- 1Veterinary Medicine, Department Population Health Sciences, Animals in Science & Society, University Utrecht, Utrecht, Netherlands
- 2Veterinary Medicine, Department Population Health Sciences, Animals in Science & Society, Former Behavior and Welfare Group, University Utrecht, Utrecht, Netherlands
Stress is an important factor in animal welfare. Hair or wool cortisol concentrations are considered to be potential long-term indicators of stress experienced by an animal. Using Swifter sheep, we investigated whether ewe parity and litter size affect the wool cortisol concentrations in ewes and their offspring. We hypothesized that multiparous ewes and their offspring would have higher wool cortisol concentrations than primiparous ewes and their offspring, that ewes with larger litters and their offspring would have lower wool cortisol concentrations than ewes with smaller litters and their offspring, that male lambs would have higher wool cortisol concentrations than female lambs, and that the wool cortisol concentrations in the wool of ewes and their lambs would be correlated. Lamb wool grows in utero during the third trimester of pregnancy. In ewes, the shave–reshave method was used so that wool samples from ewes also covered approximately the last trimester of pregnancy. Our study confirmed that litter size affected ewe wool cortisol concentrations: ewes that gave birth to larger litters (i.e., 3 or 4 lambs) had higher wool cortisol concentrations than ewes that gave birth to smaller litters (i.e., 1 or 2 lambs). There was no evidence that the wool cortisol concentrations of the ewes and their lambs were correlated. Neither litter size nor parity of the ewe affected wool cortisol in the lambs. Our study confirms that wool cortisol can be reliably measured in ewes and their newborn lambs, and suggests that it may be useful as a retrospective indicator of stress during the last trimester of pregnancy.
1 Introduction
Current housing conditions and management systems of farm animals (Nordquist et al., 2017) and the living conditions of companion animals may sometimes be suboptimal and stressful (Serpell, 2019). In particular, animals subjected to chronic stress may suffer from compromised/poor welfare. The level of cortisol, collected from sample matrices such as blood, saliva, urine, feathers, hair (Russell et al., 2015), or nails (Meyer and Novak, 2021), is often used as a physiological proxy for experienced stress (Burnett et al., 2014). Although cortisol is commonly referred to as the stress hormone, because of its role in acute and chronic stress, it is a hormone with multiple effects (Oakley and Cidlowski, 2013). Cortisol plays a major role in the central nervous system and the immune system (Miller et al., 2007; Olejniczak et al., 2022). Interestingly, cortisol concentration increases in the fetus to stimulate normal organ development and to ensure the normal timing of parturition (Magyar et al., 1980; Gootwine et al., 2007). Cortisol is also involved in, among other things: controlling the body’s use of fats, proteins, and carbohydrates (Knott et al., 2010); suppressing inflammation (Silverman et al., 2005); regulating blood pressure (Hammer and Stewart, 2006; Mohd Azmi et al., 2021) and blood glucose; and regulating the sleep–wake cycle (Buckley and Schatzberg, 2005; De Nys et al., 2022). Glucocorticoid receptors are expressed widely throughout the body (Witchel and DeFranco, 2006; Timmermans et al., 2019).
When an animal is confronted with a stressful situation, cortisol is produced by the hypothalamic-pituitary-adrenal (HPA) axis (Brydges and Braithwaite, 2008), the major stress system in the body (Frodl and O’Keane, 2013). The HPA axis is activated when the hypothalamus secretes corticotrophin-releasing hormone (CRH), which travels to the anterior pituitary gland (Miller et al., 2007). The anterior pituitary gland responds with the secretion of adrenocorticotropin hormone (ACTH) into the blood vessels. ACTH is transported via the blood to the adrenal gland, where cortisol is synthesized and released in response to ACTH. A rise in cortisol decreases CRH via a negative feedback loop. This inhibits ACTH secretion and thereby cortisol production, and homeostasis is reached again (Micale and Drago, 2018).
Cortisol can be measured in different sampling matrices: body fluids such as saliva, excretions such as feces and urine, and hair or feathers (Davenport et al., 2006; Ghassemi Nejad et al., 2014; Meyer and Novak, 2021). Each medium has its own advantages and disadvantages. For instance, the measurement of cortisol in blood is widely used, in spite of the fact that the sampling of blood may be stressful. The animal needs to be captured and restrained; these acts can influence blood cortisol concentrations. However, as Guesdon et al (2012; 2013; 2015). have demonstrated, the stress of blood sampling via a catheter can be greatly reduced by training and habituating the animals to the procedure beforehand.
Less stressful sampling methods are the collection of saliva, urine, and feces (Davenport et al., 2006; Ghassemi Nejad et al., 2014). According to Davenport et al. (2006), these methods have their own limitations (Davenport et al., 2006). Both blood and saliva samples are point measurements. This means that values from the same individual can fluctuate over time, because the value is influenced by the circadian rhythm, food intake, and the environment (Davenport et al., 2006), and by the stress response to acute adverse events. Saliva sampling is a non-invasive way of measuring only when the animals are trained, which is not possible for all animals (Davenport et al., 2006). Both urine and feces samples can give an indication of cortisol accumulation over a longer period of time: for urine, this is approximately 24 h, and for feces, this is between 10 h and 12 h (Davenport et al., 2006; Burnett et al., 2014). However, if animals are socially housed, the risk of sample contamination is high. Furthermore, all these sampling methods must be repeated multiple times to provide a good long-term overview of HPA axis activity (Davenport et al., 2006).
A relatively recent way of measuring cortisol is through the use of hair samples (Ghassemi Nejad et al., 2014; Heimbürge et al., 2019; Heimbürge et al., 2020a; Heimbürge et al., 2020b). The concentration of cortisol in hair is believed to reflect the accumulation of cortisol over the period of time that the hair has grown (Davenport et al., 2006; Ghassemi Nejad et al., 2014; Lee et al., 2015; Russell et al., 2015). Similarly, wool cortisol concentrations might serve as (retrospective) indicators of physiological stress in sheep (Fürtbauer et al., 2019; Sawyer et al., 2021). Another advantage is that this method of collecting hair or wool samples is non-invasive. However, unfortunately, it is not yet completely known how cortisol accumulates in hair and wool (Burnard et al., 2016). Cortisol can enter the hair or wool shaft by active or passive diffusion via blood, sebum, or sweat (Davenport et al., 2006; Russell et al., 2012; Stubsjøen et al., 2015). The largest proportion of cortisol accumulated in hair originates from diffusion from blood as its endogenous source (Xie et al., 2011; Meyer and Novak, 2021). For wool, a significant quantity may also originate from the sebaceous glands (Burnard et al., 2016). An additional source of cortisol may be local production of cortisol in or near the hair follicle, which influences the cortisol concentration in the hair shaft (Russell et al., 2012; Salaberger et al., 2016). In addition, the environmental conditions, location of the hair on the body, color of the hair, and animal age and health condition can have an impact on the cortisol concentrations of hair (del Rosario González-de-la-Vara et al., 2011; Burnett et al., 2014; Heimbürge et al., 2020b) and wool (Stubsjøen et al., 2015; Salaberger et al., 2016).
Cortisol in hair and wool has been measured in several species, including sheep (Ghassemi Nejad et al., 2014; Stubsjøen et al., 2015), pigs (Bacci et al., 2014; Antonides et al., 2015; van Eck et al., 2016; Roelofs et al., 2019), cows (Burnett et al., 2014; Burnett et al., 2015; van Eerdenburg et al., 2021), dogs (van der Laan et al., 2022; van Houtert et al., 2022), rhesus macaques (Davenport et al., 2006; Wooddell et al., 2021), and humans (Kalra et al., 2007; D’Anna-Hernandez et al., 2011). Previous research on humans, sheep, and pigs shows that cortisol concentration in hair and wool increases during pregnancy (D’Anna-Hernandez et al., 2011; Bacci et al., 2014; Meyer and Novak, 2021; Sawyer et al., 2021). Furthermore, studies show that multiparous cows (i.e., cows who have given birth before) have higher hair cortisol concentrations than primiparous cows (i.e., cows who have gavin birth for the first time) (Burnett et al., 2014; Burnett et al., 2015).
The average gestation period in sheep is 147 days (approximately 5 months). The fetus grows most rapidly during the final 60 days. The development of wool follicles begins toward the end of the second trimester (https://www.agric.wa.gov.au/management-reproduction/managing-pregnancy-ewes). The appearance of wool appears to be preceded by hair. According to Cloete (1939), body hair is first seen on day 90, toward the end of the second trimester, that is hair grows first, followed by wool (Duerden and Ritchie, 1924; Cloete, 1939). The fetus is completely covered with hair by approximately 116 days (Green and Winters, 1945; note that they did not differentiate between hair and wool), that is in the third trimester of pregnancy, but is completely covered with a wool fleece at birth (Duerden and Ritchie, 1924; Cloete, 1939). The wool fleece grown during the last trimester of pregnancy could therefore be considered as a long-term store of cortisol, and therefore a retrospective stress indicator for the fetus.
Collecting hair or wool samples offers the unique opportunity to obtain a direct biomarker of chronic in utero hormone exposure, predominantly accumulated during the last trimester of pregnancy (Meyer and Novak, 2021). Using this approach in studies addressing the effects of parity and litter size on prenatal cortisol accumulation, a positive association between litter size and plasma and wool cortisol—the latter representing cortisol accumulation approximately 1.5 months before lambing—was found in highly prolific Afec-Assaf sheep (Alon et al., 2021). Similar to sheep, sows’ hair cortisol level was weakly correlated with litter size (rs = 0.36), suggesting that sows carrying a larger litter had higher levels of circulating cortisol during the last weeks of pregnancy (Roelofs et al., 2019), perhaps because carrying more fetuses might have been more stressful or have caused more metabolic stress.
However, increased litter size may also accelerate the maturation of the HPA axis in the fetuses, and the resulting higher fetal cortisol concentration may diffuse into the uterus (Rutherford et al., 2013); maternal cortisol readily crosses the placenta (Rakers et al., 2020). Hence, the original source of higher cortisol concentrations may be the mother (Hantzopoulou et al., 2022), the fetus, or both. Moreover, adverse environmental conditions (e.g., exposure to thermal stress, Nazifi et al., 2003) during pregnancy may also affect the ewes’ and lambs’ cortisol concentrations.
The pregnancy status can not only influence the mother but might also influence the developing fetus. For example, if an animal is pregnant with more than one offspring, intrauterine competition takes place for nutrients and space (Casellas and Caja, 2014), which may lead to higher cortisol levels in one or all siblings. A relationship between ewe cortisol levels and litter size has previously been demonstrated, with higher wool (Alon et al., 2021) and plasma cortisol levels (Fazio, 2013) found in ewes measured post partum, that is at the end of pregnancy the ewe could be more “exhausted” and have a larger litter size.
It should be noted that several hormones, including cortisol, can affect wool production, particularly those that change during pregnancy, lactation, and aging. High levels of cortisol have been shown to inhibit wool growth, possibly through the effects of cortisol on wool follicle function (Scobie and Hynd, 1995; Weaver et al., 2021). When sheep are stressed (either by metabolic factors or by external factors), cortisol levels are elevated and, at high concentrations, have been shown to reduce wool growth.
In ewes, cortisol levels are low during pregnancy. An increase is seen at parturition. The effect on wool growth appears to be greatest in the last trimester (Hinch, 2013). Compared with non-pregnant ewes in which wool growth was constant, single- and triple-lambing ewes showed slightly reduced wool growth, and the decrease was greater in triple-lambing ewes (Ochoa Cordero et al., 2019). Alon et al. (2021) suggested that multiple-gestation pregnancies are associated with higher maternal cortisol concentrations in late pregnancy, possibly as a result of increased metabolic stress.
In fetuses, Toland Thompson and colleagues (2007) found an increase in plasma cortisol concentrations in both single and twin lambs beginning at 138 days of gestation. In twin lambs, both the peak in cortisol concentration and the period over which cortisol levels remained elevated were greater than in singletons. McDowall et al. (2013) showed that the experimental modulation of cortisol biosynthesis affected wool follicles in fetuses, an observation consistent with the notion that elevated cortisol levels in ewes (e.g., due to stress) affect wool growth in fetuses.
However, elevated cortisol levels do not fully explain the slowing of wool growth prior to parturition. Other causes may be related to cell division processes and wool protein synthesis in the follicle. Both processes may be inhibited by the action of a growth factor such as epidermal growth factor (EGF) or by a lack of nutrients (amino acids) (Hinch, 2013).
The relationship between ewe glucocorticoids, litter size, and lamb glucocorticoids has not yet been explored. One study found that birth weight and the HPA response have a converse relationship (Bloomfield et al., 2007). This suggests that larger litters should have higher plasma cortisol values, because the offspring of larger litters generally have lower birth weights (Gootwine et al., 2007). Furthermore, in sheep the plasma nutrient concentrations in twins and their mothers are lower than those of singletons and their mothers in late gestation (Gootwine et al., 2007), and it was found that twin lambs have higher levels of hypoxia and oxidative stress than singletons (Sales et al., 2018). However, one study demonstrated that plasma cortisol levels are lower in twin lambs than in singletons (Gardner et al., 2004), which is hypothesized to delay adrenocortical function in order to prolong gestation. As all of the lambs in the present study were multiples, and as mentioned above, twin lambs have been demonstrated to show hypoxia compared with singletons (Sales et al., 2018), one might expect some extent of hypoxic stress in all of them. There is some evidence that the cortisol concentration in the plasma of male fetuses in response to hypoxic stress is much higher than that of female fetuses (Giussani et al., 2011). Whether these effects generalize to other sources of stress is not yet known.
Based on published findings, we hypothesized that:
1. multiparous ewes and their offspring have higher wool cortisol concentrations than primiparous ewes and their offspring;
2. ewes with larger litters and their offspring have lower wool cortisol concentrations than ewes with smaller litter sizes and their offspring (in line with Gardner et al., 2004);
3. male lambs have a higher wool cortisol concentration than female lambs;
4. the wool cortisol concentrations in the wool of ewes and their lambs are correlated.
The aim of this study was to investigate whether the wool cortisol concentrations of ewes and their newborn lambs are affected by the parity of the ewe (primiparous vs. multiparous), the size of the litter, and the sex of the lambs. Empirical support for any or all of our hypotheses would warrant further investigation into the putative role of wool cortisol concentration as a physiological prenatal retrospective marker of stress that sheep experience during the last trimester of pregnancy.
2 Materials and methods
2.1 Ethical statement
For this study the wool of ewes and their lambs was used, which was collected in a non-invasive, pain-free manner. According to Dutch law (“Wet op de dierproeven”, the Dutch Experiments on Animals Act from 18 Dec. 2014, §1, article 1, 1b, 13a), this study did not need to be approved by an ethics committee. Nonetheless, this study was registered, and the study protocol was evaluated by the Animal Welfare Body of Utrecht University, the Netherlands.
2.2 Animals and housing
This study was a follow-up of a larger study (relevant information about the three phases of the entire study is provided in Supplementary Materials 1) in which an appropriate wool-washing method preceding the determination of cortisol in wool was determined in the third phase. The study design and the number of ewes and lambs used in the present study are depicted in Figure 1.
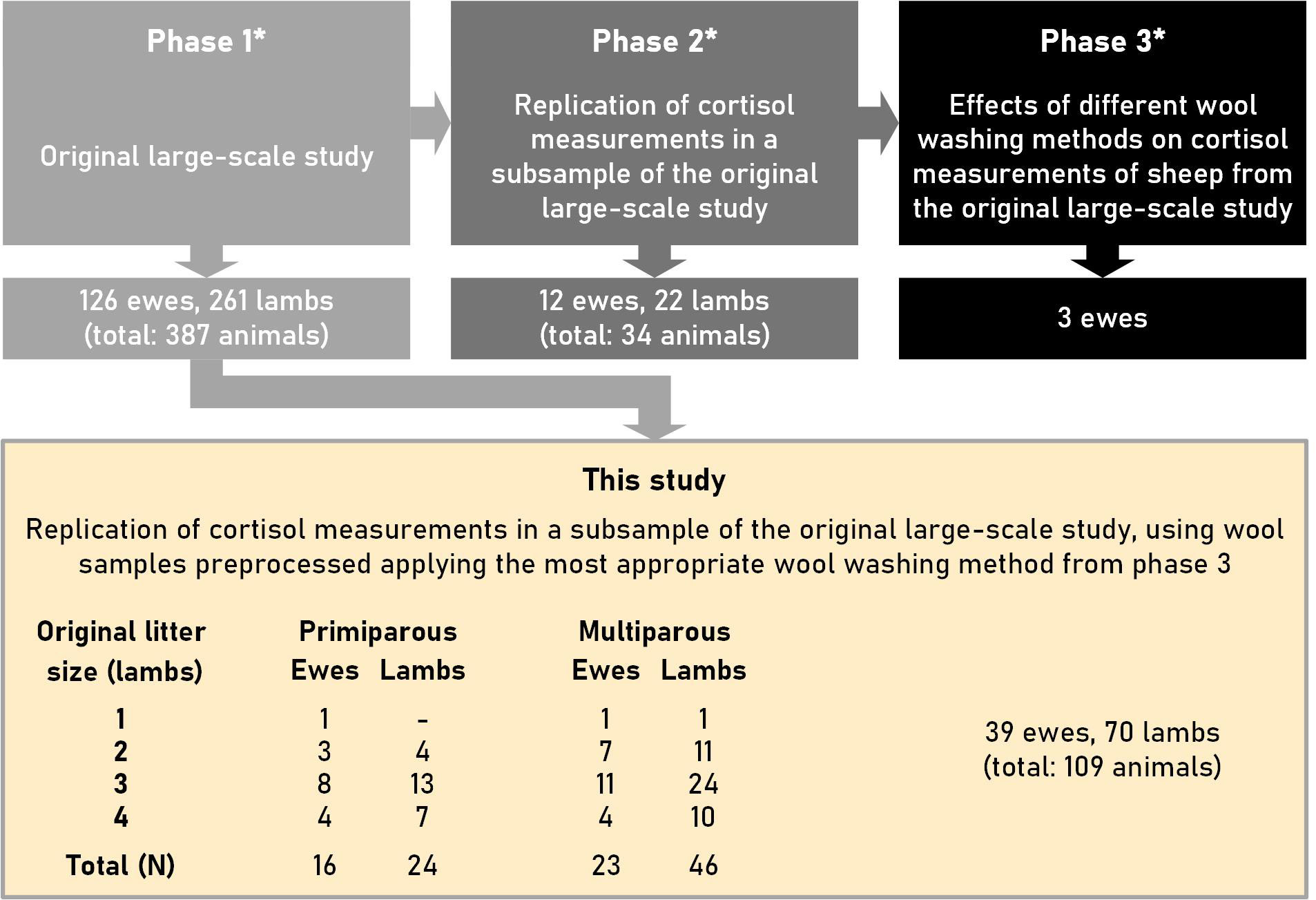
Figure 1 Design of this study, which was performed on a subsample of a large-scale study with ewes and lambs of the Swifter sheep breed. *: Phases 1 to 3 are described in more detail in Supplementary Material 1. The litter sizes (original) against parity (primiparous vs. multiparous) and the number of ewes and lambs used to determine cortisol concentrations are tabulated under “This study”.
For the cortisol measurements of this study the wool of 39 sheep (Ovis aries) and 70 lambs of the Swifter breed was used (for information about this breed see: “Swifter sheep”; Farmow Livestock, 2022; “Swifter sheep”; Wikimedia Foundation, 2022). This breed is very fertile, and ewes giving birth to singletons happens only rarely. De Vries et al. (1998) found that the litter size in Swifter sheep increases with an increase in parities. Whereas the mean litter size (± SD) was 1.68 ± 0.590 lambs for the first parity, it was 2.17 ± 0.691 lambs for the second parity, and 2.37 ± 0.733 lambs for the third parity. The present study was conducted at the education and research farm of the Faculty of Veterinary Medicine of Utrecht University. The flock of sheep is mainly kept for meat production; a smaller number of sheep are used as experimental animals in scientific research.
The sheep were kept on grasslands during the year but were housed in stables during the lambing season. The herd was administratively (not physically) divided into successive batches based on their expected lambing date. During the first part of the housing period the sheep were kept in a herd of approximately 50 individuals in a deep litter straw pen with a size of approximately 85m2. After lambing, each ewe and her lambs were kept together in a small pens and separated from the herd for approximately 2 days. These pens had a size of approximately 2–4m2.
After 2 days, the lambs were strong enough to rejoin the other ewes and lambs in another pen; the size of these pens was between 60m2 and 95m2, and between 10 and 35 ewes were kept in each pen with their lambs. If an ewe had more than two lambs, only two lambs stayed with the ewe, usually the lightest of the litter. The other lambs were housed together with the lambs of other ewes. These lambs had access to a milk bar.
2.3 Experimental design
For cortisol measurement, the ewes and lambs were categorized by parity and litter size. All sheep were born, and consequently all wool samples were collected, between the last week of February and mid-April (mean ambient temperature was 4.6°C in February, 5.4°C in March, and 14.5°C in April, according to https://www.wintergek.nl/data/lijst-gemiddelde-temperatuur-nederland). Approximately 4 weeks before lambing, all ewes were shorn. Within 48 h after parturition, an area of approximately 4cm2 was shorn on the flanks of the lambs and ewes, as close as possible to the skin, for collecting the wool samples, using disposable Prep Razors (Kai medical, AUV Veterinary Services, Servoprax GmbH Germany). The shorn area yielded a sufficient amount of wool (coat length approximately 1 cm) for cortisol analysis. All lambs and most ewes had a white coat. Two ewes were black, and one had a gray coat. Whether wool color differentially affects cortisol concentrations is not yet known. Whereas coat color affected cortisol concentrations in dog hair (Bennett and Hayssen, 2010), it did not affect them in cattle (Nedić et al., 2017). The collected wool samples were kept in the dark at all times until analysis because the cuticle structure can be damaged by natural sunlight, which can cause a decline in the cortisol concentration (Li et al., 2012; Wester and van Rossum, 2015).
2.4 Processing the wool samples
Based on results from a pilot study (see Supplementary Materials 1 for details), after a pre-wash with 20mL distilled water followed by 20mL 80% isopropanol (EMSURE, VWR International B.V. Nederland), the wool of these samples was washed with a detergent [20 g Biotex Green (Unilever)], resolved in 200mL distilled water]. Biotex Green is a washing powder that is used for material that cannot be cleaned in a washing machine. It contains enzymes that break down stains consisting of fat, proteins, or starch. Subsequently, the samples were placed in a water bath of 60°C for 1 hour. Afterwards, 20mL distilled water was added and the samples were shaken by hand for 5 s. The water solution was thrown away and 20mL of distilled water was added and shaken by hand for 5 s. This step was repeated six times. Next, 20mL 100% n-hexane (J.T. Baker/VWR) was added and the samples were put on the Roller Bank (Stuart, Boom, SRT9D) for 3 minutes at 30 RPM. After cleaning, the hair samples were dried for 7 days at 37°C in a stove (Memmert, Dépex NV, de Bilt, Holland).
The dry wool was cut into short pieces with a maximum length of 5 mm, after which 35 mg was further processed. Three metal beads of 3.2 mm (QIAGEN, BioSpec Products Lab Services BV Nederland) were added to the sample, which was bead beaten in a TissueLyser II (QIAGEN, Benelux B.V Nederland) for 15 min at 30 Hz. Afterwards the samples were centrifuged (Mikrostar 17R,VWR) at 17,000G and 4°C for 5 minutes. These two steps were repeated until all the wool in the tubes was pulverized. In general, repeating these steps twice was sufficient.
One mL of methanol (EMSURE, VWR International B.V. Nederland) was added to the sample, the beads were removed and the sample was sonicated (Sonicor Instrument Corporation – Copiague N.Y.) for 30 min, and then placed on the Roller Bank for 24 h so that the cortisol could be extracted. After 24 h the samples were centrifuged for 5 minutes at 17,000G, after which 0.6mL of the supernatant was placed into a Speedvac (Savant, automatic environmental Speedvac, AES 1000) for approximately 3 hours. The methanol evaporated in the Speedvac and as a result, only the cortisol remained.
Afterwards, 200µL of phosphate-buffered saline (PBS) provided by the Salimetrics High Sensitivity Salivary Cortisol ELISA kit was added. Subsequently, the samples were placed on the Roller Bank for another 24 h to dissolve the cortisol. The ELISA was performed in accordance with the supplier’s instructions. The samples were measured in a Multimode Detector DTX 880 (Beckman Coulter, Inc).
2.5 Statistical analysis
The data were collated in Microsoft Excel© (the data can be found in Supplementary Materials 2). The cortisol level of one lamb that one primiparous ewe had given birth to was not measured. Consequently, the cortisol measurement of this ewe was included in the analysis for cortisol values of ewes, whereas the cortisol value of the lamb was missing. All data were transferred to statistical program R version 4.0.5 (R Core Team, 2021) with library readxl. Graphs were produced with library ggplot2. A general linear model (glm) was applied to analyze the cortisol concentration in the wool samples of the dams. The explanatory variables were parity (primiparous or multiparous) and original litter size (1 or 2 vs. 3 or 4 offspring). The cortisol concentration was log transformed to meet model assumptions, which were assessed by visual inspection of the residual plots. The effect size with 95% confidence interval of the full model were presented to interpret the effect size to answer the research questions even when results are not significant. Due to the log transformation of the outcome variable, the back transformed estimated effect size should be interpreted as the ratio between the (geometric) mean of a specified category with the (geometric) mean of the reference category.
Pearson’s (product–moment) correlation coefficient was visualized in a scatterplot and calculated between the log transformed cortisol concentration of dams and offspring. The correlation has also been calculated separately for the small litter sizes and large litter sizes.
The log transformed cortisol concentrations in the wool samples of lambs were analyzed using a linear mixed effects model (Bates et al., 2015) with the explanatory factors being parity of the dams (primiparous or multiparous), original litter sizes (1 or 2 vs. 3 or 4 lambs), sex (male or female), and cortisol concentrations in the wool samples of the dam (< 30, 30.1–40, or > 40 pg/mg). Ewe IDs were added as random intercept to the model to take the correlation between lambs within ewe into account. The model assumptions were assessed by visual inspection of the residual plots and no aberrations were observed. The back transformed effect sizes (ratio between geometric means) with 95% confidence interval of the full model were presented.
Note that the results of the statistical model are reported according to the “Arrive” guidelines for animal experiments (Percie du Sert et al., 2020). The confidence interval for each parameter in the model is reported. Favoring reporting confidence intervals instead of p-values is also supported by Halsey (2019), as it allows for the reporting of the magnitude of the parameter effect with precision. Wide confidence intervals indicate that estimates of parameters are less precise. The confidence interval is loosely explained as the range of plausible values for the population parameters, based on the appropriate statistical model in this study. As we report the full model, we also indicate the importance of a factor based on the AIC for model selection (Halsey, 2019). When a factor has no statistically significant effect on the parameter estimate, the AIC will be smaller for the more parsimonious model, i.e., the model with lower AIC has better fit.
3 Results
The cortisol concentrations are summarized separately per parity (primiparous vs. multiparous) for dams and offspring (Figure 2A), and per litter size (1 or 2 lambs vs. 3 or 4 lambs) separately for dams and offspring (Figure 2B). In general, the cortisol concentration was higher in offspring compared to dams, and the offspring of multiparous dams had lower cortisol concentrations than those of primiparous dams. Larger litter sizes seemed to increase the cortisol concentration in dams and in offspring (Figure 2B), and in general, lambs had higher cortisol concentrations than dams (Figure 2C). The correlation between cortisol concentrations in the wool of dams and offspring was, at best, very weak and failed to reach statistical significance (Figure 2D). For all comparisons, a substantial overlap in cortisol concentrations was seen between groups.
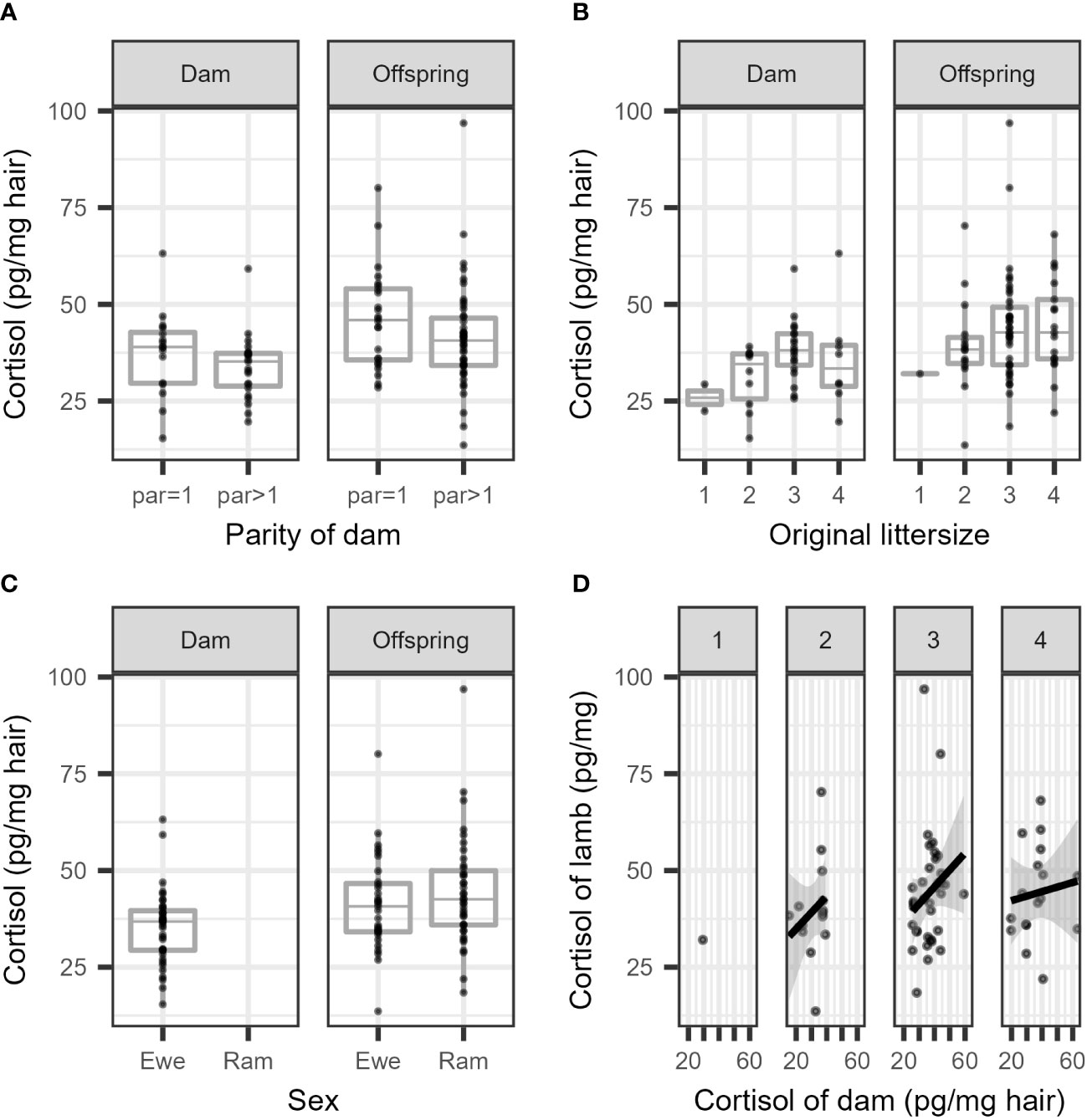
Figure 2 Boxplots of cortisol concentration in the wool are presented (A) per parity in dams and their offspring, (B) per litter size in dams and their offspring, and their (C) per sex in dams and offspring. (D) depicts a scatterplot of the cortisol concentration of dams versus related individual offspring (per litter size 1-4; untransformed measurements).
3.1 Cortisol concentration in primiparous vs. multiparous ewes
The results of the final model for cortisol concentration in ewes are presented in Table 1 (see also Figure 2A). The cortisol concentration in multiparous ewes was, on average, 6% lower than in primiparous ewes, which is not significant. The cortisol concentration in the wool of ewes with larger litters (3 or 4 lambs) was 24% higher than ewes with smaller litters (1 or 2 lambs). This difference between mean cortisol of both litter sizes is statistically significant as the ratio between group means = 1 is not included in the 95% confidence interval. This result contrasts with a decrease predicted in our hypothesis.
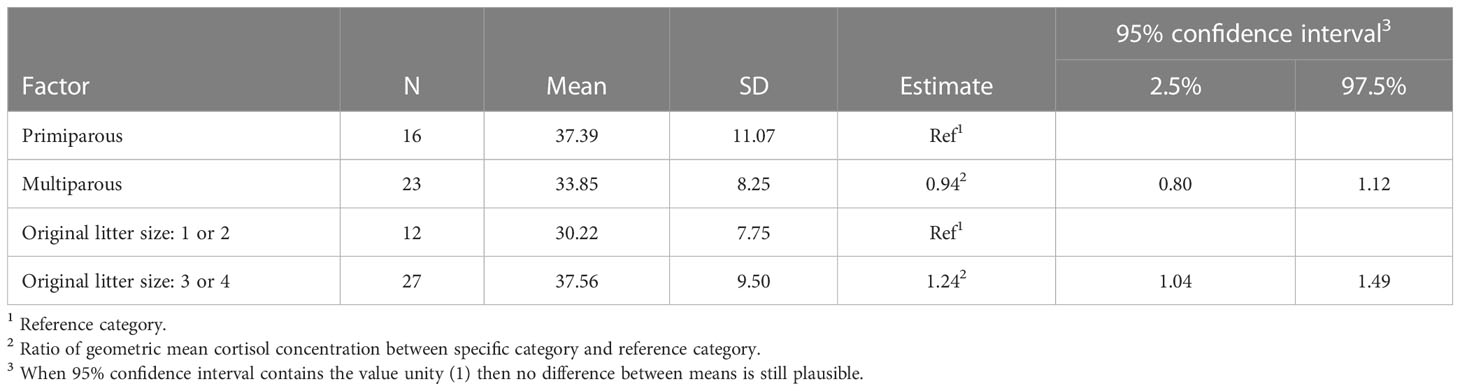
Table 1 Summary statistics and adjusted model estimates (ratio between group means) with 95% confidence intervals for mean cortisol concentration (pg/mg wool) in ewes (dams) per explanatory factor of the full model.
3.2 Cortisol concentration in offspring
Offspring of multiparous dams had a 13% lower cortisol concentration than offspring from primiparous ewes (see Table 2), though this difference was not statistically significant. The cortisol concentration in the wool of offspring from larger litters (3 or 4 lambs) was, on average, 12% higher than that of offspring from smaller litters (1 or 2 lambs). This increase was not statistically significant.
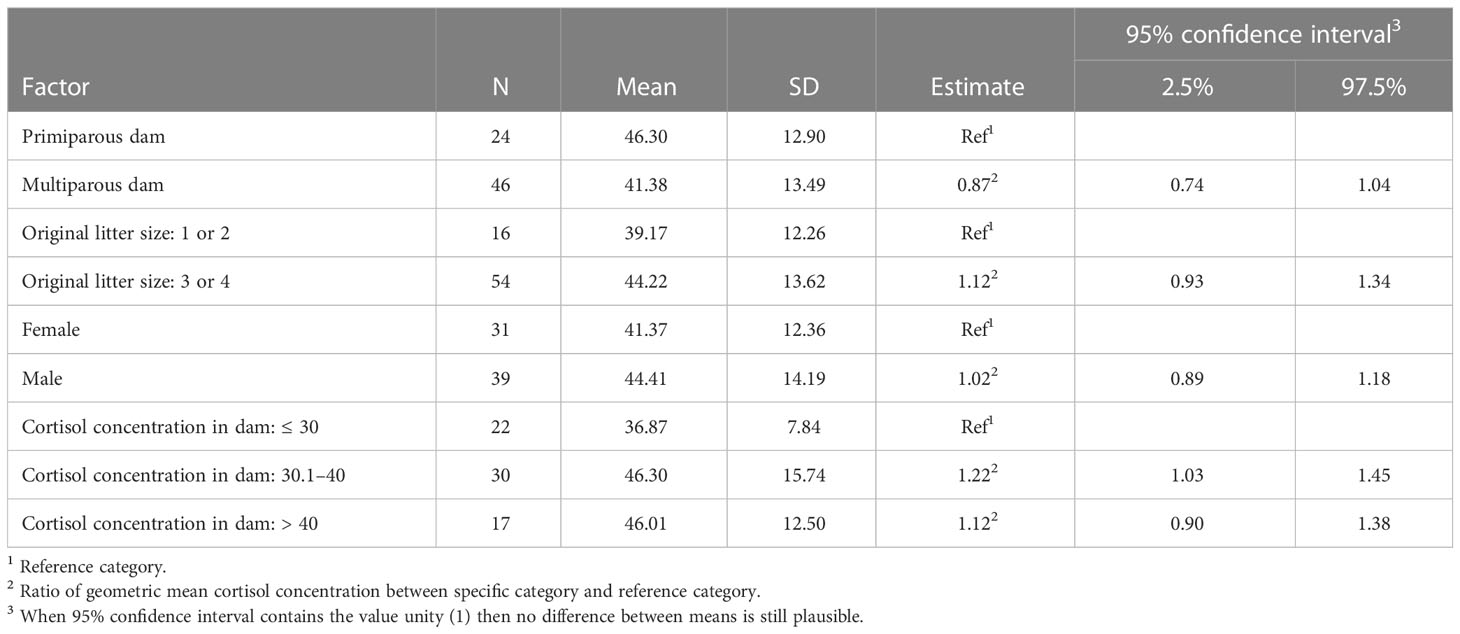
Table 2 Summary statistics and adjusted model estimates (ratio between group means) with 95% confidence intervals for mean cortisol concentration (pg/mg wool) in lambs/offspring per explanatory factor of the full model.
Sex of the lambs did not seem to affect cortisol in wool. Intermediate cortisol concentration of the dam (30.1–40 pg/mg wool) increased the cortisol concentration in offspring with 22% and with 12% when cortisol concentration of the dam exceeds 40 pg/mg wool. None of the comparisons between group means, however, was statistically significant, because the ratio unity (i.e., equal group means) was included in the 95% confidence intervals.
3.3 Association between cortisol concentration of dam and offspring
A large variation was observed between the log transformed cortisol concentrations of dams and individual offspring (Figure 2D), resulting in a low Pearson’s correlation coefficient. The estimated correlation for the full data was r = 0.24 (95% CI: 0.007 to 0.45). The correlation was r = 0.14 in small litters (1 or 2 lambs) (95% CI: 0.38 to 0.60) and r = 0.23 (95% CI: –0.04 to 0.47) in large litters (3 or 4 lambs). However, these correlations are considered to be negligible, and at best indicate a very weak association (see, e.g., Schober et al., 2018, p. 1765).
4 Discussion
The main aim of this study was to investigate the effects of parity and litter size on the concentration of cortisol in the wool of ewes and their newborn offspring. Wool was sampled shortly after parturition in Swifter ewes and their newborns. Because only one ewe in this study gave birth to a singleton (see overview table under “This study” in Figure 1), we pooled all ewes with one or two offspring into one subgroup, and all ewes with three or four offspring into a second subgroup. We expected that (1) the wool of multiparous ewes and their offspring would have higher cortisol concentrations than that of primiparous ewes and their offspring, 2) the wool of ewes with larger litters and their offspring would have lower hair cortisol concentrations than that of ewes with smaller litter sizes and their offspring, 3) the wool of male lambs would have a higher cortisol concentration than that of female lambs, and 4) the cortisol concentrations in the wool of ewes and their lambs are correlated. Of these expectations, only the hypothesized higher cortisol concentration in the wool of ewes carrying larger litters was confirmed statistically, corroborating findings by others (Alon et al., 2021). It is, however, not yet known if it is biologically significant that the wool cortisol concentration in these ewes was 24% higher than in ewes with small litters (an increase that is statistically significant but demonstrates only a weak association). This finding, however, supports our expectation that wool cortisol concentration is sensitive to pregnancy-related factors that are believed to affect stress in the ewe and/or lamb during the third trimester. However, we cannot pinpoint the exact source of stress that might have led to the increase in cortisol concentration in ewes that gave birth to larger (3 or 4 lambs) litters.
4.1 The pros and cons of measuring cortisol in hair and wool as retrospective indicator for experienced chronic stress
Salaberger and co-workers (2016) advised caution when interpreting wool cortisol concentration as a marker of chronic stress in sheep, because the local production of cortisol may have a significant influence on concentrations in the hair. In addition, Fürtbauer et al. (2019) showed that due to their structural differences, hair and wool differ with respect to cortisol uptake and storage. These differences require more fundamental research with respect to cortisol in wool, in particular its source, mode of uptake, stability over time, reliable methods of determining cortisol concentrations, and the impact of putative interfering influences. According to Burnard et al. (2016), p. 401) “there is currently insufficient evidence to conclude that cortisol concentration in hair accurately reflects long-term blood cortisol concentrations.”
Hair and wool differ in structure (length, diameter, shape, and cuticle) and function (density of the sweat and sebaceous glands, density of follicles, blood supply, and pigmentation), all of which may influence the absorption of cortisol (Burnard et al., 2016; Fürtbauer et al., 2019; Heimbürge et al., 2019). It is not yet known via which system—that is, blood, tissue around the follicle, or secretions of sweat and sebum—it is that endogenous cortisol is mainly incorporated. A study in which radiolabeled cortisol was injected intravenously and then detected in rhesus monkey hair, provided a strong indication that cortisol from the blood stream is incorporated in hair (Kapoor et al., 2018).
Wool fibers have more coarse, single-layered cuticula, and are usually very crimped and waxy, with a high ratio of secondary follicles (containing no sweat glands) to primary follicles (containing a sweat gland) (Burnard et al., 2016). The near continuous growth of wool fibers offers the opportunity to deduce a cortisol timeline. By using regrown wool in sheep from a previously shorn area, an overview of the storage of cortisol in the wool over a certain period of time can be obtained. Due to the annual shearing of wool-producing sheep, such as the Merino breed, the amount of cortisol is more or less evenly distributed over the wool (Burnard et al., 2016; Sawyer et al., 2019; Hantzopoulou et al., 2022). However, the location on the body from which the wool sample is taken can affect the concentration of cortisol measured (Fürtbauer et al., 2019).
Hair has a smooth cuticle, is usually straight or slightly wavy (sometimes frizzy), and long. Hair follicles lie deep in the skin and have a direct blood supply. Hair growth is a cyclical process that is dependent on breed, season, and location on the body. To create a timeline it is necessary to know the growth rate, and to consider the possibility of declining levels of cortisol along the hair shaft (Heimbürge et al., 2019). Cortisol can only be incorporated in growing hair via passive diffusion during the anagen (growth phase) (Heimbürge et al., 2019). Since hair follicles have a better blood flow than wool follicles, cortisol transferred via the blood supply will lead to higher cortisol concentrations in hair than in wool (Burnard et al., 2016).
Studying the human maternal–fetal stress transfer revealed that during the first two trimesters, the mother is the main source of cortisol to which the fetus is exposed, although the cortisol that the fetus is exposed to is at a significantly lower level than that observed in the mother. In the third trimester, the fetus’s own HPA axis activity may contribute to cortisol accumulation (Meyer and Novak, 2021). In ewes, the concentrations of wool cortisol increased significantly toward the end of pregnancy, and the concentration after lambing did not decrease but remained significantly higher than before pregnancy (Liggins, 1994; Sawyer et al., 2019). In human fetuses, a large increase in blood cortisol is observed around the time of parturition. Shortly after parturition, the hair cortisol levels in newborns were significantly lower than those of their mothers (Meyer and Novak, 2021).
According to Liggins (1994), fetal organs mature quickly just before parturition to support the postpartum life, in a species-dependent process tightly coupled to mechanisms initiating parturition, processes both associated with cortisol. The increase in cortisol observed in fetal circulation in late pregnancy differs between precocious animals (e.g. sheep), in whom concentrations rise sharply prepartum and altricial animals such as rodents and humans, in whom slower increases are observed (Liggins, 1994).
4.2 Determining cortisol concentrations in wool - methodological considerations
Quantification of cortisol was carried out by different systems, ELISA (enzyme-linked immunosorbent assay), RIA (radioimmunoassay), or (LC-)MS ((liquid chromatography) - mass spectrometry). Although ELISA is less specific and sensitive, it is suitable for comparative hormone studies in animals and is the method that is most commonly used (70% of published reports) (Burnard et al., 2016). Comparison of ELISA with LC-MS, despite the latter being a very specific method, showed an undervalued true cortisol level (12% lower than ELISA), which was not due to the cross-reactivity (< 1%) of the ELISA (Greff et al., 2019).
Powdered hair yields higher cortisol levels than minced hair (Davenport et al., 2006; Meyer et al., 2014; Ghassemi Nejad et al., 2019; Ghassemi Nejad et al., 2020). Numerous grinding methods have been reported, including: finely mincing with scissors, pulverizing using a ball mill, using liquid nitrogen, using a TissueLyser, or in some cases not pulverizing, and using whole non-pulverized hair (Marceau et al., 2020). Based on the information that powdered hair yields higher cortisol levels, our wool samples were pulverized using a TissueLyser (bead beater). Powdering using a bead beater is now the preferred method as it makes the extraction of cortisol more consistent than using surgical scissors (Ghassemi Nejad et al., 2019). However, it is essential to realize that effects of particle size reduction during cortisol dissolution may be less important than consistency of methodology (Greff et al., 2019).
4.3 The age and the degree of contamination of wool/hair matters—applying the shave–reshave method
Shearing ewes approximately 50 days before the expected birth date and analyzing the cortisol concentration in the regrown wool could provide a retrospective indicator of stress of ewes that covered the period in which hair grows in the fetus, i.e., the third trimester of pregnancy. Applying the shave–reshave method is crucial for using cortisol concentration as a retrospective marker of stress while ensuring that there is a sufficient sample of actively growing hairs (Heimbürge et al., 2019).
The shave–reshave method may also help to control for another source of putative bias: namely that cortisol incorporated by external contamination is enhanced in distal hair segments of sheep (Fürtbauer et al., 2019), pigs, and cattle (Otten et al., 2022). Structural damage in older wool/hair may increase their permeability, and consequently their uptake of contaminants (e.g., feces, urine, and saliva), which in turn affects the measured cortisol concentrations (Salaberger et al., 2016). Contamination may be caused by lying on soiled floors (i.e., with feces and/or urine), or by saliva, or contact with the contaminated coat of conspecifics during social interactions (Heimbürge et al., 2020a). On the other hand, a decline in cortisol concentrations from proximal to distal hair segments has been found in different species due to a “washout effect” (Kirschbaum et al., 2009; Heimbürge et al., 2019). Damage to the wool/hair may also contribute to the washout of cortisol that has been stored (Otten et al., 2022). These two opposing effects may challenge the validity of wool/hair cortisol concentration being used as a long-term retrospective marker of stress (Sharpley et al., 2013), as damage, external contaminations, and “washout effects” affect the measured cortisol concentration in old hair. Consequently, the efficient and complete removal of contaminants is a crucial step in sample selection and sample processing (see also Supplementary Material 1).
4.4 Future directions
In future studies, it would be of interest to expose subgroups experimentally to different forms of stress during the last trimester of pregnancy. Ghassemi Nejad et al. (2014) found that hair cortisol was affected by exposure to heat stress in sheep that did not have immediate access to water after eating. Because the Swifter breed used in this study is easy to handle and does not panic easily (“Swifter sheep”; Farmow Livestock, 2022; “Swifter sheep”; Wikimedia Foundation, 2022), it may make sense to perform these experiments with more stress-sensitive sheep breeds.
Another interesting factor to take into account in future research is lamb weight. A previous study has shown that the blood plasma of lambs with a lower birth weight has higher cortisol values (Bloomfield et al., 2007). In addition, it has been observed earlier that offspring in larger litters have lower birth weights (e.g., sheep: Freetly and Leymaster, 2004; pigs: Wolf et al., 2008). The effects of litter size might thus be confounded by the birth weight of lambs. Unfortunately, birth weights were not recorded in the present study.
5 Conclusions
In conclusion, it is possible to reliably measure the cortisol concentration in the wool of sheep, but before it can be used as a valid indicator of (pregnancy-related) stress in sheep, and subsequently, as a proxy for animal welfare (Otovic and Hutchinson, 2015), more research needs to be carried out. According to Weaver et al. (2021), measuring cortisol in wool fibers appears to be an excellent matrix for assessing the effects of chronic stress in sheep. Preferably, the stress level of ewes should be manipulated experimentally during (the last trimester of) pregnancy and the shave–reshave method should be used. In addition, sheep breeds that are more stress-sensitive than Swifters should be studied. The stress response should be measured using both physiological and behavioral indices, eventually including other sampling matrices that are complementary to wool. We support the view of Rooke et al. (2015) that more scientific evidence is urgently needed regarding the effects of and responses to stressors in ewes and lambs, particularly on how the effects of different maternal stressors interact. The use of wool cortisol measurements as a retrospective marker of stress in ewes and lambs could be an additional tool in the toolbox to address these questions.
Data availability statement
The original contributions presented in the study are included in the article/Supplementary Material. Further inquiries can be directed to the corresponding author.
Ethics statement
Ethical review and approval was not required for the animal study because for this study wool of ewes and their lambs was used, which was collected in a non-invasive, pain free manner. According to Dutch law (“Wet op de dierproeven”, the Dutch Experiments on Animals Act from 18 Dec. 2014, §1, article 1, 1b, 13a), this study did not need approval by an ethics committee. Nonetheless, this study was registered, and the study protocol was evaluated by the Animal welfare body of Utrecht University, The Netherlands.
Author contributions
EZ: data collection, laboratory analyses, writing (original draft and editing). JV: conceptualization, formal analysis, writing (original draft, reviewing and editing) MB: data collection, writing (original draft). FS: conceptualization, formal analysis, and writing (original draft, review, and editing) RN: conceptualization; writing: review and editing. All authors contributed to the article and approved the submitted version.
Funding
Funding for this project was provided by Utrecht University, the Netherlands.
Acknowledgments
We would like to thank Lars Ravesloot for his supervision during the essay processing; Raoul Manraj for his help with processing the first batch of hair samples; Iris Siemons, Niek van Milligen, and Romy Röring for helping to collect all hair samples; and Patricia Gadella, Wim van Brenk, and Dirk van der Heide for taking care of the sheep.
Conflict of interest
The authors declare that the research was conducted in the absence of any commercial or financial relationships that could be construed as a potential conflict of interest.
Publisher’s note
All claims expressed in this article are solely those of the authors and do not necessarily represent those of their affiliated organizations, or those of the publisher, the editors and the reviewers. Any product that may be evaluated in this article, or claim that may be made by its manufacturer, is not guaranteed or endorsed by the publisher.
Supplementary material
The Supplementary Material for this article can be found online at: https://www.frontiersin.org/articles/10.3389/fanim.2023.1056726/full#supplementary-material
References
“Swifter sheep”; Farmow Livestock (2022). Available at: https://www.farmow.com/breed/swifter-sheep (Accessed August 1, 2022).
“Swifter sheep”; Wikimedia Foundation (2022). Available at: https://second.wiki/wiki/swifter_schaf (Accessed April 6, 2022).
Alon T., Matas D., Koren L., Gootwine E. (2021). Higher cortisol and testosterone levels in sheep with larger litter sizes. Livestock Sci. 243, 5. doi: 10.1016/j.livsci.2020.104381
Antonides A., Schoonderwoerd A. C., Nordquist R. E., van der Staay F. J. (2015). Very low birth weight piglets show improved cognitive performance in the spatial cognitive holeboard task. Front. Behav. Neurosci. 9. doi: 10.3389/fnbeh.2015.00043
Bacci M. L., Nannoni E., Govoni N., Scorrano F., Zannoni A., Forni M., et al. (2014). Hair cortisol determination in sows in two consecutive reproductive cycles. Reprod. Biol. 14, 218–223. doi: 10.1016/j.repbio.2014.06.001
Bates D., Maechler M., Bolker B., Walker S. (2015). Fitting linear mixed-effects models using lme4. J. Stat. Softw 67, 1–48. doi: 10.18637/jss.v067.i01
Bennett A., Hayssen V. (2010). Measuring cortisol in hair and saliva from dogs: coat color and pigment differences. Domest. Anim. Endocrinol. 39, 171–180. doi: 10.1016/j.domaniend.2010.04.003
Bloomfield F. H., Oliver M. H., Harding J. E. (2007). Effects of twinning, birth size, and postnatal growth on glucose tolerance and hypothalamic-pituitary-adrenal function in postpubertal sheep. Am. J. Physiol-Endocrinol Metab. 292, E231–E237. doi: 10.1152/ajpendo.00210.2006
Brydges N. M., Braithwaite V. A. (2008). Measuring animal welfare: what can cognition contribute? Annu. Rev. Biomed. Sci. 10, T91–T103. doi: 10.5016/1806-8774.2008.v10pT91
Buckley T. M., Schatzberg A. F. (2005). Review: on the interactions of the hypothalamic-pituitary-adrenal (HPA) axis and sleep: normal HPA axis activity and circadian rhythm, exemplary sleep disorders. J. Clin. Endocrinol. Metab. 90 (5), 3106–3114. doi: 10.1210/jc.2004-1056
Burnard C., Ralph C., Hynd P., Hocking Edwards J., Tilbrook A. (2016). Hair cortisol and its potential value as a physiological measure of stress response in human and non-human animals. Anim. Prod Sci. 57, 401–414. doi: 10.1071/AN15622
Burnett T. A., Madureira A. M. L., Silper B. F., Nadalin A., Tahmasbi A., Veira D. M., et al. (2014). Short communication: factors affecting hair cortisol concentrations in lactating dairy cows. J. Dairy Sci. 97, 7685–7690. doi: 10.3168/jds.2014-8444
Burnett T. A., Madureira A. M. L., Silper B. F., Tahmasbi A., Nadalin A., Veira D. M., et al. (2015). Relationship of concentrations of cortisol in hair with health, biomarkers in blood, and reproductive status in dairy cows. J. Dairy Sci. 98, 4414–4426. doi: 10.3168/jds.2014-8871
Casellas J., Caja G. (2014). Fetal programming by co-twin rivalry in sheep. J. Anim. Sci. 92, 64–71. doi: 10.2527/jas.2013-6677
Cloete J. H. L. (1939). Prenatal growth in the merino sheep. Onderstepoort J. Veterinary Sci. Anim. Industry 13, 417–487.
D’Anna-Hernandez K. L., Ross R. G., Natvig C. L., Laudenslager M. L. (2011). Hair cortisol levels as a retrospective marker of hypothalamic–pituitary axis activity throughout pregnancy: comparison to salivary cortisol. Physiol. Behav. 104, 348–353. doi: 10.1016/j.physbeh.2011.02.041
Davenport M. D., Tiefenbacher S., Lutz C. K., Novak M. A., Meyer J. S. (2006). Analysis of endogenous cortisol concentrations in the hair of rhesus macaques. Gen. Comp. Endocrinol. 147, 255–261. doi: 10.1016/j.ygcen.2006.01.005
del Rosario González-de-la-Vara M., Valdez R. A., Lemus-Ramirez V., Vázquez-Chagoyán J. C., Villa-Godoy A., Romano M. C. (2011). Effects of adrenocorticotropic hormone challenge and age on hair cortisol concentrations in dairy cattle. Can. J. Veterinary Res. 75, 216–221.
De Nys L., Anderson K., Ofosu E. F., Ryde G. C., Connelly J., Whittaker A. C. (2022). The effects of physical activity on cortisol and sleep: a systematic review and meta-analysis. Psychoneuroendocrinology 143, 105843. doi: 10.1016/j.psyneuen.2022.105843
Duerden J. E., Ritchie M. I. F. (1924). Development of the merino wool fibre. South Afr. J. Sci. 21, 480–497.
Fazio E. E. (2013). “Cortisol changes in pregnant and post-partum ewes: effects of single or twin births,” in Trends in veterinary sciences. Eds. Boiti C., Ferlazzo A., Gaiti A., Pugliese A. (Berlin, Heidelberg: Springer), 51–54.
Freetly H. C., Leymaster K. A. (2004). Relationship between litter birth eight and litter size in six breeds of sheep. J. Anim. Sci. 82, 612–618. doi: 10.2527/2004.822612x
Frodl T., O’Keane V. (2013). How does the brain deal with cumulative stress? a review with focus on developmental stress, HPA axis function and hippocampal structure in humans. Neurobiol. Dis. 52, 24–37. doi: 10.1016/j.nbd.2012.03.012
Fürtbauer I., Solman C., Fry A. (2019). Sheep wool cortisol as a retrospective measure of long-term HPA axis activity and its links to body mass. Domest. Anim. Endocrinol. 68, 39–46. doi: 10.1016/j.domaniend.2018.12.009
Gardner D. S., Jamall E., Fletcher A. J. W., Fowden A. L., Giussani D. A. (2004). Adrenocortical responsiveness is blunted in twin relative to singleton ovine fetuses. J. Physiol. 557, 1021–1032. doi: 10.1113/jphysiol.2004.061796
Ghassemi Nejad J., Lee B.-H., Kim J.-Y., Kim B.-W., Chemere B., Park K.-H., et al. (2019). Comparing hair cortisol concentrations from various body sites and serum cortisol in Holstein lactating cows and heifers during thermal comfort zone. J. Veterinary Behav. 30, 92–95. doi: 10.1016/j.jveb.2018.12.007
Ghassemi Nejad J., Lohakare J. D., Son J. K., Kwon E. G., West J. W., Sung K. I. (2014). Wool cortisol is a better indicator of stress than blood cortisol in ewes exposed to heat stress and water restriction. Animal 8, 128–132. doi: 10.1017/S1751731113001870
Ghassemi Nejad J., Park K.-H., Forghani F., Lee H.-G., Lee J.-S., Sung K.-I. (2020). Measuring hair and blood cortisol in sheep and dairy cattle using RIA and ELISA assay: a comparison. Biol. Rhythm. Res. 51, 813–823. doi: 10.1080/09291016.2019.1611335
Giussani D. A., Fletcher A. J. W., Gardner D. S. (2011). Sex differences in the ovine fetal cortisol response to stress. Pediatr. Res. 69, 118–122. doi: 10.1203/PDR.0b013e3182042a20
Gootwine E., Spencer T. E., Bazer F. W. (2007). Litter-size-dependent intrauterine growth restriction in sheep. Animal 1, 547–564. doi: 10.1017/S1751731107691897
Greff M. J. E., Levine J. M., Abuzgaia A. M., Elzagallaai A. A., Rieder M. J., van Uum S. H. M. (2019). Hair cortisol analysis: an update on methodological onsiderations and clinical applications. Clin. Biochem. 63, 1–9. doi: 10.1016/j.clinbiochem.2018.09.010
Guesdon V., Ligout S., Delagrange P., Spedding M., Lévy F., Laine A.-L., et al. (2012). Multiple exposures to familiar conspecific withdrawal is a novel robust stress paradigm in ewes. Physiol. Behav. 105, 203–208. doi: 10.1016/j.physbeh.2011.08.011
Guesdon V., Malpaux B., Delagrange P., Spedding M., Cornilleau F., Chesneau D., et al. (2013). Rapid effects of melatonin on hormonal and behavioral stressful responses in ewes. Psychoneuroendocrinology 38, 1426—1434. doi: 10.1016/j.psyneuen.2012.12.011
Guesdon V., Meurisse M., Chesneau D., Picard S., Lévy F., Chaillou E. (2015). Behavioral and endocrine evaluation of the stressfulness of single-pen housing compared to group-housing and social isolation conditions. Physiol. Behav. 147, 63–70. doi: 10.1016/j.physbeh.2015.04.013
Halsey L. G. (2019). The reign of the p-value is over: what alternative analyses could we employ to fill the power vacuum? Biol. Lett. 15 (8), 20190174. doi: 10.1098/rsbl.2019.0174
Hammer F., Stewart P. M. (2006). Cortisol metabolism in hypertension. Best Pract. Res. Clin. Endocrinol. Metab. 20, 337–353. doi: 10.1016/j.beem.2006.07.001
Hantzopoulou G.-C., Sawyer G., Tilbrook A., Narayan E. (2022). Intra- and inter- sample variation in wool cortisol concentrations of Australian merino lambs between twice or single shorn ewes. Front. Anim. Sci. 3 (9). doi: 10.3389/fanim.2022.890914
Heimbürge S., Kanitz E., Otten W. (2019). The use of hair cortisol for the assessment of stress in animals. Gen. Comp. Endocrinol. 270, 10–17. doi: 10.1016/j.ygcen.2018.09.016
Heimbürge S., Kanitz E., Tuchscherer A., Otten W. (2020a). Is it getting in the hair? – cortisol concentrations in native, regrown and segmented hairs of cattle and pigs after repeated ACTH administrations. Gen. Comp. Endocrinol. 295 (113534), 10. doi: 10.1016/j.ygcen.2020.113534
Heimbürge S., Kanitz E., Tuchscherer A., Otten W. (2020b). Within a hair’s breadth – factors influencing hair cortisol levels in pigs and cattle. Gen. Comp. Endocrinol. 288 (8), 113359. doi: 10.1016/j.ygcen.2019.113359
Hinch G. (2013) Lecture 5: managing weaners and breeding ewes for wool production [WWW document]. WOOL412/512 sheep production. Available at: https://www.woolwise.com/wp-content/uploads/2017/07/WOOL-412-512-12-T-05.pdf.
Kalra S., Einarson A., Karaskov T., Van Uum S., Koren G. (2007). The relationship between stress and hair cortisol in healthy pregnant women. Clin. Invest. Med. 30, E103–E107. doi: 10.25011/cim.v30i2.986
Kapoor A., Schultz-Darken N., Ziegler T. E. (2018). Radiolabel validation of cortisol in the hair of rhesus monkeys. Psychoneuroendocrinology 97, 190–195. doi: 10.1016/j.psyneuen.2018.07.022
Kirschbaum C., Tietze A., Skoluda N., Dettenborn L. (2009). Hair as a retrospective calendar of cortisol production - increased cortisol incorporation into hair in the third trimester of pregnancy. Psychoneuroendocrinology 34, 32–37. doi: 10.1016/j.psyneuen.2008.08.024
Knott S. A., Cummins L. J., Dunshea F. R., Leury B. J. (2010). Feed efficiency and body composition are related to cortisol response to adrenocorticotropin hormone and insulin-induced hypoglycemia in rams. Domest. Anim. Endocrinol. 39, 137–146. doi: 10.1016/j.domaniend.2010.03.003
Lee D. Y., Kim E., Choi M. H. (2015). Technical and clinical aspects of cortisol as a biochemical marker of chronic stress. BMP Rep. 48, 209–216. doi: 10.5483/BMBRep.2015.48.4.275
Li J., Xie Q., Gao W., Xu Y., Wang S., Deng H., et al. (2012). Time course of cortisol loss in hair segments under immersion in hot water. Clin Chim Acta 413, 434–440. doi: 10.1016/j.cca.2011.10.024
Liggins G. C. (1994). The role of cortisol in preparing the fetus for birth. Reprod Fertility Dev. 6, 141–150. doi: 10.1071/rd9940141
Magyar D. M., Fridshal D., Elsner C. W., Glatz T., Eliot J., Klein A. H., et al. (1980). Time-trend analysis of plasma cortisol concentrations in the fetal sheep in relation to parturition. Endocrinology 107, 155–159. doi: 10.1210/endo-107-1-155
Marceau K., Wang W., Robertson O., Shirtcliff E. A. (2020). A systematic review of hair cortisol during pregnancy: reference ranges and methodological considerations. Psychoneuroendocrinology 122 (10), 104904. doi: 10.1016/j.psyneuen.2020.104904
McDowall M. L., Watson-Haigh N. S., Edwards N. M., Kadarmideen H. N., Nattrass G. S., McGrice H. A., et al. (2013). Transient treatment of pregnant merino ewes with modulators of cortisol biosynthesis coinciding with primary wool follicle initiation alters lifetime wool growth. Anim. Prod Sci. 53 (10), 1101–1111. doi: 10.1071/AN12193
Meyer J. S., Novak M. A. (2021). Assessment of prenatal stress-related cortisol exposure: focus on cortisol accumulation in hair and nails. Dev. Psychobiol 63, 409–436. doi: 10.1002/dev.22021
Meyer J., Novak M., Hamel A., Rosenberg K. (2014). Extraction and analysis of cortisol from human and monkey hair. J. Visualized Experiments 83, 1–6, e50882. doi: 10.3791/50882
Micale V., Drago F. (2018). Endocannabinoid system, stress and HPA axis. Eur. J. Pharmacol. 834, 230–239. doi: 10.1016/j.ejphar.2018.07.039
Miller G. E., Chen E., Zhou E. S. (2007). If it goes up, must it come down? chronic stress and the hypothalamic-pituitary-adrenocortical axis in humans. psychol. Bull. 133, 25–45. doi: 10.1037/0033-2909.133.1.25
Mohd Azmi N. A. S., Juliana N., Azmani S., Mohd Effendy N., Abu I. F., Mohd Fahmi Teng N. I., et al. (2021). Cortisol on circadian rhythm and its effect on cardiovascular system. Int. J. Environ. Res. Public Health 17 (15). doi: 10.3390/ijerph17240000
Nazifi S., Saeb M., Rowghani E., Kaveh K. (2003). The influences of thermal stress on serum biochemical parameters of Iranian fat-tailed sheep and their correlation with triiodothyronine (T 3), thyroxine (T 4) and cortisol concentrations. Comp. Clin. Pathol. 12, 135–139. doi: 10.1007/s00580-003-0487-x
Nedić S., Pantelić M., Vranješ-Ðurić S., Nedić D., Jovanović L., Čebulj-Kadunc, et al. (2017). Cortisol concentrations in hair, blood and milk of Holstein and busha cattle. Slovenian Veterinary Res. 54, 163–172. doi: 10.26873/SVR-398-2017
Nordquist R. E., van der Staay F. J., van Eerdenburg F. J. C. M., Velkers F. C., Fijn L., Arndt S. S. (2017). Mutilating procedures, management practices, and housing conditions that may affect the welfare of farm animals: implications for welfare research. Animals 7 (22), 12. doi: 10.3390/ani7020012
Oakley R. H., Cidlowski J. A. (2013). The biology of the glucocorticoid receptor: new signaling mechanisms in health and disease. J. Allergy Clin. Immunol. 132, 1033–1044. doi: 10.1016/j.jaci.2013.09.007
Ochoa Cordero M., Meza Herrera C. A., Vázquez G J. M., Stewart C. A., Rosales Nieto C. A., Ochoa Alfaro A. E., et al. (2019). Pregnancy and litter size, but not lamb sex, affect feed intake and wool production by merino-type ewes. Anim. 214, 11. doi: 10.3390/ani9050214
Olejniczak I., Oster H., Ray D. W. (2022). Glucocorticoid circadian rhythms in immune function. Semin. Immunopathol 44, 153–163. doi: 10.1007/s00281-021-00889-2
Otovic P., Hutchinson E. (2015). Limits to using HPA axis activity as an indication of animal welfare. ALTEX: Alternativen Zu Tierexperimenten 32, 4–50. doi: 10.14573/altex.1406161
Otten W., Heimbürge S., Tuchscherer A., Kanitz E. (2022). The age of hair matters – the incorporation of cortisol by external contamination is enhanced in distal hair segments of pigs and cattle. Animal 16 (6), 100495. doi: 10.1016/j.animal.2022.100495
Percie du Sert N., Ahluwalia A., Alam S., Avey M. T., Baker M., Browne W. J., et al. (2020). Reporting animal research: explanation and elaboration for the ARRIVE guidelines 2.0. PloS Biol. 18 (7), e3000411. doi: 10.1371/journal.pbio.3000411
Rakers F., Rupprecht S., Dreiling M., Bergmeier C., Witte O. W., Schwab M. (2020). Transfer of maternal psychosocial stress to the fetus. Neurosci. Biobehav. Rev. 117, 185–197. doi: 10.1016/j.neubiorev.2017.02.019
R Core Team (2021). R: a language and environment for statistical computing. (Vienna, Austria: R Foundation for Statistical Computing).
Roelofs S., Godding L., de Haan J. R., van der Staay F. J., Nordquist R. E. (2019). Effects of parity and litter size on cortisol measures in commercially housed sows and their offspring. Physiol. Behav. 201, 83–90. doi: 10.1016/j.physbeh.2018.12.014
Rooke J. A., Arnott G., Dwyer C. M., Rutherford K. M. D. (2015). The importance of the gestation period for welfare of lambs: maternal stressors and lamb vigour and wellbeing. J. Agric. Sci. 153, 497–519. doi: 10.1017/S002185961400077X
Russell E., Kirschbaum C., Laudenslager M. L., Stalder T., de Rijke Y., van Rossum E. F. C., et al. (2015). Toward standardization of hair cortisol measurement: results of the first international interlaboratory round robin. Ther. Drug Monit. 37, 71–77. doi: 10.1097/FTD.0000000000000148
Russell E., Koren G., Rieder M., Van Uum S. (2012). Hair cortisol as a biological marker of chronic stress: current status, future directions and unanswered questions. Psychoneuroendocrinology 37, 589—601. doi: 10.1016/j.psyneuen.2011.09.009
Rutherford K. M. D., Baxter E. M., D’Eath R. B., Turner S. P., Arnott G., Roehe R., et al. (2013). The welfare implications of large litter size in the domestic pig I: biological factors. Anim. Welfare 22, 199–218. doi: 10.7120/09627286.22.2.199
Salaberger T., Millard M., El Makarem S., Möstl E., Grünberger V., Krametter-Frötscher R., et al. (2016). Influence of external factors on hair cortisol concentrations. Gen. Comp. Endocrinol. 233, 73–78. doi: 10.1016/j.ygcen.2016.05.005
Sales F., Peralta O. A., Narbona E., McCoard S., De los Reyes M., González-Bulnes A., et al. (2018). Hypoxia and oxidative stress are associated with reduced fetal growth in twin and undernourished sheep pregnancies. Anim. 2177, 13. doi: 10.3390/ani811021
Sawyer G., Fox D. R., Narayan E. (2021). Pre- and post-partum variation in wool cortisol and wool micron in Australian merino ewe sheep (Ovis aries). PeerJ 17. doi: 10.7717/peerj.11288
Sawyer G., Webster D., Narayan E. (2019). Measuring wool cortisol and progesterone levels in breeding maiden Australian merino sheep (Ovis aries). PloS One 14 (4), e0214734. doi: 10.1371/journal.pone.0214734
Schober P., Boer C., Schwarte L. A. (2018). Correlation coefficients: appropriate use and interpretation. Anesth. Analgesia 126, 1763–1768. doi: 10.1213/ANE.0000000000002864
Scobie D. R., Hynd P. I. (1995). Reduction of mitotic rate in the wool follicle by cortisol. Aust. J. Agric. Res. 46 (2), 319–331. doi: 10.1071/AR9950319
Serpell J. A. (2019). How happy is your pet? the problem of subjectivity in the assessment of companion animal welfare. Anim. Welfare 28, 57–66. doi: 10.7120/09627286.28.1.057
Sharpley C. F., McFarlane J. R., Slominski A. (2013). Stress-linked cortisol concentrations in hair: what we know and what we need to know. Rev. Neurosci. 23, 111–121. doi: 10.1515/RNS.2011.058
Silverman M. N., Pearce B. D., Biron C. A., Miller A. H. (2005). Immune modulation of the hypothalamic-pituitary-adrenal (HPA) axis during viral infection. Viral Immunol. 18 (1), 41–78. doi: 10.1089/vim.2005.18.41
Stubsjøen S. M., Bohlin J., Dahl E., Knappe-Poindecker M., Fjeldaas T., Lepschy M., et al. (2015). Assessment of chronic stress in sheep (part i): the use of cortisol and cortisone in hair as non-invasive biological markers. Small Ruminant Res. 132, 25–31. doi: 10.1016/j.smallrumres.2015.09.015
Timmermans S., Souffriau J., Libert C. (2019). A general introduction to glucocorticoid biology. Front. Immunol. 10, 1545. doi: 10.3389/fimmu.2019.01545
Toland Thompson A. C., Hebart M. L., Penno N. M., Hynd P. I. (2007). Perinatal wool follicle attrition coincides with elevated perinatal circulating cortisol concentration in merino sheep. Aust. J. Agric. Res. 58 (7), 748–752. doi: 10.1071/AR06327
van der Laan J. E., Vinke C. M., Arndt S. S. (2022). Evaluation of hair cortisol as an indicator of long−term stress responses in dogs in an animal shelter and after subsequent adoption. Sci. Rep. 12, 5117. doi: 10.1038/s41598-022-09140-w
van Eck L. M., Antonides A., Nordquist R. E., van der Staay F. J. (2016). Testing post-weaning food motivation in low and normal birth weight pigs in a runway and operant conditioning task. Appl. Anim. Behav. Sci. 181, 83–90. doi: 10.1016/j.applanim.2016.05.010
van Eerdenburg F. J. C. M., Hof T., Doeve B., Ravesloot L., Zeinstra E. C., Nordquist R. E., et al. (2021). The relation between hair-cortisol concentration and various welfare assessments of Dutch dairy farms. Animals 11 (17), 821. doi: 10.3390/ani11030821
van Houtert E. A. E., Endenburg N., Vermetten E., Rodenburg T. B. (2022). Hair cortisol in service dogs for veterans with post-traumatic stress disorder compared to companion dogs (Canis familiaris). J. Appl. Anim. Welfare Sci. 11. doi: 10.1080/10888705.2022.2033119
Weaver S. J., Hynd P. I., Ralph C. R., Hocking Edwards J. E., Burnard C. L., Narayan E., et al. (2021). Chronic elevation of plasma cortisol causes differential expression of predominating glucocorticoid in plasma, saliva, fecal, and wool matrices in sheep. Domest. Anim. Endocrinol. 74, 105503. doi: 10.1016/j.domaniend.2020.106503
Wester V. L., van Rossum E. F. C. (2015). Clinical applications of cortisol measurements in hair. Eur. J. Endocrinol. 173, M1–M10. doi: 10.1530/EJE-15-0313
Witchel S. F., DeFranco D. B. (2006). Mechanisms of disease: regulation of glucocorticoid and receptor levels–impact on the metabolic syndrome. Nat. Clin. Pract. 2, 621–631. doi: 10.1038/ncpendmet0323
Wolf J., Žáková E., Groeneveld E. (2008). Within-litter variation of birth weight in hyperprolific Czech Large white sows and its relation to litter size traits, stillborn piglets and losses until weaning. Livestock Sci. 115, 195–205. doi: 10.1016/j.livsci.2007.07.009
Wooddell L. J., Kaburu S. S. K., Dettmer A. M. (2021). Behavioral and hormonal changes following social instability in young rhesus macaques (Macaca mulatta). J. Comp. Psychol. 135, 568–580. doi: 10.1037/com0000297
Keywords: wool cortisol, stress, Ovis aries, parity, litter size, sex differences, sheep, livestock welfare
Citation: Zeinstra EC, Vernooij JCM, Bentvelzen M, van der Staay FJ and Nordquist RE (2023) Wool cortisol as putative retrospective indicator of stress in ewes during the third trimester of pregnancy, and their newborns: effects of parity and litter size — an exploratory study. Front. Anim. Sci. 4:1056726. doi: 10.3389/fanim.2023.1056726
Received: 29 September 2022; Accepted: 09 May 2023;
Published: 31 May 2023.
Edited by:
Edward Narayan, The University of Queensland, AustraliaReviewed by:
Georgia-Constantina Hantzopoulou, The University of Queensland, AustraliaGregory John Lynn Sawyer, The University of Sydney, Australia
Elodie Chaillou, UMR7247 Physiologie de la Reproduction et des Comportements (PRC), France
Copyright © 2023 Zeinstra, Vernooij, Bentvelzen, van der Staay and Nordquist. This is an open-access article distributed under the terms of the Creative Commons Attribution License (CC BY). The use, distribution or reproduction in other forums is permitted, provided the original author(s) and the copyright owner(s) are credited and that the original publication in this journal is cited, in accordance with accepted academic practice. No use, distribution or reproduction is permitted which does not comply with these terms.
*Correspondence: Rebecca E. Nordquist, ci5lLm5vcmRxdWlzdDFAdXUubmw=
†These authors have contributed equally to this work