- 1Department of Clinical Sciences, Swedish University of Agricultural Sciences, Uppsala, Sweden
- 2Department of Animal Environment and Health, Swedish University of Agricultural Sciences, Uppsala, Sweden
- 3AgroSup Dijon, Dijon, France
Using levels of the stress hormone cortisol as an indicator for welfare is a common, but debated practice. In this observational study, hair cortisol concentration (HCC) of samples from 196 dairy calves from 7 to 302 days of age collected from 12 Swedish farms was determined using a commercially available ELISA. An assessment of animal welfare, assessed using animal-based indicators, was performed on the day of sampling. First, methodological factors with the potential to impact HCC and the effect of age were analyzed using generalized additive models. This revealed a significant peak in hair cortisol in young calves (around 50 days of age) and an association between fecal contamination of hair samples and the level of cortisol extracted. Second, associations between welfare indicators and HCC were explored using cluster analysis and regularized regression. The results show a complex pattern, possibly related to different coping styles of the calves, and indicators of poor welfare were associated with both increased and decreased hair cortisol levels. High cortisol levels were associated with potential indicators of competition, while low cortisol levels were associated with the signs of poor health or a poor environment. When running the regularized regression analysis without the contaminated hair samples and with the contaminated samples (including a contamination score), the results did not change, indicating that it may be possible to use a contamination score to correct for contamination.
Introduction
Determining the activity of the hypothalamic–pituitary–adrenocortical (HPA) axis by measuring cortisol has been used as a potential indicator of stress and welfare for many years, although the use is complicated by the complexity of the system (Mormède et al., 2007). Persistent increases in cortisol levels, indicating chronic stress, have been suggested to be the most harmful for the health and well-being of any animal, although chronic stress can also be associated with decreased cortisol levels due to adrenal exhaustion. Yet, the most common methods for measuring cortisol, such as using blood and saliva, are mainly suitable for studying acute changes during a limited time (Otovic and Hutchinson, 2015). In addition, these methods are sensitive to stress associated with sampling, and there is a natural daily variation and also adaptation to recurrent stressors, all of which may affect the results (Ladewig and Smidt, 1989; Knights and Smith, 2007). Measuring cortisol metabolites in feces or urine enable retrospective measures, unaffected by the sampling procedure, but are influenced by passage rate and require the collection of all feces or urine excreted from the animal during the time period of interest, which causes practical difficulties (Möstl and Palme, 2002). Hair cortisol, on the other hand, is a non-invasive technique used for studying retrospective cortisol levels (Lee et al., 2015; Roth et al., 2016; Burnard et al., 2017). As cortisol is integrated with the hair during its growth, this method gives a picture of the total amount of circulating cortisol over time and the sampling is not subjected to the problems of circadian variations or the interference of momentary stress during sampling (Lee et al., 2015). Previous longitudinal studies have confirmed the relationship among hair cortisol concentration (HCC), serum levels, and fecal levels of cortisol in cattle (González-de-la-Vara et al., 2011; Tallo-Parra et al., 2015).
However, there are factors that can impact the analysis of HCC. For example, the washing and extraction protocol, sampling site and type of hair, season, age, and fecal contamination can impact HCC (Davenport et al., 2006; Moya et al., 2013; Burnett et al., 2014; Meyer et al., 2014; Tallo-Parra et al., 2015; Heimbürge et al., 2020a; Otten et al., 2020). In experimental studies, it is possible to control for these aspects within the study design but in observational studies, some factors can pose a challenge and require control at the analysis stage. Such an example is urine or fecal contamination which may be unavoidable when maintaining a consistent hair sampling site. Furthermore, even if there is an effect of age, in newborn and younger calves (6 weeks of age) that have been shown to have higher HCC compared with older animals (24 weeks and 6 months of age) (Heimbürge et al., 2020b; Hayashi et al., 2021), the pattern of decrease over time between these ages remains to be investigated.
The use of cortisol as a welfare indicator also has important limitations that have been pointed out in several publications (Rushen, 1991; Korte et al., 2007; Otovic and Hutchinson, 2015). While stress and animal welfare are closely linked, high stress does not automatically cause poor welfare and low stress does not necessarily define good welfare (Botreau et al., 2007). In addition, the concept of welfare stretches beyond the pure presence or absence of stress and includes the feelings experienced by the animal (Duncan, 2005). Thus, the relationship between HCC and welfare may depend on which welfare indicator is being measured. Previous studies on cattle have shown increased levels of hair cortisol in clinically compromised cows and cows subjected to heat stress (Burnett et al., 2015; Ghassemi Nejad et al., 2017). However, only weak associations between the results of welfare assessments and HCC in dairy cows have been observed at the herd level (van Eerdenburg et al., 2021).
In this observational study, the associations among HCC, age, and various animal-based indicators of welfare in young dairy calves are explored to assess the usefulness of hair cortisol as an indicator of welfare. We also investigate the effect of potential confounders and discuss the possibilities and limitations of controlling for the effect of fecal contamination.
Materials and Methods
The cross-sectional study included 196 calves (1–43 weeks of age) from 42 pens on 12 Swedish dairy farms. These farms participated in another ongoing research project and were selected due to the presence of verotoxin-producing Escherichia coli (VTEC). A detailed description of the selection of animals for sampling can be found in Tamminen et al. (2020). In short, calves in pens, where VTEC was present, were selected for sampling using a systematic randomization process. The sampled calves were housed indoors in groups under typical Swedish conditions, most commonly on straw bedding but there were examples of calves on sawdust and slatted floors. Ethical approval for the study was granted by the regional ethical committee (Uppsala Djurförsöksetiska Nämnd, Dnr: C 85/15).
Animal-Based Assessment
The farm visit was planned so that the undisturbed animals could be observed for 20 min around morning feeding time as this is a time point of high activity (Bokkers and Koene, 2001). If multiple pens were included on a farm, the observer rotated between the pens every five min until each pen had been observed for a total of 20 min. Two out of 42 pens contained more than 20 animals and were too large to observe the whole pen from one location. In these cases, the pen was observed for 2 × 20 min from two opposite locations to account for the possibility of missing behaviors performed by animals standing far away from the assessor. The protocol, which consisted of clinical scoring, undisturbed behavioral observations, and a behavioral test, was developed based on previous publications on the assessment of calf welfare (Lidfors, 1993; Jensen and Kyhn, 2000; Bokkers and Koene, 2001; Whay et al., 2003; Windschnurer et al., 2008; Bokkers et al., 2009; Leach et al., 2009; Welfare Quality®, 2009). The full protocol is presented in Table 1. The measures consisted of a combination of long-term indicators, such as body condition score and coat condition, and measures reflect a shorter time period, such as ruminal fill. When applicable for the behavior being observed, such as mounting and licking, both performer and receiver were noted. After the undisturbed behavioral observations, fear of unknown humans was tested using a distance avoidance test. Finally, calves were restrained and individually assessed e.g., for nasal discharge, tear staining, and sampled for VTEC and hair. At this time, the reactivity of the calf to restraint was also scored. All animal-based assessments were carried out by the first author.
Analysis of HCC
Hair samples were collected from the tail of each calf (just above the tail switch) with scissors. Hair was cut as close to the skin as possible. Samples were wrapped in aluminum foil and stored dark at room temperature for 3–7 months before analysis. From 318 sampled animals, 196 samples including both individuals colonized by VTEC O157:H7 and randomly selected negative controls (1–3 controls per colonized individual) were selected for analysis of hair cortisol. The protocol for preparing and analyzing hair was based on previous studies on cattle and other species (Meyer et al., 2014; Tallo-Parra et al., 2015; Roth et al., 2016) but adapted and modified by authors. Prior to analysis, hair from the centimeter closest to the cut end of the hair (closest to the skin) was cut with scissors and placed in a 15-ml tube. Samples were washed by adding 4 ml of isopropanol, and samples were vortexed for 4 min before the isopropanol was decanted. The procedure was repeated three times. Samples were left to dry at room temperature under a fume hood for 2-3 days until all the isopropanol had evaporated. For samples where fecal contamination remained after the washing procedure, the dirtiness of the hair was scored from 1 to 4 (1= no dirt, shiny, 2 = <5 dirt particles, shiny, 3 = > 5 dirt particles, shiny, 4 = > 5 particles of dirt, dry, or dull). Approximately 50 mg of each hair sample was added to a 2-ml microtube with a screwcap. If less than 50 mg of washed hair was available, as much as possible was used. Exact duplicates were prepared for 30 samples (12%). In addition, a duplicate sample with a different degree of remaining dirt or of a different color was prepared from 21 of the individual samples. Samples were prepared farm by farm in random order. For exact duplicates, every 10th sample was selected during preparation (if the sample did not have enough hair, the 11th sample was taken). The 21 samples of different colors and dirtiness were selected based on availability during sample preparation.
Three chrome steel balls (3.2 mm in diameter, BioSpec Products, Cat. No. 11079132c) per tube were added, and the tubes were frozen in liquid nitrogen for 2 min before grinding the hair for 3 × 60 s at 6,500 RPM in a bead beater (Precellys Evolution). After grinding, 1.2 ml of methanol was added and samples placed on a rocker. After 18 h, the samples were centrifuged. The first 41 samples were centrifuged for 2 min at 7,000 g, and 0.6 ml of methanol was transferred to new 1.5-ml tubes. As the first samples had occasional hair particles in the final product, the centrifugation protocol was changed and the remaining samples were centrifuged two times After the initial centrifugation, 0.8 ml of methanol was transferred to new 1.5-ml tubes, and the samples were recentrifuged at 10,000 g for 5 min, and 0.6 ml of methanol was transferred to new 1.5-ml tubes. Methanol was evaporated at 38°C under a fume hood after the centrifugation. Phosphate-buffered saline (0.01 M PBS, pH 7.4) was added to the dried samples. The first 92 samples were dissolved in 200 μl PBS, the last 159 samples in 150 μl as the dried sample appeared to dissolve better in this volume. The concentration of cortisol was determined using an ELISA kit designed for salivary cortisol (Salimetrics Europe Ltd, Art 1-3002) according to the manufacturer's instructions (validated by Moya et al., 2013). Each sample was analyzed in duplicate, and high and low controls were run on each assay. Inter- and intraassay coefficients of variation (CV) values were calculated according to the instructions from the manufacturer (Salimetrics, 2018). Final cortisol content (pg/mg) in hair was calculated using the formula presented by Meyer et al. (2014).
Data Management and Statistical Methods
Data were entered in Excel (Microsoft Corp., Redmond, WA) and exported to R Statistical Software (R Core Team, 2018) for statistical analysis. Hair cortisol was log10-transformed to achieve normal distribution before calculation of Pearson's correlation coefficient for exact duplicates and duplicates of different dirtiness or colors. Plots of the correlation were generated using the package ggplot (Wickham, 2016). The impact of remaining dirt, age, hair color, month, and methodological changes during the process was analyzed using a generalized additive model (GAM) with a log link function in the package gamm4 (Wood, 2004; Wood and Scheipl, 2017). In this analysis, one sample from each pair of exact duplicates was excluded from the analysis, but all other remaining samples were included. A random effect of pen nested within-herd was included to account for the clustering of the observations. The model was reduced based on the change in R-squared after the removal of one variable at a time, and the final model was evaluated by residual plots.
To explore and visualize the observations of the calves and HCC, a cluster analysis of mixed data was performed using the CluMix package in which distance between individual calves was based on Gower's general similarity coefficient and variables clustered based on similarity using measures of association (Hummel et al., 2017, 2019).
The associations between hair cortisol (log10-transformed) and welfare indicators were also analyzed using regularized regression using package glmnet (Friedman et al., 2010). For animals with multiple samples, only one sample (with the lowest CV value) was included. Associations between welfare parameters and HCC were first explored excluding the samples with fecal contamination (n = 139) and second with all samples, but then with the dirtiness score included in the analysis (n = 196). There were small amounts of missing data in the welfare indicators randomly scattered across observations. The proportion of missing data per indicator was low, for the majority of indicators <1%. The indicator with the highest proportion of missing values was cleanliness body (8 missing) and distance avoidance test (7 missing at random, 23 missing by design). These were followed by cleanliness upper hind legs (6), cleanliness below hocks and coat condition (5), and ruminal fill (4). Another 10 variables were missing 1 observation. Missing values were assumed to be missing at random except for the distance avoidance test where 23 observations were missing due to the pens being too small or crowded for carrying out the test. These were treated as a separate category in the analysis. The remaining missing values were imputed using non-parametric random forest imputation in the package missForest before the elastic net regression (Stekhoven and Bühlmann, 2012; Stekhoven, 2013). Two variables (lameness and conjunctivitis) were excluded due to little variance between the calves, and qualitative indicators with several levels were reduced by grouping if some levels contained very few observations and grouping was biologically plausible (Supplementary Table S2). The counts of hairless patches and wounds or swellings were also condensed into categories (Supplementary Table S2). For many behavioral observations, a high number of calves did not perform the behavior leading to a distribution with the high frequency of zero observations. This was handled by adding a categorical variable describing if the behavior was observed and a quantitative variable with the number of times the behavior was observed (Robertson et al., 1994). The models were tuned and fitted using leave one out cross-validation using the package caret (Kuhn, 2020), and the models that generated the smallest mean squared errors were selected (James et al., 2013). The herd was included as a fixed effect to account for clustering.
Results
The cortisol levels in hair ranged from 1.57 to 138.22 pg/mg with a median of 9.19. For high control, the average CV was 9.1% and for the low control, it was 10% giving an interassay (average of high and low control) CV of 9.5% (n = 12). The mean intraassay CV was 4.3% (n = 12). These values are well within the recommendations of the manufacturer (15 and 10%, respectively). Correlation between the 30 exact duplicates was high (Pearson's correlation coefficient = 0.94) while the correlation between the 21 duplicates of different colors and dirtiness was lower (Pearson's correlation coefficient = 0.76). There was no association between HCC and colonization with VTEC O157:H7 (Tamminen, 2020).
Factors Influencing the Extraction of Cortisol
The GAM showed that the dirtiness of hair was associated with a significant increase in cortisol extracted and that the increase was larger for the dirtiest samples (Table 2). There was a significant peak in hair cortisol levels in calves around 50 days of age and a tendency for darker hair to be associated with higher HCC (Figure 1). Other parameters (storage time, number of centrifugations, methanol) did not influence the cortisol concentrations (Table 2). A preliminary analysis indicated that lower HCC was seen in the samples where 200 μl of PBS was used to dissolve the cortisol, which supported our suspicion that the dried samples did not dissolve completely. However, in a stratified analysis of samples from farms where both preparation volumes were applied, no difference was observed. It was, therefore, concluded that the effect observed was a result of farm differences and not the amount of PBS. Also, removing the PBS variable did not impact the explanatory abilities of the model, further supporting that the effect was related to farm variations. Thus, this variable was excluded from the final model.
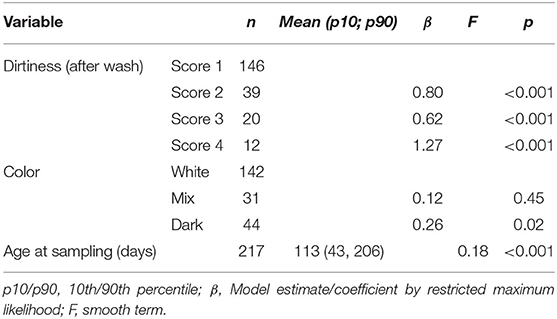
Table 2. Results of the final generalized additive mixed model on the effect of dirtiness, hair color, storage, and other laboratory-related factors on hair cortisol extracted from hair samples.
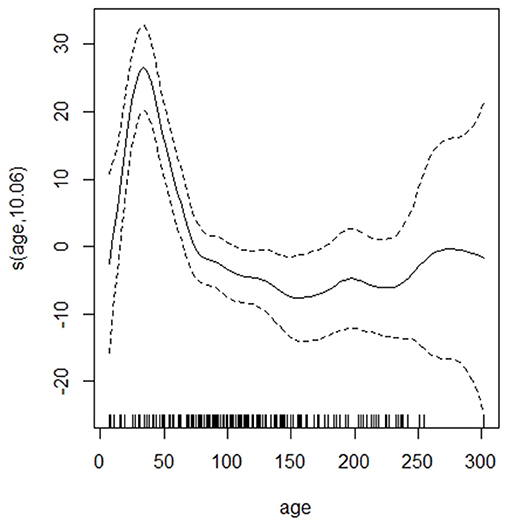
Figure 1. The association between age (in days) and hair cortisol content of the dairy calves. Dotted lines indicate 95% CIs.
Association Between HCC, Age, and Indicators of Welfare
Detailed results of the welfare and behavior assessments (including all samples regardless of contamination level) and a visualization of the cluster analysis can be found in the (Supplementary Tables S1, S2; Supplementary Figure S1). HCC clustered (as expected from the previous analysis) closely to the dirtiness score. Interestingly, hair cortisol also clustered closely to score in the distance avoidance test (which was in the same cluster as the dirtiness score) although the pattern of association between HCC and the score in the avoidance distance test was not distinguishable. Most notably, a group from Herd 1, where the distance avoidance test was missing by design (indicating a small and crowded pen), appeared to have higher HCC levels. In addition, HCC clustered close to age, herd, sex, being fed milk, and stretching (although the latter had relatively few observations). Young animals being fed milk formed a cluster that appeared to have higher HCC values compared with the older animals. Animals with poor body conditions (and poor coat conditions) also showed high similarity and clustered closely together. However, in this group, cortisol levels did not appear to increase but decreased. There was also a cluster where calves with a high frequency of coughing during the behavioral observations and with nasal discharge and coughing observed during the clinical observations appeared to have a slightly lower HCC. There was some clustering associated with the herd, but the degree of similarity within-herd differed between herds.
Regularized regression using clean samples and all samples yielded comparable models with similar R-squared (0.59 and 0.58) and mean squared error (0.42 and 0.46). In the model including all samples, the dirtiness score of hair (Scores 3 and 4) had the largest coefficients (Score 2 was also included but with a smaller coefficient). The coefficients of variables describing the association between HCC and the welfare indicators in the two models are presented in Figure 2. Variables with positive coefficients (i.e., associated with increased hair cortisol) in both models were a mixture of potential indicators for both poor and good welfare (Boissy et al., 2007; Botreau et al., 2007). Being fed milk had large coefficients in both models. The behaviors with the largest positive coefficients, i.e., affecting cortisol the most, present in both models were the qualitative and quantitative variables for being displaced and being rubbed on by others. That both the qualitative and the quantitative indicators for displacement were included in the final models which implies that the effect of being displaced on cortisol concentration increases the more calves are being displaced. Together with poor ruminal fill (model with all samples), these variables are potential indicators for competition and/or low dominance. Moderate tear staining was also included in both models. There were also variables with smaller positive coefficients not usually related to poor welfare (e.g., locomotor play, which is considered an indicator of good welfare, and grooming or comfort behaviors such as self-licking and rubbing). The coefficients of the variables associated with low cortisol levels (i.e., variables with negative coefficients) in both models were related to poor health (nasal discharge, coughing), dirtiness on the upper hind legs (indicating diarrhea), and high reactivity during sampling. Performing stereotypic behaviors, such as cross-sucking and tongue movements, also had small negative coefficients in both models.
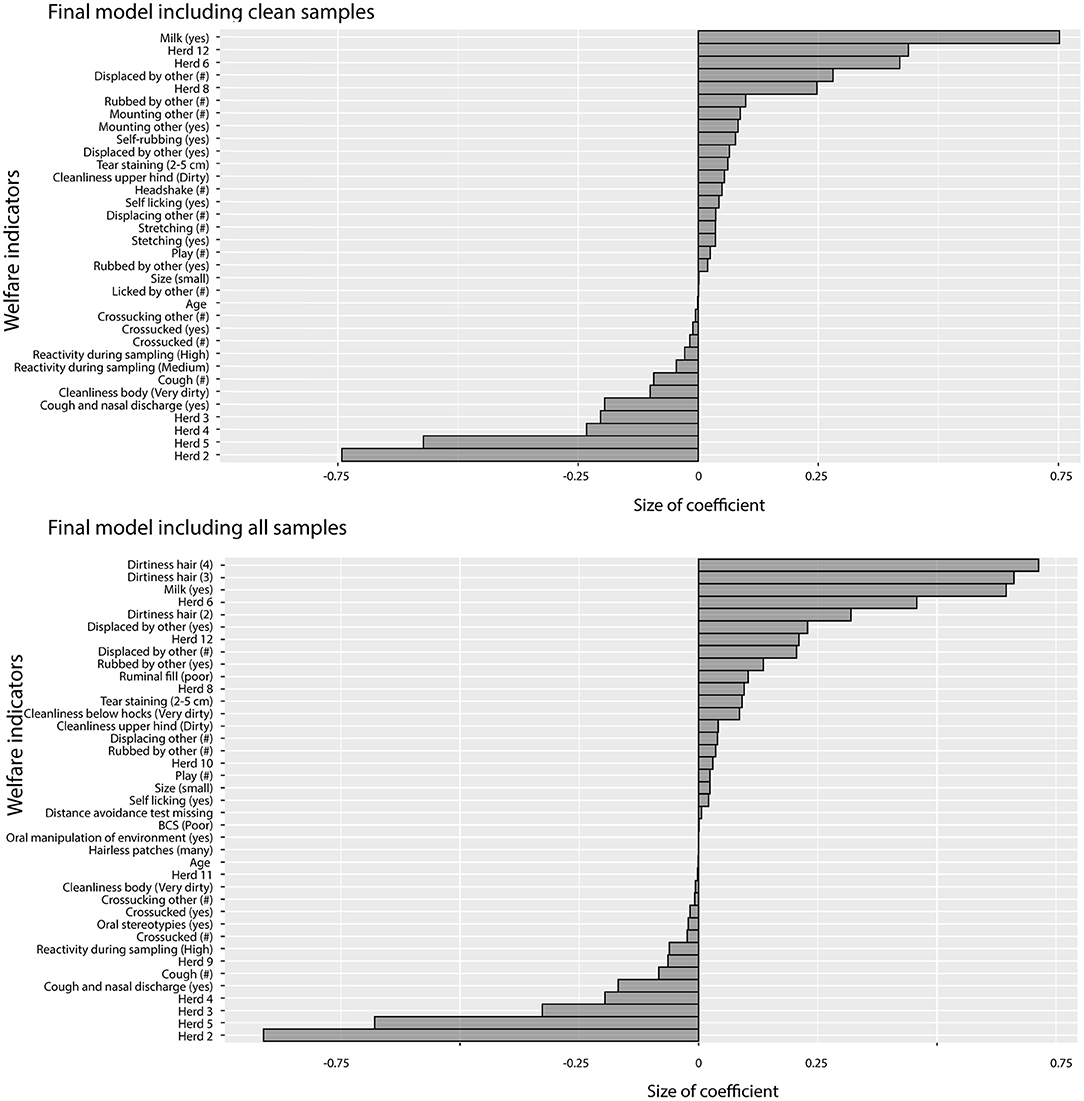
Figure 2. Coefficients from the regularized regression model that minimized root mean squared error. Positive coefficients were positively associated with HCC and negative coefficients were negatively associated with HCC. Behavioral variables marked with (#) are quantitative measures of the number of times the behavior was observed as opposed to binary variables indicating whether a behavior was observed (yes/no).
There were some differences between the two models. In the model including only clean samples, mounting behaviors had relatively large coefficients while poor ruminal fill and dirtiness below hocks had relatively large coefficients in the model with all samples. For the negative coefficients, poor cleanliness of the body had a larger negative coefficient in the model with only clean samples compared with the model with all samples. These differences are likely associated with correlations between variables (leading to different variables being picked in the different models) and in some cases (such as for mounting) a result of few observations.
Discussion
The results of this observational study display the complexity of the HPA axis and how factors related to contamination of samples, environment, and individual differences are associated with HCC. Many of these are closely interconnected and need to be considered and interpreted in association with each other.
Methodological Considerations Regarding the Extraction and Analysis of Hair Cortisol
The mean cortisol content in the samples was 15.4 pg/mg, which is in the higher range of what previous studies using an ELISA from the same manufacturer have reported (Moya et al., 2013; Burnett et al., 2014, 2015; Ghassemi Nejad et al., 2017). However, considering the peak in hair cortisol values observed in young animals this is not surprising as these other studies included older animals. As visualized in Figure 1, a significant increase in hair cortisol levels of dairy calves with a peak around 50 days of age was observed in the GAM. In the regularized regression, the variable “Fed milk,” i.e., belonging to the age group where the peak was observed, was associated with increased hair cortisol levels. The effect of young age on HCC is consistent with the increased HCC in 6-week-old calves compared with 24-week-old calves observed by Wood and Scheipl (2017).
Calves were weaned around 2 months of age on the farms included in this study, and the observed peak may be related to increased stress due to reduced milk allowance before weaning. There are also additional changes in the life of a young calf occurring during the first 2 months. For example, most of the farms kept animals in single pens for about 2 weeks before they were group housed. This transition, including social challenges and increased activity, may have influenced hair cortisol levels. Calves are also disbudded during this time period and although this is done with local analgesics in Sweden, the procedure may induce stress and pain when the analgesics wear off. Experimental studies where hair is clipped and the newly grown hair regularly sampled should provide additional information about the role of different challenges in a young calf's life.
Correlation between duplicates was high for the exact duplicates while duplicates with different levels of contamination and color showed more variations. Considering this, in combination with the results of the GAM, emphasizes that fecal contamination had a large influence on HCC and the association is supported by a dose-response curve, i.e., increasing levels of contamination are associated with a larger effect (Hill, 1965). As the risk of dirtiness on the tail is high, we suggest avoiding the use of hair from this location when studying dairy calves, contradictory to the recommendations in previous studies on cattle (Moya et al., 2013; Burnett et al., 2014; Vesel et al., 2020). Dark hair color was also associated with increased HCC while mixed samples did not differ from white hair samples. This could be a result of the relatively low number of samples with dark and mixed hair in this study, that it was more difficult to observe contamination in dark samples or be related to other unmeasured parameters (such as the thickness of hair).
Fecal contamination of the hair sample was negatively correlated with the cleanliness of the calf in the welfare assessment (i.e., the samples from clean calves were less likely to be contaminated), and the variables clustered relatively close in the cluster analysis. Due to this correlation, it is possible that some aspects of the high HCC observed in dirty samples are actually associated with calves living in a suboptimal, dirty environment. There is some support for this in cluster analysis where it appears that high HCC was generally associated with poor cleanliness, except among the younger individuals that stand out with very high cortisol levels and good cleanliness. This discrepancy between the group of young animals and others may explain the relatively large change in the coefficient for poor cleanliness of body when more samples were added in the regularized regression. With the larger sample size, the influence of young animals, and thereby the usefulness of the variable cleanliness of body, decreased. In addition, some variables with few observations, such as mounting others, also decreased in importance when additional samples were added. However, we note that the majority of the larger coefficients not related to cleanliness remained comparable between models. Thus, we argue that using a contamination score can be a way to handle samples exposed to contamination in the analysis, as long as the potential for confounding between environment and contamination is kept in mind.
Hair Cortisol as an Indicator for Welfare in Dairy Calves
It is important to note that in this study, welfare was only assessed at the one-time point and the behaviors observed during a relatively short time period. This means that calves are compared based on a snapshot during an active time period. Hair cortisol on the other hand represents an average of cortisol levels during the time of hair growth, and there are likely past events influencing hair cortisol levels that are unaccounted for in this study. Still, it is interesting that, despite the limited observation time, we find relatively short-term measures, such as poor ruminal fill and being displaced, related to increased hair cortisol levels. However, as the personality and feeding behavior of calves have been shown to be relatively constant over time (Lecorps et al., 2018; Neave et al., 2018), the short-term measures may still be related to retrospective cortisol levels. BCS is also a measure of feed intake, although more long-term, as opposed to ruminal fill, this variable was not associated with high HCC levels. In the cluster analysis, this measure clustered with another long-term measure, coat condition, and these individuals appeared to have low HCC. A possible explanation could be that this represents a group that has been exposed to stress for a longer time and exhibit a habituated cortisol response. Another possible explanation is that these long-term characteristics are associated with a poor, non-stimulating environment which causes hypostimulation (Korte et al., 2007). Longitudinal studies where confounders can be limited or controlled and HCC followed over time are needed to confirm these associations.
The usefulness of cortisol as an indicator of welfare lies in the potential to identify chronically stressed individuals struggling with coping. In this study, indicators of poor welfare were found among variables associated with high and low hair cortisol levels. The variables associated with the largest negative coefficients in the regularized regression were related to poor health while the largest positive coefficients appeared to be related to competition and dominance. However, some of the variables with smaller positive coefficients were not related to stress and poor welfare. That locomotor play was related to higher cortisol levels and may be related to activity influencing cortisol, for example, human endurance athletes have higher HCC compared with controls (Skoluda et al., 2012) or a result of the correlation between play and young age. However, the association between high reactivity and low cortisol was not anticipated as previous studies on cattle have not identified associations between HCC and temperament (Cooke et al., 2017; Lockwood et al., 2017). In many other species, fearfulness and reactivity have been associated with different coping styles and used to separate proactive and reactive individuals (Koolhaas et al., 1999). Proactive coping is characterized by a low HPA axis activity but a high sympathetic reactivity, whereas reactive coping is associated with a more active HPA axis activity and low sympathetic reactivity. The association between high reactivity and low cortisol, observed in our study, may be attributable to calves having different coping styles. The animals that were fearful and reacted violently to restraining may be the examples of proactive individuals (with a low HPA axis activity) exposed to an unfamiliar situation, whereas the reactive individuals (with high HPA axis activity) are generally more prone to handle new situations in a more passive manner (Koolhaas et al., 2007). This could explain why high cortisol levels were observed in dynamic calves interacting with other calves and the environment.
While high HCC was associated with indicators of poor and good welfare, low HCC was mainly associated with indicators related to poor welfare and reduced health. This is in agreement with a study that found lower hair cortisol levels in horses with clearly compromised welfare (Pawluski et al., 2017). It also supports the idea that the most severe forms of chronic stress may be associated with lower levels of cortisol, due to a decreased responsiveness to adrenocorticotropic hormone or habituation (Korte et al., 2007; Mormède et al., 2007). However, the association between low cortisol levels and indicators of poor clinical health (such as coughing and nasal discharge) contradicts that high cortisol levels can be useful to identify stress associated with clinical disease in calves, as previous studies on cows have suggested (Comin et al., 2013; Burnett et al., 2015). This discrepancy may also be a result of confounding due to age or environmental factors as mentioned above. A restrictive, non-stimulating environment can lead to a lower baseline of blood cortisol in healthy calves (Fisher et al., 1997). Such non-stimulating environments could be connected to environments where calves are more likely to develop coughing and other health-related problems (such as poor coat condition and BCS mentioned above). If so, low hair cortisol levels could be used as a herd level indicator of a suboptimal environment and/or poor management of calves where calves are also more likely to become sick. However, additional confirmation of these associations and increased understanding of how environment, personality, and coping styles influence hair cortisol levels are needed.
Conclusion
This study highlights the importance of fecal contamination of hair when analyzing HCC. To avoid contamination, the use of hair from the tail of dairy calves should be avoided. When contamination is present, the results suggest that contamination scores can be a way of addressing the problem in observational studies. The results also emphasize the complexity of the HPA axis in relation to different indicators of welfare and individual differences. While poor welfare can be associated with increased and decreased HCC, the results suggest that low HCC in calves could be a sign of long-term exposure to a suboptimal environment where calves are more likely to become sick. The results also point out the need to understand how different coping mechanisms affect HCC in dairy calves and propose new hypothesizes that should be explored in future studies.
Data Availability Statement
The datasets presented in this study can be found in online repositories. The names of the repository/repositories and accession number(s) can be found below: Mendeley Data, doi: 10.17632/bxzdpbx5rn.1.
Ethics Statement
The animal study was reviewed and approved by Uppsala Djurförsöksetiska Nämnd, Dnr: C 85/15. Written informed consent was obtained from the owners for the participation of their animals in this study.
Author Contributions
L-MT, LK, and UE: conceptualization. L-MT, LB, LK, and AS: methodology. L-MT and LB: investigation and curation. L-MT, AS, and LK: data validation. L-MT: formal analysis and investigation, writing—original draft preparation, visualization, and project administration. AS: resources. AS, LK, and UE: writing—review and editing. LK and UE: supervision. UE: funding acquisition. All authors have read and agreed to the published version of the manuscript.
Funding
This study was funded by the Swedish Research Council Formas (Grant 2014-61-28268-47).
Conflict of Interest
The authors declare that the research was conducted in the absence of any commercial or financial relationships that could be construed as a potential conflict of interest.
Publisher's Note
All claims expressed in this article are solely those of the authors and do not necessarily represent those of their affiliated organizations, or those of the publisher, the editors and the reviewers. Any product that may be evaluated in this article, or claim that may be made by its manufacturer, is not guaranteed or endorsed by the publisher.
Acknowledgments
The authors thank Yongzhi Guo (Department of Clinical Sciences, Swedish University of Agricultural Sciences) for technical assistance with running the analysis of hair cortisol. The authors also thank Per Jensen, Ann-Sofie Sundman, and Ann-Charlotte Svensson Holm (Department of Physics, Chemistry, and Biology, Linköping University) for graciously providing advice and helpful tips regarding the protocol for hair cortisol analysis. We additionally want to thank the dairy producers participating in this study, the students Linnea Andersson, Ellen Wik, and Claire-Lise Sol who assisted in collecting the samples, and the Swedish Research Council Formas for funding this project.
Supplementary Material
The Supplementary Material for this article can be found online at: https://www.frontiersin.org/articles/10.3389/fanim.2021.793558/full#supplementary-material
References
Boissy, A., Manteuffel, G., Jensen, M. B., Moe, R. O., Spruijt, B., Keeling, L. J., et al. (2007). Assessment of positive emotions in animals to improve their welfare. Physiol. Behav. 92, 375–397. doi: 10.1016/j.physbeh.2007.02.003
Bokkers, E. A. M., and Koene, P. (2001). Activity, oral behaviour and slaughter data as welfare indicators in veal calves: A comparison of three housing systems. Appl. Anim. Behav. Sci. 75, 1–15. doi: 10.1016/S0168-1591(01)00175-7
Bokkers, E. A. M., Leruste, H., Heutinck, L. F. M., Wolthuis-Fillerup, M., Van Der Werf, J. T. N., Lensink, B. J., et al. (2009). Inter-observer and test-retest reliability of on-farm behavioural observations in veal calves. Anim Welf. 18, 381–390.
Botreau, R., Veissier, I., Butterworth, A., Bracke, M. B. M., and Keeling, L. J. (2007). Definition of criteria for overall assessment of animal welfare. Anim. Welf. 16, 225–228. doi: 10.1016/S0008-6215(00)00097-5
Burnard, C., Ralph, C., Hynd, P., Edwards, J. H., and Tilbrook, A. (2017). Hair cortisol and its potential value as a physiological measure of stress response in human and non-human animals. Anim. Prod. Sci. 57, 401–414. doi: 10.1071/AN15622
Burnett, T. A., Madureira, A. M. L., Silper, B. F., Nadalin, A., Tahmasbi, A., Veira, D. M., et al. (2014). Short communication: factors affecting hair cortisol concentrations in lactating dairy cows. J. Dairy Sci. 97, 7685–7690. doi: 10.3168/jds.2014-8444
Burnett, T. A., Madureira, A. M. L., Silper, B. F., Tahmasbi, A., Nadalin, A., Veira, D. M., et al. (2015). Relationship of concentrations of cortisol in hair with health, biomarkers in blood, and reproductive status in dairy cows. J. Dairy Sci. 98, 4414–4426. doi: 10.3168/jds.2014-8871
Comin, A., Peric, T., Corazzin, M., Veronesi, M. C., Meloni, T., Zufferli, V., et al. (2013). Hair cortisol as a marker of hypothalamic-pituitary-adrenal axis activation in Friesian dairy cows clinically or physiologically compromised. Livest. Sci. 152, 36–41. doi: 10.1016/j.livsci.2012.11.021
Cooke, R. F., Schubach, K. M., Marques, R. S., Peres, R. F. G., Silva, L. G. T., Carvalho, R. S., et al. (2017). Effects of temperament on physiological, productive, and reproductive responses in Bos indicus beef cows. J. Anim. Sci. 95, 1–8. doi: 10.2527/JAS.2016.1098
Davenport, M. D., Tiefenbacher, S., Lutz, C. K., Novak, M. A., and Meyer, J. S. (2006). Analysis of endogenous cortisol concentrations in the hair of rhesus macaques. Gen. Comp. Endocrinol. 147, 255–261. doi: 10.1016/j.ygcen.2006.01.005
Duncan, I. J. H. (2005). Science-based assessment of animal welfare: farm animals. Rev. Sci. Tech. Off. Int. des Epizoot. 24, 483–492.
Fisher, A. D., Crowe, M. A., Prendiville, D. J., and Enright, W. J. (1997). Indoor space allowance: Effects on growth, behaviour, adrenal and immune responses of finishing beef heifers. Anim. Sci. 64, 53–62. doi: 10.1017/S135772980001554X
Friedman, J., Hastie, T., and Tibshirani, R. (2010). Regularization paths for generalized linear models via Coordinate Descent. J. Stat. Softw. 33, 1–3. doi: 10.18637/jss.v033.i01
Ghassemi Nejad, J., Kim, B. W., Lee, B. H., and Sung, K. (2017). Coat and hair color: hair cortisol and serotonin levels in lactating Holstein cows under heat stress conditions. Anim. Sci. J. 88, 190–194. doi: 10.1111/asj.12662
González-de-la-Vara, M., del, R., Valdez, R. A., Lemus-Ramirez, V., Vázquez-Chagoyán, J. C., Villa-Godoy, A., et al. (2011). Effects of adrenocorticotropic hormone challenge and age on hair cortisol concentrations in dairy cattle. Can. J. Vet. Res. 75, 216–221.
Hayashi, H., Arai, C., Ikeuchi, Y., Yamanaka, M., and Hirayama, T. (2021). Effect of growth and parturition on hair cortisol in Holstein cattle. Anim. Sci. J. 92:e13518. doi: 10.1111/asj.13518
Heimbürge, S., Kanitz, E., Tuchscherer, A., and Otten, W. (2020a). Is it getting in the hair?—Cortisol concentrations in native, regrown and segmented hairs of cattle and pigs after repeated ACTH administrations. Gen. Comp. Endocrinol. 295:113534. doi: 10.1016/j.ygcen.2020.113534
Heimbürge, S., Kanitz, E., Tuchscherer, A., and Otten, W. (2020b). Within a hair's breadth—factors influencing hair cortisol levels in pigs and cattle. Gen. Comp. Endocrinol. 288:113359. doi: 10.1016/j.ygcen.2019.113359
Hill, A. B. (1965). The environment and disease: association or causation? Proc. R. Soc. Med. 58, 295–300.
Hummel, M., Edelmann, D., and Kopp-Schneider, A. (2017). Clustering of samples and variables with mixed-type data. PLoS ONE 12:e0188274. doi: 10.1371/journal.pone.0188274
James, G., Witten, D., Hastie, T., and Tibshirani, R. (2013). An Introduction to Statistical Learning: with Applications in R, eds. G. Casella, S. Fienberg, I. Olkin (Berlin: Springer Publishing Company).
Jensen, M. B., and Kyhn, R. (2000). Play behaviour in group-housed dairy calves, the effect of space allowance. Appl. Anim. Behav. Sci. 67, 35–46. doi: 10.1016/S0168-1591(99)00113-6
Knights, M., and Smith, G. W. (2007). Decreased ACTH secretion during prolonged transportation stress is associated with reduced pituitary responsiveness to tropic hormone stimulation in cattle. Domest. Anim. Endocrinol. 33, 442–450. doi: 10.1016/j.domaniend.2006.09.001
Koolhaas, J. M., De Boer, S. F., Buwalda, B., and Van Reenen, K. (2007). Individual variation in coping with stress: a multidimensional approach of ultimate and proximate mechanisms. Brain. Behav. Evol. 70, 218–226. doi: 10.1159/000105485
Koolhaas, J. M., Korte, S. M., De Boer, S. F., Van Der Vegt, B. J., Van Reenen, C. G., Hopster, H., et al. (1999). Coping styles in animals: current in behavior and stress-physiology. Neurosci. Biobehav. Rev. 23, 925–935. doi: 10.1016/S0149-7634(99)00026-3
Korte, S. M., Olivier, B., and Koolhaas, J. M. (2007). A new animal welfare concept based on allostasis. Physiol. Behav. 92, 422–428. doi: 10.1016/j.physbeh.2006.10.018
Kuhn, M. (2020). caret: Classification and Regression Training. R package version 6.0-86. R package version 6.0-86. Available online at: https://cran.r-project.org/package=caret (accessed December 1, 2021).
Ladewig, J., and Smidt, D. (1989). Behavior, episodic secretion of cortisol, and adrenocortical reactivity in bulls subjected to tethering. Horm. Behav. 23, 344–360. doi: 10.1016/0018-506X(89)90048-2
Leach, K. A., Knierim, U., and Whay, H. R. (2009). “Cleanliness scoring for dairy and beef cattle and veal calves,” in Animal Welfare Measures of Dairy Cattle, Beef Bulls and Veal Calves—Welfare Quality Reports No. 11, eds. L. J. Keeling and B. Forkman (Cardiff), 25–30.
Lecorps, B., Kappel, S., Weary, D. M., and von Keyserlingk, M. A. G. (2018). Dairy calves' personality traits predict social proximity and response to an emotional challenge. Sci. Rep. 8, 1–9. doi: 10.1038/s41598-018-34281-2
Lee, D., Kim, E., and Choi, M. (2015). Technical and clinical aspects of cortisol as a biochemical marker of chronic stress. BMB Rep. 48, 209–216. doi: 10.5483/BMBRep.2015.48.4.275
Lidfors, L. M. (1993). Cross-sucking in group-housed dairy calves before and after weaning off milk. Appl. Anim. Behav. Sci. 38, 15–24. doi: 10.1016/0168-1591(93)90038-Q
Lockwood, S. A., Kattesh, H. G., Rhinehart, J. D., Strickland, L. G., Krawczel, P. D., Wilkerson, J. B., et al. (2017). Relationships among temperament, acute and chronic cortisol and testosterone concentrations, and breeding soundness during performance testing of Angus bulls. Theriogenology 89, 140–145. doi: 10.1016/j.theriogenology.2016.10.019
Meyer, J., Novak, M., Hamel, A., and Rosenberg, K. (2014). Extraction and analysis of cortisol from human and monkey hair. J. Vis. Exp. 2014:e50882. doi: 10.3791/50882
Mormède, P., Andanson, S., Aupérin, B., Beerda, B., Guémen,é, D., Malmkvist, J., et al. (2007). Exploration of the hypothalamic-pituitary-adrenal function as a tool to evaluate animal welfare. Physiol. Behav. 92, 317–339. doi: 10.1016/j.physbeh.2006.12.003
Möstl, E., and Palme, R. (2002). Hormones as indicators of stress. Domest. Anim. Endocrinol. 23, 67–74. doi: 10.1016/S0739-7240(02)00146-7
Moya, D., Schwartzkopf-Genswein, K. S., and Veira, D. M. (2013). Standardization of a non-invasive methodology to measure cortisol in hair of beef cattle. Livest. Sci. 158, 138–144. doi: 10.1016/j.livsci.2013.10.007
Neave, H. W., Costa, J. H. C., Weary, D. M., and von Keyserlingk, M. A. G. (2018). Personality is associated with feeding behavior and performance in dairy calves. J. Dairy Sci. 101, 1–13. doi: 10.3168/jds.2017-14248
Otovic, P., and Hutchinson, E. (2015). Limits to using HPA axis activity as an indication of animal welfare. ALTEX 32, 41–50. doi: 10.14573/altex.1406161
Otten, W., Heimbürge, S., Kanitz, E., and Tuchscherer, A. (2020). It's getting hairy – External contamination may affect the validity of hair cortisol as an indicator of stress in pigs and cattle. Gen. Comp. Endocrinol. 295:113531. doi: 10.1016/j.ygcen.2020.113531
Pawluski, J., Jego, P., Henry, S., Bruchet, A., Palme, R., Coste, C., et al. (2017). Low plasma cortisol and fecal cortisol metabolite measures as indicators of compromised welfare in domestic horses (Equus caballus). PLoS ONE 12:e182257. doi: 10.1371/journal.pone.0182257
R Core Team (2018). R: A Language and Environment for Statistical Computing. Available online at: https://www.r-project.org/ (accessed December 1, 2021).
Robertson, C., Boyle, P., Hsieh, C. C., Macfarlane, G. J., and Maisonneuve, P. (1994). Some statistical considerations in the analysis of case-control studies when the exposure variables are continuous measurements. Epidemiology 5, 164–170. doi: 10.1097/00001648-199403000-00006
Roth, L. S. V., Faresj,ö, Å., Theodorsson, E., and Jensen, P. (2016). Hair cortisol varies with season and lifestyle and relates to human interactions in German shepherd dogs. Sci. Rep. 6:19631. doi: 10.1038/srep19631
Rushen, J. (1991). Problems associated with the interpretation of physiological data in the assessment of animal welfare. Appl. Anim. Behav. Sci. 28, 381–386. doi: 10.1016/0168-1591(91)90170-3
Salimetrics (2018). Calculating Inter- and Intra-Assay Coefficients of Variability. Available online at: https://www.salimetrics.com/calculating-inter-and-intra-assay-coefficients-of-variability/ (accessed September 1, 2018).
Skoluda, N., Dettenborn, L., Stalder, T., and Kirschbaum, C. (2012). Elevated hair cortisol concentrations in endurance athletes. Psychoneuroendocrinology 37, 611–617. doi: 10.1016/j.psyneuen.2011.09.001
Stekhoven, D. J. (2013). missForest: nonparametric missing value imputation using random forest. R package version 1.4.
Stekhoven, D. J., and Bühlmann, P. (2012). Missforest-non-parametric missing value imputation for mixed-type data. Bioinformatics 28, 112–118. doi: 10.1093/bioinformatics/btr597
Tallo-Parra, O., Manteca, X., Sabes-Alsina, M., Carbajal, A., and Lopez-Bejar, M. (2015). Hair cortisol detection in dairy cattle by using EIA: protocol validation and correlation with faecal cortisol metabolites. Animal 9, 1059–1064. doi: 10.1017/S1751731115000294
Tamminen, L. M. (2020). Transmission and Dynamics of VTEC. O157:H7. Uppsala : Sveriges lantbruksuniv.
Tamminen, L. M., Hranac, C. R., Dicksved, J., Eriksson, E., Emanuelson, U., and Keeling, L. J. (2020). Socially engaged calves are more likely to be colonised by VTEC O157:H7 than individuals showing signs of poor welfare. Sci. Rep. 10, 1–12. doi: 10.1038/s41598-020-63186-2
van Eerdenburg, F. J. C. M., Hof, T., Doeve, B., Ravesloot, L., Zeinstra, E. C., Nordquist, R. E., et al. (2021). The relation between hair-cortisol concentration and various welfare assessments of dutch dairy farms. Animals 11, 1–17. doi: 10.3390/ani11030821
Vesel, U., Pavic, T., Jezek, J., Snoj, T., and Staric, J. (2020). Welfare assessment in dairy cows using hair cortisol as a part of monitoring protocols. J. Dairy Res. 87, 72–78. doi: 10.1017/S0022029920000588
Welfare Quality® (2009). “Welfare Quality® assessment protocol for cattle,” in CONSORTIUM WQ, Lelystad.
Whay, H. R., Main, D. C. J., Green, L. E., and Webster, A. J. F. (2003). An animal-based welfare assessment of group-housed calves on UK dairy farms. Anim. Welf. 12, 611–617.
Wickham, H. (2016). ggplot2: Elegant Graphics for Data Analysis. New York: Springer-Verlag. Available online at: https://ggplot2.tidyverse.org/ (accessed December 1, 2021).
Windschnurer, I., Schmied, C., Boivin, X., and Waiblinger, S. (2008). Reliability and inter-test relationship of tests for on-farm assessment of dairy cows' relationship to humans. Appl. Anim. Behav. Sci. 114, 37–53. doi: 10.1016/j.applanim.2008.01.017
Wood, S., and Scheipl, F. (2017). gamm4: generalized additive mixed models using “mgcv” and “lme4”. R package version 0.2-5. Available online at: https://cran.r-project.org/package=gamm4 (accessed December 1, 2021).
Keywords: animal welfare, cattle, HPA axis, stress, coping
Citation: Tamminen L-M, Keeling LJ, Svensson A, Briot L and Emanuelson U (2021) Unraveling the Complexity to Observe Associations Between Welfare Indicators and Hair Cortisol Concentration in Dairy Calves. Front. Anim. Sci. 2:793558. doi: 10.3389/fanim.2021.793558
Received: 12 October 2021; Accepted: 16 November 2021;
Published: 15 December 2021.
Edited by:
Edward Narayan, The University of Queensland, AustraliaReviewed by:
John VanLeeuwen, University of Prince Edward Island, CanadaKerry Lissemore, University of Guelph, Canada
Copyright © 2021 Tamminen, Keeling, Svensson, Briot and Emanuelson. This is an open-access article distributed under the terms of the Creative Commons Attribution License (CC BY). The use, distribution or reproduction in other forums is permitted, provided the original author(s) and the copyright owner(s) are credited and that the original publication in this journal is cited, in accordance with accepted academic practice. No use, distribution or reproduction is permitted which does not comply with these terms.
*Correspondence: Lena-Mari Tamminen, bGVuYS5tYXJpLnRhbW1pbmVuQHNsdS5zZQ==