. The cows grazing Legume pasture had greater hematocrit, bilirubin, cholesterol, albumin, β-carotene, retinol, and thiol groups but lower ceruloplasmin, paraoxonase, and myeloperoxidase (MPO) than those grazed Grass and Forb pastures, indicating a positive effect of Legume pasture on the liver, oxidative stress, and red blood cells. The plasma of cows in the various pastures was enriched with various isoflavonoids, especially the cows grazed on Forb and Legume pastures, which likely contributed to improving the antioxidative status of those cows. However, this effect was likely not due to the higher activation of NRF2. Overall, these results indicate that Forb and Legume pastures rich in secondary metabolites do not strongly affect the metabolism but can improve the status of the liver and the kidney and improve the efficiency of N utilization and antioxidant response, compared with the Grass pasture.
Introduction
Most pastures utilized for dairy production in temperate environments are primarily composed of perennial ryegrass and white clover (Lee et al., 2018). These pastures provide high-quality forage predominantly during the cool season but are characterized by low taxonomic and phytochemical diversity, presenting limited benefits to the cows and the environment (Distel et al., 2020). Diversification of pastures using forage species with various functional and structural attributes is often sought as a means of extending the grazing period, better balancing the supply of nutrients, improving animal health, and improving the function of soils (Distel et al., 2020). Designing diverse pastures that can adequately meet the needs of the animal, while limiting the negative environmental impact, often requires the use of Forb species containing high amounts of bioactive compounds (Distel et al., 2020). Typically, Forbs and Legumes with large phytochemical diversity are integrated into pasture systems either through diversification of the pasture mixtures or planting short-rotation herbal leys before the pasture renovations in dairy pasture systems. The Forb pastures can be managed as a supplementary forage source to Grass-clover pastures in summer or included in the seasonal sequence-grazing programs where cows switch from the Grass-based pastures in spring to the Legume or Forb pastures in summer to maintain high milk yields and improve the seasonal productivity (Moore et al., 2004).
Many alternative forages have garnered recent interest for their potential uses and benefits in the dairy production systems, such as Forb varieties, such as chicory (Cichorium intybus L.) and plantain (Plantago lanceolata L.) and Legume species, such as birds foot trefoil (Lotus corniculatus L.) and red clover (Trifolium pratense L.), (Distel et al., 2020). Both chicory and plantain can improve N utilization by reducing the rumen ammonia and urinary N levels while maintaining the production levels similar to or above those of cows grazing traditional perennial ryegrass-based pastures (Minneé et al., 2017; Wilson et al., 2020). At appropriate concentrations, the tannins and saponins promote animal health and affect the ruminal bacteria and digestion processes to reduce greenhouse gas emissions, improve nutrient utilization (Mueller-Harvey et al., 2019), and increase the milk quality in dairy herds (Campione et al., 2020). The inclusion of plantain in the dairy cow pastures can lower blood β-hydroxybutyric acid (BHB) and blood urea nitrogen, indicating a positive effect on energy metabolism and nitrogen efficiency (Pembleton et al., 2016). The use of birds foot trefoil can provide similar benefits in terms of reducing ammonia concentrations (Hymes-Fecht et al., 2013) and post-ruminal protein availability (Christensen et al., 2015). Red clover is also known to be a source of many bioactive compounds that may affect the ruminant performance, particularly isoflavones, such as formononetin and biochanin A (Sivesind and Seguin, 2005). Formononetin is broken down into equol once ingested by the animal and can be detected in both the milk (Mustonen et al., 2009) and meat (Moorby et al., 2004). In ruminants, many of the isoflavones and their derivatives have been investigated for their potential role as the phytoestrogens that can interact with estrogen receptors to modulate cell growth and proliferation (Boué et al., 2003). While this effect may be beneficial in young, growing animals as has been observed in finishing lambs (Moorby et al., 2004), it can have negative consequences for the reproductively active animals as was the case for dairy heifers that received berseem clover, a variety high in formononetin and other phytoestrogens (Hashem et al., 2016). In addition, isoflavones, such as those found in the red clover can modulate rumen N efficiency via antimicrobial interactions (Ramos-Morales et al., 2018). Polyphenols including condensed tannins and other flavonoids have also been investigated for their observed antioxidant effects, such as the ability to limit lipid peroxidation and inflammation (Theodorou et al., 2006). These antioxidant effects are likely driven by the upregulation of the nuclear factor erythroid 2-related factor 2 (NRF2), (Zhai et al., 2013; Wang et al., 2016), a transcription factor that plays a key role in activating the endogenous antioxidant response (Tonelli et al., 2018). Of interest, the NRF2 activity can be modulated in bovine mammary cells by both natural and synthetic agonists (Ford et al., 2021).
Support for a beneficial effect of Forbs in pastures was provided by a recent study in our laboratory where lactating Jersey cows grazed on Forb pastures mainly containing chicory and plantain. The cows grazing this Forb pasture achieved at par or better milk yield compared with the cows grazing Legume-based or Grass-clover pastures (Wilson et al., 2020). Interestingly, the cows grazing the Forb pastures also had lower somatic cell counts (SCC) than the cows grazing the other pastures, indicating an additional positive effect of Forb on the immune system of the mammary gland. Data obtained from that study suggested Forb pastures improved the health of the cows, perhaps through improved antioxidant activity. With the intent to assess if the improved performance in cows grazed on Forb and Legume pasture was due to better immune and metabolic status, we assessed a large panel of blood parameters associated with the metabolism and status of the liver, kidney, and antioxidant response in the samples obtained from that study. In addition, serum collected during that study was utilized in an in vitro assay to assess whether the consumption of Forb or Legume pasture mixes could modulate NRF2. It is hypothesized that the cows grazing the Forb and Legume pastures have improved metabolic and antioxidant status, additionally, the cows grazed on Forb will have improved immune status compared with the cows grazed on ryegrass-white clover pasture.
Materials and Methods
Animals and Experimental Design
All the procedures were approved by the Institutional Animal Care and Use Committee of the Oregon State University (ACUP Number: 5206). The detailed information on methodology and grazing management was presented previously (Wilson et al., 2020). Briefly, the grazing was performed at the Oregon State University Research Dairy in Corvallis, OR (44°34′N, 123°18′ W, 78 m a.s.l.), the United States, in 2019. In this study, 27 Jersey cows in mid-lactation were used in a randomized complete block design with three plots per treatment and three animals per plot (two multiparous cows and one primiparous cow). The cows (BW = 480 ± 46) were randomly assigned to each treatment based on age (3.2 ± 1.5 years), milk production (24.8 ± 6.2 L/cow/day), and days in milk (157 ± 64 days). The cows grazed either a Grass-based pasture (X Festulolium braunii cv. Perun, Festuca arundinacea cv. Rustler, Dactylis glomerata cv. Sundown, Trifolium repens cv. Domino) or a Forb-based pasture (Cichorium intybus, cv Antler, Plantago lanceolata cv. Boston, and T. repens cv. Domino) or a Legume-based pasture Trifolium pratense cv. Raven, Lotus corniculatus cv. Bruce, Trifolium alexandrinum cv. Frosty and Trifolium michelianum cv. Fixation). The grazing experiment was split into two periods (Period 1: 29 April−19 May and Period 2: 19 May−7 June) to take into effect changing the botanical and chemical composition of pastures on the measured parameters. The cows selected from the experiment were kept during the winter and early spring seasons in the free stall barn with no access to pasture and they received total mixed ration (TMR) typical of lactating dairy cows at the Oregon State University Dairy Center. The composition and chemical analysis of the TMR was previously reported (Jaaf et al., 2020). Prior to the commencement of the experiment, all the cows grazed a permanent Grass-clover pasture as one herd for a week (i.e., Baseline). The pasture was predominantly composed of tall fescue [Lolium arundinaceum (Schreb.) Darbyshand] and white clover (T. repens L.). Prior to the sampling period, the cows were allowed to adjust to their respective pastures for 2 weeks. The cows were milked two times daily (0.500 and 1,800 h) and provided a new pasture strip after each afternoon milking. After each milking, the cows received 1 kg of rolled grain mix (corn and barley mix 50:50) plus a mineral mix.
Blood Samples and Analyses
The blood samples were collected from the jugular vein into the evacuated tubes with heparin or empty (Becton Dickinson Vacutainer Systems; Becton Dickinson and Co., Franklin Lakes, NJ, USA) just after milking morning at days 0 (baseline), 15, 18, and 21 in the Period 1 and days 12, 15, and 18 in the Period 2. The plasma and serum were obtained via centrifugation at 1,900 × g for 15 min and frozen (−20°C) until analysis. The aliquots of plasma were sent to the Department of Animal Sciences, Food and Nutrition (DIANA), Università Cattolica del Sacro Cuore, Piacenza, Italy for metabolic, oxidative, and inflammatory profiling. The blood samples were processed and analyzed following the methods previously described (Mezzetti et al., 2020) if not differently specified. Energy, protein, and mineral metabolism were profiled measuring the concentration of glucose, cholesterol, non-esterified fatty acid (NEFA), BHB, creatinine, and total protein, Ca, P, Mg, and Zn. Free Ca2+ was calculated as previously described (Mir et al., 2016) using the Orrell adjusted Ca as follow: free Ca2+ = Ca mmol/L + 0.0176 × (34-albumin g/L)/2. Globulin was obtained by subtracting albumin from total protein. The positive (haptoglobin and ceruloplasmin) and negative (albumin and paraoxonase) acute-phase proteins were used to evaluate the inflammatory response. Myeloperoxidase (MPO) was determined to assess the activity of neutrophils. Liver activity and health were assessed by measuring the total bilirubin, aspartate aminotransferase (AST), and γ-glutamyl transferase (GGT). Oxidative stress was monitored by measuring the total reactive oxygen metabolites (ROM), thiol groups, ferric reducing antioxidant power (FRAP), advanced oxidation protein products (AOPP), retinol, α-tocopherol, and β-carotene. Urea data were presented previously (Wilson et al., 2020). Several ratios between the parameters were computed. The NEFA to albumin ratio (mM/mM) was used to assess the potential toxicity of free fatty acids (normal range is 0.5–1), (Høstmark, 1995). The albumin to globulin ratio was used as an index of nutrition and systemic inflammatory status (larger the number better the nutritional status and lower inflammatory status), (Deng et al., 2016). The urea to creatinine ratio was used as an indirect index of the renal glomerular filtration rate with a normal range of 40–100 (mM/mM), with ranges >100 indicating extra-renal problems, such as dehydration, and values <40 indicating kidney issues in humans (Stark, 1998; Lin and Denker, 2015).
Mass Spectrometric Analysis of Secondary Bioactive Metabolites in Plasma
Genistein, daidzein, biochanin A, formononetin, resveratrol, catechin, epicatechin, taxifolin, quercetin, kaempferol, isorhamnetin, luteolin, delphinidin, petunidin, cyanidin, equol, and peonidin (purity ≥ 98.0%), and internal standards [d4]-genistein and [d4]-daidzein (≥99.0% purity) were purchased from Cayman Chemicals (Ann Arbor, MI, USA). Prunetin (≥98.0%) and β-glucuronidase/arylsulfatase (7.1 Units/ml β-glucuronidase and 24 Units/ml arylsulfatase) were purchased from Sigma-Aldrich (St. Louis, MO, USA). Irilone (≥99.0%) was provided by the UIC Botanical Center (Chicago, IL, USA). High-performance liquid chromatography-mass spectrometry (HPLC-MS) grade acetonitrile, methanol, and formic acid were purchased from Thermo Fisher Scientific (Waltham, MA, USA). Ultrapure water was prepared using a Milli-Q water purification system (Millipore, MA, USA). All other reagents and solvents were reagent grade or better and were purchased from VWR (Visalia, CA, USA).
The secondary bioactive metabolites were measured in plasma as reported previously (Muchiri and Van Breemen, 2020). Briefly, 0.2 ml plasma, adjusted to pH 5.0 using 25 mM citrate buffer (pH 5.0), was mixed with β-glucuronidase/arylsulfatase (7.1 U/ml β-glucuronidase and 24 U/ml arylsulfatase) and incubated at 37°C for 2 h. Internal standards ([d4]-daidzein and [d4]-genistein) were added (final concentration of 250 ng/ml), and four volumes of ethanol/methanol (1:1, v/v) were added to precipitate the proteins. The mixture was vortexed for 2 min and allowed to stand at −20°C for 30 min to precipitate the proteins. After centrifugation at 18,000 × g and 4°C for 30 min, the supernatant was removed, dried under vacuum at 24°C, and reconstituted in 200 μl of the initial ultra-high performance liquid chromatography (UHPLC) mobile phase (water/acetonitrile containing 0.01% formic acid; 1:1, v/v). The aliquots of 5 μl were analyzed using ultra-high-performance liquid chromatography-tandem mass spectrometry (UHPLC-MS/MS).
Chromatographic separation of isoflavones was carried out using a Shimadzu (Kyoto, Japan) Nexera UHPLC system fitted with a Shimadzu Shim-pack XR-ODS C18 UHPLC column (1.6 μm, 2.1 mm × 75 mm). The temperature of the auto sampler was 4°C, and the column oven was 35°C. The mobile phase consisted of water (mobile phase A) and acetonitrile (mobile phase B), both containing 0.01% formic acid. The isoflavones were separated using a 6.5 min gradient method that began with 20–55% B over 2 min, followed by a 2 min hold at 55% B, and then a 0.5 min gradient to 90% B. After 1 min, the column was re-equilibrated at 20% B for 1 min before the next injection. The UHPLC system was interfaced with a Shimadzu LCMS-8,060 triple quadrupole mass spectrometer equipped with electrospray and polarity switching. Nitrogen was used as a drying gas at a flow rate of 5 L/min and for nebulization at 3 L/min. The interface and desolvation line temperatures were 400 and 300°C, respectively. The isoflavones were measured using collision-induced dissociation with selected reaction monitoring (SRM). The collision gas pressure was 230 kPa, and the SRM dwell time for each transition was 15 ms. Data acquisition and integration were carried out using Shimadzu Lab Solutions software version 5.7. The analysis of equol was carried out according to a prior published method (Saha and Kroon, 2020), except that the HPLC separation was shortened from 15 to 6 min.
The additional bioactive compounds and their bovine metabolites in plasma were analyzed using LC-MS with positive ion or negative ion electrospray on a Shimadzu LCMS-9,030 Q-ToF hybrid high-resolution mass spectrometer at a resolving power of 30,000 full widths at half maximum (FWHM). The electrospray ionization interface temperature was 300°C, and the voltages were 4.5 and −3.5 kV for positive and negative ion modes, respectively. The heat block and desolvation line temperatures were 400 and 250°C, respectively. Nitrogen was used as a drying gas at a flow rate of 10 L/min, for nebulization at 3 L/min, and as a heating gas at 10 L/min. Mass spectra and data-dependent product ion tandem mass spectra were acquired every 100 ms over the scan ranges of m/z 100–1,200 and m/z 70–1,200, respectively. During data-dependent acquisition, six dependent events were set, and the intensity threshold of 3,000 was defined. Each production tandem mass spectrum was obtained using a collision energy of 35 V with an energy spread of 17 V. The reversed-phase UHPLC separations were carried out using a Waters (Milford, MA, USA) C18 Cortecs HPLC column (2.1 mm × 50 mm, 2.7 μm) with an 18 min linear gradient from 5 to 95% acetonitrile in water containing 0.1% formic acid at a flow rate of 0.3 ml/min. The column was re-equilibrated at 5% acetonitrile for 2 min between the injections. The auto-sampler temperature was 4°C, the injection volume was 5 μl, and the column oven was 30°C. Data acquisition and integration were carried out using Shimadzu Lab Solutions software version 5.7. The peak area corresponding to the concentration of the deuterium-labeled internal standard was used as a reference for determining the concentrations of the bioactive compounds.
In vitro NRF2 Modulation Assay
The bovine mammary alveolar cells (MACT) were cultured in high-glucose Dulbecco's modified Eagle's medium (DMEM) with sodium pyruvate (Cat #25-500, Genesee Scientific, San Diego, CA, USA) and 10% fetal bovine serum (Cat #F4135, Genesee Scientific) and grown and sub-cultured as previously described (Osorio and Bionaz, 2017). The cells were passed into separate white 96-well-plates (Cat#655098, Corning, Germany) and cotransfected with the plasmids pgl4.37 (luc2P/ARE/Hygro) (Cat #E3641, Promega Corporation, Madison, WI, USA) and pRL-TK (Cat #E2241, Promega Corporation) plasmids at a 40:1 ratio in OptiMEM (Cat #11058021, Genesee Scientific) using Lipofectamine 3,000 (Cat #L3000001, Thermo Fisher Scientific, Carlsbad, CA, USA) in accordance with a previously validated method (Ford et al., 2021). Then, 24 h after transfection, the growth medium was removed and replaced with serum or DMEM for another 24 h before reading the plate. To match the experimental design, the serum of the three cows in each plot was pooled both during confinement where the cows were fed the TMR, during the Baseline period, and on day 18 of Period 1, and on day 12 of Period 2 with a n = 3/treatment. In addition, a triplicate was treated with 10 μM of the known activator of NRF2, sulforaphane (SFN), (Cat #S4441, Sigma-Aldrich) as a positive control. The dual luciferase reporter assay was performed using the Dual-Glo Luciferase Assay System kit (Cat#E2920, Promega Corporation) following the instructions of manufacturer using a Synergy HTX Multi-Mode Reader (BioTek Inc., Winooski, VT, USA) to read luminescence, at 1 mm height, 5 s integration time, and 180 gain.
Statistical Analysis
For all the parameters, outlier data were checked using PROC REG of SAS (v9.4, SAS Institute, Inc., Cary, NC, USA) before the statistical analysis. Data with a studentized t > 3.0 were removed. To account for and correct for the differences between the groups at baseline in the final dataset, all the blood parameters were corrected by the baseline (i.e., 0 days) as previously described (Rosa et al., 2017). Briefly, for each parameter data, each sample was corrected arithmetically to obtain the same average between the groups at baseline (i.e., 0 days) by subtracting from each sample the difference between the average at baseline between the Grass group and the sample group (i.e., all data were corrected by the Grass group at baseline). The baseline data were removed before the statistical analysis. To minimize the effect of cows in each plot, a geometrical mean was performed for each plot and period using the baseline-corrected data. Data were analyzed using GLIMMIX of SAS with the fixed effect of pasture treatments (Grass, Forb, and Legume), periods (Period 1 and Period 2), and their interaction with the plot (n = 3) as a random effect using the default covariate model (i.e., variance components). For the NRF2 activity, data were analyzed in three different ways: (1) as Luciferase/Renilla ratio corrected by the negative control (i.e., DMEM only), (2) as fold relative to the baseline in each group and plot separately (e.g., data from the Grass group in Plot 1 in the experimental period were divided by the Baseline value of the Plot 1 of the same group), and (3) as fold relative to TMR in each group and plot separately. The data were analyzed using GLM of SAS with pasture (Grass, Forb, and Legume), experimental period (TMR, Baseline, and Experiment), and their interaction as the main effect. For the analysis of the dataset as fold relative to Baseline and TMR, the latter was removed from the model prior to running the statistical analysis. For the analysis of the secondary compounds in plasma, two statistical analyses were performed: a GLM model was used to compare the effect of the treatments (TMR, Forb, Grass, and Legume) using block (n = 3 as geometrical of the three animals per block) as a random effect and a GLIMMIX model with pasture (Grass, Forb, and Legume), period (1 and 2), and their interaction as main effect and block as a random effect. The P-value of ≤ 0.05 was declared as significant and P-value between >0.05 and 0.10 as having a statistical tendency.
Results
Blood Parameters
Metabolism and Minerals
Pasture type had a minimal effect on the blood metabolic profile of dairy cows with few parameters affected by the period (Table 1). The cows grazing the Legumes pasture had higher hematocrit and cholesterol levels compared with those grazing Grass and Forb pastures. The plasma BHB levels of the cows grazing Grass were substantially higher than those grazing Legume and Forb pastures (P < 0.01). The BHB level of cows in the Forb group was lower than the cows in the Legume group. The cows in the Forb group had lower (P < 0.05) total blood protein content compared with the cows in the Legume and Grass groups which were similar to each other. The level of NEFA (P < 0.01) and total protein (P < 0.05) decreased from Period 1 to Period 2. Neither the pasture type nor the grazing period had an effect on the blood mineral profile (all P > 0.05). However, the blood Mg concentration tended to be greater in cows in the Legume and Forb groups than the cows in the Grass group (P = 0.10).
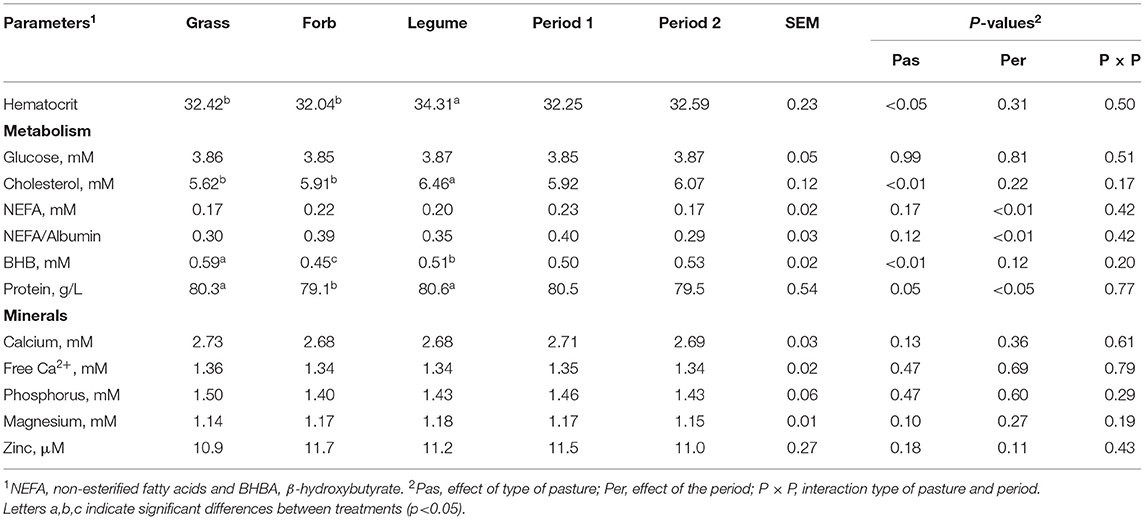
Table 1. The blood parameters associated with metabolism and mineral concentration in the dairy cows grazing Grass-, Forb-, or Legume-based pastures.
Inflammation, Immune Status, and Liver Function
The type of pasture affected several parameters related to inflammation, immune status, and liver function with few parameters affected by the grazing period (Table 2). The levels of the positive acute phase protein ceruloplasmin, the liver-derived paraoxonase, and MPO were lower while the concentration of bilirubin was higher in the Legume compared with the Grass and Forb groups (P ≤ 0.02). Compared with the other groups, the Forb had a lower level of albumin (P <0.01). The level of AST and albumin/globulin ratio of Forb and Legume was lower than the Grass (P < 0.01). No pasture type*grazing period interaction was detected for the parameters related to inflammation, immune status, and liver function (all P > 0.05).
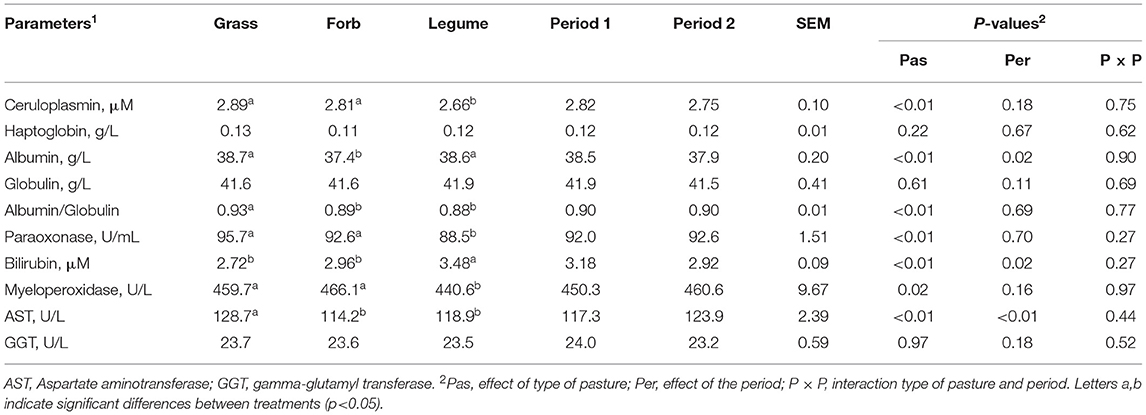
Table 2. The blood parameters associated with inflammation, immune response, and liver health in the dairy cows grazing Grass-, Forb-or Legume-based pastures.
Kidney Function
Kidney function in the cows was highly affected by the type of pasture (Table 3). The creatinine level was lower in Forb and Legume as compared with Grass (P < 0.01). The urea to creatinine ratio was different between the cows grazed on the various pastures, with the lowest level detected in Forb and the largest detected in Legume (P < 0.01).
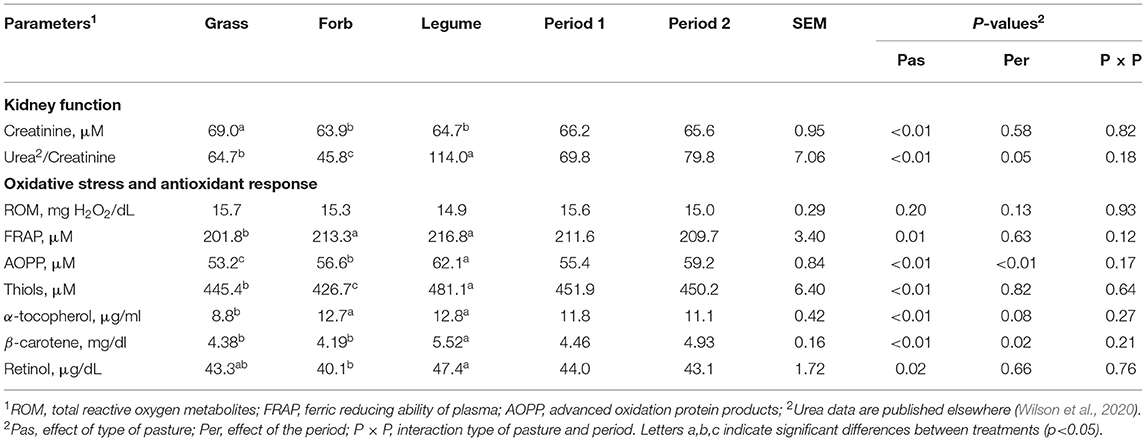
Table 3. The blood parameters associated with kidney function and oxidative status in the dairy cows grazing Grass-, Forb-or Legume-based pastures.
Oxidative Stress
All the parameters related to oxidative stress and antioxidant response, except for total ROM (P = 0.20), were affected by the type of pasture with a larger effect in the cows grazed on Legume pastures. The cows grazing Legume pastures had higher (P < 0.01) blood levels of AOPP, thiols, and β-carotene compared with the other groups and higher (P = 0.02) levels of retinol compared with those grazing Forb. The Forb and Legume groups had higher (P < 0.01) FRAP and α-tocopherol levels compared with the Grass. The Forb group had a greater (P < 0.01) AOPP concentration compared with the Grass group. Among those parameters, AOPP (P < 0.01) and β-carotene (P = 0.02) increased from Period 1 to Period 2 while α-tocopherol tended to decrease (P = 0.08) as the grazing season progressed.
NRF2 Modulation
No effects were observed in the activation of NRF2 in the MACT cells by the serum from the cows that grazed pastures or compared with the serum from cows while fed TMR (Figure 1). We detected a lower activation of NRF2 by the serum of the cows when grazing the Baseline pasture compared with the serum obtained from the cows while fed on TMR or grazing the experimental pastures.
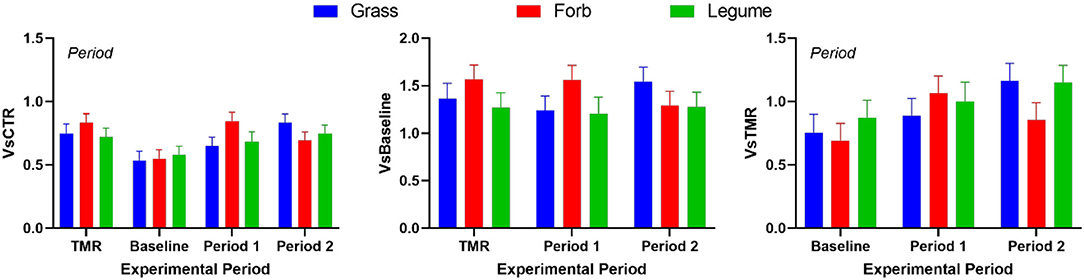
Figure 1. Nuclear factor erythroid 2-related factor 2 (NRF2) modulation measured by luciferase luminescence in mammary alveolar cells (MACT) by serum collected in the cows while fed total mixed ration (TMR), permanent pasture during the baseline, and when grazing ryegrass and white clover pasture (Grass), pastures rich in chicory and plantain (Forb), or Legume-rich pastures (Legume) (i.e., Experimental) during April 29 to May 19 (Period 1) and May 19 to June 6 (Period 2) of 2019. The cells were also treated with 10 μM sulforaphane (SFN) in Dulbecco's modified Eagle's medium (DMEM) as positive control and only DMEM as a negative control. The negative control was used to correct for variation between the plates. The data are a ratio of ARE-luciferase/TK-Renilla luciferase in each sample relative to the negative control (CTR). Except for SFN and DMEM where a minimum of three technical replicates was used, there were n = 3 biological replicates per treatment as a pool of serum from the three cows in the same grazing plot (n = 3 plots per group). Data are shown and were statistically analyzed as a ratio of luciferase/renilla relative to CTR (VsCTR) for all the groups while receiving TMR in Baseline pasture or during the two periods of the Experimental pastures; as fold relative to Baseline (VsBaseline); or as fold relative to TMR (VsTMR). The statistical effect of Period is indicated in the graph when present. The treatment of MACT cells with SFN in DMEM activated NRF2 10.93 ± 1.25 fold relative to DMEM alone.
Secondary Bioactive Metabolites in Plasma
All measured secondary bioactive compounds except for kaempferol and quercetin were detected in all the bovine samples collected (Figure 2). The plasma levels of genistein, biochanin A + prunetin, and kaempferol were highest in the Legume group (P < 0.01). The latter was mainly present in the plasma of cows in the Legume group. A metabolite, p-ethylphenol, derived from the demethylation of biochanin A to genistein followed by ring cleavage, was observed in the low ng/mL range and was highest (P < 0.05) in the Legume group but only in Period 1. Irilone and daidzein had a similar pattern, with higher plasma levels in the cows grazing compared with the cows fed with TMR and highest values in the Legume group in period 1 (P < 0.05), with irilone being more abundant in the Forb vs. Grass (P < 0.05) group in Period 1. Formononetin was almost undetectable in the TMR group and was present at a high amount in plasma of grazing cows, with the highest amount in the Forb group followed by Legume and Grass, with all the differences between the groups statistically significant (P < 0.05). Plasma concentrations of ixerisoside D were higher (P < 0.05) in Period 2 vs. Period 1 and was higher (P < 0.05) in Forb in Period 2 vs. the other groups. The concentration in plasma of delphinidin was higher during Period 1 vs. Period 2 and was lower (P < 0.05) in the cows grazing pastures compared with the cows consuming TMR. Furthermore, the concentration of the same compound was lower (P < 0.05) in the plasma of Legume vs. the other grazing groups. In contrast to this trend, the plasma concentrations of quercetin were higher in Period 2 vs. Period 1 but, as for the delphinidin, they were lower in grazing vs. TMR groups (P < 0.05). An unidentified compound with an exact mass of 267.1239 (negative ion electrospray) was observed in plasma for all the samples. The unknown compound was found at the highest concentration in the plasma of the cows in the Legume group, followed by Forb, and Grass (all differences with P < 0.05). The cows fed with TMR had a lower (P < 0.01) amount of this compound compared with the cows grazing pastures. The isoflavone aglycones were not detected in the non-hydrolyzed plasma of cows in all the groups (data not shown). Equol, the primary product of formononetin degradation in the rumen, was highest in the blood of the animals grazing Legume compared with the other groups (P < 0.05). Total equol was also higher in Period 2 when compared with Period 1, likely driven by the higher blood levels of equol-diglucuronide for all the cows regardless of the pasture type.
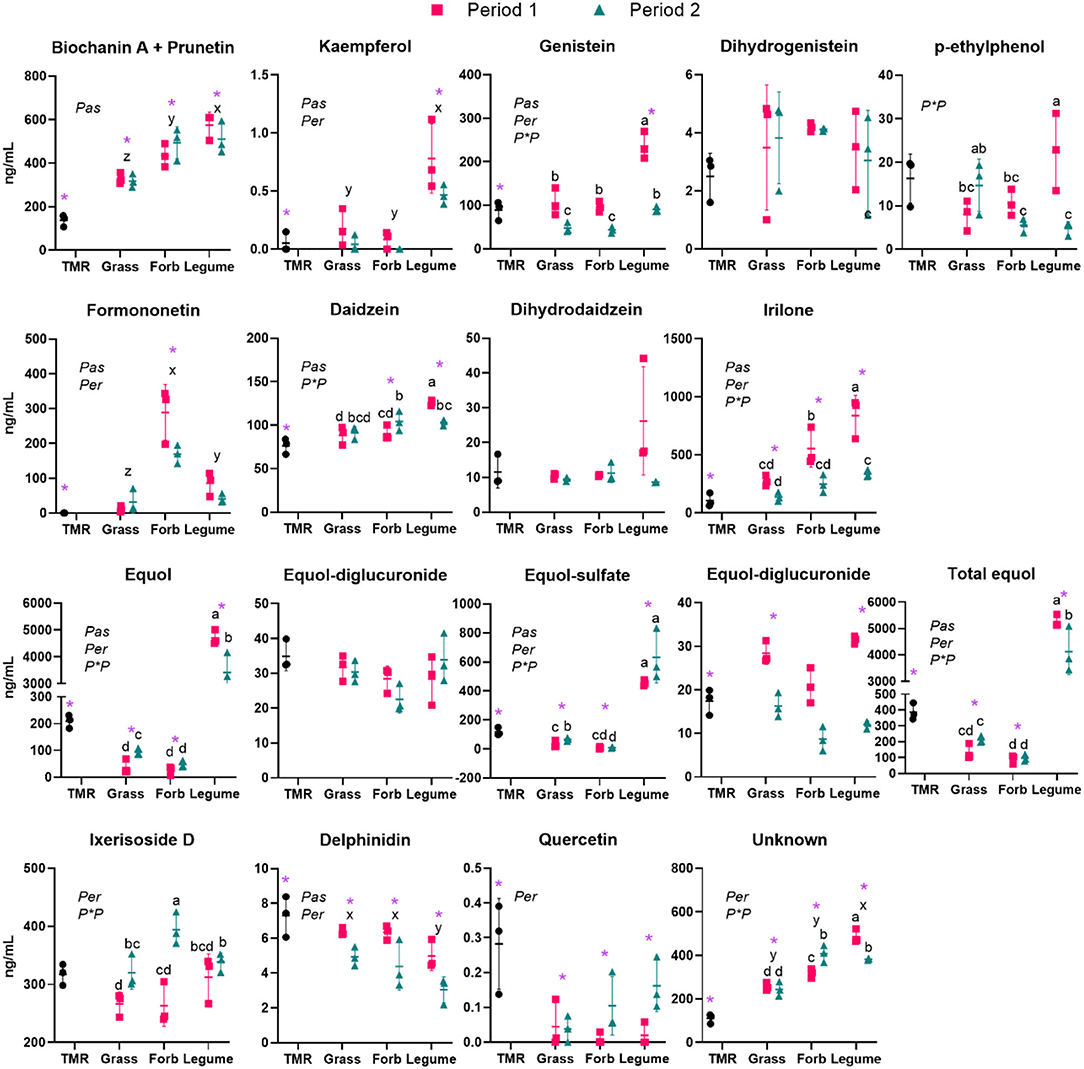
Figure 2. The plant secondary compounds present in the plasma of the cows fed TMR (this was offered to all cows before starting the pasture trial), ryegrass-white clover mix (Grass), chicory-plantain mix (Forb), and Legume mix (Legume) for two periods during 2019 spring (Period 1 = April 29 to May 19 and Period 2 = May 19 to June 6). Shown are the data point for each plot (n = 3 plots per group) in each treatment and period with mean and SD. Letters a, b, c, and d denote the statistical differences (P < 0.05) between the various Pastures at each Period when Pasture × Period was significant (P*P), letters x, y, and z denote the statistical differences (P < 0.05) between the various pastures (Pas). Purple asterisks denote the difference of various pastures with TMR.
Discussion
Effect of Pasture Composition on Metabolism
The findings regarding the nutritive quality of the pastures and the effect of pasture type on the feed intake and milk yield of the cows were reported previously (Wilson et al., 2020). Those data revealed a superior performance, indicated by higher DMI and milk production, in the cows grazing Forb and Legume compared to Grass. Thus, those data would suggest an improved metabolic situation in the cows grazing on Forb and Legume pastures vs. Grass pasture. However, the blood parameters measured only indicated a minor effect on metabolism by the various pastures. The three parameters were affected: cholesterol, BHB, and total protein. The effect on the latter is due to the level of albumin, which is discussed in another section.
Cholesterol
The increased concentration of cholesterol in the plasma of cows, especially in mid-lactation, is often associated with the increased amount of dietary lipids (Bionaz et al., 2020). The total amount of lipids ingested were likely higher in cows consuming the Legume pasture compared with the other pastures, considering that the Legume pasture had a numerically larger ether extract and the cows consuming it had a significantly higher dry matter intake compared with the other pastures (Wilson et al., 2020). Besides dietary factors, the changes in cholesterol level in the blood are associated with liver function as high-density lipoprotein (HDL), which is the most abundant lipoprotein in cattle and is exclusively produced in the liver (Bionaz et al., 2007). The paraoxonase level in blood is highly associated with the level of HDL (Rochu et al., 2010).
The cholesterol levels across all the groups were within the normal range reported in mid-lactation dairy cows (Bionaz et al., 2007; Cozzi et al., 2011) although the cows in the Legume have slightly elevated cholesterol in our study but unlikely to be biologically significant. As previously observed in the post-partum cows (Bionaz et al., 2007), a positive association between paraoxonase and HDL and total cholesterol level exist in the dairy cows (Bionaz et al., 2007). However, our results indicate lower activity of paraoxonase despite a higher level of cholesterol in cows in the Legume group. Those data indicate a likely role of the intestinal-produced cholesterol in changing the level of cholesterol in the blood in this group, rather than tied to increased liver function and hepatic HDL production. A higher level of cholesterol in the blood might be indicative of higher energy intake. This appears to be supported by a prior work where the inclusion of a Legume in pasture significantly increased the cholesterol content in the muscle of beef cattle when compared with Grass only (Jaturasitha et al., 2009). The authors in that study concluded that the higher energy in the Legume pasture was the cause of the increase in cholesterol concentration. This can be also the case in our study, where a higher feed intake and energy content in the diet was observed for the Legume vs. Grass pasture (Wilson et al., 2020).
β-Hydroxybutyric Acid
The decrease in circulating BHB noticed in the Legume and Forb group compared with the Grass group may not be tied to energetic imbalances leading to lipolysis in the adipose tissue, as the NEFA levels are not significantly different between the groups. Consequently, the changes in BHB may be attributed to the changes in ruminal volatile fatty acids (VFA) compositions, particularly butyrate, as it is converted to BHB in the rumen (Miettinen and Huhtanen, 1996; Defrain et al., 2004). Thus, the data would suggest a likely lower production of butyrate in the Forb and Legume vs. Grass group. However, without ruminal VFA data, the precise reason for this observation remains unknown. Non-fiber carbohydrates (NFC), which were higher in the Legume and Forb compared with the Grass pastures (Wilson et al., 2020), are known to increase butyrate production (Defrain et al., 2004; Whelan et al., 2014; Gao and Oba, 2016; Wang et al., 2020) although not always (Wei et al., 2018). A possible explanation is that the higher NFC in Legume-fed animals increased ruminal propionate (Roche et al., 2010; Wei et al., 2018; Min et al., 2019) augmenting hepatic glucose production (Reynolds et al., 2003); congruently, propionate infusion in the post-partum cows decreases the BHB level in the blood likely due to direct stimulation of hepatic oxidation of acetyl CoA by propionate, reducing the pool of acetyl CoA available to form BHB (Stocks and Allen, 2012). Greater hepatic glucose production would also justify the greater milk production observed in both the Forb and Legume vs. Grass cows (Wilson et al., 2020).
Legume and Forb Pasture Has a Positive Effect on Liver AST
Several liver parameters associated with inflammation were affected in the plasma of Legume vs. the other pastures, including a lower paraoxonase and ceruloplasmin. Those effects are likely not due to lower inflammatory status (Bionaz et al., 2007) considering that the haptoglobin, one of the most reactive positive acute phase proteins, was not different between the groups, and that also albumin, a negative acute phase protein, was not larger in Legume vs. Grass. The liver in Forb and Legume appeared to be in better condition compared with Grass as indicated by the lower AST in plasma, a typical parameter related to liver health (Oh and Hustead, 2011). The lower level of ceruloplasmin in cows grazing Legume vs. the other pastures is unclear. Besides inflammatory status (Mayasari et al., 2017), the level of ceruloplasmin in plasma is associated with the availability of copper in the diet, since ceruloplasmin is the major transporter of copper in the blood (Gooneratne et al., 1989). Excess of molybdenum is associated with the lower absorption of Cu in cattle (Thorndyke et al., 2020). Unfortunately, we did not measure micro minerals in the various pastures to determine if the mineral imbalance is the cause of the lower ceruloplasmin. However, the animals received a mineral mix containing a balanced amount of minerals.
Oxidative Status and, Likely, Immune System Are Improved by Legume and Forb Pastures
The present data indicated that the cows that graze Legume pastures had an improved antioxidant capacity in comparison with the cows in Grass and Forb groups as indicated by significantly higher FRAP, α-tocopherol, β-carotene, thiol groups, and retinol. Both FRAP and thiol groups have been used in previous studies as reliable indicators of overall antioxidant status in dairy cows (Bernabucci et al., 2002; Haubold et al., 2020). The elevated levels of α-tocopherol, β-carotene, and retinol can primarily be attributed to the pasture composition, since birds foot trefoil, plantain, and chicory contain similarly high amounts of α-tocopherol and β-carotene in comparison with the traditional Grass-clover pastures (Elgersma, 2015). However, the increased abundance of birds foot trefoil and red clover (at least 72%) in the Legume pastures compared with the abundance of plantain and chicory in the Forb pastures (up to 56%) likely explains why the Legume pastures had higher concentrations of β-carotene than the Forb pastures. Increased α-tocopherol and β-carotene work synergistically to help prevent the lipid peroxidation and the generation of malondialdehyde, contributing to an increase in FRAP (Krinsky, 1992; Verma et al., 2019). The increased thiols in the Legume group is also reflective of the increased antioxidant potential of the serum from the cows that graze Legume pastures, as the scavenging of free radicals in the blood by antioxidant factors, such as β-carotene prevents the formation of disulfide bonds (Banne et al., 2003). The high levels of the flavonol quercetin in the Legume group also contributed to the improved antioxidant capacity due to its activity as a scavenger of free radicals (Booth et al., 2006; Pu et al., 2015).
Despite cows in the Forb group having the lowest levels of retinol and thiols, the FRAP of these animals was significantly higher than the cows in the Grass group and similar to the cows in the Legume group. This may point toward the activity of botanical secondary compounds in the Forb pastures coming from the chicory and/or plantain. Both forage varieties are known to contain bioactive compounds that can modulate the metabolic and immune processes and function as antibiotic and anthelmintic agents (Tamura and Nishibe, 2002; Peña-Espinoza et al., 2018). Of these compounds, chicory is rich in anti-inflammatory and reactive oxygen species (ROS)-scavenging compounds, such as sesquiterpene lactones, hydroxycinnamic acids, flavonoids, and anthocyanins (Peña-Espinoza et al., 2018), which all may contribute to the elevated FRAP despite other antioxidant parameters being lower than Legume. The cows in the Forb group had higher levels of anthocyanins, such as dephinidin compared with the cows in the Legume group, which are potent antioxidants as measured using FRAP (Mueller-Harvey et al., 2019).
Of interest, AOPP, which is typically viewed as an indicator of oxidative stress, was greater in the blood of cows grazing Legume compared with the other groups, despite an overall better antioxidant status. While AOPP in humans is correlated with oxidative stress and inflammatory activity (Witko-Sarsat et al., 1998), the relationship between AOPP and antioxidant status in dairy cows seems to require further investigation. The increased α-tocopherol found in the blood of cows in Legume may have affected AOPP through the enhanced neutrophil activity as seen in the previous studies where vitamin E was supplemented to dairy cattle (Hogan et al., 1990; Politis et al., 2004).
Antioxidant Effects of Legume Pasture Likely Improved Red Blood Cells
In dairy cows, hematocrit remains relatively stable and significant reductions are often associated with chronic inflammation, dehydration, and hypoxic conditions (Roland et al., 2014). While a higher hematocrit was observed in the Legume group, there are no indications that the cows in this group were experiencing any level of significant dehydration or hypoxia. This higher hematocrit may indicate a beneficial effect of the Legume in comparison with the other two pasture types. The oxidative stress can negatively affect the hematopoiesis (Ghaffari, 2008); thus, the improved antioxidant status of the animals in the Legume group may have improved hematopoiesis. This may also be related to the elevated bilirubin levels, as bilirubin is a product of red blood cell degradation and is correlated with the red blood cell number (Chen et al., 2018). However, our data do not exclude the possibility that the cows that graze Legume pasture had a decreased clearance of bilirubin by the liver.
Forb and Legume Pastures Improve Kidney Function
Of particular interest in this investigation was the effect that the Forb pastures had on creatinine levels in the blood and, thereby, the overall urea:creatinine ratio from an N partitioning perspective. A number of studies indicated the beneficial effects of plantain in reducing the nitrous oxide emissions from grazing cows (Bryant et al., 2020). The decreased level of urea in blood observed in the cows that graze Forb pastures has been previously attributed to the increased use of urea in the rumen and an increased urine volume produced in comparison with the Legume and Grass pastures (Wilson et al., 2020). The lower urea in plasma mainly contributed to the lower urea:creatinine ratio in Forb compared with the other groups. However, besides urea, we also detected in the cows that graze Forb pastures a significant decrease of creatinine in plasma (current manuscript) and in urine [as shown in the prior manuscript (Wilson et al., 2020)].
The plasma creatinine levels in healthy animals are typically associated with muscle mass (Baxmann et al., 2008), as its production is the result of creatine catabolism mainly in the heart and skeletal muscle (Wyss and Kaddurah-Daouk, 2000). The continuous clearance of creatinine by the kidney has led to creatinine levels in the blood being used as indicators of kidney function, with the high levels of blood creatinine pointing toward the issues with glomerular filtration (Murayama et al., 2013). The low level of plasma creatinine found in the cows that graze Forb and Legume in comparison with Grass pastures points toward an effect of those pastures on the function of the kidney. For animals in Forb, this is likely caused by the plantain and chicory, as both increase the urine volume and frequency of urination in ruminants, as previously observed (Mangwe et al., 2019; Bryant et al., 2020). The secondary metabolites may be having an additional effect, as the extract from a similar plantain variety (Plantago asiatica) inhibits the angiotensin-converting enzyme (Tong et al., 2019). By this mechanism, reabsorption by the kidney would be limited and urine volume would increase. Although the creatinine levels were significantly lower in the Forb and Legume groups compared with the Grass group, all of the values remained within the normal range for dairy cattle (Cozzi et al., 2011). This, taken with the fact that the differences in urea:creatinine ratio were primarily driven by the differences in urea indicate that the positive impact of the Forb and Legume pasture varieties on kidney function may have been minimal.
Data on NRF2 Activity by Serum From the Cows Grazing Pastures Are Inconclusive
An NRF2 is an important transcription factor responsible for the upregulation of a wide range of genes associated with the cellular antioxidant defense and survival (Tonelli et al., 2018). The activation of NRF2 has been achieved with a range of natural products, some of which are known to be present in some of the plant varieties used in this study (Iranshahy et al., 2018).
We analyzed the samples in several ways to identify any effect if present; however, our data clearly indicate no statistically significant effect on the activation of NRF2 by the serum obtained from the cows grazing the various experimental pastures. This effect was somewhat surprising considering that serum from the cows grazing in those pastures contains a higher concentration of secondary compounds that are known to activate NRF2 in the monogastric animals, such as formononetin (Aladaileh et al., 2019), equol (Lin et al., 2016), and kaempferol (Yuan et al., 2021); however, all the above data were obtained in monogastric and there is a lack of information specific to bovine or ruminants. Our data also confirmed prior observation of reduced activation of NRF2 when the cells are cultivated in blood serum compared with when cultivated in DMEM with 10% fetal bovine serum (Ford et al., 2021). The reason is unclear for such an effect with possibly some effects by the presence of albumin, as previously demonstrated (Ford et al., 2021). The ex-vivo method we used is not a perfect representation of the in vivo situation but can somewhat reflect the response in vivo of the cells that are reached by the serum from the circulating blood since the cells in our model are affected by all the compounds in serum present in the extracellular space surrounding the cells. Thus, a more realistic effect on NRF2 activation in vivo can be inferred.
Blood Plasma Enriched With Pasture Plant Secondary Compounds
Formononetin is known to be very abundant in Legumes and from 3-to 180-fold more abundant than chicory (Andersen et al., 2009). We did not measure plant secondary compounds in our pastures; however, the higher amount of formononetin in plasma of the cows grazed on Forb compared with the cows grazed on Legume and higher abundance of daidzein, genistein, and equol in the latter might indicate a higher rumen metabolism of those compounds in the cows grazing Legume vs. Forb pastures. In the rumen, formononetin is quickly demethylated to daidzein and then metabolized to equol while biochanin A is demethylated to genistein (Dickinson et al., 1988). Equol is the major metabolic product of formononetin utilization by bacteria in the rumen (Dickinson et al., 1988). The high levels of equol found in the blood of the cows grazing Legume align with the previous findings where high levels of equol were found in the milk of cows grazing red clover-rich pasture (Andersen et al., 2009). However, formononetin, which was found to be highest in the milk of cows grazing the red clover pasture in a previous study (Andersen et al., 2009), was found to be low in the blood of cows grazing the Legume pasture in the current study, slightly higher than what was observed for the animals grazing Grass. The reason is unclear for the higher amount of formononetin in plasma of the cows grazed Forb; however, it is possible that Forb, containing chicory, might have inhibited the metabolism of the low amount of formononetin present in the Forb pasture to equol by inhibition of the enzymatic demethylation of formononetin. Inhibition can occur in the rumen and/or in the liver, as both the bacterial and mammalian cytochromes P450 have been shown to demethylate formononetin (Hur and Rafii, 2000; Hu et al., 2003). We do not have sufficient data to support the inhibition of formononetin metabolism or to provide an explanation of our findings.
A higher amount of isoflavonoids can compromise fertility (Reed, 2016), with equol playing an important role (Marley et al., 2011). The irilone has estrogenic activity, as observed in humans (Lutter et al., 2014). However, there is no data available on ruminants. Thus, the high concentration of irilone in plasma of the cows grazing pastures, particularly in the cows that graze Forb and Legume pastures in the current study, warrants further investigation, considering the potential effect on reproduction. Overall, our data clearly show that the plant secondary compounds are affected by the type of pasture; although the biological significance is not fully clear, it is likely that higher presence of those compounds helped to improve the antioxidant status of the animals grazed on Forb and Legume (KríŽová et al., 2019).
Limitations
A limitation of this work was the absence of the secondary compound analysis of the pasture samples. This information would have helped to interpret the results of the plant secondary compounds detected in the plasma of the cows. Unfortunately, the samples originally collected and used to measure the nutritive analysis as previously reported (Wilson et al., 2020) but were discarded by mistake and, thus, they were not available for analysis.
Conclusions
The results of the current study indicate that both the Forb and Legume pastures positively affected various degrees of liver function, antioxidant status, and kidney function in dairy cows when compared with a traditional Grass pasture. The benefits attained from these pastures can be linked to the composition of the pastures themselves and the effects of these alternative forage species within the animals, as evidenced by the changes in blood biochemical parameters, such as the plant secondary compounds. In addition, the Forb and Legume pastures seem to be imparting antioxidant benefits partly by enriching the blood with isoflavonoids. However, this effect was likely not determined by the regulation of the transcription factor NRF2.
Data Availability Statement
The original contributions presented in the study are included in the article/supplementary material, further inquiries can be directed to the corresponding author/s.
Ethics Statement
All procedures were approved by the Oregon State University Animal Care and Use Committee (ACUP Number: 5206).
Author Contributions
HF performed the NRF2 experiment, helped to perform the in vivo study, to collect the blood samples, analyzed the data, helped to interpret the data, and wrote the manuscript. SB helped to interpret the data, collect the blood, to write the manuscript, and revised the manuscript. ET performed the blood analysis, proposed the blood parameters to run, helped to interpret the data, and revised the manuscript. RM and RB analyzed plasma for botanical compounds and helped write the manuscript. MB helped to collect the blood samples, decide the blood parameters to run, interpret the data, build the graph and tables, helped writing the manuscript, and revised the final manuscript. SA conceived the idea, designed and performed the in vivo experiment, helped to interpret the data, to write the manuscript, and revised the final manuscript. All authors contributed to the article and approved the submitted version.
Funding
The funding for this study is provided by the Oregon Dairy Farmers Association, Oregon, United States.
Conflict of Interest
The authors declare that the research was conducted in the absence of any commercial or financial relationships that could be construed as a potential conflict of interest.
Publisher's Note
All claims expressed in this article are solely those of the authors and do not necessarily represent those of their affiliated organizations, or those of the publisher, the editors and the reviewers. Any product that may be evaluated in this article, or claim that may be made by its manufacturer, is not guaranteed or endorsed by the publisher.
Acknowledgments
The authors acknowledge the help of Angela Krueger, Lorena Carmona-Flores, Randi Wilson, Yunus Gultekin, Danielle Cacdac, Kaylee Marr, and Masoud Moradi for their assistance in collecting the blood samples and Giorgia Lovotti and Silvia Zini for their analysis of the plasma parameters performed in Italy. We also thank Shimadzu Scientific Instruments for providing the 9,030 QToF mass spectrometer used during this investigation.
References
Aladaileh, S. H., Hussein, O. E., Abukhalil, M. H., Saghir, S. A. M., Bin-Jumah, M., et al. (2019). Formononetin upregulates Nrf2/HO-1 signaling and prevents oxidative stress, inflammation, and kidney injury in methotrexate-induced rats. Antioxidants 8:430. doi: 10.3390/antiox8100430
Andersen, C., Nielsen, T. S., Purup, S., Kristensen, T., Eriksen, J., Soegaard, K., et al. (2009). Phyto-oestrogens in herbage and milk from cows grazing white clover, red clover, lucerne or chicory-rich pastures. Animal 3, 1189–1195. doi: 10.1017/S1751731109004613
Banne, A. F., Amiri, A., and Pero, R. W. (2003). Reduced level of serum thiols in patients with a diagnosis of active disease. J. Anti Aging Med. 6, 327–334. doi: 10.1089/109454503323028920
Baxmann, A. C., Ahmed, M. S., Marques, N. C., Menon, V. B., Pereira, A. B., Kirsztajn, G. M., et al. (2008). Influence of muscle mass and physical activity on serum and urinary creatinine and serum cystatin C. Clin. J. Am. Soc. Nephrol. 3, 348–354. doi: 10.2215/CJN.02870707
Bernabucci, U., Ronchi, B., Lacetera, N., and Nardone, A. (2002). Markers of oxidative status in plasma and erythrocytes of transition dairy cows during hot season. J. Dairy Sci. 85, 2173–2179. doi: 10.3168/jds.S0022-0302(02)74296-3
Bionaz, M., Trevisi, E., Calamari, L., Librandi, F., Ferrari, A., and Bertoni, G. (2007). Plasma paraoxonase, health, inflammatory conditions, and liver function in transition dairy cows. J. Dairy Sci. 90, 1740–1750. doi: 10.3168/jds.2006-445
Bionaz, M., Vargas-Bello-Pérez, E., and Busato, S. (2020). Advances in fatty acids nutrition in dairy cows: from gut to cells and effects on performance. J. Anim. Sci. Biotechnol. 11:110. doi: 10.1186/s40104-020-00512-8
Booth, N. L., Overk, C. R., Yao, P., Burdette, J. E., Nikolic, D., Chen, S. N., et al. (2006). The chemical and biologic profile of a red clover (Trifolium pratense L.) phase II clinical extract. J. Altern. Complement. Med. 12, 133–139. doi: 10.1089/acm.2006.12.133
Boué, S. M., Wiese, T. E., Nehls, S., Burow, M. E., Elliott, S., Carter-Wientjes, C. H., et al. (2003). Evaluation of the estrogenic effects of legume extracts containing phytoestrogens. J. Agric. Food Chem. 51, 2193–2199. doi: 10.1021/jf021114s
Bryant, R. H., Snow, V. O., Shorten, P. R., and Welten, B. G. (2020). Can alternative forages substantially reduce N leaching? Findings from a review and associated modelling. New Zealand J. Agric. Res. 63, 3–28. doi: 10.1080/00288233.2019.1680395
Campione, A., Natalello, A., Valenti, B., Luciano, G., Rufino-Moya, P. J., Avondo, M., et al. (2020). Effect of feeding hazelnut skin on animal performance, milk quality, and rumen fatty acids in lactating ewes. Animals 10:588. doi: 10.3390/ani10040588
Chen, X. T., Yang, S., Yang, Y. M., Zhao, H. L., Chen, Y. C., Zhao, X. H., et al. (2018). Exploring the relationship of peripheral total bilirubin, red blood cell, and hemoglobin with blood pressure during childhood and adolescence. J. Pediatr. (Rio J) 94, 532–538. doi: 10.1016/j.jped.2017.07.018
Christensen, R. G., Yang, S. Y., Eun, J. S., Young, A. J., Hall, J. O., and Macadam, J. W. (2015). Effects of feeding birdsfoot trefoil hay on neutral detergent fiber digestion, nitrogen utilization efficiency, and lactational performance by dairy cows1. J. Dairy Sci. 98, 7982–7992. doi: 10.3168/jds.2015-9348
Cozzi, G., Ravarotto, L., Gottardo, F., Stefani, A. L., Contiero, B., Moro, L., et al. (2011). Short communication: reference values for blood parameters in Holstein dairy cows: effects of parity, stage of lactation, and season of production. J. Dairy Sci. 94, 3895–3901. doi: 10.3168/jds.2010-3687
Defrain, J. M., Hippen, A. R., Kalscheur, K. F., and Schingoethe, D. J. (2004). Feeding lactose increases ruminal butyrate and plasma β-hydroxybutyrate in lactating dairy cows. J. Dairy Sci. 87, 2486–2494. doi: 10.3168/jds.S0022-0302(04)73373-1
Deng, Y., Pang, Q., Miao, R. C., Chen, W., Zhou, Y. Y., Bi, J. B., et al. (2016). Prognostic significance of pretreatment albumin/globulin ratio in patients with hepatocellular carcinoma. Onco. Targets. Ther. 9, 5317–5328. doi: 10.2147/OTT.S109736
Dickinson, J. M., Smith, G. R., Randel, R. D., and Pemberton, I. J. (1988). In vitro metabolism of formononetin and biochanin A in bovine rumen fluid. J. Anim. Sci. 66, 1969–1973. doi: 10.2527/jas1988.6681969x
Distel, R. A., Arroquy, J. I., Lagrange, S., and Villalba, J. J. (2020). Designing diverse agricultural pastures for improving ruminant production systems. Front. Sustain. Food Syst. 4:596869. doi: 10.3389/fsufs.2020.596869
Elgersma, A. (2015). Grazing increases the unsaturated fatty acid concentration of milk from grass-fed cows: a review of the contributing factors, challenges and future perspectives. Eur. J. Lipid Sci. Technol. 117, 1345–1369. doi: 10.1002/ejlt.201400469
Ford, H. R., Busato, S., and Bionaz, M. (2021). In vitro–In vivo hybrid approach for studying modulation of NRF2 in immortalized bovine mammary cells. Front. Vet. Sci. 2:674355. doi: 10.3389/fanim.2021.674355
Gao, X., and Oba, M. (2016). Effect of increasing dietary non-fiber carbohydrate with starch, sucrose, or lactose on rumen fermentation and productivity of lactating dairy cows. J. Dairy Sci. 99, 291–300. doi: 10.3168/jds.2015-9871
Ghaffari, S. (2008). Oxidative stress in the regulation of normal and neoplastic hematopoiesis. Antioxid. Redox Signal. 10, 1923–1940. doi: 10.1089/ars.2008.2142
Gooneratne, S. R., Buckley, W. T., and Christensen, D. A. (1989). Review of copper deficiency and metabolism in ruminants. Can. J. Anim. Sci. 69, 819–845. doi: 10.4141/cjas89-096
Hashem, N. M., El-Azrak, K. M., and Sallam, S. M. A. (2016). Hormonal concentrations and reproductive performance of Holstein heifers fed trifolium alexandrinum as a phytoestrogenic roughage. Anim. Reprod. Sci. 170, 121–127. doi: 10.1016/j.anireprosci.2016.04.012
Haubold, S., Kröger-Koch, C., Starke, A., Tuchscherer, A., Tröscher, A., Kienberger, H., et al. (2020). Effects of abomasal infusion of essential fatty acids and conjugated linoleic acid on performance and fatty acid, antioxidative, and inflammatory status in dairy cows. J. Dairy Sci. 103, 972–991. doi: 10.3168/jds.2019-17135
Hogan, J. S., Smith, K. L., Weiss, W. P., Todhunter, D. A., and Schockey, W. L. (1990). Relationships among vitamin E, selenium, and bovine blood neutrophils. J. Dairy Sci. 73, 2372–2378. doi: 10.3168/jds.S0022-0302(90)78920-5
Høstmark, A. T. (1995). Serum fatty acid/albumin molar ratio and the risk of diseases. Med. Hypotheses 44, 539–541. doi: 10.1016/0306-9877(95)90520-0
Hu, M., Krausz, K., Chen, J., Ge, X., Li, J., Gelboin, H. L., et al. (2003). Identification of CYP1A2 as the main isoform for the phase I hydroxylated metabolism of genistein and a prodrug converting enzyme of methylated isoflavones. Drug Metab. Dispos. 31, 924–931. doi: 10.1124/dmd.31.7.924
Hur, H., and Rafii, F. (2000). Biotransformation of the isoflavonoids biochanin A, formononetin, and glycitein by Eubacterium limosum. FEMS Microbiol. Lett. 192, 21–25. doi: 10.1111/j.1574-6968.2000.tb09353.x
Hymes-Fecht, U. C., Broderick, G. A., Muck, R. E., and Grabber, J. H. (2013). Replacing alfalfa or red clover silage with birdsfoot trefoil silage in total mixed rations increases production of lactating dairy cows1. J. Dairy Sci. 96, 460–469. doi: 10.3168/jds.2012-5724
Iranshahy, M., Iranshahi, M., Abtahi, S. R., and Karimi, G. (2018). The role of nuclear factor erythroid 2-related factor 2 in hepatoprotective activity of natural products: a review. Food Chem. Toxicol. 120, 261–276. doi: 10.1016/j.fct.2018.07.024
Jaaf, S., Batty, B., Krueger, A., Estill, C. T., and Bionaz, M. (2020). Selenium biofortified alfalfa hay fed in low quantities improves selenium status and glutathione peroxidase activity in transition dairy cows and their calves. J. Dairy Res. 87, 184–190. doi: 10.1017/S002202992000028X
Jaturasitha, S., Norkeaw, R., Vearasilp, T., Wicke, M., and Kreuzer, M. (2009). Carcass and meat quality of Thai native cattle fattened on Guinea grass (Panicum maxima) or Guinea grass-legume (Stylosanthes guianensis) pastures. Meat Sci. 81, 155–162. doi: 10.1016/j.meatsci.2008.07.013
Krinsky, N. I. (1992). Mechanism of action of biological antioxidants. Proc. Soc. Exp. Biol. Med. 200, 248–254. doi: 10.3181/00379727-200-43429
KríŽová, L., Dadáková, K., Kašparovská, J., and Kašparovský, T. (2019). Isoflavones. Molecules (Basel, Switzerland) 24:1076. doi: 10.3390/molecules24061076
Lee, J. M., Clark, D. A., Clark, C. E. F., Waugh, C. D., Roach, C. G., Minneé, E. M. K., et al. (2018). A comparison of perennial ryegrass-and tall fescue-based swards with or without a cropping component for dairy production: animal production, herbage characteristics and financial performance from a 3-year farmlet trial. Grass Forage Sci. 73, 340–354. doi: 10.1111/gfs.12334
Lin, J., and Denker, M. (2015). Chapter 61: Azotemia and Urinary Abnormalities. New York, NY: McGraw-Hill Education.
Lin, X., Jiang, S., Jiang, Z., Zheng, C., and Gou, Z. (2016). Effects of equol on H2O2-induced oxidative stress in primary chicken intestinal epithelial cells. Poult. Sci. 95, 1380–1386. doi: 10.3382/ps/pew034
Lutter, S., Schmalbach, K., Esch, H. L., and Lehmann, L. (2014). The isoflavone irilone contributes to the estrogenic potential of dietary supplements containing red clover. Arch. Toxicol. 88, 309–321. doi: 10.1007/s00204-013-1114-5
Mangwe, M. C., Bryant, R. H., Beck, M. R., Beale, N., Bunt, C., and Gregorini, P. (2019). Forage herbs as an alternative to ryegrass-white clover to alter urination patterns in grazing dairy systems. Anim. Feed Sci. Technol. 252, 11–22. doi: 10.1016/j.anifeedsci.2019.04.001
Marley, C., Mccalman, H., Buckingham, S., Downes, D., and Abberton, M. (2011). A Review of the Effect of Legumes on Ewe and Cow Fertility. AHDB.
Mayasari, N., Chen, J., Ferrari, A., Bruckmaier, R. M., Kemp, B., Parmentier, H. K., et al. (2017). Effects of dry period length and dietary energy source on inflammatory biomarkers and oxidative stress in dairy cows. J. Dairy Sci. 100, 4961–4975. doi: 10.3168/jds.2016-11857
Mezzetti, M., Minuti, A., Piccioli-Cappelli, F., and Trevisi, E. (2020). Inflammatory status and metabolic changes at dry-off in high-yield dairy cows. Ital. J. Anim. Sci. 19, 51–65. doi: 10.1080/1828051X.2019.1691472
Miettinen, H., and Huhtanen, P. (1996). Effects of the ratio of ruminal propionate to butyrate on milk yield and blood metabolites in dairy cows. J. Dairy Sci. 79, 851–861. doi: 10.3168/jds.S0022-0302(96)76434-2
Min, B. R., Gurung, N., Shange, R., and Solaiman, S. (2019). Potential role of rumen microbiota in altering average daily gain and feed efficiency in meat goats fed simple and mixed pastures using bacterial tag-encoded FLX amplicon pyrosequencing1. J. Anim. Sci. 97, 3523–3534. doi: 10.1093/jas/skz193
Minneé, E. M. K., Waghorn, G. C., Lee, J. M., and Clark, C. E. F. (2017). Including chicory or plantain in a perennial ryegrass/white clover-based diet of dairy cattle in late lactation: feed intake, milk production and rumen digestion. Anim. Feed Sci. Technol. 227, 52–61. doi: 10.1016/j.anifeedsci.2017.03.008
Mir, A. A., Goyal, B., Datta, S. K., Ikkurthi, S., and Pal, A. (2016). Comparison between measured and calculated free calcium values at different serum albumin concentrations. J. Lab. Physicians 8, 71–76. doi: 10.4103/0974-2727.180785
Moorby, J. M., Fraser, M. D., Theobald, V. J., Wood, J. D., and Haresign, W. (2004). The effect of red clover formononetin content on live-weight gain, carcass characteristics and muscle equol content of finishing lambs. Anim. Sci. 79, 303–313. doi: 10.1017/S1357729800090160
Moore, K. J., White, T. A., Hintz, R. L., Patrick, P. K., and Brummer, E. C. (2004). Sequential grazing of cool-and warm-season pastures. Agron. J. 96, 1103–1111. doi: 10.2134/agronj2004.1103
Muchiri, R. N., and Van Breemen, R. B. (2020). Single-laboratory validation of UHPLC-MS/MS assays for red clover isoflavones in human serum and dietary supplements. J. AOAC Int. 103, 1160–1166. doi: 10.1093/jaoacint/qsaa033
Mueller-Harvey, I., Bee, G., Dohme-Meier, F., Hoste, H., Karonen, M., Kölliker, R., et al. (2019). Benefits of condensed tannins in forage legumes fed to ruminants: importance of structure, concentration, and diet composition. Crop Sci. 59, 861–885. doi: 10.2135/cropsci2017.06.0369
Murayama, I., Miyano, A., Sasaki, Y., Kimura, A., Sato, S., and Furuhama, K. (2013). Glomerular filtration rate in Holstein dairy cows estimated from a single blood sample using iodixanol. J. Dairy Sci. 96, 5120–5128. doi: 10.3168/jds.2013-6884
Mustonen, E. A., Tuori, M., Saastamoinen, I., Taponen, J., Wähälä, K., Saloniemi, H., et al. (2009). Equol in milk of dairy cows is derived from forage legumes such as red clover. Br. J. Nutr. 102, 1552–1556. doi: 10.1017/S0007114509990857
Oh, R. C., and Hustead, T. R. (2011). Causes and evaluation of mildly elevated liver transaminase levels. Am. Fam. Physician 84, 1003–1008.
Osorio, J. S., and Bionaz, M. (2017). Plasmid transfection in bovine cells: optimization using a realtime monitoring of green fluorescent protein and effect on gene reporter assay. Gene 626, 200–208. doi: 10.1016/j.gene.2017.05.025
Pembleton, K. G., Cullen, B. R., Rawnsley, R. P., Harrison, M. T., and Ramilan, T. (2016). Modelling the resilience of forage crop production to future climate change in the dairy regions of southeastern Australia using APSIM. J. Agric. Sci. 154, 1131–1152. doi: 10.1017/S0021859615001185
Peña-Espinoza, M., Valente, A. H., Thamsborg, S. M., Simonsen, H. T., Boas, U., Enemark, H. L., et al. (2018). Antiparasitic activity of chicory (Cichorium intybus) and its natural bioactive compounds in livestock: a review. Parasites Vectors 11:475. doi: 10.1186/s13071-018-3012-4
Politis, I., Bizelis, I., Tsiaras, A., and Baldi, A. (2004). Effect of vitamin E supplementation on neutrophil function, milk composition and plasmin activity in dairy cows in a commercial herd. J. Dairy Res. 71, 273–278. doi: 10.1017/S002202990400010X
Pu, W., Wang, D., and Zhou, D. (2015). Structural characterization and evaluation of the antioxidant activity of phenolic compounds from astragalus taipaishanensis and their structure-activity relationship. Sci. Rep. 5:13914. doi: 10.1038/srep13914
Ramos-Morales, E., Rossi, G., Cattin, M., Jones, E., Braganca, R., and Newbold, C. J. (2018). The effect of an isoflavonid-rich liquorice extract on fermentation, methanogenesis and the microbiome in the rumen simulation technique. FEMS Microbiol. Ecol. 94:fiy009. doi: 10.1093/femsec/fiy009
Reed, K. F. M. (2016). Fertility of herbivores consuming phytoestrogen-containing medicago and trifolium species. Agriculture 6:35. doi: 10.3390/agriculture6030035
Reynolds, C. K., Aikman, P. C., Lupoli, B., Humphries, D. J., and Beever, D. E. (2003). Splanchnic metabolism of dairy cows during the transition from late gestation through early lactation. J. Dairy Sci. 86, 1201–1217. doi: 10.3168/jds.S0022-0302(03)73704-7
Roche, J. R., Kay, J. K., Phyn, C. V. C., Meier, S., Lee, J. M., and Burke, C. R. (2010). Dietary structural to non-fiber carbohydrate concentration during the transition period in grazing dairy cows. J. Dairy Sci. 93, 3671–3683. doi: 10.3168/jds.2009-2868
Rochu, D., Chabrière, E., and Masson, P. (2010). “Chapter 6-paraoxonase-1 and its interactions with HDL: molecular structures of PON1 and HDL,” in The HDL Handjournal, ed T. Komoda (Boston: Academic Press), 99–131. doi: 10.1016/B978-0-12-382171-3.10006-3
Roland, L., Drillich, M., and Iwersen, M. (2014). Hematology as a diagnostic tool in bovine medicine. J. Vet. Diagn. Invest. 26, 592–598. doi: 10.1177/1040638714546490
Rosa, F., Osorio, J. S., Trevisi, E., Yanqui-Rivera, F., Estill, C. T., and Bionaz, M. (2017). 2,4-thiazolidinedione treatment improves the innate immune response in dairy goats with induced subclinical mastitis. PPAR Res. 2017:7097450. doi: 10.1155/2017/7097450
Saha, S., and Kroon, P. A. (2020). A simple and rapid LC-MS/MS method for quantification of total daidzein, genistein, and equol in human urine. J. Anal. Methods Chem. 2020:2359397. doi: 10.1155/2020/2359397
Sivesind, E., and Seguin, P. (2005). Effects of the environment, cultivar, maturity, and preservation method on red clover isoflavone concentration. J. Agric. Food Chem. 53, 6397–6402. doi: 10.1021/jf0507487
Stark, J. (1998). Interpretation of BUN and serum creatinine. An interactive exercise. Crit. Care Nurs. Clin. North Am. 10, 491–496. doi: 10.1016/S0899-5885(18)30194-1
Stocks, S. E., and Allen, M. S. (2012). Hypophagic effects of propionate increase with elevated hepatic acetyl coenzyme A concentration for cows in the early post-partum period. J. Dairy Sci. 95, 3259–3268. doi: 10.3168/jds.2011-4991
Tamura, Y., and Nishibe, S. (2002). Changes in the concentrations of bioactive compounds in plantain leaves. J. Agric. Food Chem. 50, 2514–2518. doi: 10.1021/jf011490x
Theodorou, M. K., Kingston-Smith, A. H., Winters, A. L., Lee, M. R. F., Minchin, F. R., Morris, P., et al. (2006). Polyphenols and their influence on gut function and health in ruminants: a review. Environ. Chem. Lett. 4, 121–126. doi: 10.1007/s10311-006-0061-2
Thorndyke, M. P., Guimaraes, O., Tillquist, N. M., Zervoudakis, J. T., and Engle, T. E. (2020). Molybdenum exposure in drinking water vs. feed impacts apparent absorption of copper differently in beef cattle consuming a high-forage diet. Biol. Trace Elem. Res. 199, 2913–2918. doi: 10.1007/s12011-020-02440-0
Tonelli, C., Chio, I. I. C., and Tuveson, D. A. (2018). Transcriptional regulation by Nrf2. Antioxid. Redox Signal. 29, 1727–1745. doi: 10.1089/ars.2017.7342
Tong, R. C., Qi, M., Yang, Q. M., Li, P. F., Wang, D. D., Lan, J. P., et al. (2019). Extract of Plantago asiatica L. Seeds ameliorates hypertension in spontaneously hypertensive rats by inhibition of angiotensin converting enzyme. Front. Pharmacol. 10:403. doi: 10.3389/fphar.2019.00403
Verma, M. K., Jaiswal, A., Sharma, P., Kumar, P., and Singh, A. N. (2019). Oxidative stress and biomarker of TNF-α, MDA and FRAP in hypertension. J. Med. Life 12, 253–259. doi: 10.25122/jml-2019-0031
Wang, K., Jin, X. L., Shen, X. G., Sun, L. P., Wu, L. M., Wei, J. Q., et al. (2016). Effects of Chinese propolis in protecting bovine mammary epithelial cells against mastitis pathogens-induced cell damage. Mediators Inflamm. 2016:8028291. doi: 10.1155/2016/8028291
Wang, L., Zhang, G., Li, Y., and Zhang, Y. (2020). Effects of high forage/concentrate diet on volatile fatty acid production and the microorganisms involved in VFA production in cow rumen. Animals (Basel) 10:223. doi: 10.3390/ani10020223
Wei, Z., Zhang, B., and Liu, J. (2018). Effects of the dietary non-fiber carbohydrate content on lactation performance, rumen fermentation, and nitrogen utilization in mid-lactation dairy cows receiving corn stover. J. Anim. Sci. Biotechnol. 9:20. doi: 10.1186/s40104-018-0239-z
Whelan, S. J., Mulligan, F. J., Gath, V., Flynn, B., and Pierce, K. M. (2014). Short communication: effect of dietary manipulation of crude protein content and non-fibrous-to-fibrous-carbohydrate ratio on energy balance in early-lactation dairy cows. J. Dairy Sci. 97, 7220–7224. doi: 10.3168/jds.2013-7452
Wilson, R. L., Bionaz, M., Macadam, J. W., Beauchemin, K. A., Naumann, H. D., and Ates, S. (2020). Milk production, nitrogen utilization, and methane emissions of dairy cows grazing grass, forb, and legume-based pastures. J. Anim. Sci. 98:skaa220. doi: 10.1093/jas/skaa220
Witko-Sarsat, V., Friedlander, M., Nguyen Khoa, T., Capeillère-Blandin, C., Nguyen, A. T., Canteloup, S., et al. (1998). Advanced oxidation protein products as novel mediators of inflammation and monocyte activation in chronic renal failure. J. Immunol. 161, 2524–2532.
Wyss, M., and Kaddurah-Daouk, R. (2000). Creatine and creatinine metabolism. Physiol. Rev. 80, 1107–1213. doi: 10.1152/physrev.2000.80.3.1107
Yuan, Y., Zhai, Y., Chen, J., Xu, X., and Wang, H. (2021). Kaempferol ameliorates oxygen-glucose deprivation/reoxygenation-induced neuronal ferroptosis by activating Nrf2/SLC7A11/GPX4 Axis. Biomolecules 11:923. doi: 10.3390/biom11070923
Keywords: grazing, blood parameters, chicory, plantain, Legume, dairy cows, plant secondary compounds
Citation: Ford HR, Busato S, Trevisi E, Muchiri RN, van Breemen RB, Bionaz M and Ates S (2021) Effects of Pasture Type on Metabolism, Liver and Kidney Function, Antioxidant Status, and Plant Secondary Compounds in Plasma of Grazing, Jersey Dairy Cattle During Mid-lactation. Front. Anim. Sci. 2:729423. doi: 10.3389/fanim.2021.729423
Received: 23 June 2021; Accepted: 23 September 2021;
Published: 25 October 2021.
Edited by:
James Levi Klotz, Agricultural Research Service (USDA), United StatesReviewed by:
Søren Krogh Jensen, Aarhus University, DenmarkAhmed Elolimy, University of Arkansas for Medical Sciences, United States
Brittany Harlow, Agricultural Research Service (USDA), United States
Copyright © 2021 Ford, Busato, Trevisi, Muchiri, van Breemen, Bionaz and Ates. This is an open-access article distributed under the terms of the Creative Commons Attribution License (CC BY). The use, distribution or reproduction in other forums is permitted, provided the original author(s) and the copyright owner(s) are credited and that the original publication in this journal is cited, in accordance with accepted academic practice. No use, distribution or reproduction is permitted which does not comply with these terms.
*Correspondence: Serkan Ates, c2Vya2FuLmF0ZXNAb3JlZ29uc3RhdGUuZWR1