- Norwich Medical School, University of East Anglia, Norwich, Norfolk, United Kingdom
Iron deficiency is a common public health problem in the UK. This review examines the role of meat in iron nutrition, focusing on the most vulnerable groups of the UK population. Meat contains haem iron which is absorbed by a different pathway to non-haem iron found in cereals and vegetables. A summary of absorption data from studies using isotopically-labelled haem iron shows that, although there is a wide degree of variation, haem iron bioavailability is consistently higher than non-haem iron. The importance of meat alternatives, such a plant protein, insects, and biofortified crops as a supply of bioavailable iron, and the use of food iron fortification is reviewed. Finally, the consequences of excluding meat from the diet in relation to dietary iron requirements is discussed.
Introduction
Iron deficiency is a long-standing public health problem in the UK, especially in teenage girls, women of child-bearing age, and elderly men and women (Public Health England, 2020). Low iron stores are quite common, especially in teenage girls, and a smaller number have iron deficiency anaemia caused by an imbalance between dietary iron supply and physiological requirements. Approximately one fifth of the UK daily intake of iron in adults is provided from meat and meat products. Meat is also considered to be an important source of iron because it contains haem iron which is more bioavailable than non-haem iron from plants, and it enhances non-haem iron absorption from foods consumed at the same meal. On the other hand, meat is reported to have adverse effects on human health, although the impact of different types and quantities of meat is somewhat controversial (Johnston et al., 2019). In relation to iron nutrition, the consequences of avoiding meat have been examined by measuring the iron status of vegetarians and vegans, but confounding is a well-known weakness of cross-sectional data. Randomised controlled trials provide evidence of causality, but interventions have to be long enough in duration to enact a change in iron status, therefore data of this type are very limited. The drive to consume less meat, for environmental, health, economic and other reasons, has led to a growing number of meat replacement products entering the market. These include protein sources derived from soy and other plants, as well as traditional foods that are novel to the UK, such as insects. Globally, a number of iron-biofortified staple crops have been developed, including beans, cereals and potatoes, and these are being introduced into agricultural practice. However, the problem of low iron bioavailability in high phytate/polyphenol foods remains a practical constraint, and dietary advice to improve iron bioavailability is needed to ensure that iron deficiency does not become widespread in children and women who consume meat-free diets.
Iron deficiency in the UK
Population groups that are at risk of iron deficiency include infants (over 6 months of age), toddlers and young children, adolescents, women of child-bearing age, and pregnant women. The reasons for their vulnerability are the high iron requirements for growth and, in the case of women, losses associated with menstruation and/or the demands of pregnancy. According to the latest figures available from the National Diet and Nutrition Survey (Public Health England, 2020) low haemoglobin concentrations, indicative of anemia, are present in 9% of girls aged 11-18 years, 7% of women aged 18-64 years, and 17% of men and women over 65 years (Table 1). A higher percentage of girls and women have low iron stores (low plasma ferritin), 24% in girls aged 11-18 years, and 15% in women aged 18-64 years, but only 2% of the elderly have a low plasma ferritin concentration. There are two possible explanations for the apparent discordance in the elderly: either iron deficiency is not the cause of anaemia, or ferritin (an acute phase protein) may be raised due to chronic inflammation (as often found in older people) and therefore does not accurately reflect iron stores.
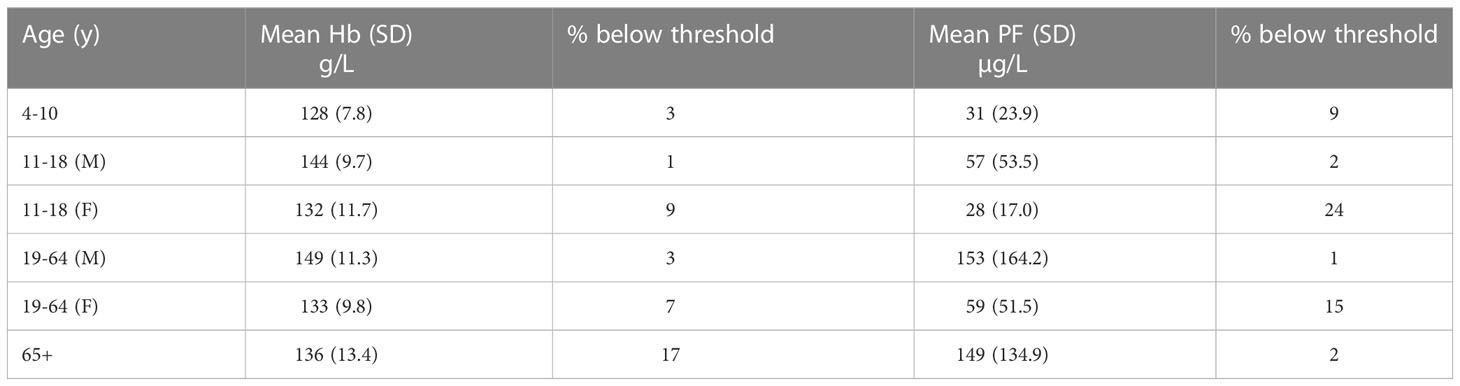
Table 1 Haemoglobin (Hb) and plasma ferritin (PF) concentrations in different age groups (NDNS years 7-8, 2014/15-2015/16) .
Contribution of meat to the iron intake of vulnerable groups of the UK population
The NDNS shows that between 2008 and 2019 there was a small reduction in iron intakes in all age groups except men aged 75 years and over. The greatest reduction was in girls aged 4-10 years (-1.4 mg/d), boys aged 11-18 years (-1.1 mg/d), men aged 65-74 years (-1.1 mg/d), and women aged 75 years and over (-1.5 mg/d).
The sources of dietary iron by food group are shown in Figure 1 for adults aged 19-65 years. Cereals made the greatest contribution to total iron intake (37-53%, depending on age group), with high fibre breakfast cereals, some of which are fortified with iron, contributing 8-15%, followed by meat and meat products (14-19%, depending on age group). The food group entitled ‘beef, veal and dishes’ contribute one quarter, and ‘chicken, turkey and dishes’ contribute another quarter of the iron intake from meat in most age groups. Burgers, kebabs and sausages were consumed in higher amounts in children and teenagers, whereas older people tended to consume more meat pies and pastries. Median consumption of red and processed meat was significantly lower in 2016-2019 than 2014-2016 for men aged 19-64 years and adults aged 65 years and over. Since 2008, there has been a reduction in mean meat consumption in all age groups of 13 g/day, 23 g/day and 19 g/day for ages 11-18 years, 19-64 years and 65 years and over, respectively. The latest data shows that mean consumption in all age or sex groups met the UK recommendation of no more than 70g per day (Scientific Advisory Committee on Nutrition, 2010).
Iron content of meat
It is generally assumed that 40% of the total iron in meat is in the form of haem iron (Monsen et al., 1978; Monsen and Balintfy, 1982), but the haem iron content of meat is, in fact, very variable, depending on the origin of the meat, and the method of cooking (Gandemer et al., 2020). Pretorius et al. (2016) reported values for the iron content of pooled samples of raw homogenized muscle from carcasses of 4 types of meat. The total haem iron content (mg/100 g fresh weight) for lamb, beef, pork and chicken was 1.32, 1.21, 0.71 and 0.58 mg/100g respectively, with haem iron (%) being reported to be 81, 77, 88 and 74% of total iron respectively. Lombardi-Boccia et al. (2002) analyzed the haem and non-haem iron content of a range of different meats before and after cooking (Table 2). The haem iron content was highest in beef, followed by lamb, pork and chicken. When chicken and turkey were cooked in the oven at 180°C for 50 min, there was a substantial loss of haem iron, ranging from 22-43%. In contrast, when cooked in a pan, the losses were lower, ranging from 1-24%. The decrease in haem iron is the result of the oxidative cleavage of the porphyrin ring which releases iron from the haem complex, which may only occur at relatively high temperatures i.e. above 85°C (Han et al., 1993). Pourkhalili et al. (2013) examined the effect of different cooking methods on the haem iron content of lamb meat. The lean raw meat was boiled at 97°C for 90 min, pan fried in oil for 20 min (internal temperature 85°C), or grilled above a flame for 10 min (internal temperature not higher than 86°C). The greatest losses occurred with boiling, and lowest losses were with grilling. An important finding from this study was that the four analytical methods, modified from Hornsey (1956), used to measure haem iron gave different results, and the authors concluded that a haem extraction solution comprised of 90% acetone, 18% water and 2% hydrochloric acid was the most appropriate because it correlated better with results for haem iron content calculated from the difference between total iron and non-haem iron.
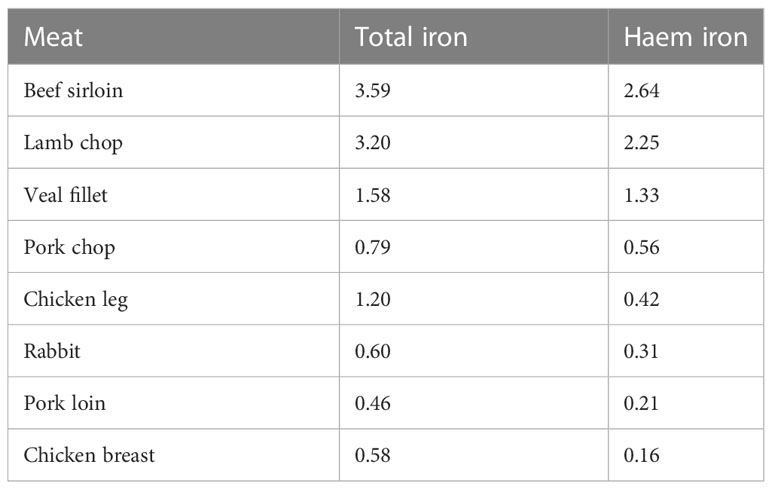
Table 2 Haem and non-haem iron content (mg/100g) of different types of cooked meat (Lombardi-Boccia et al 2002).
Gel filtration can be used to examine the distribution of iron compounds in raw meat. Clement et al. (1972) examined different types of raw meat and found that beef steak had a higher iron concentration (30 µg/g) than chicken (4.7 µg/g in breast compared with 9.0 µg/g in leg meat, which contains more myoglobin). Apart from haemoglobin and myoglobin, the other main component is ferritin (beef steak, chicken breast and chicken leg contain 3.6, 2.4 and 1.9 µg/g respectively). In veal muscle, 15-20% of the total iron was reported to be present as ferritin (Layrisse, 1973). In addition to haem iron in haemoglobin and myoglobin, ferritin, haemosiderin, and a small quantity of low molecular weight iron compounds, are present in meat (Latunde-Dada and Neale, 1986). The % of total iron present as haem in beef, lamb, pork and chicken were reported to be 77, 68, 55 and 42%, respectively. In pigeon, haem iron was 79% and 45% of total iron in breast meat and liver respectively, ferritin was 6% and 26% of total iron in breast meat and liver respectively, and haemosiderin was 14% and 25% of total iron in breast meat and liver respectively. As with haem iron, cooking changes the bioavailability of other forms of iron in meat, presumably through changes in both the solubility and composition of the different iron compounds (Latunde-Dada and Neale, 1986), therefore data obtained from raw meat is of limited use when predicting the potential contribution of different types of meat to iron nutrition.
It is important to take into account haem iron intake when assessing the impact of diet in relation to risk of iron deficiency because of its higher bioavailability (see following section). However, this is a challenging task due to the lack of analytical data on the haem iron content of foods and the discrepancy in quoted values. The haem iron values proposed by Monsen et al. (1978) and Monsen (1988) were 30-40% of iron in pork and liver, and 50-60% in beef, lamb and chicken. Schricker et al. (1982) quote 49%, 57% and 62% in pork, lamb and beef respectively. In addition, the haem iron content can vary between muscles in the same animal and according to the cooking method used (Schricker et al., 1982). In view of the lack of data on haem iron in meat, Cross et al. (2012) developed a haem iron database based on meat type, cooking method and doneness level. Meats with the highest level of haem iron, regardless of cooking method, were hamburgers (mean 10.3 µg/g) and steak (mean 9.3 µg/g). Pork had values in the range of 3.4-7.5 µg/g. Chicken had the lowest haem values but varied according to the cut of meat (mean for chicken breast 2.4 µg/g). The method used to measure total iron was inductively coupled plasma-atomic emission spectrometry (ICP-AES). Haem iron was extracted with acidified acetone (Hornsey, 1956) and analyzed by flame atomic absorption spectrometry. The remaining solid material was subjected to a total acid digestion and analyzed by ICP-AES to provide the non-haem iron. Interestingly, there was no clear effect of cooking method on haem iron, as has been reported previously. However, the extraction solution for haem iron (acetone:H2O:HCl) was 80:10:10, which differed from the 90:18:2 composition recommended by Pourkhalili et al. (2013), and the absence of certified reference materials introduces some uncertainty in the data.
Iron absorption from meat
Dietary iron is predominantly inorganic (non-haem) iron which has a well-established mechanism of absorption; ferric iron in the digestate is reduced to ferrous iron, transported across the apical membrane of the intestinal enterocyte by divalent metal-ion transporter 1 (DMT1), and either stored as ferritin in the cell or exported to the circulation via ferroportin 1 (FPN1) where it binds to plasma transferrin (Anderson and Frazer, 2017). Meat contains haem iron, either as haemoglobin in red blood cells or myoglobin in muscle cells, comprised of one ferrous atom bound to four globin molecules. Meat also contains variable amounts of ferritin and haemosiderin, both of which are less well absorbed than haem iron (Martínez-Torres et al, 1976). As is the case with non-haem iron, the efficiency of absorption of iron from ferritin is inversely related to iron status; mean absorption of radio-isotopically ferritin iron was 0.9% in normal subjects, 2.5% in subjects with moderate iron deficiency, and 5.7% in subjects with marked iron deficiency (Layrisse et al., 1975).
Haem iron absorption
The mechanism of absorption of haem iron has not yet been fully characterized (Fairweather-Tait and Sharp, 2021). It is believed to bind to the enterocyte brush border intact and then endocytosed, although the molecular details of the process are unknown, and the possibility of a haem transporter cannot be discounted. Within the enterocyte the iron is released from haem through the action of heme oxygenase and is either stored as ferritin or exported from the cells via FPN1 i.e. by the same pathway as non-haem iron (Anderson and Frazer, 2017). There are a limited number of studies investigating haem iron absorption, the majority of which were carried out several decades ago. The main limitation is that the iron has to be isotopically labelled with radio- or stable isotopes of iron in order to undertake metabolic studies.
Although the findings from different studies cannot be directly compared, an overview of the data generated from the studies undertaken using radio-isotopically labelled haem iron is given in Table 3. There is an inverse relationship between dose of haem iron and percentage absorption. Pizarro et al. (2003) reported that the absorption of radio-isotopically labelled haem iron by healthy women was both dose-related and saturable over the range tested (0.5-100 mg). At levels of haem iron typically found in a portion of meat (approximately 1 mg), mean absorption ranges from 20.7-37.3%, but at higher intakes (e.g. 5-10 mg iron) the efficiency of absorption is greatly reduced i.e. 11-16%.
As with non-haem iron, the iron status of individuals (often categorized according to serum ferritin concentration) affects iron absorption. Haem iron absorption from a burger and chips meal containing 1.2 mg radio-isotopically labelled haem iron was inversely correlated to serum ferritin concentration, with mean values of 22-25% in 57 healthy men and women (Roughead and Hunt, 2000). Haem iron absorption is upregulated when iron requirements are raised, as occurs in iron deficiency, although to a much lesser extent than non-haem iron (Cook, 1990). A summary is provided by Cook (1990) for a large set of data obtained from studies measuring haem and non-haem iron absorption from a hamburger, french fries and a milk shake meal (containing 1.4 mg haem iron and 3.4 mg non-haem iron), the two forms of iron being labelled with different radioisotopes of iron. Iron replete men absorbed 2.5% of non-haem iron compared with 26% of haem iron. However, when iron stores were depleted, absorption increased nearly 10-fold (increased to 22%) for non-haem iron, whereas haem iron absorption only doubled (increased to 47%), indicating that the adaptive response for non-haem iron is much greater than for haem iron.
As discussed earlier, cooking changes the forms of iron in meat, and the method used impacts on iron bioavailability. For example, absorption of haem iron from radio-isotopically labelled beef was 23.4 (SEM 1.0)% but when it was fried, the value fell to 19.4 (SEM 1.2)%, and when it was overcooked absorption fell to 14.5 (SEM 1.2)% (Martínez-Torres et al., 1986).
Haem iron absorption is around 50% lower when the globin chains are removed during digestion (Turnbull et al., 1962). One possible explanation is that the released hemin polymerises into large aggregates that are poorly soluble at physiological pH (Gaitán et al., 2011). Haem iron is better absorbed with food than on its own (Table 3) which may be because peptides released from food bind the liberated haem to form monomeric haem products that are readily absorbed by the mucosal cells (Conrad et al., 1966).
When deriving Dietary Reference Values (DRVs) for iron, EFSA reviewed studies on haem iron absorption in whole diets and cited the study by (Hallberg et al, 1997) in which radio-isotopically labelled rabbit haemoglobin was used to label four high bioavailability meals per day (total iron intake 13 mg/day) for five days. The mean absorption of haem iron was 35% in 12 male blood donors and 23% in 19 non-blood donors, with an overall absorption of 28.6% (Table 3). EFSA used this and other evidence to reach a conclusion that the absorption of haem iron from a Western-style diet is approximately 25% (EFSA NDAPanel, 2015).
Effect of meat on non-haem iron absorption
A number of dietary components influence the bioavailability of non-haem iron, either positively or negatively. The most common mechanism is chemical binding between the constituent and iron released from digested food, which either prevents iron from being taken up by the iron transporter protein in the duodenal mucosal cells (reducing bioavailability), or prevents iron from forming insoluble hydroxides with the increase in pH in the duodenum (increasing bioavailability). A recently conducted literature search listed inhibitors of absorption as phytic acid/phytates, polyphenols/tannins, proteins from soya beans, milk, eggs and calcium, and enhancers as ascorbic acid, lactic acid, alcohol (which stimulates iron uptake by inhibition of hepcidin expression), the presence of haem iron, and the so-called ‘meat factor’ in flesh from mammals, birds and fish, (Milman, 2020).
The enhancing effect of meat on non-haem iron absorption has been known for many years and this attribute has been used, for example, as a strategy to optimize iron absorption in children (Etcheverry et al., 2006). Cook and Monsen (1976) found that substituting egg ovalbumin in a semi-synthetic meal with beef, lamb, pork, chicken, liver and fish caused a two to four-fold increase in iron absorption by human volunteers, whereas no increase was observed with milk, cheese, or egg. Hurrell et al. (2006) reported that freeze-dried beef and chicken muscle increased iron absorption by 180% and 100%, respectively, relative to egg albumin, and suggested that the enhancing effect of muscle tissue on iron absorption was mainly protein related. A recent scoping review that systematically mapped studies on the meat factor (Consalez et al., 2022) concluded from data extracted from 77 eligible studies that the addition of muscle tissue and muscle tissue fractions to single plant-based meals steadily increased absorption of iron. There were no clear differences between red meat, poultry and fish in promoting the meat factor effect, and no evidence that milk and egg products contain the meat factor.
Attempts to fully characterise the meat factor (or factors) have been unsuccessful to date. In addition to the well-known gastric acid-promoting effect of meat (McArthur et al., 1988), proposed candidates include amino acids (Martínez-Torres and Layrisse, 1970), peptides (Taylor et al., 1986; Hurrell et al., 2006), and protein hydrolysates (Li et al., 2017). Evidence from in vitro Caco-2 cell studies suggest that glycosaminoglycans (GAG) may enhance non-haem iron bioavailability (Huh et al., 2004). However, an in vivo stable isotope study investigating the effect of GAG (3-10 times the quantity present in 150 g beef muscle) from fish and chicken muscle reported that it had no effect on iron absorption from a semi-synthetic meal of egg albumin, corn oil, maltodextrin and water. Using an initial in vitro screening approach, Armah et al. (2008) separated fractions from cooked beef after it had undergone simulated gastric and intestinal digestion and tested their effect on iron uptake into Caco-2 cells using an 55Fe label. Mass spectrometric techniques were used to identify the active fractions, and L-alpha-glycerophosphocholine (L-alpha) was found to be the compound present in the most active fraction. The effect of L-alpha on iron absorption in vivo was determined in a human stable isotope study measuring non-heme iron absorption from 2 meals of vegetarian lasagne, each containing 10 mg iron. Absorption was significantly increased from 3.4% to 5.6% by the addition of 100mg ascorbic acid to the meal, and from 3.5% to 4.9% by the addition of 46 mg L-alpha to the meal. However, in a different study, the enhancing effect of L-alpha was not observed when 70 mg L-alpha was added to maize porridge, although ascorbic acid did increase iron absorption (Troesch et al., 2009). Although phospholipids might have an enhancing effect on non-heme iron absorption, it is clear that there is no single component of meat that can be identified as the meat factor, and that the enhancing effect of meat on non-haem iron absorption is probably a combination of factors.
The potential contribution of meat alternatives and fortified/biofortified plant foods towards iron nutrition
Alternatives to meat, such as the soy protein Tofu, have been consumed as traditional foods for a long time, and more recently, other meat alternatives, including textured vegetable protein and mycoprotein (Quorn) have entered the market (Thavamani et al., 2020). The acknowledgement that diets containing high intakes of meat have a negative impact on the environment and adverse consequences for human health (Godfray et al., 2018) has stimulated the search for alternative sources of protein to replace meat. Many plant-based meat alternatives have been developed in recent years, including cultured meat, plant protein concentrates, and insect products. Iron is one of the key nutrients in meat and although some plant-based meat analogues are reported to have a higher content of iron than meat (Bryngelsson et al., 2022) others may not (Ismail et al., 2020), and, in fact, the bioavailability of iron is the overriding factor when assessing the quality of a meat alternative in relation to iron nutrition.
Phytate, widely found in plant foods but not meat, dramatically reduces iron absorption; many high iron plant foods, such as pulses, wholegrains and some green leafy vegetables (kale, spinach) contain high levels of phytate (Milman, 2020). An example of a novel plant protein that contains high levels of both iron and phytate is duckweed (Wolffia globosa), a traditional food consumed in Asian countries. A human intervention study in healthy men and women, in which diets containing duckweed were consumed for 6 months, reported no detrimental effect on iron status (Yaskolka Meir et al., 2019). Duckweed is currently under consideration for approval as a novel food by the Advisory Committee on Novel Foods and Processes (https://acnfp.food.gov.uk/ACNFPMeeting_June2021).
Like many plant foods, edible insects have a lower ecological footprint than meat, and are an alternative to meat as they contain high levels of protein and iron. Iron occurs predominantly in non-haem forms, bound to ferritin and transferrin and other transport and storage proteins (Mwangi et al., 2018). In a stable isotope absorption study undertaken in iron-depleted women, Mwangi et al. (2022) found that iron absorption from intrinsically labelled house crickets (Acheta domesticus) added to maize porridge was low (~3%) which they suggested might be due to the presence of chitin and other inhibitors in the cricket biomass. A study of similar design examined iron absorption from mealworm larvae (Tenebrio molitor) and found it was similar to maize porridge (~5%); processing of the T. molitor biomass to reduce the chitin content did not increase iron bioavailability (Hilaj et al., 2022). The authors conclude that T. molitor iron is absorbed from the common nonheme iron pool and could therefore be a useful source of dietary iron.
One of the strategies for addressing the long-standing global problem of iron deficiency has been food iron fortification, but there have been several technical challenges, notably the low bioavailability of iron compounds that can be added to foods without causing adverse organoleptic changes (Hurrell, 2022). Micronutrient deficiencies and the move towards lower meat consumption have led to the development of breeding programmes for biofortified staple food crops. The goal is to generate food crops with a higher iron content, although bioavailability is another important consideration (La Frano et al., 2014). It has been shown, for example, that the phytic acid content of plant foods such as biofortified beans has a strongly negative impact on iron absorption (Petry et al., 2014). Absorption from biofortified purple potatoes was lower than from biofortified yellow potatoes, presumably because of the presence of polyphenols which inhibit iron absorption. Conversely, the absorption of iron from biofortified sweet potatoes, which do not contain phytate or polyphenols, was high (Jongstra et al., 2020).
Vegetarian and vegan diets
Iron is listed as one of the nutrients of concern in a plant-based diet (Craig et al., 2021). A systematic review of the intake and adequacy of vegan diets was recently published (Bakaloudi et al., 2021) and, in general, the intake of iron in vegan diets is no lower than other categories of diet, presumably because of the high iron content of widely consumed vegan foods such as green-leafy vegetables, grains, nuts and beans. Neufingerl and Eilander (2021) undertook a systematic review and analysed data from 38 studies that reported on iron intake (30 assessed intake from foods only). The mean iron intake was higher in vegans (21.0 mg/d) compared to vegetarians (15.3 mg/d) and meat eaters (13.9 mg/d), independent of supplement intake. Fabricius et al. (2021) used a risk-benefit assessment approach to estimate the health impact of substituting unprocessed red meat by pulses in the Danish diet, including the impact of substitution on adequacy of iron intake. There was a small decrease in the proportion of individuals below the average requirement in men and post-menopausal women (men: 32–30%), women>51: 35–33%), but no change for menstruating women (women<52: 75%); no predictions were made about changes in iron status, which would, of course, be an extremely challenging task because of the overriding effect of bioavailability. A modelling study on the unintended consequences of switching from animal- to plant-based foods, based on data from NHANES 2018-2018, predicted that dietary requirements for iron are met with flexitarian, vegetarian and vegan diets comprised of either traditional or novel plant-based foods (Tso and Forde, 2021).
The systematic review by Neufingerl and Eilander (2021) examined data on iron stores (serum/plasma ferritin concentration) in vegans, vegetarian and omnivores, from 17 studies (10 of which excluded supplement users). Iron status was higher in meat-eaters (55.5 µg/L) than in vegetarians (33.8 µg/L) and vegans (31.3 µg/L) and was lowest in vegetarian women (24.3 µg/L). However, mean haemoglobin values were similar and adequate across the three dietary patterns (i.e., 139/136/140 g/L for meat-eater, vegans and vegetarians, respectively). Another meta-analysis of 24 cross-sectional studies found that vegetarians are more likely to have lower iron stores than non-vegetarians, the results being more pronounced in men than premenopausal women (Haider et al., 2018). However, caution must be used when interpreting data from cross-sectional studies because associations are not necessarily causal. Potential confounders include the observation that people who eat more vegetables tend to have healthier diets overall, and could have other healthier lifestyle factors, such as having a higher socioeconomic and educational status, smoking less, being more physically active, and having a lower BMI. In addition, the length of time for iron stores to become depleted varies between individuals, depending on the size of body iron stores at the onset of the dietary change, their physiological requirements and capacity to absorb iron, which may be related to genotype.
There are very few randomized controlled trials on the effects of eliminating meat from the diet on iron status, and most of them were too short to demonstrate an effect on ferritin or haemoglobin. Wells et al. (2003) carried out a 12-week dietary intervention in older men undertaking resistive training. Total iron intake was similar between the group consuming a vegetarian or a beef-containing diet but at the end of the 12 weeks, although serum ferritin had decreased in both groups, the meat-consuming group had an increased haemoglobin and haematocrit compared with the vegetarian group. Another RCT investigating the effect of meat on iron status was a study in New Zealand toddlers, aged 12-20 months at baseline (Szymlek-Gay et al., 2009). The children were given red meat, iron-fortified milk or cow’s milk for 20 weeks. Serum ferritin and body iron were significantly higher in the fortified milk group and serum ferritin was significantly higher in the red meat group than the cow’s milk group. The authors concluded that an increased intake of red meat prevents the decline in iron status observed in toddlers not given additional iron.
Food fortification and biofortification
Iron fortification of foods has been undertaken for a very long time, with differing degrees of success. The first major challenge is the development of technical solutions to allow highly bioavailable forms of iron to be added to foods without adverse organoleptic changes; the second is the physiological response to infection and inflammation which causes a rise in hepcidin and instigates a barrier to iron absorption (Hurrell, 2022).
A more sustainable solution to the widespread problem of iron deficiency is to increase the nutrient density of foods by conventional plant or animal breeding, agronomic management, or genetic engineering. This is known as biofortification. Iron is amongst the most common micronutrients in plant crop biofortification programmes. Whilst there are successful examples of meat biofortified with both vitamin D (Neill et al., 2021) and selenium (D’Amato et al., 2020), there have been no attempts to increase its already high iron content. Therefore, the focus is on producing biofortified plant food crops.
Plant breeding programmes have managed to double the iron content of common beans, but the high phytic acid content of beans reduces iron bioavailability and impedes the effectiveness of bean iron biofortification, highlighting the importance of reducing the phytic acid content of food crops (Petry et al., 2015). Potatoes are low in phytic acid and this may be one of the reasons why the iron bioavailability of iron in yellow fleshed potato (containing 0.33 mg iron/100g fresh weight) was found to be remarkably high; the geometric mean absorption from a meal was 28.4% (95% CI 23.5%, 34.2%). The geometric mean absorption of iron from biofortified purple flesh potato (containing 0.69 mg iron/100g fresh weight) was much lower, namely 13.3% (95% CI 10.6%, 16.6%), probably due to the presence of polyphenols (Jongstra et al., 2020). The higher iron content of the biofortified potato meant that the total iron absorbed was similar between the two species of potato, a good illustration of the advantage conferred by biofortification.
Dietary requirements
In 1998, the World Health Organization and the Food and Agriculture Organization proposed dietary iron bioavailability values for setting DRVs of 15, 10 or 5%, depending on the composition of the diet (Food Agriculture Organization of the United Nations, & World Health Organization, 1988). The highest bioavailability value is for diversified diets with generous amounts of meat and/or foods rich in ascorbic acid. The lowest bioavailability is for diets based on cereals, tubers and legumes with little or no meat or ascorbic acid-containing fruits and vegetables.
Craig et al. (2021) published guidelines for health professionals in relation to plant-based diets in which they stated that vegetarians, and especially vegans, should consume a well-balanced diet, regularly use fortified foods and/or supplements, and pay special attention to calcium, iron, vitamin D, and vitamin B12. When the US Dietary Reference Intakes (DRIs) were derived, approximately 20 years ago, there were separate recommendations for iron intake by vegetarians (Institute of Medicine, 2003). The DRIs for iron are derived factorially whereby physiological requirements, estimated from the sum of iron losses plus iron needed for growth, are converted into dietary intakes with an assumed dietary iron absorption of 18%. However, for plant-based diets they assume that absorption is lower, at around 10%, so they estimate that dietary iron requirements for vegetarians are 1.8 times higher than omnivores. The evidence to support this includes (1) a radioisotope study by Hunt and Roughead (1999) in which non-haem absorption was measured over 2 days and where there was a 6-fold difference in absorption from a lacto-ovo-vegetarian diet compared with a nonvegetarian diet, and (2) a longer-term trial which found a two-fold difference in iron absorption between low bioavailability Western-style diets (mainly vegetarian) and high bioavailability diets (containing meat) consumed for a period of 2 weeks (Cook et al., 1991).
In 2015, EFSA published updated Dietary Reference Values for iron (EFSA NDA Panel, 2015) in which they concluded that the bioavailability of iron from European vegetarian diets is not substantially different from diets containing meat and other flesh foods. The evidence used to reach this conclusion included a randomized controlled trial in 21 women, aged 20-42 years, in which the effect of consuming a lacto-ovo-vegetarian or omnivorous diet for eight weeks on serum ferritin concentrations was determined. There was no difference in iron status between the groups fed a Western-style vegetarian diet or a diet containing meat, indicating that the iron bioavailability of the two diets was similar (Hunt and Roughead, 1999). One possible weakness of this study is the short time duration, which may not have been long enough to elicit a change in serum ferritin. The UK DRVs which were published in 1991 (Department of Health, 1991) used the FAO/WHO value of 15% bioavailability (Food Agriculture Organization of the United Nations, & World Health Organization, 1988) to convert iron requirements to dietary reference values. However, they cautioned that iron in diets containing little or no meat is less well absorbed and that people habitually consuming such diets may need a higher iron intake, although they noted that “data do not exist to quantify this precisely”.
It is possible that the current, and anticipated, reduction in meat consumption may have an impact on iron status. Dietary patterns are constantly evolving in response to food security, changes in lifestyle, and the types and availability of different foods. Meat has a long-established role in the UK diet in relation to its contribution towards well-absorbed dietary iron. It is possible that results of older surveys evaluating the adequacy of dietary iron intake to meet physiological requirements may no longer be pertinent if dietary iron bioavailability falls. Careful monitoring of the iron status of vulnerable groups (particularly teenage girls and women of child-bearing age) is warranted to confirm that they have the capacity to upregulate the efficiency of iron absorption from diets of lower bioavailability.
Author contributions
The author confirms being the sole contributor of this work and has approved it for publication.
Funding
The author is funded by the University of East Anglia.
Conflict of interest
The author declares that the research was conducted in the absence of any commercial or financial relationships that could be construed as a potential conflict of interest.
Publisher’s note
All claims expressed in this article are solely those of the author and do not necessarily represent those of their affiliated organizations, or those of the publisher, the editors and the reviewers. Any product that may be evaluated in this article, or claim that may be made by its manufacturer, is not guaranteed or endorsed by the publisher.
References
Anderson G. J., Frazer D. M. (2017). Current understanding of iron homeostasis. Am. J. Clin. Nutr. 106 (Suppl 6), 1559S–1566S. doi: 10.3945/ajcn.117.155804
Armah C. N., Sharp P., Mellon F. A., Pariagh S., Lund E. K., Dainty J. R., et al. (2008). L-alpha-glycerophosphocholine contributes to meat’s enhancement of nonheme iron absorption. J. Nutr. 138, 873–877. doi: 10.1093/jn/138.5.873
Bakaloudi D. R., Halloran A., Rippin H. L., Oikonomidou A. C., Dardavesis T. I., Williams J., et al. (2021). Intake and adequacy of the vegan diet. A systematic Rev. evidence. Clin. Nutr. 40, 3503–3521. doi: 10.1016/j.clnu.2020.11.035
Bezwoda W. R., Bothwell T. H., Charlton R. W., Torrance J. D., MacPhail A. P., Derman D. P., et al. (1983). The relative dietary importance of haem and non-haem iron. S. Afr. Med. J. 64, 552–556.
Björn-Rasmussen E., Hallberg L., Isaksson B., Arvidsson B. (1974). Food iron absorption in man. applications of the two-pool extrinsic tag method to measure heme and nonheme iron absorption from the whole diet. J. Clin. Invest. 53, 247–255. doi: 10.1172/JCI107545
Bryngelsson S., Moshtaghian H., Bianchi M., Hallström E. (2022). Nutritional assessment of plant-based meat analogues on the Swedish market. Int. J. Food Sci. Nutr. 73, 889–901. doi: 10.1080/09637486.2022.2078286
Clement N., Torrance J. D., Bothwell T. H., Charlton R. W. (1972). Iron compounds in muscle. S. Afr. J. Med. Sci. 37, 7–14.
Conrad M. E., Cortell S., Williams H. L., Foy A. L. (1966). Polymerization and intraluminal factors in the absorption of hemoglobin-iron. J. Lab. Clin. Med. 68, 659–668.
Consalez F., Ahern M., Andersen P., Kjellevold M. (2022). The effect of the meat factor in animal-source foods on micronutrient absorption: a scoping review. Adv. Nutr. 2, nmac089. doi: 10.1093/advances/nmac089
Cook J. D. (1990). Adaptation in iron metabolism. Am. J. Clin. Nutr. . 51, 301–308. doi: 10.1093/ajcn/51.2.301
Cook J. D., Dassenko S. A., Lynch S. R. (1991). Assessment of the role of nonheme-iron availability in iron balance. Am. J. Clin. Nutr. 54, 717–722. doi: 10.1093/ajcn/54.4.717
Cook J. D., Monsen E. R. (1976). Food iron absorption in human subjects. III. comparison of the effect of animal proteins on nonheme iron absorption. Am. J. Clin. Nutr. 29, 859–867. doi: 10.1093/ajcn/29.8.859
Craig W. J., Mangels A. R., Fresán U., Marsh K., Miles F. L., Saunders A. V., et al. (2021). The safe and effective use of plant-based diets with guidelines for health professionals. Nutrients. 13, 4144. doi: 10.3390/nu13114144
Cross A. J., Harnly J. M., Ferrucci L. M., Risch A., Mayne S. T., Sinha R. (2012). Developing a heme iron database for meats according to meat type, cooking method and doneness level. Food Nutr. Sci. 3, 905–913. doi: 10.4236/fns.2012.37120
D’Amato R., Regni L., Falcinelli B., Mattioli S., Benincasa P., Dal Bosco A., et al. (2020). Current knowledge on selenium biofortification to improve the nutraceutical profile of food: a comprehensive review. J. Agric. Food Chem. 68 (14), 4075–4097. doi: 10.1021/acs.jafc.0c00172
Department of Health (1991). “Dietary reference values for food energy and nutrients for the united kingdom,” in Report on health and social subjects no 41 (London: HMSO).
EFSA NDA, Panel (2015). Scientific opinion on dietary reference values for iron. EFSA J. 13:4254, 115 pp. doi: 10.2903/j.efsa.2015.4254
Etcheverry P., Hawthorne K. M., Liang L. K., Abrams S. A., Griffin I. J. (2006). Effect of beef and soy proteins on the absorption of non-heme iron and inorganic zinc in children. J. Am. Coll. Nutr. 25, 34–40. doi: 10.1080/07315724.2006.10719512
Fabricius F. A., Thomsen S. T., Fagt S., Nauta M. (2021). The health impact of substituting unprocessed red meat by pulses in the Danish diet. Eur. J. Nutr. 60, 3107–3118. doi: 10.1007/s00394-021-02495-2
Fairweather-Tait S., Sharp P. (2021). Iron. Adv. Food Nutr. Res. 96, 219–250. doi: 10.1016/bs.afnr.2021.01.002
Food Agriculture Organization of the United Nations, & World Health Organization (1988). Requirements of vitamin a, iron, folate and vitamin B12: report of a joint FAO/WHO expert consultation (Rome: Food and Agriculture Organization of the United Nations).
Gaitán D., Flor S., Saavedra P., Miranda C., Olivares M., Arredondo M., et al. (2011). Calcium does not inhibit the absorption of 5 milligrams of nonheme or heme iron at doses less than 800 milligrams in nonpregnant women. J. Nutr. 141, 1652–1656. doi: 10.3945/jn.111.138651
Gaitán D., Olivares M., Lönnerdal B., Brito A., Pizarro F. (2012). ). non-heme iron as ferrous sulfate does not interact with heme iron absorption in humans. Biol. Trace Elem. Res. 150, 68–73. doi: 10.1007/s12011-012-9496-4
Gandemer G., Scislowski V., Portanguen S., Kondjoyan A. (2020). The impact of cooking of beef on the supply of heme and non-heme iron for humans. Food Nutr. Sc. 11, 629–648. doi: 10.4236/fns.2020.117045
Godfray H. C. J., Aveyard P., Garnett T., Hall J. W., Key T. J., Lorimer J., et al. (2018). Meat consumption, health, and the environment. Science 361 (6399), eaam5324. doi: 10.1126/science.aam5324
Haider L. M., Schwingshackl L., Hoffmann G., Ekmekcioglu C. (2018). The effect of vegetarian diets on iron status in adults: a systematic review and meta-analysis. Crit. Rev. Food Sci. Nutr. 58, 1359–1374. doi: 10.1080/10408398.2016.1259210
Hallberg L., Hultén L., Gramatkovski E. (1997). Iron absorption from the whole diet in men: how effective is the regulation of iron absorption? Am. J. Clin. Nutr. 66, 347–356. doi: 10.1093/ajcn/66.2.347
Hallberg L., Rossander-Hulthèn L., Brune M., Gleerup A. (1993). Inhibition of haem-iron absorption in man by calcium. Br. J. Nutr. 69, 533–540. doi: 10.1079/BJN19930053
Han D. K. W., McMillan K. W., Godber J. S., Bidner T. D., Younathan M. T., Marshall D. L., et al. (1993). Iron distribution in heated beef and chicken muscles. J. Fd. Sc. 58, 697–700. doi: 10.1111/j.1365-2621.1993.tb09337.x
Hilaj N., Zimmermann M. B., Galetti V., Zeder C., Murad Lima R., Hammer L., et al. (2022). The effect of dechitinization on iron absorption from mealworm larvae (Tenebrio molitor) flour added to maize meals: stable-isotope studies in young females with low iron stores. Am. J. Clin. Nutr. 116, 1135–1145. doi: 10.1093/ajcn/nqac210
Hornsey I. I. C. (1956). The colour of cooked cured pork. estimation of the nitric oxide-haem pigments. J. Fd. Agric. Sc. 7, 534–540. doi: 10.1002/jsfa.2740070804
Huh E. C., Hotchkiss A., Brouillette J., Glahn R. P. (2004). Carbohydrate fractions from cooked fish promote iron uptake by caco-2 cells. J. Nutr. 134, 1681–1689. doi: 10.1093/jn/134.7.1681
Hunt J. R., Roughead Z. K. (1999). Nonheme-iron absorption, fecal ferritin excretion, and blood indexes of iron status in women consuming controlled lactoovovegetarian diets for 8 wk. Am. J. Clin. Nutr. 69, 944–952. doi: 10.1093/ajcn/69.5.944
Hurrell R. F. (2022). Ensuring the efficacious iron fortification of foods: a tale of two barriers. Nutrients. 14, 1609. doi: 10.3390/nu14081609
Hurrell R. F., Reddy M. B., Juillerat M., Cook J. D. (2006). Meat protein fractions enhance nonheme iron absorption in humans. J. Nutr. 136, 2808–2812. doi: 10.1093/jn/136.11.2808
Institute of Medicine (2003). Dietary reference intakes for vitamin a, vitamin K, arsenic, boron, chromium, copper, iodine, iron, manganese, molybdenum, nickel, silicon, vanadium, and zinc (Washington, DC: National Academies Press).
Ismail I., Hwang Y. H., Joo S. T. (2020). Meat analog as future food: a review. J. Anim. Sci. Technol. 62, 111–120. doi: 10.5187/jast.2020.62.2.111
Johnston B. C., Zeraatkar D., Han M. A., Vernooij R. W. M., Valli C., Dib R. E., et al. (2019). Unprocessed red meat and processed meat consumption: dietary guideline recommendations. Ann. Internal Med 171, 756–764. doi: 10.7326/M19-1621
Jongstra R., Mwangi M. N., Burgos G., Zeder C., Low J. W., Mzembe G., et al. (2020). Iron absorption from iron-biofortified sweetpotato is higher than regular sweetpotato in Malawian women while iron absorption from regular and iron-biofortified potatoes is high in Peruvian women. J. Nutr. 150, 3094–3102. doi: 10.1093/jn/nxaa267
La Frano M. R., de Moura F. F., Boy E., Lönnerdal B., Burri B. J. (2014). Bioavailability of iron, zinc, and provitamin a carotenoids in biofortified staple crops. Nutr. Rev. 72, 289–307. doi: 10.1111/nure.12108
Latunde-Dada G. O., Neale R. J. (1986). Pigeon (Columba l.) meat iron solubility and availability for absorption in rats. Br. J. Nutr. 55, 409–418. doi: 10.1079/bjn19860047
Layrisse M., Martínez-Torres C., Cook J. D., Walker R., Finch C. A. (1973). Iron fortification of food: its measurement by the extrinsic tag method. Blood. 41, 333–352. doi: 10.1182/blood.V41.3.333.333
Layrisse M., Martínez-Torres C., Renzy M., Leets I. (1975). Ferritin iron absorption in man. Blood 45, 689–698. doi: 10.1182/blood.V45.5.689.689
Li Y., Jiang H., Huang G. (2017). Protein hydrolysates as promoters of non-haem iron absorption. Nutrients 9, 609. doi: 10.3390/nu9060609
Lombardi-Boccia G., Domingues B. M., Aguzzi A. (2002). Total heme and non-heme iron in raw and cooked meats. J. Food Sc. 67, 1–4. doi: 10.1111/j.1365-2621.2002.tb08715.x
Lynch S. R., Skikne B. S., Cook J. D. (1989). Food iron absorption in idiopathic hemochromatosis. Blood. 74, 2187–2193. doi: 10.1182/blood.V74.6.2187.bloodjournal7462187
Martínez-Torres C., Layrisse M. (1970). Effect of amino acids on iron absorption from a staple vegetable food. Blood 35, 669–682. doi: 10.1182/blood.V35.5.669.669
Martínez-Torres C., Leets I., Taylor P., Ramírez J., del Valle Camacho M., Layrisse M. (1986). Heme, ferritin and vegetable iron absorption in humans from meals denatured of heme iron during the cooking of beef. J. Nutr. 116, 1720–1725. doi: 10.1093/jn/116.9.1720
Martínez-Torres C., Renzi M., Layrisse M. (1976). Iron absorption by humans from hemosiderin and ferritin, further studies. J. Nutr. 106, 128–135. doi: 10.1093/jn/106.1.128
McArthur K. E., Walsh J. H., Richardson C. T. (1988). Soy protein meals stimulate less gastric acid secretion and gastrin release than beef meals. Gastroenterology 95, 920–926. doi: 10.1016/0016-5085(88)90164-3
Milman N. T. (2020). A review of nutrients and compounds, which promote or inhibit intestinal iron absorption: making a platform for dietary measures that can reduce iron uptake in patients with genetic haemochromatosis. J. Nutr. Metab. Sep 14, 7373498. doi: 10.1155/2020/7373498
Monsen E. R. (1988). Iron nutrition and absorption: dietary factors which impact iron bioavailability. J. Am. Diet. Assoc. 88, 786–790. doi: 10.1016/S0002-8223(21)07902-5
Monsen E. R., Balintfy J. L. (1982). Calculating dietary iron bioavailability: refinement and computerization. J. Am. Diet. Assoc. 80, 307–311. doi: 10.1016/S0002-8223(21)08469-8
Monsen E. R., Hallberg L., Layrisse M., Hegsted D. M., Cook J. D., Mertz W., et al. (1978). Estimation of available dietary iron. Am. J. Clin. Nutr. 31, 134–141. doi: 10.1093/ajcn/31.1.134
Mwangi M. N., Oonincx D. G. A. B., Hummel M., Utami D. A., Gunawan L., Veenenbos M., et al. (2022). Absorption of iron from edible house crickets: a randomized crossover stable-isotope study in humans. Am. J. Clin. Nutr. 116, 1146–1156. doi: 10.1093/ajcn/nqac223
Mwangi M. N., Oonincx D. G. A. B., Stouten T., Veenenbos M., Melse-Boonstra A., Dicke M., et al. (2018). Insects as sources of iron and zinc in human nutrition. Nutr. Res. Rev. 31, 248–255. doi: 10.1017/S0954422418000094
Neill H. R., Gill C. I. R., McDonald E. J., McRoberts W. C., Pourshahidi L. K. (2021). Vitamin d biofortification of pork may offer a food-based strategy to increase vitamin d intakes in the UK population. Front. Nutr. 8. doi: 10.3389/fnut.2021.777364
Neufingerl N., Eilander A. (2021). Nutrient intake and status in adults consuming plant-based diets compared to meat-eaters: a systematic review. Nutrients. 14, 29. doi: 10.3390/nu14010029
Petry N., Boy E., Wirth J. P., Hurrell R. F. (2015). Review: the potential of the common bean (Phaseolus vulgaris) as a vehicle for iron biofortification. Nutrients. 7 (2), 1144–1173. doi: 10.3390/nu7021144
Petry N., Egli I., Gahutu J. B., Tugirimana P. L., Boy E., Hurrell R. (2014). Phytic acid concentration influences iron bioavailability from biofortified beans in Rwandese women with low iron status. J. Nutr. 144, 1681–1687 doi: 10.3945/jn.114.192989
Pizarro F., Olivares M., Hertrampf E., Mazariegos D. I., Arredondo M. (2003). Heme-iron absorption is saturable by heme-iron dose women. J. Nutr. 133, 2214–2217. doi: 10.1093/jn/133.7.2214
Pourkhalili A., Mirlohi M., Rahimi E. (2013). Heme iron content in lamb meat is differentially altered upon boiling, grilling, or frying as assessed by four distinct analytical methods. Sc. Wld. J., 374030. doi: 10.1155/2013/374030
Pretorius B., Schönfeldt H. C., Hall N. (2016). Total and haem iron content in lean meat cuts and the contribution to the diet. Fd. Chem. 193, 97–101. doi: 10.1016/j.foodchem.2015.02.109
Public Health England (2020). Available at: https://www.gov.uk/government/statistics/ndns-results-from-years-9-to-11-2016-to-2017-and-2018-to-2019.
Roughead Z. K., Hunt J. R. (2000). Adaptation in iron absorption: iron supplementation reduces nonheme-iron but not heme-iron absorption from food. Am. J. Clin. Nutr. 72, 982–989. doi: 10.1093/ajcn/72.4.982
Roughead Z. K., Zito C. A., Hunt J. R. (2005). Inhibitory effects of dietary calcium on the initial uptake and subsequent retention of heme and nonheme iron in humans: comparisons using an intestinal lavage method. Am. J. Clin. Nutr. 82, 589–597. doi: 10.1093/ajcn.82.3.589
Schricker B. R., Miller D. D., Stouffer J. R. (1982). Measurement and content of nonheme and total iron in muscle. J. Fd. Sc. 47, 740–743. doi: 10.1111/j.1365-2621.1982.tb12704.x
Szymlek-Gay E. A., Ferguson E. L., Heath A. L., Gray A. R., Gibson R. S. (2009). Food-based strategies improve iron status in toddlers: a randomized controlled trial12. Am. J. Clin. Nutr. 90, 1541–1551. doi: 10.3945/ajcn.2009.27588
Taylor P. G., Martínez-Torres C., Romano E., Layrisse M. (1986). The effect of cysteine-containing peptides released during meat digestion on iron absorption in humans. Am. J. Clin. Nutr. 43, 68–71. doi: 10.1093/ajcn/43.1.68
Thavamani A., Sferra T. J., Sankararaman S. (2020). Meet the meat alternatives: the value of alternative protein sources. Curr. Nutr. Rep. 9, 346–355. doi: 10.1007/s13668-020-00341-1
Troesch B., Egli I., Zeder C., Hurrell R. F., de Pe S., Zimmermann M. B. (2009). Optimization of a phytase-containing micronutrient powder with low amounts of highly bioavailable iron for in-home fortification of complementary foods. Am. J. Clin. Nutr. 89, 539–544. doi: 10.3945/ajcn.2008.27026
Tso R., Forde C. G. (2021). Unintended consequences: nutritional impact and potential pitfalls of switching from animal- to plant-based foods. Nutrients. 13, 2527. doi: 10.3390/nu13082527
Turnbull A., Cleton F., Finch C. A. (1962). Iron absorption. IV. the absorption of hemoglobin iron. J. Clin. Inv. 41 Nutr. Rev. 47, 51–53. doi: 10.1111/j.1753-4887.1989.tb02786.x
Wells A. M., Haub M. D., Fluckey J., Williams D. K., Chernoff R., Campbell W. W. (2003). Comparisons of vegetarian and beef-containing diets on hematological indexes and iron stores during a period of resistive training in older men. J. Am. Diet. Assoc. 103, 594–601. doi: 10.1053/jada.2003.50112
Yaskolka Meir A., Tsaban G., Zelicha H., Rinott E., Kapla A., Youngster I., et al. (2019). A green-Mediterranean diet, supplemented with mankai duckweed, preserves iron-homeostasis in humans and is efficient in reversal of anemia in rats. J. Nutr. 149, 1004–1011. doi: 10.1093/jn/nxy321
Keywords: iron deficiency, iron intake from meat, iron bioavailability, meat alternatives, haem iron
Citation: Fairweather-Tait S (2023) The role of meat in iron nutrition of vulnerable groups of the UK population. Front. Anim. Sci. 4:1142252. doi: 10.3389/fanim.2023.1142252
Received: 11 January 2023; Accepted: 04 April 2023;
Published: 19 April 2023.
Edited by:
Ian Givens, University of Reading, United KingdomCopyright © 2023 Fairweather-Tait. This is an open-access article distributed under the terms of the Creative Commons Attribution License (CC BY). The use, distribution or reproduction in other forums is permitted, provided the original author(s) and the copyright owner(s) are credited and that the original publication in this journal is cited, in accordance with accepted academic practice. No use, distribution or reproduction is permitted which does not comply with these terms.
*Correspondence: Susan Fairweather-Tait, cy5mYWlyd2VhdGhlci10YWl0QHVlYS5hYy51aw==