- Department of Animal Science, Iowa State University, Ames, IA, United States
Zinc is a trace mineral of interest for optimizing growth in feedlot cattle due to its roles in many physiological functions, including growth. Twenty-four Angus-cross steers (467 ± 13 kg) were used to assess the effects of supplemental Zn and ractopamine hydrochloride (RAC) on trace mineral concentrations and muscle gene expression. Four GrowSafe-equipped pens were randomly assigned to treatments (1 pen of six steers/treatment): 0 (CON), 60 (LOW), 120 (MED) or 180 (HI) mg supplemental Zn/kg DM (Availa-Zn, Zinpro). Dietary Zn treatments were initiated on d 0 and RAC supplementation (300 mg·steer·-1·d-1; Actogain45, Zoetis) began on d 53. Blood, liver and muscle (longissimus thoracis) samples were collected from all steers on d -4, 48, and 67. The LOW treatment was removed from gene expression analyses due to < 3 steers being represented for 14 of 22 genes. Data were analyzed using ProcMixed of SAS with the fixed effect of treatment and steer as the experimental unit; orthogonal linear and quadratic contrast statements were used to compare treatments. On d 48 and 67, there were linear and quadratic trends for plasma Zn to be greater in Zn-supplemented steers than CON (P ≤ 0.10). On d 48, there was a tendency for a quadratic decrease on the expression of SLC30A4 (P ≤ 0.07) but no other differences due to treatment. On d 67, several genes involved in Zn transport and storage (MTA1, SLC39A7, SLC39A8, SLC39A9, SLC39A10, SLC39A13) were decreased (P ≤ 0.08), suggesting increased growth influences intracellular Zn trafficking and demands.
Introduction
Zinc plays a key role in many physiological functions including growth. Increasing Zn supplementation to feedlot cattle may support growth of cattle receiving growth promoting technologies such as anabolic implants or beta agonists (Genther-Schroeder et al., 2016a; Genther-Schroeder et al., 2016b). In the beta-agonist pathway, cyclic adenosine monophosphate is a secondary cellular signal and is broken down by phosphodiesterase, resulting in decreased effectiveness of the beta-agonist pathway, but this negative feedback can be decreased by Zn through the inhibition of PDE (von Bülow et al., 2005).
Zinc movement is highly dynamic within the cell, with transport facilitated by ZIP (into the cytosol) and ZnT (out of cytosol) transporters. The effects of Zn supplementation to cattle fed the beta agonist, ractopamine hydrochloride, on the expression of Zn transporters and storage mechanisms in muscle has not been studied. The objective of this study was to characterize how Zn machinery expression in muscle changes with increasing Zn supplementation before and during the ractopamine feeding period. It was hypothesized that genes involved in Zn trafficking in the muscle will be upregulated during the ractopamine period and Zn supplementation will affect this response.
Materials and methods
All methods were approved by the Iowa State University Institutional Animal Care and Use Committee (IACUC # 8-15-8073-B). Twenty-four Angus crossbred steers from a single ranch were identified by Genemax Gain (Zoetis, Parsippany, NJ) scores of 3, 4, and 5 (i.e., having more genetic potential for gain) and selected for this 84-day study. Steers were acclimated to GrowSafe bunks (GrowSafe Systems Ltd., Airdrie, AB, Canada) for 24 days and were transitioned to a common finishing diet 21 days prior to trial initiation. On d 0, steers were stratified by BW into pens of 6, and pens randomly assigned to treatment (1 pen/treatment), and implanted with a component TE-IS with Tylan (80 mg trenbolone acetate, 16 mg estradiol USP, 29 mg tylosin tartrate; Elanco Animal Health, Greenfield, IN). All steers received ractopamine hydrochloride (RAC) at 300 mg· steer·-1·d-1 (Actogain, Zoetis) starting on d 53. Dietary treatments included a control (CON) with no supplemental Zn and increasing Zn supplementation at 60 mg/kg DM (LOW), 120 mg/kg DM (MED), or 180 mg/kg DM (HI). The supplemental Zn source was a Zn amino acid complex (AvailaZn, donated by Zinpro Corporation, Eden Prairie, MN). Dietary treatments were delivered as part of the total mixed ration (TMR), delivered once daily via wagon mixer. On a dry matter basis TMR included 62% cracked corn, 25% modified distiller grains, 8% hay, 3% dried distiller grains used as a carrier for treatments, RAC and other micronutrients. Trace minerals and vitamins were added at NASEM (2016) recommended concentrations, except Zn. Weekly TMR samples were collected for DM determination, and dried samples were composited and subsequently analyzed for nutrient analysis at a commercial lab (Dairyland INC, Arcadia, WI; methods 990.03 and 920.39; AOAC, 1996). The control diet analyzed at 14.8% crude protein, 15.7% neutral detergent fiber, 5% ether extract and 35 mg Zn/kg DM.
Steers were weighed on d -1, 0, 28, 52, 53, 69, 80 and 81. Blood, muscle, and liver biopsies were collected on d -4, 48, and 67. Blood was collected in K2EDTA coated blood tubes (Becton, Dickinson and Company), stored on ice until centrifugation at 1,200 × g for 20 minutes at 4°C, aliquoted, and stored at -20°C until analysis. Muscle biopsies (<0.5g) were collected from the longissimus thoracis using methods described by Pampusch et al. (2008). Liver biopsies (approximately 1g) were completed using methods described by Engle and Spears (2000). Liver and muscle biopsies were flash frozen and stored at -80°C until analysis. Liver, TMR and plasma trace mineral concentrations were determined using inductively coupled plasma optical emission spectroscopy (ICP OES; Optima 7000; PerkinElmer, Waltham, MA) using methods described in Pogge and Hansen (2013). RNA was isolated from longissimus thoracis and cDNA synthesized as described in Rients et al. (2023). Quantitative real-time PCR was performed using Fluidigm Biomark HD system as described in Reichhardt et al. (2023). Primers utilized for qPCR are found in Supplementary Table 1. The LOW treatment was removed from analysis of gene expression data due to < 3 steers being represented for 14 of the 22 analyzed genes due to poor reads. Western blot analysis was not completed in this study so data presented are gene expression and further studies may be needed to compare with protein expression.
Statistical analysis
Data were analyzed using the Mixed procedure of SAS 9.4 (Cary, NC) with the fixed effect of treatment and steer was the experimental unit. Orthogonal linear and quadratic contrast statements were utilized, with coefficients based on supplemental Zn concentrations created using ProcIML. Initial plasma and liver TM concentrations were used as a covariate in subsequent analysis. Outliers were evaluated on an individual animal basis and removed if more than three standard deviations away from the treatment mean. For variables most treatments had no outliers, with a maximum of 2 outliers removed from a single treatment. The least-squared means and SEM are reported. Statistical significance was determined at a P ≤ 0.05, and a statistical tendency was determined at 0.05 < P ≤ 0.1.
Results
Trace mineral concentrations
Plasma Fe concentrations quadratically increased from CON to LOW treatments, then plateaued on d 48 (P = 0.02; Table 1) but was unaffected by treatment on d 67 (P = 0.46). Plasma Cu concentrations quadratically decreased from CON to LOW treatments, then leveled off on d 48 (P = 0.04). On d 67, plasma Cu concentrations tended to be quadratically increased, with the MED treatment having the highest concentration (P = 0.07). On d 48, plasma Zn concentrations linearly increased with Zn supplementation (P = 0.01), best explained by the quadratic trend (P = 0.10) where plasma Zn increased from CON to LOW and plateaued. On d 67, there was a linear and quadratic trend for plasma Zn concentrations to be increased from CON to LOW and are similar in MED and HI (P = 0.10).
Liver Mn concentrations tended to linearly increase on d 48 driven by MED and HI (P = 0.07; Table 2) but were not affected by treatment on d 67 (P ≥ 0.61). As Zn supplementation increased, liver Cu linearly decreased on d 48 and d 67 (P < 0.01). Liver Zn was not affected by treatment on d 48 (P ≥ 0.25) but tended to quadratically increase on d 67 where concentrations decreased from CON to MED, and then increased in HI (P = 0.08). Liver Fe was linearly increased on d 67 (P < 0.01) but was not affected by treatment on d 48 (P ≥ 0.12).
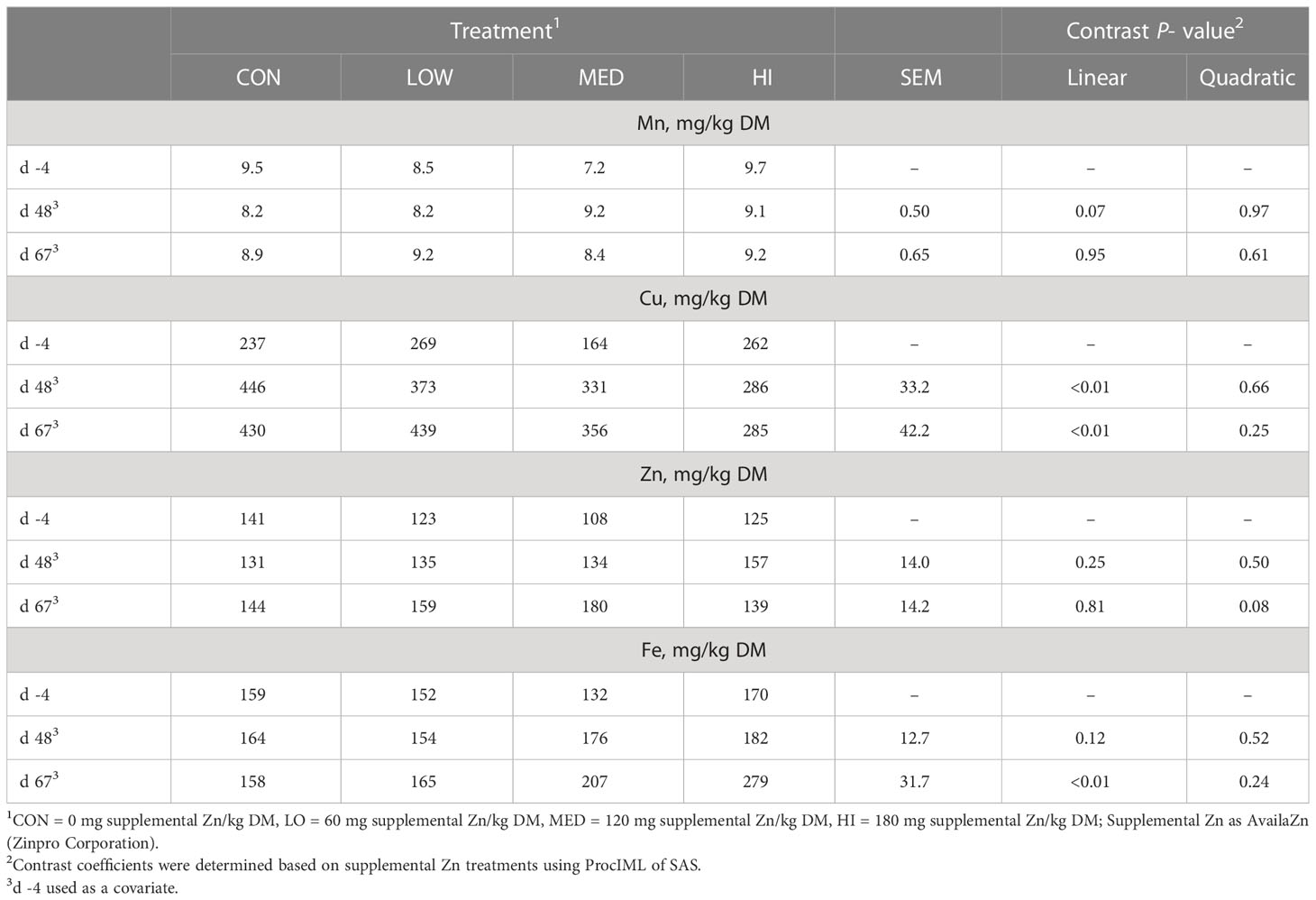
Table 2 Effects of Zn supplementation on the liver trace mineral concentrations of finishing beef steers.
Zn transport and storage gene expression
On d 48, there was a tendency for a quadratic decrease in SLC30A4 expression (P = 0.07; Table 3). There was a linear decrease in the expression of SLC39A8, SLC39A9, SLC39A10 and SLC39A13 on d 67 (P ≤ 0.05; Table 4). Additionally, the expression of MT1A, SLC30A4, SLC39A1 and SLC39A7 tended to linearly decrease on d 67 (P ≤ 0.10). There were no other differences in muscle gene expression (P > 0.10).
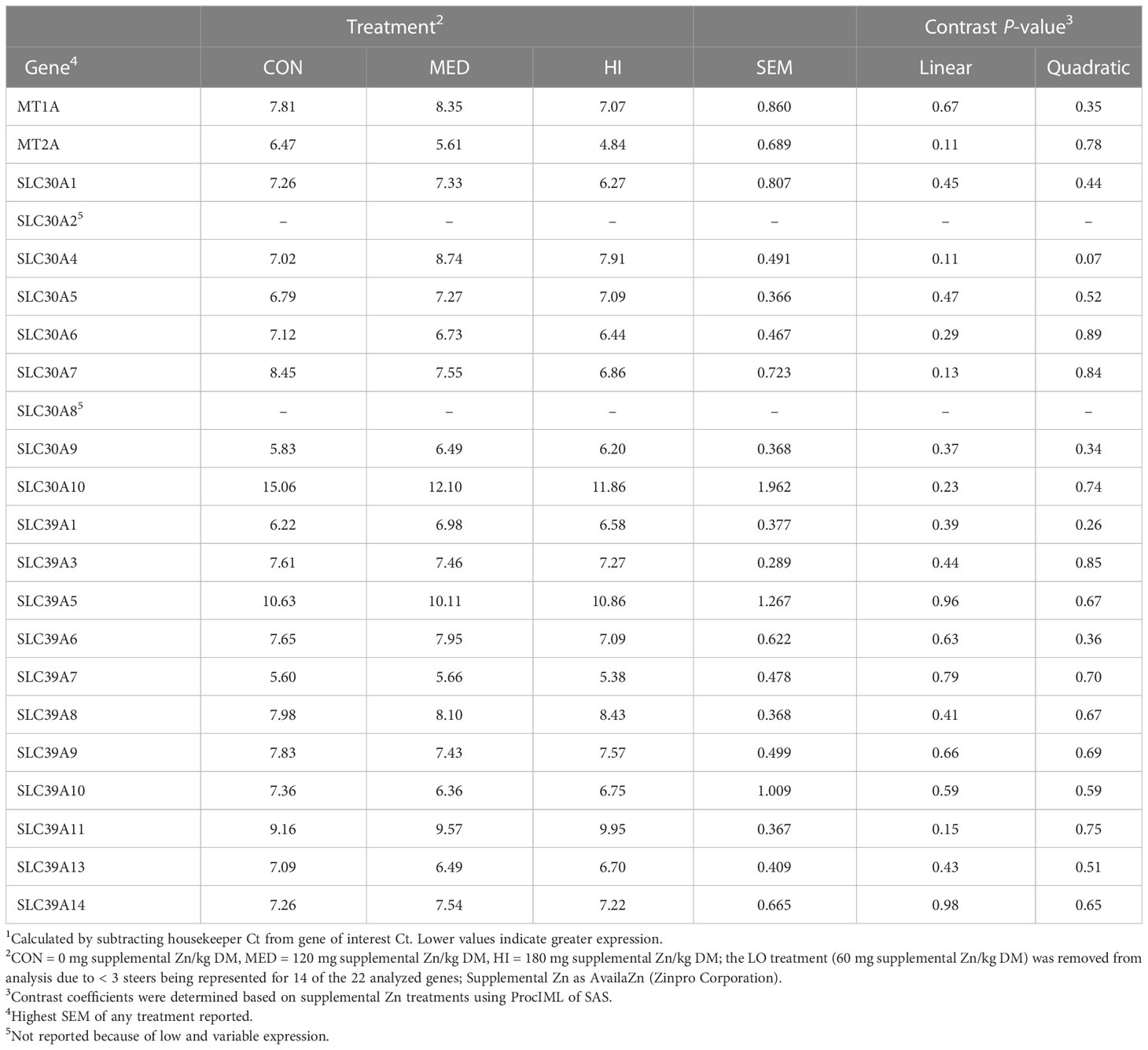
Table 3 Effect of supplementing Zn for 48 d (prior to start of ractopamine feeding on d 53) on delta Ct values1 of genes involved in Zn storage and transport in the muscle of finishing beef steers.
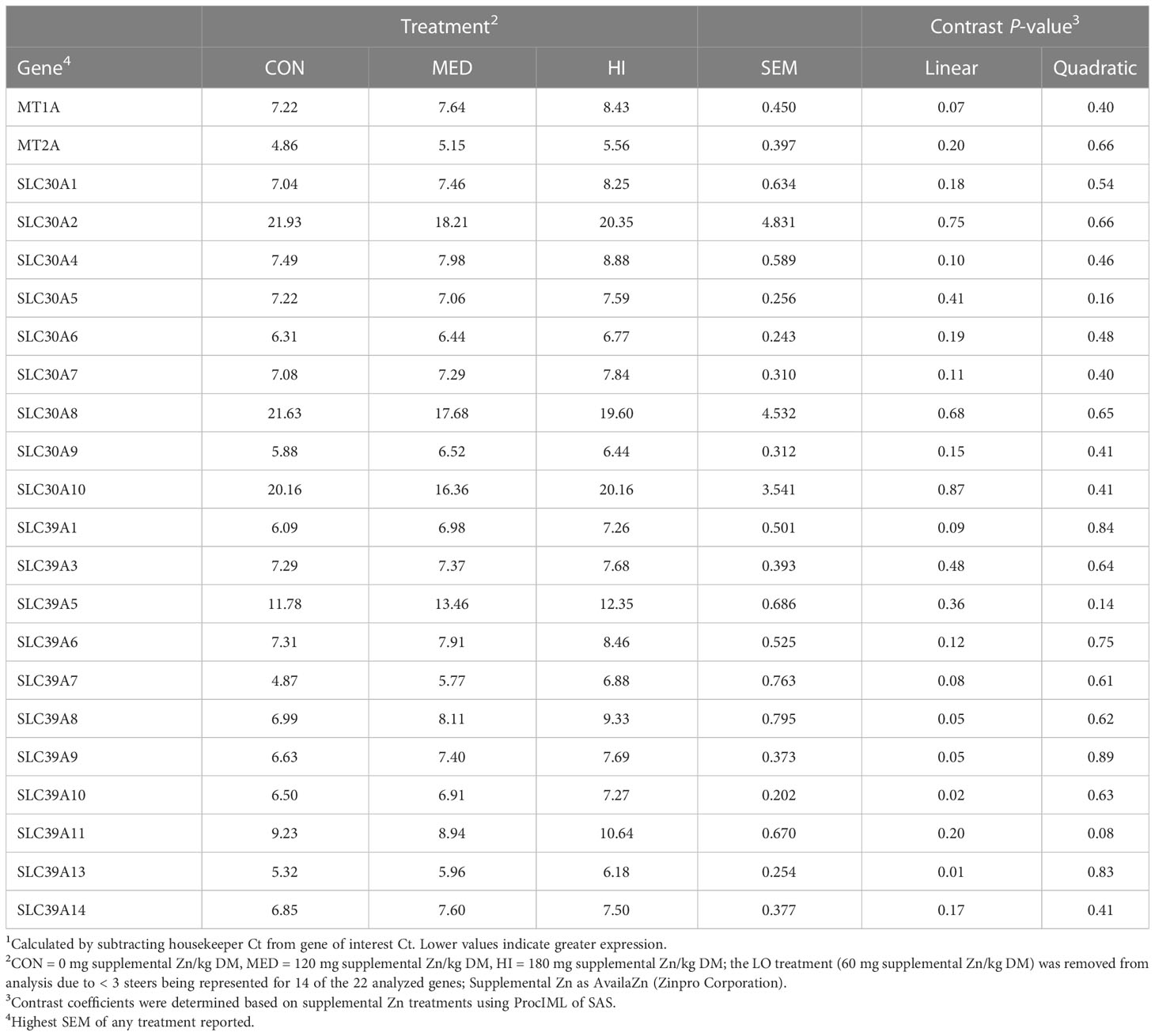
Table 4 Effect of supplementing Zn for 67 d (14 d after the start of ractopamine feeding on d 53) on delta Ct values1 of genes involved in Zn storage and transport in the muscle of finishing beef steers.
Discussion
Ractopamine hydrochloride increases protein synthesis (Anderson et al., 1990), resulting in greater muscle accretion (Mersmann, 1998). We have previously observed feeding high concentrations of Zn (120 mg supplemental Zn/kg DM) supports growth of RAC-fed steers but not non-RAC steers (Genther-Schroeder et al., 2016b). Additionally, N and Zn retention were found to be positively correlated in late-stage finishing steers (Carmichael et al., 2018). Because Zn is critical for protein synthesis (Oberleas et al., 1969; Williams and Chesters, 1970), additional Zn supplementation may support these demands in RAC-fed cattle. In the present study, we observed decreases in the patterns of gene expression of Zn transporters in muscle of RAC-fed cattle, which may help explain the improvements in performance previously observed in Zn and RAC fed cattle.
ZIP transporters are members of the solute carrier 39 (SLC39) superfamily and once translated, the membrane-bound proteins move Zn into the cytoplasm (Jeong and Eide, 2013). ZnT transporters are encoded by the gene solute carrier 30 (SLC30) family and are responsible for the movement of Zn across membranes out of the cytoplasm (Huang and Tepaamorndech, 2013). In the current study, limited differences in Zn transporter gene expression in the muscle were noted between dietary Zn treatments prior to RAC feeding (d 48). However, genes encoding for ZIP1, ZIP7, ZIP8, ZIP9, and ZIP13 linearly decreased as dietary Zn increased after 14 d of RAC feeding (d 67). Although growth performance of these cattle is not shown in this paper, across all cattle average daily gain prior to RAC was 1.97 ± 0.27 kg/day and during the RAC period increased to 2.24 ± 0.29 kg/day (mean ± SD). Some of these transporters, ZIP1 and ZIP8, have previously been found on the plasma membrane of cells (Gaither and Eide, 2001; Besecker et al., 2008) while others, ZIP7, ZIP9, ZIP13, and ZnT7, have previously been found on the golgi apparatus membrane (Kirschke and Huang, 2003; Huang et al., 2005; Bin et al., 2011), where Zn incorporation into metalloenzymes is thought to occur (Eide, 2006). Overall, the linear decreases in ZIP transporters on d 67 are driven by greater expression in non-Zn supplemented steers, suggesting increased Zn trafficking into the muscle cytoplasm during the RAC period. With the increased protein synthesis demands associated with RAC, observing changes in gene expression of transporters previously found to be associated with organelles where proteins are synthesized further supports the need for Zn in RAC induced growth. The lower expression of Zn trafficking genes in cattle supplemented with 60 to 180 mg Zn/kg DM may suggest they had adequate Zn stores available to respond to RAC-induced protein synthesis demands.
While plasma Zn concentrations were increased in Zn-supplemented cattle compared to the control on d 48 and 67, there was minimal difference in plasma Zn concentrations within steers receiving Zn supplementation. Zinc supplementation above 100 mg Zn/kg DM has been found to increase plasma zinc concentrations compared to non-supplemented controls, with no further rises noted as Zn supplementation increased up to 200 mg Zn/kg DM (Huerta et al., 2002; Messersmith et al., 2019). Plasma Zn remains constant unless there is significant demand on the Zn pool or below adequate concentrations in the diet (Wieringa et al., 2015). The lower plasma Zn concentrations in control steers around 1.05 mg/L may be insufficient during periods of high growth. However, Zn supplemented steers had plasma Zn concentrations greater than 1.2 mg/L which may be sufficient to meet the increased growth demands induced by RAC feeding. With a greater concentration of Zn in the plasma of supplemented steers, more Zn is available for movement into the muscle via ZIP transporters. However, in control steers with lower plasma Zn concentrations, gene expression of ZIP transporters may be increased to allow for greater opportunity for Zn trafficking to meet demands in the muscle cell. Messersmith et al. (2022) found plasma Zn to increase in Zn-supplemented steers over non-supplemented controls, and that Zn-fed steers had greater ADG in response to anabolic implant. More work is needed to understand the plasma Zn concentration needed to support optimal growth in cattle.
Data from this study shed light on the effects of RAC feeding and Zn supplementation on the Zn trafficking within muscle cells. The increased expression of Zn trafficking genes in control steers during the RAC period but not the pre-RAC period suggests 35 mg Zn/kg DM may be insufficient to optimally support growth induced by RAC. This may be why cattle growth is sometimes improved in response to Zn supplementation concurrent with growth promoting technologies such as beta agonists or anabolic implants (Messersmith et al., 2019; Messersmith et al., 2022). Without Zn supplementation, Zn trafficking genes are upregulated during periods of increased growth further indicating the need for Zn in muscle. Further research is needed to better understand Zn movement throughout the muscle during periods of increased growth.
Data availability statement
The original contributions presented in the study are included in the article/Supplementary Materials, further inquiries can be directed to the corresponding author/s.
Ethics statement
The animal study was reviewed and approved by Iowa State University Institutional Animal Care and Use Committee (IACUC #8-15-8073-B).
Author contributions
Conceptualization, SH, OG-S. Funding acquisition, SH. Investigation, RW. Data curation and analysis, ED, RW, ER. Writing- original draft preparation, ER. Writing- review and editing, SH, OG-S, ED, RW. All authors contributed to the article and approved the submitted version.
Funding
Funding for this research was provided by USDA grant number 2015-06420.
Conflict of interest
All authors were employees of Iowa State University during the time the experiment was conceived, conducted, data analyzed, and the manuscript written. Current author affiliations include RWC with Great Plains Livestock Consulting, Inc., ELD with Kemin Industries, Inc., and OGS with Land O’Lakes, Inc.
The authors declare that the research was conducted in the absence of any commercial or financial relationships that could be construed as a potential conflict of interest.
Publisher’s note
All claims expressed in this article are solely those of the authors and do not necessarily represent those of their affiliated organizations, or those of the publisher, the editors and the reviewers. Any product that may be evaluated in this article, or claim that may be made by its manufacturer, is not guaranteed or endorsed by the publisher.
Supplementary material
The Supplementary Material for this article can be found online at: https://www.frontiersin.org/articles/10.3389/fanim.2023.1191230/full#supplementary-material
References
Anderson P. T., Helferich W. G., Parkhill L. C., Merkel R., Bergen W. G. (1990). Metabolism and hormonal regulation ractopamine increases total and myofibrillar protein synthesis in cultured rat myotubes J. Nutr. 1, 1677–1683. doi: 10.1093/jn/120.12.1677
Besecker B., Bao S., Bohacova B., Papp A., Sadee W., Knoell D. L. (2008). The human zinc transporter SLC39A8 (Zip8) is critical in zinc-mediated cytoprotection in lung epithelia. Am. J. Physiol. Lung Cell Mol. Physiol. 294, 1127–1136. doi: 10.1152/ajplung.00057.2008.-Zinc
Bin B. H., Fukada T., Hosaka T., Yamasaki S., Ohashi W., Hojyo S., et al. (2011). Biochemical characterization of human ZIP13 protein: a homo-dimerized zinc transporter involved in the spondylocheiro dysplastic ehlers-danlos syndrome. J. Biol. Chem. 286, 40255–40265. doi: 10.1074/jbc.M111.256784
Carmichael R. N., Genther-Schroeder O. N., Deters E. L., Jackson T. D., Messersmith E. M., VanValin K. R., et al. (2018). The influence of supplemental zinc and dietary fiber concentration on mineral retention of beef steers. Trans. Anim. Sci 96, 2939–2948. doi: 10.1093/tas/txz032
Eide D. J. (2006). Zinc transporters and the cellular trafficking of zinc. Biochim. Biophys. Acta Mol. Cell Res. 1763, 711–722. doi: 10.1016/j.bbamcr.2006.03.005
Engle T. E., Spears J. W. (2000). Effects of dietary copper concentration and source on performance and copper status of growing and finishing steers. J. Anim. Sci. 78, 2446–2451. doi: 10.2527/2000.7892446x
Gaither L. A., Eide D. J. (2001). The human ZIP1 transporter mediates zinc uptake in human K562 erythroleukemia cells. J. Biol. Chem. 276, 22258–22264. doi: 10.1074/jbc.M101772200
Genther-Schroeder O. N., Branine M. E., Hansen S. L. (2016a). The influence of supplemental zn-amino acid complex and ractopamine hydrochloride feeding duration on growth performance and carcass characteristics of finishing beef cattle. J. Anim. Sci. 94, 4338–4345. doi: 10.2527/jas.2015-0159
Genther-Schroeder O. N., Branine M. E., Hansen S. L. (2016b). The effects of increasing supplementation of zinc-amino acid complex on growth performance, carcass characteristics, and inflammatory response of beef cattle fed ractopamine hydrochloride. J. Anim. Sci. 94, 3389–3398. doi: 10.2527/jas.2015-0209
Huang L., Kirschke C. P., Zhang Y., Yan Y. Y. (2005). The ZIP7 gene (Slc39a7) encodes a zinc transporter involved in zinc homeostasis of the golgi apparatus. J. Biol. Chem. 280, 15456–15463. doi: 10.1074/jbc.M412188200
Huang L., Tepaamorndech S. (2013). The SLC30 family of zinc transporters-a review of current understanding of their biological and pathophysiological roles. Mol. Aspects Med. 34, 548–560. doi: 10.1016/j.mam.2012.05.008
Huerta M., Kincaid R. L., Cronrath J. D., Busboom J., Johnson A. B., Swenson C. K. (2002). Interaction of dietary zinc and growth implants on weight gain, carcass traits and zinc in tissues of growing beef steers and heifers. Anim. Feed Sci. Technol. 95, 15–32. doi: 10.1016/S0377-8401(01)00334-0
Jeong J., Eide D. J. (2013). The SLC39 family of zinc transporters. Mol. Aspects Med. 34, 612–619. doi: 10.1016/j.mam.2012.05.011
Kirschke C. P., Huang L. (2003). ZnT7, a novel mammalian zinc transporter, accumulates zinc in the golgi apparatus. J. Biol. Chem. 278, 4096–4102. doi: 10.1074/jbc.M207644200
Mersmann H. J. (1998). Overview of the effects of β-adrenergic receptor agonists on animal growth including mechanisms of action. J. Anim. Sci. 76, 160–172. doi: 10.2527/1998.761160x
Messersmith E. M., Niedermayer E. K., Crawford G. I., Hansen S. L. (2019). “Effect of zn supplementation on performance, carcass characteristics, and liver mineral concentration on heifers receiving a single revalor-XH or a revalor-200/Revalor-200 re-implant program,” in Proceedings of Plains Nutrition Council Spring Conference Plains nutrition council meeting in 2019 in San Antonio TX.
Messersmith E. M., Smerchek D. T., Hansen S. L. (2022). Effects of increasing supplemental zinc in beef feedlot steers administered a steroidal implant and beta agonist. Transl. Anim. Sci. 6, 1–10. doi: 10.1093/tas/txac029
Oberleas D., Prasad A. S., Ii, ‘ D. (1969). Growth as affected by zinc and protein nutrition. Am. J. Clin. Nutr. 22, 1304–1314. doi: 10.1093/ajcn/22.10.1304
Pampusch M. S., White M. E., Hathaway M. R., Baxa T. J., Chung K. Y., Parr S. L., et al. (2008). Effects of implants of trenbolone acetate, estradiol, or both, on muscle insulin-like growth factor-I, insulin-like growth factor-I receptor, estrogen receptor-ά, and androgen receptor messenger ribonucleic acid levels in feedlot steers. J. Anim. Sci. 86, 3418–3423. doi: 10.2527/jas.2008-1085
Pogge D. J., Hansen S. L. (2013). Supplemental vitamin c improves marbling in feedlot cattle consuming high sulfur diets. J. Anim. Sci. 91, 4303–4314. doi: 10.2527/jas2012-5638
Reichhardt C. C., Cuthbert J. M., Motsinger L. A., Brady T. J., Briggs R. K., Thomas A. J., et al. (2023). Anabolic implants alter abundance of mRNA involved in muscle growth, metabolism, and inflammation in the longissimus of Angus steers in the feedlot. Domest Anim. Endocrinol. 82, 106773. doi: 10.1016/j.domaniend.2022.106773
Rients E. L., Deters E. L., McGill J. L., Belknap C. R., Hansen S. L. (2023). Effects of feeding a saccharomyces cerevisiae fermentation product and ractopamine hydrochloride to finishing beef steers on growth performance, immune system, and muscle gene expression. J. Anim. Sci. 101, 1–11. doi: 10.1093/jas/skac311
von Bülow V., Rink L., Haase H. (2005). Zinc-mediated inhibition of cyclic nucleotide phosphodiesterase activity and expression suppresses TNF-α and IL-1β production in monocytes by elevation of guanosine 3′,5′-cyclic monophosphate. J. Immunol. 175, 4697–4705. doi: 10.4049/jimmunol.175.7.4697
Wieringa F. T., Dijkhuizen M. A., Fiorentio M., Laillou A., Berger J. (2015). Determination of zinc status in humans: which indicator should we use? Nutrients 7, 3252–3263. doi: 10.3390/nu7053252
Keywords: beef cattle, gene expression, muscle, ractopamine hydrochloride, zinc, zinc transport
Citation: Rients EL, Wyatt RN, Deters EL, Genther-Schroeder ON and Hansen SL (2023) Zinc supplementation and ractopamine hydrochloride impact gene expression of zinc transporters in finishing beef steers. Front. Anim. Sci. 4:1191230. doi: 10.3389/fanim.2023.1191230
Received: 21 March 2023; Accepted: 06 June 2023;
Published: 20 June 2023.
Edited by:
Kendall C. Swanson, North Dakota State University, United StatesReviewed by:
Jon Schoonmaker, Purdue University, United StatesTerry Engle, Colorado State University, United States
Copyright © 2023 Rients, Wyatt, Deters, Genther-Schroeder and Hansen. This is an open-access article distributed under the terms of the Creative Commons Attribution License (CC BY). The use, distribution or reproduction in other forums is permitted, provided the original author(s) and the copyright owner(s) are credited and that the original publication in this journal is cited, in accordance with accepted academic practice. No use, distribution or reproduction is permitted which does not comply with these terms.
*Correspondence: Stephanie L. Hansen, c2xoYW5zZW5AaWFzdGF0ZS5lZHU=
†Present address: Remy N. Wyatt, Great Plains Livestock Consulting, Inc., Eagle, NE, United States
Erin L. Deters, Kemin Industries, Inc., Human Nutrition and Health North America, Des Moines, IA, United States
Olivia N. Genther-Schroeder, Land O’Lakes, Inc., Arden Hills, MN, United States