- 1Department of Animal Sciences, Isfahan (Khorasgan) Branch, Islamic Azad University, Isfahan, Iran
- 2Department of Animal Sciences, College of Agriculture, Isfahan University of Technology, Isfahan, Iran
- 3Animal Science Research Department, Isfahan Agriculture and Natural Resources Research and Education Center, Agriculture Research, Education and Extension Organization (AREEO), Isfahan, Iran
- 4Department of Animal Science, College of Agriculture and Natural Resources, University of Tehran, Karaj, Iran
- 5Bahadoran Laboratory of Animal Nutrition and Feed Science, Isfahan, Iran
Introduction: Aromatic and herbal plants usage as feed additives have become a new tendency in dairy cows’ nutrition to enhance animal performance. This experiment was performed to study the effects of supplementing fennel seed powder (FSP) to diets during early lactation on performance, milk fatty acid (FA) profile, and rumen fermentation of Holstein dairy cows.
Methods: Twenty-four primiparous Holstein dairy cows (10 ± 3 d in milk, 30 ± 2.1 Kg of milk/d, 610 ± 31 Kg body weight; mean ± SE) were balanced for actual milk yield and calving date (n = 8 per treatment) in a complete randomized design. Animals were allocated randomly to diets containing 0 g/d (0FSP), 25 g/d (25FSP), or 50 g/d (50FSP) FSP, individually top-dressed over the total mixed ration. The experimental period was 45 d consisting of the first 15 d for adaptation and the final 30 d for data collection and sampling.
Results and discussion: Dry matter intake responded quadratically to FSP feeding, and cows fed 25FP treatment had greater DMI than 0FSP treatment. The average ruminal pH value decreased linearly as FSP increased in diets. Ruminal valerate and isovalerate proportion increased linearly as FSP inclusion in diets increased, while acetate proportion decreased and acetate:propionate ratio tended to decrease linearly. Increasing FSP in diets linearly increased serum glucose, globulin, and total protein concentrations. Milk yield increased linearly as FSP inclusion in diets increased, whereas milk composition was unaffected. Increasing FSP in diets linearly increased de novo and mixed FA and decreased preformed FA in milk. Dietary treatments did not affect saturated FA, whereas unsaturated FA, mono and poly unsaturated FA linearly decreased with increasing FSP inclusion in diets. Moreover, the content of C18:0 tended to decrease, and C18:1 cis-9 decreased linearly as FSP inclusion increased. Also, increasing the FSP level in diets decreased linearly non-esterified fatty acids and acetone concentrations in the milk. It could be concluded that FSP addition at 50 g/d could enhance performance of early lactating cows.
Introduction
Cows experience negative nutrient balances after calving for several weeks because dry matter intake (DMI) is less than needed to support milk yield. This imbalance between nutrient intake and demand in early lactation leads to negative energy balance (NEB) and energy mobilization from body fat tissue (Hashemzadeh-Cigari et al., 2015). Mobilization of adipose tissue during NEB increased concentration of non-esterified fatty acids (NEFA) and beta-hydroxybutyric acid (BHBA) during early lactation, which is associated with metabolic and immune dysfunctions such as ketosis and lipidosis. Therefore, dietary strategies should be considered to optimize the metabolism rate of lactating cows during the early lactation period.
Feed additives are used in dairy cow nutrition for different reasons, including covering needs from essential nutrients, increasing feed intake, optimizing feed utilization, and improving overall performance. Phytogenics, as bioactive plant compounds, have been used as feed additives to promote productivity and prevent diseases in human and animal nutrition (Rochfort et al., 2008). The beneficial effects of phytogenics on animals’ performance may be associated with their positive effect on intake (Saeedi et al., 2017) and nutrient digestion (Mahmoud et al., 2020; Fahim et al., 2021) or their characteristics as antibacterial, antioxidative, and immunostimulation (Hashemzadeh et al., 2022).
Fennel (Foeniculum vulgare) is an aromatic plant belonging to the Apiaceae family. Limonene, anethole, α-pinene, fenchone, estragole, and fenchol are the main phytoconstituents of fennel (Hajalizadeh et al., 2019). Because of these essential oil and bioactive compounds, fennel has aromatic (due to essential oils such as fenchone, trans-anethole, estragole, and limonene), antioxidant and anti-inflammatory (due to flavonoids, essential oils, phenolic acids, hydroxycinnamic acids, coumarin, and tannin), estrogenic (due to photoanethole and dianethole), antimicrobial (due to 1,3-benzenediol, oleic acid, undecanal, linoleic acid, 5-hydroxy-furanocoumarin, and 2,4-undecadienal) properties (Badgujar et al., 2014; Kargar et al., 2021; Nowroozinia et al., 2022). The fennel antioxidants inhibit the gastrointestinal tract contractions induced by acetylcholine and histamine (Badgujar et al., 2014; Kooti et al., 2015). Also, essential oils in fennel decrease gas production in the gastrointestinal by regulating motility of intestinal smooth muscle (Kooti et al., 2015).
Moreover, it was reported in humans that fennel has positive effects on some metabolism-related disorders, including regulating the appetite and improving hyperlipidemia, mainly by influencing the expression of insulin and leptin receptors (Zakernezhad et al., 2021). Also, estrogens are crucial hormonal regulators of systemic energy homeostasis via regulating adipose tissue metabolism. Estrogens seem to display beneficial actions on insulin and glucose metabolism through the suppression of adipose lipolysis resulting in decreased circulating free FAs levels and the modulation of expression and/or secretion of adipocytokines for the improvement of insulin sensitivity (Kim et al., 2014). Furthermore, Samadi-Noshahr et al. (2021) reported that FSP had hypolipidemic effects via its antioxidant properties and ability to stimulate insulin secretion. These results indicate that FSP may prevent body fat mobilization in fresh cows by increasing insulin sensitivity and reducing lipolysis.
Mahmoud et al. (2020) observed improved milk yield with fennel seed powder (FSP) addition at 0.7% of the diet of crossbreed lactating cows for 90 days. Furthermore, FSP supplementation increased yield of milk and energy-corrected milk (ECM) by 10.5 and 16%, respectively, in early lactating buffaloes (Fahim et al., 2021). Also, Attari et al. (2018) evaluated the effects of supplementing dairy cows (30 days in milk) with a photogenic mixture containing FSP at 0.03% of body weight for 63 days. They reported that FSP addition increased actual and component-corrected milk yield without affecting milk composition. Saeedi et al. (2017) observed that supplementation of FSP to the diet of Holstein dairy calves for two weeks increased average daily gain and decreased the age of weaning. Furthermore, Hajalizadeh et al. (2019) stated that FSP addition at 1.5% of diet to growing lambs for 80 days increased DMI and final body weight.
van Haelst et al. (2008) reported that the mobilization of adipose tissue happens earlier than the development of ketosis in the early lactation cows and mobilized FAs are transferred into milk fat; hence, milk long-chain FAs profile change may be a valuable tool for early diagnosis of hyperketonemia. Also, Churakov et al. (2021) indicated that milk FAs could be used to address NEB in early lactation cows and concentrations of C18:0 and C18:1 cis-9 are the best variables for early detection of cows with severe NEB. Moreover, it was reported that supplementation of FSP affected milk fatty acid (FA) profile. Mahmoud et al. (2020) reported increased omega-3, polyunsaturated FAs, and conjugated linoleic acid (CLA), while decreased saturated FAs proportions in lactating cows received fennel at 0.7% of diet. For these reasons, milk FAs profile was measured in the current experiment to investigate the effect of FSP on the energy balance of early lactation dairy cows.
To our knowledge, no data are available on the effects of FSP on performance, milk FAs profile, rumen fermentation, and blood parameters of early lactating cows. We hypothesized that feeding FSP would improve performance without increasing body fat mobilization in dairy cows. Therefore, the objective of the present study was to investigate the impact of increasing levels of FSP as feed additives on DMI, milk yield and FAs profile, rumen fermentation, and some blood parameters of dairy cows during early lactation.
Materials and methods
All animal procedures were approved by the Animal Care and Use Committee of Isfahan University of Technology (IUT, Iran; IACUC #2019/B15) as set by the Iranian Council of Animal Care (1995). The experiment was performed from January to March 2022, at the Saberi poor Animal Husbandry Farm, Nazar Abad, Karaj, Iran (35.9030’N, 50.6630’E).
Animals and treatments
Twenty-four primiparous Holstein dairy cows (10 ± 3 days in milk, 30 ± 2.1 kg of milk/d, 610 ± 31 kg of body weight) were blocked by actual milk yield and calving date (n = 8 per treatment) in a complete randomized design. Cows were housed in individual pens (4 × 4 m) with concrete floors cleaned regularly and fed a total mixed ration (TMR) ad libitum intake. Dietary treatments were as follows: 1- diet containing 0 g of FSP (0FSP); 2- diet containing 25 g FSP (25FSP); 3- diet containing 50 g of FSP (50FSP). The FSP dosage was chosen from previous studies (Kargar et al., 2021; Nowroozinia et al., 2022), in which 1.5 and 3 g/d FSP (0.041 and 0.082 g FSP/d per kg BW; mean calf’s BW of 36.3 kg) significantly improved the average daily gain and health indicators in dairy calves. Accordingly, we used 0.041 and 0.082 g FSP/d per kg of cow BW, corresponding to 25 and 50 g/d of FSP per cow, with the mean cow’s BW of 610 kg.
Chemical components of FSP were measured according to the method described by Ansari et al. (2022). Briefly, FSP (30 g; n = 3) was subjected to hydrodistillation in 1,000 mL of distilled water for 3 h using a Clevenger-type apparatus (HeatingMantle; Sci FineTech Co.). After dehydration over anhydrous sodium sulfate (CAS #7757–82–6), the percentage of oil was calculated. The prepared oil was stored in sealed vials in the dark at 4°C and then subjected to GC and GC-MS analyses to identify of constituents (Ansari et al., 2022; Table 1).
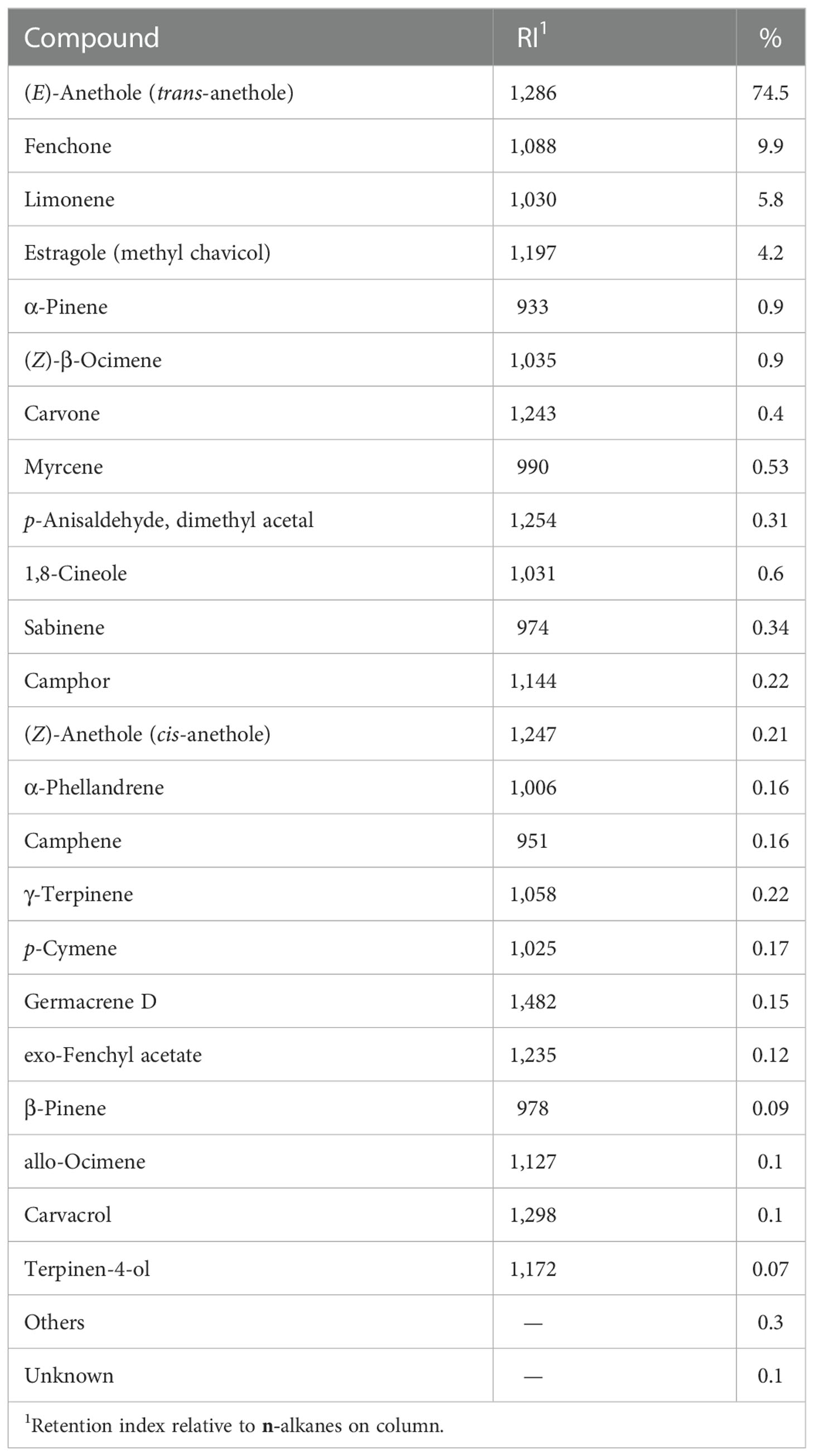
Table 1 Chemical components based on the total essential oil (%) distilled from fennel (Foeniculum vulgare) seed (n = 3)..
The forage-to-concentrate ratio of the basal diet was 39:61 on a DM basis, with alfalfa hay and corn silage as the forage sources. Before feeding, alfalfa hay was chopped with a theoretical length of 30 mm using a harvesting machine with a screen size regulator (model NPC260, Niroupayeh Co., Karaj, Iran). Corn silage was used from the silo and sampled weekly for DM content, and its inclusion in the TMR has been adjusted accordingly. Corn and barely grains were ground using a hammer mill with 2 and 3 mm screen sizes, respectively (model AHM80/55, Avartin Co., Tehran, Iran). The TMR was prepared daily and offered twice at 08:00 and 16:00 in an equal amount to ensure 5 to 10% feed refusals. Ingredients and chemical composition of diets are shown in Table 2. Diets were formulated to meet or exceed nutrient requirements stipulated by the NRC (2001). Fennel seed powder was dispersed into 200 g of ground corn grain (as-fed basis) and top-dressed twice daily by mixing into the top portion of the TMR at each feeding. Before feeding, FSP was ground to pass through a 1-mm screen in a Wiley mill (Ogawa Seiki Co. Ltd., Tokyo, Japan). Experimental period was 45 d and cows were adapted to experimental diets during the first 15 d of the trial and the last 30 d for sampling and data collection. Cows had free access to fresh water and diets and were milked thrice daily.
Sampling, measurements, and analyses
Feed intake: The amounts of TMR offered was weighed daily during the sampling period for each cow, and orts were recorded daily every morning before feeding. The TMR and orts samples were taken daily and dried at 68°C for 48 h in a forced-air oven for DMI calculation. Feed intake was recorded by subtracting the total amount of TMR offered daily from that of refusals. Moreover, dried TMR samples were ground to pass a 1-mm screen in a mill (Ogaw Seiki CO., Ltd., Tokyo, Japan) and analyzed (AOAC, 2002) for DM (method 925.40), CP (method 2001.11), ether extract (method 920.39), and ash (method 942.05). Neutral detergent fiber (NDF) content was determined with the methods described by Van Soest et al. (1991) using heat stable α-amylase.
Rumen parameters: Rumen fluid was sampled on day 37 of study at 3 h after the morning feed. Rumen content collecting was performed through the rumen using a custom-designed stomach pump and a tube at one end into a 500 mL container as described by Geishauser (1993). The first 200 mL of fluid pumped out was discarded, and the subsequent was filtered with 4 layers of cheesecloth and immediately after filtration pH was analyzed with a portable pH meter (HI 8318; Hanna Instruments, Cluj-Napoca, Romania). After that, two ruminal fluid subsamples were taken and mixed with chilled 25% (wt/vol) metaphosphoric acid (H3PO4) and stored at -20°C for later determination of volatile fatty acids (VFA) and NH3-N (Heidari et al., 2022). After thawing, the colorimetric phenol-hypochlorite method was used to determine NH3-N concentration (Broderick and Kang, 1980). Also and for VFA analysis, another rumen subsample was thawed and centrifuged at 10,000 × g at 4°C for 20 min and analyzed using gas chromatography (0.25 × 0.32, 0.3 μm i.d. fused silica capillary, model No. CP-9002 Vulcanusweg 259 a.m., Chrompack, Delft, the Netherlands), as described by Bal et al. (2000).
Blood parameters: Blood samples were collected from the coccygeal vein on days 20 and 40 into heparinized tubes (7 mL per sample; Vacutainer, Becton Dickinson, Franklin Lakes, NJ) 3 h after the morning feeding and then immediately placed in iced water. The samples were centrifuged at 3000 rpm for 15 min. The obtained plasma was aliquoted in 1.5-mL microtubes and stored at -20°C until further analyses. After experimental period, plasma samples were thawed and concentrations of glucose (Kit No. 93008), total protein (Kit No. 9304), cholesterol (Kit No. 2118), albumin (Kit No. 9307), and triglyceride (Kit No. 2109) were determined via an automatic analyzer (Alcyon-300 Auto analyzer; DRG Instruments GmbH, Marburg, Germany) using commercially available kits (Pars Azmoon, Tehran, Iran). Serum globulin was determined by subtracting albumin from total plasma protein (Ansari et al., 2022).
Milk yield and composition: Milk yield was recorded thrice daily at 0900, 1700, and 0100 h. Yields were recorded in the last 30 d of the experimental period and averaged to determine the daily mean milk yield. Milk was sampled on d 41 until 45 of study, and samples were preserved with potassium dichromate and held at 4°C pending analysis. Milk samples were submitted to Ideh Sazan Rojan Alvand Co. (Alborz, Iran) for fat, true protein, lactose, solids not-fat (SNF), total solids (TS), milk urea nitrogen (MUN), NEFA, BHBA, and FAs analyses using Fourier-transform mid-infrared spectroscopy (FTIR) of CombiScope FTIR 600 HP (Delta Instruments, Drachten, The Netherlands). This method could be helpful for evaluating the energy status and NEB in early lactating cows (McParland and Berry, 2016; Daneshvar et al., 2021). Milk composition for each day was calculated from the composite of each milking corrected for milk yield.
Yields of 3.5% fat corrected milk (FCM, kg/d) and energy corrected milk (ECM, kg/d) were calculated according to the following equations as described by Dairy Records Management Systems (2014):
Feed efficiency (FE) was calculated as actual and component-corrected milk yield (kg/d) divided by DMI (kg/d).
Statistical analysis
Data were analyzed as a complete randomized design using the mixed model procedure of SAS (2002). The statistical model applied included the effects of treatment as a fixed effect and cow within treatment as a random effect, and time (day) entered the model as a repeated statement. Treatments were balanced for actual milk yield and calving date. The following model was used: Yijk = µ + Ti + Dj + (TD)ij + C(T)ik + Eijk, where Y is the dependent variable; Ti is the fixed effect of treatment (i = 1, 2, 3); Dj is the fixed effect of time (j = 1, 2, 3, ……, 30); (TD)ij is the interaction effect between time and treatment; C(T)ik is the random effect of cow within treatment; and Eijk is the residual error. The data were checked for normality and where necessary, suitable Box-Cox transformations were identified using the TRANSREG procedure to normalize the distribution of a particular dataset. The different variance-covariance matrices (Huynh-Feldt (HF), compound symmetry (CS), Toeplitz (TOEP), and first-order autoregressive) were tested and the lowest corrected Akaike’s information criterion (AIC) was used for choosing the best matrix. Linear and quadratic contrasts were tested. Significance was declared at P< 0.05, and trends at P< 0.10.
Results
Dry matter intake and rumen fermentation parameters are indicated in Table 3. The DMI responded quadratically to FSP feeding (P = 0.040), and cows fed 25FSP treatment had greater DMI than 0FSP treatment. Ruminal pH decreased linearly as FSP increased in diets, and the average value for cows fed 0FSP, 25FSP, and 50FSP diets were 7.05, 6.86, and 6.79, respectively (P = 0.044). No differences in ruminal NH3-N and total VFA concentrations occurred among treatments. Valerate (P = 0.001) and isovalerate (P = 0.012) proportion increased linearly as FSP inclusion in diets increased, while acetate proportion decreased (P = 0.022) and acetate:propionate ratio tended to decrease (P = 0.072) linearly.
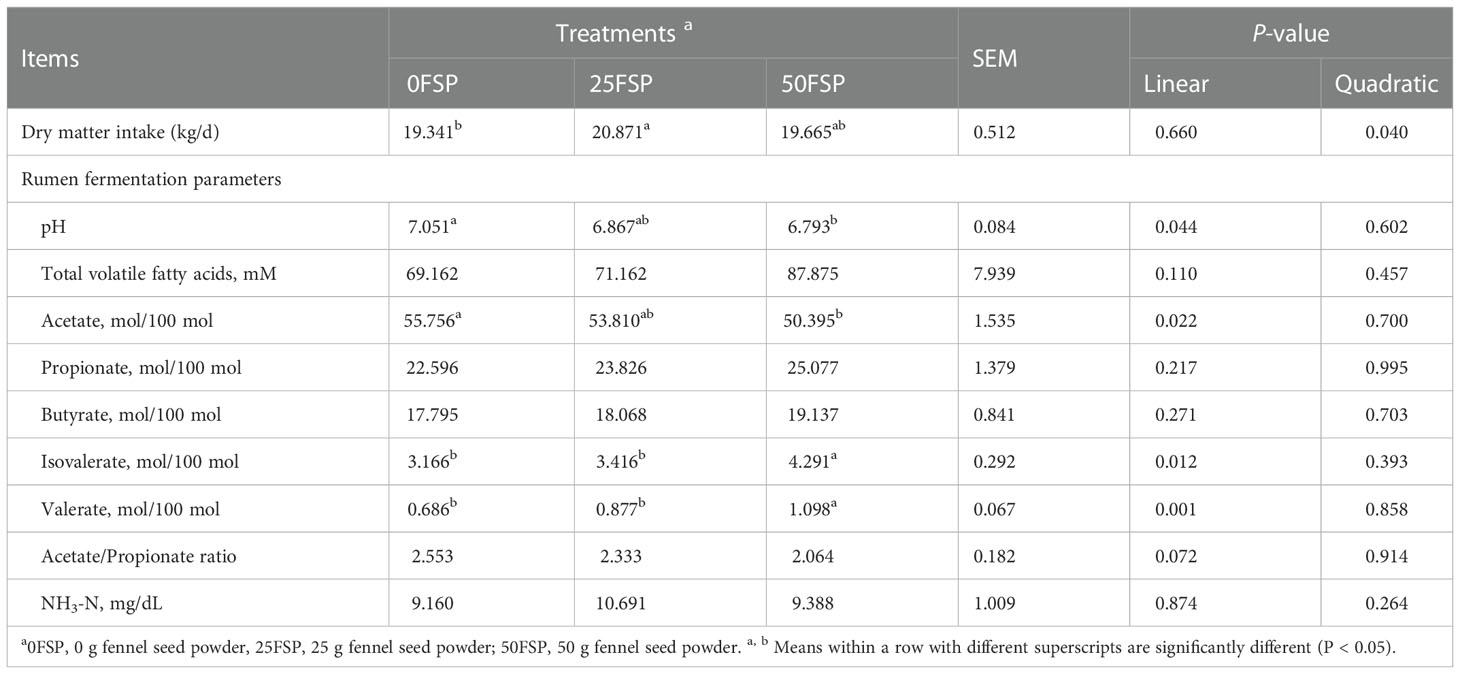
Table 3 Dry matter intake and rumen fermentation parameters of early lactating Holstein dairy cows (n = 8 per treatment) fed a basal diet supplemented with fennel seed powder (FSP).
Increasing FSP in diets linearly increased plasma glucose (P = 0.008), globulin (P = 0.005), and TP (P = 0.08) concentrations, whereas plasma cholesterol (P = 0.53) and triglyceride (P = 0.15) concentrations were not affected (Table 4).
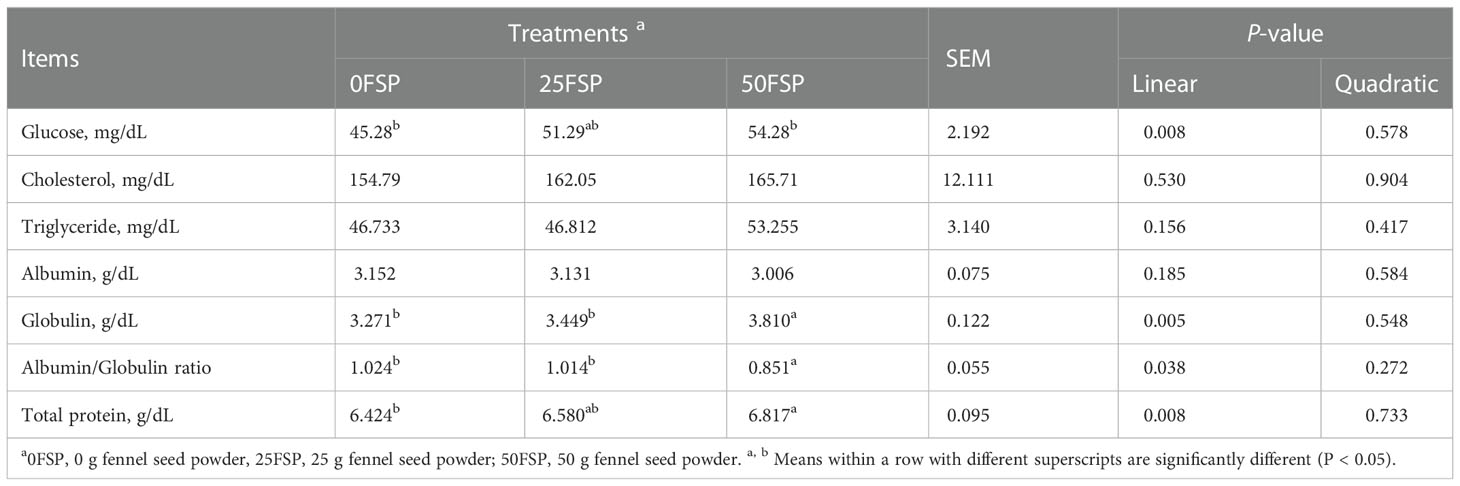
Table 4 Blood parameters of early lactating Holstein dairy cows (n = 8 per treatment) fed a basal diet supplemented with fennel seed powder (FSP).
As shown in Table 5, milk yield increased linearly as FSP inclusion in diets increased. Increasing FSP in diets linearly increased yields of milk fat (P = 0.017), protein (P = 0.028), lactose (P = 0.014), and TS (P = 0.008), whereas milk concentrations of fat, protein, lactose, and TS were not affected. Treatments had no effects on MUN concentration which averaged 12.4 mg/dL. There was a quadratic effect for FE in cows fed increasing amounts of FSP (P< 0.001), with cows fed 25FSP having lower FE compared with cows fed 50FSP (P< 0.001).
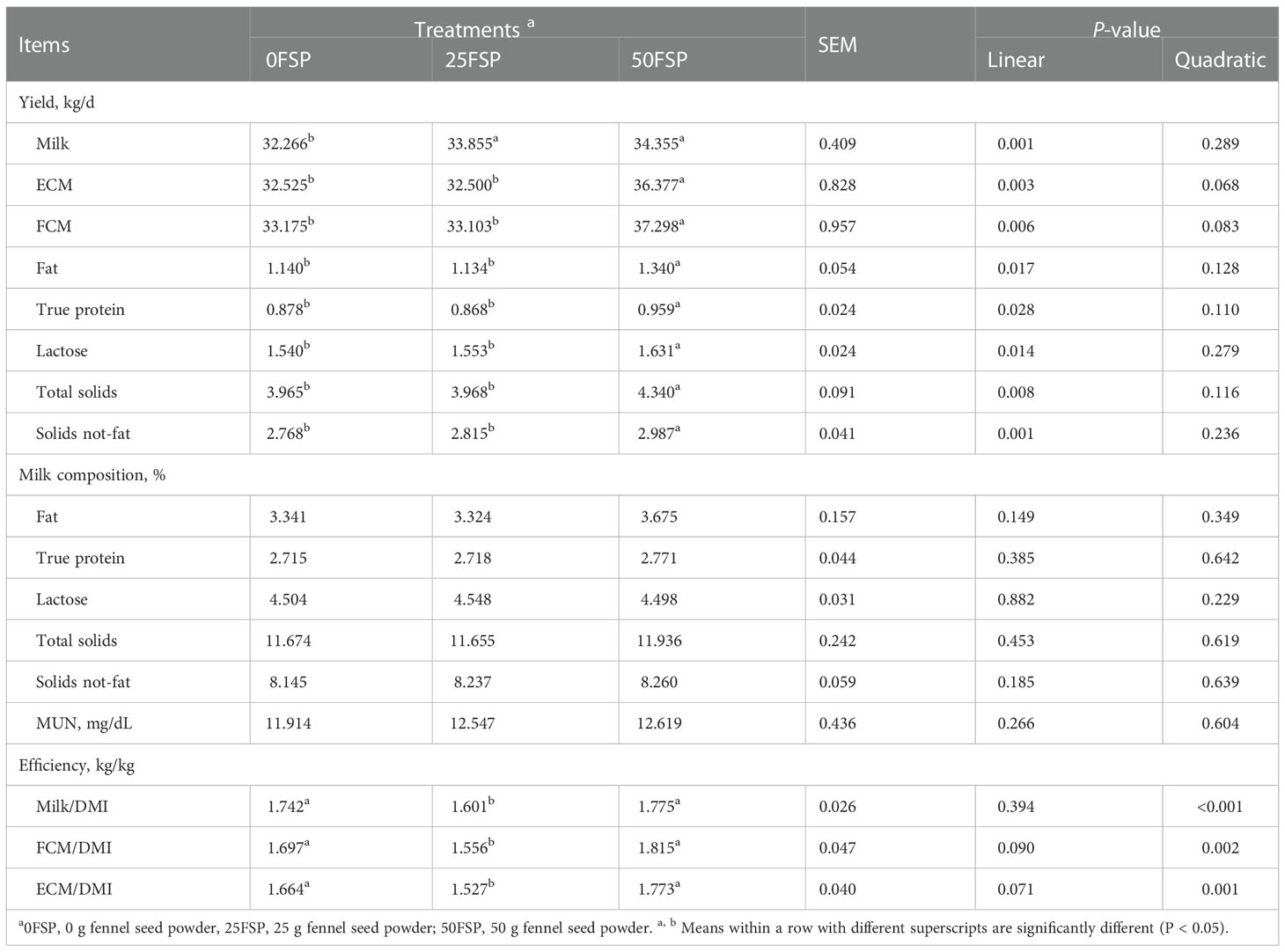
Table 5 Milk yield and composition of early lactating Holstein dairy cows (n = 8 per treatment) fed a basal diet supplemented with fennel seed powder (FSP).
Increasing FSP in diets linearly increased de novo (P< 0.001), mixed (P = 0.064), and decreased preformed (P = 0.002) milk FAs, whereas free FAs were not affected by FSP inclusion (Table 6). Milk saturated FAs were not affected by dietary treatments, whereas unsaturated FAs (P = 0.001), mono unsaturated FAs (P< 0.001), and poly unsaturated FAs (P = 0.032) linearly decreased with increasing FSP inclusion in diets. Moreover, content of C18:0 tended to decrease and C18:1 cis-9 decreased in milk as FSP inclusion increased linearly. Also, increasing FSP levels in diets decreased NEFA (P = 0.008) and acetone (P = 0.023) concentrations in milk linearly.
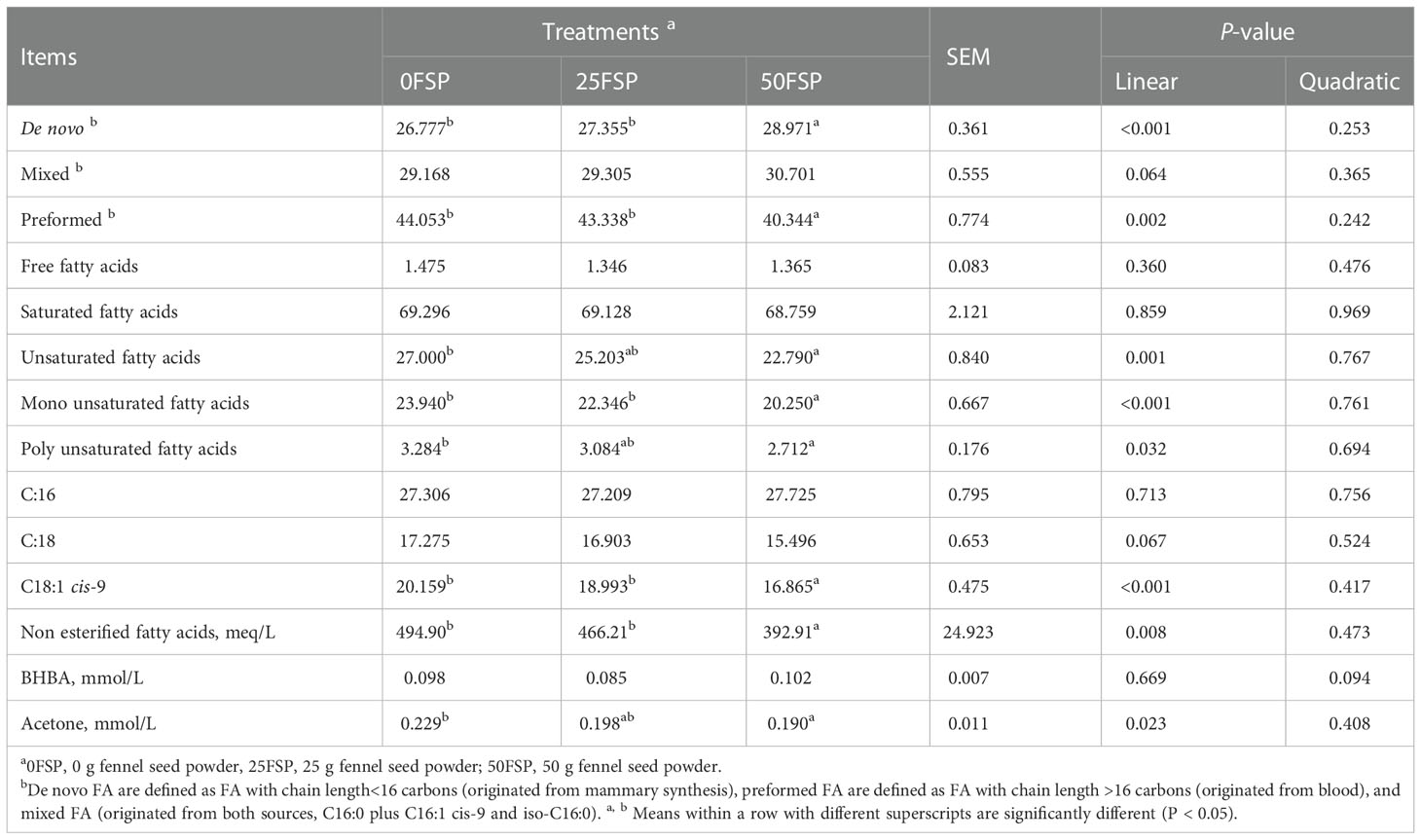
Table 6 Fatty acids profile (g/100 g total fatty acids) in milk of early lactating Holstein dairy cows (n = 8 per treatment) fed a basal diet supplemented with fennel seed powder (FSP).
Discussion
In contrast to our finding, FSP supplementation did not affect feed intake in early lactating Egyptian buffaloes (Fahim et al., 2021), whereas it increased DMI in dairy calves (Fahim et al., 2021; Kargar et al., 2021), lamb (Hajalizadeh et al., 2019), and Mohabadi dairy goats (Yari et al., 2018). Fennel seed powder contains anethole, limonene, α-pinene, fenchol, fenchone, and estragole (Hajalizadeh et al., 2019) and can stimulate the appetite due to both aroma and flavor agents (Kargar et al., 2021), resulting that diet being more palatable and therefore increasing DMI. It was reported that DMI was increased by a flavoring diet (Saeedi et al., 2017; Kargar et al., 2021). Moreover, FSP improved the digestibility of nutrients (Mahmoud et al., 2020; Fahim et al., 2021), and it could increase DMI due to lower rumen fill (Allen, 2014). It is difficult to explain inconsistent results on DMI. The nutrient composition of the experimental diets, kind and stage of animal, and FSP dosage across different studies were different. For example, early lactation dairy cows were used in the current experiment compared with mid-lactation dairy cows and early lactation buffaloes used by Mahmoud et al. (2020) and Fahim et al. (2021), respectively. Moreover, those experiments (Mahmoud et al., 2020; Fahim et al., 2021) used only one level of FSP in contrast to the present experiment, which used two levels of FSP. The quadratic response of DMI to increasing levels of FSP indicates that various levels of FSP had different effects on intake and performance of dairy cows, and it needs further research to find the best dosage of FSP.
Lower ruminal pH in cows fed FSP may be explained by lower acetate concentration and acetate:propionate ratio. By increasing the digestibility and rate of fermentation (Mahmoud et al., 2020; Fahim et al., 2021), fermentation byproducts production such as total and individual VFAs will increase, leading to a ruminal pH decrease (Church, 1988). In agreement with our finding, calves fed a diet with 0.8% FSP decreased ruminal pH compared to a control group (Saeedi et al., 2017). Moreover, Busquet et al. (2006) indicated that cows supplemented with plant extracts had lower ruminal pH than in control, which showed plant extracts’ impact on production of ruminal VFA. In contrast, Santos et al. (2015) in dairy calves and Yari et al. (2018) in Mohabadi dairy goats observed no significant effects on rumen pH by supplementing essential oils.
Feeding diets containing FSP did not affect ruminal NH3-N concentration. According to Santos et al. (2015) and Saeedi et al. (2017), ruminal NH3-N concentration increased when plant extracts were added to dairy calves. However, feeding FSP to Mohabadi dairy goats decreased rumen NH3-N concentration (Yari et al., 2018). Benchaar et al. (2006) showed that rumen NH3-N concentration and protein digestibility did not change by supplementing a mixture of essential oils (0.75 and 2 g/d) in dairy cows. Moreover, Castillejos et al. (2007) indicated that feeding a blend of different essential oils, including limonin and thymol, had no effect on rumen NH3-N concentration but increased total VFA production. They suggested that supplementing essential oils for a long time may cause ruminal micro-organisms adaptation and become resistant to the effects of ingredients in essential oils.
In agreement with our finding, FSP addition decreased acetate proportion and acetate:propionate ratio and increased propionate and total VFA production in Mohabadi dairy goats (Yari et al., 2018) and dairy calves (Saeedi et al., 2017). Furthermore, essential oils supplementation increased the relative abundance of propionate and valerate, whereas it decreased acetate levels in beef cattle (Zhang et al., 2021). Essential oils can interact with microbial cell membranes and inhibit the growth of some gram-positive and gram-negative bacteria. As a result of such inhibition, the addition of some plant extracts to the rumen results in an inhibition of deamination and methanogenesis, resulting in lower acetate and higher propionate concentrations (Calsamiglia et al., 2007). The lower acetate levels in the rumen denote less methane because methanogens could use acetate to produce methane (Cobellis et al., 2016), and the low acetate:propionate ratio indicates a high energy efficiency in feed (Liu et al., 2020). On the other hand, it has been reported that the effects of pure herbal essential oils on rumen fermentation related to ruminal pH (Cardozo et al., 2005), essential oil level (Benchaar et al., 2006), and basal diet (Calsamiglia et al., 2007). Finding the optimum composition and level will prepare an optimum situation for rumen fermentation (Calsamiglia et al., 2007).
Plasma protein represents the total amount of protein present in the blood. It also reflects the two major groups of proteins, i.e., albumin and globulin. The increase in TP is due to greater plasma globulin concentration in FSP-fed cows in the current experiment. Also, Mahmoud et al. (2020) reported that the increase in blood TP may be due to the increase in protein digestibility in FSP-fed animals reported by Mahmoud et al. (2020) and Fahim et al. (2021). The same result was observed by Galbat et al. (2014), that the serum TP concentration was increased by adding some medicinal plants to the ration of dairy goats.
Plasma globulin increment with phytogenic addition may be evidence of their merit to increase the immunity status of animals due to inhibiting the growth of pathogens or decreasing their toxins in the gastrointestinal ecosystem (Windisch et al., 2008). Also, Bhatt (2015) indicated that most phytogenics have anti-inflammatory, antibacterial, antiviral, anthelmintic, antioxidant, or coccidiostats properties, which may improve the immune system. Results are agreed with those reported by Mahmoud et al. (2020) and Lakhani et al. (2019), who showed enhanced plasma TP and globulin concentrations when FSP was fed to dairy cows and calves, respectively. In disagreement with our finding, Fahim et al. (2021) reported that FSP had no effect on plasma TP and globulin concentration of buffaloes. The linear increase of plasma globulin level with the addition of FSP caused a linear decrease in the ratio of albumin to globulin.
The increase in plasma glucose concentration probably is related to the increase in DM and OM digestibility reported by other studies (Mahmoud et al., 2020; Fahim et al., 2021). In contrast to our finding, plasma glucose levels did not change in buffalos (Fahim et al., 2021) or decreased in dairy calves (Lakhani et al., 2019) when the FSP was added to diets. These variations between experiments might be due to variations in experimental design, doses supplemented, diets, species, and physiological stage of experimental animals.
Similar to our findings, FSP supplementation increased milk and ECM yield in early lactating buffaloes (Fahim et al., 2021) and crossbred lactating cows (Mahmoud et al., 2020). Also, Attari et al. (2018) observed that FSP supplementation at 0.03% of body weight for 63 days in dairy cows increased milk yield without affecting milk composition. In the current experiment, feed efficiency increased in 50FSP treatment due to increased milk yield without affecting feed intake with supplementations of FSP, in agreement with Fahim et al. (2021).
Milk yield increase may result from improved total tract nutrient digestibility, as mentioned by Fahim et al. (2021) and Mahmoud et al. (2020). Moreover, Windisch et al. (2008) indicated that herbal additives have an improved effect on the productivity and health status of the animal by keeping the digestive tract stability through the prevention of the pathogens’ growth and decreasing animal’s exposure to toxins. Furthermore, it can suppose that milk yield improvement is a result of increasing rumen VFA concentration with FSP supplementation in the current experiment.
Milk fat content increased by FSP addition in other studies (Mahmoud et al., 2020; Fahim et al., 2021). Despite a linear decrease in rumen acetate production, FSP addition did not affect milk fat content in the present study. Differences in the results may be related to the species of animal, dose of supplements, basal diets, or physiological stage. Many factors affect milk fat content, such as genetics, stage of lactation, concentrate intake, amount and composition of dietary fat, protein and energy intake, and seasonal effects. Energy intake influences milk fat composition in several ways. In a negative energy balance, dietary supply of glucose decrease, causing lower synthesis of short-chain FAs by mammary tissue and increased mobilization of adipose tissue FAs. This short-chain FAs decrease probably leads to lower milk fat (Palmquist et al., 1993). In our experiment, FSP-fed cows had lower ruminal acetate proportion but higher de novo and mixed FAs in milk, and this shows that the increase in milk de novo and mixed FAs levels despite the decrease in ruminal acetate level could maintain the milk fat. The current data showed insignificant effect of FSP on milk composition. These results are consistent with those found by Mahmoud et al. (2020); Fahim et al. (2021), and Attari et al. (2018), who reported that FSP had no significant effect on milk composition.
In a meta-analysis study, Daning et al. (2021) reported that using essential oils at a specific dosage has significant effects on milk and FCM yield, and feed efficiency. However, it does not affect the milk composition such as protein, lactose, fat, and MUN.
Supplementation with FSP affected milk FAs profile. Mahmoud et al. (2020) observed increased omega-3, polyunsaturated FAs, and CLA, while decreased saturated FAs proportions in lactating cows received FSP at 0.7% of diet. Furthermore, FSP addition increased proportions cis-9, trans-11 C18:2, proportion trans-10, cis-12 C18:2, and total CLA; however, it decreased polyunsaturated FAs without affecting the concentration of C16:0 in early lactating buffalo (Fahim et al., 2021).
In the present study, milk FAs profile and ketone bodies were evaluated by the FTIR analysis. This method could be useful for evaluating the energy status of cows (McParland and Berry, 2016), especially in early lactating period (Daneshvar et al., 2021). Lower preformed and higher de novo and mixed FAs in milk of FSP-fed cows might be associated with less BW mobilization than 0FSP cows. Preformed FAs originated from diet and body fat mobilization. As a result, Palmquist et al. (1993) reported that high amounts of preformed FAs in mammary glands inhibit the production of de novo FAs. Furthermore, concentration of milk preformed FAs was higher than in mid-lactation dairy cows, as reported by Rico et al. (2014). These findings may be the consequence of greater body fat mobilization and NEB of early lactation cows included in the current experiment, as a similar scenario had been reported by Daneshvar et al. (2021). Also, estrogens are crucial hormonal regulators of systemic energy homeostasis via regulating adipose tissue metabolism. Estrogens seem to display beneficial actions on insulin and glucose metabolism through the suppression of adipose lipolysis resulting in decreased circulating free FAs levels and the modulation of expression and/or secretion of adipocytokines for the improvement of insulin sensitivity (Kim et al., 2014). Furthermore, Samadi-Noshahr et al. (2021) reported that FSP had hypolipidemic effects via its antioxidant properties and ability to stimulate insulin secretion. These results indicate that FSP may prevent body fat mobilization in fresh cows by increasing insulin sensitivity and reducing lipolysis.
Gross et al. (2011) demonstrated that long-chain FAs concentrations in milk, especially unsaturated FAs, C18:1 cis-9, and C18:0 could be used as indicators for evaluating energy status in dairy cows. Moreover, Churakov et al. (2021) indicated that milk FAs could be used as a tool to address NEB in early lactation cows, and concentrations of C18:0 and C18:1 cis-9 are the best variables for early detection of cows with severe NEB. Also, van Haelst et al. (2008) reported that the mobilization of adipose tissue happens sooner than the ketosis development in early lactation cows, and mobilized FAs are transferred into milk fat; hence, milk long-chain FAs profile change may be a useful tool for primary diagnosis of ketosis. In the current experiment, FSP addition decreased milk content of unsaturated FAs, C18:1 cis-9, and C18:0 compared with 0FSP diet, which is consistent with the lower milk acetone and NEFA. It is argued that FTIR predictions for acetone and NEFA are valuable for screening cows on subclinical ketosis, especially when used in combination with other indicators, and can serve in the evaluation of the herd health status with respect to subclinical ketosis (de Roos et al., 2007; Bach et al., 2019). In agreement with our findings, de Roos et al. (2007) and Bach et al. (2019) showed that cows with higher body fat mobilization had higher milk ketone bodies and lower de novo FAs, which are promising indicators of subsequent disease or removal in early lactation. Data from the current study indicated that adding FSP increased concentrations of de novo and mixed FAs, while concentrations of performed and unsaturated FAs decreased. These results, together, indicate that FSP-fed cows had a lower body fat mobilization, and FSP may improve metabolic statements in early lactation cows.
Supplementing 50 g FSP to early lactating Holstein dairy cows increased milk production and plasma glucose and globulin and reduced body fat mobilization indicated by lower milk long-chain FAs; therefore, FSP addition is proposed as an economically affordable strategy to improve performance and health outcomes.
Conclusions
Adding 50 g of FSP increased milk yield in early lactating Holstein cows without any negative effect on feed intake. Supplementations did not influence milk composition, including fat, protein, lactose, and TS. Moreover, FSP supplementation increased concentrations of de novo and mixed FAs and decreased preformed FAs in the milk. Also, unsaturated FAs linearly decreased with increasing FSP inclusion in the diets. Furthermore, increasing the FSP level in the diets decreased the NEFA and acetone concentrations linearly in milk. The ruminal pH value and acetate proportion decreased linearly as FSP increased in diets. Increasing FSP in the diets linearly increased plasma glucose, globulin, and TP concentrations, indicating the positive effect of FSP addition on animal health. The results of this study showed that addition of FSP could enhance the performance of early lactating cows. Also, the results of the milk FAs profile and ketone bodies explained that FSP has a positive effect on body fat mobilization and could decrease the risk of ketosis in early lactating cows. In agreement with our hypothesis, FSP feeding improved performance of early lactating Holstein cows without increasing body fat mobilization. Further investigations are essential to understand better how FSP impacts digestibility, blood parameters, and body condition score change in transition dairy cows (pre and postpartum).
Data availability statement
The raw data supporting the conclusions of this article will be made available by the authors, without undue reservation.
Ethics statement
All animal procedures were conducted with protocols approved by the Animal Care and Use Committee of the Iranian Council of Animal Care (1995).
Author contributionss
All authors have made a substantial direct and intellectual contribution to the work ranging from inception of the project idea, funding, project implementation and management, data collection and analysis, writing, and editing of the manuscript. All authors contributed to the article and approved the submitted version.
Conflict of interest
The authors declare that the research was conducted in the absence of any commercial or financial relationships that could be construed as a potential conflict of interest.
Publisher’s note
All claims expressed in this article are solely those of the authors and do not necessarily represent those of their affiliated organizations, or those of the publisher, the editors and the reviewers. Any product that may be evaluated in this article, or claim that may be made by its manufacturer, is not guaranteed or endorsed by the publisher.
References
Allen M. S. (2014). Drives and limits to feed intake in ruminants. Anim. Prod. Sci. 54, 1513–1524. doi: 10.1071/AN14478
Ansari M., Kargar S., Eslami M. A., Falahati R., Albenzio M., Caroprese M., et al. (2022). Potential benefits of early-life supplementation of liquid feed with fennel (Foeniculum vulgare) seeds or oregano (Origanum vulgare) leaves on growth, health, and blood metabolites in Holstein dairy calves. J. Dairy Sci. 105, 6639–6653. doi: 10.3168/jds.2022-21776
AOAC (2002). Official methods of analysis of AOAC international. 17th ed (Gaithersburg, MD: AOAC International).
Attari V., Basiri S. H., Taheri V. (2018). Effects of feeding fennel, black seed and black caraway plant on milk yield, growth hormone and prolactin in lactating Holstein cows. Anim. Sci. Rea. 28, 169–179.
Bach K. D., Barbano D. M., McArt J. A. A. (2019). Association of mid-infrared-predicted milk and blood constituents with early-lactation disease, removal, and production outcomes in Holstein cows. J. Dairy Sci. 102, 10129–10139. doi: 10.3168/jds.2019-16926
Badgujar S. B., Patel V. V., Bandivdekar A. H. (2014). Foeniculum vulgare mill: A review of its botany, phytochemistry, pharmacology, contemporary application, and toxicology. BioMed. Res. Int. 2014, 842674. doi: 10.1155/2014/842674
Bal M. A., Shaver R. D., Jirovec A. G., Shinners K. J., Coors J. G. (2000). Crop processing and chop length of corn silage: effects on intake, digestion, and milk production by dairy cows. J. Dairy Sci. 83, 1264–1273. doi: 10.3168/jds.S0022-0302(00)74993-9
Benchaar C., Petit H. V., Berthianume R., Whyte T. D., Chouinard P. Y. (2006). Effects of addition of essential oils and monensin premix on digestion, ruminal fermentation, milk production and milk composition in dairy cows. J. Dairy Sci. 89, 4352–4365. doi: 10.3168/jds.S0022-0302(06)72482-1
Bhatt N. (2015). Herbs and herbal supplements, a novel nutritional approach in animal nutrition. Iranian J. Appl. Anim. Sci. 5, 497–516.
Broderick G. A., Kang J. H. (1980). Automated simultaneous determination of ammonia and total amino acids in ruminal fluid and In vitro media. J. Dairy Sci. 63, 64–75. doi: 10.3168/jds.S0022-0302(80)82888-8
Busquet M., Calsamiglia S., Ferret A., Kamel C. (2006). Plant extracts effect in vitro rumen microbial fermentation. J. Dairy Sci. 89, 761–771. doi: 10.3168/jds.S0022-0302(06)72137-3
Calsamiglia S., Busquet M., Cardozo P. W., Castillejos L., Ferret A. (2007). Invited review: Essential oils as modifiers of rumen microbial fermentation. J. Dairy Sci. 90, 2580–2595. doi: 10.3168/jds.2006-644
Cardozo P. W., Calsamiglia S., Ferret A., Kamel C. (2005). Screening for the effects of natural plant extracts at different pH on in vitro rumen microbial fermentation of a high-concentrate diet for beef cattle. J. Anim. Sci. 83, 2572–2579. doi: 10.2527/2005.83112572x
Castillejos L., Calsamiglia S., Ferret A., Losa R. (2007). Effects of dose and adaptation time of a specific blend of essential oils compounds on rumen fermentation. Anim. Feed Sci. Technol. 132, 186–201. doi: 10.1016/j.anifeedsci.2006.03.023
Churakov M., Karlsson J., Edvardsson Rasmussen A., Holtenius K. (2021). Milk fatty acids as indicators of negative energy balance of dairy cows in early lactation. Animal 15, 100253. doi: 10.1016/j.animal.2021.100253
Church D. C. (1988). The ruminant animal: Digestive physiology and nutrition (Englewood Cliffs, NJ: Prentice-Hall).
Cobellis G., Trabalza-Marinucci M., Yu Z. (2016). Critical evaluation of essential oils as rumen modifiers in ruminant nutrition: a review. Sci. Total Environ. 545, 556–568. doi: 10.1016/j.scitotenv.2015.12.103
Dairy Records Management Systems (2014). DHI glossary (Raleigh, NC: Dairy Records Management Systems).
Daneshvar D., Ghasemi E., Hashemzadeh F., Kowsar R., Khorvash M. (2021). Feeding diets varying in starch concentration supplemented with palmitic acid or stearic acid: Effects on performance, milk fatty acid profile, and metabolic parameters of postpartum dairy cows. Anim. Feed Sci. Technol. 279, 115015. doi: 10.1016/j.anifeedsci.2021.115015
Daning D. R. A., Yusiati L. M., Hanim C., Widyobroto B. P. (2021). Meta-analysis of the effect of essential oil usage towards the production and milk composition of dairy cow. IOP Conf. Ser.: Earth Environ. Sci. 733, 012105. doi: 10.1088/1755-1315/733/1/012105
de Roos A. P. W., van den Bijgaart H. J. C. M., Hørlyk J., de Jong G. (2007). Screening for subclinical ketosis in dairy cattle by Fourier transform infrared spectrometry. J. Dairy Sci. 90,1761–1766. doi: 10.3168/jds.2006-203
Fahim N. H., Kholif A. E., Azzaz H. H. (2021). Fennel and ginger improved nutrient digestibility and milk yield and quality in early lactating Egyptian buffaloes. Ann. Anim. Sci. 22, 255–270. doi: 10.2478/aoas-2021-0008
Galbat S. A., El-Shemy A., Madpoli A. M., Maghraby O. M. A. L., El-Mossalami E. I. (2014). Effects of some medicinal plants mixture on milk performance and blood components of Egyptian dairy goats. Middle East J. Appl. Sci. 4, 942–948.
Geishauser T. (1993). An instrument for the collection and transfer of ruminal fluid and for the administration of water soluble drugs in adult cattle. Bovine Pract. 27, 38–42. doi: 10.21423/BOVINE-VOL1993NO27P27-42
Gross J., van Dorland H. A., Bruckmaier R. M., Schwarz F. J. (2011). Milk fatty acid profile related to energy balance in dairy cows. J. Dairy Res. 78, 479–488. doi: 10.1017/S0022029911000550
Hajalizadeh Z., Dayani O., Khezri A., Tahmasbi R., Mohammadabadi M. R. (2019). The effect of adding fennel (Foeniculum vulgare) seed powder to the diet of fattening lambs on performance, carcass characteristics and liver enzymes. Small Rumin. Res. 175, 72–77. doi: 10.1016/j.smallrumres.2019.04.011
Hashemzadeh-Cigari F., Ghorbani G. R., Khorvash M., Riasi A., Taghizadeh A., Zebeli Q. (2015). Supplementation of herbal plants differently modulated metabolic profile, insulin sensitivity, and oxidative stress in transition dairy cows fed various extruded oil seeds. Prev. Vet. Med. 118, 45–55. doi: 10.1016/j.prevetmed.2014.10.013
Hashemzadeh F., Rafeie F., Hadipour A., Rezadoust M. H. (2022). Supplementing a phytogenic-rich herbal mixture to heat-stressed lambs: Growth performance, carcass yield, and muscle and liver antioxidant status. Small Rumin. Res. 206, 106596. doi: 10.1016/j.smallrumres.2021.106596
Heidari M., Ghorbani G. R., Hashemzadeh F., Ghasemi E., Panahi A., Rafiee H. (2022). Feed intake, rumen fermentation and performance of dairy cows fed diets formulated at two starch concentrations with either conventional urea or slow-release urea. Anim. Feed Sci. Techno 290, 115366. doi: 10.1016/j.anifeedsci.2022.115366
Iranian Council of Animal Care (1995). Guide to the care and use of experimental animals (Isfahan, Iran: Isfahan University of Technology).
Kargar S., Nowroozinia F., Kanani M. (2021). Feeding fennel (Foeniculum vulgare) seed as potential appetite stimulant to newborn Holstein dairy calves: Effects on meal pattern, ingestive behavior, oro-sensorial preference, and feed sorting. Anim. Feed Sci. Technol. 278, 115009. doi: 10.1016/j.anifeedsci.2021.115009
Kim J. H., Cho H. T., Kim Y. J. (2014). The role of estrogen in adipose tissue metabolism: insights into glucose homeostasis regulation. Endocr. J. 61, 1055–1067. doi: 10.1507/endocrj.EJ14-0262
Kooti W., Moradi M., Ali-Akbari S., Sharafi-Ahvazi N., Asadi-Samani M., AshtaryLarky D. (2015). Therapeutic and pharmacological potential of foeniculum vulgare mill: A review. J. Herb Pharm. 4, 1–9.
Lakhani N., Kamra D. N., Lakhani P., Alhussien M. N. (2019). Immune status and haematobiochemical profile of buffalo calves supplemented with phytogenic feed additives rich in tannins, saponins and essential oils. Trop. Anim. Health Prod. 51, 565–573. doi: 10.1007/s11250-018-1727-z
Liu X., Sha Y., Dingkao R., Zhang W., Lv W., Wei H., et al. (2020). Interactions between rumen microbes, VFAs, and host genes regulate nutrient absorption and epithelial barrier function during cold season nutritional stress in Tibetan sheep. Front. Microbiol. 11, 593062. doi: 10.3389/fmicb.2020.593062
Mahmoud A. E. M., Rahmy H. A. F., Ghoneem W. M. A. (2020). Role of caraway, fennel and melissa addition on productive performance of lactating frisian cows. Pakistan J. Biol. Sci. 23, 1380–1389. doi: 10.3923/pjbs.2020.1380.1389
McParland S., Berry D. P. (2016). The potential of Fourier transform infrared spectroscopy of milk samples to predict energy intake and efficiency in dairy cows. J. Dairy Sci. 99, 4056–4070. doi: 10.3168/jds.2015-10051
Nowroozinia F., Kargar S., Akhlaghi A., Raouf Fard F., Bahadori-Moghaddam M., Kanani M., et al. (2022). Feeding fennel (Foeniculum vulgare) seed as a potential appetite stimulant for Holstein dairy calves: Effects on growth performance and health. J. Dairy Sci. 105, 654–664. doi: 10.3168/jds.2021-20221
NRC (2001). Nutrient requirements of dairy cattle. 7th ed (Washington, DC, USA: National Academy of Sciences).
Palmquist D. L., Beaulieu A. D., Barbano D. M. (1993). Feed and animal factors influencing milk fat composition. J. Dairy Sci. 76, 1753–1771. doi: 10.3168/jds.S0022-0302(93)77508-6
Rico J. E., Allen M. S., Lock A. L. (2014). Compared with stearic acid, palmitic acid increased the yield of milk fat and improved feed efficiency across production level of cows. J. Dairy Sci. 97, 1057–1066. doi: 10.3168/jds.2013-7432
Rochfort S., Parker A. J., Dunshea F. R. (2008). Plant bioactives for ruminant health and productivity. Phytochem 69, 299–322. doi: 10.1016/j.phytochem.2007.08.017
Saeedi S., Dayani O., Tahmasbi R., Khezri A. (2017). Effect of supplementation of calf starter with fennel powder on performance, weaning age and fermentation characteristics in Holstein dairy calves. J. Anim. Physiol. Anim. Nutr. (Berl) 101, 81–87. doi: 10.1111/jpn.12511
Samadi-Noshahr Z., Hadjzadeh M.-A., Moradi-Marjaneh R., Khajavi-Rad A. (2021). The hepatoprotective effects of fennel seeds extract and trans-anethole in streptozotocin-induced liver injury in rats. Food Sci. Nutr. 9, 1121–1131. doi: 10.1002/fsn3.2090
Santos F. H. H., De Paula M. R., Lezier D., Silva J. T., Santos G., Bittar C. M. M. (2015). Essential oils for dairy calves: effects on performance, scours, rumen fermentation and intestinal fauna. Animal 9, 958–965. doi: 10.1017/S175173111500018X
van Haelst Y. N. T., Beeckman A., van Knegsel A. T. M., Fievez V. (2008). Short communication: elevated concentrations of oleic acid and longchain fatty acids in milk fat of multiparous subclinical ketotic cows. J. Dairy Sci. 91, 4683–4686. doi: 10.3168/jds.2008-1375
Van Soest P. J., Robertson J. B., Lewis B. A. (1991). Methods for dietary fiber, neutral detergent fiber, and nonstarch polysaccharides in relation to animal nutrition. J. Dairy Sci. 74, 3583–3597. doi: 10.3168/jds.S0022-0302(91)78551-2
Windisch W., Schedle K., Plitzner C., Kroismayr A. (2008). Use of phytogenic products as feed additives for swine and poultry. J. Anim. Sci. 86, E140–E148. doi: 10.2527/jas.2007-0459
Yari M., Atalou H., Pirmohammadi R., Khalilvandi-Behroozyar H., Alijoo Y. (2018). Effects of fennel seed (foeniculum vulgare) powder in corn or barley grain based diets on milk production and composition, ruminal fermentation and some blood parameters in mohabadi dairy goats pre- and post-partum. Anim. Sci. Rea. 28, 141–158.
Zakernezhad F., Barati M., Sanadgol N., Movahhedi M., Majd A., Golab F. (2021). The association between fennel extract, serum lipid profile, and leptin receptor expression. BCN 12, 711–720. doi: 10.32598/bcn.2021.998.2
Keywords: essential oils, fennel seed, herbal additives, early lactation cow, negative energy balance
Citation: Moosavi-Zadeh E, Rahimi A, Rafiee H, Saberipour H and Bahadoran R (2023) Effects of fennel (Foeniculum vulgare) seed powder addition during early lactation on performance, milk fatty acid profile, and rumen fermentation parameters of Holstein cows. Front. Anim. Sci. 4:1097071. doi: 10.3389/fanim.2023.1097071
Received: 13 November 2022; Accepted: 03 January 2023;
Published: 17 January 2023.
Edited by:
Rodolpho Martin Do Prado, Federal University of Rio Grande do Sul, BrazilReviewed by:
Hossam H. Azzaz, National Research Centre, EgyptCaren Paludo Ghedini, Federal University of Rio Grande do Sul, Brazil
Ana Schogor, Santa Catarina State University, Brazil
Copyright © 2023 Moosavi-Zadeh, Rahimi, Rafiee, Saberipour and Bahadoran. This is an open-access article distributed under the terms of the Creative Commons Attribution License (CC BY). The use, distribution or reproduction in other forums is permitted, provided the original author(s) and the copyright owner(s) are credited and that the original publication in this journal is cited, in accordance with accepted academic practice. No use, distribution or reproduction is permitted which does not comply with these terms.
*Correspondence: Hassan Rafiee, harafiee@yahoo.com