Dietary Pharmacological Zinc and Copper Enhances Voluntary Feed Intake of Nursery Pigs
- 1Department of Animal Science, Iowa State University, Ames, IA, United States
- 2Department of Veterinary Diagnostic and Production Animal Medicine, Iowa State University, Ames, IA, United States
- 3United States Department of Agriculture: Agricultural Research Service (USDA-ARS) National Laboratory for Agriculture and the Environment, Ames, IA, United States
- 4Zinpro Corporation, Eden Prairie, MN, United States
The objective of the three experiments herein were to characterize the effect of pharmacological zinc and copper concentrations on nursery pig feed intake, stomach ghrelin, energy and nutrient digestibility, and mineral retention in post-weaned pigs. In Expt. 1, 300 weaned pigs were allotted across three dietary treatments (n = 10 pens/treatment) and fed in two diet phases (P1 and P2) lasting 7 and 14 days, respectively. Treatments were: (1) Control diet with no pharmacological minerals in P1 and P2, CON; (2) CON + 3,000 mg/kg Zn and 200 mg/kg Cu (P1), no pharmacological minerals in P2, ZC-CON; and (3) CON + 3,000 mg/kg Zn and 200 mg/kg Cu (P1), CON + 2,000 mg/kg Zn and 200 mg/kg Cu (P2); ZC. Over the 21-day test period, ZC pigs had 15% higher ADG and 13–24% ADFI compared to the CON and ZC-CON pigs (P < 0.05). ZC-CON and ZC pig daily feed intakes were 29 and 73% higher by day 5 and 7 post-weaning, respectively, compared to the CON pigs (P < 0.0001). However, removing pharmacological minerals in P2 abruptly decreased ZC-CON daily feed intake within 24 h to similar intakes as the CON compared to the ZC pigs (0.17, 0.14, and 0.22 kg/d, respectively, P < 0.05). Dietary pharmacological minerals increased stomach fundus ghrelin-positive cells than CON pigs at day 7 (P = 0.005) and day 21 (P < 0.001). However, fasting plasma total and acyl-ghrelin concentrations did not differ from a control in response to zinc oxide daily drenching (Expt. 2). Expt. 3 showed that zinc and copper to have moderate to low retention; however, pharmacological zinc and copper diets increased zinc (P < 0.05) and copper retention (P = 0.06) after 28 days post-weaning compared to control pigs. Pharmacological zinc and copper did not improve digestible energy, metabolizable energy or nitrogen balance. Altogether, dietary pharmacological zinc and copper concentrations improve growth rates and mineral retention in nursery pigs. This improved performance may partially be explained by increased stomach ghrelin abundance and enhanced early feed intake in newly weaned pigs fed pharmacological concentrations of zinc and copper.
Introduction
Weaning is a critical period of pig production during which 19–28-day-old piglets are removed from the sow and littermates, transported to nursery facilities, and transitioned from milk to solid feed (Lalles et al., 2004). Commercial pigs are typically weaned during a critical period of gastrointestinal tract development, and the subsequent weaning stress can result in villus atrophy and crypt hyperplasia, as well as negative impacts on intestinal barrier function, intestinal absorption and secretion, immune system activation, and the enteric nervous system (Lalles et al., 2004; Moeser et al., 2017). Further, weaning stress commonly manifests a reduction in early voluntary feed intake (McCracken et al., 1995) and growth rates (Campbell et al., 2013). To combat this transition stress, newly weaned pigs are initially provided with highly palatable diets with readily digestible ingredients such as lactose and animal based proteins to maximize feed intake, energy and nutrient digestibility, and growth (Maxwell and Carter, 2001). Further, in many parts of the world, post-weaning diarrhea (PWD) further impairs nursery pig growth and health (Amezcua et al., 2002; Fairbrother et al., 2005; Rhouma et al., 2017). To combat PWD and enhance nursery pig performance, protocols in the United States and elsewhere use pharmacological concentrations of zinc and copper in diets. These two minerals, and especially zinc, are essential trace mineral for pigs, and serve as components of several metalloenzymes, providing structural integrity and/or participating in the enzymatic reaction (Gropper and Smith, 2013). To meet mineral requirements, zinc and copper are typically formulated in nursery pig diets at 80–100 and 5–6 mg/kg, respectively (NRC, 2012). However, pharmacological concentrations of zinc in pig diets are under intense scrutiny by the European Union and are being phased out due to antimicrobial resistance and environmental toxicity concerns (Yazdankhah et al., 2014; Debski, 2016).
Pharmacological concentrations of zinc can be quantified as amounts in a diet greater than the nutritional requirement of the pigs. Pharmacological zinc (1,000–3,000 mg/kg) is commonly added to the feed in the form of zinc oxide (ZnO) and is used to control PWD and improve growth rates (Poulsen, 1995; Carlson et al., 1999; Hill et al., 2000; Zhu et al., 2017). However, alternatives to pharmacological zinc in nursery pig diets are now desired. Though the mode of action by which pharmacological Zn improves nursery pig growth is not entirely clear, potential modes of action may include mitigating decreases in intestinal integrity and permeability (Zhang and Guo, 2009; Hu et al., 2013; Song et al., 2015) and modulating gut microbiota by reducing lactic acid bacteria and increasing coliform counts (Hojberg et al., 2005). Further, zinc is critical for the development and function of the immune system (Kloubert et al., 2018) where it has been hypothesized that pharmacological zinc may improve nursery pig growth via modulation of the immune system (Goswami et al., 2005) and by downregulating the mRNA expression of proinflammatory cytokines (Hu et al., 2013; Zhu et al., 2017). Pharmacological zinc has also consistently been associated with reduced incidence of PWD (Carlson et al., 2004; Ou et al., 2007; Heo et al., 2010), thereby improving nutrient utilization and water retention by young pigs. In addition to zinc, pharmacological concentrations of dietary copper (100–200 mg/kg copper) have consistently been shown to improve nursery pig growth performance and feed efficiency, irrespective of copper source (Cromwell et al., 1998; Ma et al., 2015). Further, copper is routinely formulated in North American nursery pig diets above requirement (~10–250 mg/kg) and this may also be in combination with pharmacological zinc to improve growth rates and reduce PWD (Flohr et al., 2016). Similar to zinc, the mode of action by which pharmacological coppers improved nursery pig growth is elusive. Dietary pharmacological copper has been shown to have antimicrobial properties (Hojberg et al., 2005) and increases anabolic hormone signaling (Wang et al., 2016) in nursery pigs.
One mode of action that has partially been examined is the orexigenic effects of zinc. Early work in nursery pigs reported that feeding high levels of zinc from ZnO (1,000–5,000 mg/kg) stimulated voluntary feed intake by 113% compared to pigs fed the basal diets (Hahn and Baker, 1993). Mechanistically, pharmacological zinc has been shown to stimulate stomach ghrelin secretions (Yin et al., 2009) and increase plasma ghrelin concentrations (Zhang and Guo, 2008) in young pigs. Altogether, these data suggest that pharmacological zinc augments early weaned pig growth via an orexigenic effect on voluntary feed intake. Thus, the objective of the study herein was to characterize the effect of pharmacological zinc and copper on early nursery pig voluntary feed intake, growth performance, energy and nutrients digestibility and balance. It was hypothesized that pharmacological zinc and copper would enhance voluntary feed intake and that this is driven by increased stomach ghrelin abundance and elevated plasma ghrelin concentrations in nursery pigs. Further, we hypothesized that pharmacological zinc and copper would increase nitrogen retention in nursery pigs. To assess these hypotheses three experiments were conducted in newly weaned nursery pigs.
Materials and Methods
All animal procedures were approved by the Iowa State University Institutional Animal Care and Use Committee (IACUC protocol #20-106, #7-17-8575-S, and #21-021) and adhered to the guidelines for ethical and humane use of animals for research.
Experimental Design, Animals, and Diets
Experiment 1
A total of 300 mixed sex pigs, weaned at ~19–24 d of age [5.70 ± 1.0 kg BW; Camborough (1050) × 337, Hendersonville, TN], were randomly selected to evaluate the effect of pharmacological zinc and copper on daily feed intake in newly weaned pigs. Pigs were randomly allocated across 30 pens with each pen containing five gilts and five barrows. The pens were then assigned to one of three dietary treatments that were fed over 2 phases (phase 1: d 0–7, phase 2: d 8–21). The dietary treatments were: (1) Control diet with no added pharmacological minerals (CON, n = 10), (2) CON + 3,000 mg/kg Zn and 200 mg/kg Cu (phase 1), and no pharmacological minerals in phase 2 (ZC-CON), and (3) CON + 3,000 mg/kg Zn and 200 mg/kg Cu in phase 1 and 2,000 mg/kg Zn and 200 mg/kg Cu in phase 2 (ZC). Pharmacological zinc was added in the form of ZnO and pharmacological copper was added in the form of copper sulfate at the expense of corn. The corn-soybean meal, mash-based diets were formulated to be isocaloric and isonitrogenous within phases 1 and 2, and met or exceeded nutrient requirements for this size pig based on (NRC, 2012) guidelines (Table 1). Diets were ad libitum fed throughout the 21-d experiment and water was offered at all times.
Individual pig body weight was recorded weekly (d 0, 7, 14, and 21) to calculate pen average daily gain (ADG). Pen feed disappearance was recorded daily from d 0 to 14 to determine daily feed intake, and feed disappearance recorded weekly between d 12 and 21 to determine average daily feed intake (ADFI) within each phase and overall (d 0–21). ADG and ADFI were used to determine feed efficiency (Gain:Feed, G:F).
On d 7 and 21, the median BW pig from half the pens in each treatment (n = 5 pigs per treatment) was chosen and euthanized via captive bolt followed by exsanguination. Thereafter, a liver sample (~10 g) was collected and snap frozen until trace mineral analysis was conducted at the Iowa State University Veterinary Diagnostic Laboratory (ISUVDL). Sections of stomach fundus, jejunum (~ 60 cm distal from the pyloric sphincter) and ileum (36 cm proximal from the ileal-cecal junction) were excised and fixed in 10% neutral buffered formalin for 24 h and then transferred to 70% ethanol and stored until further analysis.
Experiment 2
To test the hypothesis that ZnO administration will elevate fasting blood ghrelin concentrations in nursery pigs, 20 mixed sex pigs (7.7 ± 1.16 kg BW), 4 days post-weaning were randomly selected and placed in group pens. All pigs were fed the phase 1 control diet (Table 1) ad libitum and had free access to water at all times. On four consecutive mornings, all pigs received a 10 mL oral gavage of either tap water (CON; n = 10 pigs) or a ZnO slurry (ZN; containing 20 mg Zn/mL; n = 10 pigs). All pigs were fasted overnight on the third day and on the fourth day fasting blood samples (8 mL) were collected via jugular venipuncture into vacutainer plasma EDTA and serum collection tubes (BD, Franklin Lakes, NJ). Thereafter, pigs were euthanized by captive bolt, exsanguinated, and liver samples (~10 g) collected and frozen. Blood samples were centrifuged (2000 × g for 10 min at 4°C), plasma and serum collected, aliquoted, and stored at −80°C until analysis. Liver and serum samples were submitted to the ISUVDL for zinc and trace mineral analysis. Fasting total ghrelin (TSZ ELISA, Framingham, MA) and acylated ghrelin (Bertin Corp., Rockville, MD) concentrations were determined using commercially available ELISA kits according to manufacturer's instructions. Total ghrelin was reported in ng/mL, while acylated ghrelin was reported in pg/mL.
Experiment 3
Experiment 3 examined the effects of pharmacological zinc and copper on nutrient and energy digestibility in nursery pigs. One hundred weaned barrows and gilts (BW 6.36 ± 0.85 kg) where randomly assigned to 20 pens (mixed sex; 5 pigs/pen). Pens were then randomly assigned to one of two dietary treatments: (1) Control with no growth-promoting additives (CON) and (2) CON + pharmacological zinc and copper (ZC). Diets (Table 2) were fed in 2 phases in the nursery and pharmacological miner concentration was reduced each phase to avoid toxicity (Burrough et al., 2019): phase 1 (d 0–14) with ZC zinc and copper at 2,000 and 200 mg/kg, respectively, and phase 2 (d 15–28) with ZC zinc and copper at 1,600 and 57 mg/kg, respectively. Zinc and copper concentrations in the ZC diets were reduced between phases 1 and 2 to avoid any mineral toxicity and negative growth effects that could occur feeding beyond 28 days (Burrough et al., 2019). Diets within each phase were formulated to meet or exceed the nutrient requirements for this size pig (NRC, 2012). On d 28, 16 barrows were randomly selected (n = 8/treatment; BW 12.75 ± 1.04 kg) and moved into individual metabolism crates (0.53 × 0.71 m). While in metabolism crates, barrows continued eating the phase 2 treatment diets (Table 2) which contained titanium dioxide as an indigestible marker. Pigs were fed twice daily, equivalent to 3.5% of their average BW at d28 (~85% of ad libitum) that consisted of 225 g of feed at 0700 h and 225 g of feed at 1700 h, with free access to water. Any feed refusal was recorded each day.
After a 3-d adaptation period to the metabolism crate, a 3-d total collection period was undertaken. Over this collection period, urine and feces were collected to determine apparent total tract digestibility (ATTD) and balance of GE, N, Zn, and Cu as previously described (Kerr et al., 2009). Briefly, total urine and feces were collected twice daily during the collection period. To limit microbial growth and ammonia loss, 10 mL of 6 N HCl was added to urine collection containers. During the collection period, urine and feces was pooled within pig and stored at −20°C. Thereafter, urine was thawed, weighed to determine output, and then a subsample was collected, filtered, and stored at −20°C for further analysis. Pooled fecal samples were dried at 70°C for 48 h, weighed, ground through a 2-mm screen, and stored for further analysis.
Trace Mineral Analysis
For serum and liver trace mineral analysis, samples were analyzed for zinc and copper using inductively coupled plasma-mass spectrometry (ICP-MS; Analytik Jena, Wodburn, MA) in collisional reaction interface mode with hydrogen as the skimmer gas. Liver samples were processed and analyzed on a wet tissue basis per laboratory standard operating protocols as described by Burrough et al. (2019). Briefly, 1.0 g of sample was weighed into a 50 mL digestion tube and 10 mL of 70% nitric acid was added. The samples were digested in a microwave digester, and then filtered twice (Whatman #40 filter paper, GE Healthcare Life Sciences, Buckinghamshire, UK) and diluted to 25 mL with 18 MΩ water. A final 1:10 dilution was achieved using 1% nitric acid and analyzed by ICP-MS. Analysis of zinc and copper in feed, feces, and urine was determined using Method 985.01 (AOAC International, 2005) for inductively coupled plasma spectrometry sample digestion. Briefly, ~2 g of feed and ~0.3 g of fecal samples were dry-ashed to remove organic matter. Urine, ashed feed and fecal samples were then treated with 16 M nitric acid to remove any remaining organic matter. All zinc and copper concentrations were reported as mg/kg on a wet-weight basis after digestion or on a dry matter basis for digestibility and retention.
Intestinal Morphology
Formalin fixed jejunal and ileal sections were paraffin-embedded into blocks, sectioned and stained with hematoxylin and eosin for evaluation of intestinal morphology as described by Helm et al. (2020). Briefly, images were taken at 20X magnification using a DP80 Olympus Camera mounted on an OLYMPUS BX 53/43 microscope (Olympus Scientific, Waltham, MA). Fifteen to twenty well-orientated villus and crypt pairs were measured for height and depth, respectively, in μm using OLYMPUS CellSens Dimension 1.16 software (Olympus Scientific, Waltham, MA). Villus to crypt ratios were also calculated and reported.
Immunohistochemistry
The abundance of ghrelin in stomach sections were assessed as described by Paxton et al. (2014). Briefly, a commercial monoclonal mouse anti-ghrelin antibody (Cat. No. MAB10404; Millipore, Billerica, MA) was used for immunohistochemistry (IHC) to assess presence of ghrelin in fundic cells. IHC was performed on a BOND RX fully automated stainer (Leica Biosystems, Buffalo Grove, IL, USA) using the manufacturer's recommended protocol (Protocol F). The primary antibody was diluted 1:2,000 in a commercial diluent (BOND Primary Antibody Diluent) and was detected with a commercial secondary antibody (PowerVision Poly-HRP Anti-Mouse IgG, Leica Biosystems, Buffalo Grove, IL, USA). Slides were imaged at 20X magnification as described for morphology measurements for a total of 10 images per pig throughout the fundus. Images were imported into and analyzed in HALO image analysis software (HALO™. Indica Labs, Inc., Corrales, NM, USA). Briefly, for each image, a 10,138 μm2 section of fundic tissue was outlined as the region of interest (ROI), and the software then counted both the total cells, and stained cells in each ROI. Ghrelin-positive fundic cells were reported as number of ghrelin-positive cells per area.
Energy and Nutrient Apparent Total Tract Digestibility and Balance
Gross energy (GE) of the feed, feces, and urine was determined using an isoperbolic bomb calorimeter (Model 6200, Parr Instrument Company, Moline, IL) using benzoic acid as a standard. For feed and fecal samples, ~1.0 g of sample was pelleted prior to determination of GE. For analysis of urine, 0.5 g of dried cellulose was weighed into a crucible, and 1 mL of filtered urine was pipetted onto the cellulose. Crucibles were dried for 24 h at 50°C. This process was repeated 2 additional times for a total of 3 mL of urine dried onto 0.5 g of cellulose. For calculation of urine GE, GE of dried cellulose was determined and subtracted from the determined GE of urine samples. The GE of the diet was then multiplied by the total feed intake over the 3-d collection period and divided by the days on feed to determine daily GE intakes. Apparent digestible energy (DE) was calculated by subtracting the GE of feces from the GE of intake. Metabolizable energy (ME) was calculated by subtracting urinary GE from apparent DE and was reported as a percent of DE. Nitrogen content of feed, feces, and urine was determined using a thermo-combustion analyzer (Variomax; Elementar Analysensysteme GmbH, Hanau, Germany). Zinc and copper were assayed in feed and feces as described above. Apparent total tract digestibility of Zn and Cu is reported a percent of intake, and retention was reported as a percent of digested Zn or Cu. Apparent total tract digestibility (ATTD) coefficients and nutrient and energy retention were calculated as previously described (Adeola, 2001). Furthermore, nitrogen digestibility was reported as both a percent of intake as well as total grams absorbed (N intake g—fecal N g), and N retention was reported as a percent of digestible N and total grams retained (N Absorbed g—urinary N g).
Statistical Analysis
Statistical analysis of all data was performed in SAS 9.4 (SAS Institute, Cary, NC). The following mixed model was fitted to quantitative parameters for performance, trace mineral analysis, intestinal morphology, blood parameters, ATTD and nutrient retention data:
wherein Yij = the phenotype measured on pen (Expt. 1 performance) or animal (Expt. 1, 2, and 3) j; Ti = fixed effect of treatment (Expt. 1: CON, ZC-CON, ZC; Expt. 2: CON, ZN; Expt. 3: CON, ZC); and eij = error term of pen or animal j subjected to treatment i eij ~ N(0, ).
The following mixed model was fitted to quantitative parameters for daily feed intake (Expt. 1):
wherein Yijk = the phenotype measured on pen k; Ti = fixed effect of treatment (Expt. 1: CON, ZC-CON, ZC); and eij = error term of animal k subjected to treatment i eij ~ N(0, ). For daily feed intake data, pen k at day j was used as a repeated measure.
Least square means were determined using the LS means statement and data are presented as Least Squares means with a pooled standard error. For all analyses, differences were considered significant when P < 0.05 and a tendency when 0.05 ≤ P ≤ 0.10.
Results
Experiment 1
Experiment 1 was designed to evaluate the effect of pharmacological zinc and copper on daily feed intake and stomach ghrelin abundance in newly weaned pigs. Weaned pigs were fed diets with or without growth-promoting pharmacological levels of zinc and copper for 21 d and the performance data is reported in Table 3. In the ZC-CON treatment group, pharmacological minerals were only fed for the first 7 d. By d 7 post-weaning, the ZC and ZC-CON pigs were 7% heavier than the CON (P = 0.037). At the end of phase 2 (d 21) the ZC pigs were 10% heavier than the CON pigs, with the ZC-CON treatment being intermediate (P = 0.050). Over the first 14 d post-weaning, daily feed disappearance was assessed (Figure 1). As expected, daily feed intake increased significantly over the test period (P < 0.0001). Accordingly, and in agreement with our hypothesis, the ZC-CON and ZC pigs had 29% to 73% higher daily feed intakes compared to CON pigs between day 5 and 7 (P < 0.05). Interestingly, following the phase 2 diet change at d 7 from pharmacological zinc and copper to the CON treatment diet in the ZC-CON pigs, d 8 feed intake immediately and significantly decreased to similar amounts to that of the CON pigs, while the ZC feed intake remained greater (0.14 and 0.17 vs. 0.22 kg, respectively, P = 0.001, Figure 1). These data suggest an orexigenic effect of zinc oxide and which was confirmed in phase 1 where there was a 29% increase in ADFI in the ZC-CON and ZC pigs compared to the CON pigs (P = 0.007, Table 3). Phase 2 ADFI was also increased 17% in the ZC treatment compared to the CON and ZC-CON pigs (P = 0.016, Table 3). Overall (d 0–21), we reported a 13 and 24% increase in ADFI in the ZC pigs compared to the ZC-CON and CON pigs, respectively (P = 0.012, Table 3).
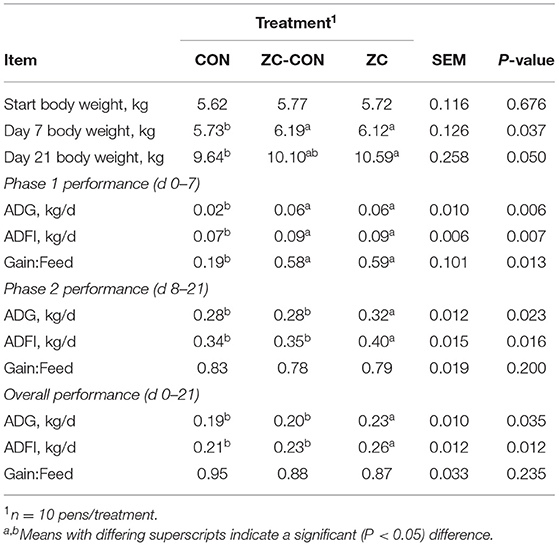
Table 3. Growth performance of nursery pigs fed a control diet for 3 weeks (CON), a diet with 3,000 mg/kg Zn for 1 week followed by a control diet for 2 weeks (ZC-CON), and a diet with 3,000 mg/kg Zn for 1 week followed by a diet with 2,000 mg/kg Zn for 2 weeks (ZC) (Expt. 1).
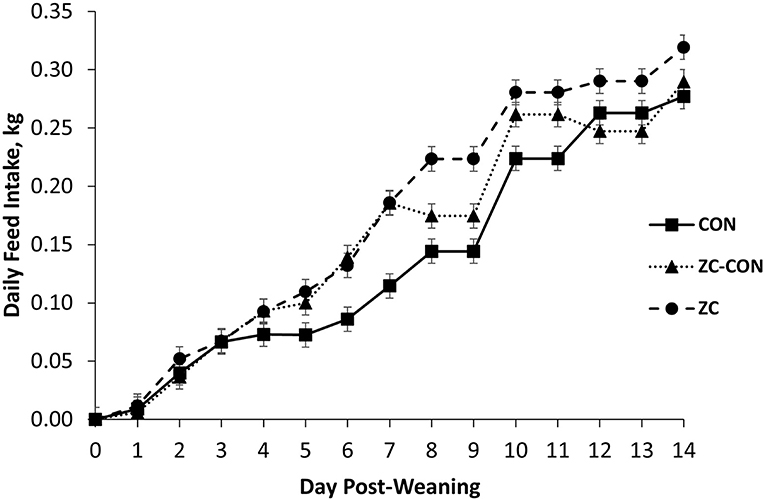
Figure 1. Post-weaning daily feed intake in nursery pigs fed diets containing no pharmacological zinc or copper (CON), pharmacological zinc and copper (ZC) or phase 1 (day 0–7) diets containing pharmacological zinc and copper, and CON in phase 2 (day 8–14; ZC-Con). Day P < 0.0001, Treatment P < 0.0001 and Day × Treatment P < 0.0001. n = 10 pens/treatment.
In support of the body weight and feed intake findings, the ZC-CON and ZC pigs had an ADG of 0.06 kg/d compared to 0.02 kg/d in CON pigs in phase 1 (P = 0.0006, Table 3). In phase 2, continuous pharmacological dietary zinc (ZC) pig ADG was increased over the CON and ZC-CON pig treatments by 17% (P = 0.023). Overall (d 0–21), ZC pigs exhibited a 15 and 21% increase in ADG compared to ZC-CON and CON pigs, respectively (P = 0.035, Table 3). Feed efficiency as determined by G:F, was not different among treatments in phase 2 or overall (P > 0.05). However, ZC and ZC-CON pig G:F was increased 210% from the CON in phase 1 (P = 0.013, Table 3).
The ability of the diets to enrich serum and liver zinc concentrations are reported in Table 4. Unsurprisingly, pharmacological zinc and copper resulted in increased liver zinc concentrations at d 7 and 21 in the ZC-CON and ZC pigs compared to the CON pigs (P < 0.0001 and P = 0.0142, respectively). However, liver Cu concentrations tended to be reduced in the ZC-CON and ZC pigs compared to CON pigs at d 7 (P = 0.073). By d 21, ZC pigs had increased liver Cu concentrations compared to ZC-CON and CON pigs (P = 0.006).
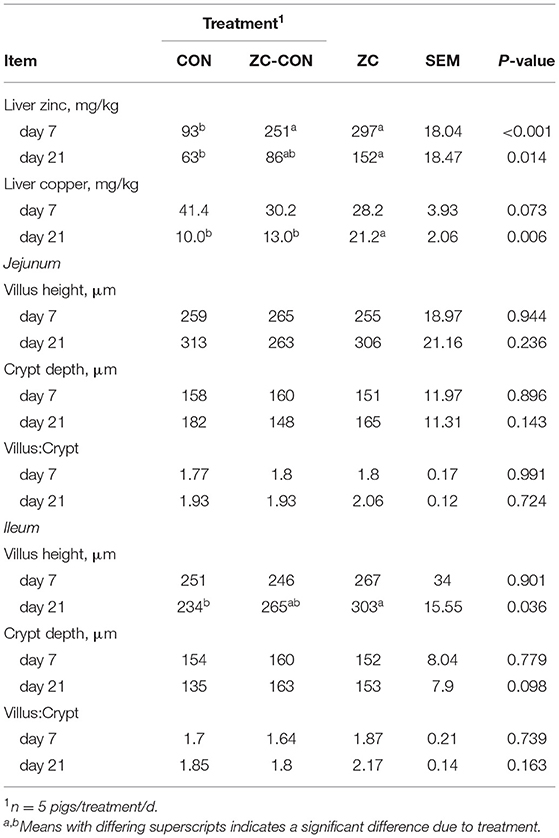
Table 4. Liver zinc concentrations, jejunum and ileum morphology and ghrelin positive fundic cells at d 7 and d 21 in pigs fed a control (CON) diet for 21 d, a diet containing pharmacological zinc and copper for 7 d followed by a control diet for 14 d (ZC-CON), or a diet containing pharmacological zinc and copper for 21 d (ZC) (Expt. 1).
Jejunum and ileum morphology were assessed at d 7 and 21 (Table 4). No jejunal villus height, crypt depth, or villus to crypt ratio difference between treatments were observed at d 7 or d 21. Additionally, no ileum morphology differences at d 7 were reported between treatments. However, ZC pig ileum villi lengths were 25% longer at d 21 compared to the CON pig (P = 0.036), with ZC-CON pigs being intermediate. Further, there was tendency for crypt depth to be increased at d 21 in the ZC and ZC-CON treatments compared to CON pigs (P = 0.098, Table 4).
To test the hypothesis that dietary pharmacological zinc augments stomach ghrelin abundance, ghrelin-positive stomach fundic cells were assessed by IHC at d 7 and d 21 (Figures 2, 3). The abundance of ghrelin-positive fundic cells per area was greater in ZC-CON pigs compared to CON pigs, with ZC pigs being intermediate at d 7 (P = 0.005, Figure 2). At d 21, the abundance of ghrelin-positive cells per area in ZC pigs was greater than ZC-CON pigs, and ZC and ZC-CON pigs exhibited a greater abundance of ghrelin-positive cells per area than CON pigs (P < 0.001, Figure 2).
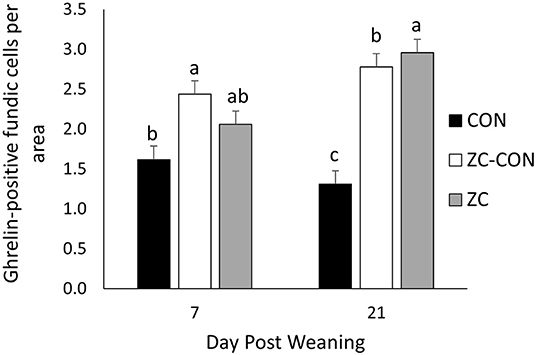
Figure 2. Ghrelin-fundic positive cells between the CON, ZC-CON, and ZC treatment groups at d 7 and 21. At d7, pigs in the ZC-CON group exhibited more ghrelin-positive cells per area (10,138 μm2) than the CON pigs (2.44 vs. 1.62 cells, respectively, P = 0.005), with ZC pigs being intermediate (2.06 cells). At d 21, pigs in the ZC group exhibited 126% more ghrelin-positive cells per area compared to the CON pigs and 61% more ghrelin-positive cells per area compared to the ZC-CON pigs (P < 0.001).
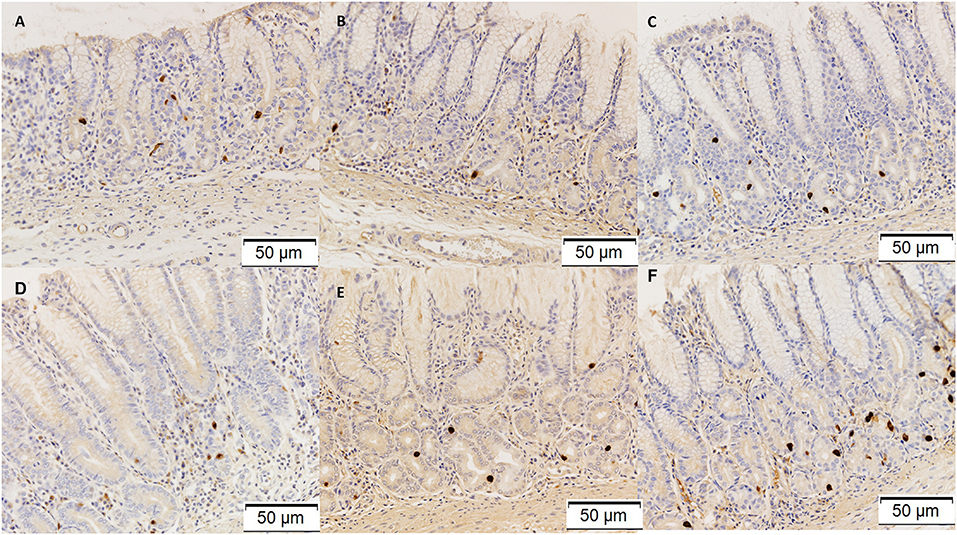
Figure 3. Representative immunohistochemistry staining for ghrelin (brown) in the fundus in post-weaned pigs fed without or with pharmacological zinc and copper. Images (A–C) represents day 7 and (D–F) represents day 21 post weaned stomach samples. Pigs were fed a control diet in both phases [CON, images (A,D)]. Images (B,E) are stomach sections from pigs were fed pharmacological zinc and copper in phase 1, and a control diet in phase 2 (ZC-CON). Pigs were fed pharmacological zinc and copper in both phases (ZC) are represented in images (C,F).
Experiment 2
To further assess the specific effects of pharmacological zinc on feed intake regulation, nursery pigs were given a bolus drench of ZnO daily for 4 days. On d 4, blood samples were collected to evaluate the ability of zinc to increase fasting ghrelin concentrations. As expected, short term ZnO administration increased both serum and liver zinc concentrations by 200 and 119%, respectively, compared to the controls (P ≤ 0.05; Table 5). However, fasting plasma total and acyl-ghrelin concentrations did not differ between treatments.
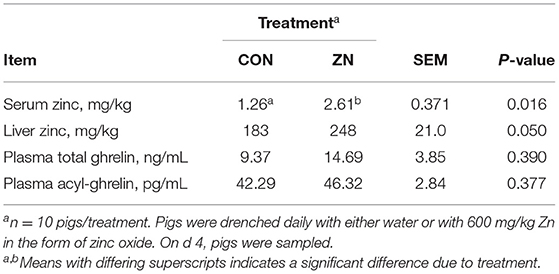
Table 5. Plasma total ghrelin and acyl-ghrelin of pigs in a fasted state following 4 d of a zinc oxide bolus (ZN) or a saline bolus (CON) drench (Expt. 2).
Experiment 3
This experiment evaluated the effect of pharmacological zinc and copper on nutrient digestibility and retention. Following 28 d of feeding pharmacological zinc and copper, total intake and excretion of energy, nitrogen, zinc, and copper were assessed (Table 6). Although not different between the CON and ZC pigs (P > 0.05), dietary copper and zinc retention as a percent of intake was low at ~54–59% and 36–42%, respectively. However, ZC pigs had increased zinc retention as a percent of digested Zn compared to the CON treatment group (P = 0.048), and copper retention tended to be increased as well (P = 0.060). In terms of mineral balance, both absorbed and retained zinc and copper were greater in the ZC pigs compared to the CON pigs (P < 0.0001). There were no treatment effects on DE as a percent of GE or on ME as a percent of DE. Nitrogen intake and absorption (g/d) did not differ between the CON and ZC treatments. Urinary and fecal nitrogen excretions and nitrogen retention did not differ between treatments (P > 0.05, Table 6).
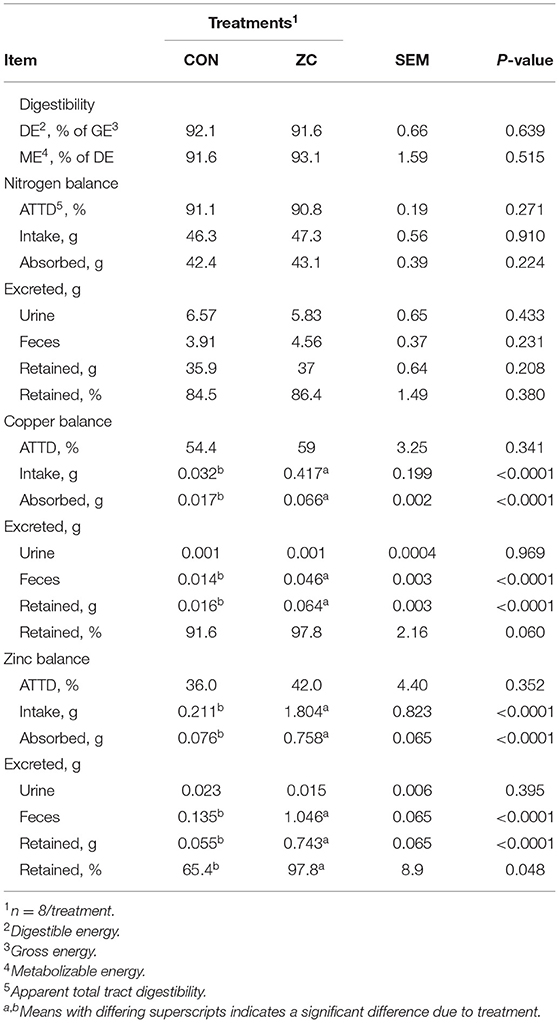
Table 6. Energy digestibility, mineral digestibility, and Nitrogen balance of pigs fed a control diet (CON) vs. pigs fed a diet containing pharmacological levels of Zn and Cu (ZC) (Expt. 3).
Discussion
The negative impacts of weaning stress ultimately lead to reduced voluntary feed intake that contributes to the observed decrease in growth rates (Campbell et al., 2013). To encourage nursery pig post-weaning feed intake, phase feeding programs are typically implemented with highly palatable and easily digestible ingredients that meet the pig's nutrient and energy needs. According to the NRC (2012), the recommended dietary inclusion of zinc for nursery pigs is ~80 mg/kg; however, in many parts of the world zinc is commonly added at supra-nutritional or pharmacological concentrations (i.e., >1,000 mg/kg) to the nursery diet in the form of ZnO to improve growth rates and control PWD (Poulsen, 1995; Carlson et al., 1999; Hill et al., 2000; Sales, 2013; Zhu et al., 2017). In addition to zinc, pharmacological concentrations of dietary copper (i.e., 100–200 mg/kg copper) are also routinely used to improve nursery pig growth performance and feed efficiency in combination with pharmacological zinc (Ma et al., 2015). However, high concentrations of zinc and copper in pig diets has come under intense scrutiny due to antimicrobial resistance and environmental toxicity concerns (Yazdankhah et al., 2014; Debski, 2016). As such, alternatives are desired and the mode of action by which pharmacological minerals (i.e., zinc and copper) improves growth in weaned pigs warrants explanation. There is extensive literature regarding possible modes of action of pharmacological zinc including mitigating the impact of weaning stress on intestinal integrity and permeability (Zhang and Guo, 2009), modulation of the immune system (Goswami et al., 2005), alteration of the gut microbiota (Hojberg et al., 2005) and reduced incidence of PWD (Poulsen, 1995; Zhu et al., 2017). Similar to zinc, pharmacological copper has been shown to have antimicrobial properties (Hojberg et al., 2005) and increases anabolic hormone signaling (Wang et al., 2016) in nursery pigs. A relatively under-investigated area, as it relates to both weaning stress and mode of action of pharmacological minerals, is the orexigenic or appetite regulation potential of therapeutic zinc and copper. However, unlike zinc (Yin et al., 2009), copper does not appear to have any orexigenic effects that enhance appetite. As early feed intake is critical for weaning transition (McCracken et al., 1995), the objective of the study was to characterize the effect of pharmacological zinc and copper on early nursery pig voluntary feed intake, growth performance, digestibility and nitrogen balance.
In nursery pig diets containing pharmacological zinc concentrations, improvements in ADG and ADFI has been routinely reported (Hahn and Baker, 1993; Carlson et al., 1999; Hill et al., 2000; Mavromichalis et al., 2000; Yin et al., 2009). The growth performance results herein agree with this body of work, in which 3,000 and 2,000 mg/kg zinc in the form of ZnO improved early nursery pig growth in both phase 1 and phase 2, respectively. A primary driver of growth is the supply of energy and nutrients to support lean tissue and bone accretion. Thus, we hypothesized that pharmacological zinc would enhance voluntary feed intake and that this orexigenic phenotype is a result of increased stomach ghrelin abundance and elevated plasma ghrelin concentrations. Although previous work reports that reduced feed intake in conjunction with weaning stressors contributes to reduced growth, and adequate feed intake following weaning may counteract the deleterious effects of weaning (McCracken et al., 1995), early feed intake has been poorly characterized in post-weaned pigs. In the current study (Expt. 1), daily feed intake of pigs fed pharmacological zinc was significantly higher compared to CON pigs for the first 14-d period. This increased voluntary feed intake was particularly evident between d 4 and d 14 post-weaning. Interestingly, when pigs were switched to phase 2 diets containing no pharmacological zinc (ZC-CON), within 24 h voluntary feed intake was attenuated by 27% from the ZC pigs. Thereafter, ZC-CON feed intakes were similar to the CON. These findings are indicative of a potential mode of action of pharmacological Zn that is modulating early post-weaning voluntary feed intake.
The ability of dietary zinc to enhance voluntary feed intake may be through the orexigenic signaling action of ghrelin. The ghrelin hormone, a peptide hormone found in the X/A-like cells in the oxyntic mucosa of the gastric fundus, and is secreted into the circulation (Date et al., 2000). Two major forms of ghrelin are found in the stomach and plasma: the acylated form and the desacylated form (Hosoda et al., 2000). The expression and eventual secretion of ghrelin is increased by fasting and reduced by meal intake (Kojima and Kangawa, 2002). In its acylated form, this peptide crosses the blood brain barrier and can act on orexigenic neurons to enhance appetite stimulation (Wren et al., 2000; Asakawa et al., 2001; Cowley et al., 2003). In the brain, the arcuate nucleus of the hypothalamus contains two distinct neuronal populations: anorexigenic neurons and orexigenic neurons (Sohn, 2015) which are regulated by hormones to voluntary feed intake via control of hunger and satiety (Small and Bloom, 2004). Interestingly, ZnO has been shown to increased ghrelin secretion in cultured gastric mucosal cells (Yin et al., 2009). Furthermore, oral administration of zinc to mildly zinc-deficient rats stimulated food intake within 20 min, and increased hypothalamic neuropeptide Y and orexin mRNA expression (Ohinata et al., 2009). Ohinata et al. (2009) additionally found that oral zinc administration did not stimulate feed intake in vagotomized rats, indicating that the effect of zinc on feed intake is likely mediated through the afferent vagus nerve. It has been shown that the orexigenic action of ghrelin is also abolished in vagotomized rodents (Asakawa et al., 2001; Inui et al., 2004; Ohinata et al., 2009); thus, the orexigenic action of zinc appears to be related with ghrelin signaling (Suzuki et al., 2011). Interestingly, the literature is sparse in regards to direct appetite or orexigenic effects of dietary copper. As such, we speculate that the appetite responses presented herein may predominantly be a result of dietary pharmacological zinc administration.
In weaned pigs, pharmacological zinc has been shown to elevate plasma acylated and serum total ghrelin (Zhang and Guo, 2008; Yin et al., 2009). In the current study, quantification of ghrelin-positive cells in the fundus of the stomach using IHC, indicated that the inclusion of pharmacological minerals increased the abundance of ghrelin-positive cells per unit area. This was not surprising, as feed intake was increased significantly due to the inclusion of pharmacological minerals by d 4, and this increase was maintained during the study. To further test the hypothesis of pharmacological zinc enhancing ghrelin in post-weaned pigs, a second experiment was undertaken. For 4 d, pigs were given a bolus drench of either 200 mg/d zinc in the form of ZnO or a water control. Contrary to the previous reports that showed fasting plasma acyl-ghrelin and serum total ghrelin to be increased in nursery pigs in response to dietary pharmacological zinc (Zhang and Guo, 2008; Yin et al., 2009), herein we were unable to show any significant augmentation of fasting plasma total and acylated ghrelin concentrations after the daily ZnO bolus treatments. However, evaluation of serial post-prandial ghrelin concentration changes post bolus of ZnO is needed to truly understand the signaling interaction between zinc and ghrelin. In addition to its orexigenic actions, circulating ghrelin is the endogenous ligand for growth hormone secretagogue receptor 1a, which stimulates the release of growth hormone (Kojima et al., 1999; Chanoine, 2005; Khatib et al., 2014). Although not evaluated herein, the increase in ADG reported in response to ZnO could be via this anabolic signaling mechanism.
Weaning is commonly associated with changes in small intestinal morphology, an indirect measure of nutrient absorptive capacity, such reduced villus height, and increased crypt depth (Hampson, 1986; Cera et al., 1988; van Beers-Schreurs et al., 1998; Liu et al., 2002; Brown et al., 2006; Montagne et al., 2007). Villus atrophy and crypt hyperplasia are indicative of reduced absorptive capacity in the small intestine (Pluske et al., 1997), where it is thought that the reduced nutrients available in the lumen of the intestine due to reduced feed intake at weaning contributes to the altered morphology (Cera et al., 1988; McCracken et al., 1995; Pluske et al., 1996; Spreeuwenberg et al., 2001). Our group has previously reported that villus lengths in the ileum are positively correlated with both ADG and ADFI at d 7 and 21 post-weaning (De Mille and Gabler, 2021). In the present study, despite differences in feed intake, morphology did not differ across dietary treatment at d 7. These results are in agreement with Hedemann et al. (2006), who reported that 2,500 mg/kg zinc did not significantly alter intestinal morphology measures in nursery pigs. However, it has been reported that pharmacological zinc can be beneficial to small intestinal morphology, particularly in the case of increased villus lengths (Li et al., 2001; Hu et al., 2013; Wang et al., 2017). In agreement, in the current study by 21 days post-weaning, ileal villus height was increased in pigs fed continuous pharmacological minerals.
The physiological changes in the gastrointestinal tract at weaning can also antagonize digestion and absorption, reducing growth performance of nursery pigs (Boudry et al., 2004; Lalles et al., 2004). Therefore, ATTD and balance of GE, N, zinc, and copper were determined to evaluate digestive function. Herein, it was observed that treatment did not impact DE, ME, or N balance in 28-day post-weaned nursery pigs. However, pharmacological minerals did significantly increase zinc retention of absorbed zinc and tended to increase copper retention of absorbed copper. These data are in partial agreement with these results, Buff et al. (2005) observed that 2,000 mg/kg zinc numerically increases the retention of zinc in late nursery pigs. Further, the retention of zinc and copper from the diet ranges from 5 to 40% (Combs et al., 1966; Houdijk et al., 1999; Rincker et al., 2005), and it is worth noting here, that in the present study zinc absorption ranged between 36 and 42%, while copper absorption ranged between 54 and 59%. This poor absorption and retention rate highlights the concerns regarding excess minerals in manure becoming an environmental contaminant when applied to soil (Payne et al., 1988; Anderson et al., 1991). Despite this low retention, liver and serum zinc and copper concentrations were increased by dietary pharmacological concentrations of zinc and copper and support what has previously been reported in similar age pigs (Burrough et al., 2019). In terms of ATTD, Dove (1995) reported that the addition of 250 mg/kg copper increased ATTD of fat, dry matter, and ash, as well as retention of energy and N. However, in nursery pigs fed diets containing 1,500 or 2,500 mg/kg zinc, decreased digestibility of dry matter, crude protein, calcium, and phosphorous, with no impact on energy, were reported when compared to a control diet (Han and Thacker, 2010). This is in contrast to Lei and Kim (2018), whom reported increased ATTD of DM and N in nursery pigs fed 2,500 mg/kg zinc. Despite these conflicting reports, improved growth performance in nursery pigs fed pharmacological zinc and copper may not be related to changes in nutrient digestibility.
In conclusion, the data presented herein show that pharmacological mineral inclusion in the nursery pig diet promotes growth in the face of weaning stress. Feed intake was determined daily for 14 d, and most interestingly, a significant reduction in feed intake occurred within 24 h of removing pharmacological minerals from the diet. Although we cannot rule out the orexigenic contribution of pharmacological dietary copper on augmenting feed intake post-weaning, based on previous work linking ghrelin and zinc (Zhang and Guo, 2008; Ohinata et al., 2009; Yin et al., 2009), we hypothesize that pharmacological zinc primarily enhances voluntary feed intake of nursery pigs via ghrelin production in the gastric mucosa. The abundance of ghrelin-positive fundic cells per area were increased at both d 7 and d 21 due to the inclusion of pharmacological minerals, supporting our hypothesis. However, pharmacological mineral inclusion did not alter plasma total or acylated ghrelin at either d. Pharmacological minerals did increase villus heights in the ileum at d 21, but aside from the improvements in zinc and copper retention, nutrient digestibility was not augmented by treatment. Therefore, pharmacological minerals augmented voluntary feed intake in nursery pigs, and appears to be related to ghrelin abundance in the fundus.
Data Availability Statement
The raw data supporting the conclusions of this article will be made available by the authors, without undue reservation.
Ethics Statement
The animal study was reviewed and approved by Iowa State University Institutional Animal Care and Use Committee.
Author Contributions
CD, BK, and NG performed live animal experiments. CD, BK, EB, and NG performed laboratory experiments. CD, WS, BK, EB, and NG wrote the manuscript. All authors contributed to the design of the study, interpretation of the results, and assisted with manuscript editing.
Funding
Funding for this research was provided by the USDA National Institute of Food and Agriculture, Agriculture and Food Research Initiative Competitive Grant #2019-67015-29486. The funders were not involved in study design, data collection, analysis, and interpretation of data, writing the manuscript, or decision to submit the article for publication.
Conflict of Interest
WS is employed by Zinpro Corporation.
The remaining authors declare that the research was conducted in the absence of any commercial or financial relationships that could be construed as a potential conflict of interest.
Publisher's Note
All claims expressed in this article are solely those of the authors and do not necessarily represent those of their affiliated organizations, or those of the publisher, the editors and the reviewers. Any product that may be evaluated in this article, or claim that may be made by its manufacturer, is not guaranteed or endorsed by the publisher.
Acknowledgments
The authors would like to thank Trey Faaborg and Tim Hicks at the ISU Swine Nutrition Farm for help with the pig work. The authors would also like to thank Dr. Emma Helm, Kayla Miller, and the undergraduate students of the Gabler Laboratory for aiding in sample collection and slide imaging.
References
Adeola, O.. (2001). “Digestion and balance techniques in pigs,” in Swine Nutrition, 2nd Edn., eds A. J. Lewis and L. L. Southern (New York, NY: CRC Press), 903–916.
Amezcua, R., Friendship, R. M., Dewey, C. E., Gyles, C., and Fairbrother, J. M. (2002). Presentation of postweaning Escherichia coli diarrhea in southern Ontario, prevalence of hemolytic E. coli serogroups involved, and their antimicrobial patterns. Can. J. Vet. Res. 66, 73–78.
Anderson, M. A., McKenna, J. R., Martens, D. C., Donohue, S. J., Kornegay, E. T., and Lindemann, M. D. (1991). Long-term effects of copper rich swine manure application on continuous corn production. Commun. Soil Sci. Plant Anal. 22, 993–1002. doi: 10.1080/00103629109368468
AOAC International. (2005). Official Methods of Analysis of AOAC International, Official Method 985.01. Gaithersburg, MD: AOAC.
Asakawa, A., Inui, A., Kaga, O., Yuzuriha, H., Nagata, T., Ueno, N., et al. (2001). Ghrelin is an appetite-stimulatory signal from stomach with structural resemblance to motilin. Gastroenterology 120, 337–345. doi: 10.1053/gast.2001.22158
Boudry, G.l, Pron, V., Le Hrou-Luron, I., Lalls, J. P., and Sve, B. (2004). Weaning induces both transient and long-lasting modifications of absorptive, secretory, and barrier properties of piglet intestine. J. Nutr. 134, 2256–2262. doi: 10.1093/jn/134.9.2256
Brown, D. C., Maxwell, C. V., Erf, G. F., Davis, M. E., Singh, S., and Johnson, Z. B. (2006). The influence of different management systems and age on intestinal morphology, immune cell numbers and mucin production from goblet cells in post-weaining pigs. Vet. Immunol. Immunopathol. 111, 187–198. doi: 10.1016/j.vetimm.2005.12.006
Buff, C. E., Bollinger, D. W., Ellersieck, M. R., Brommelsiek, W. A., and Veum, T. L. (2005). Comparison of growth performance and zinc absorption, retention, and excretion in weanling pigs fed diets supplemented with zinc-polysaccharide or zinc oxide. J. Anim. Sci. 83, 2380–2386. doi: 10.2527/2005.83102380x
Burrough, E. R., De Mille, C., and Gabler, N. K. (2019). Zinc overload in weaned pigs: tissue accumulation, pathology, and growth impacts. J. Vet. Diagn. 31, 537–545. doi: 10.1177/1040638719852144
Campbell, J. M., Crenshaw, J. D., and Polo, J. (2013). The biological stress of early weaned piglets. J. Anim. Sci. Biotechnol. 4, 19. doi: 10.1186/2049-1891-4-19
Carlson, D., Poulsen, H. D., and Sehested, J. (2004). Influence of weaning and effect of post weaning dietary zinc and copper on electrophysiological response to glucose, theophylline and 5-HT in piglet small intestinal mucosa. Comp. Biochem. Physiol. Part A Mol. Integr. Physiol. 137, 757–765. doi: 10.1016/j.cbpb.2004.02.011
Carlson, M. S., Hill, G. M., and Link, J. E. (1999). Early- and traditionally weaned nursery pigs benefit from phase-feeding pharmacological concentrations of zinc oxide: effect on metallothionein and mineral concentrations. J. Anim. Sci. 77, 1199–1207. doi: 10.2527/1999.7751199x
Cera, K. R., Mahan, D. C., Cross, R. F., Reinhart, G. A., and Whitmoyer, R. E. (1988). Effect of age, weaning and postweaning diet on small intestinal growth and jejunal morphology in young swine. J. Anim. Sci. 66, 574–584. doi: 10.2527/jas1988.662574x
Chanoine, J. P.. (2005). Ghrelin in growth and development. Horm. Res. 63, 129–138. doi: 10.1159/000084688
Combs, G. E., Ammerman, C. B., Shirley, R. L., and Wallace, H. D. (1966). Effect of source and level of dietary protein on pigs fed high-copper rations. J. Anim. Sci. 25, 613–616. doi: 10.2527/jas1966.253613x
Cowley, M. A., Smith, R. G., Diano, S., Tschop, M., Pronchuk, N., Grove, K. L., et al. (2003). The distribution and mechanism of action of ghrelin in the CNS demonstrates a novel hypothalamic circuit regulating energy homeostasis. Neuron 37, 649–661. doi: 10.1016/S0896-6273(03)00063-1
Cromwell, G. L., Lindemann, M. D., Monegue, H. J., Hall, D. D., and Orr, D. E. Jr. (1998). Tribasic copper chloride and copper sulfate as copper sources for weanling pigs. J. Anim. Sci. 76, 118–123. doi: 10.2527/1998.761118x
Date, Y., Kojima, M., Hosoda, H., Sawaguchi, A., Mondal, M. S., Suganuma, T., et al. (2000). Ghrelin, a novel growth hormone-releasing acylated peptide, is synthesized in a distinct endocrine cell type in the gastrointestinal tracts of rats and humans. Endocrinology 141, 4255–4261. doi: 10.1210/endo.141.11.7757
De Mille, C. M., and Gabler, N. K. (2021). 271 investigating the relationship between nursery pig performance and markers of intestinal morphology and integrity (abstract). J. Anim. Sci. 99, 100–101. doi: 10.1093/jas/skab054.161
Debski, B.. (2016). Supplementation of pigs diet with zinc and copper as alternative to conventional antimicrobials. Pol. J. Vet. Sci. 19, 917–924. doi: 10.1515/pjvs-2016-0113
Dove, C. R.. (1995). The effect of copper level on nutrient utilization of weanling pigs. J. Anim. Sci. 73, 166–171. doi: 10.2527/1995.731166x
Fairbrother, J. M., Nadeau, E., and Gyles, C. L. (2005). Escherichia coli in postweaning diarrhea in pigs: an update on bacterial types, pathogenesis, and prevention strategies. Anim. Health Res. Rev. 6, 17–39. doi: 10.1079/AHR2005105
Flohr, J. R., DeRouchey, J. M., Woodworth, J. C., Tokach, M. D., Goodband, R. D., and Dritz, S. S. (2016). A survey of current feeding regimens for vitamins and trace minerals in the US swine industry. J. Swine Health Prod. 24, 290–303.
Goswami, T. K., Bhar, R., Jadhav, S. E., Joardar, S. N., and Ram, G. C. (2005). Role of dietary zinc as a nutritional immunomodulator. Asian Australas. J. Anim. Sci. 18, 439–452. doi: 10.5713/ajas.2005.439
Gropper, S. S., and Smith, J. (2013). “Essential trace and ultratrace minerals,” in Advanced Nutrition and Human Metabolism, 6th Edn., eds J. L. Dorsey, J. Hemphill, E. A. Kirk, S. E. Nizielski, S. K. Reaves and K. P. Shelnutt (Belmont, CA: Wadsworth, Cengage Learning), 481–546.
Hahn, J. D., and Baker, D. H. (1993). Growth and plasma zinc responses of young pigs fed pharmacologic levels of zinc. J. Anim. Sci. 71, 3020–3024. doi: 10.2527/1993.71113020x
Hampson, D. J.. (1986). Alterations in piglet small intestinal structure at weaning. Res. Vet. Sci. 40, 32–40. doi: 10.1016/S0034-5288(18)30482-X
Han, Y.-K., and Thacker, P. A. (2010). Effects of antibiotics, zinc oxide, or a rare earth mineral-yeast product on performance, nutrient digestibility and serum parameters in weanling pigs. Asian Australas. J. Anim. Sci. 23, 1057–1065. doi: 10.5713/ajas.2010.90569
Hedemann, M. S., Jensen, B. B., and Poulsen, H. D. (2006). Influence of dietary zinc and copper on digestive enzyme activity and intestinal morphology in weaned pigs. J. Anim. Sci. 84, 3310–3320. doi: 10.2527/jas.2005-701
Helm, E. T., Curry, S. M., De Mille, C. M., Schweer, W. P., Burrough, E. R., and Gabler, N. K. (2020). Impact of viral disease hypophagia on pig jejunal function and integrity. PLoS ONE 15, e0227265. doi: 10.1371/journal.pone.0227265
Heo, J. M., Kim, J. C., Hansen, C. F., Mullan, B. P., Hampson, D. J., Maribo, H., et al. (2010). Effects of dietary protein level and zinc oxide supplementation on the incidence or post-weaning diarrhea in weaner pigs challenged with an enterotoxigenic strain of Escherichia coli. Livest. Sci. 133, 210–213. doi: 10.1016/j.livsci.2010.06.066
Hill, G. M., Cromwell, G. L., Crenshaw, T. D., Dove, C. R., Ewan, R. C., Knabe, D. A., et al. (2000). Growth promotion effects and plasma changes from feeding high dietary concentrations of zinc and copper to weanling pigs (regional study). J. Anim. Sci. 78, 1010–1016. doi: 10.2527/2000.7841010x
Hojberg, O., Canibe, N., Poulsen, H. D., Hedemann, M. S., and Jensen, B. B. (2005). Influence of dietary zinc oxide and copper sulfate on the gastrointestinal ecosystem in newly weaned piglets. Appl. Environ. Microbiol. 71, 2267–2277. doi: 10.1128/AEM.71.5.2267-2277.2005
Hosoda, H., Kojima, M., Matsuo, H., and Kangawa, K. (2000). Ghrelin and des-acyl ghrelin: two major forms of rat ghrelin peptide in gastrointestinal tissue. Biochem. Biophys. Res. Commun. 279, 909–913. doi: 10.1006/bbrc.2000.4039
Houdijk, J. G. M., Bosch, M. W., Tamminga, S., Verstegen, M. W. A., Berenpas, E. B., and Knoop, H. (1999). Apparnt ileal and total-tract nutrient digestion by pigs as affected by dietary nondigestible oligosaccharides. J. Anim. Sci. 77, 148–158. doi: 10.2527/1999.771148x
Hu, C., Song, J., Li, Y., Luan, Z., and Zhu, K. (2013). Diosmectite-zinc oxide composite improves intestinal barrier function, modulates expression of pro-inflammatory cytokines and tight junction protein in early weaned pigs. Br. J. Nutr. 110, 681–688. doi: 10.1017/S0007114512005508
Inui, A., Asakawa, A., Bowers, C. Y., Mantovani, G., Laviano, A., Meguid, M. M., et al. (2004). Ghrelin, appetite, and gastric motility: the emerging role of the stomach as an endocrine organ. FASEB J. 18, 439–456. doi: 10.1096/fj.03-0641rev
Kerr, B. J., Weber, T. E., Dozier, W. A. I., and Kidd, M. T. (2009). Digestible and metabolizable energy content of crude glycerin originating from different sources in nursery pigs. J. Anim. Sci. 87, 4042–4049. doi: 10.2527/jas.2008-1676
Khatib, N., Gaidhane, S., Gaidhane, A. M., Khatib, M., Simkhada, P., Gode, D., et al. (2014). Ghrelin: ghrelin as a regulatory peptide in growth hormone secretion. J. Clin. Diagn. 8, MC13–MC17. doi: 10.7860/JCDR/2014/9863.4767
Kloubert, V., Blaabjerg, K., Dalgaard, T. S., Poulsen, H. D., Rink, L., and Wessels, I. (2018). Influence of zinc supplementation on immune parameters in weaned pigs. J. Trace Elements Med. Biol. 49, 231–240. doi: 10.1016/j.jtemb.2018.01.006
Kojima, M., Hosoda, H., Date, Y., Nakazato, M., Matsuo, H., and Kangawa, K. (1999). Ghrelin is a growth-hormone-releasing acylated peptide from stomach. Nature 402, 656–660. doi: 10.1038/45230
Kojima, M., and Kangawa, K. (2002). Ghrelin, an orexigenic signaling molecule from the gastrointestinal tract. Curr. Opin. Pharmacol. 2, 665–668. doi: 10.1016/S1471-4892(02)00220-5
Lalles, J., Boudry, G., Favier, C., Le Floc'h, N., Luron, I., Montagne, L., et al. (2004). Gut function and dysfunction in young pigs: physiology. Anim. Res. 53, 301–316. doi: 10.1051/animres:2004018
Lei, X. J., and Kim, I. H. (2018). Low dose of coated zinc oxide is as effective as pharmacological zinc oxide in promoting growth performance, reducing fecal scores, and improving nutrient digestibility and intestinal morphology in weaned pigs. Anim. Feed Sci. Technol. 245, 117–125. doi: 10.1016/j.anifeedsci.2018.06.011
Li, B. T., Van Kessel, A. G., Caine, W. R., Huang, S. X., and Kirkwood, R. N. (2001). Small intestinal morphology and bacterial populations in ileal digesta and feces of newly weaned pigs recieving a high dietary level of zinc oxide. Can. J. Anim. Sci. 81, 511–516. doi: 10.4141/A01-043
Liu, T., Peng, J., Xiong, Y., Zhou, S., and Cheng, X. (2002). Effects of dietary glutamine and glutamate supplementation on small intestinal structure, active absorption and DNA, RNA concentrations in skeletal muscle tissue of weaned piglets during d 28 to 42 of age. Asian Australas. J. Anim. Sci. 15, 238–242. doi: 10.5713/ajas.2002.238
Ma, Y. L., Zanton, G. I., Zhao, J., Wedekind, K., Escobar, J., and Vazquez-Anon, M. (2015). Multitrial analysis of the effects of copper level and source on performance in nursery pigs. J. Anim. Sci. 93, 606–614. doi: 10.2527/jas.2014-7796
Mavromichalis, I., Peter, C. M., Parr, T. M., Ganessunker, D., and Baker, D. H. (2000). Growth-promoting efficacy in young pigs of two sources of zinc oxide having either a high or a low bioavailability of zinc. J. Anim. Sci. 78, 2896–2902. doi: 10.2527/2000.78112896x
Maxwell, C. V. Jr., and Carter, S. D. (2001). “Feeding the weaned pig,” in Swine Nutrition, 2nd Edn., eds A. J. Lewis and L. L. Southern (CRC Press LLC), 682–715.
McCracken, B. A., Gaskins, H. R., Ruwe-Kaiser, P. J., Klasing, K. C., and Jewell, D. E. (1995). Diet-dependent and diet-independent metabolic responses underlie growth stasis of pigs at weaning. J. Nutr. 125, 2838–2845.
Moeser, A. J., Pohl, C. S., and Rajput, M. (2017). Weaning stress and gastrointestinal barrier development: implications for lifelong gut health in pigs. Anim. Nutr. 3, 313–321. doi: 10.1016/j.aninu.2017.06.003
Montagne, L., Boudry, G., Favier, C., and Huerou-Luron, I. L. (2007). Main intestinal markers associated with the changes in gut architecture and function in piglets after weaning. Br. J. Nutr. 97, 45–57. doi: 10.1017/S000711450720580X
Ohinata, K., Takemoto, M., Kawanago, M., Fushimi, S., Shirakawa, H., Goto, T., et al. (2009). Orally administered zinc increases food intake via vagal stimulation in rats. J. Nutr. 139, 611–616. doi: 10.3945/jn.108.096370
Ou, D., Li, D., Cao, Y., Li, X., Yin, J., Qiao, S., et al. (2007). Dietary supplementation with zinc oxide decreases expression of the stem cell factor in the small intestine of weanling pigs. J. Nutr. Biochem. 18, 820–826. doi: 10.1016/j.jnutbio.2006.12.022
Paxton, B. E., Alley, C. L., Crow, J. H., Burchette, J., Weiss, C. R., Kraitchman, D. L., et al. (2014). Histopathologic and immunohistochemical sequelae of bariatric embolization in a porcine model. J. Vasc. Interv. Radiol. 25, 455–461. doi: 10.1016/j.jvir.2013.09.016
Payne, G., Martens, D., Kornegay, E. T., and Lindemann, M. (1988). Availability and form of copper in three soils following eight annual applications of copper-enriched swine manure. J. Environ. Qual. 17, 740–746. doi: 10.2134/jeq1988.00472425001700040038x
Pluske, J., Williams, I., and Aherne, F. (1996). Villous height and crypt depth in piglets in response to increases in the intake of cows' milk after weaning. Anim. Sci. 62, 145–158. doi: 10.1017/S1357729800014429
Pluske, J. R., Hampson, D. J., and Williams, I. H. (1997). Factors influencing the structure and function of the small intestine in the weaned pig: a review. Livest. Prod. Sci. 51, 215–236. doi: 10.1016/S0301-6226(97)00057-2
Poulsen, H. D.. (1995). Zinc oxide for weanling piglets. Acta Agric. Scand. A Anim. Sci. 45, 159–167. doi: 10.1080/09064709509415847
Rhouma, M., Fairbrother, J. M., Beaudry, F., and Letellier, A. (2017). Post weaning diarrhea in pigs: risk factors and non-colistin-based control strategies. Acta Vet. Scand. 59, 1–19. doi: 10.1186/s13028-017-0299-7
Rincker, M. J., Hill, G. M., Link, J. E., Meyer, A. M., and Rowntree, J. E. (2005). Effects of dietary zinc and iron supplementation on mineral excretion, body composition, and mineral status of nursery pigs. J. Anim. Sci. 83, 2762–2774. doi: 10.2527/2005.83122762x
Sales, J.. (2013). Effects of pharmacological concentrations of dietary zinc oxide on growth of post-weaning pigs: a meta-analysis. Biol. Trace Elem. Res. 152, 343–349. doi: 10.1007/s12011-013-9638-3
Small, C. J., and Bloom, S. R. (2004). Gut hormones and the control of appetite. Trends Endocrinol. Metab. 15, 259–263. doi: 10.1016/j.tem.2004.06.002
Sohn, J.-W.. (2015). Network of hypothalamic neurons that control appetite. BMB Rep. 48, 229–233. doi: 10.5483/BMBRep.2015.48.4.272
Song, Z. H., Xiao, K., Ke, Y. L., Jiao, L. F., and Hu, C. H. (2015). Zinc oxide influences mitogen-activated protein kinase and TGF- β1 signaling pathways, and enhances intestinal barrier integrity in weaned pigs. Innate Immun. 21, 341–348. doi: 10.1177/1753425914536450
Spreeuwenberg, M. A. M., Verdonk, J. M. A. J., Gaskins, H. R., and Verstegen, M. W. A. (2001). Small intestine epithelial barrier function is compromised in pigs with low feed intake at weaning. J. Nutr. 131, 1520–1527. doi: 10.1093/jn/131.5.1520
Suzuki, H., Asakawa, A., Li, J. B., Tsai, M., Amitani, H., Ohinata, K., et al. (2011). Zinc as an appetite stimulator - the possible role of zinc in the progression of diseases such as cachexia and sarcopenia. Rec. Pat. Food, Nutr. Agric. 3, 226–231. doi: 10.2174/2212798411103030226
van Beers-Schreurs, H. M. G., Nabuurs, M. J. A., Vellenga, L., Valk, H. J. K.-v. d., Wensing, T., and Breukink, H. J. (1998). Weaning and the weanling diet influence the villous height and crypt depth in the small intestine of pigs and alter the concentrations of short-chain fatty acids in the large intestine and blood. J. Nutr. 128, 947–953. doi: 10.1093/jn/128.6.947
Wang, C., Zhang, L., Su, W., Ying, Z., He, J., Zhong, X., et al. (2017). Zinc oxide nanoparticles as a substitute for zinc oxide or colistin sulfate: effects on growth, serum enzymes, zinc deposition, intestinal morphology and epithelial barrier in weaned piglets. PLoS ONE 12, e0181136. doi: 10.1371/journal.pone.0181136
Wang, J., Zhu, X., Guo, Y., Wang, Z., Zhao, B., Yin, Y., et al. (2016). Influence of dietary copper on serum growth-related hormone levels and growth performance of weanling pigs. Biol. Trace Elem. Res. 172, 134–139. doi: 10.1007/s12011-015-0574-2
Wren, A. M., Small, C. J., Ward, H. L., Murphy, K. G., Dakin, C. L., Taheri, S., et al. (2000). The novel hypothalamic peptide ghrelin stimulates food intake and growth hormone secretion. Endocrinology 141, 4325–4328. doi: 10.1210/endo.141.11.7873
Yazdankhah, S., Rudi, K., and Bernhoft, A. (2014). Zinc and copper in animal feed - development of resistance and co-resistance to antimicrobial agents in bacteria of animal origin. Microb. Ecol. Health Dis. 25. doi: 10.3402/mehd.v25.25862
Yin, J., Li, X., Li, D., Yue, T., Fang, Q., Ni, J., et al. (2009). Dietary supplementation with zinc oxide stimulates ghrelin secretion from the stomach of young pigs. J. Nutr. Biochem. 20, 783–790. doi: 10.1016/j.jnutbio.2008.07.007
Zhang, B., and Guo, Y. (2008). The growth-promoting effect of tetrabasic zinc chloride is associated with elevated concentration of growth hormone and ghrelin. Asian Australas. J. Anim. Sci. 21, 1473–1478. doi: 10.5713/ajas.2008.80057
Zhang, B., and Guo, Y. (2009). Supplemental zinc reduced intestinal permeability by enhancing occludin and zonula occludens protein-1 (ZO-1) expression in weaning piglets. Br. J. Nutr. 102, 687–693. doi: 10.1017/S0007114509289033
Keywords: nursery pig, zinc, feed intake (FI), ghrelin, digestibility
Citation: De Mille CM, Burrough ER, Kerr BJ, Schweer WP and Gabler NK (2022) Dietary Pharmacological Zinc and Copper Enhances Voluntary Feed Intake of Nursery Pigs. Front. Anim. Sci. 3:874284. doi: 10.3389/fanim.2022.874284
Received: 11 February 2022; Accepted: 24 March 2022;
Published: 25 April 2022.
Edited by:
Katie Wood, University of Guelph, CanadaReviewed by:
Michael Azain, University of Georgia, United StatesGuofeng Han, Nanjing Agricultural University, China
Crystal L. Levesque, South Dakota State University, United States
Copyright © 2022 De Mille, Burrough, Kerr, Schweer and Gabler. This is an open-access article distributed under the terms of the Creative Commons Attribution License (CC BY). The use, distribution or reproduction in other forums is permitted, provided the original author(s) and the copyright owner(s) are credited and that the original publication in this journal is cited, in accordance with accepted academic practice. No use, distribution or reproduction is permitted which does not comply with these terms.
*Correspondence: Nicholas K. Gabler, ngabler@iastate.edu