- Department of Animal and Poultry Sciences, Virginia Tech, Blacksburg, VA, United States
Previous work determined that bovine interleukin-6 (IL6) increases inner cell mass (ICM), primitive endoderm (PE), and total cell number in in vitro produced (IVP) bovine blastocysts. Another IL6 family member, leukemia inhibitory factor (LIF), has the potential to produce the same effects of IL6 due to the presence of its receptor in bovine blastocysts. We compared the abilities of LIF and IL6 to increase ICM cell numbers in day 7, 8, and 9 IVP bovine blastocysts. Supplementation with 100 ng/ml LIF from day 5 onward improved blastocyst formation rates on days 7 and 8 similar to what was observed when supplementing 100 ng/ml IL6. However, LIF supplementation did not cause an increase in ICM numbers like was observed after supplementing IL6. On day 9, increases in PE cell numbers were detected after LIF supplementation, but 300 ng/ml LIF was required to achieve the same effect on PE numbers that was observed by providing 100 ng/ml IL6. Collectively, these results show that LIF can mimic at least some of the effects of IL6 in bovine blastocyst.
Introduction
Leukemia inhibitory factor (LIF) is a well-recognized pluripotency factor in mouse and human embryonic stem cells (ESCs) and induced pluripotent stem cells (iPSCs), where it facilitates the maintenance of cells in a state reminiscent of inner cell mass (ICM) or epiblast (EPI) cells (Fernandes et al., 2010; Fraga et al., 2011; Fernandez-Alonso et al., 2017). In the mouse, LIF supplementation also improves embryo development (Mitchell et al., 1994; Kauma and Matt, 1995; Fedorcsak and Storeng, 2003), is essential for implantation (Nicola and Babon, 2015), and promotes the development of primitive endoderm (PE) (Morgani and Brickman, 2015). The roles for LIF are less well-defined during early embryogenesis in cattle. Blastocyst development, hatching, and/or post-thaw embryo recovery were increased following LIF supplementation in some studies (Yamanaka et al., 1999; Neira et al., 2010; Kocyigit and Cevik, 2015, 2017) but not others (Fukui and Matsuyama, 1994; Sirisathien and Brackett, 2003; Vejlsted et al., 2005; Rodriguez et al., 2007). Equivocal effects of LIF supplementation on total and trophectoderm (TE) cell numbers are also noted (Fukui and Matsuyama, 1994; Yamanaka et al., 1999; Sirisathien and Brackett, 2003; Vejlsted et al., 2005; Rodriguez et al., 2007; Kocyigit and Cevik, 2015, 2017). Interestingly, studies have not detected improvements in ICM cell numbers after LIF exposure, but rather, two studies detected reduced ICM numbers and hypoblast development (Vejlsted et al., 2005; Rodriguez et al., 2007). Some but not all of these discrepancies may be explained by the source and purity of LIF proteins and by using low concentrations of LIF.
Our interest in examining the actions of LIF during bovine embryogenesis was prompted by discovering that interleukin-6 (IL6), a member of the same cytokine family as LIF, contains embryotrophic activities within in vitro-produced (IVP) bovine embryos. IL6 did not greatly affect blastocyst development, but it altered embryo morphology by increasing ICM cell numbers and increasing PE numbers as the ICM cells differentiated into PE and EPI lineages (Wooldridge and Ealy, 2019, 2021; Wooldridge et al., 2019). These findings are noteworthy because several reports have indicated that bovine IVP blastocysts contain fewer ICM cells than in vivo-derived blastocysts (Iwasaki et al., 1990; Knijn et al., 2003), and poor post-transfer development of the embryonic disk and yolk sac, which develops from the ICM and PE, respectively, likely contributes to pregnancy failures in cattle (Bertolini et al., 2002; Fischer-Brown et al., 2004; Alberto et al., 2013; Mess et al., 2017).
Members of the IL6 cytokine family include IL6, LIF, other interleukins (IL11, IL27, IL31), cardiotrophin-1, ciliary neurotrophic factor and oncostatin M. This cytokine family utilizes a common signal-transducing receptor subunit, termed IL6ST (also known as GP130), and ligand-specific α-receptor subunits to mediate intracellular signaling events (Rose-John, 2018). The bovine blastocyst contains transcripts for IL6ST and the α-receptor subunits recognizing IL6 (IL6R) and LIF (LIFR) (Wooldridge et al., 2019). Various downstream intracellular signaling pathways can be mediated by IL6 family cytokines, but arguably the best known signaling system for this cytokine family involves Janus kinase (JAK)-induced phospho-activation of signaling transductor and activator of transcription 3 (STAT3) (Johnson et al., 2018). There is rapid STAT3 phosphorylation, dimerization and nuclear localization within the bovine ICM cells after exposure to IL6 (Wooldridge et al., 2019). Moreover, IL6 requires JAK2 activity to influence ICM and PE development (Wooldridge et al., 2019; Wooldridge and Ealy, 2021).
The effect of IL6 on bovine blastocysts is intriguing, and this prompted us to wonder about whether other members of the IL6 cytokine family could have a similar effect. Due to the recent availability of a bovine recombinant LIF (Kingfisher Biotech), we sought to clarify some of the previous discordance of LIF supplementation on bovine embryos, and to compare the effects of LIF with IL6. Herein we report on the ability of LIF to increase ICM and PE cell numbers in bovine preimplantation embryos.
Materials and Methods
Materials
Unless specified otherwise, reagents were purchased from ThermoFisher Chemical Company (Waltham, MA, USA). No animals were used for this work. All studies were completed on slaughterhouse-derived materials that followed humane slaughter practices according to USDA guidelines.
Bovine embryos were produced by in vitro maturation, fertilization and culture procedures described previously (Zhang et al., 2010; Wooldridge and Ealy, 2019). Cumulus-oocyte complexes (COCs) were harvested both beef and dairy ovaries purchased from Brown Packing Company (Gaffney, SC, USA) and transported to the laboratory in 0.9% [w/v] saline containing antibiotic-antimycotic mix (100 U/ml penicillin, 100 μg/ml streptomycin, 250 ng/ml amphotericin B). Upon arrival, COCs were collected by slashing small and medium-sized follicles (1–8 mm) from ovaries selected for the presence of developing follicles (i.e., presumably from cycling cattle) and then were placed in groups of 20–35 in 500 μl maturation media covered in light mineral oil (Cooper Surgical Inc., Trumbull, CT, USA). No effort was made to account for COC donor breed. The COCs were matured for 21–24 h at 38.5°C in 5% CO2 in humidified air. For fertilization, the COCs were washed in HEPES-SOF before being placed in groups of 150–200 in 3 ml SOF-FERT covered in light mineral oil. Sperm were isolated using a BoviPureTM density gradient (Nidacon; Spectrum Technologies Healdsburg, CA, USA), washed once in SOF-FERT, and then added to the fertilization media at a concentration of 1 million sperm/ml fertilization media. After 14–18 h at 38.5°C in 5% CO2 in humidified air, presumptive zygotes were denuded by gentle pipetting and before being placed in groups of ~25 in 50 μl SOF-BE1 covered by light mineral oil. Zygotes were incubated at 38.5°C in 5% CO2, 5% O2, and 90% N2 in humidified air. In most cases 25–30 zygotes were placed into each drop, but in a few cases, zygotes were group-cultured with as few as 18 zygotes and as many as 32 zygotes. Blastocyst formation was assessed on days 7 and 8 post-fertilization as the percentage of cleaved embryos that formed blastocysts. Blastocysts were then also categorized as “regular” (blastocoel of >50% the embryo's mass, but no increase in embryo diameter), or “advanced” (having an obvious increase in diameter; this included both expanded and hatched blastocysts).
LIF and IL6 Stock Preparation
Concentrated stocks (10 μg/ml) of LIF (recombinant bovine; Kingfisher Biotech, St. Paul, MN, USA) and IL6 (recombinant bovine; Kingfisher Biotech) were prepared in SOF containing 1% [w/v] bovine serum albumin (BSA). Control treatment stocks contained SOF with 1%BSA. Stocks were frozen in single-use aliquots at −80°C. Embryos were not removed from their original drops. In most studies, treatments were administered either at day 5 post-fertilization to all embryos regardless of stage of development by the addition of 2 μl of stock solution to each SOF-BEI drop containing embryos to achieve in-droplet final concentrations of 0, 100, or 300 ng/ml, depending on the study. In one study (immunofluorescence work), IL6 or LIF was administered 30 min prior to fixation and staining.
Immunofluorescence
On days 7 and 8, a representative sampling of regular and expanded blastocysts were selected (i.e., a similar proportion of regular or advanced blastocysts as observed for that treatment group in each replicate). On day 9, only expanded and hatched blastocysts were selected. In no studies were “early” blastocysts (having a small cavity, <50% of the embryo's mass) used for analysis.
The CDX2 staining was completed as described previously by this group (Wooldridge and Ealy, 2019). In brief, blastocysts were fixed in 4% [w/v] paraformaldehyde, permeabilized using 0.25% [v/v] Triton-X and blocked with 10% [v/v] Horse Serum. Embryos were then incubated with anti-CDX2 primary antibody (Biogenex, AM392-5M, sold ready-to-use) and incubated with either donkey anti-mouse FITC or Alexa Fluor 647, depending on the study (Invitrogen, A16018 or A31571, 1:200 dilution for either). Both secondaries performed well.
The CDX2, NANOG, and GATA6 co-staining studies were completed as described previously by this group (Wooldridge and Ealy, 2021). Blastocysts were fixed in 4% [w/v] paraformaldehyde, permeabilized with 0.5% Triton-X in Dulbecco's Phosphate Buffered Saline (DPBS) and blocked with 10% [v/v] horse serum. Due to antibody overlap, two rounds of primary and secondary antibody incubations were completed. First, blastocysts were incubated with rabbit anti-GATA6 (Cell Signaling Technology, Danvers, MA; 5851T; 1:500) and mouse anti-NANOG (eBioscience; 14-5768-82; 1:200) for 1 h at room temperature, then blastocysts were incubated with donkey anti-rabbit IgG (Alexafluor 555; 1:200) and donkey anti-mouse IgG (Alexafluor 647; 1:200). Blastocysts were then incubated with mouse anti-CDX2 antibody (same as above) for 1 h at room temperature and finally exposed to donkey anti-mouse IgG (Alexafluor 488; 1:500).
At the end of each staining protocol, embryos were incubated with 1 μg/ml DAPI for 5 min at room temperature then washed in PBS-PVP and then either flattened on a glass slide lined with petroleum jelly for cell counting or not flattened for z-series acquisition and use for capturing images used in Figure 3. Staining was visualized with an Eclipse Ti-E inverted microscope equipped with an X-cite 120 epifluorescence illumination system and DS-L3 digital camera. Images were captured with NIS-Elements Software (Nikon Instruments, Melville, NY, USA), and cell counting was completed with the cell counter plugin in the program FIJI (ImageJ) (Wooldridge and Ealy, 2019). For the day 7 and 8 studies, CDX2+ nuclei were designated as TE and CDX− nuclei that were DAPI+ were considered ICM. For the day 9 studies, nuclei staining only with NANOG were considered EPI cells, while those nuclei that stained only with GATA6 were considered PE cells. Nuclei that contained staining for both NANOG and GATA6 were termed undifferentiated (UN) ICM cells. A few TE cells stained weakly for GATA6. Because of this, only nuclei that were CDX2− were considered as GATA6+ ICM cells.
Statistical Analyses
All studies were completed using a complete randomized block design with similar numbers of embryos. In most cases the same number of embryos were used within the same replicate study. Analyses were completed by least-squares ANOVA using the general linear model of the Statistical Analysis System (Proc GLM; SAS for Windows, version 9.4; SAS Institute Inc., Cary, NC, USA). Individual comparisons were partitioned further, when necessary, by using the Probability of difference (PDIFF) test of SAS. Replicate was used as the experimental unit for blastocyst formation studies. Percentage data (e.g., blastocyst formation rates) were arcsine-transformed before analysis but are presented as non-transformed means and SEM. The Tukey honestly significant difference test was used for all blastocyst formation data. Embryo was considered the experimental unit for cell counting studies. Statistical significance was determined at P ≤ 0.05 and a statistical tendency was defined as P > 0.05 and ≤ 0.1.
Results
LIF Increases Advanced Blastocyst Formation but Not ICM Cell Number
The effect of IL6 or LIF supplementation on blastocyst development and cell numbers were determined in a study where embryos were supplemented with either 100 ng/ml IL6, 100 ng/ml LIF or carrier only (1% [w/v] BSA) beginning at day 5 (Figure 1). On day 7, neither LIF nor IL6 supplementation affected the percentage of cleaved embryos that formed blastocysts (Figure 1A). On day 8, total blastocyst formation was not affected by LIF or IL6, but the percentage of advanced blastocysts was greater (P < 0.05) in both LIF and IL6-supplemented embryos when compared with controls (Figure 1A).
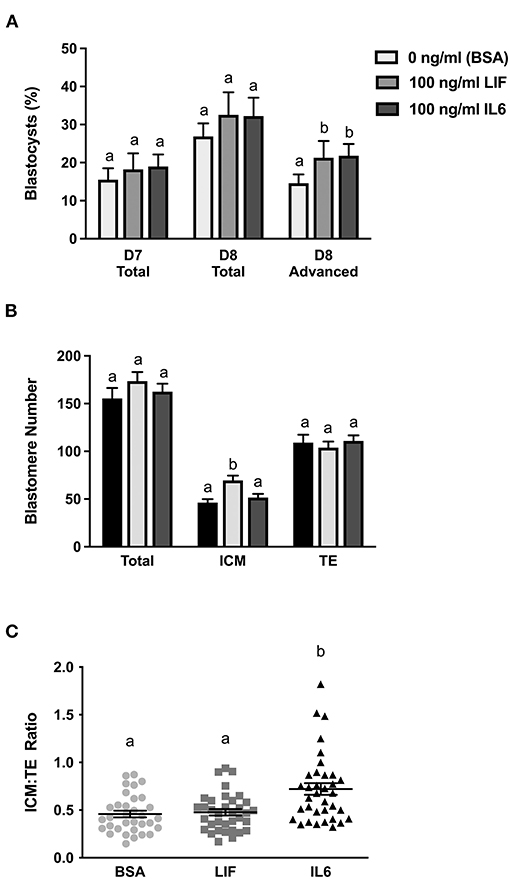
Figure 1. Comparison of LIF vs. IL6 supplementation on blastocyst formation (n = 18–32 zygotes/drop; 2–4 drops/treatment/replicate; 8 replicates) and day 8 cell numbers (n = 33–35 blastocysts/treatment over 7 replicates). (A) Effects of 100 ng/ml IL6 or 100 ng/ml LIF supplementation on total blastocyst formation at day 7 and total and advanced blastocyst formation at day 8. (B) Effects of 100 ng/ml IL6 or 100 ng/ml LIF supplementation on total, inner cell mass (ICM), and trophectoderm (TE) cell numbers in day 8 blastocysts. (C) The effects of 100 ng/ml IL6 or 100 ng/ml LIF supplementation on ICM:TE ratios of day 8 blastocysts. Different superscripts within each panel indicate differences (P < 0.05).
A subset of representative blastocysts from each treatment were immuno-stained for CDX2 and DAPI to determine total, ICM and TE cell numbers at day 8 (Figure 1B). Total and TE numbers were not affected by LIF or IL6 supplementation. Number of ICM cells were not affected by LIF supplementation but were increased (P < 0.05) following IL6 supplementation (Figure 1B). Also, supplementation with LIF did not affect the ICM:TE ratio whereas IL6 increased (P < 0.05) the ICM:TE ratio (Figure 1C).
A follow-up study examined whether LIF or IL6 supplementation beginning on day 5 post-fertilization affected total, ICM, and TE numbers in day 7 blastocysts (Figure 2). Supplementation with LIF did not affect total, ICM or TE numbers whereas increases (P < 0.05) in total and ICM numbers but not TE numbers were detected in IL6-supplemented blastocysts (Figure 2A). The ICM:TE ratio was not affected by LIF supplementation but was increased (P < 0.05) in IL6-supplemented blastocysts (Figure 2B).
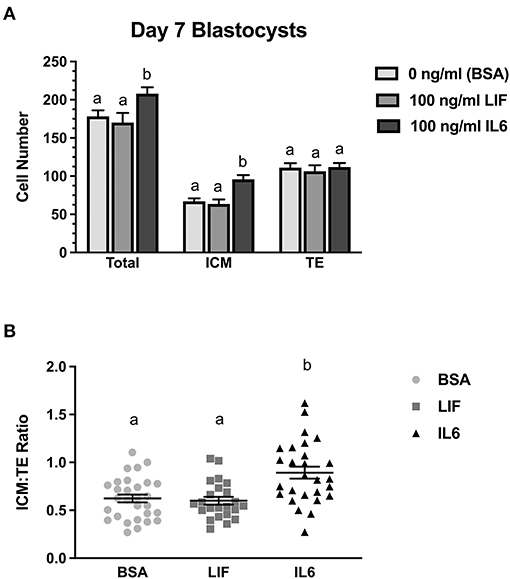
Figure 2. Comparison of LIF vs. IL6 supplementation on day 7 blastocyst cell numbers (n = 23–29 blastocyst/treatment/timepoint over 3 replicates). (A) Effects of 100 ng/ml IL6 or 100 ng/ml LIF on total, inner cell mass (ICM), and trophectoderm (TE) cell numbers in day 7 blastocysts. (B) Effects of 100 ng/ml IL6 or 100 ng/ml LIF on ICM:TE ratios of day 7 blastocysts. Different superscripts within each panel indicate differences (P < 0.05).
LIF Can Influence PE Lineage Development
Two studies were completed to examine whether LIF supplementation beginning at day 5 post-fertilization influences the number and distribution of EPI and PE cells within the ICM at day 9 (Figures 3A,B). Representative images of immunostaining for markers of EPI (NANOG+), PE (GATA6+), and TE (CDX2+) are presented in Figure 3C. A few GATA6:CDX2 dual-positive nuclei identified in this study. This was rare. Usually only 1–2 nuclei for every 5–10 blastocysts examined. The reason for this dual positivity remained unclear, but they were always located within the TE region, so they were considered TE and were not included as GATA6+ PE cells. In the first study (Figure 3A), total ICM numbers (either NANOG+ or GATA6+ or dual positive) and EPI cell numbers were not different between LIF-supplemented and control blastocysts, but there was a tendency for increased (P = 0.07) PE cell numbers in LIF-supplemented blastocysts when compared with control blastocysts. The IL6-supplemented blastocysts exhibited greater (P < 0.05) ICM, PE, and UN cell numbers but similar EPI cell numbers when compared with control blastocysts. No differences in PE, EPI, or UN cells were detected for LIF vs. IL6-treated blastocysts, respectively, but there was a tendency (P = 0.09) for IL6-supplemented blastocysts to contain more total ICM cells than LIF-supplemented blastocysts. No differences in TE cell numbers were detected between controls and LIF-treated blastocysts, but there was a tendency (P = 0.09) for increased TE cell numbers in IL6-treated blastocysts when compared with controls. No difference in TE cell number was observed between LIF and IL6-treated blastocysts.
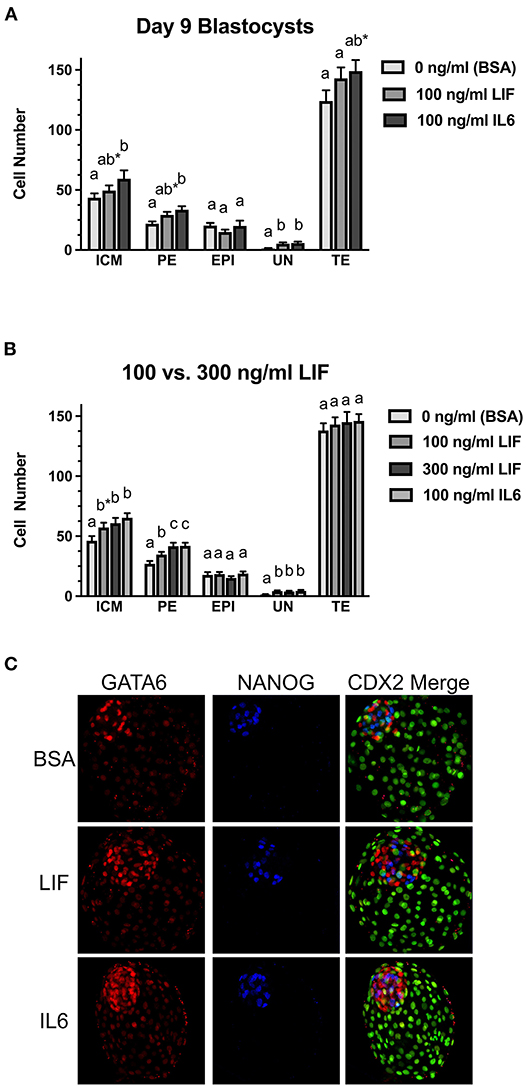
Figure 3. Comparison of LIF vs. IL6 supplementation on day 9 blastocyst cell numbers. (A) Effects of 100 ng/ml IL6 or 100 ng/ml LIF on inner cell mass (ICM), primitive endoderm (PE), epiblast (EPI), undetermined (UN), and trophectoderm (TE) cell numbers in day 9 blastocysts (n = 27–29 blastocyst/treatment/timepoint over 3 replicates). (B) Effects of 100 ng/ml IL6 or either 100 or 300 ng/ml LIF on ICM, PE, EPI, UN, and TE cell numbers in day 9 blastocysts (n = 36–45 blastocysts/treatment over 3 replicates). Different superscripts for each endpoint indicate differences (P < 0.05). The asterisks (*) indicate a tendency for a difference between the control (BSA only) and 100 ng/ml LIF within the ICM (P = 0.09) and a tendency for a difference between the control (BSA only) and 100 ng/ml IL6 within the TE (P = 0.09). (C) Examples of day 9 blastocysts treated with BSA (control, no treatment), 300 ng/ml LIF (LIF) or 100 ng/ml IL6 (IL6). Green signals represent CDX2+ cells in the far-right column.
The second study examined whether providing a greater LIF concentration (300 ng/ml) beginning on day 5 post-fertilization could influence ICM cell numbers in day 9 blastocysts (Figure 3B). This LIF concentration did not affect the percentage of blastocysts (data not shown). When compared to the controls, there was a tendency (P = 0.07) for greater total ICM numbers in blastocysts exposed to 100 ng/ml LIF, and greater (P < 0.05) total ICM numbers were observed in blastocysts treated with 300 ng/ml LIF or 100 ng/ml IL6 groups. Total ICM numbers did not differ among the IL6 and two LIF treatment groups. PE cell numbers were greater (P < 0.05) in blastocysts supplemented with 100 ng/ml or 300 ng/ml LIF or 100 ng/ml IL6. Also, PE cell numbers were greater (P < 0.05) for the 300 ng/ml LIF and 100 ng/ml IL6 treatments than the 100 ng/ml LIF treatment. No changes in EPI cell numbers were observed among the treatments but increases (P < 0.05) in UN cell numbers were detected in blastocysts supplemented with 100 or 300 ng/ml LIF or 100 ng/ml IL6. None of the treatments affected TE cell number in this study.
Discussion
Published reports have failed to glean definitive actions of LIF on blastocyst formation and composition, hypoblast development, and cryoprotection in cattle (Fukui and Matsuyama, 1994; Yamanaka et al., 1999; Sirisathien et al., 2003; Vejlsted et al., 2005; Rodriguez et al., 2007; Kocyigit and Cevik, 2015, 2017). Some of the problems with defining LIF actions may be attributed to differences in culture media formulations and culture conditions, the addition of undefined media components (e.g., serum), and the concentrations of LIF examined (range: 2–100 ng/ml). Also, most of the previous studies utilized recombinant human or mouse LIF preparations. Amino acid sequence identity is fairly similar for LIF between these species and the bovine (89 and 73% identity of human and mouse LIF, respectively), so cross-species activities for these various LIF proteins may not be a major concern, but the use of bovine recombinant LIF in this work optimized opportunities for detecting biological effects. It also allowed us to compare LIF activity with recombinant bovine IL6. Protein concentration was used herein because the proteins have a similar mass (19.8 kDa for IL6; 20.7 kDa for LIF).
No efforts were taken to ensure that LIF and IL6 contained similar specific activities (i.e., similar ability to act on a standardized cell line). However, the recombinant bovine LIF protein used for this work was biologically active in bovine embryos. This LIF protein increased the percentage of expanded and hatched blastocysts on day 8 and increased PE and UN numbers. No dose-response studies were completed for LIF. Previous dose-response studies completed with IL6 found that concentrations lower than 100 ng/ml failed to consistently affect ICM cell numbers in bovine blastocysts (Wooldridge and Ealy, 2019). Therefore, this work focused on comparing this effective concentration for IL6 with equal or greater concentrations of LIF.
This work found that LIF cannot mimic IL6's ability to influence ICM development in blastocysts at days 7 and 8 post-fertilization. The scarcity of LIFR transcripts offers a potential reason why LIF failed to act in day 7 and 8 blastocysts. RNA-sequencing detected LIFR transcripts in day 8 bovine blastocysts, but its abundance was low and was ~60-fold less than the abundances of IL6R and IL6ST (Wooldridge et al., 2019). Therefore, it appears that LIF can act before the blastocyst stage in bovine embryos, but the absence of LIFR abundance in day 7–8 blastocysts limits it actions at this time. We have not examined LIFR expression profiles after this time. The present findings indicate the PE is a target of LIF, so perhaps LIFR expression is restricted to this cell type in the blastocyst. A more complete picture of the timing of LIFR expression and its localization pattern within the ICM and TE is needed to clarify how LIF may function before and after day 7–8 of development. Also, a greater focus on LIF responses within the TE should be considered in future work. The percentage of expanded and hatched blastocysts at day 8 were increased with LIF treatment. Unfortunately, TE cell numbers were not recorded at day 9, but in retrospect, more attention could have been paid to describing how LIF influences the TE. In the mouse, the TE is the primary target for LIF (Lavranos et al., 1995).
It is noteworthy to mention that consistent responses in ICM and total cell numbers were observed after IL6 and LIF supplementation across studies examining blastocysts at days 7, 8, or 9. Variations in cell numbers were detected across these studies. Such study-to-study variations are not uncommon. However, they may also reflect a phenomenon we have observed in previous work, where a decrease in ICM numbers is observed with extended blastocyst culture in SOF-BE1 medium (Wooldridge and Ealy, 2021), the same media used in this work. We suspect this reflects insufficiencies in the SOF-BE1 formation to support ICM development. It will be interesting to explore how profound ICM responses to IL6 treatment using media formulations reported by others that support ICM development in blastocysts (Ramos-Ibeas et al., 2020; Isaac and Pfeffer, 2021).
A second notable outcome of this work was observing that LIF can mimic IL6's actions on PE development. Greater LIF concentrations were required to elicit an effect that was similar to IL6, but nonetheless, LIF was able to increase PE numbers in day 9 blastocysts. Further work is needed to determine if increasing IL6 concentrations would produce greater PE cell number responses, although a previous study found no added benefit to supplementing 200 ng/ml IL6 when compared with the 100 ng/ml treatment (Wooldridge and Ealy, 2019). Determination of PE and EPI occurs randomly throughout the ICM at days 8 and 9 in bovine blastocysts based on ICM cell sensitivity to FGF2 and FGF4 (Yang et al., 2011; Kuijk et al., 2012). Initially, the EPI and PE lineages produce a scattered, “salt and pepper” distribution of differentiated cells within the ICM when stained for PE and EPI markers (see Figure 3C; Yamanaka et al., 2010; Kuijk et al., 2012). However, soon after their specification, PE cells migrate to the base of the ICM to form the hypoblast layer, which will then expands underneath the TE to form the yolk sac (Hosoe et al., 2019). The present work did not extend the blastocyst cultures to the point where hypoblast formation occurred, but another group failed to see a positive effect of LIF supplementation on hypoblast development in IVP bovine blastocysts cultured for extended periods (Hosoe et al., 2019), although a significantly lower LIF concentration was tested in that study (20 ng/ml). Moreover, IVP embryo degeneration occurred in these cultures, suggesting that embryo transfer may be needed to adequately test the potential benefits of IL6 and LIF on hypoblast and yolk sac development.
One shortcoming of using the recombinant bovine IL6 and LIF proteins was that the specific activity of both proteins was not determined by the manufacturer. In retrospect, we should have completed dose-response studies on a bovine cell line to evaluate the minimal effective concentration needed to stimulate cell proliferation or activate pSTAT3Y705. However, the bovine LIF protein used herein contained biological activity. When provided at 100 ng/ml, LIF promoted advanced blastocyst development at day 8, increased the number of UN ICM cells, and tended to increase ICM and PE numbers at day 9. Another potential limitation was not using confocal microscopy or z-stacking to capture blastocysts for nuclei counting. The ICM region is of special concern because these cells are closely packed together. Flattening the embryos before capturing the images may have prevented us from counting and categorizing every ICM nucleus. Thus, it is possible that ICM, PE, EPI, and UN numbers were underdetermined. We propose, however, that there was no treatment bias associated with this underestimation, if it existed.
The potential mechanism for IL6 and LIF-mediated expansion of the PE was not pursued in this project. However, current and previous work by this laboratory (Wooldridge and Ealy, 2021) found no evidence that IL6 or LIF causes fewer EPI cells to form, so it seems more likely that these cytokines are acting after PE specification rather than before the EPI/PE specification event has taken place. Moreover, there is evidence in the mouse blastocyst that LIF functions after PE lineage specification, where it specifically functions to limit PE cell apoptosis rather than promoting PE proliferation (Morgani et al., 2013; Morgani and Brickman, 2015).
It is interesting to note that redundancies in IL6 and LIF action on PE has also been observed in mice. Both IL6 and LIF can control PE lineage development in PE lines derived from primed mouse ESCs (Morgani and Brickman, 2015). However, LIF appears to be the primary player in controlling mouse PE development. Greater IL6 concentrations are needed to produce the same effects as LIF on these cells (Morgani and Brickman, 2015). The opposite was seen in the present work, where IL6 could increase PE development at lower concentrations than LIF. Therefore, the actions of IL6 and LIF on PE development are similar in the mouse and cow, but the primary mediator of this action differs between the two species. It is not clear in the cow or mouse whether signaling from any IL6 family member is necessary for normal yolk sac development. Loss of Il6st function in the mouse causes embryonic lethality, although a link with poor yolk sac development has not been explored (Yoshida et al., 1996). Loss of Lifr function contains a partial embryonic lethal, with an underrepresentation of Lifr−/− embryos in pregnancies at embryonic day 3.5 (blastocyst stage) and 9.5 (post-implantation) (Ware et al., 1995). No adverse pregnancy events occur in Il6r-deficient mice (McFarland-Mancini et al., 2010).
Transcription profiling work completed in bovine blastocysts (Wooldridge et al., 2019) also supports the idea that IL6 is the preferred ligand within the IL6 cytokine family for controlling PE lineage development in cattle. The level of IL6 and IL6R mRNA expression far exceeds that of any other IL6 family ligand/receptor combination. Also, transcript abundance for IL6R is similar with that of IL6ST whereas other receptor subunits have markedly lower expression. The expression of LIFR, for example, is 50-times less than that for IL6R and IL6ST. Also, LIF mRNA expression is undetectable in bovine blastocysts. It is unclear if endometrial-derived LIF influences bovine embryogenesis, but we cannot discount this possibility given that endometrial LIF mediates implantation in other species (Nichols et al., 2001).
Neither LIF nor IL6 affected EPI cell numbers. The absence of IL6 effect was also observed in earlier work (Wooldridge and Ealy, 2021). This suggests that LIF and IL6 are unable to affect EPI cell proliferation and/or survival. However, LIF and IL6 were equally effective at increasing UN cell numbers. For IL6, this could very well-represent its ability to influence ICM cell numbers prior to EPI/PE specification, as observed recently in blastocysts examined at days 7 and 8 post-fertilization (Wooldridge et al., 2019). However, LIF did not influence ICM numbers at day 7 or 8 in this work. Therefore, it is more likely that LIF and IL6 may be limiting or delaying ICM cell differentiation. This speculation is in-line with LIF's activity as a pluripotency factor (Fernandes et al., 2010; Fraga et al., 2011; Fernandez-Alonso et al., 2017).
It remains unclear how IL6 and LIF may act within the ICM because tight junctions within the TE create a diffusion barrier for many molecules. A direct effect of IL6 on the ICM appears to occur for IL6 given that STAT3 activation and nuclear localization is observed within 30 min (Wooldridge and Ealy, 2019, 2021; Wooldridge et al., 2019). However, the mechanism of IL6 delivery is unclear. Transcytosis is a possible mechanism for IL6 transport. The bovine TE contains both subunits of the IL6 receptor (IL6R and IL6ST) (Ozawa et al., 2012). Alternatively, IL6 can modify tight junction permeability to permit its passage through the intestinal epithelium (Suzuki et al., 2011; Al-Sadi et al., 2014). Perhaps a similar mechanism is utilized in the TE. Similar mechanisms have not been described for LIF. Alternatively, perhaps the ICM-mediated actions of IL6 and LIF are indirect, and these cytokines act on the TE to modulate effector molecules that then act on the ICM.
In conclusion, LIF could not mimic IL6's ability to increase ICM cell numbers at day 8 post-fertilization but it could replicate the actions of IL6 on the PE when administered at a greater concentration than what is required to detect an effect for IL6. Both embryokines increased PE cell numbers, although IL6 was more effective at accomplishing this than LIF. Exploring the roles for IL6, LIF and potentially other IL6 cytokine family members in yolk sac development in the cow is important because poor yolk sac development exists in a subset of transferred IVP bovine embryos (Alberto et al., 2013; Mess et al., 2017). Also, there is a high incidence of pregnancy losses in inseminated cattle during the time when the yolk sac functions as the primary placental source of nutrients (Wiltbank et al., 2016; Ealy et al., 2019; Reese et al., 2020). It remains untested whether providing IL6 or LIF to IVP before transfer will improve yolk sac development, but the notable effects of IL6 and LIF on PE suggest that this cytokine family play at least a facilitative role in mediating PE development in the bovine conceptus.
Data Availability Statement
The raw data supporting the conclusions of this article will be made available by the authors, without undue reservation.
Author Contributions
AE conceived the studies, oversaw the completion of the studies and statistical analyses, and wrote the final version of the manuscript. LW assisted with conceiving the studies, performed the experiments, analyzed the data, and wrote the initial draft of the manuscript. All authors contributed to the article and approved the submitted version.
Funding
This work includes Agriculture and Food Research Initiative Competitive Grant Nos. 2017-67015-26461, 2018-67030-28727, and 2021-67015-34485 from the USDA National Institute of Food and Agriculture and by the National Institute of Health grant number R21-OD026516-01. Fellowship support was provided by Agriculture and Food Research Initiative Competitive Grant No. 2018-67011-27993 from the USDA National Institute of Food and Agriculture.
Conflict of Interest
The authors declare that the research was conducted in the absence of any commercial or financial relationships that could be construed as a potential conflict of interest.
Publisher's Note
All claims expressed in this article are solely those of the authors and do not necessarily represent those of their affiliated organizations, or those of the publisher, the editors and the reviewers. Any product that may be evaluated in this article, or claim that may be made by its manufacturer, is not guaranteed or endorsed by the publisher.
Acknowledgments
Authors thank Dr. Matthew Utt, Dr. Bo Harstine and Select Sires, Inc. (Plain City, OH) for donating the bovine semen used for this work. The authors also thank graduate and undergraduate students at Virginia Tech for assisting with bovine in vitro embryo production.
References
Alberto, M. L., Meirelles, F. V., Perecin, F., Ambrosio, C. E., Favaron, P. O., Franciolli, A. L., et al. (2013). Development of bovine embryos derived from reproductive techniques. Reprod. Fertil. Dev. 25, 907–917. doi: 10.1071/RD12092
Al-Sadi, R., Ye, D., Boivin, M., Guo, S., Hashimi, M., Ereifej, L., et al. (2014). Interleukin-6 modulation of intestinal epithelial tight junction permeability is mediated by JNK pathway activation of claudin-2 gene. PLoS ONE 9:e85345. doi: 10.1371/journal.pone.0085345
Bertolini, M., Beam, S. W., Shim, H., Bertolini, L. R., Moyer, A. L., Famula, T. R., et al. (2002). Growth, development, and gene expression by in vivo- and in vitro-produced day 7 and 16 bovine embryos. Mol. Reprod. Dev. 63, 318–328. doi: 10.1002/mrd.90015
Ealy, A. D., Wooldridge, L. K., and McCoski, S. R. (2019). Post-transfer consequences of in vitro-produced embryos in cattle. J. Anim. Sci. 97, 2555–2568. doi: 10.1093/jas/skz116
Fedorcsak, P., and Storeng, R. (2003). Effects of leptin and leukemia inhibitory factor on preimplantation development and STAT3 signaling of mouse embryos in vitro. Biol. Reprod. 69, 1531–1538. doi: 10.1095/biolreprod.103.019034
Fernandes, A. M., Meletti, T., Guimaraes, R., Stelling, M. P., Marinho, P. A., Valladao, A. S., et al. (2010). Worldwide survey of published procedures to culture human embryonic stem cells. Cell Transplant. 19, 509–523. doi: 10.3727/096368909X485067
Fernandez-Alonso, R., Bustos, F., Williams, C. A. C., and Findlay, G. M. (2017). Protein kinases in pluripotency-beyond the usual suspects. J. Mol. Biol. 429, 1504–1520. doi: 10.1016/j.jmb.2017.04.013
Fischer-Brown, A. E., Lindsey, B. R., Ireland, F. A., Northey, D. L., Monson, R. L., Clark, S. G., et al. (2004). Embryonic disc development and subsequent viability of cattle embryos following culture in two media under two oxygen concentrations. Reprod. Fertil. Dev. 16, 787–793. doi: 10.1071/rd04026
Fraga, A. M., Souza de Araujo, E. S., Stabellini, R., Vergani, N., and Pereira, L. V. (2011). A survey of parameters involved in the establishment of new lines of human embryonic stem cells. Stem Cell Rev. Rep. 7, 775–781. doi: 10.1007/s12015-011-9250-x
Fukui, Y., and Matsuyama, K. (1994). Development of in vitro matured and fertilized bovine embryos cultured in media containing human leukemia inhibitory factor. Theriogenology 42, 663–673. doi: 10.1016/0093-691x(94)90383-t
Hosoe, M., Furusawa, T., Hayashi, K. G., Takahashi, T., Hashiyada, Y., Kizaki, K., et al. (2019). Characterisation of bovine embryos following prolonged culture in embryonic stem cell medium containing leukaemia inhibitory factor. Reprod. Fertil. Dev. 31, 1157–1165. doi: 10.1071/RD18343
Isaac, E., and Pfeffer, P. L. (2021). Growing cattle embryos beyond day 8 - an investigation of media components. Theriogenology 161, 273–284. doi: 10.1016/j.theriogenology.2020.12.010
Iwasaki, S., Yoshiba, N., Ushijima, H., Watanabe, S., and Nakahara, T. (1990). Morphology and proportion of inner cell mass of bovine blastocysts fertilized in vitro and in vivo. J. Reprod. Fertil. 90, 279–284. doi: 10.1530/jrf.0.0900279
Johnson, D. E., O'Keefe, R. A., and Grandis, J. R. (2018). Targeting the IL-6/JAK/STAT3 signalling axis in cancer. Nat. Rev. Clin. Oncol. 15, 234–248. doi: 10.1038/nrclinonc.2018.8
Kauma, S. W., and Matt, D. W. (1995). Coculture cells that express leukemia inhibitory factor (LIF) enhance mouse blastocyst development in vitro. J. Assist. Reprod. Genet. 12, 153–156. doi: 10.1007/bf02211386
Knijn, H. M., Gjorret, J. O., Vos, P. L., Hendriksen, P. J., van der Weijden, B. C., Maddox-Hyttel, P., et al. (2003). Consequences of in vivo development and subsequent culture on apoptosis, cell number, and blastocyst formation in bovine embryos. Biol. Reprod. 69, 1371–1378. doi: 10.1095/biolreprod.103.017251
Kocyigit, A., and Cevik, M. (2015). Effects of leukemia inhibitory factor and insulin-like growth factor-I on the cell allocation and cryotolerance of bovine blastocysts. Cryobiology 71, 64–69. doi: 10.1016/j.cryobiol.2015.05.068
Kocyigit, A., and Cevik, M. (2017). Leucemia inhibitory factor; investigating the time-dependent effect on viability of vitrified bovine embryos. Reprod. Domest. Anim. 52, 1113–1119. doi: 10.1111/rda.13040
Kuijk, E. W., van Tol, L. T., Van de Velde, H., Wubbolts, R., Welling, M., Geijsen, N., et al. (2012). The roles of FGF and MAP kinase signaling in the segregation of the epiblast and hypoblast cell lineages in bovine and human embryos. Development 139, 871–882. doi: 10.1242/dev.071688
Lavranos, T. C., Rathjen, P. D., and Seamark, R. F. (1995). Trophic effects of myeloid leukaemia inhibitory factor (LIF) on mouse embryos. J. Reprod. Fertil. 105, 331–338. doi: 10.1530/jrf.0.1050331
McFarland-Mancini, M. M., Funk, H. M., Paluch, A. M., Zhou, M., Giridhar, P. V., Mercer, C. A., et al. (2010). Differences in wound healing in mice with deficiency of IL-6 versus IL-6 receptor. J. Immunol. 184, 7219–7228. doi: 10.4049/jimmunol.0901929
Mess, A. M., Carreira, A. C. O., Marinovic de Oliveira, C., Fratini, P., Favaron, P. O., Barreto, R., et al. (2017). Vascularization and VEGF expression altered in bovine yolk sacs from IVF and NT technologies. Theriogenology 87, 290–297. doi: 10.1016/j.theriogenology.2016.09.012
Mitchell, M. H., Swanson, R. J., Hodgen, G. D., and Oehninger, S. (1994). Enhancement of in vitro murine embryo development by recombinant leukemia inhibitory factor. J. Soc. Gynecol. Investig. 1, 215–219. doi: 10.1177/107155769400100307
Morgani, S. M., and Brickman, J. M. (2015). LIF supports primitive endoderm expansion during pre-implantation development. Development 142, 3488–3499. doi: 10.1242/dev.125021
Morgani, S. M., Canham, M. A., Nichols, J., Sharov, A. A., Migueles, R. P., Ko, M. S., et al. (2013). Totipotent embryonic stem cells arise in ground-state culture conditions. Cell Rep. 3, 1945–1957. doi: 10.1016/j.celrep.2013.04.034
Neira, J. A., Tainturier, D., Pena, M. A., and Martal, J. (2010). Effect of the association of IGF-I, IGF-II, bFGF, TGF-beta1, GM-CSF, and LIF on the development of bovine embryos produced in vitro. Theriogenology 73, 595–604. doi: 10.1016/j.theriogenology.2009.10.015
Nichols, J., Chambers, I., Taga, T., and Smith, A. (2001). Physiological rationale for responsiveness of mouse embryonic stem cells to gp130 cytokines. Development 128, 2333–2339. doi: 10.1242/dev.128.12.2333
Nicola, N. A., and Babon, J. J. (2015). Leukemia inhibitory factor (LIF). Cytokine Growth Factor Rev. 26, 533–544. doi: 10.1016/j.cytogfr.2015.07.001
Ozawa, M., Sakatani, M., Yao, J., Shanker, S., Yu, F., Yamashita, R., et al. (2012). Global gene expression of the inner cell mass and trophectoderm of the bovine blastocyst. BMC Dev. Biol. 12:33. doi: 10.1186/1471-213X-12-33
Ramos-Ibeas, P., Lamas-Toranzo, I., Martinez-Moro, A., de Frutos, C., Quiroga, A. C., Zurita, E., et al. (2020). Embryonic disc formation following post-hatching bovine embryo development in vitro. Reproduction 160, 579–589. doi: 10.1530/REP-20-0243
Reese, S. T., Franco, G. A., Poole, R. K., Hood, R., Fernadez Montero, L., Oliveira Filho, R. V., et al. (2020). Pregnancy loss in beef cattle: a meta-analysis. Anim. Reprod. Sci. 212:106251. doi: 10.1016/j.anireprosci.2019.106251
Rodriguez, A., De Frutos, C., Diez, C., Caamano, J. N., Facal, N., Duque, P., et al. (2007). Effects of human versus mouse leukemia inhibitory factor on the in vitro development of bovine embryos. Theriogenology 67, 1092–1095. doi: 10.1016/j.theriogenology.2006.11.015
Rose-John, S. (2018). Interleukin-6 family cytokines. Cold Spring Harb. Perspect. Biol. 10:a028415. doi: 10.1101/cshperspect.a028415
Sirisathien, S., and Brackett, B. G. (2003). TUNEL analyses of bovine blastocysts after culture with EGF and IGF-I. Mol. Reprod. Dev. 65, 51–56. doi: 10.1002/mrd.10263
Sirisathien, S., Hernandez-Fonseca, H. J., Bosch, P., Hollet, B. R., Lott, J. D., and Brackett, B. G. (2003). Effect of leukemia inhibitory factor on bovine embryos produced in vitro under chemically defined conditions. Theriogenology 59, 1751–1763. doi: 10.1016/s0093-691x(02)01258-x
Suzuki, T., Yoshinaga, N., and Tanabe, S. (2011). Interleukin-6 (IL-6) regulates claudin-2 expression and tight junction permeability in intestinal epithelium. J. Biol. Chem. 286, 31263–31271. doi: 10.1074/jbc.M111.238147
Vejlsted, M., Avery, B., Gjorret, J. O., and Maddox-Hyttel, P. (2005). Effect of leukemia inhibitory factor (LIF) on in vitro produced bovine embryos and their outgrowth colonies. Mol. Reprod. Dev. 70, 445–454. doi: 10.1002/mrd.20221
Ware, C. B., Horowitz, M. C., Renshaw, B. R., Hunt, J. S., Liggitt, D., Koblar, S. A., et al. (1995). Targeted disruption of the low-affinity leukemia inhibitory factor receptor gene causes placental, skeletal, neural and metabolic defects and results in perinatal death. Development 121, 1283–1299. doi: 10.1242/dev.121.5.1283
Wiltbank, M. C., Baez, G. M., Garcia-Guerra, A., Toledo, M. Z., Monteiro, P. L., Melo, L. F., et al. (2016). Pivotal periods for pregnancy loss during the first trimester of gestation in lactating dairy cows. Theriogenology 86, 239–253. doi: 10.1016/j.theriogenology.2016.04.037
Wooldridge, L. K., and Ealy, A. D. (2019). Interleukin-6 increases inner cell mass numbers in bovine embryos. BMC Dev. Biol. 19:2. doi: 10.1186/s12861-019-0182-z
Wooldridge, L. K., and Ealy, A. D. (2021). Interleukin-6 promotes primitive endoderm development in bovine blastocysts. BMC Dev. Biol. 21:3. doi: 10.1186/s12861-020-00235-z
Wooldridge, L. K., Johnson, S. E., Cockrum, R. R., and Ealy, A. D. (2019). Interleukin-6 requires JAK to stimulate inner cell mass expansion in bovine embryos. Reproduction 158, 303–312. doi: 10.1530/REP-19-0286
Yamanaka, K., Kudo, T., Kimura, N., Amano, T., and Itagaki, Y. (1999). Effect of bovine leukemia inhibitory factor on hatching and numbers of inner cell mass and trophectoderm of bovine intact and biopsied blastocysts. Anim. Sci. J. 70, 444–450. doi: 10.2508/chikusan.70.444
Yamanaka, Y., Lanner, F., and Rossant, J. (2010). FGF signal-dependent segregation of primitive endoderm and epiblast in the mouse blastocyst. Development 137, 715–724. doi: 10.1242/dev.043471
Yang, Q. E., Fields, S. D., Zhang, K., Ozawa, M., Johnson, S. E., and Ealy, A. D. (2011). Fibroblast growth factor 2 promotes primitive endoderm development in bovine blastocyst outgrowths. Biol. Reprod. 85, 946–953. doi: 10.1095/biolreprod.111.093203
Yoshida, K., Taga, T., Saito, M., Suematsu, S., Kumanogoh, A., Tanaka, T., et al. (1996). Targeted disruption of gp130, a common signal transducer for the interleukin 6 family of cytokines, leads to myocardial and hematological disorders. Proc. Natl. Acad. Sci. U.S.A. 93, 407–411. doi: 10.1073/pnas.93.1.407
Keywords: embryo, blastocyst, primitive endoderm, interleukin-6, leukemia inhibitory factor
Citation: Wooldridge LK and Ealy AD (2021) Leukemia Inhibitory Factor Stimulates Primitive Endoderm Expansion in the Bovine Inner Cell Mass. Front. Anim. Sci. 2:796489. doi: 10.3389/fanim.2021.796489
Received: 16 October 2021; Accepted: 03 December 2021;
Published: 20 December 2021.
Edited by:
Erin E. Connor, University of Delaware, United StatesReviewed by:
Bjorn Oback, AgResearch Ltd, New ZealandJoao Henrique Moreira Viana, Brazilian Agricultural Research Corporation (EMBRAPA), Brazil
Copyright © 2021 Wooldridge and Ealy. This is an open-access article distributed under the terms of the Creative Commons Attribution License (CC BY). The use, distribution or reproduction in other forums is permitted, provided the original author(s) and the copyright owner(s) are credited and that the original publication in this journal is cited, in accordance with accepted academic practice. No use, distribution or reproduction is permitted which does not comply with these terms.
*Correspondence: Alan D. Ealy, ZWFseUB2dC5lZHU=