- 1Hill’s Pet Nutrition, Topeka, KS, United States
- 2Department of Grain Science & Industry, Kansas State University, Manhattan, KS, United States
- 3Department of Food, Nutrition, Dietetics and Health, Kansas State University, Olathe, KS, United States
As the demand for dog food production continues to rise, along with the number of dogs per household in the US, sustainable ingredients must be considered for the pet food industry. Consumers have a negative perspective when it comes to animal by-products in their pet’s food. However, animal by-products contribute a quality source of dietary protein, vitamins, and minerals which contribute to nutritionally complete pet foods. Along with being nutritionally complete, foods must also appeal to the dog while eating. The objective of this study was to produce formulas utilizing four different chicken protein sources, and to evaluate the effects of those protein sources on palatability in dogs. Five treatments were produced, through extrusion, using fresh mechanically deboned chicken at two different inclusion levels: (FMDC14 and FMDC25), dried chicken (DC), chicken meal (CM), and chicken by-product meal (CBPM). Four trained panels of twenty-five adult dogs, made up of majority Beagles, randomly divided by sex, were used for palatability testing using the two-bowl test. Based on the palatability results from this study, the CBPM treatment was preferred (p<0.05) over the FMDC14, FMDC25, and DC treatments. The CBPM treatment was at parity to the CM treatment (p>0.05). It can be concluded that the CBPM treatment was considered palatable to the dogs.
Introduction
As the number of dogs per household continues to rise, so does the demand for more dog food. Sales are predicted to rise 5% from 2020 to 2021 and are expected to continue to increase for the next five years (APPA, 2021). The COVID-19 pandemic, along with humanization and premiumization trends, are driving this increase in sales (Sprinkle, 2021). Many pet owners consider their pets as members of the family, and consumer preference has shifted towards certain food ingredients to be more “human-grade” and less processed (Meineri et al., 2021). With the addition of more fresh chicken in pet food formulas, this puts a strain on the global demand for chicken protein sources and may begin to compete with the human food industry (Okin, 2017) (Nijdam et al., 2012). As the demand for dog food increases, animal-based protein supplies are increasing demand, and could have an ecological impact on current food systems.
As pet food production continues to rise, the utilization of more sustainable ingredients must be considered, especially in regard to protein sources. Sustainability, defined from a pet food industry standpoint, is described as the ability to produce enough food for the growing pet population, while providing sufficient nutrition, and leaving the smallest environmental footprint possible. To provide a sustainable future, the shift to more sustainable ingredients must be discussed. Chicken has the lowest environmental impact when compared to other animal protein sources, and chicken by-products can be used within the pet food industry (Knight, 2020). By utilizing by-products, the environmental effects of organic waste disposal are reduced (Gooding and Meeker, 2016). Unfortunately, the pet owners’ uncertainty regarding by-products tends to lead to a negative perception and are often labeled as poor-quality ingredients (Laflamme et al., 2014). Consumers often presume that these secondary products provide little benefit and often avoid purchasing products with these ingredients on the label. The definition of by-products is the “clean secondary products produced in addition to the principal product” therefore, chicken by-product meal will include “ground, rendered, clean parts of slaughtered poultry, such as heads, feet, undeveloped eggs and intestines, exclusive of feathers” (AAFCO, 2021). By-products, when rendered properly, provide quality protein and trace minerals to canine foods (Donadelli et al., 2019). Other chicken protein sources defined by AAFCO include chicken meat, or fresh mechanically deboned chicken: “the clean combination of flesh and skin without accompanying bone, derived from the parts or whole carcasses of poultry or a combination thereof, exclusive of feathers, heads, feet and entrails,” dried chicken: which includes everything prior but is dried, and chicken meal which includes everything prior, also dried, and can be accompanied with or without bone. All four are used as protein sources in the pet food industry and provide good nutrition (AAFCO, 2021). Protein quality is determined by measuring how well the amino acid profile and bioavailability of those amino acids match the requirements of the dog’s life stage (Donadelli et al., 2019). While previous studies have measured digestibility between fresh poultry and poultry by-products used in formulas, canine preference between the two ingredients, or other forms of chicken has not been measured (Murray et al., 1997).
Aside from the food product being nutritionally balanced, the product must also be palatable and accepted by the dog. Unlike humans, dogs consume their daily nutritional requirements from a single food. Therefore, it is imperative that the foods are palatable enough for a pet to consume an adequate volume to meet their nutritional requirements, and consumption is the primary indicator used in the pet food industry to evaluate the palatability of products (Aldrich and Koppel, 2015). A complete profile of finished food products, including texture, flavor, and aroma, are needed to help decipher canine preference (Koppel, 2014). Previous studies have measured canine food preference through odor, taste, and texture: (Houpt et al., 1978; Griffin, 2003; Walker et al., 2006; Neufield, 2012; Samant et al., 2021). Impact of ingredient inclusion on food preference of dogs has been previously researched including soybean inclusion (Félix et al., 2012), fiber inclusion (Koppel et al., 2015), vegetable ingredient-based formulas (Callon et al., 2017), and raw versus cooked meats (Tsai, 2019). Still, a limited number of studies can be found with a focus on food preference of dogs for ingredients themselves. A deeper understanding of the preference of protein sources by dogs may further support the use of by-products in pet foods, and further convince consumers of their benefits. Therefore, it was the objective of this study to produce formulas utilizing four different chicken protein sources, and to evaluate the effects of those protein sources on canine palatability.
Materials and methods
Formulation
Five experimental formulas were formulated using the computer software, Concept 5 (Creative Formulation Concepts, LLC Annapolis, MD, USA) with four different chicken protein sources; fresh mechanically deboned chicken (FMDC; BHJ USA Inc. Saint Joseph, MO, USA), dried chicken (DC; Protein Solutions, LLC. Joplin, MO, USA), chicken meal (CM; Tyson Foods. Clarksville, AR, USA), and chicken by-product meal (CBPM; Darling International Inc. Nishnabotna, MO, USA). The CM, DC, and CBPM formulas were formulated at an ingredient inclusion level of 14%. The fresh mechanically deboned chicken formulas were formulated at two separate inclusion rates: a 14% fresh mechanically deboned chicken (FMDC14) inclusion rate and a 25% fresh mechanically deboned chicken (FMDC25) inclusion rate. The FMDC14 formula was formulated to match the volume (14%) of the chicken ingredients in the CM, DC, and CBPM formulas, while the FMDC25 formula was formulated to have similar crude protein levels as the CM, DC, and CBPM formulas (25%). The fat content of each formula was standardized, using soybean oil. The remainder of each formula was comprised of brewer’s rice, rice protein concentrate, cellulose, beet pulp, vitamins, minerals, and an antioxidant blend (Table 1). Lactic acid was added to dry formulas to lower the food’s pH to maximize microbial stability (Hand et al., 2010; Montelongo et al., 2012). The five experimental formulas were formulated to meet AAFCO (2021) canine maintenance nutritional requirements.
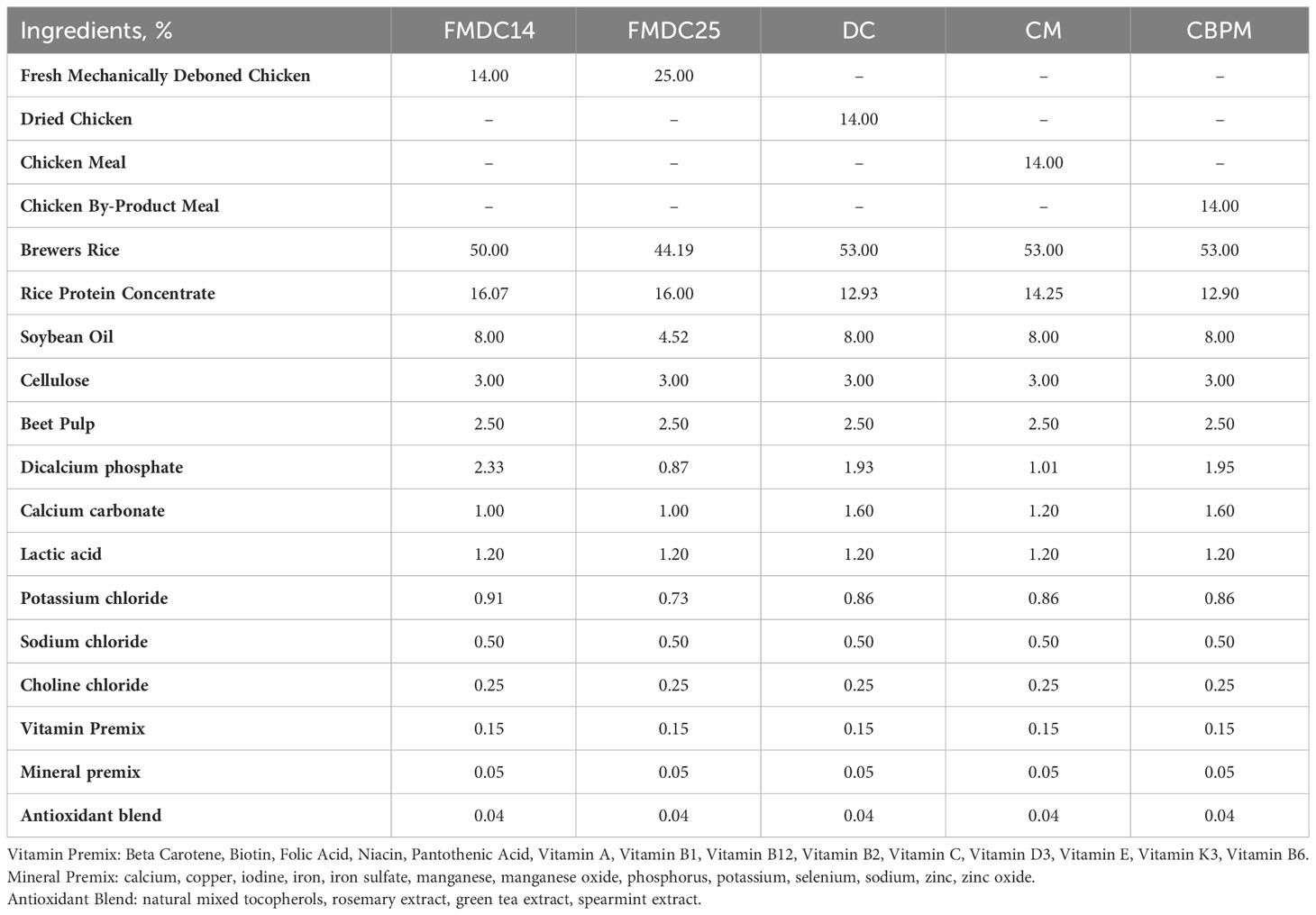
Table 1 Formula Composition: fresh mechanically deboned chicken 14% (FMDC14), fresh mechanically deboned chicken 25% (FMDC25), dried chicken (DC), chicken meal (CM), chicken by-product meal (CBPM).
Formula production
Five experimental formulas were produced in the Hill’s Experimental Food Lab at the Hill’s Pet Nutrition Center (Topeka, KS, USA). All dry ingredients were weighed, and then mixed with a ribbon blender (Wenger Mfg., Sabetha, KS, USA). Ingredients were mixed for five minutes, and then transferred to the hammer mill (Jacobs Corporation, Harlan, IA, USA) to be ground to a consistent particle size (No. 16 mesh sieve). Once complete, the dry ingredients were added to the preconditioner (Wenger Mfg., Sabetha, KS, USA) at a feed rate of 14-14.5 lb./min. In the preconditioner, moisture, steam, lactic acid, and choline chloride were added to the dry ingredients and mixed with paddles at 235-240 rpm. Temperature of the mixture reached 185°F at the throat of the preconditioner before exiting to the extruder.
A single screw extruder (Model X-85; Wenger Mfg., Sabetha, KS, USA) with a common medium shear pet food screw profile was used. After extrusion, the product was conveyed to a dual pass dryer (AeroDry; Buhler Aeroglide, Cary, NC, USA) set at 150-155°F to obtain a final moisture of less than 10%. After drying, the product was collected and transferred to a ribbon mixer where soybean oil was added manually and mixed for three minutes to achieve equal distribution. Finally, the finished product was packaged in poly-lined plastic bags, (15-pounds per bag), passed through a metal detector, and stored in ambient conditions until used for scheduled testing.
Kibble analysis
All five experimental formulas were analyzed for moisture, protein, and fat utilizing near-infrared spectroscopy (NIR; Foss NIRS DS2500, Eden Prairie, MN, USA) in the Hill’s Experimental Food Lab for quality control purposes. NIR analysis was used to measure moisture, protein, and fat levels during production, therefore when production hit a steady state, the product could then be collected. Bulk density was analyzed at regular intervals during production until the density reached the target measurement of 23 lb./ft3. A final NIR analysis was conducted after the soybean oil was topically applied, to ensure the target application has been met. In the Hill’s Food Science Lab, twenty kibbles from each experimental formula were measured for diameter, cut length, weight, and analyzed for texture. Digital calipers (Mitutoyo ABSOLUTE A500-171, Aurora, IL, USA) were used to measure the diameter and cut length in millimeters while an analytical scale (Ohaus Explorer Scales, Pleasant Prairie, WI, USA) was used to measure the weight in grams. Quality tests were also performed on the formulas to determine water activity and pH levels. Water activity was measured using a water activity meter (Aqua Lab 4TEV, Pullman, WA, USA). To increase shelf life, dry dog foods tend to have a water activity below 0.60 (Samant et al., 2021). The pH was measured by mixing 10 g of ground kibbles with 90 mls of water. The mixture was stirred intermittently for 20 minutes, then allowed to settle for 5 minutes before measuring the pH with a probe (VWR Symphony SP70P, Radnor, PA, USA).
Texture analysis was performed to evaluate the kibbles for hardness using a TA.XT plus Texture Analyzer (Texture Technologies Corporation, Hamilton, MA, USA), equipped with 50-kg load cells. The procedure used was a modified version from the method used by Dogan and Kokini (2007) and has been used in other studies (Manbeck et al., 2017; Alvarenga et al., 2018; Smith, 2018). A 25 mm cylindrical probe was used for a compression test with a pre-test speed of 2 mm/s, a test speed of 1 mm/s, a post-test speed of 10 mm/s, and a strain level of 50%. A total of 20 kibbles, from each formula, were measured for hardness. The first peak fracture force (kg) was taken as a measure of hardness for each kibble analyzed.
A final composite sample of each formula was sent to a commercial laboratory, (Eurofins Scientific, Inc., Des Moines, IA, USA) for a full nutritional panel analysis. A combination of methods organized by the Association of Official Agricultural Chemists (AOAC) and Eurofins proprietary methods were used to perform nutritional analyses to Hill’s Standards.
Palatability testing procedure
This study used the same method as Hall et al. (2018) to measure palatability using the trained canine panel at the Hill’s Pet Nutrition Center (Topeka, KS, USA). All canine use was approved by the Institution Animal Care and Use Committee, Hill’s Pet Nutrition Center (Topeka, KS, USA) (Hill’s IACUC #CP26/permit number: 590). Four trained panels of 25 adult dogs, made up of majority Beagles (average age 10.8 +/- 2.9 years and average weight 8.1 +/- 2.8 kgs), randomly divided by sex, were used for palatability testing. Dogs were deemed to be in good health, trained for palatability testing, calibrated annually, and were able to discriminate between foods. Dogs were provided with access to water ad libitum. Test formulas were fed double blind using non descriptive labels, represented by internal lot codes, by trained animal care technicians who have no previous knowledge of the test formulas.
Palatability was measured for the five formulas (FMDC14, FMDC25, DC, CM, and CBPM) using the two-bowl test, fed one meal per day in the morning, over the duration of two days for a total of two meals. For each day of the palatability test, caloric intake was set to meet each dog’s daily needs based on their weight. The feeding time allotment was set for 30 minutes. Bowls were placed on the designated feeding scales, which are programed to allow each dog to only consume their daily caloric intake from either one bowl or a combination of some from each bowl. These bowls were then presented simultaneously to each dog. The test was considered complete once each dog consumed enough calories to maintain their body weight and/or after 30 minutes had expired. On the second day of testing, the bowls were switched to avoid side bias. Once feeding was complete, the final weight from each bowl was recorded. Intake ratio and first bite were recorded during the palatability test. The first bite was measured for each dog at the beginning of feeding. Load scales under each bowl helped determine which food had the first bite by measuring the change in weight. The change in weight of the bowls was monitored continuously to also assess the consumption rate. At the end of the test, the intake ratio (IR) was calculated using the following equation (Eq. 1) and the bowl with the most food consumed was considered the preferred food (Griffin, 2003):
Statistical analysis
The model statement for kibble measurements contained cut length, diameter, and weight as fixed variables. The model statement for the texture analysis contained hardness. All means were separated using Fisher’s Least Significant Difference (LSD) with a significance of (α = 0.05). LSDs are shown with superscript letters to determine (p<0.05) differences between samples.
For palatability equivalency testing, food consumption was measured using the IR. A Student’s t-test was conducted to classify the IR results of the overall panel. A p-value< 0.05 expresses that the IR of the test was greater than 0.50 (Vondran, 2013). The first bite data was analyzed using a Chi2 test.
Results and discussion
Formulation
Experimental formulas were formulated to ensure limited impacts of the other ingredients on palatability (Table 1). No palatants or animal fat were added to keep the base formulas as bland as possible so that the protein is not diluted or masked. To accommodate the differences in the nutritional matrix of the different chicken proteins, minor adjustments to brewer’s rice, rice protein concentrate, and soybean oil were made. The use of bland or flavor neutral ingredients, (brewer’s rice, rice protein concentrate, and soybean oil), in the formulas should not affect palatability with any minor changes to meet nutritional requirements. The other ingredients, cellulose, beet pulp, lactic acid, choline chloride, vitamins, minerals, and the antioxidant blend were held as constant as possible across all five experimental formulas.
Production
All five formulas were batched and produced on the same day to minimize variability by ensuring raw materials were from the same supplier lot codes. During extrusion, all processing parameters were held constant to limit physical differences to the finished food for palatability testing. All five formulas were successful runs, producing usable finished products for palatability, analytical, and other scheduled testing.
While all five formulas were successful and collected, the FMDC25 formula did not reach the target of 25% inclusion of fresh mechanically deboned chicken. This was most likely due to the increased moisture contribution. When sufficient steam was added to reach the target temperature, the mixture developed a doughy texture in the extruder that was not able to form an extruded kibble. The maximum level of fresh mechanically deboned chicken inclusion reached was 22%. Further changes in the processing parameters may have allowed the 25% level of inclusion but would have added additional variables to the study design.
Kibble analysis
Result of the NIR testing collected post-production for quality control purposes are reported in Table 2. The FMDC25 formula yielded the highest bulk density (23.44 kg/m3) and highest moisture (9.2%). This was most likely due to the elevated levels of water from the high rate of inclusion of fresh mechanically deboned chicken. However, the FMDC14 formula measured the lowest in moisture (6.4%), which was most likely a result of the drying step. Measured protein was lowest in the FMDC14 (21.62%) formula because of the lower protein contribution from the fresh mechanically deboned chicken ingredient (due to higher moisture dilution) while the CM formula had the highest protein (26.68%) because it had the highest concentration of protein as an ingredient. Fat % was lowest for the FMDC25 formula due to less soybean oil added compared to the FMDC14, DC, CM, and CBPM formulas. This may be an indirect result of the higher fat content in the fresh mechanically deboned chicken ingredient at that high level of inclusion.

Table 2 Kibble NIR measurements (As Fed) and density post-production of canine formulas: fresh mechanically deboned chicken 14% (FMDC14), fresh mechanically deboned chicken 25% (FMDC25), dried chicken (DC), chicken meal (CM), chicken by-product meal (CBPM).
Kibble diameter, cut length, mass, and hardness values are provided in Table 3. The cut length of the kibbles differed (p<0.05) between experimental formulas. The FMDC25 kibbles measured the largest at 5.44 mm while the DC kibbles measured the smallest at 4.76 mm. The kibble diameter between the CBPM, CM, DC, and FMDC14 kibbles were not different (p>0.05) from each other (ranging between 12.53-12.89mm) but did differ (p<0.05) from FMDC25, which measured the smallest at 12.06 mm. Kibble mass also differed (p<0.05) between formulas. Mass was similar between the CM, FMDC14, and FMDC25 kibbles (0.30 g) while the CBPM kibbles weighed a little more (0.32 g) and the DC kibbles weighed a little less (0.28 g).

Table 3 Kibble measurements and texture analysis: fresh mechanically deboned chicken 14% (FMDC14), fresh mechanically deboned chicken 25% (FMDC25), dried chicken (DC), chicken meal (CM), chicken by-product meal (CBPM).
Hardness in this study was defined as the peak force (kg) of each kibble compression. Hardness in pet food is measured as the amount of force that is needed to initially fracture a kibble which simulates a first bite for dogs. According to Dogan and Kokini (2007), the force needed to initially fracture a kibble is related to the internal structure which includes porosity, size of pores, and cell wall thickness. Texture can influence the final product’s palatability; therefore, it was important that each formula was similar in hardness (Koppel et al., 2015). Each formula was statistically different (p<0.05) however, with the largest difference seen between the DC (28.31 kg of force) kibbles and the CM (25.82 kg of force) kibbles. Dunsford et al. (2002) concluded that smaller kibble cell walls reinforce each other, thus requiring more force to break the kibble. This may be the case for the DC formula in this study since it measured the smallest for cut length. Processing variables have also been shown to influence hardness and texture of formulas (Alvarenga et al., 2018).
Quality check results for all five canine formulas have been provided in Table 4. Water activity (aw) results for the kibbles ranged from 0.37 to 0.58, with the FMDC25 formula measured the highest at 0.58 aw whereas the FMDC14 formula measured the lowest at 0.37 aw. The CM, CBPM, and DC formulas measured around 0.50 aw. The pH for the FMDC14 formula measured the lowest at 4.78 while the FMDC25, DC, CM, and CBPM formulas measured around a pH of 5.

Table 4 Kibble quality analysis: fresh mechanically deboned chicken 14% (FMDC14), fresh mechanically deboned chicken 25% (FMDC25), dried chicken (DC), chicken meal (CM), chicken by-product meal (CBPM).
Nutrient analysis results of the five canine formulas have been provided in Table 5. This analysis provides insight on how the formulas are nutritionally comparable to one another. To ensure all products were similar nutritionally, each formula’s predicted (As Fed) nutritional requirements were compared before production. Analytical results conclude that nutrients analyzed above the AAFCO minimum requirements for adult dogs at maintenance.
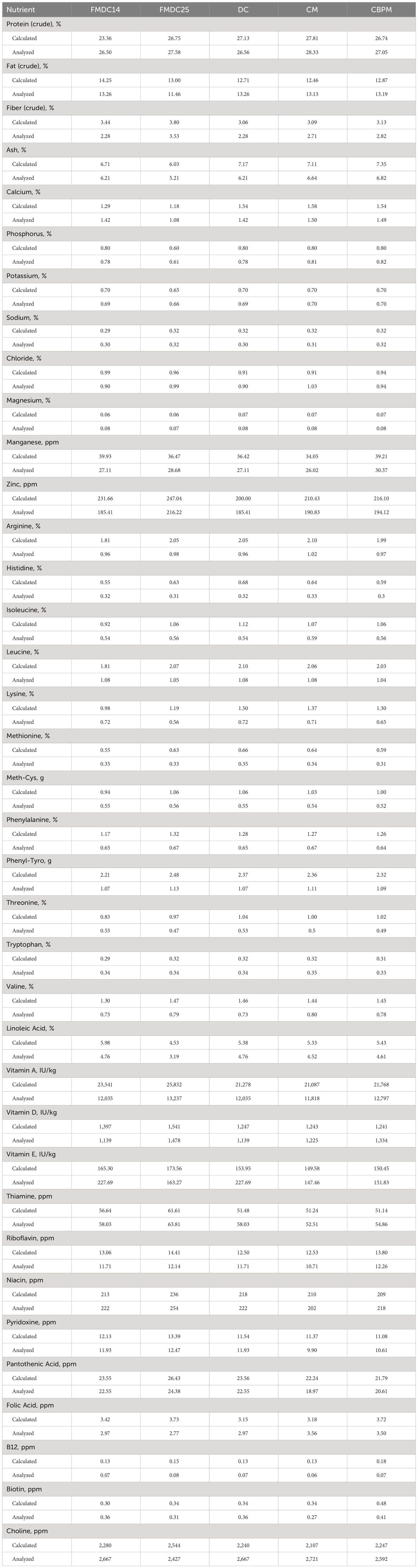
Table 5 Nutrient composition analysis (DMB): fresh mechanically deboned chicken 14% (FMDC14), fresh mechanically deboned chicken 25% (FMDC25), dried chicken (DC), chicken meal (CM), chicken by-product meal (CBPM).
By design, all the formulas were very similar with the primary difference being the different chicken sources. Processing parameters were kept as close as possible to maintain focus on the impact of protein sources on palatability. The impact of changing processing parameters could be a topic for future research.
Palatability results
For each palatability test, the CBPM formula was considered the test food (food A) and the DC, CM, FMDC14, and FMDC25 formulas were considered the control foods (food B). The palatability and first consumption results are concluded in Table 6. The CBPM formula was preferred (p<0.05) over the FMDC14 formula, FMDC25 formula, and the DC formula. The CBPM formula was a parity (p = 0.1427) with the CM formula. When evaluating which first bite of food was taken by each dog, there was a difference (p<0.05) between each palatability test: the CBPM versus the CM formula (32 vs. 18), the CBPM vs the DC formula (33 vs. 17), the CBPM vs the FMDC14 formula (41 vs. 9), and the CBPM vs the FMDC25 formula (39 vs. 11) over the two-day trial. The majority of the first bites from the dog panels were statistically significant in the CBPM formula (Table 6). It is uncertain if these results are related to aroma preference for the CBPM formula as it has been discussed that first consumption/approach responses are very subjective and are not the best indicators of palatability. In addition, these methods data can often be difficult to measure, and the repeatability of these measures is questionable (Griffin, 2003).
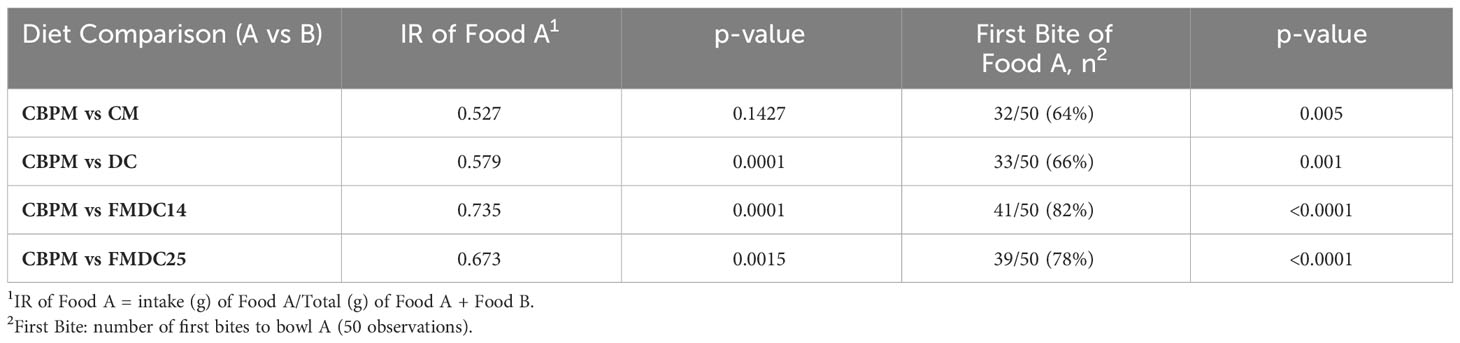
Table 6 The effect of experimental formulas on canine palatability: fresh mechanically deboned chicken 14% (FMDC14), fresh mechanically deboned chicken 25% (FMDC25), dried chicken (DC), chicken meal (CM), chicken by-product meal (CBPM).
Some of these palatability results were unexpected when compared to previous studies. According to (Li et al., 2017) dogs have a higher taste preference for fat, however in this study DC (12.86%) and FMDC14 (12.8%) had the highest fat levels but were less preferred compared to CBPM (11.23%). We also know that too much fat can lead to faster oxidation, giving a rancid smell that is unappealing to dogs (Hand et al., 2010). Since the products were produced on the same day, and stored in identical conditions, we believe oxidation is not at play, and that with the similar levels of fat, we are seeing the impact of the different protein sources.
According to Hand et al. (2010), dogs prefer consistent, larger kibbles. All formulas were similar in diameter size, apart from the FMDC25 formula that measured different (p<0.05). The smallest cut length kibble, the DC formula, may have influenced texture, as it measured the hardest (28.31 kg). The FMDC25 formula was the next hardest (27.49 kg) in texture, however this may be correlated to having a more “spongey” texture, as seen in Alvarenga et al. (2018), because it measured the highest in moisture (9.2%) and bulk density (23.44kg/m3). The FMDC25 formula also had the lowest starch content which may have led to these results. Results of this study may further indicate that minor differences in the kibble analysis may not have an impact on canine palatability. Previous research suggests that odors might be the primary drivers in a dogs’ food choice (Houpt et al., 1978; Houpt and Smith, 1981; Hall et al., 2017; Horowitz, 2017; Basque et al., 2019). A dog’s food selection is highly driven by smell based on the physiology of their highly developed nasal cavities. Houpt et al. (1978) found that when dogs were presented with a bland diet supplemented with a meat odor, they preferred it over a controlled diet with no odor. Manabe et al. (2010) determined that oils and short chain fatty acids are recognized in a special section of the olfactory bulb, and that this strong odor may be a driving factor behind a dog’s liking. Determining the sensory characteristics and the aromatic compounds within the five formulas may help better understand the preference from the palatability tests.
Conclusion
Testing of the four different chicken proteins presented challenges in keeping processing constant between formulas. Using a high amount of fresh mechanically deboned chicken proved to be difficult in forming a finished product at the target moisture and density while maintaining similar extrusion processing. Based on the palatability results from this study, the CBPM formula was preferred over the FMDC14, FMDC25, and DC formulas and was a palatability parity to the CM formula. The CM was most likely a parity to that of CBPM because the composition of the two raw materials are very similar. The definitive palatability results indicate that the minor differences in the kibble analysis may not have had an impact on canine palatability, and that the aroma from the different chicken protein sources did. It can be concluded that the CBPM formula in this study is highly palatable and in addition to being nutritionally beneficial, may provide increased food enjoyment by pets. Future studies should continue to analyze animal by-products as the need for more sustainable protein sources for the pet food industry are necessary.
Data availability statement
The original contributions presented in the study are included in the article/supplementary material. Further inquiries can be directed to the corresponding author.
Ethics statement
The animal study was approved by Institution Animal Care and Use Committee, Hill’s Pet Nutrition Center (Topeka, KS, USA) (Hill’s IACUC #CP26/permit number: 590). The study was conducted in accordance with the local legislation and institutional requirements.
Author contributions
CS: Writing – original draft, Writing – review & editing. NR: Writing – review & editing. CA: Writing – review & editing. MT: Writing – review & editing.
Funding
The author(s) declare financial support was received for the research, authorship, and/or publication of this article. Work was funded by and performed at the Pet Nutrition Center, Hill’s Pet Nutrition, Inc., Topeka, Kansas, USA.
Conflict of interest
Authors CS and NR are employed by Hill’s Pet Nutrition, Inc.
The remaining authors declare that the research was conducted in the absence of any commercial or financial relationships that could be construed as a potential conflict of interest.
The authors declare that this study received funding from Hill’s Pet Nutrition, Inc. The funder had the following involvement in the study: study design, collection, analysis, interpretation of data, the writing of this article, and the decision to submit it for publication.
Publisher’s note
All claims expressed in this article are solely those of the authors and do not necessarily represent those of their affiliated organizations, or those of the publisher, the editors and the reviewers. Any product that may be evaluated in this article, or claim that may be made by its manufacturer, is not guaranteed or endorsed by the publisher.
Abbreviations
FMDC, fresh mechanically deboned chicken; DC, dried chicken; CM, chicken meal; CBPM, chicken by-product meal; IR, intake ratio; AAFCO, Association of American Feed Control Officials; NIR, near-infrared spectroscopy.
References
AAFCO (2021). “Model regulations for pet food and specialty pet food under the model bill,”. Ed. Cook S. (Champaign, IL: Association of American Feed Controls Officials, Inc).
Aldrich G. C., Koppel K. (2015). Pet food palatability evaluation: A review of standard assay techniques and interpretation of results with a primary focus on limitations. Anim. (Basel) 5 (1), 43–55. doi: 10.3390/ani5010043
Alvarenga I. C., Ou Z., Thiele S., Alavi S., Aldrich C. G. (2018). Effects of milling sorghum into fractions on yield, nutrient composition, and their performance in extrusion of dog food. J. Cereal Sci. 82, 121–128. doi: 10.1016/j.jcs.2018.05.013
APPA (2021) Pet industry market size, trends & ownership statistics. American Pet Products Association, Inc. Available at: http://www.americanpetproducts.org/press_industrytrends.asp.
Basque C., Cambou S., Peron F., Le Paih L., Marzin C., Hanaoka K., et al. (2019). Food preference and olfactory discrimination tests: A complementary approach to understand the drivers of hedonic responses in dogs. J. Sensory Stud. 34 (2), e12483–e12n/a. doi: 10.1111/joss.12483
Callon M. C., Cargo-Froom C., DeVries T. J., Shoveller A. K. (2017). Canine food preference assessment of animal and vegetable ingredient-based diets using single-pan tests and behavioral observation. Front. Veterinary Sci. 4. doi: 10.3389/fvets.2017.00154
Dogan H., Kokini J. L. (2007). Psychophysical markers for crispness and influence of phase behavior and structure. J. Texture Stud. 38 (3), 324–354. doi: 10.1111/j.1745-4603.2007.00100.x
Donadelli R. A., Aldrich C. G., Jones C. K., Beyer R. S. (2019). The amino acid composition and protein quality of various egg, poultry meal by-products, and vegetable proteins used in the production of dog and cat diets. Poultry Sci. 98 (3), 1371–1378. doi: 10.3382/ps/pey462
Dunsford B., Plattner B., Greenbury D., Rokey G. (2002). The influence of extrusion processing on petfood palatability (Inc: Watt Publishing).
Félix A. P., Carvalho M. P., Alarça L. G., Brito C. B. M., Oliveira S. G., Maiorka A. (2012). Effects of the inclusion of carbohydrates and different soybean meals in the diet on palatability, digestibility and faecal characteristics in dogs. Anim. Feed Sci. Technol. 174 (3–4), 182–189. doi: 10.1016/j.anifeedsci.2012.03.013
Gooding C. H., Meeker D. L. (2016). Review: Comparison of 3 alternatives for large-scale processing of animal carcasses and meat by-products. Prof. Anim. Scientist 32 (3), 259–270. doi: 10.15232/pas.2015-01487
Hall N. J., Péron F., Cambou S., Callejon L., Wynne C. D. (2017). Food and food-odor preferences in dogs: A pilot study. Chem. Senses 42 (4), 361–370. doi: 10.1093/chemse/bjx016
Hall J. A., Vondran J. C., Vanchina M. A., Jewell D. E. (2018). When fed foods with similar palatability, healthy adult dogs and cats choose different macronutrient compositions. J. Exp. Biol. 221. doi: 10.1242/jeb.173450
Hand M. S., Thatcher C. D., Remillard R. L., Roudebush P., Novotny B. J. (2010). Small Animal Clinical Nutrition (5th edition.). Copyright 2010, 200, 1987, 1984, 1983. (Edison, New Jersey: The Mark Morris Institute)
Horowitz A. (2017). Smelling themselves: Dogs investigate their own odours longer when modified in an “olfactory mirror” test. Behav. Processes 143, 17–24. doi: 10.1016/j.beproc.2017.08.001
Houpt K. A., Hintz H. F., Shepherd P. (1978). The role of olfaction in canine food preferences. Chem. Senses 3 (3), 281–290. doi: 10.1093/chemse/3.3.281
Houpt K. A., Smith S. L. (1981). Taste preferences and their relation to obesity in dogs and cats. Can. Veterinary J. 22 (4), 77–85.
Knight R. (2020) USDA ERS - Livestock and Meat Domestic Data. Available at: https://www.ers.usda.gov/data-products/livestock-and-meat-domestic-data/.
Koppel K. (2014). Sensory analysis of pet foods: Sensory analysis of pet foods. J. Sci. Food Agric. 94 (11), 2148–2153. doi: 10.1002/jsfa.6597
Koppel K., Monti M., Gibson M., Alavi S., DonFrancesco B. D., Carciofi A. C. (2015). The effects of fiber inclusion on pet food sensory characteristics and palatability. Anim. (Basel) 5 (1), 110–125. doi: 10.3390/ani5010110
Laflamme D., Izquierdo O., Eirmann L., Binder S. (2014). Myths and misperceptions about ingredients used in commercial pet foods. Veterinary Clinics North America. Small Anim. Pract. 44 (4), 689–698. doi: 10.1016/j.cvsm.2014.03.002
Li H., Smith S., Aldrich G., Koppel K. (2017). Preference ranking procedure proposal for dogs: A preliminary study. J. Sensory Stud. 33 (1), e12307. doi: 10.1111/joss.12307
Manabe Y., Matsumura S., Fushiki T. (2010). “Preference for high-fat food in animals,” in Fat Detection: Taste, Texture, and Post Ingestive Effects. Eds. Montmayeur J.-P., le Coutre J. (Boca Raton, Florida: CRC Press/Taylor & Francis). Available at: http://www.ncbi.nlm.nih.gov/books/NBK53543/.
Manbeck A. E., Aldrich C. G., Alavi S., Zhou T., Donadelli R. A. (2017). The effect of gelatin inclusion in high protein extruded pet food on kibble physical properties. Anim. Feed Sci. Technol. 232, 91–101. doi: 10.1016/j.anifeedsci.2017.08.010
Meineri G., Candellone A., Tassone S., Peiretti P. G., Longato E., Pattono D., et al. (2021). Effects of “fresh mechanically deboned meat” inclusion on nutritional value, palatability, shelf-life microbiological risk and digestibility in dry dog food. PloS One 16 (4), e0250351–e0250351. doi: 10.1371/journal.pone.0250351
Montelongo L. J., Pope B. K., Martinez S. B. (2012). Pet Food Compositions Having Antimicrobial Activity (Patent No. WO 2012/018913 A1). (Alexandria, Virginia: The United States Patent and Trademark Office (USPTO)).
Murray S. M., Patil A. R., Fahey G. C., Merchen N. R., Hughes D. M. (1997). Raw and rendered animal by-products as ingredients in dog diets. J. Nutr. 128 (12), 2812S–2815S. doi: 10.1093/jn/128.12.2812S
Neufield K. (2012). Synergies of different flavours for dog and cat food: Olfactory sense better developed. Kraftfutter 3–4, 15–18.
Nijdam D., Rood T., Westhoek H. (2012). The price of protein: Review of land use and carbon footprints from life cycle assessments of animal food products and their substitutes. Food Policy 37 (6), 760–770. doi: 10.1016/j.foodpol.2012.08.002
Okin G. S. (2017). Environmental impacts of food consumption by dogs and cats. PloS One 12 (8), e0181301–e0181301. doi: 10.1371/journal.pone.0181301
Samant S. S., Crandall P. G., Jarma Arroyo S. E., Seo H.-S. (2021). Dry pet food flavor enhancers and their impact on palatability: A review. Foods 10 (11), 2599. doi: 10.3390/foods10112599
Smith S. C. (2018). Next-generation distillers dried grain as a potential dietary ingredient in dog and cat diets (Manhattan, Kansas: Master's Thesis) Kansas State University).
Sprinkle D. (2021) PetfoodIndustry.com. US pet industry reset: Pet spending during COVID-19. Available at: https://www.petfoodindustry.com/articles/10186-us-pet-industry-reset-pet-spending-during-covid-19.
Tsai W.-L. (2019). Understanding pet food consumers with various sensory analysis methods. (Master's Thesis) (Manhattan, Kansas: Kansas State University).
Vondran J. C. (2013). A two-pan feeding trial with companion dogs considerations for future testing. (Master's Thesis) (Manhattan, Kansas: Kansas State University).
Keywords: canine, chicken by-product meal, chicken protein, dog food, palatability
Citation: Shields CJ, Rozzi NL, Aldrich CG and Talavera MJ (2023) Effects of different chicken protein sources on palatability in dry adult dog food. Front. Anim. Sci. 4:1292658. doi: 10.3389/fanim.2023.1292658
Received: 11 September 2023; Accepted: 02 November 2023;
Published: 24 November 2023.
Edited by:
Luciano Pinotti, University of Milan, ItalyReviewed by:
Attawit Kovitvadhi, Kasetsart University, ThailandApolônio Gomes Ribeiro, Federal University of Paraíba, Brazil
Copyright © 2023 Shields, Rozzi, Aldrich and Talavera. This is an open-access article distributed under the terms of the Creative Commons Attribution License (CC BY). The use, distribution or reproduction in other forums is permitted, provided the original author(s) and the copyright owner(s) are credited and that the original publication in this journal is cited, in accordance with accepted academic practice. No use, distribution or reproduction is permitted which does not comply with these terms.
*Correspondence: Martin J. Talavera, dGFsYXZlcmFAa3N1LmVkdQ==