Animal Welfare Assessment: Quantifying Differences Among Commercial Medium and Fast Growth Broiler Flocks
- 1Department of Animal Production, NEIKER-Basque Institute for Agricultural Research and Development Basque Research and Technology Alliance, Arkaute, Spain
- 2Instituto de Investigaciones Biológicas y Tecnológicas (IIByT), Facultad de Ciencias Exactas, Físicas y Naturales (FCEFyN), Consejo Nacional de Investigaciones Científicas y Técnicas y Universidad Nacional de Córdoba (CONICET-UNC), Córdoba, Argentina
- 3IKERBASQUE, Basque Foundation for Science, Bilbao, Spain
A combined welfare assessment protocol, including indicators from the Welfare Quality® and AWIN® EU funded projects, was tested on commercial fast and medium growth commercial broiler flocks to determine differences in their assessments as measured with the used of animal welfare indicators. Ten commercial fast (Ross 308, Cobb 500, or a mix of both) and 10 medium growth (Hubbard JA × Ross 308), mixed sex commercial flocks were assessed at 32 and 48 days of age, respectively. Two observers simultaneously collected data on each flock. Observations included transect walks on central and wall areas to assess the AWIN® welfare indicators, bedding quality, environmental parameters and positive behaviors, all of them collected with the i-WatchBroiler app. According to the WQ protocol, welfare assessment indicators including the human avoidance tests, gait score, body weight and hock burns were also measured on each flock. Novel object tests were also carried out. The results of the study show that fast growth flocks had a higher incidence of welfare issues shown by the higher percentage of immobile, lame, sick, featherless, and tail wounded birds. Positive behaviors such as play fighting, wing flapping and running were more frequently observed in medium growth flocks on central locations, while fast growth flocks had a more limited expression of such behaviors. Fast growth flocks also had worse gait scores. Medium growth flocks expressed a different response to behavioral tests depending on the house location, likely attributable to their better mobility and welfare state, and also to the smaller stocking densities at which they were housed, while on the other hand the behavior of fast growth broilers during tests was similar regardless house location, being likely affected by mobility problems and the higher stocking density specific to their management. These results provide quantitative evidences on the differences in animal welfare assessment outcomes in fast and medium growth broilers. Nevertheless, results also suggest that some of the test responses were associated with the physical state and movement ability of the birds and house location that limit their response capacity. Such limitations should be considered when interpreting animal welfare assessment outcomes. These results add to previously published scientific evidences showing the potential of the method and app technology for practical on-farm broiler welfare assessment, including positive indicators, with farmers, technical personnel, certification bodies or scientist as potential end-users.
Introduction
Animal welfare considerations have raised awareness and concerns about livestock production conditions among consumers and the society at large (Alonso et al., 2020). This has been a main broiler industry driver toward a (re)transition to more welfare-oriented production systems while still able to maintain profitability. Broiler production has traditionally based system profitability on a gradual reduction in the length of production cycles to reach market body weight and on increased feed efficiency (Havenstein et al., 2003), relying on an intensive genetic selection for these two traits (McKay, 2009). While this has brought unarguable benefits for human population worldwide through making high quality protein available at reasonable prices (Tavárez and Solis de los Santos, 2016), collateral consequences for broilers have also emerged, evidenced specially by the gradual degradation of their physical condition, health, and overall animal welfare (Rauw et al., 1998). Faster growth rates have resulted in a higher prevalence of leg problems and reduced mobility (Kestin et al., 2001), which are very difficult (if not impossible) to compensate by parallel improvements of chicken housing and management conditions.
It has been suggested that valid animal welfare assessment should be founded on animal-based measures or indicators (Main et al., 2007). A wide collection of animal-based indicators is available for broilers, many of which are already integrated within different welfare assessment protocols such as the Welfare Quality®, and in welfare auditing schemes such as Welfair™ (www.animalwelfair.com/en/). Nevertheless, interpretation of welfare indicators needs caution, particularly if we consider that broilers may have problems in effectively expressing their motivations. In fact, broilers' behavior and movements depend strongly on their physical ability to perform them normally, which is often limited in fast growth birds (Bokkers and Koene, 2003). Broiler behavior and movement are, in addition, affected by factors such as density and location within the house under assessment (Leone and Estevez, 2008; Mallapur et al., 2009; Buijs et al., 2010). Indeed, the distribution of broilers inside a house is not homogeneous, as individuals will tend to stay close to walls to seek protection (Newberry and Hall, 1990; Estevez et al., 1997), especially those showing health/welfare problems (BenSassi et al., 2019a). All these aspects may influence the results of welfare assessments, and therefore, they must be carefully considered when interpreting assessment outcomes.
Recent technological developments aid in performing broiler welfare assessments under experimental and commercial conditions (Ben Sassi et al., 2016). Among them, the i-WatchBroiler app, designed for mobile devices, was created to facilitate on-farm welfare assessments. The i-WatchBroiler app is based on the adaptation of the transect walk methodology to poultry rearing conditions (Marchewka et al., 2013, 2015). With the app, observers can record the frequencies of birds displaying any of a list of defined welfare issues. Frequencies are standardized with respect to the expected number of birds present on the transect during the assessment. Both the sampling method and the app have already proven their usefulness and practicality to assess broiler welfare in commercial environments (BenSassi et al., 2019a,b).
In broiler chicken farming, a production shift toward the use of slower growth rate genetic lines and the use of lower stocking densities is taking place. This should greatly help to overcome some of the detrimental animal welfare issues of intensive broiler production (Bessei, 2006). Many studies have already pointed out the welfare benefits of raising slow or medium growth strains with respect to fast growth strains (Wilhelmsson et al., 2019; Dixon, 2020; Rayner et al., 2020; Dawson et al., 2021; Torrey et al., 2021). These advantages have just started to be objectively confirmed in experimental farms representing production systems evaluating fast and slow growth broiler strains (Rayner et al., 2020; Dawson et al., 2021; Torrey et al., 2021). Nevertheless, welfare assessments on commercial flocks of fast and medium/slow growth genetic strains are still lacking, even though they are necessary to confirm these experimental results.
The aim of this study was to quantitatively assess the differences in animal welfare outcomes of commercial broiler flocks differing in growth rates, using the most relevant, currently available poultry welfare assessment protocols. The study also considered the effect of management and environmental parameters that have been associated with broiler welfare outcomes. We hypothesized that welfare assessment outcomes would confirm the expected increased welfare status in slower growing flocks. In the case of behavioral measures, we expected them to be more closely linked to the actual biological capabilities of broilers according to their genetic potential rather than to their actual motivation to perform the assessed behaviors.
Materials and Methods
Animals
The study was conducted between July and December 2020. The welfare of 20 commercial broiler flocks, reared in Northern Spain for the same integrating company, was assessed using a protocol that included indicators from both the AWIN® and Welfare Quality® projects. Half of the assessed flocks corresponded to fast growth broilers (n = 10), and the other half to a medium growth strain (n = 10).
The welfare of fast growth flocks was assessed at 32.3 ± 0.3 (mean ± se) days of age, always before bird clearance. Cumulative mean flock mortality the day of the assessment was 3.6 ± 0.2%. Flocks were all of mixed sexes, comprised the genetic lines Ross 308, Cobb 500, or a mix of both, and had an initial mean flock size of 26,500 ± 2,018 birds. Initial stocking density of assessed flocks was, on average, 16.5 ± 0.3 broilers/m2. Houses were 107 ± 5 m long and 15 ± 0.6 m wide, all used some type of forced ventilation (longitudinal or transversal) and used artificial (fluorescent or LED) lighting. Broilers were provided with at least 6 h of darkness/day, a mean of 4.5 ± 0.5 h of continuous darkness/day, ad libitum access to feed through pan feeders, and fresh drinking water through nipple drinkers. House floors were covered with wood shavings or rice hulls as bedding material.
Medium growth flocks were assessed at 47.8 ± 0.6 (mean ± se) days of age, when mean cumulative mortality was 2.1 ± 0.2%. Flocks (mixed sexes) were all a Hubbard JA × Ross 308 cross, with a mean initial size of 14,790 ± 1,525 birds. Initial stocking density of assessed flocks was, on average, 11.7 ± 0.1 broilers/m2. Houses were 90 ± 8 m long and 14 ± 0.6 m wide. Seven out of the 10 houses had some type of forced ventilation (longitudinal or transversal), while 3 had natural ventilation. Houses were provided with fluorescent or LED lighting that was in some cases combined with natural light. Flocks had 6.4 ± 0.2 h of total darkness/day, 5.4 ± 0.4 h of continuous darkness/day, and had ad libitum access to feed through pan feeders, and to fresh drinking water through nipple drinkers. Bedding material was wood shavings, rice hulls, or chopped straw.
Data Collection
All welfare assessments started around 9 a.m. and were carried out by two trained observers using a structured assessment protocol. An introduction of the study was first given to the farmer and veterinary in charge of the farm. Then a questionnaire, based on farm written records of the flock to be assessed and of the facilities, was completed previous to entering the broiler house. Then, a visual inspection of the flock was done to detect visual signs of thermal stress, either huddling indicating hypothermia (Gilbert et al., 2010) or panting and wing elevation indicating hyperthermia (Lara and Rostagno, 2013). After this, the observers measured house length and width, divided the house into the corresponding number of transects and measured the width of each of them (Marchewka et al., 2013; BenSassi et al., 2019b). The distance between 3 consecutive feeders that defined the observation patches to assess positive behaviors was also measured (Rayner et al., 2020). All distances were measure with a laser meter (GLM 250 VF Professional, Robert Bosch GmbH, Germany). All required farm and flock information was introduced in the i-WatchBroiler app installed on a mobile device prior to start the welfare flock assessment.
After collecting all this required information, the on-farm data collection started. Each observer collected data in the areas delimited by one central and one wall transect, pseudo-randomly assigned to each observer and flock prior to start data collection to comply with transect assessment needs (Marchewka et al., 2015). Distance between both observers' transects was maximized to avoid interference, being at least one transect away.
Observer 1 performed a series of three consecutive rounds of data collection within the area delimited by the two assigned transects. During the first round, using the transect method with the i-WatchBroiler app, a central and a wall transect were assessed for AWIN® welfare indicators, bedding quality and environmental parameters. Transect method inter-observer reliability and overall robustness of the assessment had already been determined in our previous studies (Marchewka et al., 2013, 2015; BenSassi et al., 2019a,c). Collected AWIN® welfare indicators included number of birds immobile, lame, small, dirty, sick, featherless, with head, back, and tail wounds, terminally ill, and dead. Indicators were identified and collected as defined in Marchewka et al. (2013). Environmental parameters were also collected at the beginning, middle, and end of each transect walk. Parameters collected included environmental temperature (T, °C), relative humidity (RH, %), light intensity (LI, lux), and CO2 and NH3 concentrations (ppm), that were all collected with portable meters (AZ 7755, AZ Instrument Corp., Taiwan for T, RH, and CO2; GasAlert NH3 Extreme, BW Technologies, Canada for NH3; and MT-912, Urceri, China for LI). Bedding quality was also scored at the same 3 locations per transect, using the 5-point score defined in the Welfare Quality® protocol for poultry (2009).
In a second round, the same assigned transects were assessed for positive behaviors according to the definitions from Rayner et al. (2020). Four observations were carried out on different, evenly distributed observation patches per transect. The observer walked through each transect and then turned to observe the patch from a distance of 3 m during 15 s. For each assessed patch the number of birds displaying positive behaviors (“worm” running, play fighting, wing flapping, jumping, running, ground scratching, vertical wing shaking and perching) were collected. Finally, in a third round, broiler fear response was assessed by means of avoidance tests (Forkman et al., 2007). A series of 21 avoidance tests were carried out as described in the Welfare Quality® protocol for poultry (2009). At the end of each test the number of broilers within a circle of 1 m around the observer was registered. After this, Observer 1 performed gait score assessments on 150 broilers using the scale defined by Kestin et al. (1992). Birds were randomly selected and observed from a distance following the procedures as described by Dawkins et al. (2004) and Marchewka et al. (2015). In this case, the total house area was sampled, although care was taken to avoid perturbation of the broilers being simultaneously assessed by Observer 2.
Simultaneously, Observer 2 assessed fear and exploratory motivation through novel object tests. These observations were delimited to the two transect areas assigned to Observer 2. Novel object tests were carried out as described by de Jong and Gunnink (2019), on 21 locations evenly distributed within the assigned areas. Each test consisted in inserting a novel object, a 50 cm red plastic stick, into the bedding and then walking back 3 m. Each test lasted for 4 min, during which latency (s) for the first broiler to get within a circle of 0.5 m around the object, the latency (s) to touch the object, the number of broilers within 0.5 m of the novel object at 30-s intervals, and the total number of object contacts (n) were collected.
Finally, after the completion of all observations, both observers collected body weight and hock burns on 100 birds. Body weight and hock burns of 100 broilers were assessed by both observers. Broilers were fenced out in groups of 25 birds at four different areas of the house to have a proper representation of the whole flock. Birds were then individually caught by one observer, who weighed them and then presented them to the other observer for hock burn assessment. The observer assessing hock burns was always the same across all flocks. Hock burns of both legs were scored according to the 5-point score defined in the Welfare Quality® protocol for poultry (Welfare Quality, 2009). Only the highest score was retained. After this, each broiler was released and the next broiler was assessed.
Variable Calculation and Statistical Analysis
AWIN® welfare indicators were expressed as their occurrence relative to the estimated number of broilers present in each assessed transect (%), estimated by the transect size relative to the total house size and flock size on the day of assessment. In addition, aggregated welfare indicators (% of mobility problems, health problems, lesions, and welfare index) were calculated as described in BenSassi et al. (2019b). Each positive behavior was expressed as its occurrence relative to the estimated number of broilers present on each patch (%), which was obtained considering the patch area relative to the total house area, and the flock size during the assessment. A positive behavior diversity index, corresponding to the number of different positive behaviors simultaneously observed on each patch, was also calculated.
Data were analyzed using SAS 9.4 (SAS Institute, NC, USA). Data normality and variance homoscedasticity of all continuous, dependent variables were tested. In cases where these were not met, a lognormal distribution (latency of the first broiler within a circle of 0.5 m around the object, total number of object contacts), a gamma distribution (latency to touch the object), and a Poisson distribution (jumps and other behaviors) were assumed. The effects of the flock type (medium vs. fast growth), house location (central vs. wall transect), and their interaction on dependent variables were tested by means of mixed model ANOVAs using the GLIMMIX procedure. For the number of broilers within 0.5 m of the novel object, time after beginning the test and its interaction with main fixed effects were also tested by repeated measures mixed model ANOVA. For the variables collected during the avoidance and novel object tests, house width (m) was also included in models as a covariable and retained when statistically significant (P < 0.05). All models included the farm as a random effect. Least square means were computed in case of statistically significant effects (P < 0.05), with P-values adjusted for multiple comparisons by Tukey range tests.
Bedding quality, gait score, hock burn score, and behavior diversity were analyzed assuming an ordered multinomial response distribution and a cumulative logit link function. The effects of the genetic strain and house location were tested, and the odds ratios of each independent variable level with respect to the reference level were estimated, as well as their 95% confidence interval. Models also included a farm random effect.
Ethical Statement
Farms met the requirements of the Spanish legislation on the protection of chickens produced for meat purposes (Real Decreto 692/2010), and the study complied with Spanish legislation relative to the use of animals for experimentation and scientific purposes (Real Decreto, 53/2013).
Results
The results of the AWIN® welfare indicators assessment according to the transect walk method are presented in Table 1. Higher percentages of immobile, lame, sick and featherless birds, and of birds with tail wounds (all P < 0.05) were found in fast growth flocks. Aggregation of welfare indicators showed that fast growth flocks had more mobility problems and lesions, and a worse welfare index (all P < 0.05). Percentages of dead birds (P = 0.089) and with health problems (P = 0.097) tended to be higher on wall locations (Table 1). The interaction between genetic strain and house location was not significant for any welfare indicator (P > 0.05 in all cases).
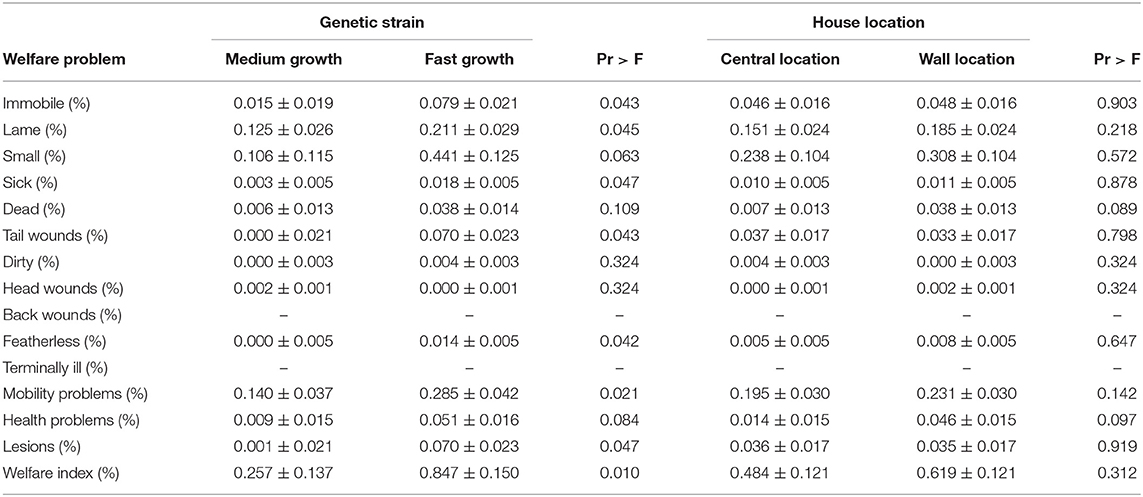
Table 1. Effect (mean ± se) of broiler genetic strain (medium vs. fast growth broilers) and of the house location where welfare assessments were carried out (central vs. wall locations) on the occurrence of welfare problems/transect detected during welfare assessments.
Regarding positive behaviors assessed during transect walks, results (Table 2) show a significant flock type (fast or medium growth flocks) by house location interaction for play fighting, running (P < 0.01) and wing flapping (P < 0.05). Much higher percentages of positive behaviors were observed in medium growth flocks at central locations, specially wing flapping, running and play fighting as compared to fast growth strains. Jumps and other positive behaviors were rare (Table 2), and jumps were not affected by any of the studied variables. The odds of observing higher positive behavior diversity was 2.46 times larger (95% confidence limits of 1.12 and 5.40; P = 0.026) for medium with respect to fast growth flocks, and 4.07 times higher (95% confidence limits of 2.21 and 7.47; P < 0.001) in central with respect to wall locations.
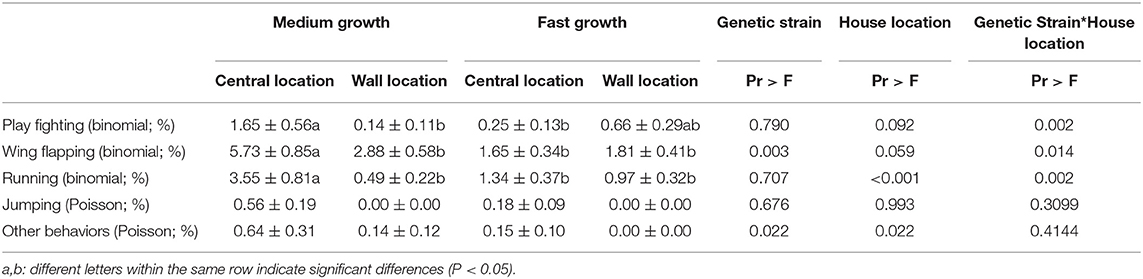
Table 2. Effect (mean ± se) of broiler genetic strain (medium vs. fast growth broilers), of the house location where welfare assessments were carried out (central vs. wall locations), and of their interaction on the occurrence of positive behaviors detected during welfare assessments.
During avoidance tests the number of broilers remaining close to the observer was found to be highest in fast growth flocks on wall locations (Figure 1; P < 0.001). The smallest number of broilers remaining close to the observer corresponded to medium growth flocks on central locations, with medium growth flocks assessed on wall locations showing intermediate values. For the novel object tests, the number of broilers within 0.5 m of the object was highest for medium growth flocks at central locations, while the lowest was also in medium growth flocks at wall locations (flock type by house location interaction P < 0.001; Figure 2). As shown in Figure 3, the interaction between the flock type and sampling time was significant (P = 0.048). When comparing sampling times within each genetic strain, the number of birds within 0.5 m of the object stabilized earlier in medium growth flocks (at 120 s, compared to 150 s of fast growth flocks). On the other hand, no significant differences were detected when fast and medium growth flocks were compared within each sampling time (every 30 s). Latency for the first broiler within 0.5 m around the object was also affected by flock type and house location with medium growth flocks on wall locations showing longer latencies (Figure 4; P = 0.003). Latency to contact the novel object (Figure 5) was, overall, longer on wall than on central locations (P = 0.001). The total number of novel object contacts was significantly higher in medium than in fast growth flocks (3.0 ± 0.3 vs. 1.2 ± 0.3 contacts, respectively; P = 0.006) and tended to be higher on central than on wall locations (3.7 ± 0.3 vs. 1.9 ± 0.3 contacts, respectively; P = 0.086).
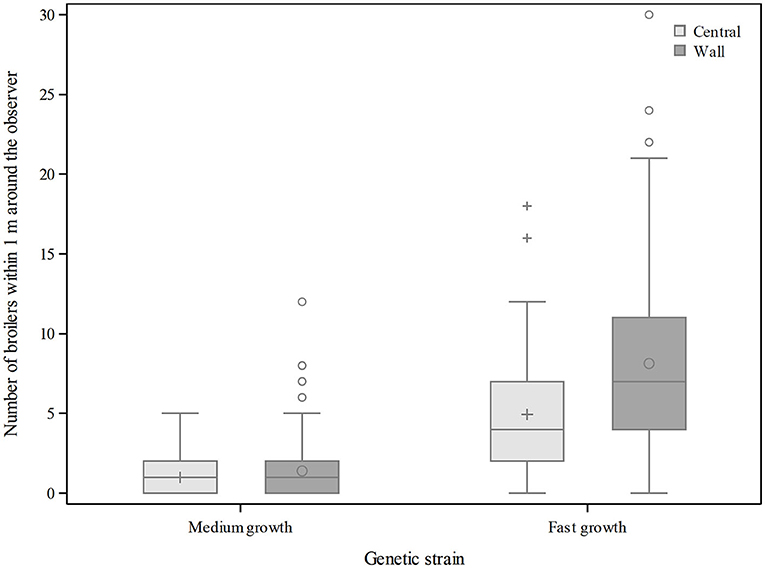
Figure 1. Interaction between the genetic strain and the assessment house location on the number of broilers within 1 m of the observer during avoidance tests. Boxes show interquartile ranges, and whiskers represent minimum and maximum values. Symbols (+, °) inside boxes indicate the mean value, while symbols (+, °) outside whiskers represent outliers.
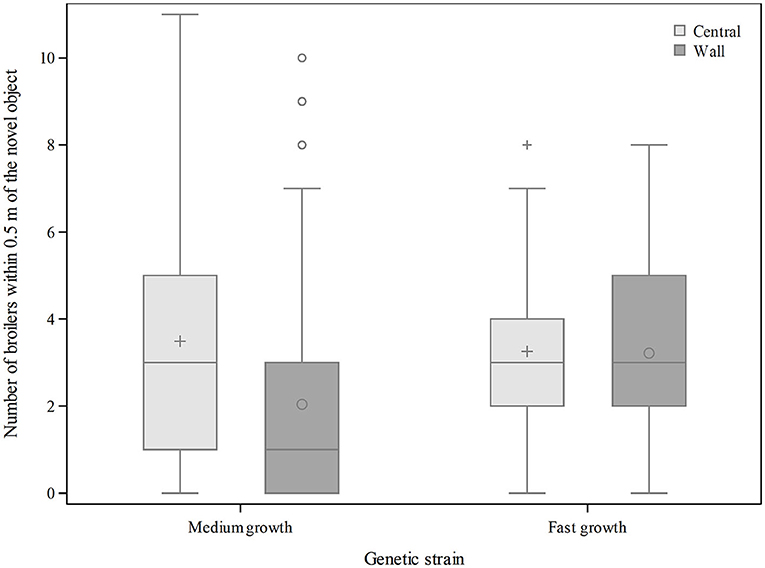
Figure 2. Interaction between the genetic strain and the assessment house location on the number of broilers within 0.5 m of the novel object. Boxes show interquartile ranges, and whiskers represent minimum and maximum values. Symbols (+, °) inside boxes indicate the mean value, while symbols (+, °) outside whiskers represent outliers.
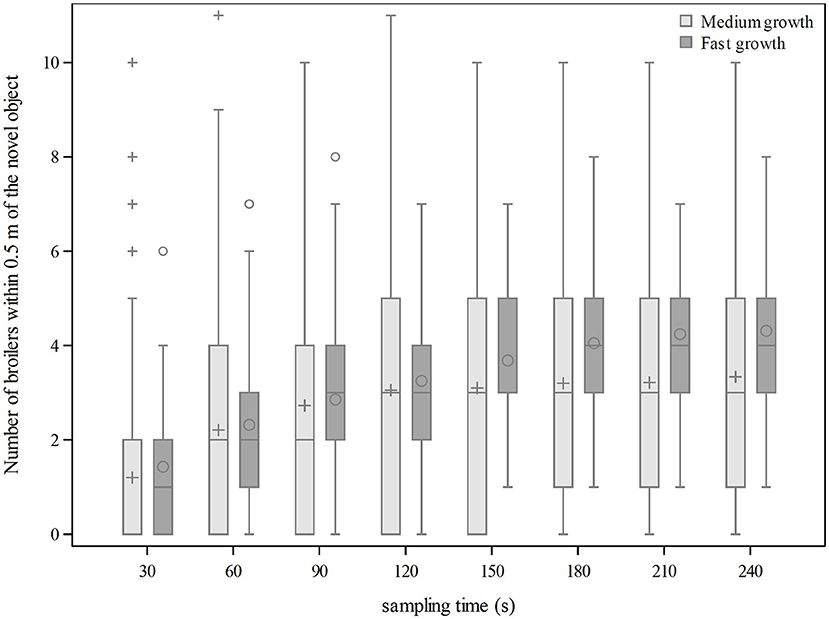
Figure 3. Interaction between the genetic strain and the sampling time on the number of broilers within 0.5 m of the novel object. Boxes show interquartile ranges, and whiskers represent minimum and maximum values. Symbols (+, °) inside boxes indicate the mean value, while symbols (+, °) outside whiskers represent outliers.
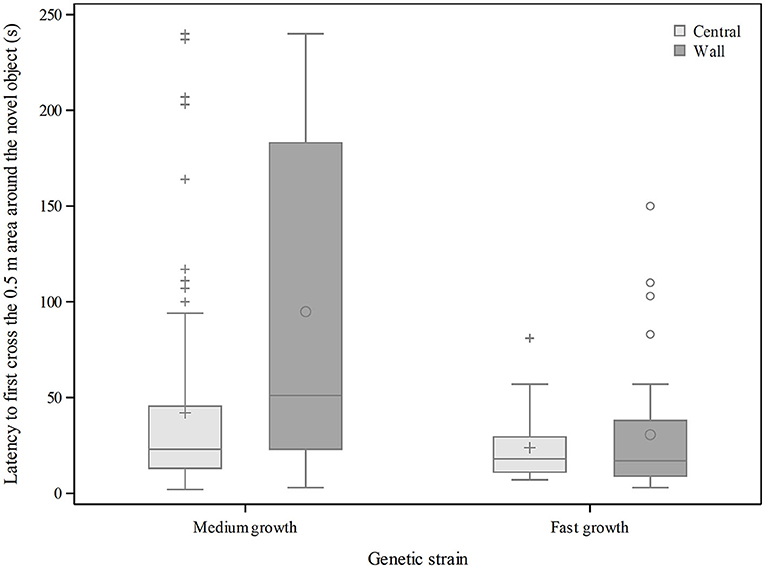
Figure 4. Interaction between the genetic strain and the assessment house location on the latency to first cross the 0.5 m area around the novel object. Boxes show interquartile ranges, and whiskers represent minimum and maximum values. Symbols (+, °) inside boxes indicate the mean value, while symbols (+, °) outside whiskers represent outliers.
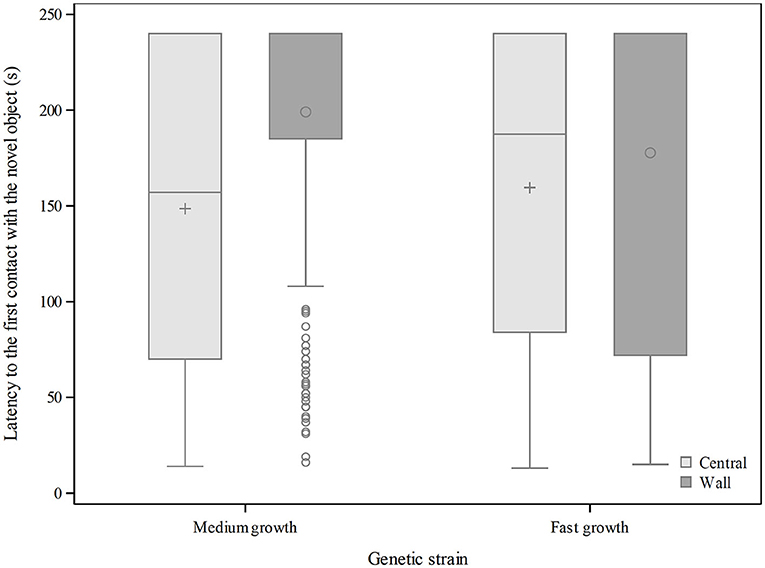
Figure 5. Interaction between the genetic strain and the assessment house location on the latency to the first contact with the novel object. Boxes show interquartile ranges, and whiskers represent minimum and maximum values. Symbols (+, °) inside boxes indicate the mean value, while symbols (+, °) outside whiskers represent outliers.
Average hock burn scores did not differ between flock type (0.20 ± 0.07 on average; P = 0.267). The odds of obtaining higher hock burn scores (i.e., higher hock burn severity) did not differ between medium and fast growth flocks (odds ratio of 1.36, 95% confidence limits of 0.76 and 2.45; P = 0.308). Mean gait scores were 0.7 ± 0.1 and 1.4 ± 0.1, for medium and fast growth flocks, respectively (P < 0.001), and 1.1 ± 0.1 and 1.0 ± 0.1 for central and wall locations, respectively (P = 0.078). The risk of higher gait scores (i.e., chickens with more severe leg problems) was significantly affected by the flock type, so that risk was 4.5 times higher for fast growth flocks than for medium growth flocks (95% confidence limits being 2.5 and 7.9; P < 0.001). In addition, the risk of observing birds with worst gait scores was 1.2 times higher at central than at wall locations (95% confidence limits being 1.0 and 1.4; P = 0.047).
Table 3 shows the differences between the housing environments of medium and fast growth flocks. Mean house T was lower for medium as compared to fast growth flocks (P < 0.001), and the opposite was found for CO2 concentration (P = 0.006). Mean RH was lower in central locations (P = 0.010), whereas LI was lower in wall locations (P = 0.005). Bedding quality did not differ between flock types and house locations, with mean bedding score being 1.80 ± 0.17. The odds of obtaining higher bedding scores (i.e., worse bedding quality) did not differ between flock types (odds ratio of 2.175, 95% confidence limits being 0.461 and 10.267, when comparing medium vs. fast growth flocks; P = 0.296) or house locations (odds ratio of 1.392, 95% confidence limits being 0.691 and 2.805, when comparing central vs. wall locations; P = 0.351).
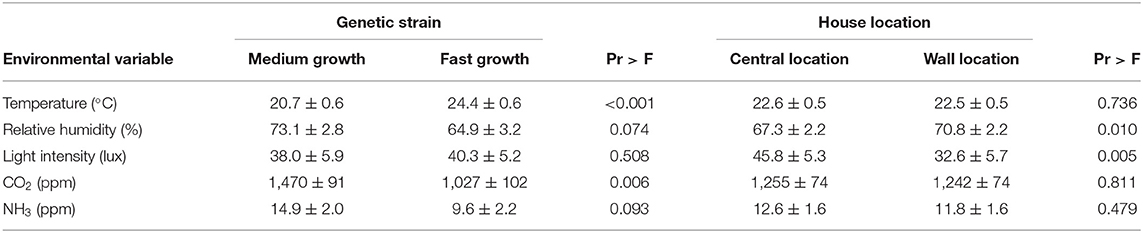
Table 3. Effect (mean ± se) of broiler genetic strain (medium vs. fast growth broilers) and of the house location where welfare assessments were carried out (central vs. wall locations) on house environmental variables measured during welfare assessments.
Discussion
For objective and rigorous animal welfare assessments on commercial farms, the combination of both animal and resource-based indicators, together with the use of indicators that account for all animal welfare principles, are recommended. Welfare assessments on commercial farms can be influenced by interfering factors inherent to current production conditions (BenSassi et al., 2019a,b), or inherent to the animals per-se. In this context, the present study aimed at quantitatively assess the differences in animal welfare outcomes of commercial broiler flocks, differing in growth rates, using the most relevant, currently available poultry welfare assessment protocols, the Welfare Quality® and AWIN® protocols. The transect method, already validated for inter-observer reliability and method validity to assess the AWIN® welfare indicators (Marchewka et al., 2013, 2015) and positive behaviors (Rayner et al., 2020), was use for flock assessment. In addition, bedding quality, environmental parameters were collected along each transect. The division of each house under study into transects was also used to systematize data collection for indicators described in the Welfare Quality® Poultry protocol (2009).
The results of the assessment with the transect method provide quantitative evidences of a higher deterioration in the welfare status of fast growing flocks, as shown by the incidence of broilers with wounds, mobility and health problems that were significantly higher as compared to those observed in medium growth flocks. However, the results for fast growing flocks also showed a lower incidence of welfare issues as compared to the results obtained in previous studies using the transect method by Marchewka et al. (2013) and BenSassi et al. (2019a) in fast growth broiler flocks within the same region. The improvement in welfare status of the most recently studied flocks may be explained by the fact that all farms participating in the study are regularly audited for animal welfare, and therefore they already need to keep flocks under good welfare standards.
Fast growth has been associated to several important welfare concerns (Bassler et al., 2013). Among others, fast growth is known to cause increased bone disorders and pathologies leading to lameness (Shim et al., 2012; Wilhelmsson et al., 2019), and of immune-related problems (Cheema et al., 2003). The differences among fast and slow growth birds show that, even if health status has improved for fast growth birds, still they show a reduced welfare status when compared to slower growth birds. Besides genetic factors that predispose to increase welfare issues in fast growth broilers, part of the effects may also be linked with the higher rearing densities in which they are maintained, which is known to be associated to a higher presence of skin scratches (Allain et al., 2009) and other health problems (Ventura et al., 2010), as is the case in this study. These results are not too surprising, but they serve to confirm and quantify the magnitude in welfare status of flocks varying in growth rate and rearing density, as measured with the transect method. To which extent genetics and rearing density contribute to the negative effects in welfare status cannot be teased apart in this study.
Although differences in the prevalence of welfare indicators between house locations were hardly remarkable among fast and medium growth flocks, the percentages of dead broilers and health problems tended to be higher in wall transects. In commercial flocks, it is commonly observed that dead birds tend concentrate in areas close to the sidewalls and house ends (Tabler et al., 2002). Previous studies using the transect sampling methodology (Marchewka et al., 2013; BenSassi et al., 2019a) already highlighted the fact that the highest prevalence of welfare issues occurred in wall transectss. This is explained by the fact that birds with problems will tend to navigate toward the perimetral areas of the house, seeking for a perceived protective effect of walls (Newberry and Hall, 1990; Cornetto and Estevez, 2001). From a practical standpoint, when applying welfare assessment protocols, this result imply that both central and wall locations should be assessed in order to capture a truly representative picture of the flock welfare status.
By using the transect method and the i-WatchBroiler app we also determined that diversity and prevalence in the expression of positive behaviors were higher in medium growth flocks, showing that not only these birds had less welfare issues but they also had a better capacity to express positive behaviors (Boissy et al., 2007). Our results agree with those of Rayner et al. (2020), who reported a reduced expression of positive behaviors in fast growth broilers. Nevertheless, and similarly to our case, in that study the treatment using fast growth broilers also used higher stocking densities, while slow growth treatments combined both high and low stocking densities. Therefore, in both studies, it is difficult to ascertain whether the lowest expression of positive behaviors was due to the faster growth rate, to the higher stocking density or, most probably, to a combination of both. On the other hand, in another recent study comparing fast and slow growth strains, stocking density was maintained constant for all treatments (Dawson et al., 2021). Despite that behaviors were not explicitly defined as positive behaviors, their results indicated that slow growth birds spent more time walking and interacting with enrichment materials. This was attributed to the reduced physical abilities of fast growth birds, which suggests that they experienced more difficulties to express a wider behavioral repertoire as compared to slow growth birds. Considering these supporting literature, it might be suggested that, in our study, the expression of positive behaviors was likely more prevalent in medium growth flocks because their reduced growth rate and body weight facilitated the physical possibility to express high activity behaviors. However, it must be realistically accepted that in this study the effects of lower stocking densities may have added to the better physical abilities of the birds.
In regard of these positive welfare indicators, our results showed that their expression was strongly associated with house location, with lower performance observed in wall transects. This effect may be associated with the higher relative bird densities normally observed in wall areas (Newberry and Hall, 1990; Cornetto and Estevez, 2001), due to the “wall trapping effect” as defined by Estevez and Christman (2006). Less space would be available in wall transects, therefore reducing the possibilities for the birds to express behaviors that are energetic but that also require larger open spaces for their performance. This “wall trapping effect” would be responsible for the establishment of a gradient of possibilities of movement (higher in the center and lower near walls) that also informs about broilers' welfare status. Thus, the assessment of negative welfare incidences as well as of positive welfare must be assessed both in wall and central house locations if a representative picture of the whole flock is pursued.
Fear tests, such as the novel object and the avoidance tests, are designed to determine animals' behavioral response to any danger threatening their integrity such as the presence of unknown persons or objects (Forkman et al., 2007). They might require well controlled, experimental conditions to permit drawing accurate conclusions but, still, solid conclusions may be difficult to extract, as interpretation of behavioral tests is, as previously stated, “multifaceted” (Kulke et al., 2021). In any case, the novel object and the avoidance tests have been adapted to and used in commercial environments (de Jong and Gunnink, 2019), and have even been adopted in broiler welfare assessment protocols and auditing schemes. In our study, relevant interactions between flock type and house location were detected for the novel object tests. Fast growth broilers showed a similar response, irrespective of whether assessment was on wall or central transects. Medium growth broilers on central locations approached the object faster and in larger numbers, and tended to first contact the object faster than those on wall locations. Furthermore, the number of medium growth broilers close to the novel object stabilized before the number of fast growth broilers. This may be classically interpreted as medium growth flocks displaying less fear of the novel object and higher curiosity toward it, although lower densities may have also played an important role on this overall effect. Low densities result in fewer disturbances among broilers and facilitate their mobility (Buijs et al., 2010; Leone et al., 2010). In this study, lower densities linked to medium growth strains would have promoted higher freedom for bird movement (Leone and Estevez, 2008). However, this does not explain the differences among wall and central locations in the response found in medium growth flocks. The explanation for this may relate to the wall presence reducing the possible positions from which broilers could access the novel object. This is contrary to what was observed in central house locations as birds have equal chances of getting to the novel object from any of the 360° around the object. Another explanation may be that the potentially lower welfare status of broilers assessed on peripheral areas might have influenced their behavioral response during tests. This remains to be tested, and for this individual movement tracking technologies might be of interest. On the other hand, in fast growth flocks results of the novel object did not depend on the house location. Perhaps both higher mobility problems and higher densities would have already limited broiler movement and ability to react to the presence of the novel object.
Results of the avoidance tests appear to further confirm our hypothesis relative to the underlying mechanisms of the behavioral response during novel object tests. A higher number of broilers remained close to the observer in the case of fast growth flocks, which was particularly evident on wall locations. Although avoidance tests are designed to determine levels of animal fear (Forkman et al., 2007), it is likely that, as previously suggested, a perfect relationship between test outcomes and animal fear may only be sustained under experimental setups, while in commercial conditions other factors may interfere with the outcome. Our results, suggest that movement difficulties of fast growth broilers due to the combination of their reduced physical ability and high rearing density, in addition to the effect of the walls (as a barrier and as a “trap”) may affect to some extent the outcomes of some of the test that require bird movement.
Mean bedding quality did not differ between flock type or house locations, with score ranging between 1 and 2 according to the Welfare Quality® scale. These values can be considered satisfactory and are lower than the average score of 2.3 reported for commercial flocks from different European countries (de Jong et al., 2016). As previously indicated, all farms participating in the study undergo periodical welfare audits, with bedding quality being one of the inspected parameters, besides being a factor with potential to have a major impact on other welfare indicators. Thus, farmers need to make the effort to maintain bedding under best possible conditions, and this may explain the overall good results regarding this aspect.
Despite the good bedding quality, we found that the risk of obtaining worse gait scores was higher in fast growth flocks in agreement with Dixon (2020) and Rayner et al. (2020), as both studies found that gait scores were higher in fast growth than in slower growing flocks and supporting the idea that faster growth rates result in more leg and mobility problems (Kestin et al., 2001). It is worth highlighting the fact that, although statistical significance was small, the risk of observing higher gait scores was higher in central house locations. This may be explained by the fact that the complete house area was sampled for gait scores and, given that peripheral house locations represent a smaller proportion of the total house area, fewer birds were therefore sampled for gait score on peripheral areas. This may have resulted in the probabilities of observing birds with higher gait scores being smaller on peripheral areas. Given the close link between poor bedding quality and increased hock burn severity (de Jong et al., 2014), overall good bedding results likely explain why hock burn scores were on average low and independent from the tested effects.
Environmental variables, particularly RH, CO2, and NH3 concentrations, suggest that better ventilation systems were used in houses in which fast growth birds were reared. This may be attributed to their higher level of technification, as all of them had some type of forced ventilation, while 30% of medium growth houses exclusively depended on natural ventilation. However, in these facilities, wall locations were characterized by having a high RH and lower light intensity, suggesting some degree of ventilation systems' inefficiency and a heterogeneous distribution of the lighting prioritizing central locations.
The transect method has previously confirmed its robustness (BenSassi et al., 2019c) and sensitivity to different environmental and management aspects (BenSassi et al., 2019b). The new information generated in this study confirms the effectiveness of the transect method and associated technology as a tool to quantify the level of welfare problems that might be associated to specific genetic strains. Added to the previously generated scientific information, and to the advantages of welfare assessment on a freely available electronic support, this has also clear, straightforward data processing and management benefits. These advantages can particularly benefit farmers and technical personnel interested in monitoring on-farm broiler welfare because it requires less time, involves no bird catching and results can be directly obtained after assessment. In addition, the technology allows for centralized, massive data collection and processing that may be the basis for improved management and to data based strategic decisions.
In conclusion, the use of the transect sampling method and associated welfare assessment technology, allowed us to detect that fast growth flocks had more mobility and health problems, more lesions, and a reduced prevalence and diversity in the expression of positive behaviors with respect to medium growth flocks. The differences among flocks are interpreted as clear evidences of reduced welfare status of fast growing birds, as compared to medium growth, the effect that was to some extent magnified by the higher densities in which fast growth broilers are reared. The transect method and associated technology (the app i-watchbroiler) allowed for an easy and efficient welfare assessment, and offered enough resolution as to be able to detect clear differences in welfare status, in addition to the collection of positive indicators, adding further support to their usefulness under commercial practice. The results of the novel object and avoidance tests suggest that behavioral responses were modulated by the birds' physical ability to move, the rearing density that characterize fast growth production systems, and by the location where tests were conducted. Therefore, the results of these tests should be interpreted with caution when conducted on commercial flocks, on birds with different physical abilities and under different management practices, especially density.
Data Availability Statement
The datasets presented in this study can be found in online repositories. The names of the repository/repositories and accession number(s) can be found below: Figshare repository (doi: 10.6084/m9.figshare.19071185).
Ethics Statement
Farms met the requirements of the Spanish legislation on the protection of chickens produced for meat purposes (Real Decreto 692/2010), and the study complied with Spanish legislation relative to the use of animals for experimentation and scientific purposes (Real Decreto, 53/2013).
Author Contributions
IE, XA, and FN: conceptualization, formal analysis, investigation, methodology, and supervision. XA and FN: data collection. XA: writing—original draft preparation. FN and IE: writing—reviewing and editing. All authors contributed to the article and approved the submitted version.
Funding
The authors acknowledge the Science, Innovation and Universities Ministry of Spain (Grant TECHWEL, RTI2018-096034-R-C22) for the financial support to conduct the study and salary provision for FN.
Conflict of Interest
The authors declare that the research was conducted in the absence of any commercial or financial relationships that could be construed as a potential conflict of interest.
Publisher's Note
All claims expressed in this article are solely those of the authors and do not necessarily represent those of their affiliated organizations, or those of the publisher, the editors and the reviewers. Any product that may be evaluated in this article, or claim that may be made by its manufacturer, is not guaranteed or endorsed by the publisher.
Acknowledgments
The authors wish to thank the collaboration of the company Grupo AN, especially Javier Lacalle and all the veterinarians and farmers involved, for allowing us access to their flocks.
References
Allain, V., Mirabito, L., Arnould, C., Colas, M., Le Bouquin, S., Lupo, C., et al. (2009). Skin lesions in broiler chickens measured at the slaughterhouse: relationships between lesions and between their prevalence and rearing factors. Br. Poult. Sci. 50, 407–417. doi: 10.1080/00071660903110901
Alonso, M. E., González-Montaña, J. R., and Lomillos, J. M. (2020). Consumers' concerns and perceptions of farm animal welfare. Animals 10:385. doi: 10.3390/ani10030385
Bassler, A. W., Arnould, C., Butterworth, A., Colin, L., de Jong, I. C., Ferrante, V., et al. (2013). Potential risk factors associated with contact dermatitis, lameness, negative emotional state, and fear of humans in broiler chicken flocks. Poult. Sci. 92, 2811–2826. doi: 10.3382/ps.2013-03208
Ben Sassi, N., Averós, X., and Estevez, I. (2016). Technology and poultry welfare. Animals 6, 62. doi: 10.3390/ani6100062
BenSassi, N., Averós, X., and Estevez, I. (2019a). The potential of the transect method for early detection of welfare problems in broiler chickens. Poult. Sci. 98, 522–532. doi: 10.3382/ps/pey374
BenSassi, N., Averós, X., and Estevez, I. (2019c). Broiler chickens on-farm welfare assessment: estimating the robustness of the transect sampling method. Front. Vet. Sci. 6:236. doi: 10.3389/fvets.2019.00236
BenSassi, N., Vas, J., Vasdal, G., Averós, X., Estevez, I., and Newberry, R. (2019b). On-farm broiler chicken welfare assessment using transect sampling reflects environmental inputs and production outcomes. PLoS ONE 14, e0214070. doi: 10.1371/journal.pone.0214070
Bessei, W. (2006). Welfare of broilers: a review. Worlds Poult. Sci. J. 62, 455–466. doi: 10.1079/WPS2005108
Boissy, A., Manteuffel, G., Jensen, M. B., Moe, R. O., Spruijt, B., Keeling, L. J., et al. (2007). Assessment of positive emotions in animals to improve their welfare. Physiol. Behav. 92, 375–397. doi: 10.1016/j.physbeh.2007.02.003
Bokkers, E. A. M., and Koene, P. (2003). Behaviour of fast- and slow growing broilers to 12 weeks of age and the physical consequences. Appl. Anim. Behav. Sci. 81, 59–72. doi: 10.1016/S0168-1591(02)00251-4
Buijs, S., Keeling, L. J., Vangestel, C., Baert, J., Vangeyte, J., and Tuyttens, F. A. M. (2010). Resting or hiding? Why broiler chickens stay near walls and how density affects this. Appl. Anim. Behav. Sci. 124, 97–103. doi: 10.1016/j.applanim.2010.02.007
Cheema, M. A., Qureshi, M. A., and Havenstein, G. B. (2003). A comparison of the immune response of a 2001 commercial broiler with a 1957 random bred broiler strain when fed representative 1957 and 2001 broiler diets. Poult. Sci. 82, 1519–1529. doi: 10.1093/ps/82.10.1519
Cornetto, T. L., and Estevez, I. (2001). Influence of vertical panels on use of space by domestic fowl. Appl. Anim. Behav. Sci. 71, 141–153. doi: 10.1016/S0168-1591(00)00171-4
Dawkins, M. S., Donnelly, C. A., and Jones, T. A. (2004). Chicken welfare is influenced more by housing conditions than by stocking density. Nature 427, 342–344. doi: 10.1038/nature02226
Dawson, L. C., Widowski, T. M., Liu, Z., Edwards, A. M., and Torrey, S. (2021). In pursuit of a better broiler: a comparison of the inactivity, behavior, and enrichment use of fast- and slower growing broiler chickens. Poult. Sci. 100, 101451. doi: 10.1016/j.psj.2021.101451
de Jong, I. C., and Gunnink, H. (2019). Effects of a commercial broiler enrichment programme with or without natural light on behaviour and other welfare indicators. Animal 13, 384–391. doi: 10.1017/S1751731118001805
de Jong, I. C., Gunnink, H., and van Harn, J. (2014). Wet litter not only induces footpad dermatitis but also reduces overall welfare, technical performance, and carcass yield in broiler chickens. J. Appl. Poult. Res. 23, 51–58. doi: 10.3382/japr.2013-00803
de Jong, I. C., Hindle, V. A., Butterworth, A., Engel, B., Ferrari, P., Gunnink, H., et al. (2016). Simplifying the Welfare Quality® assessment protocol for broiler chicken welfare. Animal 10, 117–127. doi: 10.1017/S1751731115001706
Dixon, L. M. (2020). Slow and steady wins the race: the behaviour and welfare of commercial faster growing broiler breeds compared to a commercial slower growing breed. PLoS ONE 15, e0231006. doi: 10.1371/journal.pone.0231006
Estevez, I., and Christman, M. C. (2006). Analysis of movement and use of space of animals in confinement: the effect of sampling effort. Appl. Anim. Behav. Sci. 97, 221–240. doi: 10.1016/j.applanim.2005.01.013
Estevez, I., Newberry, R., and Arias de Reyna, L. (1997). Broiler chickens, a tolerant social system? Etología 5, 19–29.
Forkman, B., Boissy, A., Meunier-Salaün, M.-C., Canali, E., and Jones, R. B. (2007). A critical review of fear tests used on cattle, pigs, sheep, poultry and horses. Physiol. Behav. 92, 340–374. doi: 10.1016/j.physbeh.2007.03.016
Gilbert, C., McCafferty, D., Le Maho, Y., Martrette, J.-M., Giroud, S., Blanc, S., et al. (2010). One for all and all for one: the energetic benefits of huddling in endotherms. Biol. Rev. 85, 545–569. doi: 10.1111/j.1469-185X.2009.00115.x
Havenstein, G. B., Ferket, P. R., and Qureshi, M. A. (2003). Growth, livability, and feed conversion of 1957 versus 2001 broilers when fed representative 1957 and 2001 broiler diets. Poult. Sci. 82, 1500–1508. doi: 10.1093/ps/82.10.1500
Kestin, S. C., Gordon, S., Su, G., and Sørensen, P. (2001). Relationships in broiler chickens between lameness, liveweight, growth rate and age. Vet. Rec. 148, 195–197. doi: 10.1136/vr.148.7.195
Kestin, S. C., Knowles, T. G., Tinch, A. E., and Gregory, N. G. (1992). Prevalence of leg weakness in broiler chickens and its relationship with genotype. Vet. Rec. 131, 190–194. doi: 10.1136/vr.131.9.190
Kulke, K., Kemper, N., and Stracke, J. (2021). Boys (toms) don't try. Behaviour of turkeys in a novel object test – Influence of age and sex. Appl. Anim. Behav. Sci. 244, 105484. doi: 10.1016/j.applanim.2021.105484
Lara, L. J., and Rostagno, M. H. (2013). Impact of heat stress on poultry production. Animals 3, 356–369. doi: 10.3390/ani3020356
Leone, E. H., Christman, M. C., Douglass, L., and Estevez, I. (2010). Separating the impact of group size, density, and enclosure size on broiler movement and space use at a decreasing perimeter to area ratio. Behav. Processes 83, 16–22. doi: 10.1016/j.beproc.2009.08.009
Leone, E. H., and Estevez, I. (2008). Use of space in the domestic fowl: separating the effects of enclosure size, group size, and density. Anim. Behav. 76, 1673–1682. doi: 10.1016/j.anbehav.2008.08.004
Main, D. C. J., Whay, H. R., Leeb, C., and Webster, A. J. F. (2007). Formal animal-based welfare assessment in UK certification schemes. Anim. Welfare 16, 233–236.
Mallapur, A., Miller, C., Christman, M. C., and Estevez, I. (2009). Short-term and long-term movement patterns in confined environments by domestic fowl: influence of group size and enclosure size. Appl. Anim. Behav. Sci. 117, 28–34. doi: 10.1016/j.applanim.2008.11.005
Marchewka, J., Estevez, I., Vezzoli, G., Ferrante, V., and Makagon, M. M. (2015). The transect method: a novel approach to on-farm welfare assessment of commercial turkeys. Poult. Sci. 94, 7–16. doi: 10.3382/ps/peu026
Marchewka, J., Watanabe, T. T. N., Ferrante, V., and Estevez, I. (2013). Welfare assessment in broiler farms: transect walks versus individual scoring. Poult. Sci. 92, 2588–2599. doi: 10.3382/ps.2013-03229
McKay, J. C. (2009). “The genetics of modern commercial poultry,” in Biology of breeding poultry. Poultry Science Symposium, ed P. M. Hocking (Wallingford, Oxon: CABI Publishing), 3–9.
Newberry, R. C., and Hall, J. W. (1990). Use of pen space by broiler chickens: effects of age and pen size. Appl. Anim. Behav. Sci. 25, 125–136. doi: 10.1016/0168-1591(90)90075-O
Rauw, W. M., Kanis, E., Noordhuizen-Stassen, E. N., and Grommers, F. J. (1998). Undesirable side effects of selection for high production efficiency in farm animals: a review. Livestock Prod. Sci. 56, 15–33. doi: 10.1016/S0301-6226(98)00147-X
Rayner, A. C., Newberry, R. C., Vas, J., and Mullan, S. (2020). Slow-growing broilers are healthier and express more behavioural indicators of positive welfare. Sci. Rep. 10, 1–14. doi: 10.1038/s41598-020-72198-x
Real Decreto 53/2013. Por el que se establecen las normas básicas aplicables para la protección de los animales utilizados en experimentación y otros fines científicos, incluyendo la docencia. BOE de 1 de febrero de 2013 34, 11370–11421. Available online at: https://www.boe.es/eli/es/rd/2013/02/01/53.
Real Decreto 692/2010. Por el que se establecen las normas mínimas para la protección de los pollos destinados a la producción de carne y se modifica el Real Decreto 1047/1994, de 20 de mayo, relativo a las normas mínimas para la protección de terneros. BOE de 3 de Junio de 2010 135 47986–47995. Availble online at: https://www.boe.es/eli/es/rd/2010/05/20/692.
Shim, M. Y., Karnuah, A. B., Anthony, N. B., Pesti, G. M., and Aggrey, S. E. (2012). Genetics, the effects of broiler chicken growth rate on valgus, varus, and tibial dyschondroplasia. Poult. Sci. 91, 62–65. doi: 10.3382/ps.2011-01599
Tabler, G. T., Berry, I. L., Xin, H., and Barton, T. L. (2002). Spatial distribution of death losses in broiler flocks. J. Appl. Poult. Res. 11, 388–396. doi: 10.1093/japr/11.4.388
Tavárez, M. A., and Solis de los Santos, F. (2016). Impact of genetics and breeding on broiler production performance: a look into the past, present, and future of the industry. Anim. Front. 6, 37–41. doi: 10.2527/af.2016-0042
Torrey, S., Mohammadigheisar, M., Nascimento dos Santos, M., Rotchschild, D., Dawson, L. C., Liu, Z., et al. (2021). In pursuit of a better broiler: growth, efficiency, and mortality of 16 strains of broiler chickens. Poult. Sci. 100, 100955. doi: 10.1016/j.psj.2020.12.052
Ventura, B. A., Siewerdt, F., and Estevez, I. (2010). Effects of barrier perches and density on broiler leg health, fear and performance. Poult. Sci. 89, 1574–1583. doi: 10.3382/ps.2009-00576
Welfare Quality. (2009). The Welfare Quality® assessment protocol for poultry (broilers, laying hens). The Welfare Quality® Consortium, Lelystad, Netherlands.
Wilhelmsson, S., Yngvession, J., Jönsson, L., Gunnarsson, S., and Wallenbeck, A. (2019). Welfare Quality® assessment of a fast-growing and a slower-growing broiler hybrid, reared until 10 weeks and fed a low-protein, high-protein or mussel-meal diet. Livest. Sci. 219, 71–79. doi: 10.1016/j.livsci.2018.11.010
Keywords: chicken, genetics, house location, transect, welfare
Citation: Averós X, Nazar FN and Estevez I (2022) Animal Welfare Assessment: Quantifying Differences Among Commercial Medium and Fast Growth Broiler Flocks. Front. Anim. Sci. 3:868851. doi: 10.3389/fanim.2022.868851
Received: 03 February 2022; Accepted: 21 March 2022;
Published: 12 April 2022.
Edited by:
Suresh Neethirajan, Wageningen University and Research, NetherlandsReviewed by:
Rebecca E. Nordquist, Utrecht University, NetherlandsSabine G. Gebhardt-Henrich, University of Bern, Switzerland
Copyright © 2022 Averós, Nazar and Estevez. This is an open-access article distributed under the terms of the Creative Commons Attribution License (CC BY). The use, distribution or reproduction in other forums is permitted, provided the original author(s) and the copyright owner(s) are credited and that the original publication in this journal is cited, in accordance with accepted academic practice. No use, distribution or reproduction is permitted which does not comply with these terms.
*Correspondence: Xavier Averós, xaveros@neiker.eus; Inma Estevez, iestevez@neiker.eus