- Department of Animal Science, Institute of Agriculture, University of Tennessee, Knoxville, TN, United States
Introduction: High quality semen is essential for reproductive efficiency. Nutrition and environmental factors impact the ejaculate components, like cytokines, that are essential for pregnancy establishment. We hypothesized that differing nutritional periods and body condition scores would affect seminal plasma cytokine concentrations of bulls.
Methods: Mature Angus bulls (n=11) were individually housed and randomly assigned to either over-fed (n=5) or restricted (n=6) treatment pathways. Bulls were fed different volumes of a single ration creating 8 individual treatment periods. Body weight and body condition scores were taken every 14 d to manage intake volumes. Ejaculates were collected every 84 d to determine seminal plasma cytokine profiles. A complete randomized design was used to evaluate seminal plasma cytokines after each nutritional treatment. Initial cytokine concentrations and volume of the ejaculate were included as covariates.
Results: All cytokines returned to initial concentrations following maintenance treatments at an ideal body condition score of 6. Nutritional treatments affected (P ≤ 0.05) IFN-γ, IL-8, MIP-1α, MIP-1β, TNF-α, IL-1β, and VEGF-A. However, TNF-α, IFN-γ, and MIP-1α had the greatest impact on cytokine profile.
Discussion: Nutritional levels and adiposity altered seminal plasma cytokine concentrations which could potentially impact the inflammatory balance of the uterus and the immune responses necessary for pregnancy establishment.
1. Introduction
A cow-calf producer’s primary income is highly dependent on bull fertility since profits are based on the number of calves born each year (Taylor and Field, 1995). Monitoring bull fertility is critical to maximizing reproductive efficiency in beef production (Butler et al., 2020). One infertile bull is more detrimental than one infertile cow due to the number of offspring that bulls produce in a given breeding season (Kastelic, 2013). Bull management for maximizing fertility includes proper nutrition to produce bulls that fit industry demands. However, bull fertility and reproductive success can become limiting when issues such as inadequate nutrition, injuries, or infections are present (Kastelic, 2013). Correct nutritional management of bulls is critical due to sires experiencing variable periods of nutrition throughout the year. Therefore, understanding the complete impacts of nutrition on seminal plasma (SP) and male fertility could help increase reproductive efficiency.
Seminal plasma is the non-cellular portion of the ejaculate composed of cytokines, amino acids, enzymes, hormones, ions, sugars, lipids, antioxidants, and proteins that act as a nutritional, protection, and transport media for spermatozoa as it travels through the female reproductive tract (Juyena and Stelletta, 2012). Moreover, SP moieties can be impacted by many factors including nutrition (Binder et al., 2015; Watkins et al., 2018; Schjenken et al., 2021). Restricted diets can decrease the amount of SP volume produced in each ejaculate (Singh et al., 2018), while overfeeding increases the production of insulin-like growth factor 1, potentially influencing sperm production and libido of rams (Fourie et al., 2004; Selvaraju et al., 2012). Pro-inflammatory cytokines are also elevated when high energy diets are fed (Eckel and Ametaj, 2016). Therefore, nutritional levels, both over and under appropriate nutrition, will influence SP components and potentially reproductive efficiency.
Cytokines are a diverse group of signaling proteins involved in a multitude of immunological functions (Chen et al., 2018). Cytokines are known to be pleiotropic and synergistic, acting in cascading pathways to create a strong biological effect in a given tissue (Kany et al., 2019). Recently, SP was found to target female tissues in humans, mice, and cattle to elicit an immunological response needed for pregnancy establishment (Robertson et al., 2009; Bromfield, 2014). Specifically, cytokine signaling activates structural modifications in female tissues through the recruitment of leukocytes, macrophages, dendritic cells (Robertson et al., 1997), and regulatory T cells (Tsuda et al., 2019). The cytokines within SP cause cascading signals in the endometrium to promote the inflammatory immune response that will in turn facilitate pregnancy establishment, embryo tolerance, and fetal development in many species including in humans, mice, and cattle (Tremellen et al., 1998; Robertson, 2005). Furthermore, complex cytokine networks have important roles in a wide range of reproductive processes such as maternal-fetal interaction by interferon-τ, uterine expansion by Interleukin (IL)-1β and IL-8 (Orsi and Tribe, 2008), and cervical remodeling by IL-10 to prepare for parturition (Van Engelen et al., 2009). At copulation, SP stimulates the release of IL-10 in the female reproductive tract, potentially for the immunological balance needed to avoid rejection of the spermatozoa and subsequent embryo (Denison et al., 1999). The balance of pro- and anti-inflammatory cytokines is crucial for the survival of the spermatozoa in the female tract and the tolerance of the fetus. Since nutrition may impact the overall inflammatory state of SP and immune responses within the female after intercourse, it is crucial to understand how different diets affect cytokines within the SP. Therefore, the hypothesis of the current study is that differing levels of nutrition and body condition score (BCS) will alter cytokine concentrations within the SP of beef bulls.
2. Materials and methods
All experimental procedures were approved by the University of Tennessee Institutional Animal Care and Use Committee.
2.1. Experimental design and sample collection
The experimental design and sample collection (Figure 1) was previously reported in detail (Harrison et al., 2022). Briefly, mature Angus bulls (n = 11; Body Weight [BW] = 738 kg; BCS = 6; Age = 4 years) were purchased from a single farm in South Dakota, individually housed (2.44-m by 12.19-m paddock), and provided ad-libitum water, mineral supplement (CO-OP Supreme Cattle Mineral; Tennessee Farmers Cooperative; Lavergne, TN), and a single ration at varying levels to achieve targeted BW and BCS goals. The diet consisted of 35% grass hay, 35% cracked corn, 20% dried distillers grains, and 10% soybean meal resulting in a diet that was 80.3% TDN, 1.92 Mcal/kg NEm, 1.28 Mcal/kg NEg, and 19% crude protein. Prior to treatment assignment, all bulls were required to pass a breeding soundness exam (BSE). Pre-treatment average scrotal circumference was 38.52 ± 1.45 cm (34 cm minimum), forward progressive motility averaged 45.8 ± 7.3% (30% minimum), morphological defects were 19.9 ± 5.9%, 5.9 ± 3.9%, 0.64 ± 1.02%, and 26.5 ± 6.1% for head, mid piece, tail, and total (70% minimum), respectively (Harrison et al., 2022). The experimental design consisted of two nutritional pathways (over-fed [n = 5] and restricted [n = 6]) and four nutritional planes (gain, loss, maintenance at a suboptimal BCS, and maintenance at the ideal BCS) to achieve eight individual treatments. The treatments included: gain to obesity (GO), maintenance at an obese BCS (BCS = 8; MO), loss after obesity (LO), maintenance at ideal adiposity (BCS = 6) after obesity (IMO), loss from nutrient restriction (LR), maintenance at a low BCS (BCS = 4; MR), gain after nutrient restriction (GR), and maintenance at ideal adiposity after nutrient restriction (IMR). Individual non-shrunk BW and BCS (1 = emaciated and 9 = obese, (Wagner et al., 1988)) was taken every 14 d to monitor changes within bulls. Semen was collected every 84 d for cytokine concentrations. The ejaculate was centrifuged in the lab for 5 min at 2500 × G to pellet spermatozoa at the bottom of the tube. The SP was then aliquoted into 2 mL microcentrifuge tubes and stored at -80°C until cytokine analyses could be conducted.
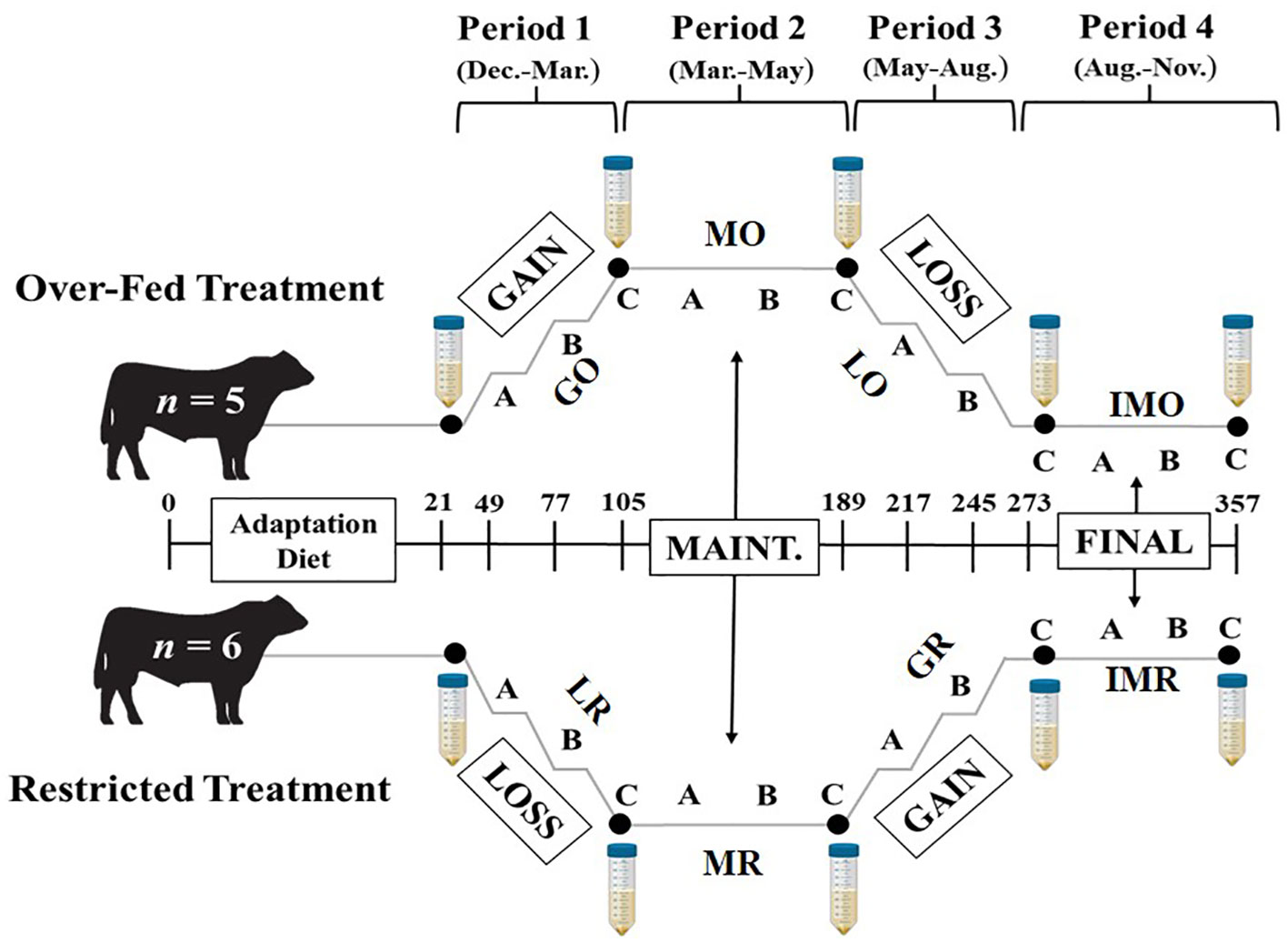
Figure 1 Project timeline with two nutrition pathways: Over-Fed and Restricted, with four respective nutritional treatments per pathway. Sample collections followed a 21 d diet adaptation period prior to treatments. The treatments include: Gain to Obesity (GO), Maintenance at Obese BCS (MO), Loss after Obesity (LO), Maintenance at Ideal Adiposity after Obesity (IMO), Loss from Nutrient Restriction (LR), Maintenance at Restriction BCS (MR), Gain after Nutrient Restriction (GR), and Maintenance at Ideal Adiposity after Nutrient Restriction (IMR). Each treatment includes semen collection for cytokine concentrations for initial and every 84 d (large falcon tubes).
2.2. Cytokine quantification
Cytokine concentrations of IL-1α, IL-1β, tumor necrosis factor (TNF)-α, interferon (IFN)-γ, IL-4, IL-6, IL-10, IL-17A/cytotoxic T-lymphocyte-associated antigen 8 (CTLA8), IL-36 receptor antagonist (RA)/interleukin 1 family member 5 (IL-1F5), IL-8/C-X-C motif ligand 8 (CXCL-8), monocyte chemoattractant protein (MCP)-1/C–C motif chemokine ligand (CCL)-2, macrophage inflammatory protein (MIP)-1α/CCL-3, MIP-1β/CCL-4, and vascular endothelial growth factor (VEGF)-A were quantified within SP using the MILLIPLEX MAP Bovine Cytokine/Chemokine Magnetic Bead Panel (MilliporeSigma; Burlington, MA) according to the manufacturer protocol. The plates were analyzed on a Luminex 200 instrument (Luminex; Austin, TX) at the University of Tennessee Institute of Agriculture Genomics Hub.
2.3. Statistical analyses
A complete randomized design was implemented in GLIMMIX procedure of SAS 9.4 (SAS Institute; Cary, NC) to determine the effects of nutritional treatments on cytokine concentrations. The experimental unit was individual bull. The fixed effect was treatment (i.e. nutritional period) and the random effect was bull within treatment. Initial cytokine concentrations prior to the onset of treatments as well as the volume of each ejaculate were included as covariates for cytokine analyses but were removed from the model if found to be non-significant (P > 0.05). Normality was assessed by the Shapiro-Wilk statistic > 0.8 and the Kolmogorov-Smirnov test > 0.2. Cytokine concentrations that were not normally distributed were log transformed to achieve normality and were reported as back transformed means and standard errors. Interleukin-4, IL-6, IP-10, and MCP-1 were not able to achieve normality with or without transformation and were removed from further concentration analysis. Means were considered different when P ≤ 0.05 with a tendency at P ≤ 0.10.
For cytokine profile analyses, log-transformed cytokine concentrations were analyzed using MetaboAnalyst 5.0 (Pang et al., 2021) to identify differences in cytokine profiles amongst nutritional treatments. The chemometrics analysis using partial least squares discriminant analysis (PLS-DA) was utilized to evaluate nutritional treatments. Variable importance in projections (VIP), known as a weighted sum of squares of the partial least square loadings, was also performed on cytokines within PLS-DA to determine which cytokines were most influential in the resulting profile.
3. Results
Nutritional plane effects on BW, BCS, and gross fertility (i.e. motility and morphology) measurements have been previously reported (Harrison et al., 2022). Initial cytokine concentrations, from the sample prior to the onset of treatment, was included in the model as a covariate but only remained in the models, due to a significant impact (P ≤ 0.04), on IFN-γ and TNF-α. The volume of the ejaculate, for each bull, was also included as a covariate; however, only found to be impactful (P = 0.04) for the analysis of IFN-γ. All cytokine concentrations returned to values similar to initial concentrations during the IMO and IMR treatments with the exception of MIP-1α and VEGF-A. Nutritional treatments influenced (P < 0.05) IFN-γ, IL-8, MIP-1α, MIP-1β, TNF-α, IL-1β and VEGF-A. Interferon-γ concentrations were influenced by nutritional treatments (P < 0.01), with concentrations decreasing the greatest amount during the IMO treatment with concentrations similar to the IMR treatment. Both IMO and IMR concentrations were similar to concentrations prior to the onset of treatments. The greatest concentration occurred during the MO treatment when bulls on the over-fed pathway were maintained at a BCS of 8 (Figure 2A). Nutritional treatments also affected (P < 0.0001) IL-8 (Figure 2B) and MIP-1α (P < 0.0001; Figure 2C) similarly with the IMO and IMR treatments having the greatest concentrations and the lowest concentrations during the GR treatment. However, IL-8 concentrations returned to levels similar to initial cytokine concentrations during the IMO and IMR treatments. Conversely, MIP-1α did not return to concentrations observed at the onset of treatments. Concentrations of MIP-1β were greatest (P < 0.01) during the IMR treatment with similar concentrations for the IMO treatment, and both treatments not different from concentrations prior to the onset of treatments. The most reduced concentrations for MIP-1β occurred during the GR and LO treatments which is the dietary restriction period of both pathways (Figure 2D). The pro-inflammatory cytokines, TNF-α (P < 0.0001; Figure 2E) and IL-1β (P = 0.05; Figure 2F), followed similar trends with the greatest concentrations occurring during the IMO and IMR treatments, of which both were not different from initial concentrations. Tumor necrosis factor-α decreased in concentration but was not different from the remaining treatments. However, IL-1β had the lowest concentrations during the LO, MR and GR treatments. The angiogenic cytokine, VEGF-A, was influenced by nutritional treatments (P ≤ 0.02) and had the greatest increase in concentration during the IMO and MO treatments when bulls on the over-fed pathway were on diets which supported maintenance of BW and BCS. Similar concentrations did occur during the IMR and LO treatments; however, VEGF-A did not return to levels observed prior to the onset of nutritional treatments. The greatest decrease in VEGF-A concentrations occurred during the LR treatment which was similar to concentrations during the GO treatment (Figure 2G).
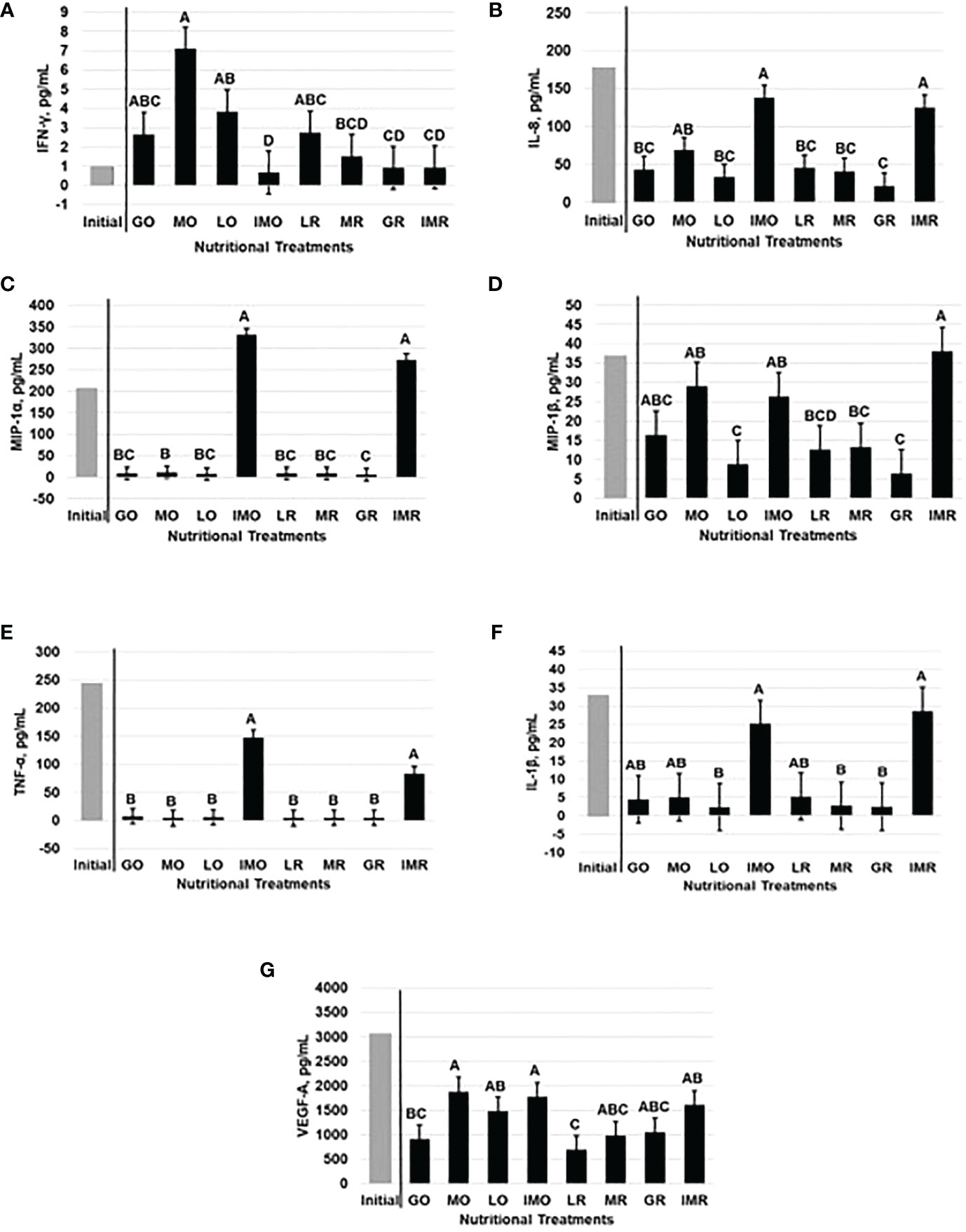
Figure 2 Seminal plasma concentrations (n=11; Least Square Mean ± SEM) of IFN-γ (A), IL-8 (B), MIP-1α (C), and MIP-1β (D) TNF-α (E), IL-1β (F), and VEGF-A (G) were affected by nutritional treatments. ABCDBars that do not share a letter denotes differences at P ≤ 0.05.
To establish cytokine profiles for each nutritional treatment, a PLS-DA was created within MetaboAnalyst 5.0. Nutritional treatments appeared to be impactful on SP cytokine profiles with overlap trends occurring between some groups (Figure 3A). Specifically, the IMO, IMR and initial nutritional treatments which were similar via overlapping ellipses but completely separate ellipses from the GR, LO, LR, MO and MR treatments. The only profile that stretched over both of the groups was the SP profile for the GO treatment. Variable importance in the projection scores were generated to determine the cytokine levels of contribution to these profiles during each nutritional period. All of the cytokines were included within the PLS-DA but the cytokines with < 0.5 VIP score included: IL-6, MCP-1, VEGF-A, IL-4, IL-8, MIP-1β and IL-10. These cytokines were deemed relatively non-impactful to the overall profile of the SP (Figure 3B). Cytokines identified as moderately impactful (VIP score = > 0.5 and < 1.0) included: IP-10, IL-17α, and IL-1α (Figure 3B) and cytokines, (TNF-α, IFN-γ, MIP-1α, IL-1β and IL-36RA) with a VIP scores > 1, were highly influential on the cytokine profiles of each nutritional period (Figure 3B). Tumor necrosis factor-α had the greatest impact (VIP score > 2) on the SP profile among all nutritional treatments and was greatest during the initial period prior to treatments and the lowest during the MO treatment (Figure 3B). In contrast, IFN-γ (VIP score > 1.5; Figure 3B) had the greatest impact during the MR treatment and the lowest during the IMO treatment. Macrophage inflammatory protein-1α (VIP score > 1.5; Figure 3B) had the most influence during the IMO treatment and the lowest impact during the GR treatment. The cytokines, IL-1β and IL-36RA, followed a similar prevalence pattern with greatest impacts (VIP score = 1.5) during the initial period and the least during the LO treatment for IL-1β and the MR treatment for IL-36RA; however, IL-1β had a greater overall impact (Figure 3B).
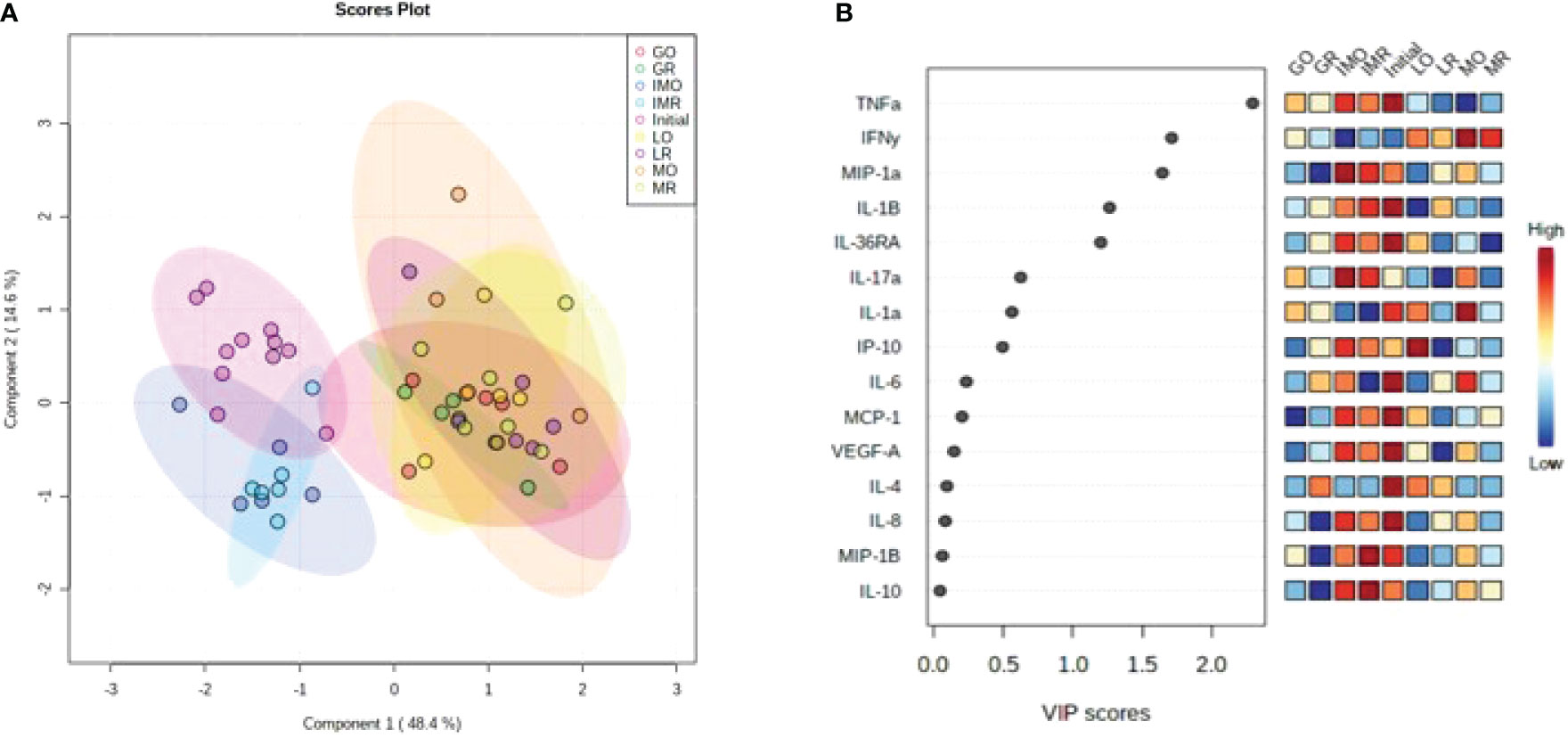
Figure 3 Analysis of cytokine profiles based on nutritional treaments: (A) Partial least squares discriminant analysis visualizing differences in inflammatory cytokines during differing nutritional treatments and (B) Variable importance in the projection for individual cytokines plot. A single ellipse represents a 95% confidence interval within that nutritional treatment.
4. Discussion
Sire management, through the control of nutrition and other environmental factors, is imperative to ensure optimal fertility and advance overall herd genetics. Nutritional effects have been reported to impact the ejaculate in boars, rams, and bulls (Brown, 1994). Nutrition can also play a role in influencing the components of SP, specifically cytokines (Eckel and Ametaj, 2016). Cytokines rarely act individually, more so as a network of highly influential, cascading protein molecules to control local and systemic inflammation (Dinarello, 1989). Many cytokines and chemokines have been reported to be altered by seminal plasma in the endometrium of cows (Ibrahim et al., 2019) including IL-8 and IL-1β. The current study found IL-8, IL-1β, IFN-γ, MIP-1α, MIP-1β, TNF-α, and VEGF-A, to be impacted by nutritional levels and may be indicative of a role in reproductive success.
Interferon-γ is known as a pleiotropic cytokine that can have both pro- and anti-inflammatory effects, and has been found in the uterus during early pregnancy (Murphy et al., 2009). Within the maternal endometrium, IFN-γ is abundantly produced by uterine natural killer cells as well as trophoblasts to initiate endometrial vasculature remodeling, angiogenesis, and the maintenance of the placenta in mice (Murphy et al., 2009). Interestingly, IFN-γ in SP has been linked to female infertility in humans outside of sperm and male parameters (Robertson et al., 2003). Concentrations of IFN-γ in the current dataset were greatest during periods of nutritional perturbation. This may be indicative of the nutritional stress influencing IFN-γ concentrations in bovine SP which could impact the ability to produce viable embryos and establish a pregnancy. In support of these results, the immune-regulatory concentrations of IFN-γ in humans have generally been detected at low levels within SP; however, was substantially elevated when a disease or infection was present (Leutscher et al., 2005; Vanpouille et al., 2016). Indicating that fluctuations in cytokine concentrations occur in response to external and internal factors as the body attempts to return back to homeostasis throughout pregnancy.
Tumor necrosis factor-α is an acute phase cytokine responsible for mediating acute inflammatory reactions to diseases and infection sites (Burger and Dayer, 2002). The pro-inflammatory properties of TNF-α are known to hinder sperm motility and functional capacity by increasing chemotactic activity and inducing the immuno-cascade effects of neutrophils (Hill et al., 1989). In contrast, TNF-α has been found to be essential for early pregnancy establishment (Toder et al., 2003). Yet the overproduction of TNF-α may cause early embryonic loss or implantation failure in humans (Saito et al., 2010; Alijotas-Reig et al., 2017). Bovine TNF-α also incites inflammation by promoting neutrophil recruitment when induced by lipopolysaccharides (Sohn et al., 2007). Interleukin-1β has similar effects on semen quality and establishment of pregnancy as TNF-α (Gruschwitz et al., 1996). Interluekin-1β is also responsible for activating the innate immune response by mediating recruitment and activation of macrophages and neutrophils (Ott et al., 2007). The highly correlated pro-inflammatory cytokines, TNF-α and IL-1β (Eggert-Kruse et al., 2007), had similar cytokine concentration trends in the current study, but were also found to be impactful on overall cytokine profiles during each nutritional period. The current study identified TNF-α to have the greatest impact (VIP score) on cytokine profiles within SP during different nutritional periods. These closely associated and impactful pro-inflammatory cytokines are potentially needed and, thus, play important roles in pregnancy establishment.
Chemokines are a family of small cytokines, mostly known for their role in chemotaxis (Hughes and Nibbs, 2018), inflammation, immune surveillance, and angiogenesis (Dimberg, 2010). Many chemokines are known to be pro-inflammatory, while others are thought to control cell migration for normal tissue growth and maintenance (Hughes and Nibbs, 2018). Interleukin-8 is a potent chemokine involved in leukocyte migration and cell activation for events associated with inflammation (Nederlof et al., 2017). Interleukin-8 decreased substantially during the GR treatment in comparison to the greatest concentration following the IMO and IMR treatments. In humans, IL-8 concentrations have been reported to be present in high concentrations within SP of healthy fertile men (Politch et al., 2007), and, thus, may indicate excessive BW gain in bulls is detrimental to fertility. In contrast, IL-8 has also been known to dramatically increase in response to bacterial and viral infections which may negatively affect spermatozoa resulting in poor sperm motility within the ejaculate (Eggert-Kruse et al., 2001). Therefore, a certain level of this immuno-stimulatory chemokine from nutritional stress could result in potential pregnancy failures and diminished herd outcomes.
The pro-inflammatory chemokines, MIP-1α and MIP-1β, are known as chemoattractants of monocytes and macrophages which contribute to the regulation of uterine macrophage populations of mice (Robertson et al., 1998). However, MIP-1α and MIP-1β have not been extensively researched in cattle. The current study identified MIP-1α to have an influential impact (VIP score) on the ejaculate during different nutritional periods; however, MIP-1β was deemed relatively non-impactful on the cytokine profiles within SP. Previous research has reported increases in MIP-1α and MIP-1β concentrations are indicators of infection or disease (Garzino-Demo et al., 1999; O'Grady et al., 1999; Chaisavaneeyakorn et al., 2003). Macrophage inflammatory protein-1α demonstrated significant concentrations within decidual tissue increases within humans who underwent abortions (Freis et al., 2018) and overall pregnancy losses within the first 10 weeks of gestation (Park et al., 2010). On the male side, humans with HIV expressed lower concentrations of MIP-1α and MIP-1β released by cytotoxic T cells (Cocchi et al., 2000). Thus, concentrations of MIP-1α and MIP-1β may increase acutely but long-term stress from diseases, like HIV (Cocchi et al., 2000), or nutritional stress (current dataset) results in lower concentrations altering the impacts of these chemokines on physiological functions. The increase of the pro-inflammatory chemokines MIP-1α and MIP-1β within SP may be detrimental to pregnancy outcomes due to early embryo loss.
Immuno-suppressive cytokines, IL-10 and IL-36RA, play important roles in inhibiting the synthesis of pro-inflammatory cytokines (Zhang and An, 2007). More specifically to reproduction, anti-inflammatory cytokines create the overall immuno-suppressive state of the mucosal environment to aid in sperm survival within the oviduct (Torres-Poveda et al., 2014). In humans, concentrations of IL-10 were greater in healthy male individuals demonstrating the natural immuno-tolerant environment for sperm of the female reproductive tract (Camejo, 2003). The immuno-suppressive functions of IL-10 also prevent the rejection of the semi-allogenic fetus (Chatterjee et al., 2014) and promote conceptus attachment to the uterine endometrium in dairy and beef cattle (Odhiambo et al., 2009). Our study demonstrated IL-10 to be non-influential to the overall ejaculate and the concentrations were not influenced by differing periods of nutritional stress. This potentially indicates that the desired immuno-tolerant levels for sperm survival can be maintained even under nutritional stress. The antagonistic effects of IL-36RA, a member of the IL-1 super family, inhibits inflammation by inhibiting IL-36 from binding to IL-36R (Yi et al., 2016). The antagonistic effects from IL-36RA impede signals between the toll/interleukin-1 receptor domains which inhibits NF-κB signaling cascade (Murrieta-Coxca et al., 2019). The roles of the IL-36 cytokine family remain mostly unknown in pregnancy; however, the immuno-suppressive effects of IL-36RA could promote a balanced uterine environment for successful pregnancy establishment. Our findings quantified IL-36RA to be influential on the cytokine profiles within SP under different nutritional periods; however, concentrations of IL-36RA were found to not be influenced by the nutritional treatments. This may demonstrate that IL-36RA has a more important role in creating the immuno-suppressive environment compared to IL-10 to increase sperm survival and to promote embryo establishment.
Angiogenic cytokines can induce endothelial cell activation and proliferation for the creation of new blood vessels from precursor cells (Ucuzian et al., 2010). Proper function of VEGF-A is critical during every step of placental growth and vascular formation to provide enough blood and nutrients to the growing fetus (Chen and Zheng, 2014). Thus, VEGF-A being present in the greatest concentrations within SP is not entirely surprising. Furthermore, research has demonstrated a decrease of VEGF-A in the SP of obese humans causing a negative effect on semen quality (Zhu et al., 2021). Our study agrees with these results since VEGF-A had one of the lowest VIP impacts of all the cytokines presented, more specifically during the LR and GO treatment. Angiogenic cytokine VEGF-A did not play a significant role in any of the cytokine profiles yet had the greatest overall concentrations of all cytokines evaluated. The drastically higher concentrations of VEGF-A in SP may indicate a role in angiogenesis in the developing fetus, causing the overall impact of this cytokine to be low. These concentrations decreased during the LR and GO treatments when bulls deviated from their initial basal BCS, demonstrating the potential decrease in semen quality which may be a cause of pregnancy failures.
The focus of the current study was to elucidate the impacts of nutrition. However, the authors admit that it is possible that some of the results could have been, in part, influenced by photoperiod. Most mammalian immune and endocrine function have been reported to be impacted by photoperiod to varying extents (Reiter, 1983; Dahl et al., 2002). The current study did take place over the course of an entire year and, thus, the bulls were exposed to different photoperiods. Scrotal circumference and spermatogenesis measurements were altered by nutrition but not impacted by time of year in which the sample was taken (Harrison et al., 2022). The cytokines quantified in the current dataset, MIP-1α, IL-1β, IL-8, and TNF-α, that were impacted by nutritional treatment responded, in varying extents, to nutritional perturbations similarly compared with ideal nutrition and BCS. The exceptions to this were the changes observed in IFN-γ, MIP-1β, and VEGF-A concentrations but these changes also did not correlate with seasonal changes in photoperiod. Therefore, photoperiod does not appear to impact cytokines in seminal plasma but more direct research studies would need to be conducted to completely elucidate the photoperiodic effect.
In conclusion, cytokines and chemokines fluctuated between the nutritional periods, potentially in response to nutritional stress. The cytokines, TNF-α, IFN-γ, and MIP-1α had the greatest impact on the overall profile of SP during nutritional periods. Moreover, this dataset demonstrated a similarity between cytokine profiles when the animal gains or loses BW or is maintained at an abnormal BCS. The cytokines: IFN-γ, MIP-1β, IL-1β, IL-8, and TNF-α, during the IMO and IMR treatments, returned to the initial concentrations and profiles. Therefore, varying nutritional levels can influence the immunological substrates within SP of mature bulls which could potentially affect the sire’s ability to successfully establish pregnancy. However, these changes are not permanent and will return under correct nutritional management. Further studies are still required to fully understand the long-term impacts of nutrition on SP, the uterine responses to differing cytokine profiles, and how these cytokines influence the establishment of pregnancy all of which could alter reproductive efficiency and success.
Data availability statement
The raw data supporting the conclusions of this article will be made available by the authors, without undue reservation.
Ethics statement
The animal study was reviewed and approved by University of Tennessee Animal Care and Use Committee 2713-0819.
Author contributions
TH conducted the study, collected all samples, analyzed the data, and was the primary author for the manuscript. EC, KB, and TA-S assisted in sample collection, data analyses, and provided editorial comments to the manuscript. RP assisted in cytokine analysis and provided editorial comments to the manuscript. LGSc aided in study design, statistical analyses, and provided editorial comments. LGSt, and FS provided study guidance and editorial comments. KM designed and supervised the study and data management as well as provided editorial comments to the manuscript. All authors reviewed and approved the final version of the manuscript.
Funding
This work was funded by the University of Tennessee Institute of Agriculture, University of Tennessee Department of Animal Science, and the United States Department of Agriculture, National Institute of Food and Agriculture, Capacity Grant No. 1019048.
Acknowledgments
The authors would like to thank Hugh Moorehead, Matt Backus, Kevin Thompson and the Middle Tennessee Research and Education Center of Lewisburg for help in animal care and collecting samples, and Sujata Agarwal and the Genomics Hub for her help in performing cytokine analysis. Additionally, the authors would like to thank the state of Tennessee through UT AgResearch, the Department of Animal Science for providing funding and support for this research.
Conflict of interest
The authors declare that the research was conducted in the absence of any commercial or financial relationships that could be construed as a potential conflict of interest.
Publisher’s note
All claims expressed in this article are solely those of the authors and do not necessarily represent those of their affiliated organizations, or those of the publisher, the editors and the reviewers. Any product that may be evaluated in this article, or claim that may be made by its manufacturer, is not guaranteed or endorsed by the publisher.
References
Alijotas-Reig J., Esteve-Valverde E., Ferrer-Oliveras R., Llurba E., Gris J. M. (2017). Tumor necrosis factor-alpha and pregnancy: focus on biologics. Clin. Rev. Allerg Immu 53 (1), 40–53. doi: 10.1007/s12016-016-8596-x
Binder N. K., Sheedy J. R., Hannan N. J., Gardner D. K. (2015). Male obesity is associated with changed spermatozoa Cox4il mRNA level and altered seminal vesicle fluid composition in a mouse model. Mol. Hum. Reprod. 21, 424–434. doi: 10.1093/molehr/gav010
Bromfield J. J. (2014). Seminal fluid and reproduction: much more than previously thought. J. Assist. Reprod. Genet. 31 (6), 627–636. doi: 10.1007/s10815-014-0243-y
Brown B. (1994). A review of nutritional influences on reproduction in boars, bulls and rams. Reprod. Nutr. Dev. 34 (2), 89–114. doi: 10.1051/rnd:19940201
Burger D., Dayer J. M. (2002). Cytokines, acute-phase proteins, and hormones: IL-1 and TNF-alpha production in contact-mediated activation of monocytes by T lymphocytes. Ann. NY Acad. Sci. 966, 464–473. doi: 10.1111/j.1749-6632.2002.tb04248.x
Butler M. L., Bormann J. M., Weaber R. L., Grieger D. M., Rolf M. M. (2020). Selection for bull fertility: A review. Transl. Anim. Sci. 4 (1), 423–441. doi: 10.1093/tas/txz174
Camejo M. I. (2003). Relation between immunosuppressive PGE(2) and IL-10 to pro-inflammatory IL-6 in seminal plasma of infertile and fertile men. Arch. Androl 49 (2), 111–116. doi: 10.1080/01485010390129232
Chaisavaneeyakorn S., Moore J. M., Mirel L., Othoro C., Otieno J., Chaiyaroj S. C., et al. (2003). Levels of macrophage inflammatory protein 1 alpha (MIP-1 alpha) and MIP-1 beta in intervillous blood plasma samples from women with placental malaria and human immunodeficiency virus infection. Clin. Diagn. Lab. Immunol. 10 (4), 631–636. doi: 10.1128/cdli.10.4.631-636.2003
Chatterjee P., Chiasson V. L., Bounds K. R., Mitchell B. M. (2014). Regulation of the anti-inflammatory cytokines interleukin-4 and interleukin-10 during pregnancy. Front. Immunol. 5, 253. doi: 10.3389/fimmu.2014.00253
Chen L., Deng H., Cui H., Fang J., Zuo Z., Deng J., et al. (2018). Inflammatory responses and inflammation-associated diseases in organs. Oncotarget 9 (6), 7204–7218. doi: 10.18632/oncotarget.23208
Chen D. B., Zheng J. (2014). Regulation of placental angiogenesis. Microcirculation 21 (1), 15–25. doi: 10.1111/micc.12093
Cocchi F., DeVico A. L., Yarchoan R., Redfield R., Cleghorn F., Blattner W. A., et al. (2000). Higher macrophage inflammatory protein (MIP)-1α and MIP-1β levels from CD8+ T cells are associated with asymptomatic HIV-1 infection. Proc. Natl. Acad. Sci. U.S.A. 97 (25), 13812–13817. doi: 10.1073/pnas.240469997
Dahl G. E., Auchtung T. L., Kendall P. E. (2002). Photoperiodic effects on endocrine and immune function in cattle. Reproduction. Supplement 59, 191–201.
Denison F. C., Grant V. E., Calder A. A., Kelly R. W. (1999). Seminal plasma components stimulate interleukin-8 and interleukin-10 release. Mol. Hum. Reprod. 5 (3), 220–226. doi: 10.1093/molehr/5.3.220
Dimberg A. (2010). Chemokines in angiogenesis. Curr. Top. Microbiol. Immunol. 341, 59–80. doi: 10.1007/82_2010_21
Dinarello C. A. (1989). Interleukin-1 and its biologically related cytokines. Adv. Immunol. 44, 153–205. doi: 10.1016/S0065-2776(08)60642-2
Eckel E. F., Ametaj B. N. (2016). Invited review: Role of bacterial endotoxins in the etiopathogenesis of periparturient diseases of transition dairy cows. J. Dairy Sci. 99 (8), 5967–5990. doi: 10.3168/jds.2015-10727
Eggert-Kruse W., Boit R., Rohr G., Aufenanger J., Hund M., Strowitzki T. (2001). Relationship of seminal plasma interleukin (IL)-8 and IL-6 with semen quality. Hum. Reprod. 16 (3), 517–528. doi: 10.1093/humrep/16.3.517
Eggert-Kruse W., Kiefer I., Beck C., Demirakca T., Strowitzki T. (2007). Role for tumor necrosis factor alpha (TNF-alpha) and interleukin 1-beta (IL-1beta) determination in seminal plasma during infertility investigation. Fertil. Steril. 87 (4), 810–823. doi: 10.1016/j.fertnstert.2006.08.103
Fourie P., Schwalbach L., Neser F., van der Westhuizen C. (2004). Scrotal, testicular and semen characteristics of young dorper rams managed under intensive and extensive conditions. Small Rumin. Res. 54 (1-2), 53–59. doi: 10.1016/j.smallrumres.2003.10.011
Freis A., Schlegel J., Daniel V., Jauckus J., Strowitzki T., Germeyer A. (2018). Cytokines in relation to hCG are significantly altered in asymptomatic women with miscarriage - a pilot study. Reprod. Biol. Endocrinol. 16 (1), 93. doi: 10.1186/s12958-018-0411-5
Garzino-Demo A., Moss R. B., Margolick J. B., Cleghorn F., Sill A., Blattner W. A., et al. (1999). Spontaneous and antigen-induced production of HIV-inhibitory β-chemokines are associated with AIDS-free status. Proc. Natl. Acad. Sci. U.S.A. 96 (21), 11986–11991. doi: 10.1073/pnas.96.21.11986
Gruschwitz M. S., Brezinschek R., Brezinschek H. P. (1996). Cytokine levels in the seminal plasma of infertile males. J. Androl 17 (2), 158–163. doi: 10.1002/j.1939-4640.1996.tb01765.x
Harrison T. D., Chaney E. M., Brandt K. J., Ault-Seay T. B., Schneider L. G., Strickland L. G., et al. (2022). The effects of differeing nutritional levels and body condition score on scrotal circumference, motility, and morphology of bovine sperm. Trans. Anim. Sci. 6, 1–7. doi: 10.1093/tas/txac001
Hill J. A., Cohen J., Anderson D. J. (1989). The effects of lymphokines and monokines on human sperm fertilizing ability in the zona-free hamster egg penetration test. Am. J. Obstet Gynecol. 160 (5), 1154–1159. doi: 10.1016/0002-9378(89)90179-8
Hughes C. E., Nibbs R. J. B. (2018). A guide to chemokines and their receptors. FEBS J. 285 (16), 2944–2971. doi: 10.1111/febs.14466
Ibrahim L. A., Rizo J. A., Fontes P. L. P., Lamb G. C., Bromfield J. J. (2019). Seminal plasma modulates expression of endometrial inflammatory meditators in the bovine. Biol. Reprod. 100, 660–671. doi: 10.1093/violre/loy226
Juyena N. S., Stelletta C. (2012). Seminal plasma: An essential attribute to spermatozoa. J. Androl 33 (4), 536–551. doi: 10.2164/jandrol.110.012583
Kany S., Vollrath J. T., Relja B. (2019). Cytokines in inflammatory disease. Int. J. Mol. Sci. 20 (23), 6008. doi: 10.3390/ijms20236008
Kastelic J. (2013). Male Involvement in fertility and factors affecting semen quality in bulls. Anim. Front. 3, 20–25. doi: 10.2527/af.2013-0029
Leutscher P. D., Pedersen M., Raharisolo C., Jensen J. S., Hoffmann S., Lisse I., et al. (2005). Increased prevalence of leukocytes and elevated cytokine levels in semen from schistosoma haematobium–infected individuals. J. Infect. Dis. 191 (10), 1639–1647. doi: 10.1086/429334
Murphy S. P., Tayade C., Ashkar A. A., Hatta K., Zhang J., Croy B. A. (2009). Interferon gamma in successful pregnancies. Biol. Reprod. 80 (5), 848–859. doi: 10.1095/biolreprod.108.073353
Murrieta-Coxca J. M., Rodríguez-Martínez S., Cancino-Diaz M. E., Markert U. R., Favaro R. R., Morales-Prieto D. M. (2019). IL-36 cytokines: regulators of inflammatory responses and their emerging role in immunology of reproduction. Int. J. Mol. Sci. 20 (7), 1649. doi: 10.3390/ijms20071649
Nederlof I., Meuleman T., van der Hoorn M. L. P., Claas F. H. J., Eikmans M. (2017). The seed to success: The role of seminal plasma in pregnancy. J. Reprod. Immunol. 123, 24–28. doi: 10.1016/j.jri.2017.08.008
O'Grady N. P., Tropea M., Preas 2. H.L., Reda D., Vandivier R. W., Banks S. M., et al. (1999). Detection of macrophage inflammatory protein (MIP)-1alpha and MIP-1beta during experimental endotoxemia and human sepsis. J. Infect. Dis. 179 (1), 136–141. doi: 10.1086/314559
Odhiambo J., Poole D., Hughes L., Dejarnette J., Inskeep E., Dailey R. (2009). Pregnancy outcome in dairy and beef cattle after artificial insemination and treatment with seminal plasma or transforming growth factor beta-1. Theriogenology 72 (4), 566–571. doi: 10.1016/j.theriogenology.2009.04.013
Orsi N. M., Tribe R. M. (2008). Cytokine networks and the regulation of uterine function in pregnancy and parturition. J. Neuroendocrinol 20 (4), 462–469. doi: 10.1111/j.1365-2826.2008.01668.x
Ott L. W., Resing K. A., Sizemore A. W., Heyen J. W., Cocklin R. R., Pedrick N. M., et al. (2007). Tumor necrosis factor-alpha- and interleukin-1-induced cellular responses: coupling proteomic and genomic information. J. Proteome Res. 6 (6), 2176–2185. doi: 10.1021/pr060665l
Pang Z., Chong J., Zhou G., de Lima Morais D. A., Chang L., Barrette M., et al. (2021). MetaboAnalyst 5.0: narrowing the gap between raw spectra and functional insights. Nucleic Acids Res. 49, W388–W396. doi: 10.1093/nar/gkab382
Park D. W., Lee H. J., Park C. W., Hong S. R., Kwak-Kim J., Yang K. M. (2010). Peripheral blood NK cells reflect changes in decidual NK cells in women with recurrent miscarriages. Am. J. Reprod. Immunol. 63 (2), 173–180. doi: 10.1111/j.1600-0897.2009.00777.x
Politch J. A., Tucker L., Bowman F. P., Anderson D. J. (2007). Concentrations and significance of cytokines and other immunologic factors in semen of healthy fertile men. Hum. Reprod. 22 (11), 2928–2935. doi: 10.1093/humrep/dem281
Reiter R. J. (1983). Pineal gland: An intermediary between the environment and the endocrine system. Psychoneuroendocrinology 8 (1), 31–40. doi: 10.1016/0306-4530(83)90039-2
Robertson S. A. (2005). Seminal plasma and male factor signalling in the female reproductive tract. Cell Tissue Res. 322 (1), 43–52. doi: 10.1007/s00441-005-1127-3
Robertson S. A., Allanson M., Mau V. J. (1998). Molecular regulation of uterine leukocyte recruitment during early pregnancy in the mouse. Placenta 19, 101–119. doi: 10.1016/S0143-4004(98)80009-X
Robertson S. A., Guerin L. R., Bromfield J. J., Branson K. M., Ahlström A. C., Care A. S. (2009). Seminal fluid drives expansion of the CD4+ CD25+ T regulatory cell pool and induces tolerance to paternal alloantigens in mice. Biol. Reprod. 80 (5), 1036–1045. doi: 10.1095/biolreprod.108.074658
Robertson S. A., Mau V. J., Hudson S. N., Tremellen K. P. (1997). Cytokine-leukocyte networks and the establishment of pregnancy. Am. J. Reprod. Immunol. 37 (6), 438–442. doi: 10.1111/j.1600-0897.1997.tb00257.x
Robertson S., Sharkey D., Tremellen K., Dekker G. (2003). Elevated interferongamma in seminal plasma from male partners of women with recurrent miscarriage. J. Soc. Gynaecol Invest. 10, 359A.
Saito S., Nakashima A., Shima T., Ito M. (2010). Th1/Th2/Th17 and regulatory T-cell paradigm in pregnancy. Am. J. Reprod. Immunol. 63 (6), 601–610. doi: 10.1111/j.1600-0897.2010.00852.x
Schjenkin J. E., Moldenhauer L. M., Sharkey D. J., Chan H. Y., Shin P. Y., Fullston T., et al. (2021). High-fat diet alters male seminal plasma composition to impair female immune adaptation for pregnancy in mice. Endocrinology. 162, 1–20. doi: 10.1210/endocr/bqab123
Selvaraju S., Sivasubramani T., Raghavendra B. S., Raju P., Rao S. B., Dineshkumar D., et al. (2012). Effect of dietary energy on seminal plasma insulin-like growth factor-I (IGF-I), serum IGF-I and testosterone levels, semen quality and fertility in adult rams. Theriogenology 78 (3), 646–655. doi: 10.1016/j.theriogenology.2012.03.010
Singh A., Rajak S., Kumar P., Kerketta S., Yogi R. (2018). Nutrition and bull fertility: A review. J. Entomol Zool Stud. 6 (6), 635–643.
Sohn E. J., Paape M. J., Connor E. E., Bannerman D. D., Fetterer R. H., Peters R. R. (2007). Bacterial lipopolysaccharide stimulates bovine neutrophil production of TNF-alpha, IL-1beta, IL-12 and IFN-gamma. Vet. Res. 38 (6), 809–818. doi: 10.1051/vetres:2007033
Taylor R., Field T. (1995). Achieving cow/calf profitability through low-cost production. Proc. Range Beef Cow Symposium, 199.
Toder V., Fein A., Carp H., Torchinsky A. (2003). TNF-alpha in pregnancy loss and embryo maldevelopment: a mediator of detrimental stimuli or a protector of the fetoplacental unit? J. Assist. Reprod. Genet. 20 (2), 73–81. doi: 10.1023/A:1021740108284
Torres-Poveda K., Bahena-Román M., Madrid-González C., Burguete-García A. I., Bermúdez-Morales V. H., Peralta-Zaragoza O., et al. (2014). Role of IL-10 and TGF-β1 in local immunosuppression in HPV-associated cervical neoplasia. World J. Clin. Oncol. 5 (4), 753. doi: 10.5306/wjco.v5.i4.753
Tremellen K., Seamark R., Robertson S. (1998). Seminal transforming growth factor-1 stimulates granulocyte- macrophage colony-stimulating factor production and inflammatory cell recruitment in the murine uterus. Biol. Reprod. 58, 1217–1225. doi: 10.1095/biolreprod58.5.1217
Tsuda S., Nakashima A., Shima T., Saito S. (2019). New paradigm in the role of regulatory T cells during pregnancy. Front. Immun. 10. doi: 10.3389/fimmu.2019.00573
Ucuzian A. A., Gassman A. A., East A. T., Greisler H. P. (2010). Molecular mediators of angiogenesis. J. Burn Care Res. 31 (1), 158–175. doi: 10.1097/BCR.0b013e3181c7ed82
Van Engelen E., De Groot M., Breeveld-Dwarkasing V., Everts M., van der Weyden G., Taverne M., et al. (2009). Cervical ripening and parturition in cows are driven by a cascade of pro-inflammatory cytokines. Reprod. Domest Anim. 44 (5), 834–841. doi: 10.1111/j.1439-0531.2008.01096.x
Vanpouille C., Introini A., Morris S. R., Margolis L., Daar E. S., Dube M. P., et al. (2016). Distinct cytokine/chemokine network in semen and blood characterize different stages of HIV infection. AIDS 30 (2), 193. doi: 10.1097/QAD.0000000000000964
Wagner J. J., Lusby K. S., Oltjen J. W., Rakestraw J., Wettemann R. P., Walters L. E. (1988). Carcass composition in mature hereford cows: estimation and effect on daily metabolizable energy requirement during winter. J. Anim. Sci. 66 (3), 603–612. doi: 10.2527/jas1988.663603x
Watikins A. J., Dias I., Tsuro H., Allen D., Emes R. D., Moreton J., et al. (2018). Paternal diet programs offspring health through sperm- and seminal plasma-specific pathways in mice. PNAS. 115, 10064–10069. doi: 10.1073/pnas.1806333115
Yi G., Ybe J. A., Saha S. S., Caviness G., Raymond E., Ganesan R., et al. (2016). Structural and functional attributes of the interleukin-36 receptor. J. Biol. Chem. 291 (32), 16597–16609. doi: 10.1074/jbc.M116.723064
Zhang J. M., An J. (2007). Cytokines, inflammation, and pain. Int. Anesthesiol Clin. 45 (2), 27–37. doi: 10.1097/AIA.0b013e318034194e
Keywords: adiposity, bull, cytokines, nutrition, seminal plasma
Citation: Harrison TD, Chaney EM, Brandt KJ, Ault-Seay TB, Payton RR, Schneider LG, Strickland LG, Schrick FN and McLean KJ (2023) The effects of nutritional level and body condition score on cytokines in seminal plasma of beef bulls. Front. Anim. Sci. 3:1078960. doi: 10.3389/fanim.2022.1078960
Received: 24 October 2022; Accepted: 12 December 2022;
Published: 04 January 2023.
Edited by:
Mohan Mondal, ICAR-National Dairy Research Institute, Kalyani, IndiaReviewed by:
Seyed Abbas Rafat, University of Tabriz, IranGeorge Perry, Texas A&M AgriLife Research, United States
Copyright © 2023 Harrison, Chaney, Brandt, Ault-Seay, Payton, Schneider, Strickland, Schrick and McLean. This is an open-access article distributed under the terms of the Creative Commons Attribution License (CC BY). The use, distribution or reproduction in other forums is permitted, provided the original author(s) and the copyright owner(s) are credited and that the original publication in this journal is cited, in accordance with accepted academic practice. No use, distribution or reproduction is permitted which does not comply with these terms.
*Correspondence: Kyle J. McLean, a21jbGVhMTBAdXRrLmVkdQ==
†Present address: Kiernan J. Brandt, South Dakota State University, Brookings, SD, United States