- 1Department of Animal Science, State University of Maringa, Maringá, Brazil
- 2BIOMIN Holding GmbH, Getzersdorf, Austria
This study evaluated the influence of a blended phytogenic feed additive on feed intake, feeding behavior, nutrient digestibility, and growth performance during feedlot adaptation, early, and late finishing periods as well as carcass traits. Twenty-six crossbred F1 Angus × Nellore bulls (19 mo ± 4 d) were housed in individually pens and fed a high-forage receiving diet for 7 days. At the end of the receiving period, bulls were weighted [initial shrunk body weight (SBW) 363 ± 20 kg], blocked by SBW and randomly assigned to two treatments; Control: without any additive or PHY: 150 ppm of a phytogenic feed additive fed throughout the adaptation and finishing phases. Bulls were transitioned through four steps over 18 days to a high-grain finishing diet (as % DM, 64% rehydrated corn grain silage, 19% corn gluten feed, 13% sugar cane bagasse and 4% minerals, urea, and vitamins mix). The finishing phase lasted 68 days, with mid-point measurements dividing early and late finishing period. The PHY group DMI was greater during adaptation and late finishing periods (P < 0.05), with a tendency during early finishing period (P = 0.06). Number of daily meals was similar between treatments (P = 0.52), but an increased meal length was noted for PHY group (P < 0.05), which contributed to their greater DMI. Diet digestibility remained similar between groups during the finishing periods (P > 0.1). Ruminitis scores were low and liver abscess similar between treatments. Final SBW tended to be higher (P = 0.09) and hot carcass weight was greater for the PHY group (P < 0.05), with no differences on dressing percentage, ribeye area and marbling score. In conclusion, the PHY treatment had positive effects on intake and carcass weight, without increasing metabolic disorders.
Introduction
Beef production intensification is steeply increasing in Brazil, mainly in the last two decades. Among intensification strategies, feeding of high concentrate diets during the finishing phase have been adopted as a tool for shortening the production period and improve meat quality. As a consequence of intensive practices, cattle are subject to higher metabolic load and more vulnerable to health challenges, particularly during the initial receiving period, that impacts animal productivity and welfare (Brown et al., 2006). Challenges include transportation, mixing of animals, introducing new environments and diet transition (Cooke, 2017). Resilience against these challenges is often further compounded by low initial feed intake, contributing to low immunocompetence and the occurrence of clinical and subclinical disease, which impact overall performance (Lippolis et al., 2017).
Application of phytogenic feed additives (PFA) in animal production has currently received emphasis as a potential alternative to improve performance while meeting consumer desire to reduce none therapeutic pharmaceutical application in the food chain (Runjaic-Antic et al., 2010; Valenzuela-Grijalva et al., 2017). Phytogenic feed additives contain phytochemical compounds from a variety of herbs, spices and traditional medicinal plants, are purported to have a broad range of therapeutic activities, including anti-microbial, anti-inflammatory, anti-oxidative and anti-carcinogenic effects, as well as a capacity of enhancing gastric secretions and stimulating appetite (Bento et al., 2013; Nazzaro et al., 2013; Drong et al., 2017). The mechanisms underlying the observed effects are attributed to the diversity in chemical structures of phytochemicals and their interaction with various host receptors (Williams et al., 2020). Response to use of phytogenic feed additives for fattening ruminants varies, with reported improvements in feed efficiency (Valero et al., 2014), reduced liver abscess severity, improvements in DMI and ADG (Souza et al., 2019) as well as modulate protein utilization and methane production (Soltan et al., 2018), whereas others have reported no effect on performance (Rivaroli et al., 2017; Michels et al., 2018).
Given their potential complexity and inherent variability between sources, efficacy of PFA cannot be generalized between species, or indeed, production phase, thus must therefore be systematically evaluated within the application context. This study was undertaken to evaluate the effects of a blended PFA containing volatile and non-volatile phytochemicals on feeding behavior, performance and carcass traits of crossbred F1 Angus × Nellore bulls during different periods of feedlot finishing, with the assumption of improving animal performance over non-additive control bulls.
Materials and Methods
Animals, Facilities, and Feeding
This study was conducted as a randomized block design at the Iguatemi Experimental Farm of the State University of Maringá, Maringá, Brazil. All animal procedures were approved by the Animal Care and Use Committee (Protocol no. 6672311019).
Twenty-six crossbreed bulls (F1 Angus × Nellore) entered the trial (initial body weight of 363 ± 20 kg and 19 mo ± 4 d of age), after being raised under grazing conditions. Animals were acclimatized to the facilities during the first 7 days, in which animals were fed a high-forage receiving diet. At trial start on day 8, animals were blocked according to the shrunk body weight (SBW), housed in individual pens, and randomly allocated to two treatments within block (13 pens/treatment). Dietary treatments were a diet without any additive (Control) or a diet containing a phytogenic feed additive treatment (PHY; Digestarom®, BIOMIN Holding GmbH, Austria) applied at 150 ppm as per manufacturer recommendations. Digestarom® consists of 485 g/kg aromatic ingredients and is characterized as a blended of herbs, spices and their extracts or pure compounds that includes licorice, caraway, cinnamon, and vanilla. Dietary treatments were applied for a total of 86 days (d 8–d 94) and bulls were slaughtered at day 94 to assess the impact of the phytogenic feed additive on body weight gain, liver and rumen health, and carcass traits.
Pens (2 × 4 m) were located outdoors (open stable), with concrete floor, roof covering and exposed to ambient temperatures (avg. min. 20.5°C to avg. max. 30.0°C) and humidity (avg. 76%). Pens were equipped with individual feed bunk and water bowls. Animals had ad libitum access to water and feed, offered as a total mixed ration (TMR). The experimental diet during the first 7 days (Receiving diet) was based on 55% corn silage and 45% concentrate. Within the following 18 days, the diet was adapted in three steps of 6 days each (Adapt diets 1–3), to increase the proportion of concentrates to a level of 87% (Finishing diet), by decreasing 4%-units of physically effective NDF and increasing 2%-units of TDN at each step. The composition of the experimental diets is described in Table 1. Experimental diets were mixed and offered twice daily in amounts of 3% in excess of daily intake. The experimental PFA was pre-mixed with minerals and vitamins, urea and limestone and incorporated into the TMR during each feeding. No other additives were used in the experimental diets.
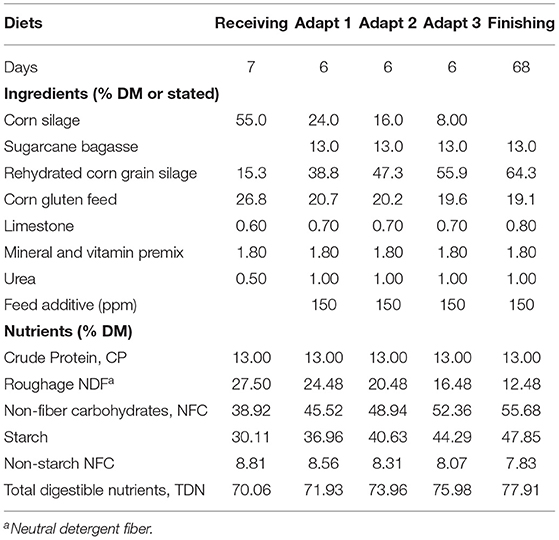
Table 1. Composition of experimental diets according to feeding phase of crossbred finishing bulls, over a trial period of in total 94 days (dry matter basis DM, unless otherwise stated).
Measurements and Animal Health
Shrunk body weight of animals was measured after 16 h of solid fasting four times during trial period (d 8–d 94): at trial start (d 8), after the adaptation period (d 25), in the middle of the finishing period (d 57) and at the end of the experiment (d 94). Offered and refused feed was measured daily and individual daily dry matter intake (DMI) was calculated as offered minus refused feed (DM basis). Variation in individual DMI was calculated as the difference of DMI between 2 days (Bevans et al., 2005). Average daily gain (ADG), average dry matter intake (DMI) and average feed efficiency (ADG/DMI) were calculated for the adaptation period, early, and late finishing period and the complete trial period. Once during the finishing period (d 55–56), individual feeding behavior (eating time, ruminating time, total chewing time, meal bolus, meal length, meal size, meal interval) was measured during a consecutive 48 h period by visual evaluation every 5 min (Maekawa et al., 2002). In addition to feeding behavior, sorting of the diet was determined by the particle sorting index (Leonardi and Armentano, 2003), measured during the adaptation period (d 24), in the middle of the finishing period (d 56) and at the end of the experiment (d 89). Sorting was estimated using a Penn State Particle Separator (19, 8, 4 mm and pan). Apparent total tract digestibility was measured at the beginning (d 37–40) and at the end (d 78–81) of the finishing period, using iNDF as internal marker (Huhtanen et al., 1994).
At the final weighing, carcass traits were evaluated by ultrasound (Aloka SSD500; 17-cm, 3.5-MHz probe). Ribeye area and back fat thickness were measured between the 12th and 13th rib transversally to the Longissimus muscle. Marbling score (1–10) was recorded from the 11th to 13th rib longitudinally to the Longissimus muscle. Biceps femoris fat thickness was also recorded. Afterward, animals were transported 110 km to a commercial abattoir and slaughtered according to animal welfare and slaughter practices established by the local sanitary inspection. After slaughter, carcass traits were evaluated by measuring hot carcass weight and dressing percentage. After evisceration, liver abscesses were scored according to incidence and severity as following: score 0 (without abscess), 1 (one or two abscesses with <2.5 cm or abscess scars), 2 (three to four abscesses <2.5 cm), and 3 (one abscess >2.5 cm or several small abscesses) (Brink et al., 1990). Rumen epithelium was scored according to the lesion incidence (0–10), where zero indicated no lesions or abnormalities and ten, severe lesions all over the rumen wall (Bigham and McManus, 1975). No signs of disease and mortality were observed during the trial period.
Statistical Analysis
Data was analyzed using the Mixed procedure of SAS v.9.4 (Cary, NC, USA). The model included a random effect of block and a fixed effect of treatment. In addition to the overall trend, data was analyzed separately for adaptation, early finishing, and late finishing periods as well as overall feeding period. Liver abscess and ruminitis scores were compared by Chi-square test. Significance was considered at P ≤ 0.05 and tendencies were considered for 0.05 < P ≤ 0.10.
Results
Adaptation Period, Day 8–25
During the initial adaptation period, DMI of the PHY bulls was greater than the Control bulls (P < 0.05, Table 2), with no noted difference in intake variation between the groups. By the end of the adaptation period, PHY bulls averaged 10 kg heavier than the Control bulls (P = 0.103). Evaluation of particle sorting index indicates the PHY group selected for mid-size particles (P < 0.05) and a tendency to sort against smaller particles (P = 0.08), with no influence of treatment on long particle selection. However, sorting index values between the groups for small and mid-size particles were within 1% of each other, suggesting minor or no biological relevance.
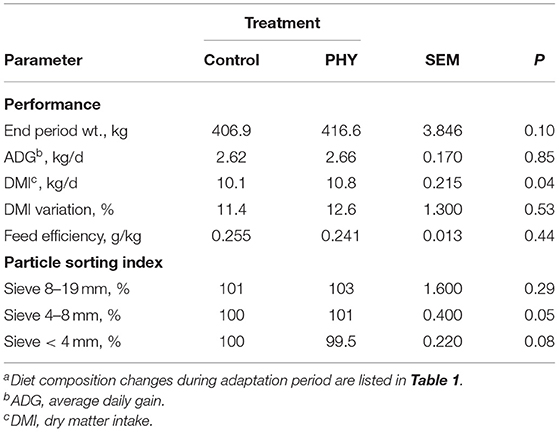
Table 2. Comparison of dry matter intake, average daily gain, and particle sorting index of crossbred finishing bulls during the adaptation period: from day 8 to day 25a.
Early Finishing Period, Day 26–57
Intake of the PHY bulls tended to be greater during the first half of the finishing period (P = 0.06, Table 3), with no difference in intake variation or particle sorting index. By the end of the feeding period, PHY bulls were 18.1 kg heavier than the Control bulls (P < 0.05). Measured parameters for apparent total tract digestibility were similar between the two treatments. In agreement, feed efficiency did not differ between the two groups.
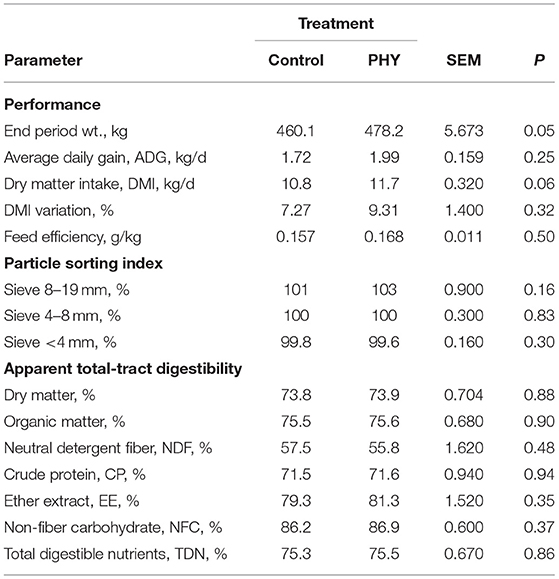
Table 3. Comparison of dry matter intake, average daily gain, particle sorting index, and apparent total tract digestibility of crossbred finishing bulls during finishing early finishing period: from day 26 to 57.
Late Finishing Period, Day 58–94
During the second half of the finishing period, the DMI of the PHY bulls was greater than that of the Control bulls (P < 0.05, Table 4). The sorting index and apparent total tract digestibility parameters were unaffected by treatment. Feed efficiency was similar between the treatments.
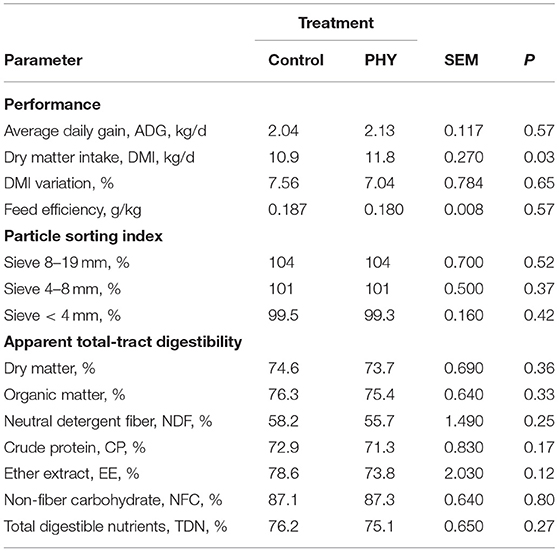
Table 4. Comparison of dry matter intake, average daily gain, particle sorting index, and apparent total tract digestibility of crossbred finishing bulls during late finishing period: from day 58 to 94.
Feeding Behavior During Finishing Period
Total eating, chewing and rumination time per 48 h period were similar between treatments (Table 5). The meal duration was greater (P < 0.05) for the PHY bulls, with similar eating and chewing rate. Combined, these observations may account for the observed differences in DMI during different stages of the trial.
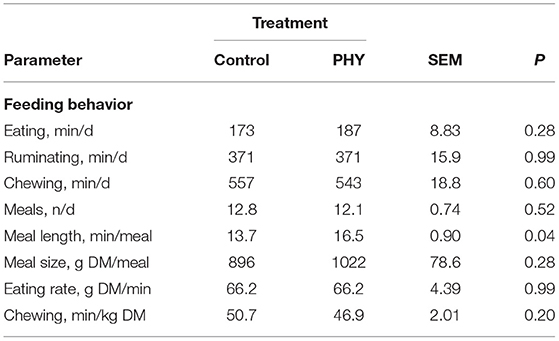
Table 5. Comparison of feeding behavior over 48 h of crossbred finishing bulls measured at mid-point of finishing period.
Overall Trial Period, Day 8–94
By the end of the trial, PHY bulls had a tendency toward heavier SBW (P = 0.09, Table 6), supported by a higher DMI (P < 0.05) throughout the trial. Overall, there were no noted differences in DMI variation or particle sorting index. Feed efficiency was similar between the treatments throughout the trial period, with the PHY bulls having a numerically greater ADG, lending to the observed live weights.
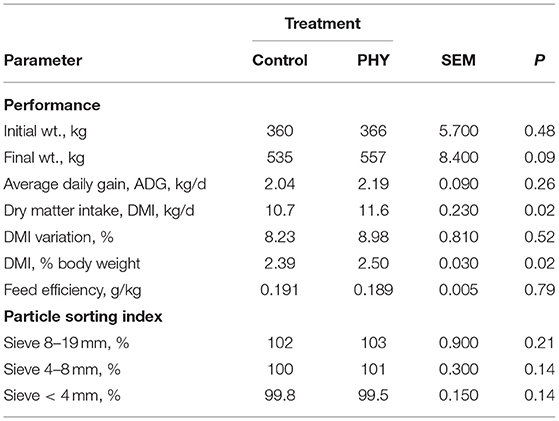
Table 6. Comparison of overall trial dry matter intake, average daily gain and particle sorting index, and apparent total tract digestibility of crossbred finishing bulls from day 8 to 94.
Carcass Traits and Wellness Evaluation
Comparison of hot carcass weights indicated that bulls from the PHY treatment were on average 15 kg heavier than those of the Control bulls (P < 0.05, Table 7). No significant differences were noted in dressing percentage, ribeye area, fat depth measurements, or marbling scores. Evaluation of wellness by ruminitis scores at slaughter indicated both groups were similar, and only one bull from the Control group had notable liver abscesses.
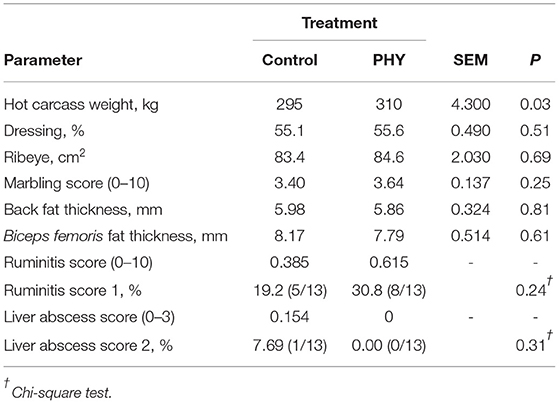
Table 7. Comparison of carcass traits, ruminitis score, and liver abscess score of crossbred finishing bulls.
Discussion
Adaptation Period
In this trial, crossbred F1 Angus × Nellore bulls were acclimatized to the individual penning and fed a forage-based receiving diet for 7 days prior to beginning the adaptation diets. Stimulating early feed intake during the adaptation period can often prove to be challenging as calves are adjusting to the new diets and experiencing multiple compounding stressors that can impair intake and added pressure on the immune system (Duff and Galyean, 2007). Diet changes during the adaptation period from receiver diet to a high starch finishing diet can often result in repeated bouts of sub- or clinical ruminal acidosis that can result in reduced intake or even put animals off feed (Brown et al., 2006). Furthermore, low ruminal pH is a catalyst for the release of gram-negative bacteria associated lipopolysaccharides and bioactive amines, e.g., histamine, that can cause inflammation and suppress anti-oxidative response, leading to impaired tight junction function in the gastro-intestinal tract, more commonly known as leaky gut (Abaker et al., 2017; Aschenbach et al., 2019). The effectiveness of the immune system to cope with these challenges relies on adequate nutrient intake, including energy, protein, minerals and vitamins, hence low intake can suppress immune response. In conjunction, purported anti-inflammatory, and anti-oxidant properties of phytogenic compounds can bolster the immune system and enhance gut integrity as discussed in the following section.
No acidosis or other clinical illnesses were reported in either group during the adaptation diet period, however bulls in the PHY group did have higher DMI, which may indicate animals were coping better with the increasing starch levels. Changes in diet starch level during adaptation are characterized by an increase in total volatile fatty acid production, which affect the instances and severity of sub-acute acidosis bouts as well as contributing to greater metabolic oxidative pressure, resulting in lower DMI and performance (Schwartzkopf-Genswein and Moya, 2015; Wiese et al., 2017). Although the 40 g/d difference in ADG is not substantial, early gains achieved from smoother transition diets are noted to impact overall finishing performance (Brown et al., 2006). Physiological effects of the PFA to stimulate greater intake during this challenging period of adaptation to a high starch diet, in spite of known negative feedback mechanisms of propionate on intake in relation to hepatic oxidation, are discussed in more detail below.
Early and Late Finishing Period
Following adaptation period, the finishing period was divided into early and late stages to investigate intake and performance parameters. Intake patterns indicating a tendency (P = 0.06) in early finishing and greater intake (P = 0.02) in the latter stage of the PHY group suggests early performance gains during adaptation were carried forward. Moreover, the consistency in performance suggests that the effects of PHY treatment on intake continued until the end to the trial. In contrast, a review by Simitzis (2017) found that PFA often do not influence DMI or ADG in ruminants. As during adaptation, the particle sorting index was not indicative of a feeding behavior effect that could account for differences in DMI. However, observations taken at the mid-point of the finishing period indicated those in the PHY group spend more time at the feed bunk (P = 0.04), however, 14% difference in intake per visit was not significant (P > 0.1). In connection, PHY bulls were heavier by the mid-point (P = 0.05), however, differences in feed efficiency or ADG were not sufficient to be significant in either finishing period owing to limited group size and variance among individuals.
Overall Performance and Carcass Characteristics
By the end of the feeding period, final weights of the Control group match closely with the Brazilian industry averages of 526.5 kg live weight after 96.4 days on feed, averaging 2.3% body weight intake (Pinto and Millen, 2018). Whereas, by the end of the trial, bulls of the PHY group tended to be heavier than Control (P = 0.09), principally in part to maintaining a 0.1% greater DMI as % body weight throughout the trial (P = 0.02). As such, with dressing percentage of Control and PHY groups similar, carcass yield was 15 kg greater for the PHY group (P < 0.03).
Feeding conditions typical of a feedlot setting are prone to induce prolonged durations of low rumen pH, lending to increased prevalence of ruminitis and liver abscesses (Amachawadi and Nagaraja, 2016). Evaluation of welfare aspects indicated that ruminitis were low and comparable between present groups and concur with reported prevalence of 20–30% under commercial conditions (Malafaia et al., 2016), in spite of the PHY group consuming nearly 1 kg/d DM more feed throughout the trial. Likewise, liver abscess scores were low and similar between treatments in this trial, taking small group size into account, being comparable to the low prevalence (2.54%) at slaughter in a large Brazilian slaughter survey (Vechiato et al., 2011). Low instances of liver abscesses in Brazilian feedlot systems has been attributed to shorter time on feed (94 d in this trial), compared to up to 200 d, with a 10–20% liver abscess rate typical of US feedlot systems (Vechiato et al., 2011; Reinhardt and Hubbert, 2015; Samuelson et al., 2016; Pinto and Millen, 2018). Given the greater daily intake, representing more starch intake (>450 g/d over the Control), suggests greater capacity of the PHY group to cope with corresponding fermentations effects on gut integrity and immunity, without negatively impacting animal welfare.
From the slaughter data, measurements of ribeye area indicated no influence of treatment other than what could be accounted for by the difference in hot carcass weight. Although not evaluated in this trial, supplementation of essential oils during the finishing phase have consistently improved meat shelf-life (Castillo et al., 2013; Mucha and Witkowska, 2021), as well as consumer preference (Guerrero et al., 2018; Ornaghi et al., 2020).
Influence of Phytogenic Feed Additive on Metabolism
Given that phytogenic feed additives are comprised of common aromatic plant sources, their application in animal production are often erroneously overlooked as simple flavoring agents. Purported bioactivities of phytogenic compounds are largely considered to be a product of their chemical structure and interaction with receptors (Shaaban and El-Ghorab, 2012; Simitzis, 2017). As such, efficacy of a given plant can also be affected as levels of volatile and non-volatile phytochemical compounds can vary by source material, whether leaves, root, bark or peels, as well as geographic origin, season and stage of maturity (Chrysargyris et al., 2020). The PFA used in this trial contained a blend of herbs, spices and essential oils that include licorice, caraway, cinnamon, and vanilla. Physiological effects of a complex PFA, as in this trial, cannot be attributed to a single ingredient given the potential for additive or synergistic effects, however, many positive characteristics have been attributed to these ingredients. Licorice has been demonstrated to enhance tight-junction proteins, thereby improving gut integrity when exposed to inflammatory stress (Bachinger et al., 2019). Cinnamaldehyde, the main essential oil of cinnamon, applied at a range between 0.4 and 1.6 g/d, has been noted to have dose dependent effects on DMI and rumen organic matter digestibility (Yang et al., 2010). Furthermore, as a traditional medicinal plant, caraway has long been used for relief from various gastro-intestinal and respiratory related problems through spasmolytic, expectorant, digestive and anti-rheumatic effects (Al-Essa et al., 2010). In dairy application, feeding caraway seed pulp up to 10% DMI had positive influences toward milk and milk fat yield, as well as DMI, through possible benefits on rumen motility and pH stability (Moheghi et al., 2010). In lambs, crushed caraway fed at 30 ppm DM promoted feed intake and weight gain, while lowering markers for oxidative stress (Kaki et al., 2018). Essential oils of vanilla are more commonly associated with palatability enhancement, whereby inclusion at 0.2% stimulated earlier DMI, which contributed to rumen development and diet adaption, enabling calves to maintain an ADG advantage post diet transition (Fathi et al., 2009). Furthermore, Brand et al. (2019), feeding a blended PFA of similar composition as this trial at 0.5 and 1.0 g/d, reported a reduction in liver abscesses and variable as well as positive, influences on DMI and ADG of feedlot steers. However, Meyer et al. (2009) reported neither blends containing either thymol, eugenol, and vanilla or linalool, α-pinene and guaiacol fed at 1 g/d influenced DMI, ADG or gain: feed ratio, illustrating the complexity in understanding the efficacy of essential oils and the necessity to evaluate each on individual merit.
Ultimately, producers strive for greater intakes as this will impact weight gain and final weights. The mechanisms governing intake, and the potential of the essential oil blend to override these inhibitory signals, has commercial application both toward improving step-up diet adaptation and reduced total days on feed. In animals fed high concentrate diets, Allen (2020) focuses on the oxidation of fuels in the liver having a central role in regulating feed intake patterns. Propionate, readily produced via fermentation of starch, is considered to be the main volatile fatty acid governing intake via negative feedback mechanisms as part of the hepatic oxidation theory (Allen et al., 2009). From feedlot perspective, high propionate levels induced by typical diets likely exceed the liver's gluconeogenic capacity, leading to oxidation of propionate, producing abundant ATP, which has a negative feedback on intake levels (Allen et al., 2009). Drawing parallels to observed higher DMI in this trial, in the face of presumed high propionate levels, this would be counter intuitive, suggesting an interruption or compensation of this mechanism with the supplementation of the PHY additive. Extrapolating from the observations of Allen et al. (2009), whereby animals fed PFA maintained greater intake and could cope with higher fermentable starch diets, and ergo increased in pressure from oxidation of propionate, it is likely that antioxidative and anti-inflammatory attributes of the combined phytogenic compounds help mitigate the metabolic stress challenge. Further research is needed to understand the mechanisms governing intake of both receiver and acclimatized feedlot animals.
Alternatively, enhanced absorption or clearance of elevated ruminal volatile fatty acid concentrations, typical of starch fermentation, may contribute to slow changes in rumen pH that otherwise would contribute to rumen dysbiosis, acidosis, inflammation, and inhibition of intake. Absorption of volatile fatty acids via rumen wall are thought to be governed by blood flow, with kinetics favoring in the order of acetate < propionate < butyrate (Storm et al., 2012). As the concentration gradient shifts, rumen motility contributes to enhance volatile fatty acids absorption via mixing of contents and replenishing the rumen: intraepithelial interface (Allen et al., 2009). This may modulate the influx of fuels to the liver, reducing the formation of ATP and associated intake inhibition signal (Allen, 2020). Enhanced rumen motility and mitigation of pH decline under high-grain feeding conditions by a blended PFA was reported by Kröger et al. (2017), however, the study did not indicate if contractions led to greater rumen epithelium blood flow. The peristaltic effects of caraway as part of the current PFA may simulate rumen contractions, to facilitate VFA absorbance and mitigate pH induced fluctuations. Furthermore, high starch diets lead to a shift toward amylolytic microbiota can also increase circulating lipopolysaccharide endotoxin levels, triggering an acute phase protein response, e.g., haptoglobin and elevating inflammatory cytokines, e.g., TNF-α, Il-6 (Zhao et al., 2018). In traditional medicine, licorice has been associated with enhanced mucin release, which may serve a protective role against inflammatory bioamines associated with low rumen pH. Prolonged elevated levels of TNF-α can induce insulin-resistance or “desensitization of tissues” contributing to gluconeogenesis inhibition as demonstrated in steers (Kushibiki et al., 2001), which in turn would lead back to heightened ATP production from the oxidation of propionate and suppression of intake as outlined previously. The role of the present PFA toward enhanced DMI, whether via rumen motility and volatile fatty acid clearance or antioxidative and anti-inflammatory effects, remain to be elucidated. However, using the same PFA as the present study, Wichramasinghe et al. (2021), demonstrated greater DMI and reduced D-lactate, haptoglobin, and lipopolysaccharide binding protein expression during chronic heat stress conditions.
Conclusion
This research shows that a blended PFA that includes licorice, caraway, cinnamon and vanilla can enhance DMI and carcass weight of crossbred F1 Angus × Nellore bulls. The physiological mechanisms contributing to consistent higher DMI remain to be elucidated, however, the positive outcome demonstrates the potential for beef production free of pharmaceutical additives. A larger scale trial is warranted to confirm effects of this essential oil blend, with respects to economic implications of either reducing days on feed or greater yield of saleable meat.
Data Availability Statement
The raw data supporting the conclusions of this article will be made available by the authors, without undue reservation.
Ethics Statement
The animal study was reviewed and approved by the University of Maringá Animal Care and Use Committee (Protocol no. 6672311019).
Author Contributions
JD leads the project and prepared the statistical analyses. FP participated in samples collection, animal feeding during experiment, and conducted the laboratory analyses. All coauthors revised, provided inputs, and approved the final version of the manuscript.
Conflict of Interest
TT and IM are employed by BIOMIN Holding GmbH, a company that manufactures and trades feed additives. However, this circumstance did not influence the design of the study or bias the analysis and evaluation of the results.
The remaining authors declare that the research was conducted in the absence of any commercial or financial relationships that could be construed as a potential conflict of interest.
Publisher's Note
All claims expressed in this article are solely those of the authors and do not necessarily represent those of their affiliated organizations, or those of the publisher, the editors and the reviewers. Any product that may be evaluated in this article, or claim that may be made by its manufacturer, is not guaranteed or endorsed by the publisher.
Acknowledgments
We are grateful to all students of the GESF team and Iguatemi Experimental Farm crew from the State University of Maringá for their support with cattle care, sample collections, and analyses.
References
Abaker, J. A., Xu, T. L., Jin, D., Chang, G. J., Zhang, K., and Shen, X. Z. (2017). Lipopolysaccharide derived from the digestive tract provokes oxidative stress in the liver of dairy cows fed a high-grain diet. J. Dairy Sci. 100, 666–678. doi: 10.3168/jds.2016-10871
Al-Essa, M. K., Shafagoj, Y. A., Mohammed, F. I., and Afifi, F. U. (2010). Relaxant effect of ethanol extract of Carum carvi on dispersed intestinal smooth muscle cells of the guinea pig. Pharm. Biol. 48, 76–80. doi: 10.3109/13880200903046161
Allen, M., Bradford, B., and Oba, M. (2009). Board invited review: the hepatic oxidation theory of the control of feed intake and its application to ruminants. J. Anim. Sci. 87, 3317–3334. doi: 10.2527/jas.2009-1779
Allen, M. S. (2020). Review: control of feed intake by hepatic oxidation in ruminant animals: integration of homeostasis and homeorhesis. Animal 14, s55–s64. doi: 10.1017/S1751731119003215
Amachawadi, R. G., and Nagaraja, T. G. (2016). Liver abscesses in cattle: a review of incidence in Holsteins and of bacteriology and vaccine approaches to control in feedlot cattle. J. Anim. Sci. 94, 1620–1632. doi: 10.2527/jas.2015-0261
Aschenbach, J. R., Zebeli, Q., Patra, A. K., Greco, G., Amasheh, S., and Penner, G. B. (2019). Symposium review: the importance of the ruminal epithelial barrier for a healthy and productive cow. J. Dairy Sci. 102, 1866–1882. doi: 10.3168/jds.2018-15243
Bachinger, D., Mayer, E., Kaschubek, T., Schieder, C., König, J., and Teichmann, K. (2019). Influence of phytogenics on recovery of the barrier function of intestinal porcine epithelial cells after a calcium switch. J. Anim. Physiol. Anim. Nutr. 103, 210–220. doi: 10.1111/jpn.12997
Bento, M. H. L., Ouwehand, A., Tiihonen, K., Lahtinen, S., Nurminen, P., Saarinen, M., et al. (2013). Essential oils and their use in animal feeds for monogastric animals-effects on feed quality, gut microbiota, growth performance and food safety: a review. Vet. Med. 58, 449–458. doi: 10.17221/7029-VETMED
Bevans, D. W., Beauchemin, K. A., Schwartzkopf-Genswein, K. S., McKinnon, J. J., and McAllister, T. A. (2005). Effect of rapid or gradual grain adaptation on subacute acidosis and feed intake by feedlot cattle. J. Anim. Sci. 83, 1116–1132. doi: 10.2527/2005.8351116x
Bigham, M., and McManus, W. (1975). Whole wheat grain feeding of lambs. V.* Effects of roughage and wheat grain mixtures. Aust. J. Agric. Res. 26, 1053–1062. doi: 10.1071/AR9751053
Brand, T., Hünerberg, M., McAllister, T. A., He, M., Saleem, A. M., Shen, Y., et al. (2019). Impact of a phytogenic feed additive on growth performance, feed intake, and carcass traits of finishing steers. Transl. Anim. Sci. 3, 1162–1172. doi: 10.1093/tas/txz109
Brink, D. R., Lowry, S. R., Stock, R. A., and Parrott, J. C. (1990). Severity of liver abscesses and efficiency of feed utilization of feedlot cattle. J. Anim. Sci. 68, 1201–1207. doi: 10.2527/1990.6851201x
Brown, M. S., Ponce, C. H., and Pulikanti, R. (2006). Adaptation of beef cattle to high-concentrate diets: performance and ruminal metabolism1. J. Anim. Sci. 84, E25–E33. doi: 10.2527/2006.8413_supplE25x
Castillo, C., Pereira, V., Abuelo, A., and Hernández, J. (2013). Effect of supplementation with antioxidants on the quality of bovine milk and meat production. Sci. World J. 2013:616098. doi: 10.1155/2013/616098
Chrysargyris, A., Mikallou, M., Petropoulos, S., and Tzortzakis, N. (2020). Profiling of essential oils components and polyphenols for their antioxidant activity of medicinal and aromatic plants grown in different environmental conditions. Agronomy 10, 727–755. doi: 10.3390/agronomy10050727
Cooke, R. F. (2017). Invited paper: nutritional and management considerations for beef cattle experiencing stress-induced inflammation Prof. Anim. Sci. 33, 1–11. doi: 10.15232/pas.2016-01573
Drong, C., Buehler, S., Frahm, J., Hüther, L., Meyer, U., Soosten, D., et al. (2017). Effects of body condition, monensin, and essential oils on ruminal lipopolysaccharide concentration, inflammatory markers, and endoplasmatic reticulum stress of transition dairy cows. J. Dairy Sci. 100, 2751–2764. doi: 10.3168/jds.2016-11819
Duff, G. C., and Galyean, M. L. (2007). Board-invited review: recent advances in management of highly stressed, newly received feedlot cattle. J. Anim. Sci. 85, 823–840. doi: 10.2527/jas.2006-501
Fathi, M. H., Riasi, A., and Allahresani, A. (2009). The effect of vanilla flavored calf starter on performance of Holstein calves. J. Anim. Feed Sci. 18, 412–419. doi: 10.22358/jafs/66416/2009
Guerrero, A., Rivaroli, D. C., Sañudo, C., Campo, M. M., Valero, M. V., Jorge, A. M., et al. (2018). Consumer acceptability of beef from two sexes supplemented with essential oil mix. Anim. Prod. Sci. 58, 1700–1707. doi: 10.1071/AN15306
Huhtanen, P., Kaustell, K., and Jaakkola, S. (1994). The use of internal markers to predict total digestibility and duodenal flow of nutrients in cattle given six different diets. Anim. Feed Sci. Technol. 48, 211–227. doi: 10.1016/0377-8401(94)90173-2
Kaki, S., Moeini, M., Hozhabri, F., and Nikousefat, Z. (2018). The use of crushed caraway (Carum carvi) and black seed (Nigella sativa) additives on growth performance, antioxidant status, serum components and physiological responses of Sanjabi lambs. Iran. J. Appl. Anim. Sci. 8, 439–444.
Kröger, I., Humer, E., Neubauer, V., Reisinger, N., Aditya, S., and Zebeli, Q. (2017). Modulation of chewing behavior and reticular pH in nonlactating cows challenged with concentrate-rich diets supplemented with phytogenic compounds and autolyzed yeast. J. Dairy Sci. 100, 9702–9714. doi: 10.3168/jds.2017-12755
Kushibiki, S., Hodate, K., Shingu, H., Ueda, Y., Shinoda, M., Mori, Y., et al. (2001). Insulin resistance induced in dairy steers by tumor necrosis factor alpha is partially reversed by 2,4-thiazolidinedione. Domest. Anim. Endocrinol. 21, 25–37. doi: 10.1016/S0739-7240(01)00102-3
Leonardi, C., and Armentano, L. E. (2003). Effect of quantity, quality, and length of alfalfa hay on selective consumption by dairy cows. J. Dairy Sci. 86, 557–564. doi: 10.3168/jds.S0022-0302(03)73634-0
Lippolis, K., Cooke, R., Schumaher, T., Brandao, A., Silva, L., Schubach, K., et al. (2017). Physiologic, health, and performance responses of beef steers supplemented with an immunomodulatory feed ingredient during feedlot receiving. J. Anim. Sci. 95, 4945–4957. doi: 10.2527/jas2017.1837
Maekawa, M., Beauchemin, K. A., and Christensen, D. A. (2002). Effect of concentrate level and feeding management on chewing activities, saliva production, and ruminal pH of lactating dairy cows. J. Dairy Sci. 85, 1165–1175. doi: 10.3168/jds.S0022-0302(02)74179-9
Malafaia, P., Tiago, A., Costa, R., Souza, V., Costa, D., Costa, A., et al. (2016). Major health problems and their economic impact on beef cattle under two different feedlot systems in Brazil. Pesq. Vet. Bras. 36:837. doi: 10.1590/s0100-736x2016000900008
Meyer, N. F., Erickson, G. E., Klopfenstein, T. J., Greenquist, M. A., Luebbe, M. K., Williams, P., et al. (2009). Effect of essential oils, tylosin, and monensin on finishing steer performance, carcass characteristics, liver abscesses, ruminal fermentation, and digestibility. J. Anim. Sci. 87, 2346–2354. doi: 10.2527/jas.2008-1493
Michels, A., Neumann, M., Mattos Leão, G. F., Reck, A. M., Bertagnon, H. G., Lopes, L. S., et al. (2018). Isoquinoline alkaloids supplementation on performance and carcass traits of feedlot bulls. Asian-Australas. J. Anim. Sci. 31, 1474–1480. doi: 10.5713/ajas.17.0868
Moheghi, M., Tahmasbi, A., Naserian, A., and Omid, A. (2010). Assessment of nutritional value of caraway-seed pulp for feeding holstein dairy cattle. J. Anim. Vet. Adv. 9, 306–310. doi: 10.3923/javaa.2010.306.310
Mucha, W., and Witkowska, D. (2021). The applicability of essential oils in different stages of production of animal-based foods. Molecules 26, 3798–3818. doi: 10.3390/molecules26133798
Nazzaro, F., Fratianni, F., De Martino, L., Coppola, R., and De Feo, V. (2013). Effect of essential oils on pathogenic bacteria. Pharmaceuticals 6, 1451–1474. doi: 10.3390/ph6121451
Ornaghi, M. G., Guerrero, A., Vital, A. C. P., de Souza, K. A., Passetti, R. A. C., Mottin, C., et al. (2020). Improvements in the quality of meat from beef cattle fed natural additives. Meat Sci. 163:108059. doi: 10.1016/j.meatsci.2020.108059
Pinto, A., and Millen, D. (2018). Nutritional recommendations and management practices adopted by feedlot cattle nutritionists: the 2016 Brazilian survey. Can. J. Anim. Sci. 99, 392–407. doi: 10.1139/cjas-2018-0031
Reinhardt, C. D., and Hubbert, M. E. (2015). Control of liver abscesses in feedlot cattle: a review. Prof. Anim. Sci. 31, 101–108. doi: 10.15232/pas.2014-01364
Rivaroli, D., Do Prado, R., Ornaghi, M., Mottin, C., Ramos, T., Guerrero, A., et al. (2017). Essential oils in the diet of crossbred (½ Angus vs. ½ Nellore) bulls finished in feedlot on animal performance, feed efficiency and carcass characteristics. J. Agric. Sci. 9:205. doi: 10.5539/jas.v9n10p205
Runjaic-Antic, D., Pavkov, S., and Levic, J. (2010). Herbs in a sustainable animal nutrition. Biotechnol. Anim. Husbandry 26, 203–214. doi: 10.2298/BAH1004203R
Samuelson, K., Hubbert, M., Galyean, M., and Löest, C. A. (2016). Nutritional recommendations of feedlot consulting nutritionists: the 2015 New Mexico State and Texas Tech University survey. J. Anim. Sci. 94, 2648–2663. doi: 10.2527/jas.2016-0282
Schwartzkopf-Genswein, K., and Moya, D. (2015). “Relationship between feeding behaviour, ruminal pH, performance and welfare in feedlot cattle,” in 5th Brazilian Nutrition Conference, Dracena 8–9. p. 1–21.
Shaaban, H., and El-Ghorab, A. (2012). Bioactivity of essential oils and their volatile aroma components: review. J. Essent. Oil Res. 24, 203–212. doi: 10.1080/10412905.2012.659528
Simitzis, P. E. (2017). Enrichment of animal diets with essential oils—A great perspective on improving animal performance and quality characteristics of the derived products. Medicines 4:35. doi: 10.3390/medicines4020035
Soltan, Y. A., Natel, A. S., Araujo, R. C., Morsy, A. S., and Abdalla, A. L. (2018). Progressive adaptation of sheep to a microencapsulated blend of essential oils: ruminal fermentation, methane emission, nutrient digestibility, and microbial protein synthesis. Anim. Feed Sci. Technol. 237, 8–18. doi: 10.1016/j.anifeedsci.2018.01.004
Souza, K. A., Monteschio, J. D, Mottin, C., Ramos, T. R., Pinto, L. A. M., Eiras, C. E., et al. (2019). Effects of diet supplementation with clove and rosemary essential oils and protected oils (eugenol, thymol and vanillin) on animal performance, carcass characteristics, digestibility, and ingestive behavior activities for Nellore heifers finished in feedlot. Livest. Sci. 220, 190–195. doi: 10.1016/j.livsci.2018.12.026
Storm, A. C., Kristensen, N. B., and Hanigan, M. D. (2012). A model of ruminal volatile fatty acid absorption kinetics and rumen epithelial blood flow in lactating Holstein cows. J. Dairy Sci. 95, 2919–2934. doi: 10.3168/jds.2011-4239
Valenzuela-Grijalva, N. V., Pinelli-Saavedra, A., Muhlia-Almazan, A., Domínguez-Díaz, D., and González-Ríos, H. (2017). Dietary inclusion effects of phytochemicals as growth promoters in animal production. J. Anim. Sci. Technol. 59:8. doi: 10.1186/s40781-017-0133-9
Valero, M. V., Prado, R. M., Zawadzki, F., Eiras, C. E., Madrona, G. S., Prado, I. N., et al. (2014). Propolis and essential oils additives in the diets improved animal performance and feed efficiency of bulls finished in feedlot. Acta Sci. Ani. Sci. 36, 419–426. doi: 10.4025/actascianimsci.v36i4.23856
Vechiato, T. F., Maschio, W., Bom, L. C., Lopes, P. D., and Ortolani, E. L. (2011). Retrospective study of liver abscesses in beef cattle slaughtered in a Brazilian abattoir. Braz. J. Vet. Res. Anim. Sci. 48, 384–391. doi: 10.11606/S1413-95962011000500005
Wichramasinghe, H. K., Stepanchenko, N., Oconitrillo, M. J., Silva, J. V. V., Goetz, B. M., Abeyta, M. A., et al. (2021). “Phytogenics as a Potential Strategy to Mitigate the Negative Effects of Heat Stress” in American Society of Animal Science, Luisville, KY.
Wiese, B. I., Hendrick, S., Campbell, J. G., McKinnon, J. J., Beauchemin, K. A., McAllister, T. A., et al. (2017). Defining risk for low reticuloruminal pH during the diet transition period in a commercial feedlot in western Canada. J. Anim. Sci. 95, 420–435. doi: 10.2527/jas2016.0969
Williams, A. R., Andersen-Civil, A. I. S., Zhu, L., and Blanchard, A. (2020). Dietary phytonutrients and animal health: regulation of immune function during gastrointestinal infections. J. Anim. Sci. 98, 1–11. doi: 10.1093/jas/skaa030
Yang, W. Z., Ametaj, B. N., Benchaar, C., and Beauchemin, K. A. (2010). Dose response to cinnamaldehyde supplementation in growing beef heifers: ruminal and intestinal digestion1. J. Anim. Sci. 88, 680–688. doi: 10.2527/jas.2008-1652
Keywords: beef cattle, carcass, essential oils, feed intake, growth performance, plant extracts
Citation: Piran Filho FA, Turner TD, Mueller I and Daniel JLP (2021) Influence of Phytogenic Feed Additive on Performance of Feedlot Cattle. Front. Anim. Sci. 2:767034. doi: 10.3389/fanim.2021.767034
Received: 30 August 2021; Accepted: 27 September 2021;
Published: 22 October 2021.
Edited by:
Ana Guerrero Barrado, Universidad CEU Cardenal Herrera, SpainReviewed by:
Rodrigo Augusto Cortez Passetti, Rehagro, BrazilRodrigo De Nazaré Santos Torres, São Paulo State University, Brazil
Copyright © 2021 Piran Filho, Turner, Mueller and Daniel. This is an open-access article distributed under the terms of the Creative Commons Attribution License (CC BY). The use, distribution or reproduction in other forums is permitted, provided the original author(s) and the copyright owner(s) are credited and that the original publication in this journal is cited, in accordance with accepted academic practice. No use, distribution or reproduction is permitted which does not comply with these terms.
*Correspondence: Joao Luiz Pratti Daniel, jlpdaniel@uem.br