- 1Department of Biological Sciences, University of Cape Town, Cape Town, South Africa
- 2Biodiversity and Development Institute, Cape Town, South Africa
- 3University of the Third Age (U3A) Stilbaai Bird Group, Stilbaai, South Africa
Citizen science data are rapidly transforming the conservation landscape. Targeted participatory citizen science initiatives generate nuanced data capable of monitoring trends in populations and generating early warnings for species and habitats experiencing significant declines. In the Hessequa Atlasing Area, Western Cape, South Africa, citizen science 'atlasers' involved with the Second Southern African Bird Atlas Project (SABAP2) have worked with scientific leadership since 2014 to improve the quality of bird atlas data in their region for species monitoring. In this study, we used reporting rates from SABAP2 checklists in the Hessequa Atlasing Area to calculate changes in range size and relative abundance for the 165 most commonly reported species in the region. We used a seven-tier alert system and broad habitat categories to sort species by priority for conservation action. Our results showed that wetland and marine associated species are experiencing the greatest declines in range and relative abundance in the Hessequa Atlasing Area, whilst urban and grassland associated species are largely increasing. We discuss how observed changes in populations may be used to guide conservation action and provide recommendations for scientists and non-scientific community members on engaging with and responding to the changes highlighted in each of the seven alert levels provided.
1 Introduction
Conservation is facing a ‘wicked’ problem (Sharman and Mlambo, 2012). Biodiversity loss, the extinction of species and ecological interactions, threatens the integrity and functioning of populations and ecosystems, both human and non-human, globally (Díaz et al., 2019; Hochkirch et al., 2021; CBD, 2022; Isbell et al., 2023). Conservation scientists are asked to advise and implement strategies to mitigate losses, but are faced with data gaps and biases that impede effective action (Jetz et al., 2011; Chambers et al., 2016; Parsons, 2016; Proença et al., 2017). Recent research has called for improved monitoring efforts to address data gaps, prioritise focus, and strengthen conservation measures (Proença et al., 2017; Siddig, 2019); a potential solution may lie in the field of citizen science. Through collecting large quantities of species data at vast geographic scales and often from under-sampled regions, citizen scientists may contribute towards the population monitoring needed to mitigate the current biodiversity crisis.
Crucially, citizen science data may support the development of early warning systems. These systems are often constructed with the goal of disaster risk management, i.e., floods, landslides, or drought; however, in the long term, biodiversity loss may be considered a disaster of equal if not greater magnitude than any natural disaster (Barnard et al., 2017). In the context of species conservation, early warning systems may be broadly described as monitoring schema implemented with the goal of detecting significant changes among species, populations, and habitats (Jetz et al., 2019). In conservation, warning systems are already widely utilised; however, many of these are retrospective rather than prospective (Schmeller et al. (2018). For instance, the International Union for Conservation of Nature (IUCN) Red List of Threatened Species (2022) has been used internationally to raise awareness, prioritise conservation intervention, incentivise funding, and inform management and policy decisions for over 50 years (Betts et al., 2020). Although crucial, IUCN assessments occur at long intervals, and are thus likely to detect patterns late rather than early, because assessments are based on changes that have already occurred. These classifications also tend to bias interventions towards rare species and those with a high immediate extinction risk (Luther et al., 2016; Baker et al., 2018; Christie et al., 2020), potentially missing common species and species experiencing moderate to slow declines, and overlooking the importance of preventing common species from becoming rare (e.g. Dirzo et al., 2014; Ceballos et al., 2017; Weeks et al., 2022). The value of an early warning system lies in its ability to inform proactive rather than remedial conservation measures, enabling thorough evaluation and strategic response instead of rapid and reactive short-term solutions (Luther et al., 2016; Schmeller et al., 2018). Schmeller et al. (2018) identify eight biodiversity variables essential to early detection which include, among others, abundance, ecosystem heterogeneity, and range dynamics. Some recently trialled systems have focused on early detection by monitoring specific biomes, i.e. tropical rainforests (Rovero and Ahumada, 2017), or implementing warning systems for low genetic diversity among indicator species in threatened habitats (Zimmerman et al., 2022), but for many taxa, particularly in the Global South, these baseline data are either scarce (Boakes et al., 2010; Feeley et al., 2016; Hoveka et al., 2020; Oliver et al., 2021; von der Heyden, 2022), or are collected and housed by non-local institutions in first-world nations (Cresswell, 2018; Stephenson and Stengel, 2020; Asase et al., 2021; de Vos, 2022; Miller et al., 2023). Citizen science offers an opportunity to collect and interpret baseline data at a regional scale, and thus is gaining traction as a facilitator of localised monitoring systems in the Global South (Gossa et al., 2015; State of India’s Birds [SoIB], 2020; Asase et al., 2021; Cyvin, 2022).
To date, citizen science project data have supported early warning systems for several taxa internationally, including butterflies (Stenoien et al., 2018), plants (García et al., 2021), fish (Poursanidis and Zenetos, 2013; Giovos et al., 2019), and microalgae (Hardison et al., 2019). The involvement of citizen scientists in maintaining these systems has led to the development of a new term, the participatory early warning system (e.g. Marchezini et al., 2018), referring to ground-level engagement of community members in collecting data that ultimately contribute towards the wellbeing of their immediate surroundings. In 2005, The United Nations together with the World Conference on Disaster Reduction adopted the “Hyogo Framework for Action 2005-2015: Building the Resilience of Nations and Communities to Disasters” as a set of strategic guidelines for detecting and mitigating the impacts of natural disasters. The framework emphasised the importance of “early warning systems that are people centred, in particular systems whose warnings are timely and understandable to those at risk (…) including guidance on how to act upon warnings” (UNISDR, 2005, para. 17, ii.d.9). In addition to engaging the general public in conservation, participatory systems also support a decentralised conservation narrative by encouraging collaboration, data collection, and communication with and within local scientific communities (Asase et al., 2021).
While participatory early warning systems do carry important social significance, to be effective as warning tools, data collection must be rigorous enough to meet both scientific standards and established monitoring targets. Within southern Africa, multiple citizen science projects already contribute to conservation decision-making (Barnes, 1998; Barnes, 2000; Robertson et al., 2010; Barnard et al., 2017); still, the role of citizen science data in informing early warning systems in southern Africa remains largely unexplored within scientific literature (Chambers et al., 2016). Several projects hold potential to strike the necessary balance between supporting a participatory early warning system and collecting meaningful data, and perhaps the strongest candidate is the Second Southern African Bird Atlas Project (SABAP2), a long-term citizen science initiative launched in 2007. Crucially from a data science perspective, SABAP2 offers a historically robust and consistently updated dataset for analysis (Underhill et al., 2017; Lee et al., 2022).
Although the quality of citizen science data is often called into question (e.g. Bird et al., 2014; Kamp et al., 2016; Johnston et al., 2021), the SABAP2 protocol is designed to mitigate the effects of spatial and observer bias by restricting participation to skilled birders and using gamification to encourage participants to both atlas in their ‘home’ region and visit regions with poor coverage (Ainsley and Underhill, 2017; Underhill et al., 2017; Brown and Williams, 2019; Daniel and Underhill, 2023). As noted by Johnston et al. (2021), the statistical strength of citizen science data is improved by using comprehensive checklists which require participants to list every species they encounter. SABAP2 employs a strict data collection protocol in which observers work in grid cells of 5×5 minutes latitude by longitude (pentads), spending two or more hours visiting as many habitat types as possible in the pentad and compiling a complete species checklist, thus enabling scientists to infer the pseudo-absence of non-detected species. The temporal dimensions of SABAP2 data quality have also been tested; coordinated systematic atlasing efforts have been demonstrated to provide nuanced and up-to-date representations of species presence (Daniel and Underhill, 2023).
The quality of SABAP2 data has been substantiated, yet it remains to be seen how these data may inform conservation action at the species level. Can SABAP2 data generate meaningful early warnings for populations, and if so, how might they then facilitate intervention? Trends in SABAP2 data are readily detected via reporting rates: the proportion of checklists on which a species is recorded in a grid cell (Harrison and Underhill, 1997). Although some seasonal and interannual variation of reporting rates is expected, sharp or continued increase or decline may indicate an underlying problem. Identifying potential aberrances early through monitoring allows scientists and policy makers to carefully assess the situation, taking into consideration any environmental or anthropogenic changes which may correspond with or contribute to the population change, and act accordingly.
In this study, we tested the ability of systematically collected SABAP2 data from the Hessequa Atlasing Area, South Africa, to detect significant trends in populations of 165 locally common avian species. Using changes in range and relative abundance calculated from reporting rates, we sorted species into seven alert categories for early warnings, and searched for patterns between alert categories and species habitat preferences. We discuss the broader significance of the patterns we detected, including how warnings for these species may be utilised to trigger effective response. Finally, we discuss the broader social impacts of implementing a participatory warning system, and provide recommendations for employing citizen science projects as conservation tools for generating early warnings and enabling thoughtful social engagement.
2 Methods
Generating citizen science data for modelling often requires compromising data quality (i.e. temporal proximity, observer expertise, standardised protocol) in favour of quantity (Isaac et al., 2014; Daniel and Underhill, 2023). Ensuring that species records are current, accurate, and collected systematically is more time-intensive and requires more specialised skills than opportunistic data collection (Boersch-Supan et al., 2019). SABAP2 was designed jointly by a team of biologists and statisticians to maximise both data quantity and quality, implementing a strict protocol to improve data integrity whilst operating at a geographic scale which feasibly allows for complete regional coverage by local citizen science participants (Underhill et al., 2017). We used reporting rates from SABAP2 checklists collected as part of a trialled monitoring scheme to calculate changes in the range and relative abundance of species in the Hessequa Atlasing Area, our region of interest.
2.1 Reporting rates as a monitoring tool
Detecting trends in species populations requires a tool sensitive enough to detect subtle shifts, and blunt enough to characterise large changes. For SABAP2 data, reporting rates offer a suitable starting point. The reporting rate for a species is defined as the proportion of checklists on which it has been recorded; the concept dates back to Linsdale (1928), who intuited that reporting rate would provide an index of abundance. Following Linsdale’s work, the next important quantitative development was made by Temple and Temple (1984, 1986), who showed that where both count data and reporting rates were available, these two measures were closely correlated using Spearman’s rank correlation coefficient. The use of this measure of correlation demonstrates an understanding of the reality that reporting rates are monotonically related to abundance, and the relationship is not linear (Underhill et al., 1992). This concept was further advanced by Griffioen (2001), who recognised that mathematical ecology developed by Nachman (1981) could be applied to the relationship between abundance, denoted by N , and reporting rate R . Using data originating in the Australian Bird Count project (Ambrose, 1991), he demonstrated that the relationship between the log(N) and log(−log(1−R)) was linear. The theoretical implications of Griffioen’s (2001) results are explored in Underhill (submitted).
Although straightforward, the use of reporting rates to measure population trends is not without risk. Potential biases inherent within the data invariably challenge the validity of results. SABAP2 was preceded by another atlas project, SABAP1, with a more relaxed protocol and coarser spatial and temporal resolutions (Underhill et al., 1992; Harrison et al., 1997). While reporting rates from SABAP1 did generally reflect estimates of actual abundance, inconsistencies in sampling effort were an accompanying caveat to utilising the data in this way (Robertson et al., 1995). The problem of observer effort remains a contemporary concern in any atlas data analysis (e.g. Szabo et al., 2010; Huntley et al., 2012; Szabo et al., 2012), along with seasonal and behavioural variations in the conspicuousness of a species and potential misidentifications by observers (Harrison and Underhill, 1997).
Robertson et al. (2010) list seven qualities which should ideally characterise a data-strong atlas project: A spatial scale appropriate to the study taxon; the highest possible spatial and temporal resolution; the highest possible taxonomic resolution (e.g. species level identifications rather than family level); the highest possible demographic resolution (e.g. inclusive of life stage and age information); a standardised protocol with a reliable indication of sampling effort; a well-described sampling protocol; and the greatest possible number of unique sampling units (e.g. pentads). Several of these components were already included in SABAP1, and either carried over or were introduced into SABAP2. The spatial resolution of the project was increased from quarter-degree units of 15×15 minutes latitude by longitude to the pentad (5×5 minutes latitude by longitude), and the temporal resolution increased from a monthly to a five-day interval. By introducing a minimum 2-hour atlasing protocol, SABAP2 reduced the uncertainty surrounding sampling effort. To minimise the chances of missing inconspicuous or seasonally gregarious species, a dimension of thoroughness was added to the sampling protocol by requiring ‘atlasers’ to visit as many habitat types as possible within each pentad. Finally, to address the problem of misidentification, new SABAP2 checklists are vetted automatically against existing records for the region, and panels of regional identification experts are tasked with reviewing incoming checklists and querying unusual records (Brooks et al., 2022). While the precautions built into SABAP2 certainly do not eliminate bias, they are designed to minimise its presence in checklists; thus, SABAP2 reporting rates can be considered a reasonably robust metric for monitoring avian population trends (e.g. Lee et al., 2017).
2.2 The Hessequa Atlasing Area and monitoring effort
Although SABAP2 data quality is strong overall, its weakness is temporal quality. Data are not collected at the regular intervals necessary for biodiversity monitoring. This is true of many large-scale citizen science initiatives (e.g. Kelling et al., 2015); checklists remain opportunistic, meaning there is no guarantee that a pentad will be atlased with any regularity. This challenges the utility of SABAP2 as a monitoring tool, since old checklists for a pentad may not provide accurate representations of the species currently within the pentad, and inconsistent sampling diminishes the ability to detect reliable population trends for individual species (Daniel and Underhill, 2023). In 2014, citizen science participants in the Hessequa Atlasing Area, a region within the Western Cape, South Africa, collaborated with leading scientists to implement a seasonal data collection strategy, ongoing as of January 2023 (van Rooyen, 2018; van Rooyen and Underhill, 2020). Collection efforts were designed with the intention of generating data of sufficient temporal quality for use in monitoring; three complete monitoring cycles (2014–2017, 2018–2019 and 2020–2021) were available for detecting trends in regional populations at the time of this study.
The Hessequa Atlasing Area (Hessequa) is a region comprising 75 pentads within the Western Cape, South Africa. The region lies in the Hessequa Municipality and is bordered to the north and south respectively by the Langeberg Mountains and the sea. Although Hessequa contains some patches of natural vegetation, particularly in the mountains and along the coast, most of the region is agriculturally transformed with land used for both livestock farming (cattle and sheep) and crop production (barley, canola and wheat) (van Rooyen and Underhill, 2020).
In 2014, atlasers in Hessequa joined forces with scientific leadership at the University of Cape Town to launch a regional-scale monitoring project. From 2014 until December 2017, each pentad in Hessequa was atlased at least once per year, and twice per year in 2016 and 2017. From 2017 onwards, the project ran in two-year cycles, dividing Hessequa into a chessboard pattern for the four austral seasons (van Rooyen, 2018; van Rooyen and Underhill, 2020; Daniel and Underhill, 2023). Over each subsequent two-year cycle, half of the pentads in the region were visited for fieldwork in summer and winter during year one, and autumn and spring during year two. The remaining pentads received fieldwork in autumn and spring during year one, and summer and winter during year two. Thus, following a standard two-year cycle, every pentad in Hessequa was visited at least once in each season. Fieldwork was ongoing as of December 2022.
2.3 Generating rapid alerts using systematic bird atlas data
When considering how to use reporting rates in generating early warning systems, it is convenient to classify species of interest into alert categories based on one or more criteria (i.e. increasing/decreasing range, distribution, abundance) to aid in the communication of results. At the regional and national level, these categorisations also serve as valuable tools for comparison against larger international classification schema such as the IUCN Red List (2022), providing a deeper understanding of the status of species within a geographic region (e.g. Jiang et al., 2020). The criteria, however, are not hard categories; they fall along a continuum, and the boundaries for categories can be adjusted. In this study, we use seven categories (Table 1): large decrease in range (red); large decrease in relative abundance (amber); moderate decrease in relative abundance (yellow); stable relative abundance (green); moderate to large increase in relative abundance of ecologically neutral or positive species (blue); moderate to large increase in either range or relative abundance of ecologically negative species (e.g. alien species and agricultural pests) (purple); and moderate to large increase in range of ecologically neutral or positive species (pink). The final two categories were included for contextual relevance. Many pentads in Hessequa encompass privately owned farmland, and because our aim was to generate practical recommendations for the region, we elected to distinguish species which may be of particular relevance to local landowners.
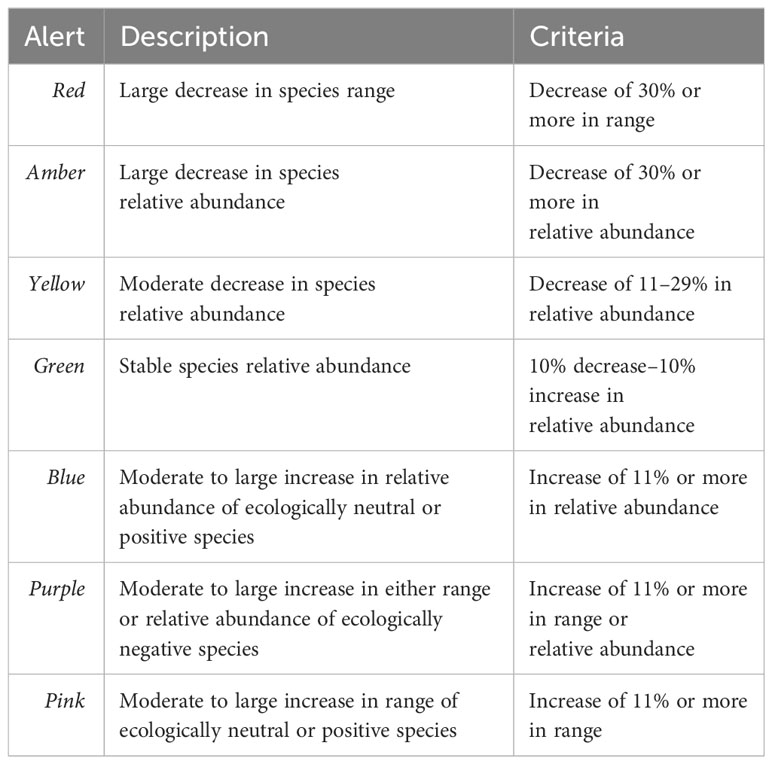
Table 1 Seven alert level categories for species populations based on changes in range and relative abundance.
2.4 Selecting species of interest and characterising changes
In the interest of providing actionable information, we focused on species for which mitigation measures could realistically be achieved in the region (i.e. the most commonly occurring species). Thus, rare species (species with low reporting rates) are excluded from this analysis: we chose a cut-off reporting rate of 5%. This reporting rate was calculated over the Hessequa Atlasing Area for the entire study period 2014–2021. Although 5% may well represent an overly inclusive low limit, we chose to err on the side of caution and therefore anticipate that some species included in this analysis will be evaluated as “too rare” in the study area to warrant an alert, or for subsequent intervention to be meaningful.
Having selected the species of interest, we calculated the two main criteria needed to classify species into categories: Change in range, and change in relative abundance. For change in range, we calculated the numbers of pentads occupied by each species in the three time periods 2014−2017, 2018–2019 and 2020–2021. For the sake of brevity, in this paper, we only present results based on a comparison of ratios between the first and third time periods (2014–2017 and 2020–2021). We could have also compared the first to second or second to third time period reporting rates, and indeed, in a workshop regarding mitigative actions in response to atlas data, these results would be considered. As the period for which data from systematic atlasing gets longer, it will be feasible to examine trends and evaluate which species are characterised by large increases and decreases in abundance (i.e. the species which are prone to irruptions) and for which short term changes in reporting rates represent statistical ‘noise’ and not ‘signal.’ Given the relatively short study period, we did not a priori expect many, or even any, species to display large changes in range size; rather, we included this category for the sake of completeness. Decreases in range size are widely considered positive correlates of increased extinction risk for a species (Mace et al., 2008; Gaston and Fuller, 2009; Lee and Jetz, 2010; Staude et al., 2019), though only one among a complex suite of species-specific contributors (Orme et al., 2006; Cardillo et al., 2008; Chichorro et al., 2019; Hernández-Yáñez et al., 2022). A few studies have examined the relationship between range decrease and species declines among birds (e.g. Lee and Jetz, 2010); however, birds constitute a challenging taxon since many species are migratory, nomadic, or semi-nomadic in response to weather events (Runge et al., 2015). While a significant and persistent change in range may indeed reflect, for instance, species decline, slight and variable changes cannot be considered reliable indicators of population trends. In these cases, a more sensitive tool such as relative abundance is often used as a complementary metric to better characterise population trends (Lawton, 1993; McGill and Collins, 2003; Huntley et al., 2012).
For species which showed negligible to moderate changes in range (0–29% increase or decrease), we estimated changes in relative abundance. To determine changes in relative abundance, we found the set of pentads in which each selected species had been recorded during the study period, i.e. the range of the species within the Hessequa Atlasing Area. For each of these pentads, we calculated C, the change in relative abundance, as described by Underhill (submitted).
where R1 is the reporting rate for the species in the grid cell for the first period of interest, and R2 is the reporting rate for the second period. We calculated the value of C for each pentad in the range, and summarised the value by calculating the median. To avoid a logarithm of zero, we changed the value of reporting rates of 0 and 1 to 0.01 and 0.99 respectively (because the summary statistic is the median, this does not impact results).
Finally, we classified each of the species of interest by habitat association, adapting the broad categories described by Chittenden et al. (2018). Species were categorised based on habitat preferences as Marine, Wetland, Thicket, Forest, Grassland, Fynbos, Urban, Montane, or Generalist. Species associated with more than one habitat were counted in all relevant habitat types; for example, Pied Kingfisher was counted as both a Wetland and a Marine species. Habitat categories were kept broad to encompass a variety of habitat sub-types. Thus, Marine included coastal habitat, Grassland included agricultural fields and Urban included suburban areas and gardens.
3 Results
A total of 1,951 checklists were submitted to the SABAP2 project for the Hessequa Atlasing Area between 2014 and 2021, containing a total of 109,273 records of species distribution. They represented a total of 323 species, of which 165 had reporting rates exceeding 5% for the time period.
3.1 Species experiencing greatest declines: Red, Amber and Yellow Alerts
Of the 165 species with reporting rates exceeding 5%, 23 species showed range declines exceeding 30%, and were thus categorised as Red Alerts (Table 2). Among these, six species showed range declines exceeding 50%, and four of these were Wetland associated.

Table 2 Range and relative abundance changes for the 165 species in the Hessequa Atlasing Area with reporting rates exceeding 5%.
Of the 132 species whose changes in range were moderate to negligible, 29 species showed decreases in relative abundance exceeding 30% (Table 2); these were assigned an Amber Alert. 26 species showed decreases in relative abundance between 11–29%, and were assigned a Yellow Alert.
By habitat preference, most species in the Red and Amber Alert categories were Grassland, Urban, and Wetland associated. However, these categories also contained the largest proportion of Marine (17 species) and Wetland (51) species. Of the 23 Red alert species, 14 were associated with Wetlands, five with Marine, and five with Forest habitat (Table 2; Figure 1). Wetland associated species were also the most prevalent in the Amber Alert category (15 species), closely followed by 11 Grassland species. Yellow Alert species were predominantly Grassland and Fynbos associated, followed by Wetland.
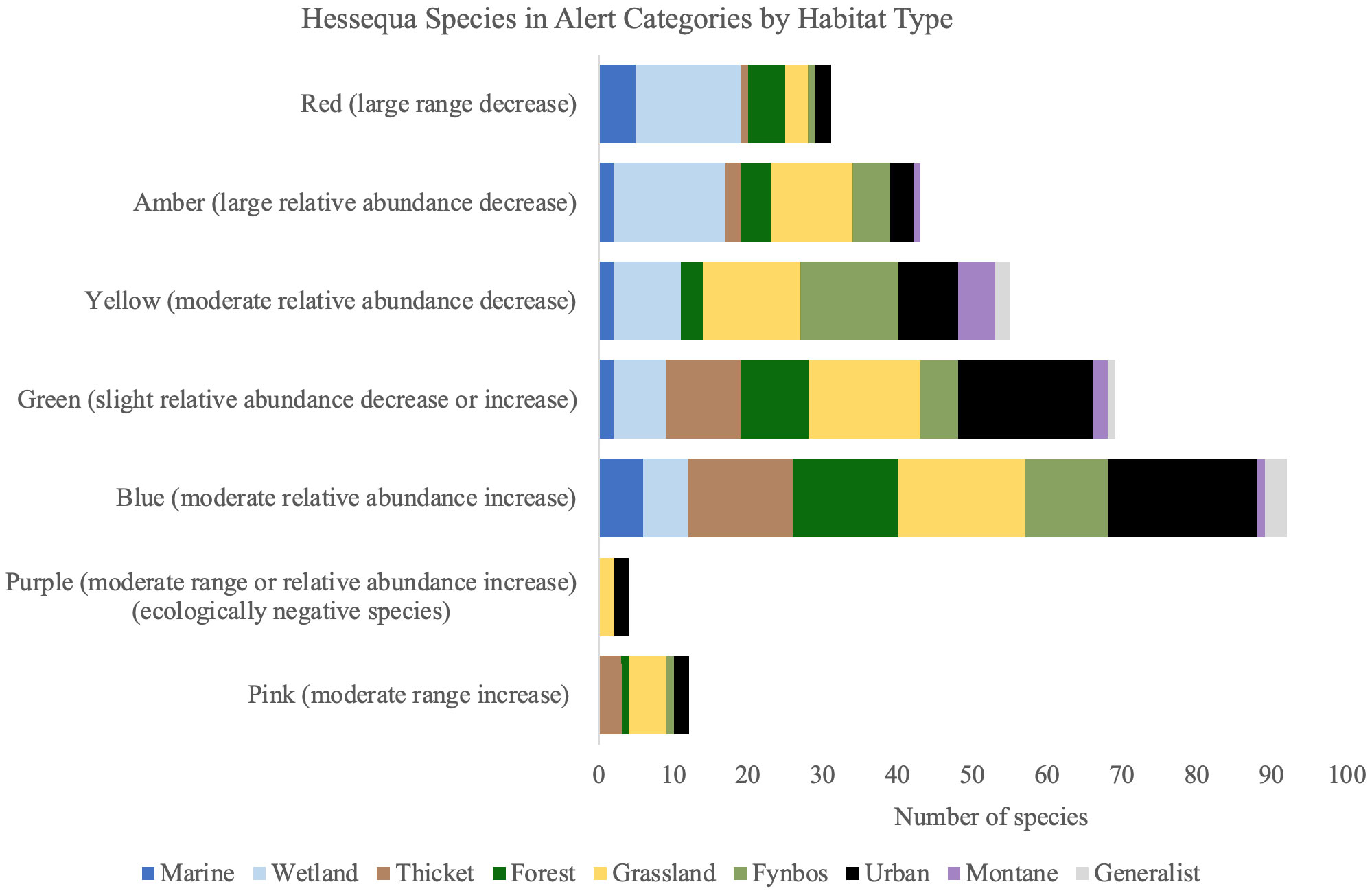
Figure 1 Habitat associations for 165 bird species in the Hessequa Atlasing Area, sorted into seven alert categories. Note the greatest concentration of species are those showing stable or slightly increasing populations, and populations decreasing in range and relative abundance are predominantly Wetland, Marine, and Grassland associated.
3.2 Stable and increasing species
77 species were categorised as either Green (30 species) or Blue (47) Alert levels. The most common habitat type among both Green and Blue Alert species was Urban, followed by Grassland; for Blue Alert species, these were closely followed by Thicket and Forest (Figure 1). Three potentially ecologically negative species were categorised as Purple Alerts, with increases in relative abundance exceeding 30%. All three Purple Alert species were associated with Urban and Grassland habitats (Table 2; Figure 1). Seven ecologically neutral or positive species were categorised as Pink Alerts, with range increases exceeding 11%. Among these, five species were Grassland associated, followed by a mix of Thicket, Fynbos, and Urban associated species (Table 2; Figure 1).
4 Discussion
4.1 Species statuses in a global context
Species categorised under Red and Amber Alerts may reasonably be considered the most vulnerable, and thus most in need of rapid intervention. The declines in observed range far exceeded our initial expectations; we anticipated range decreases to be largely insignificant, and that the more sensitive relative abundance metric would be necessary to categorise trends for most species. That six species showed declines exceeding 50% was an unexpected and sobering result. Our findings uncovered two realities: First, species ranges are undergoing rapid and drastic changes at a local level in Hessequa, and second, consistent citizen science monitoring efforts are capable of detecting these changes.
19 of the 23 species experiencing greatest declines in the Red Alert category were Wetland or Marine associated. This pattern carried over into the Amber category, where the relative abundance of Wetland species decreased by as much as 62%, as in the case of the Red-knobbed Coot (F. cristata). These declines are consistent with global predictions and parallel international studies; wetlands are diminishing worldwide (Maclean et al., 2011; Prigent et al., 2012), and despite some increases in populations of wetland birds in Europe and North America (Gaget et al., 2018; Rosenberg et al., 2019; Kamp et al., 2020; Lee et al., 2022), these localised increases are not necessarily reflected elsewhere (e.g. Maclean et al., 2011; SoIB, 2020).
Species in the Yellow Alert category can be considered at-risk; these are species to watch carefully. 26 species in the Hessequa Atlasing Area fell into this category, comprising 16% of the species included in the study. Species in the Yellow category were distributed evenly across habitat types, with the strongest concentrations in Grassland, Fynbos, and Wetland habitats. These concentrations were unsurprising; a growing body of research has identified Grassland birds as among the most vulnerable groups globally (Lee et al., 2017; Correll et al., 2019; Rosenberg et al., 2019; Vaccaro et al., 2019; Marques et al., 2020; Burns et al., 2021), with distinctions between species dependent on native grassland and those able to utilise transformed grassland habitats such as agricultural fields and cattle pasture. We observed the greatest declines in Grassland species which were dependent either exclusively on natural Grasslands or on a mixture of Grassland and a second non-Urban habitat such as Fynbos or Montane. Again, this result was unsurprising; habitat specialists are more broadly threatened by habitat conversion than generalists (Clavel et al., 2011; Le Viol et al., 2012; Sweeney and Jarzyna, 2022). However, for those species able to utilise converted land, no single type of agricultural transformation appears to be conclusively “better” for avian diversity—factors supporting the greatest diversity of Grassland species vary significantly by context, rendering blanket recommendations inadequate (e.g. Batáry et al., 2006; Gil-Tena et al., 2015; Vaccaro et al., 2019; Sasaki et al., 2020). Thus, any conservation measures on behalf of local Grassland species must take local landscape into consideration.
Green and Blue Alert species comprised roughly 47% of the species included in our analysis. This is an encouraging statistic, suggesting that for nearly half of the most common species in Hessequa, populations are either stable or moderately increasing. Green and Blue categories offer insights into the factors supporting successful populations; for instance, Urban, Grassland (transformed), and Thicket associated species were more prevalent in these two categories than any other, suggesting that these habitat types may be expanding, well-managed, or alternatively are suitable as secondary habitat choices for several species. However, more targeted examination is needed to determine the underlying drivers of increasing populations, and to determine whether any of these may be linked to corresponding population declines among Red, Amber, or Yellow Alert species. For instance, some Thicket species may be increasing due to the establishment of invasive Acacia thickets (Rogers and Chown, 2013); such novel ecosystems invariably produce mixed conservation outcomes, highlighting the importance of monitoring at both the species and landscape level in parallel. Finally, Blue and Green Alert categories also included species classified as Near Threatened by BirdLife: Agulhas Long-billed Lark (C. brevirostris), Knysna Woodpecker (C. notata), and South Africa’s national bird, the Blue Crane (A. paradiseus), all of which showed increases in relative abundance ranging from 16 to 30% in Hessequa. It is important to note, however, that regional increases do not necessarily imply species-wide increases; this concept is explored later in this paper.
Three species were categorised as both ecologically negative and showing a large increase in range or relative abundance. Note that “negative” does not necessarily denote “harmful;” introduced and alien invasive species were categorised as ecologically negative regardless of the magnitude of threat posed to local ecosystems. Two of the three species identified, House Sparrow (P. domesticus) and Common Starling (S. vulgaris), are non-native to South Africa. The third, Red-billed Quelea (Q. quelea), is a native species that has expanded its range southwards into the Western Cape (Oschadleus and Underhill, 2006), and is widely considered an agricultural pest, responsible for economically significant grain crop damage (Berruti, 2000; Oduntan et al., 2015).
Though introduced species are a topic of contention in ecology, the implications of expanding House Sparrow and Common Starling populations in southern Africa are little known. House Sparrows were first introduced to southern Africa in the 1900s and spread rapidly between human settlements (Msimanga, 2001). Although the species is considered invasive, current knowledge of any potentially negative impacts of House Sparrows in South Africa is limited; a study in KwaZulu-Natal showed that local populations were concentrated in heavily transformed urban environments (e.g. shopping centres and industrial areas), and thus may not compete with or threaten native species (Magudu and Downs, 2015). Like House Sparrows, Common Starlings were introduced; 18 birds were brought to Cape Town in 1897 by Cecil Rhodes, and within 50 years, were abundant across many parts of the Western Cape (Winterbottom and Liversidge, 1954). Although they are often found in dense aggregations, some evidence indicates that populations of Common Starlings in South Africa do not necessarily persist within a region once established (Ivanova and Symes, 2018); thus, like House Sparrows, they may not pose serious threat to native avian species.
As an agricultural pest species, Red-billed Quelea pose a social-ecological challenge, and we observed a range increase of 14% and an estimated increase in relative abundance of 102% in Hessequa. Although these numbers certainly seem to indicate a rapidly growing population, there are important details to note regarding the species’ biology and phenology. Red-billed Queleas are nomadic and migratory across their range in response to rainfall events (Elliott, 1990; Oschadleus, 2000; Dallimer and Jones, 2002) and require a specific suite of environmental conditions to establish breeding colonies (Cheke et al., 2007). Climate patterns in the southern Western Cape are highly variable from year to year and are further complexified by the presence of microclimates (Ward et al., 2021). During our seven-year study period, Hessequa’s erratic climate may have provided suitable quelea conditions over several consecutive years, with no guarantee of the conditions persisting. Alternatively, microclimates may create enough variation within Hessequa to support quelea populations year-round; this is not far-fetched, as Red-billed Quelea have been observed breeding in the Western Cape (Oschadleus, 2015). Once again, our findings showcase the value of local monitoring. Paired with climate variables and predictive models, data like those from Hessequa could inform farmers across the Red-billed Quelea's range regarding when and where populations are most likely to arrive or persist, potentially preventing large-scale crop damage.
4.2 How can an alert categories inform action?
Population change does not occur in isolation. Shifts in range and relative abundance are inextricably linked to landscape ecology, climate, and anthropogenic activity. In general, the changes we observed followed a broad trajectory, with Wetland and Marine species experiencing the greatest declines, followed by Grassland and Fynbos species with moderate declines to moderate increases, and finally Urban, (agricultural) Grassland, Forest, and Thicket species remaining stable or showing significant increases. These habitat-level observations bring opportunities to explore the nuances in observed trends: Why did some Grassland species increase and others decrease? Are decreasing species habitat or dietary specialists, and are increasing species able to utilise multiple habitat and food types? Such questions lead to a deeper understanding of the niches which species occupy within a habitat, which in turn enhances our ability to design meaningful interventions.
Perhaps the best primary application for Red and Amber (or indeed any) species lists is as a tool for awareness; through facilitated workshops, local citizens and citizen science participants can be made aware of the species they are losing, and introduced, where relevant, to the habitats they share with birds (e.g. Senabre Hidalgo et al., 2021). Workshops can also incorporate community reflection, with interdisciplinary conservation practitioners working together to guide communities in considering their relationships to the declining species: Are people aware of these birds, where they live, and how they move through the world? What, if anything, makes them important to members of the community? Are there elements of cultural heritage or tradition linked to the species? What would be sacrificed in losing the species within the region? Such questions encourage individuals to consider their citizenship in ecosystems and more-than-human communities, paving pathways towards integrative experiences of nature.
A secondary purpose for Red and Amber Alerts is as an advisory tool for conservation scientists, providing guidance for determining which species are at greatest risk, prioritising and refining management, and structuring education and outreach. For instance, Wetland bird population trends are strongly associated with effective governance, and countries with weaker conservation governance see greater population declines (Amano et al., 2017; Gaget et al., 2018). Additionally, data deficiencies in countries with poor governance may skew understandings of actual population statuses and threats (Amano et al., 2017; Lee et al., 2022). In these contexts, local projects like the SABAP2 monitoring in Hessequa are crucial; if Wetland bird populations are known to be closely linked with conservation governance, SABAP2 data can be used to not only observe population trends in relation to land change, but also to monitor the effectiveness of governance and interventions (e.g. Linz, 2020).
Yellow Alerts are arguably the most critical for conservationists to consider: which species are beginning to decline, and where are they? Identifying these species early on allows scientists to stage preventative interventions rather than attempting to halt or reverse already significant declines. For Hessequa, since the Yellow Alert list was relatively short (26 species), it may be feasible to craft individually targeted studies, perhaps incentivising postgraduate research or community-led citizen science projects that investigate the factors influencing observed declines for a particular species. As well as bolstering current knowledge surrounding the species at risk, such projects also encourage a deeper civic awareness of local conservation challenges, and offer opportunities for local communities to form unique relationships with specific target species in their region (e.g. Kobori et al., 2016). There is evidence that meaningful connections with one element of non-human nature facilitate a broader and healthier relationship with non-human nature as a whole (Evans et al., 2005; Toomey and Domroese, 2013) and may even increase species advocacy (Forrester et al., 2017). Yellow Alert species may provide an ideal starting point for local non-scientist community members to engage with the rest of the natural world.
With the rise of concepts such as Essential Biodiversity Variables and increasing understandings of the importance of species diversity in ecosystems, it is quickly apparent that monitoring data are valuable for all species and populations—rare, threatened, stable, and increasing (Pereira et al., 2013; McGeoch and Latombe, 2015; Weeks et al., 2022). In this way, Green and Blue categories can still be considered ‘Alerts,’ as they provide critical baseline data for species that are doing well, but can also trigger further investigation for species potentially beginning to experience significant population increases. For instance, in our study, Blue Crane relative abundance was estimated to have increased by 16% between 2014 and 2021. This species is a national icon and is listed as Vulnerable by the IUCN RedList (2022) with a declining global population trend; thus, continued regional increases over a seven-year period are an encouraging statistic. Patterns like these for Green and Blue Alert species can inform research initiatives focussed on the factors supporting population increases, potentially enhancing conservation interventions for the species in other parts of their range.
Similarly, Purple Alerts are important for understanding the local implications of a species population increase. For potentially problematic species (i.e. Red-billed Quelea), a Purple Alert deepens existing knowledge of species movements and can open opportunities to engage with the people who are most affected by them. There is also opportunity for broader ecological learning; for instance, although further research is needed to understand the implications of House Sparrow and Common Starling expansion in South Africa, it is known that both species have experienced substantial declines across their native ranges which cover much of continental Europe and Asia (Crick et al., 2002; Freeman et al., 2007). Reasons for these declines remain unclear (De Laet and Summers-Smith, 2007; Heldbjerg et al., 2016; Heldbjerg et al., 2019; Balmori, 2021), but as native populations decline, there is much to be learned from South Africa’s invasive populations. Studies of genetic diversity, dispersal strategies, and post-invasion adaptations help to build an understanding of the factors and traits influencing invasion success (Berthouly-Salazar et al., 2013; Hanson et al., 2020; Stuart et al., 2022; Stuart et al., 2023).
Finally, for ecologically non-negative species, Pink Alerts can provide a clear indication of the habitat types expanding within a region, whilst improving understanding of the species diversity these habitats can support. As was the case in Hessequa, it is very possible that many species in this category will inhabit novel ecosystems. Mixed landscapes are a critical frontier in conservation, and Pink Alert species lists may be particularly valuable when paired with landscape change analysis and species lists from Red and Amber Alerts; taken together, the categories depict how different species respond to shared changes in habitat.
4.3 Citizen science as a monitoring tool
In every case, true data are preferable to modelled population estimates, and through their data collection efforts, citizen science participants make the notion of true data a real possibility. Although citizen science data are capable of meeting monitoring requirements, they may also be used in conjunction with existing management tools to improve understanding of population trends. For instance, when used in partnership with SABAP2 data, the alternative threat categories identified in BirdLife South Africa’s 2018 publication, The State of South Africa’s Birds (Taylor and Peacock, 2018), the IUCN Red List (2022), or the classification scheme for endemic birds proposed by Lee et al. (2017) can situate regional monitoring efforts in a broader national or international context, enhancing the applied value of both datasets.
Furthermore, the ability of these categories to serve as a tool for informing both scientific and non-scientific communities makes them a valuable asset to conservation research, especially as the need for interdisciplinary collaboration, inclusivity, and community integration becomes increasingly apparent (Tallis and Lubchenco, 2014; Gavin et al., 2015; Raymond et al., 2022). Besides the importance of the data themselves, it is also necessary to acknowledge the role of regional-scale monitoring efforts in shaping community-level conservation. As noted by Kobori et al. (2016), “A future in which national experiments are launched to address specific environmental or biodiversity problems would certainly bode well for engaging the public in the problems of the day.” Beyond collecting critical monitoring data, participatory early warning systems (alerts) encourage local awareness of environmental challenges and offer a sense of investment and ownership in regional-scale conservation (Seng, 2012; Weise et al., 2019; Tabor and Holland, 2020). In Hessequa, a small community of bird atlasers were able to generate data of sufficient quality to detect local declines. The success here suggests that implementing similar projects elsewhere may strengthen existing knowledges of species distributions, ranges, and abundance, but may also strengthen community support for the conservation measures taken on their behalf. Our study in Hessequa demonstrates the power of placing citizen science at the forefront of applied conservation; a well-structured and community-led initiative has the potential to inform individuals, decisions, and policy.
4.4 Caveats regarding regional change and rare species
When considering changes in species range and relative abundance, it is necessary to keep sight of a global context. A regional increase might indicate an overall increase in relative abundance across a species’ entire range, but it might also equate to a regional decrease in relative abundance elsewhere. In fact, this appears to be the case for the Blue Crane; this species is relatively new in the Western Cape, where recent transformations of Fynbos and Renosterveld to artificial grassland have supported the movement of Blue Cranes into the province (McCann et al., 2007; Young and Harrison, 2020). As the concentration of Blue Cranes in the Western Cape increased, populations in eastern grassland habitats experienced sharp declines, largely driven by land transformation (McCann et al., 2007), and as of 2009, roughly half of South Africa’s Blue Crane population were estimated to inhabit artificial grasslands in the Western Cape (Pettifor et al., 2009). Thus, while Blue Cranes in the Hessequa region are increasing in relative abundance, populations in other provinces are declining.
Regional changes may also reflect range changes for a species as a whole. For instance, the Knysna Warbler (B. sylvaticus) in our study area showed a decrease in relative abundance of 91% and is primarily associated with Thicket and Forest habitats. SABAP2 data have indicated general declines in South Africa’s Forest bird species (Cooper et al., 2017), but Lee et al. (2017) found that Knysna Warbler populations were moving eastward in southern Africa. These uncertainties reiterate the importance of context surrounding an alert; local declines must be vetted against range-wide trends and species biology to understand whether the observed changes are anthropogenic or stochastic in origin (Prochazka et al., 2023). For the Knysna Warbler, several factors may be at play, as birds are likely moving eastwards in response to habitat degradation in their historic range and are also threatened by low habitat connectivity within their current range (Lee et al., 2017). Untangling the truth requires monitoring at both small and large spatial scales, and a combination of current and historic data.
Because we elected to omit rare species from our study in order to focus on the core avifaunal composition of the region, it is also necessary to consider the species we may have missed in our analyses. Some of the omitted species may have narrow ranges, and their local populations dwindled below our 5% reporting rate cut-off during the study period. This possibility is cause for concern, as it may have resulted in overlooking species most in need of rapid intervention. However, of the 158 species omitted from this study, the overwhelming majority were species at the periphery of their ranges in Hessequa. Meaningful conservation interventions for these species would be better focussed in the core of their ranges. Many of the species we omitted were also vagrants or species for whom there is no suitable permanent habitat in Hessequa, though a few were cryptic species with low detection probabilities (i.e. Fynbos Buttonquail). A summary table of the omitted species is available in Appendix 1.
5 Conclusion
The value of this research is twofold: it provides valuable insight into the state of Hessequa’s avian populations, and demonstrates the potential for citizen science as a monitoring tool to generate early warnings for biodiversity loss. Using systematically collected atlasing data, we were able to detect significant changes in range and relative abundance for the most commonly reported species in the Hessequa region and categorise these changes by cause for conservation concern. We found that Wetland, Marine and Grassland species in particular are experiencing severe declines and noted increases for several transformed Grassland and Urban associated species. Our alert levels can guide both conservationists and community members in understanding and engaging with the status of local species. Crucially, alert levels may be used to prioritise regional conservation interventions for particular species or habitats, and support community involvement in species conservation. These results are a testament to the value of regional-scale monitoring; in a seven-year period, citizen science participants collected a sufficient quality and quantity of data to support robust statistical analysis with meaningful conservation applications. Participatory citizen science initiatives may benefit from structuring data collection in ways similar to the Hessequa project; our research outcomes suggest that this style of monitoring benefits the scientific community (improving knowledge of species statuses), local communities (structuring conservation outreach and encouraging individuals to think about species in new ways), and ultimately, the species themselves (informing conservation intervention). Such collaborative and targeted initiatives may comprise the necessary future of an inclusive and interdisciplinary conservation.
Data availability statement
The original contributions presented in the study are included in the article/Supplementary Material. Further inquiries can be directed to the corresponding author.
Author contributions
KAD wrote the introduction, methods, results, discussion, and conclusion. LGU contributed to the methods and provided comments and feedback on the remainder of the manuscript. JAVR assisted in proofreading and revising the manuscript, and coordinated the bird atlasing efforts in Stilbaai which made this research possible. All authors contributed to the article and approved the submitted version.
Funding
The author(s) declare that no financial support was received for the research, authorship, and/or publication of this article.
Acknowledgments
We are deeply grateful to the U3A Stilbaai Bird Group for their remarkable efforts in species monitoring, and for consistently pushing the boundaries of citizen science.
Conflict of interest
The authors declare that the research was conducted in the absence of any commercial or financial relationships that could be construed as a potential conflict of interest.
Publisher’s note
All claims expressed in this article are solely those of the authors and do not necessarily represent those of their affiliated organizations, or those of the publisher, the editors and the reviewers. Any product that may be evaluated in this article, or claim that may be made by its manufacturer, is not guaranteed or endorsed by the publisher.
Supplementary material
The Supplementary Material for this article can be found online at: https://www.frontiersin.org/articles/10.3389/fbirs.2024.1214800/full#supplementary-material
References
Ainsley J., Underhill L. G. (2017). Gamification (persuasive design) in the second Southern African bird atlas project. Die Vogelwelt 137, 19–22.
Amano T., Székely T., Sandel B., Nagy S., Mundkur T., Langendoen T., et al. (2017). Successful conservation of global waterbird populations depends on effective governance. Nature 553 (7687), 199–202. doi: 10.1038/nature25139
Ambrose S. (1991). Australian Bird Count instruction sheet (Melbourne: Royal Australian Ornithologists’ Union).
Asase A., Mzumara-Gawa T. I., Owino J. O., Peterson A. T., Saupe E. (2021). Replacing “parachute science“ with “global science” in ecology and conservation biology. Conserv. Sci. Pract. 4 (5), e517. doi: 10.1111/csp2.517
Baker D. J., Garnett S. T., O'Connor J., Ehmke G., Clarke R. H., Woinarski J. C. Z., et al (2018). Conserving the abundance of nonthreatened species. Cons. Biol. 33 (2), 319–328. doi: 10.1111/cobi.13197
Balmori A. (2021). Electromagnetic pollution as a possible explanation for the decline of house sparrows in interaction with other factors. Birds 2 (3), 329–337. doi: 10.3390/birds2030024
Barnard P., Altwegg R., Ebrahim I., Underhill L. G. (2017). Early warning systems for biodiversity in southern Africa–How much can citizen science mitigate imperfect data? Biol. Conserv. 208, 183–188. doi: 10.1016/j.biocon.2016.09.011
Barnes K. N. (1998). The important bird areas of southern Africa (Johannesburg, South Africa: BirdLife South Africa).
Barnes K. N. (2000). The Eskom red data book of birds of South Africa, Lesotho and Swaziland (Johannesburg, South Africa: BirdLife South Africa).
Batáry P., Báldi A., Erdős S. (2006). Grassland versus non-grassland bird abundance and diversity in managed grasslands: local, landscape and regional scale effects (Dordrecht, Netherlands: Vertebrate Conservation and Biodiversity), 45–55.
Berruti A. (2000). The pest status and biology of the Red-billed Quelea in the Bergville-Winterton area of South Africa. Workshop Res. Priorities Migrant Pests Agric. South. Afr. 3 (37), 113–118.
Berthouly-Salazar C., Hui C., Blackburn T. M., Gaboriaud C., Van Rensburg B. J., Van Vuuren B. J., et al. (2013). Long-distance dispersal maximizes evolutionary potential during rapid geographic range expansion. Mol. Ecol. 22 (23), 5793–5804. doi: 10.1111/mec.12538
Betts J., Young R. P., Hilton-Taylor C., Hoffmann M., Rodríguez J. P., Stuart S. N., et al. (2020). A framework for evaluating the impact of the IUCN Red List of threatened species. Conserv. Biol. 34 (3), 632–643. doi: 10.1111/cobi.13454
Bird T. J., Bates A. E., Lefcheck J. S., Hill N. A., Thomson R. J., Edgar G.J., et al. (2014). Statistical solutions for error and bias in global citizen science datasets. Biol. Conserv. 173, 144–154. doi: 10.1016/j.biocon.2013.07.037
Boakes E. H., McGowan P. J., Fuller R. A., Chang-qing D., Clark N. E., O'Connor K., et al. (2010). Distorted views of biodiversity: spatial and temporal bias in species occurrence data. PloS Biol. 8 (6), e1000385. doi: 10.1371/journal.pbio.1000385
Boersch-Supan P. H., Trask A. E., Baillie S. R. (2019). Robustness of simple avian population trend models for semi-structured citizen science data is species-dependent. Biol. Conserv. 240, 108286. doi: 10.1016/j.biocon.2019.108286
Brooks M., Rose S., Altwegg R., Lee A. T. K., Nel H., Ottosson U., et al. (2022). The African Bird Atlas Project: a description of the project and BirdMap data-collection protocol. Ostrich 93 (4), 223–232. doi: 10.2989/00306525.2022.2125097
Brown E. D., Williams B. K. (2019). The potential for citizen science to produce reliable and useful information in ecology. Conserv. Biol. 33 (3), 561–569. doi: 10.1111/cobi.13223
Burns F., Eaton M. A., Burfield I. J., Klvaňová A., Šilarová E., Staneva A., et al. (2021). Abundance decline in the avifauna of the European Union reveals cross-continental similarities in biodiversity change. Ecol. Evol. 11 (23), 16647–16660. doi: 10.1002/ece3.8282
Cardillo M., Mace G. M., Gittleman J. L., Jones K. E., Bielby J., Purvis A. (2008). The predictability of extinction: biological and external correlates of decline in mammals. Proc. R. Soc. B: Biol. Sci. 275 (1641), 1441–1448. doi: 10.1098%2Frspb.2008.0179
CBD (Convention on Biological Diversity) (2022). Kunming-Montreal Global biodiversity framework (Montreal, Canada: United Nations Environment Programme), 7–19.
Ceballos G., Ehrlich P. R., Dirzo R. (2017). Biological annihilation via the ongoing sixth mass extinction signaled by vertebrate population losses and declines. Proc. Natl. Acad. Sci. 114 (30), E6089–E6096. doi: 10.1073/pnas.1704949114
Chambers L. E., Barnard P., Poloczanska E. S., Hobday A. J., Keatley M. R., Allsopp N., et al. (2016). Southern hemisphere biodiversity and global change: Data gaps and strategies. Austral Ecol. 42 (1), 20–30. doi: 10.1111/aec.12391
Cheke R. A., Venn J. F., Jones P. J. (2007). Forecasting suitable breeding conditions for the red-billed quelea Quelea quelea in southern Africa. J. Appl. Ecol. 44 (3), 523–533. doi: 10.1111/j.1365-2664.2007.01295.x
Chichorro F., Juslén A., Cardoso P. (2019). A review of the relation between species traits and extinction risk. Biol. Conserv. 237, 220–229. doi: 10.1016/j.biocon.2019.07.001
Chittenden H., Davies G., Weiersbye I. (2018). Roberts Bird Guide (Johannesburg, South Africa: Jacana Media).
Christie A. P., Amano T., Martin P. A., Petrovan S. O., Shackelford G. E., Simmons B. I., et al. (2020). The challenge of biased evidence in conservation. Conserv. Biol. 35 (1), 249–262. doi: 10.1111/cobi.13577
Clavel J., Julliard R., Devictor V. (2011). Worldwide decline of specialist species: Toward a global functional homogenization? Front. Ecol. Environ. 9 (4), 222–228. doi: 10.1890/080216
Cooper T. J., Wannenburgh A. M., Cherry M. I. (2017). Atlas data indicate forest dependent bird species declines in South Africa. Bird Conserv. Int. 27 (3), 337–354. doi: 10.1017/S095927091600040X
Correll M. D., Strasser E. H., Green A. W., Panjabi A. O. (2019). Quantifying specialist avifaunal decline in grassland birds of the Northern Great Plains. Ecosphere 10 (1), e02523. doi: 10.1002/ecs2.2523
Cresswell W. (2018). The continuing lack of ornithological research capacity in almost all of West Africa. Ostrich 89 (2), 123–129. doi: 10.2989/00306525.2017.1388301
Crick H. Q., Robinson R. A., Appleton G. F., Clark N. A., Rickard A. D. (2002). Investigation into the causes of the decline of starlings and house sparrows in Great Britain. BTO Res. Rep. 290, 1–305.
Cyvin J. B. (2022). Can we to a greater extent involve citizens in environmental research? Front. Environ. Sci. 10. doi: 10.3389/fenvs.2022.874559
Dallimer M., Jones P. J. (2002). Migration orientation behaviour of the red-billed quelea Quelea quelea. J. Avian Biol. 33 (1), 89–94. doi: 10.1034/j.1600-048X.2002.330114.x
Daniel K. A., Underhill L. G. (2023). Temporal dimensions of data quality in bird atlases: The case of the Second Southern African Bird Atlas Project. Citizen Sci. Theory Pract. 8 (1), 31. doi: 10.5334/cstp.578
De Laet J., Summers-Smith J. D. (2007). The status of the urban house sparrow Passer domesticus in north-western Europe: a review. J. Ornithology 148 (Suppl. 2), 275–278. doi: 10.1007/s10336-007-0154-0
de Vos A. (2022). Stowing parachutes, strengthening science. Conserv. Sci. Pract. 4 (5), e12709. doi: 10.1111/csp2.12709
Díaz S., Settele J., Brondízio E. S., Ngo H. T., Agard J., Arneth A., et al. (2019). Pervasive human-driven decline of life on Earth points to the need for transformative change. Science 366 (6471), eaax3100. doi: 10.1126/science.aax3100
Dirzo R., Young H. S., Galetti M., Ceballos G., Isaac N. J., Collen B. (2014). Defaunation in the anthropocene. Science 345 (6195), 401–406. doi: 10.1126/science.1251817
Elliott C. C. H. (1990). The migrations of the Red-billed Quelea Quelea quelea and their relation to crop damage. Ibis 132 (2), 232–237. doi: 10.1111/j.1474-919X.1990.tb01041.x
Evans C., Abrams E., Reitsma R., Roux K., Salmonsen L., Marra P. P. (2005). The neighborhood nestwatch program: Participant outcomes of a citizen-science ecological research project. Conserv. Biol. 19 (3), 589–594. doi: 10.1111/j.1523-1739.2005.00s01.x
Feeley K. J., Stroud J. T., Perez T. M. (2016). Most ‘global’ reviews of species’ responses to climate change are not truly global. Diversity Distributions 23 (3), 231–234. doi: 10.1111/ddi.12517
Forrester T. D., Baker M., Costello R., Kays R., Parsons A. W., McShea W. J. (2017). Creating advocates for mammal conservation through citizen science. Biol. Conserv. 208, 98–105. doi: 10.1016/j.biocon.2016.06.025
Freeman S. N., Robinson R. A., Clark J. A., Griffin B. M., Adams S. Y. (2007). Changing demography and population decline in the Common Starling Sturnus vulgaris: A multisite approach to Integrated Population Monitoring. Ibis 149 (3), 587–596. doi: 10.1111/j.1474-919X.2007.00684.x
Gaget E., Galewski T., Jiguet F., Le Viol I. (2018). Waterbird communities adjust to climate warming according to conservation policy and species protection status. Biol. Conserv. 227, 205–212. doi: 10.1016/j.biocon.2018.09.019
García M. B., Silva J. L., Tejero P., Pardo I. (2021). Detecting early-warning signals of concern in plant populations with a citizen science network. Are threatened and other priority species for conservation performing worse? J. Appl. Ecol. 58 (7), 1388–1398. doi: 10.1111/1365-2664.13890
Gaston K. J., Fuller R. A. (2009). The sizes of species’ geographic ranges. J. Appl. Ecol. 46 (1), 1–9. doi: 10.1111/j.1365-2664.2008.01596.x
Gavin M. C., McCarter J., Mead A., Berkes F., Stepp J. R., Peterson D., et al. (2015). Defining biocultural approaches to conservation. Trends Ecol. Evol. 30 (3), 140–145. doi: 10.1016/j.tree.2014.12.005
Gil-Tena A., De Cáceres M., Ernoult A., Butet A., Brotons L., Burel F. (2015). Agricultural landscape composition as a driver of farmland bird diversity in Brittany (NW France). Agriculture Ecosyst. Environ. 205, 79–89. doi: 10.1016/j.agee.2015.03.013
Giovos I., Kleitou P., Poursanidis D., Batjakas I., Bernardi G., Crocetta F., et al. (2019). Citizen-science for monitoring marine invasions and stimulating public engagement: A case project from the eastern Mediterranean. Biol. Invasions 21 (12), 3707–3721. doi: 10.1007/s10530-019-02083-w
Gossa C., Fisher M., Milner-Gulland E. J. (2015). The research–implementation gap: How practitioners and researchers from developing countries perceive the role of peer-reviewed literature in conservation science. Oryx 49 (1), 80–87. doi: 10.1017/S0030605313001634
Griffioen P. (2001). Temporal changes in the distributions of bird species in eastern Australia (Australia: La Trobe University). Unpublished PhD thesis.
Hanson H. E., Mathews N. S., Hauber M. E., Martin L. B. (2020). The house sparrow in the service of basic and applied biology. Elife 9, e52803. doi: 10.7554/eLife.52803.sa2
Hardison D. R., Holland W. C., Currier R. D., Kirkpatrick B., Stumpf R., Fanara T., et al. (2019). HABscope: A tool for use by citizen scientists to facilitate early warning of respiratory irritation caused by toxic blooms of Karenia brevis. PloS One 14 (6), e0218489. doi: 10.1371/journal.pone.0218489
Harrison J. A., Allan D. G., Underhill L. G., Herremans M., Tree A. J., Parker V., et al. (1997). The Atlas of Southern African Birds, Vol. 1 (Johannesburg: BirdLife South Africa).
Harrison J. A., Underhill L. G. (1997). “). Introduction and methods,” in The Atlas of Southern African Birds, vol. 1 . Eds. Harrison J. A., Allan D. G., Underhill L. G., Herremans M., Tree A. J., Parker V., Brown C. J. (BirdLife South Africa: Johannesburg), xliii–xlxiv.
Heldbjerg H., Fox A. D., Lehikoinen A., Sunde P., Aunins A., Balmer D. E., et al. (2019). Contrasting population trends of Common Starlings (Sturnus vulgaris) across Europe. Ornis Fennica 96, 153–168. doi: 10.51812/of.133957
Heldbjerg H., Fox A. D., Levin G., Nyegaard T. (2016). The decline of the Starling Sturnus vulgaris in Denmark is related to changes in grassland extent and intensity of cattle grazing. Agriculture Ecosyst. Environ. 230, 24–31. doi: 10.1016/j.agee.2016.05.025
Hernández-Yáñez H., Kim S. Y., Che-Castaldo J. P. (2022). Demographic and life history traits explain patterns in species vulnerability to extinction. PloS One 17 (2), e0263504. doi: 10.1371/journal.pone.0263504
Hochkirch A., Samways M. J., Gerlach J., Böhm M., Williams P., Cardoso P., et al. (2021). A strategy for the next decade to address data deficiency in neglected biodiversity. Conserv. Biol. 35 (2), 502–509. doi: 10.1111/cobi.13589
Hoveka L. N., van der Bank M., Bezeng B. S., Davies T. J. (2020). Identifying biodiversity knowledge gaps for conserving South Africa’s endemic flora. Biodiversity Conserv. 29, 2803–2819. doi: 10.1007/s10531-020-01998-4
Huntley B., Altwegg R., Barnard P., Collingham Y. C., Hole D. G. (2012). Modelling relationships between species spatial abundance patterns and climate. Global Ecol. Biogeography 21 (6), 668–681. doi: 10.1111/j.1466-8238.2011.00701.x
Isaac N. J., van Strien A. J., August T. A., de Zeeuw M. P., Roy D. B. (2014). Statistics for citizen science: Extracting signals of change from noisy ecological data. Methods Ecol. Evol. 5 (10), 1052–1060. doi: 10.1111/2041-210X.12254
Isbell F., Balvanera P., Mori A. S., He J. S., Bullock J. M., Regmi G. R., et al. (2023). Expert perspectives on global biodiversity loss and its drivers and impacts on people. Front. Ecol. Environ. 21 (2), 94–103. doi: 10.1002/fee.2536
IUCN (2022) The IUCN Red List of Threatened Species (Version 2022-2). Available at: https://www.icunredlist.org (Accessed 1 June 2023).
Ivanova I. M., Symes C. T. (2018). Common starling Sturnus vulgaris expansion in South Africa. Biodiversity Observations 9 (9), 1–6. doi: 10.15641/bo.v9i0.502
Jetz W., McGeoch M. A., Guralnick R., Ferrier S., Beck J., Costello M. J., et al. (2019). Essential biodiversity variables for mapping and monitoring species populations. Nat. Ecol. Evol. 3 (4), 539–551. doi: 10.1038/s41559-019-0826-1
Jetz W., McPherson J. M., Guralnick R. P. (2011). Integrating biodiversity distribution knowledge: Toward a global map of life. Trends Ecol. Evol. 27 (3), 151–159. doi: 10.1016/j.tree.2011.09.007
Jiang Z., Jiang J., Wang Y., Zhang E., Zhang Y., Cai B. (2020). Significance of country red lists of endangered species for biodiversity conservation. Biodiversity Sci. 28 (5), 558–565. doi: 10.17520/biods.2020149
Johnston A., Hochachka W. M., Strimas-Mackey M. E., Ruiz Gutierrez V., Robinson O. J., Miller E. T., et al. (2021). Analytical guidelines to increase the value of community science data: An example using eBird data to estimate species distributions. Diversity Distributions 27 (7), 1265–1277. doi: 10.1111/ddi.13271
Kamp J., Frank C., Trautmann S., Busch M., Dröschmeister R., Flade M., et al. (2020). Population trends of common breeding birds in Germany 1990–2018. J. Ornithology 162 (1), 1–15. doi: 10.1007/s10336-020-01830-4
Kamp J., Oppel S., Heldbjerg H., Nyegaard T., Donald P. F. (2016). Unstructured citizen science data fail to detect long-term population declines of common birds in Denmark. Diversity Distributions 22 (10), 1024–1035. doi: 10.1111/ddi.12463
Kelling S., Fink D., La Sorte F. A., Johnston A., Bruns N. E., Hochachka W. M. (2015). Taking a ‘Big Data’ approach to data quality in a citizen science project. Ambio 44, 601–611. doi: 10.1007/s13280-015-0710-4
Kobori H., Dickinson J. L., Washitani I., Sakurai R., Amano T., Komatsu N., et al. (2016). Citizen science: A new approach to advance ecology, education, and conservation. Ecol. Res. 31, 1–19. doi: 10.1007/s11284-015-1314-y
Lawton J. H. (1993). Range, population abundance and conservation. Trends Ecol. Evol. 8 (11), 409–413. doi: 10.1016/0169-5347(93)90043-O
Lee A. T., Altwegg R., Barnard P. (2017). Estimating conservation metrics from atlas data: the case of southern African endemic birds. Bird Conserv. Int. 27 (3), 323–336. doi: 10.1017/S0959270916000307
Lee A. T., Brooks M., Underhill L. G. (2022). The SABAP2 legacy: A review of the history and use of data generated by a long-running citizen science project. South Afr. J. Sci. 118 (1–2), 1–4. doi: 10.17159/sajs.2022/12030
Lee T. M., Jetz W. (2010). Unravelling the structure of species extinction risk for predictive conservation science. Proc. R. Soc. B: Biol. Sci. 278 (1710), 1329–1338. doi: 10.1098/rspb.2010.1877
Le Viol I., Jiguet F., Brotons L., Herrando S., Lindström Å., Pearce-Higgins J. W., et al. (2012). More and more generalists: Two decades of changes in the European avifauna. Biol. Lett. 8 (5), 780–782. doi: 10.1098/rsbl.2012.0496
Linsdale J. M. (1928). A method of showing relative frequency of occurrence of birds. Condor 30 (3), 180–184. doi: 10.2307/1363275
Linz G. M. (2020). Modeling migratory nongame birds: a plea for data. Human-Wildlife Interact. 14 (3), 538–540. doi: 10.26077/4983-4fc9
Luther D. A., Brooks T. M., Butchart S. H., Hayward M. W., Kester M. E., Lamoreux J., et al. (2016). Determinants of bird conservation-action implementation and associated population trends of threatened species. Conserv. Biol. 30 (6), 1338–1346. doi: 10.1111/cobi.12757
Mace G. M., Collar N. J., Gaston K. J., Hilton-Taylor C., Akçakaya H. R., Leader-Williams N., et al. (2008). Quantification of extinction risk: IUCN's system for classifying threatened species. Conserv. Biol. 22 (6), 1424–1442. doi: 10.1111/j.1523-1739.2008.01044.x
Maclean I. M., Wilson R. J., Hassall M. (2011). Predicting changes in the abundance of African wetland birds by incorporating abundance–occupancy relationships into habitat association models. Diversity Distributions 17 (3), 480–490. doi: 10.1111/j.1472-4642.2011.00756.x
Magudu K., Downs C. T. (2015). The relative abundance of invasive house sparrows (Passer domesticus) in an urban environment in South Africa is determined by land use. Afr. J. Wildlife Res. 45 (3), 354–359. doi: 10.3957/056.045.0354
Marchezini V., Horita F. E. A., Matsuo P. M., Trajber R., Trejo-Rangel M. A., Olivato D. (2018). A review of studies on Participatory Early Warning Systems (P-EWS): Pathways to support citizen science initiatives. Front. Earth Sci. 6, 184. doi: 10.3389/feart.2018.00184
Marques A. T., Moreira F., Alcazar R., Delgado A., Godinho C., Sampaio H., et al. (2020). Changes in grassland management and linear infrastructures associated to the decline of an endangered bird population. Sci. Rep. 10 (1), 1–11. doi: 10.1038/s41598-020-72154-9
McCann K., Theron L. J., Morrison K. (2007). Conservation priorities for the Blue Crane (Anthropoides paradiseus) in South Africa—the effects of habitat changes on distribution and numbers. Ostrich J. Afr. Ornithology 78 (2), 205–211. doi: 10.2989/OSTRICH.2007.78.2.14.94
McGeoch M. A., Latombe G. (2015). Characterizing common and range expanding species. J. Biogeography 43 (2), 217–228. doi: 10.1111/jbi.12642
McGill B., Collins C. (2003). A unified theory for macroecology based on spatial patterns of abundance. Evolutionary Ecol. Res. 5 (4), 469–492.
Miller J., White T. B., Christie A. P. (2023). Parachute conservation: Investigating trends in international research. Conserv. Lett. 16 (3), e12947. doi: 10.1111/conl.12947
Msimanga A. O. (2001). The evolution of the house sparrow (Passer domesticus) in southern Africa (South Africa: University of KwaZulu Natal). Unpublished PhD.
Nachman G. (1981). A mathematical model of the functional relationship between density and spatial distribution of a population. J. Anim. Ecol. 50 (2), 453–460. doi: 10.2307/4066
Oduntan O. O., Shotuyo A. L. A., Akinyemi A. F., Soaga J. A. (2015). Human-wildlife conflict: A view on red-billed quelea (Quelea quelea). Int. J. Mol. Evol. Biodiversity 5 (2), 1–4. doi: 10.5376/ijmeb.2015.05.0002
Oliver R. Y., Meyer C., Ranipeta A., Winner K., Jetz W. (2021). Global and national trends, gaps, and opportunities in documenting and monitoring species distributions. PloS Biol. 19 (8), e3001336. doi: 10.1371/journal.pbio.3001336
Orme C. D. L., Davies R. G., Olson V. A., Thomas G. H., Ding T. S., Rasmussen P. C., et al. (2006). Global patterns of geographic range size in birds. PloS Biol. 4 (7), e208. doi: 10.1371/journal.pbio.0040208
Oschadleus H. D. (2000). “Red-billed quelea movements in southern Africa shown by ringing recoveries in the SAFRING database,” in Cheke R. A., Rosenberg L. J., Kieser M. E. Eds. Workshop on Research Priorities for Migrant Pests of Agriculture in Southern Africa, Plant Protection Research Institute, Pretoria, South Africa, 24–26 March 1999. 125–135. Chatham, UK: Natural Resources Institute.
OsChadleus H. D. (2015). First red-billed quelea breeding record in the winter rainfall region of South Africa. Ostrich 86 (3), 295–296. doi: 10.2989/00306525.2015.1069413
OsChadleus H. D., Underhill L. G. (2006). Range expansion of the red-billed quelea, Quelea quelea, into the Western Cape, South Africa: Research in action. South Afr. J. Sci. 102 (1), 12–13.
Parsons E. C. M. (2016). Why IUCN should replace “data deficient“ conservation status with a precautionary “assume threatened“ status—a cetacean case study. Front. Mar. Sci. 3, 193. doi: 10.3389/fmars.2016.00193
Pereira H. M., Ferrier S., Walters M., Geller G. N., Jongman R. H., Scholes R. J., et al. (2013). Essential biodiversity variables. Science 339 (6117), 277–278. doi: 10.1126/science.1229931
Pettifor R. A., Shaw K., Theron L. J., Morrison K., Botha B., Franke U., et al. (2009). “The importance of the Western Cape sub-population of blue cranes to their global population in the face of climate change: Implications for conservation,” in Proceedings of the 12th Pan African Ornithological Congress 2008. Eds. Harebottle D. M., Craig A. J. F. K., Anderson M. D., Rakotomanana H., Muchai S. M. (Cape Town, South Africa: Animal Demography Unit).
Poursanidis D., Zenetos A. (2013). The role played by citizen scientists in monitoring marine alien species in Greece. Cahiers Biologie Mar. 54, 419–426.
Prigent C., Papa F., Aires F., Jimenez C., Rossow W. B., Matthews E. (2012). Changes in land surface water dynamics since the 1990s and relation to population pressure. Geophysical Res. Lett. 39 (8). doi: 10.1029/2012GL051276
Prochazka B. G., Coates P. S., O'Donnell M. S., Edmunds D. R., Monroe A. P., Ricca M. A., et al. (2023). A targeted annual warning system developed for the conservation of a sagebrush indicator species. Ecol. Indic. 148, 110097. doi: 10.1016/j.ecolind.2023.110097
Proença V., Martin L. J., Pereira H. M., Fernandez M., McRae L., Belnap J., et al. (2017). Global biodiversity monitoring: from data sources to essential biodiversity variables. Biol. Conserv. 213, 256–263. doi: 10.1016/j.biocon.2016.07.014
Raymond C. M., Cebrian-Piqueras M. A., Andersson E., Andrade R., Schnell A. A., Romanelli B. B., et al. (2022). Inclusive conservation and the Post-2020 Global Biodiversity Framework: Tensions and prospects. One Earth 5 (3), 252–264. doi: 10.1016/j.oneear.2022.02.008
Robertson M. P., Cumming G. S., Erasmus B. F. N. (2010). Getting the most out of atlas data. Diversity Distributions 16 (3), 363–375. doi: 10.1111/j.1472-4642.2010.00639.x
Robertson A., Simmons R. E., Jarvis A. M., Brown C. J. (1995). Can bird atlas data be used to estimate population size? A case study using Namibian endemics. Biol. Conserv. 71 (1), 87–95. doi: 10.1016/0006-3207(94)00024-K
Rogers A. M., Chown S. L. (2013). Novel ecosystems support substantial avian assemblages: the case of invasive alien Acacia thickets. Diversity Distributions 20 (1), 34–45. doi: 10.1111/ddi.12123
Rosenberg K. V., Dokter A. M., Blancher P. J., Sauer J. R., Smith A. C., Smith P. A., et al. (2019). Decline of the North American avifauna. Science 366 (6461), 120–124. doi: 10.1126/science.aaw1313
Rovero F., Ahumada J. (2017). The Tropical Ecology, Assessment and Monitoring (TEAM) Network: an early warning system for tropical rain forests. Sci. Total Environ. 574, 914–923. doi: 10.1016/j.scitotenv.2016.09.146
Runge C. A., Tulloch A., Hammill E., Possingham H. P., Fuller R. A. (2015). Geographic range size and extinction risk assessment in nomadic species. Conserv. Biol. 29 (3), 865–876. doi: 10.1111/cobi.12440
Sasaki K., Hotes S., Kadoya T., Yoshioka A., Wolters V. (2020). Landscape associations of farmland bird diversity in Germany and Japan. Global Ecol. Conserv. 21, e00891. doi: 10.1016/j.gecco.2019.e00891
Schmeller D. S., Weatherdon L. V., Loyau A., Bondeau A., Brotons L., Brummitt N., et al. (2018). A suite of essential biodiversity variables for detecting critical biodiversity change. Biol. Rev. 93 (1), 55–71. doi: 10.1111/brv.12332
Senabre Hidalgo E., Perelló J., Becker F., Bonhoure I., Legris M., Cigarini A. (2021). “Participation and co-creation in citizen science. Chapter 11,” in The Science of Citizen Science. Ed. Vohland K., et al. (Cham, Switzerland: Springer), 199–218. doi: 10.1007/978-3-030-58278-4
Seng D. S. C. (2012). Improving the governance context and framework conditions of natural hazard early warning systems. J. Integrated Disaster Risk Manage. 2 (1), 1–25. doi: 10.5595/idrim.2012.0020
Sharman M., Mlambo M. C. (2012). Wicked: The problem of biodiversity loss. GAIA-Ecological Perspect. Sci. Soc. 21 (4), 274–277. doi: 10.14512/gaia.21.4.10
Siddig A. A. (2019). Why is biodiversity data-deficiency an ongoing conservation dilemma in Africa? J. Nat. Conserv. 50, 125719. doi: 10.1016/j.jnc.2019.125719
SoIB (2020) State of India's birds 2020: Range, trends and conservation status. The SoIB Partnership: 50. Available at: https://www.stateofIndiasbirds.in (Accessed 15 June 2023).
Staude I. R., Navarro L. M., Pereira H. M. (2019). Range size predicts the risk of local extinction from habitat loss. Global Ecol. Biogeography 29 (1), 16–25. doi: 10.1111/geb.13003
Stenoien C., Nail K. R., Zalucki J. M., Parry H., Oberhauser K. S., Zalucki M. P. (2018). Monarchs in decline: a collateral landscape-level effect of modern agriculture. Insect Sci. 25 (4), 528–541. doi: 10.1111/1744-7917.12404
Stephenson P. J., Stengel C. (2020). An inventory of biodiversity data sources for conservation monitoring. PloS One 15 (12), e0242923. doi: 10.1371/journal.pone.0242923
Stuart K. C., Hofmeister N. R., Zichello J. M., Rollins L. A. (2023). Global invasion history and native decline of the common starling: Insights through genetics. Biol. Invasions 25 (5), 1291–1316. doi: 10.1007/s10530-022-02982-5
Stuart K. C., Sherwin W. B., Austin J. J., Bateson M., Eens M., Brandley M. C., et al. (2022). Historical museum samples enable the examination of divergent and parallel evolution during invasion. Mol. Ecol. 31 (6), 1836–1852. doi: 10.1111/mec.16353
Sweeney C. P., Jarzyna M. A. (2022). Assessing the synergistic effects of land use and climate change on terrestrial biodiversity: Are generalists always the winners? Curr. Landscape Ecol. Rep. 7 (4), 41–48. doi: 10.1007/s40823-022-00073-8
Szabo J. K., Fuller R. A., Possingham H. P. (2012). A comparison of estimates of relative abundance from a weakly structured mass-participation bird atlas survey and a robustly designed monitoring scheme. Ibis 154 (3), 468–479. doi: 10.1111/j.1474-919X.2012.01229.x
Szabo J. K., Vesk P. A., Baxter P. W., Possingham H. P. (2010). Regional avian species declines estimated from volunteer-collected long-term data using List Length Analysis. Ecol. Appl. 20 (8), 2157–2169. doi: 10.1890/09-0877.1
Tabor K. M., Holland M. B. (2020). Opportunities for improving conservation early warning and alert systems. Remote Sens. Ecol. Conserv. 7 (1), 7–17. doi: 10.1002/rse2.163
Tallis H., Lubchenco J. (2014). Working together: a call for inclusive conservation. Nature 515 (7525), 27–28. doi: 10.1038/515027a
Taylor M. R., Peacock F. (2018). State of South Africa’s Birds Report 2018 (Johannesburg, South Africa: BirdLife South Africa).
Temple S. A., Temple A. J. (1984). Results of using checklist-records to monitor Wisconsin birds: A WSO research project. Passenger Pigeon 46 (2), 61–70.
Temple S. A., Temple A. J. (1986). Geographic distribution and patterns of relative abundance of Wisconsin birds: a WSO research project. Passenger Pigeon 48, 58–68.
Toomey A. H., Domroese M. C. (2013). Can citizen science lead to positive conservation attitudes and behaviors? Hum. Ecol. Rev. 20 (1), 50–62.
Underhill L. G., Brooks M., Loftie-Eaton M. (2017). The Second Southern African Bird Atlas Project: Protocol, process, product. Vogelwelt 137, 64–70.
Underhill L. G., Prŷs-Jones R. P., Harrison J. A., Martinez P. (1992). Seasonal patterns of occurrence of Palaearctic migrants in southern Africa using atlas data. Ibis 134, 99–108. doi: 10.1111/j.1474-919X.1992.tb04739.x
UNISDR (2005). “Hyogo framework for action 2005-2015: Building the resilience of nations and communities to disasters,” in World Conference on Disaster Reduction (A/CONF.206/6). Hyogo, Japan: United Nations, Kobe.
Vaccaro A. S., Filloy J., Bellocq M. I. (2019). What land use better preserves taxonomic and functional diversity of birds in a grassland biome? Avian Conserv. Ecol. 14 (1). doi: 10.5751/ACE-01293-140101
van Rooyen J. A. (2018). Systematic atlasing in Hessequa – Moving from mapping to monitoring. Biodiversity Observations 9 (10), 1–13. doi: 10.15641/bo.v9i0.508
van Rooyen J. A., Underhill L. G. (2020). Systematic atlasing in Hessequa: Report on the first cycle of seasonal monitoring. Biodiversity Observations 11 (3), 1–14. doi: 10.15641/bo.933
von der Heyden S. (2022). Environmental DNA surveys of African biodiversity: State of knowledge, challenges, and opportunities. Environ. DNA 5 (1), 12–17. doi: 10.1002/edn3.363
Ward C., Cundill G., Midgley G., Jarre A. (2021). Drawing on diverse knowledge systems to enhance local climate understanding in the southern Cape, South Africa. Ecol. Soc. 26 (4.10). doi: 10.5751/ES-12712-260410
Weeks B. C., Naeem S., Lasky J. R., Tobias J. A. (2022). Diversity and extinction risk are inversely related at a global scale. Ecol. Lett. 25 (3), 697–707. doi: 10.1111/ele.13860
Weise F. J., Hauptmeier H., Stratford K. J., Hayward M. W., Aal K., Heuer M., et al. (2019). Lions at the gates: Trans-disciplinary design of an early warning system to improve human-lion coexistence. Front. Ecol. Evol. 6 (2019), 242. doi: 10.3389/fevo.2018.00242
Winterbottom J. M., Liversidge R. (1954). The European Starling in the south west Cape. Ostrich 25 (2), 89–96. doi: 10.1080/00306525.1954.9633410
Young D. J., Harrison J. A. (2020). Trends in populations of blue crane Anthropoides paradiseus in agricultural landscapes of Western Cape, South Africa, as measured by road counts. Ostrich 91 (2), 158–168. doi: 10.2989/00306525.2020.1781702
Keywords: bird atlas, reporting rates, citizen science, early warning system, SABAP2, species monitoring
Citation: Daniel KA, Underhill LG and van Rooyen JA (2024) Bird atlas in action: using citizen science data to generate population trend alerts in Hessequa, South Africa. Front. Bird Sci. 3:1214800. doi: 10.3389/fbirs.2024.1214800
Received: 30 April 2023; Accepted: 04 January 2024;
Published: 19 January 2024.
Edited by:
Christoph Randler, University of Tübingen, GermanyReviewed by:
Ross Hill, Bournemouth University, United KingdomDavid Thomson, United Arab Emirates University, United Arab Emirates
Copyright © 2024 Daniel, Underhill and van Rooyen. This is an open-access article distributed under the terms of the Creative Commons Attribution License (CC BY). The use, distribution or reproduction in other forums is permitted, provided the original author(s) and the copyright owner(s) are credited and that the original publication in this journal is cited, in accordance with accepted academic practice. No use, distribution or reproduction is permitted which does not comply with these terms.
*Correspondence: Karis A. Daniel, ZG5sa2FyMDA2QG15dWN0LmFjLnph