- Sewall Laboratory, Virginia Tech, Department of Biological Sciences, Blacksburg, VA, United States
Urbanization is projected to grow rapidly in the coming decades. Several consequences of this form of anthropogenic change for ecosystems and individuals are described by the fields of urban ecology and urban ecophysiology, respectively. Of the environmental variables that could pose challenges for urban-living animals, energy limitations may be especially important, particularly for animals with critical periods of growth, such as nestling songbirds. Many studies document reduced arthropod prey availability for songbirds living in urban habitats, which can compromise the growth and condition of nestlings, possibly because of protein limitation. In our study system of replicate urban and rural song sparrow (Melospiza melodia) populations, there is lower arthropod biomass in urban habitats, as well as higher rates of brood parasitism by brown-headed cowbirds (Molothrus ater), which can cause additional nutritional limitation. Therefore, we hypothesized that urban nestling song sparrows may be protein-limited and experience compromised growth and body condition relative to rural nestlings. We tested this hypothesis with two studies. First, we took body measurements during the first 10 days of development from rural nestlings, urban nestlings without brood parasites, and urban nestlings with brood parasites to see if groups differed in growth (n = 348 nestlings over 6 years). Second, we collected nestling plasma samples (n = 43) from a subset of the birds measured in the first study and then used liquid chromatography-mass spectrometry (LC-MS) to determine concentrations of free amino acids. We found rural nestlings had greater change over time in body mass and tarsus length compared to both urban groups. However, we found no differences in amino acid concentrations across these nestling groups. Thus, urbanization appears to affect growth rates in song sparrows, especially when brood parasites are present in a nest, but we found no evidence supporting amino acids as the mechanistic link for these differences in our system.
1 Introduction
Urbanization of native ecosystems is increasing rapidly (Seto et al., 2012), and urban ecology aims to describe interactions among organisms and their environment within expanding cities and suburbs. A general consensus in this field is that urbanization alters every level of ecology, from ecosystem services and biodiversity to species interactions (McKinney, 2002). In parallel, urban ecophysiology examines the physiology and condition of animals in modified habitats to understand how urbanization impacts individual health (Isaksson, 2018; Bonier, 2012). While urban ecophysiology has provided important insights, the risk of drawing inferences about how harmful urban habitats are to different species based on physiological biomarkers is often cited as a challenge to this field (Reid et al., 2024; Iglesias-Carrasco et al., 2020; Isaksson, 2020; Isaksson, 2015; Meillere et al., 2015; Beaulieu and Costantini, 2014; Partecke et al., 2006). Linking studies of physiology with ecological measures that better define the challenges organisms face in urban habitats and defining the fitness consequences associated with physiological measures can help contextualize the physiological differences documented by urban ecophysiologists (Chatelain et al., 2021; Fenoglio et al., 2020; Isaksson, 2020; Bang et al., 2012; Faeth et al., 2005). Of the ecological variables that could pose challenges for urban-living animals, energy limitations may be especially important for understanding overall physical condition and has been the conceptual basis for understanding individual “health”, and therefore could link ecological variation to individual physiology (e.g., allostatic load; Coogan et al., 2018; McEwen and Wingfield, 2003).
Many studies examining the ecological and physiological effects of urbanization have focused on urban songbirds because many species have readily adapted to urban environments, but still face challenges associated with these altered habitats. For instance, urban areas have been associated with higher densities of brood parasites (Rodewald, 2009), such as brown-headed cowbirds (Molothrus ater). Cowbirds lay their eggs in the nests of other species in order for their young to be raised by host parents, often to the detriment of host offspring (Fenoglio et al., 2017; Payne, 1998, 1977). Brood parasitism can limit nutrition by increasing competition among nestlings for food parents provide (Ladin et al., 2015; Boonekamp et al., 2014; Lorenzana and Sealy, 1999; Payne, 1977). The few studies investigating brood parasitism and urbanization have shown mixed results, with some finding urbanization was associated with increased vulnerability to brood parasitism (Lane et al., 2023; Rodewald, 2009; Burhans and Thompson, 2006; Tewksbury et al., 2006; Chace et al., 2003), while other studies found decreasing brood parasitism with increasing urbanization (Buxton et al., 2018; Rodewald et al., 2013). However, the negative effects of brood parasitism on host offspring are well documented; host nestlings that survive the presence of a brood parasite are generally smaller and less developed when they fledge (Lane et al., 2023; Lorenzana and Sealy, 1999; Dearborn et al., 1998; Payne, 1977), greatly decreasing the likelihood they will survive the post-fledging period (Monros et al., 2003).
In addition to potential pressure from brood parasites, many urban ecology studies show that fewer arthropod prey are available in urban areas, and that arthropods are a critical source of protein for songbirds (Chatelain et al., 2023; Fenoglio et al., 2020; Razeng and Watson, 2015; Bennett and Lovell, 2014; Ramsay and Houston, 2003), though other studies have found generally no differences between habitats (Martinson and Raupp, 2013) or greater arthropod abundance in urban areas (Faeth et al, 2011). Several species of songbirds seasonally shift their diet to feed their young a higher proportion of protein-rich arthropods during the breeding season (Martin et al., 1951; Judd, 1901). Any nutritional limitation in urban areas may be especially detrimental to developing nestlings (Reid et al., 2024) because they experience a rapid period of post-hatching growth (i.e. a “critical period”). For example, a study comparing urban and rural nestling crows found that urban young had lower mass, lower plasma calcium, and lower plasma protein (Heiss et al., 2009). Extensive research on “developmental stress” in songbirds, including our study species of song sparrows (Melospiza melodia), has shown that limited nutrition during critical periods of growth can compromise nestling survival and have life-long effects on body condition, behavior, and fitness (Gil et al., 2019; Wingfield et al., 2017; Peters et al., 2014; Maness and Anderson, 2013; Schmidt et al., 2012; Spencer and MacDougall-Shackleton, 2011; Monaghan, 2008; Cockburn, 2006; MacDonald et al., 2006; Searcy et al., 2004; Nowicki et al., 2002; Martin, 1987; Ricklefs, 1979). Though caloric limitation alone can impair nestling development, protein limitation is especially harmful (Plavnik and Hurwitz, 1989; Ricklefs, 1979) and could result from decreased arthropod availability in urban habitats (Chandel, 2021; Wu et al., 2012).
While overall protein limitation can impair growth, deficits of the essential amino acids, which cannot be synthesized and must be acquired through diet, have consequences such as reduced body size, developmental deformities, and death (Alagawany et al., 2021; Baker, 2009; Murphy and Pearcy, 1993; Almquist, 1952). The essential amino acids, and especially lysine, methionine, and arginine, are also important for muscle development and growth as they have been found to be the most growth-limiting amino acids in birds (Langlois and McWilliams, 2021; Chen et al., 2013; Tesseraud et al., 2001; Klasing, 1998). Other amino acids are not considered essential because they can be synthesized, but supplementation studies suggest they have benefits for early development and songbirds are sensitive to the limitation of these amino acids (Langlois and McWilliams, 2021). Animals also benefit from non-essential amino acids obtained through diet as they can supplement endogenous synthesis to meet physiological demands (Wu et al., 2012). Though most of the work on amino acids in birds has focused on laboratory or captive studies, one study in herring gulls found differences in adult body condition and different amino acid profiles across sites with variable food resources (Hebert et al., 2002). Expanding studies of how environmental conditions are associated with plasma amino acid profiles of free-living birds, especially in the context of urbanization and brood parasitism, may advance understanding of how this mechanism could link ecological and physiological states.
Our long-term study system of urban and rural song sparrows (described in Davies and Sewall, 2016) presents a unique opportunity to investigate how higher brood parasite prevalence (Lane et al., 2023) and lower arthropod biomass at our urban sites (VanDiest et al., 2024) impact nestling growth, condition, and amino acid profiles. To examine how differences in brood parasitism and arthropod availability associated with urbanization affect physiological markers of condition and the development of urban song sparrow nestlings, we performed two studies. First, we used a multi-year study of nestling song sparrow growth and development at our urban and rural sites to determine if urbanization and/or brood parasitism compromised growth, measured as the change in morphometrics over the first 10 days of development. In our second study, we compared free amino acid concentrations in plasma collected from song sparrow nestlings in rural nests without brood parasites, urban nests without brood parasites, and urban nests with brood parasites (rural nests are rarely parasitized and therefore could not be included in comparisons; Lane et al., 2023). We predicted that brood-parasitized urban nestlings would have the slowest growth and the lowest concentrations of circulating amino acids in plasma, while rural nestlings would have the fastest growth and highest concentrations of amino acids. This approach aimed to resolve how two facets of urban ecology, increased brood parasitism and reduced availability of arthropod prey, are associated with nestling amino acid profiles, as well as growth and condition.
2 Methods
2.1 Field site selection
We conducted our studies across 3 urban and 3 rural sites in Southwest Virginia from 2017 to 2022. Our study sites were determined to be the most urban or most rural sites near Blacksburg, Virginia using methods described in Seress et al., 2014 (for more details please see Davies et al., 2018; Goodchild et al., 2022, and VanDiest et al., 2024).
2.2 Nestling measurements
2.2.1 Nestling morphometrics
We located nests using behavioral observations and systematic searching from mid-April through early July during the breeding seasons of 2017–2022 (Lane et al., 2023; Martin and Geupel, 1993). We took measurements from all nestlings present during each visit to the nest. We typically measured nestlings on days 0–1 (n = 159), 5–6 (n = 244), and 8–9 (n = 163). However, some nestlings were measured on day 2 (n = 91), day 3 (n = 102), day 4 (n = 76), day 7 (n = 54), and day 10 (n = 44). Only nestlings that were measured more than once were included in our analysis of growth (n = 348). This sample size included 89 rural nestlings from 32 nests, 183 urban nestlings from 61 nests without brood parasites, and 78 urban nestlings that experienced brown-headed cowbird brood parasitism from 35 nests. Rural nests rarely experienced brood parasitism in our system (n = 2), and therefore, brood parasitized rural nests were excluded as a nestling group. We took measurements of nestling body mass, tarsus length, wing chord, and pin feather length using a digital scale (Pesola digital pocket scale item # MS500) to the nearest hundredth of a gram, or digital calipers (Fisherbrand™ Traceable™ digital carbon fiber calipers) to the nearest tenth of a mm. To account for the difficulty in measuring nestling tarsus and wing chord, five repeat measurements were taken per visit for each metric, the highest and lowest values were dropped, and the three middle values were averaged. Each of these measurements were meant to represent a different metric of growth or development (Cheng and Martin, 2012). We used body mass as it is a measure that incorporates “everything” as a metric of size and alludes to condition. We then used tarsus as a measure of structural growth, and the wing chord and pin were meant to be metrics of readiness to fledge, as wing and pin development have been shown to predict survival of the post fledging period (Jones et al., 2020; Martin et al., 2018). All nestlings were marked upon first measurement with a colored marker (typically day 0–2) so that individuals could be identified for subsequent measurements and tracked across the growth period, before being banded (day 5–6).
2.2.2 Nestling plasma amino acid sampling
We took blood samples (approximately 50µl) from a sub-group of nestlings in 2020–2022 for analysis of plasma amino acid concentrations. This sample size included 13 rural nestlings from 10 nests, 15 urban nestlings from 15 nests without brood parasites, and 15 urban nestlings that experienced brown-headed cowbird brood parasitism from 14 nests. Again, rural nests with brood parasitism were rare (n = 2), so we excluded this nestling group. We collected blood samples via brachial venipuncture with a 26 gauge needle when nestlings were 9 days old +/− 1 day (2 urban with cowbird brood parasites on day 8, 1 rural nestling day 8, 3 urban nestlings without brood parasites day 10). We performed all blood collection between 0655 and 1232 hours from May 10th to July 25th each year. We took blood samples from all nestlings measured in each nest once they reached appropriate age, but due to the difficulty in obtaining rural samples, only a subset of nestlings sampled were used in order to match sample sizes by group. All animals were handled under the relevant federal and state permits from USDS, USFWS, VA DGIF, and IACUC at Virginia Tech.
2.3 Sample preparation
We diluted nestling plasma 1:10 with liquid chromatography-mass spectrometry (LCMS) grade methanol and, after incubating at −20°C, we further diluted the mixture 1:10 with our mobile phase that also contained LCMS grade methanol. We used Sigma-Aldrich amino acid standards (cat.A9906) to establish which amino acids we could detect in our plasma samples and used Cambridge Isotope Laboratories Canonical Amino Acid Mix (MSK-CAA-1) as our internal standards. We used a Raptor Polar X (2.7μM, 100mm x 2.1mm [cat.#9311A12]) column maintained at 35°C, and a Raptor Polar EXP guard column cartridge (2.7μM, 5mm x 2.1mm [cat.#9311A0252]), before quantifying free amino acids in samples using a Shimadzu mass spectrometer. We ran all analytes in positive mode.
Data processing was performed with Lab Solutions V software. We checked peaks and retention times to ensure that the program correctly identified analytes in each sample. Alpha-aminoadipic acid, cystine, homocysteine, hydroxylysine, 3-methylhistidine, beta-alanine, beta-aminoisobutyric acid, and anserine were included in the standards mixture but were not at detectable levels (<0.5 μM) in any of the nestling samples and were thus excluded from analysis. We conducted analyses on a remaining panel of 27 amino acids and their derivatives. For samples with values below the lower detection limit, we set their values to one-half of the lower detection limit for the given amino acid.
2.4 Statistical analysis
2.4.1 Nestling growth statistical analysis – long term study
All statistics were carried out in Program R (R Core Team, 2023, number 4.3.1). To determine if habitat type affected change in morphometrics over time (i.e., growth), we ran four linear mixed effects models (LMMs) with either body mass, tarsus length, average wing chord, or pin feather length as response variables using the ‘lme4’ package (version 1.1-34; Bates et al., 2014). Significance was tested with the package ‘lmerTest’ (Kuznetsova et al., 2017), and all model assumptions were checked with the ‘check_model’ function in the ‘performance’ package (Lüdecke et al., 2021). Nestling group (rural, urban without brood parasite, or urban with brood parasite), age (classified as a continuous variable), and the interaction between nestling group and age were included as fixed effects. “Individual nestling identity” was nested within “nest identity” for random effects to account for the non-independence of samples from the same nest and repeated measures, respectively. However, for the pin feather length model, only nestling identity was included as a random effect to avoid singularity issues with the model.
2.4.2 Nestling growth statistical analysis – amino acid subset
We used similar models to the long term study to assess the changes in the same four morphometrics over time. We once again ran four LMMs with either body mass, tarsus length, wing chord, or pin feather as response variables. Nestling group (rural, urban without brood parasite, or urban with brood parasite), age (classified as a continuous variable), and the interaction between nestling group and age were included as fixed effects. Nest identity was included as the random effect.
2.4.3 Amino acid study statistical analysis
Amino acids can be interrelated because many of them are synthesized from one another. Therefore, to determine if nestlings from different habitats and with different brood parasite status differed in their amino acid panels, we performed a principal component analysis (PCA) including all 27 amino acids and their derivatives. Adequate concentrations of non-essential amino acids can alleviate amino acid deficits during development in addition to the direct consequences a lack of essential amino acids incurs, so all 27 amino acids and their derivatives were included in our analysis. To investigate how the nestling group may affect amino acid composition, we used the resulting first principal component (PC1) as the response variable in a linear model with the nestling group and year as fixed effects.
3 Results
3.1 Nestling growth
3.1.1 Nestling growth – long term study
We found significant differences in growth (i.e., morphometric measures at different time points) among nestling groups based on two of our morphometric measurements (Table 1). Specifically, there was a significant interaction between nestling group and nestling age, indicating that nestlings from different groups changed morphometrics differently over time for body mass and tarsus length, but not for wing chord or pin-feather length (Figure 1; Supplementary Figure S1). Specifically, both urban nestling groups had poorer growth of body mass and tarsus length compared to rural birds.
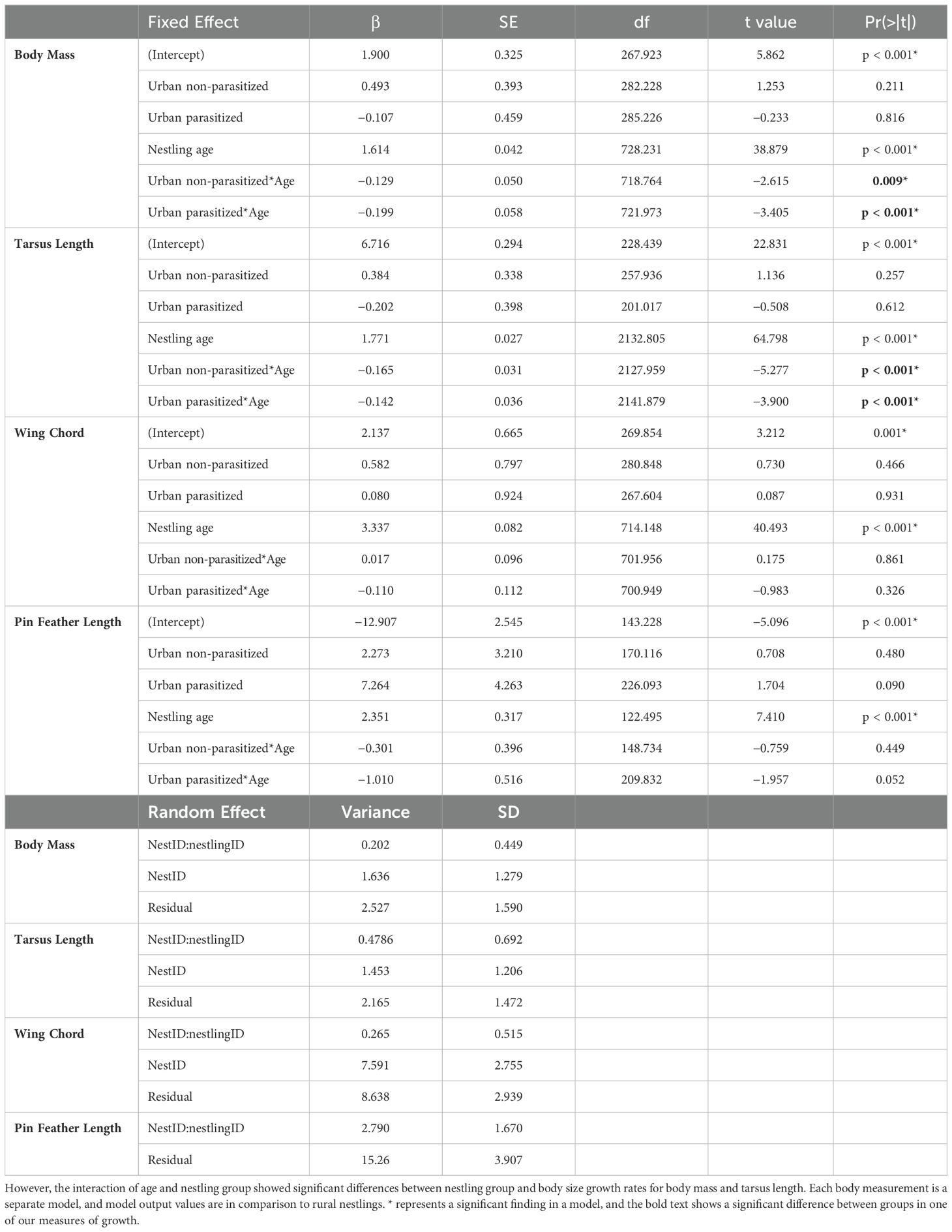
Table 1. In the growth study using the long-term dataset we found no significant differences in body mass, wing chord, or pin feather length by nestling group alone.
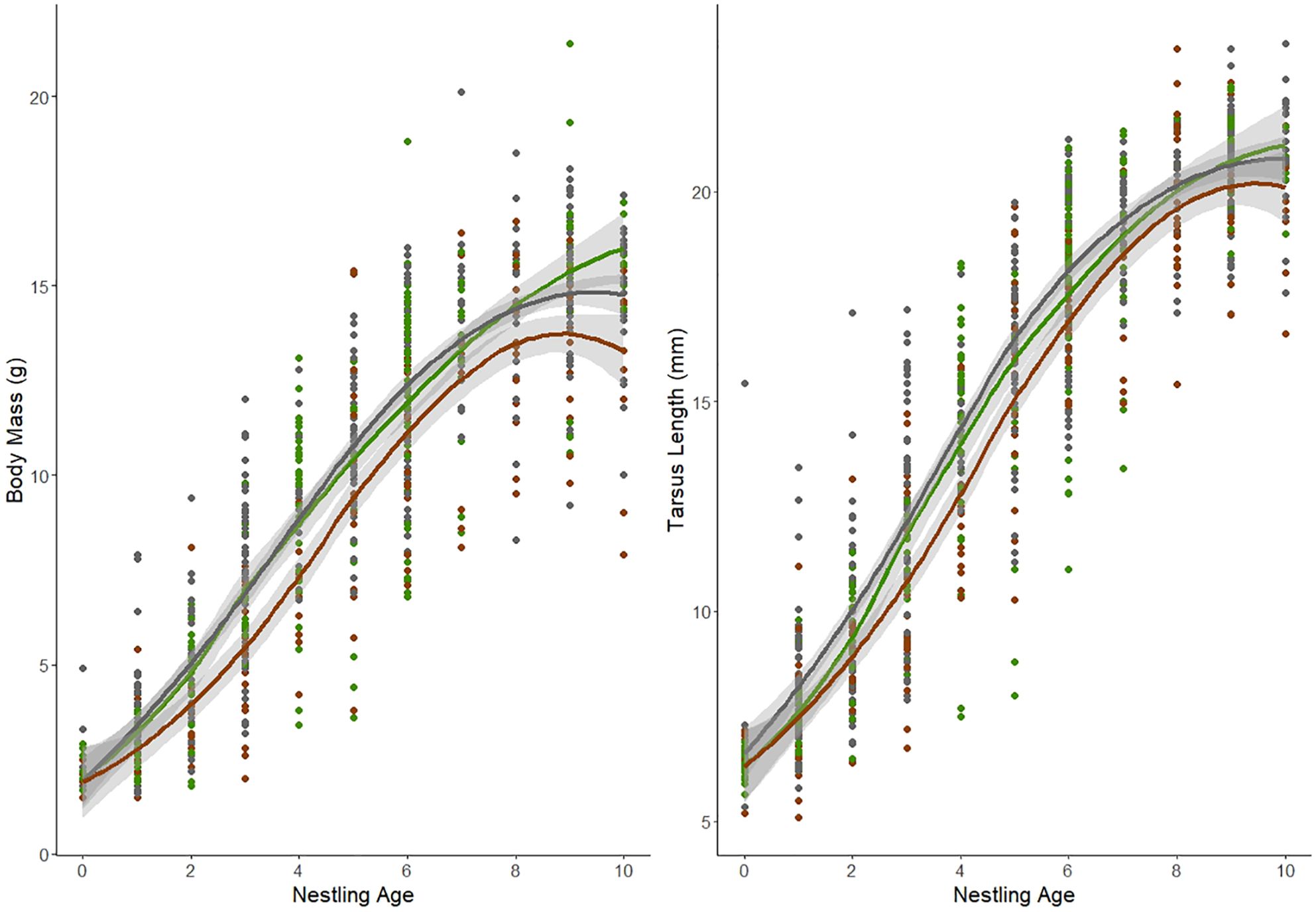
Figure 1. The effects of nestling group and age on body mass and tarsus length for all song sparrow nestlings we measured multiple times from 2017 to 2022. Green represents rural nestlings (n = 89), dark grey represents urban non-brood parasitized nestlings (n = 183), and brown represents urban brood-parasitized nestlings (n = 78). Standard error is represented by the light grey field surrounding each line. Rural nestlings had increased change over time of body mass and tarsus length compared to both urban nestling groups. Wing chord and pin feather length figures can be found in Supplementary Figure S1.
3.1.2 Nestling growth – amino acid subset
We found no significant differences in growth (body mass, tarsus length, wing chord, and pin feather length) by nestling group in the subset of nestlings sampled for amino acids (please see Supplementary Table S1 for the full model outputs).
3.2 Nestling amino acid concentrations
The first principal component for the amino acid profile explained 35.66% of the variation in plasma concentrations of all 27 amino acids and their derivatives (Table 2; Figure 2). Loadings for the first principal component were generally higher in essential amino acids compared to non-essential amino acids (Table 2). Phenylalanine, isoleucine, leucine, proline, valine, and lysine were the highest loading essential amino acids, while tyrosine was the only non-essential amino acid with high loading (Table 2). There were no significant differences among nestling groups in the composite measure of amino acid concentrations when PC1 was used as a response variable in a linear model (Supplementary Table S2: (Urban non-parasitized: β = −0.455, SE = 1.259, t value = −0.362, Pr(>|t|) = 0.720; Urban parasitized: β = 0.842, SE = 1.200, t value = 0.701, Pr(>|t|) = 0.487). Please see Table 3 for the concentrations of all amino acids for each nestling group.
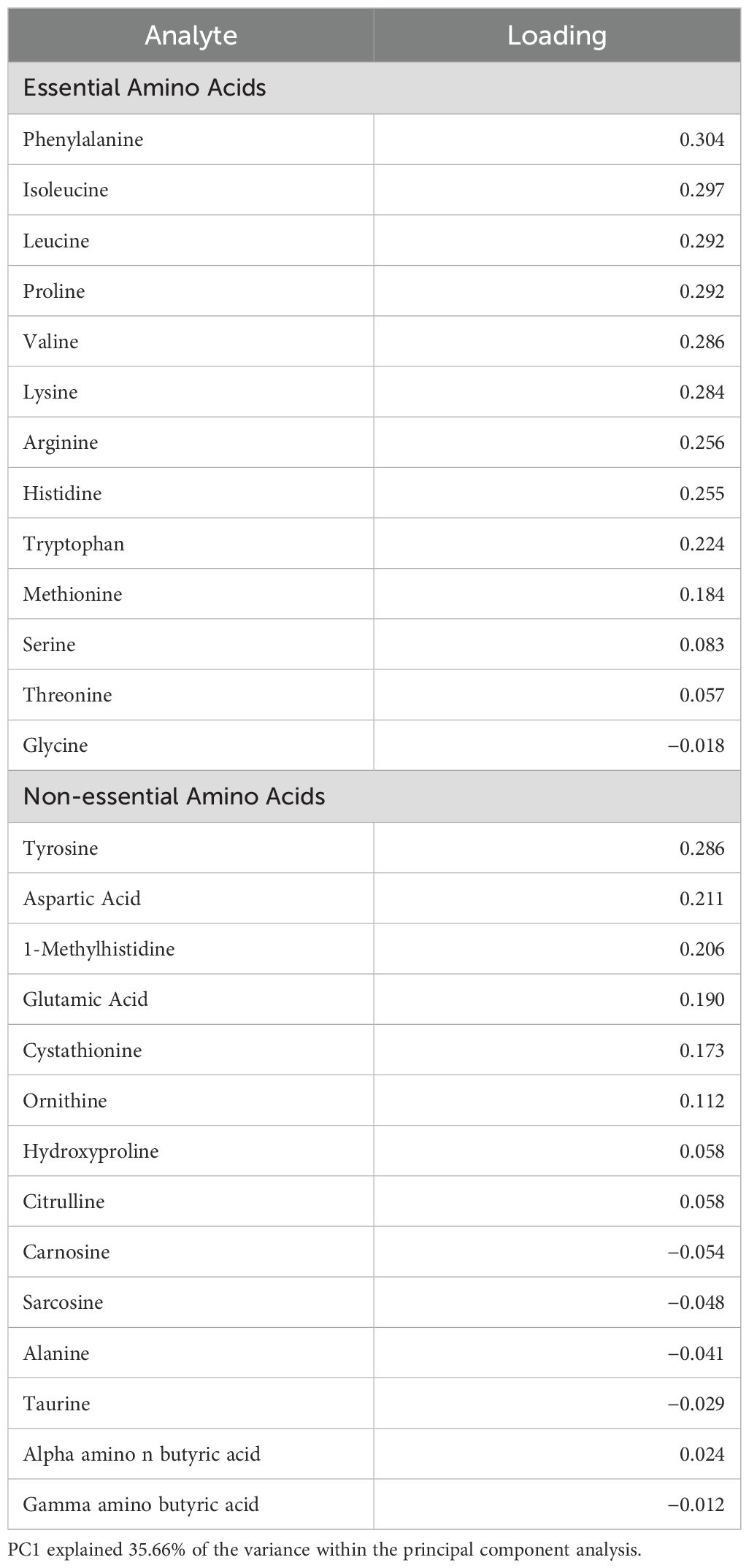
Table 2. Principal component 1 (PC1) loadings from essential and non-essential amino acids and their derivatives in nestlings.
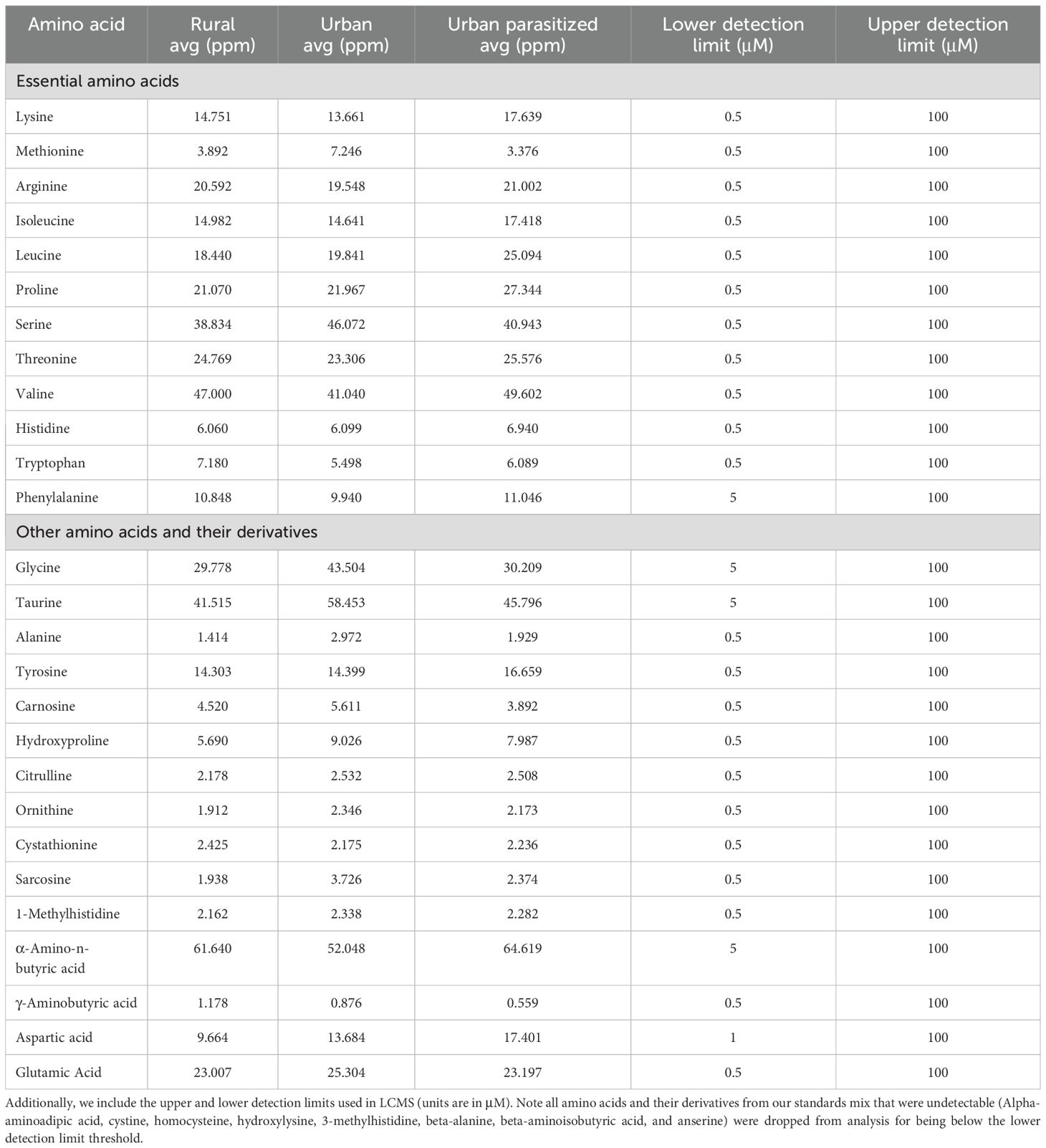
Table 3. The average concentrations of all analytes in our three nestling groups (n=15 for both urban groups and n=13 for rural).
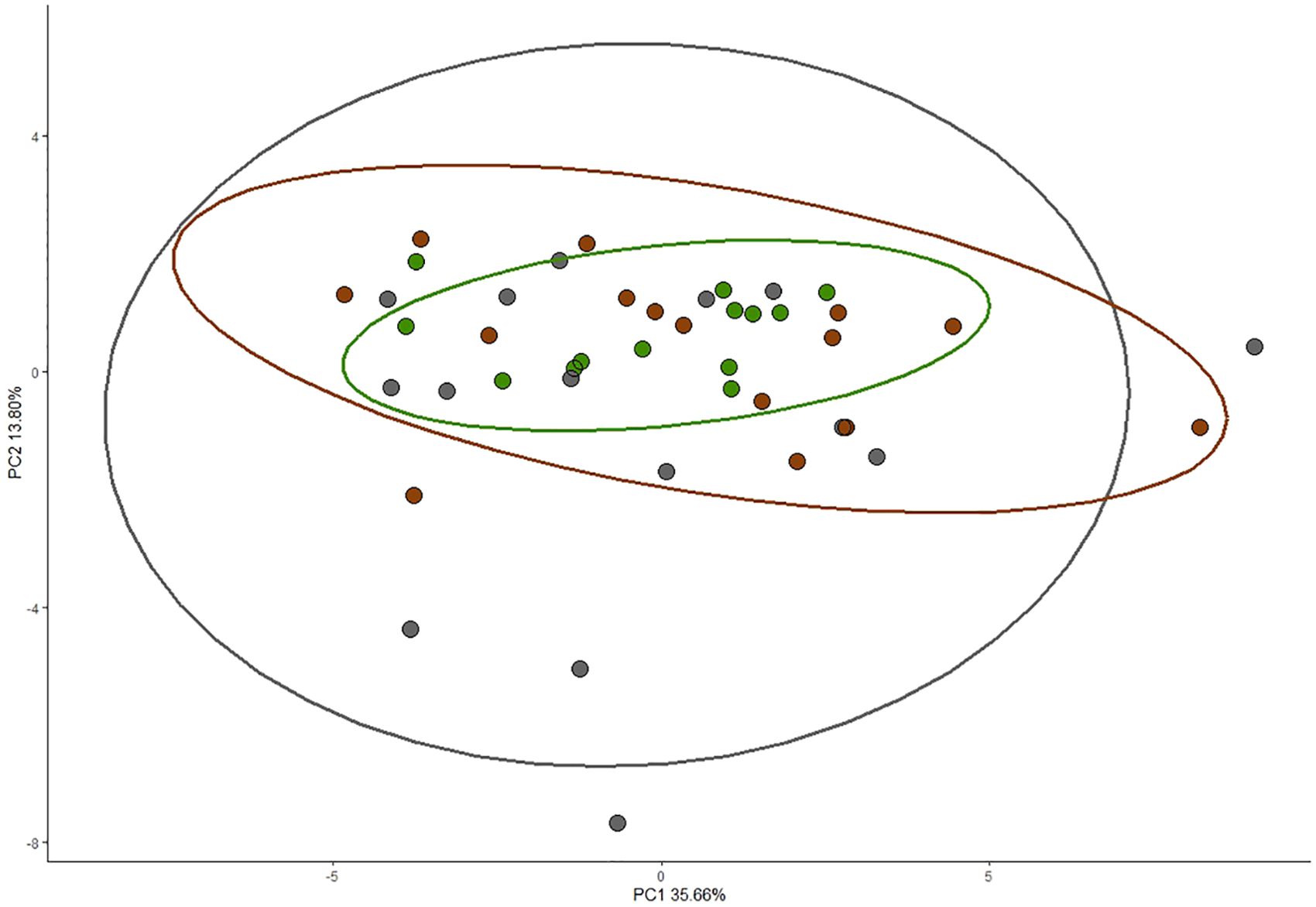
Figure 2. Score plot of all amino acids by nestling group, with ellipses encompassing 95% of the dataset. The closer points are to one another, the more similar a nestling’s free plasma amino acid profiles are. Green represents rural nestlings (n = 13), dark grey represents urban nestlings (n = 15), and brown represents urban brood-parasitized nestlings (n = 15). Principal component one (PC1) explains 35.66% of the variation in this principal component analysis, while principal component two explains 13.80% of the variation. PC1 did not significantly differ by nestling group.
4 Discussion
In our study system of song sparrows, urban sites have higher rates of brood parasitism by brown-headed cowbirds (Lane et al., 2023) and lower arthropod biomass and abundance (VanDiest et al., 2024), raising the possibility that urban nestlings are nutritionally limited. Energy deficits caused by brood parasites or limited nutrition, including protein, can reduce the body size of nestling songbirds (Heiss et al., 2009; Searcy et al., 2004; Nowicki et al., 2002; Ricklefs, 1979; Payne, 1977), presenting an opportunity to examine relationships among facets of urbanization and individual physiology and condition. We hypothesized that differences in brood parasitism and prey availability associated with urbanization could have negative consequences for the growth of nestlings in urban habitats, and that amino acids are one of the mechanisms that mediate these effects. We predicted that urban nestlings from nests with brown-headed cowbird chicks would have the slowest growth (measured as morphometric values over the first 10 days of development) and lowest concentrations of free amino acids in their plasma. Consistent with our predictions, we found that urban nestlings from nests with a brown-headed cowbird brood parasite had significantly lower body mass and tarsus length over time, and a non-significant trend for lower pin feather length over time, indicating slower growth compared to rural nestlings (Figure 1; Table 1). We also found that nestlings from urban nests without brood parasites had lower body mass and tarsus length across time points (i.e., growth) compared to rural nestlings, though not to the same degree as parasitized urban nestlings. This suggests that while urbanization has an effect on growth differences between groups, brood parasitism, which is effectively unique to urban nests in our system, is the larger driver of these differences. Though some studies have found no differences between body condition or growth of urban and rural nestlings (Heppner et al., 2023), our findings are consistent with several other studies reporting slower growth and lower body size or condition in nestling and fledgling songbirds in urban environments [great tits (Parus major; Corsini et al., 2020; Seress et al., 2018; Bailly et al., 2016), blue tits (Cyanistes caeruleus; Corsini et al., 2020; Bailly et al., 2016), house sparrows (Passer domesticus; Seress et al., 2012; Meillere et al., 2015), red-winged starlings (Onychognathus morio; Catto et al., 2021), and American crows (Corvus brachyrhynchos; Heiss et al., 2009)]. We saw significant differences in tarsus and mass development between both urban groups and the rural group (Figure 1), but not in wing development which was a nonsignificant trend (Supplementary Figure S1). This could be due to differential investment in growth metrics by nestlings. With limited access to nutrients, nestlings may invest the resources they have in aspects of growth that are more important to survival. Therefore, nestlings in the parasitized and urban groups could be investing more in wing and feather development, rather than structural size or body mass, because wing and pin development at fledge has been shown to predict survival of the post fledging period (Jones et al., 2020; Martin et al., 2018). Additionally, decreasing body size while increasing wing development could increase nimbleness, aiding in predator avoidance (Macleod et al., 2005). However, reduced body mass at fledge results in lower nestling energy reserves, so this increased agility comes at a cost. Reduced energy reserves demand increased foraging time for fledglings and adults, exposing both to potential predators.
There is little information about minimum amino acid requirements for nestling songbirds, but it is unlikely the birds in our study experienced deficits as they did not display pathologies associated with amino acid limitation (i.e., deformities or death) and mean concentrations of our composite amino acid metric did not vary significantly among groups (Table 3; Supplementary Figure S2). However, studies in chickens suggest that, very generally, “more is better” for the essential amino acids lysine, methionine, and arginine, which are associated with enhanced muscle development and growth (Langlois and McWilliams, 2021; Chen et al., 2013; Tesseraud et al., 2001; Klasing, 1998). Further, a study in wild herring gulls found that birds from colonies with less protein-rich prey had lower concentrations of plasma amino acids and lower body condition (Larus argentatus; Hebert et al., 2002), though the differences in life history and physiology between gulls and sparrows should be considered to avoid specious assumptions.
It is possible that we failed to find differences in amino acid profiles because, though we found differences in morphometrics in nestlings from different groups in our larger study (Figure 1), we could only measure plasma amino acids in a subset of nestlings, and this reduced sample size may limit detection of more subtle differences. A smaller sample size obscuring our findings is supported by the subset of nestlings measured for the amino acid study not having any significant differences in growth (Supplementary Table S1). Though we conclude that nestlings in urban habitats, even with cowbird brood parasites in their nests, had adequate amino acid availability, survivorship bias should be considered when interpreting our results. Specifically, because we sampled song sparrow nestlings for the amino acid study after monitoring their growth and just before they fledged, we did not sample nestlings that died, and thus we would not have detected pathologically low amino acid levels. Our failure to detect differences in amino acids among nestling groups cannot rule out the possibility that younger song sparrow nestlings, or nestlings that did not survive, differed in plasma amino acid concentrations at earlier points in development. Collectively, our study suggests that free amino acid concentrations may only be depleted in plasma when an animal is experiencing severe protein deficits, making comparisons of plasma amino acids most relevant when nestlings are at risk of starvation.
5 Conclusions
The growing field of urban ecophysiology will be advanced by placing biomarkers and measures of individual condition in the context of ecological variation examined by urban ecology (Isaksson et al., 2024; Sumasgutner et al., 2023; Iglesias-Carrasco et al., 2020; Isaksson, 2020; Monaghan, 2008; Shochat et al., 2006). Additionally, studies of the effects of urbanization on individuals that are most vulnerable to environmental variation, such as developing young, may provide insights into the effects of anthropogenic environmental change on the health of wildlife (Bailly et al., 2016; Seress et al., 2012). In this study, we hypothesized that nestlings in urban habitats, which face higher rates of brood parasitism by brown-headed cowbirds and lower availability of arthropod prey, may have slower growth, and such slower growth might be associated with lower plasma amino acids, an essential nutrient that supports growth. Consistent with several other studies (Lorenzana and Sealy, 1999; Dearborn et al., 1998; Payne, 1998, 1977), we found that urban nestlings from nests with brood parasites grew more slowly (Figure 1). Contrary to our predictions and a previous study on plasma protein in urban nestling crows (Heiss et al., 2009), we did not find differences in nestling amino acid profiles (Figure 2). Though a larger sample size that includes younger nestlings that may not survive to fledge, or a study system with greater nutrient limitation might detect variation in plasma amino acids, our current results do not support the hypothesis that amino acid availability is a mechanistic link between ecological conditions and individual health. Our findings do, however, encourage future work linking urban ecology and ecophysiology by pursuing biometrics that could serve as mechanisms linking individual health to ecological variation including urbanization.
Data availability statement
The datasets presented in this study can be found in online repositories. The names of the repository/repositories and accession number(s) can be found below: https://github.com/vandiesti/Amino-Acid-Code.
Ethics statement
The animal study was approved by Institutional Animal Care and Use Committee at Virginia Tech. The study was conducted in accordance with the local legislation and institutional requirements.
Author contributions
IV: Conceptualization, Data curation, Formal analysis, Funding acquisition, Investigation, Methodology, Project administration, Writing – original draft, Writing – review & editing, Visualization. SL: Conceptualization, Data curation, Investigation, Methodology, Project administration, Writing – original draft, Writing – review & editing. TF: Data curation, Investigation, Methodology, Project administration, Writing – review & editing, Conceptualization. KS: Conceptualization, Funding acquisition, Methodology, Project administration, Resources, Supervision, Writing – original draft, Writing – review & editing.
Funding
The author(s) declare that financial support was received for the research and/or publication of this article. This work was supported by the National Science Foundation (Grant numbers IOS 1652496 and IOS 2114288 to KBS) and funding independently obtained by IJV from The Society for Integrative and Comparative Biology, the Global Change Center at Virginia Tech, the Virginia Tech Ralph E. Carlson Memorial Scholarship in Ornithology, and the Virginia Society of Ornithology.
Acknowledgments
We would like to thank W. Hopkins, L. Belden, and E. Gilbert for their guidance with study design and feedback throughout this study. Additionally, R. Helm and S. Hildreth at the Virginia Tech core facilities were endlessly helpful with LCMS method creation and data collection. We also thank C. Moore, J. Green, and C. Tury for their assistance with nest searching and sample collection. Additionally, we thank C McLaughlin for comments and support.
Conflict of interest
The authors declare that the research was conducted in the absence of any commercial or financial relationships that could be construed as a potential conflict of interest.
Generative AI statement
The author(s) declare that no Generative AI was used in the creation of this manuscript.
Publisher’s note
All claims expressed in this article are solely those of the authors and do not necessarily represent those of their affiliated organizations, or those of the publisher, the editors and the reviewers. Any product that may be evaluated in this article, or claim that may be made by its manufacturer, is not guaranteed or endorsed by the publisher.
Supplementary material
The Supplementary Material for this article can be found online at: https://www.frontiersin.org/articles/10.3389/fbirs.2025.1566509/full#supplementary-material
References
Alagawany M., Elnesr S. S., Farag M. R., Tiwari R., Yatoo M. I., Karthik K., et al. (2021). Nutritional significance of amino acids, vitamins and minerals as nutraceuticals in poultry production and health–a comprehensive review. Veterinary Q. 41, 1–29. doi: 10.1080/01652176.2020.1857887
Almquist H. J. (1952). Amino acid requirements of chickens and Turkeys—A review. Poultry Sci. 31, 966–981. doi: 10.3382/ps.0310966
Bailly J., Scheifler R., Berthe S., Clément-Demange V., Leblond M., Pasteur B., et al. (2016). From eggs to fledging: Negative impact of urban habitat on reproduction in two tit species. J. Ornithology 157, 377–392. doi: 10.1007/s10336-015-1293-3
Baker D. H. (2009). Advances in protein-amino acid nutrition of poultry. Amino Acids 37, 29–41. doi: 10.1007/s00726-008-0198-3
Bang C., Faeth S. H., Sabo J. L. (2012). Control of arthropod abundance, richness, and composition in a heterogeneous desert city. Ecol. Monogr. 82, 85–100. doi: 10.1890/11-0828.1
Bates D., Mächler M., Bolker B., Walker S. (2014). Fitting Linear Mixed-Effects Models using lme4. J. Stat. Softw. 67, 1–48. doi: 10.18637/jss.v067.i01
Beaulieu M., Costantini D. (2014). Biomarkers of oxidative status: Missing tools in conservation physiology. Conserv. Physiol. 2, 1–16. doi: 10.1093/conphys/cou014
Bennett A. B., Lovell S. T. (2014). A comparison of arthropod abundance and arthropod mediated predation services in urban green spaces. Insect Conserv. Diversity 7, 405–412. doi: 10.1111/icad.2014.7.issue-5
Bonier F. (2012). Hormones in the city: Endocrine ecology of urban birds. Hormones Behav. 61, 763–772. doi: 10.1016/j.yhbeh.2012.03.016
Boonekamp J. J., Mulder G. A., Salomons H. M., Dijkstra C., Verhulst S. (2014). Nestling telomere shortening, but not telomere length, reflects developmental stress and predicts survival in wild birds. Proc. R. Soc. B: Biol. Sci. 281. doi: 10.1098/rspb.2013.3287
Burhans D. E., Thompson F. R. (2006). Songbird abundance and parasitism differ between urban and rural shrublands. Ecol. Appl. 16, 394–405. doi: 10.1890/04-0927
Buxton V. L., Santymire R. M., Benson T. J. (2018). Mixed effects of urbanization on density, nest survival, and nestling corticosterone of a generalist passerine. Ecosphere 9. doi: 10.1002/ecs2.2517
Catto S., Sumasgutner P., Amar A., Thomson R. L., Cunningham S. J. (2021). Pulses of anthropogenic food availability appear to benefit parents, but compromise nestling growth in urban red-winged starlings. Oecologia 197, 565–576. doi: 10.1007/s00442-021-05033-3
Chace J. F., Walsh J. J., Cruz A., Prather J. W., Swanson H. M. (2003). Spatial and temporal activity patterns of the brood parasitic brown-headed cowbird at an urban/wildland interface. Landsc. Urban Plan. 64. doi: 10.1016/S0169-2046(02)00220-7
Chandel N. S. (2021). Amino acid metabolism. Cold Spring Harbor Perspect. Biol. 13, 1–17. doi: 10.1101/cshperspect.a040584
Chatelain M., Massemin S., Zahn S., Kurek E., Bulska E., Szulkin M. (2021). Urban metal pollution explains variation in reproductive outputs in great tits and blue tits. Sci. Total Environ. 11, 145966. doi: 10.1016/j.scitotenv.2021.145966
Chatelain M., Rüdisser J., Traugott M. (2023). Urban-driven decrease in arthropod richness and diversity associated with group-specific changes in arthropod abundance. Front. Ecol. Evol. 11. doi: 10.3389/fevo.2023.980387
Chen Y. P., Chen X., Zhang H., Zhou Y. M. (2013). Effects of dietary concentrations of methionine on growth performance and oxidative status of broiler chickens with different hatching weight. Br. Poultry Sci. 54, 531–537. doi: 10.1080/00071668.2013.809402
Cheng Y.-R., Martin T. E. (2012). Nest predation risk and growth strategies of passerine species: grow fast or develop traits to escape risk? Am. Nat. 180, 285–295. doi: 10.1086/667214
Cockburn A. (2006). Prevalence of different modes of parental care in birds. Proc. R. Soc. B: Biol. Sci. 273, 1375–1383. doi: 10.1098/rspb.2005.3458
Coogan S. C. P., Raubenheimer D., Zantis S. P., Machovsky-Capuska G. E. (2018). Multidimensional nutritional ecology and urban birds. Ecosphere 9, 1–16. doi: 10.1002/ecs2.2018.9.issue-4
Corsini M., Schöll E. M., Di Lecce I., Chatelain M., Dubiec A., Szulkin M. (2020). Growing in the city: Urban evolutionary ecology of avian growth rates. Evolutionary Appl. 14, 69–84. doi: 10.1111/eva.13081
Davies S., Beck M. L., Sewall K. B. (2018). Territorial aggression in urban and rural Song Sparrows is correlated with corticosterone, but not testosterone. Hormones Behav. 98, 8–15. doi: 10.1016/j.yhbeh.2017.11.010
Davies S., Sewall K. B. (2016). Agonistic urban birds: Elevated territorial aggression of urban song sparrows is individually consistent within a breeding period. Biol. Lett. 12. doi: 10.1098/rsbl.2016.0315
Dearborn D. C., Anders A. D., Thompson F. R. III (1998). Effects of Cowbird Parasitism on Parental Provisioning and Nestling Food Acquisition and Growth. Available online at: https://www.jstor.org/stable/1370273?seq=1&cid=pdf-referencereferences_tab_contents (Accessed April 28, 2024).
Faeth S. H., Bang C., Saari S. (2011). Urban biodiversity: Patterns and mechanisms. Ann. New York Acad. Sci. 1223, 69–81. doi: 10.1111/j.1749-6632.2010.05925.x
Faeth S. H., Warren P. S., Shochat E., Marussich W. A. (2005). Trophic dynamics in urban communities. BioScience 55, 399. doi: 10.1641/0006-3568(2005)055[0399:TDIUC]2.0.CO;2
Fenoglio M. S., Rossetti M. R., Videla M. (2020). Negative effects of urbanization on terrestrial arthropod communities: A meta-analysis. Global Ecol. Biogeography 29, 1412–1429. doi: 10.1111/geb.13107
Fenoglio M. S., Werenkraut V., Morales J. M., Salvo A. (2017). A hierarchical multi-scale analysis of the spatial relationship between parasitism and host density in urban habitats. Austral Ecol. 42, 732–741. doi: 10.1111/aec.2017.42
Gil D., Alfonso-Iñiguez S., Pérez-Rodríguez L., Muriel J., Monclús R. (2019). Harsh conditions during early development influence telomere length in an altricial passerine: Links with oxidative stress and corticosteroids. J. Evolutionary Biol. 32, 111–125. doi: 10.1111/jeb.2019.32.issue-1
Goodchild C. G., VanDiest I. J., Lane S. J., Beck M., Ewbank H., Sewall K. B. (2022). Variation in hematological indices, oxidative stress, and immune function among male song sparrows from rural and low-density urban habitats. Front. Ecol. Evol. 10. doi: 10.3389/fevo.2022.817864
Hebert C. E., Laird Shutt J., Ball R. O. (2002). Plasma amino acid concentrations as an indicator of protein availability to breeding Herring Gulls (Larus argentatus). Auk 119, 185–200. doi: 10.1093/auk/119.1.185
Heiss R. S., Clark A. B., Mcgowan K. J. (2009). Growth and nutritional state of American Crow nestlings Growth urban and rural habitats between vary. Ecol. Appl. 19, 829–839. doi: 10.1890/08-0140.1
Heppner J. J., Krause J. S., Ouyang J. Q. (2023). Urbanization and maternal hormone transfer: Endocrine and morphological phenotypes across ontogenetic stages. Gen. Comp. Endocrinol. 333. doi: 10.1016/j.ygcen.2022.114166
Iglesias-Carrasco M., Aich U., Jennions M. D., Head M. L. (2020). Stress in the city: meta-analysis indicates no overall evidence for stress in urban vertebrates. Proc. R. Soc. B: Biol. Sci. 287, 20201754. doi: 10.1098/rspb.2020.1754
Isaksson C. (2015). Urbanization, oxidative stress and inflammation: A question of evolving, acclimatizing or coping with urban environmental stress. Funct. Ecol. 29, 913–923. doi: 10.1111/1365-2435.12477
Isaksson C. (2018). “Impact of urbanization on birds,” in Bird Species. Fascinating Life Sciences. (Cham: Springer), 235–257. doi: 10.1007/978-3-319-91689-7_13
Isaksson C. (2020). Urban ecophysiology: Beyond costs, stress and biomarkers. J. Exp. Biol. 223, 1–10. doi: 10.1242/jeb.203794
Isaksson C., Ziegler A-K., Powell D., Gudmundsson A., Andersson M. N., Rissler J. (2024). Transcriptome analysis of avian livers reveals different molecular changes to three urban pollutants: Soot, artificial light at night and noise. Environ. Pollution 358, 1–11. doi: 10.1016/j.envpol.2024.124461
Jones T. M., Benson T. J., Ward M. P. (2020). Does the size and developmental stage of traits at fledging reflect juvenile flight ability among songbirds? Funct. Ecol. 34, 799–810. doi: 10.1111/1365-2435.13513
Kuznetsova A., Brockhoff P. B., Christensen R. H. B. (2017). lmerTest package: tests in linear mixed effects models. J. Stat. Software 82, 1–26. doi: 10.18637/jss.v082.i13
Ladin Z. S., D’Amico V., Jaisi D. P., Shriver W. G. (2015). Is brood parasitism related to host nestling diet and nutrition? Auk 132, 717–734. doi: 10.1642/AUK-15-11.1
Lane S. J., VanDiest I. J., Brewer V. N., Linkous C. R., Fossett T. E., Goodchild C. G., et al. (2023). Indirect effects of urbanization: consequences of increased aggression in an urban male songbird for mates and offspring. Front. Ecol. Evol. 11. doi: 10.3389/fevo.2023.1234562
Langlois L. A., McWilliams S. R. (2021). Essential amino acid requirements of granivorous and omnivorous songbirds and the provision of natural foods. J. Ornithology. 163, 151–163. doi: 10.1007/s10336-021-01915-8
Lorenzana J. C., Sealy S. G. (1999). A meta-analysis of the impact of parasitism by the brown-headed cowbird on its hosts. Stud. Avian Biol. 18, 241–253.
Lüdecke D., Ben-Shachar M., Patil I., Waggoner P., Makowski D. (2021). performance: an R package for assessment, comparison and testing of statistical models. J. Open Source Software 6, 3139. doi: 10.21105/joss.03139
MacDonald I. F., Kempster B., Zanette L., MacDougall-Shackleton S. A. (2006). Early nutritional stress impairs development of a song-control brain region in both male and female juvenile song sparrows (Melospiza melodia) at the onset of song learning. Proc. R. Soc. B: Biol. Sci. 273, 2559–2564. doi: 10.1098/rspb.2006.3547
Macleod R., Barnett P., Clark J. A., Cresswell W. (2005). Body mass change strategies in blackbirds Turdus merula: The starvation-predation risk trade-off. J. Anim. Ecol. 74, 292–302. doi: 10.1111/j.1365-2656.2005.00923.x
Maness T. J., Anderson D. J. (2013). Predictors of juvenile survival in birds. Ornithological Monogr. 78, 1–55. doi: 10.1525/om.2013.78.1.1
Martin T. (1987). Food as a limit on breeding birds: A life-history perspective. Annu. Rev. Ecology Evolution Systematics 18, 453–487. doi: 10.1146/annurev.es.18.110187.002321
Martin T. E., Geupel G. R. (1993). Nest-Monitoring Plots: Methods for Locating Nests and Monitoring Success (Métodos para localizar nidos y monitorear el éxito de estos). Source: J. Field Ornithology 64, 507–519. Available online at: http://www.jstor.org/stable/4513862 (Accessed November 20, 2024).
Martin T. E., Tobalske B., Riordan M. M., Case S. B., Dial K. P. (2018). Age and performance at fledging are a cause and consequence of juvenile mortality between life stages. Sci. Adv. 4, 1–8. doi: 10.1126/sciadv.aar1988
Martin A. C., Zim H., Neilson A. (1951). American Wildlife & Plants A Guide to Wildlife Food Habits. (New York, NY: McGraw-Hill Book Company, Inc.).
Martinson H., Raupp M. (2013). A meta-analysis of the effects of urbanization on ground beetle communities. Ecosphere 4, 1–24. doi: 10.1890/ES12-00262.1
McEwen B. S., Wingfield J. C. (2003). The concept of allostasis in biology and biomedicine. Hormones Behav. 43, 2–15. doi: 10.1016/S0018-506X(02)00024-7
McKinney M. L. (2002). Urbanization, biodiversity, and conservation. BioScience 52, 883–890. doi: 10.1641/0006-3568(2002)052[0883:UBAC]2.0.CO;2
Meillere A., Brischoux F., Parenteau C., Angelier F. (2015). Influence of urbanization on body size, condition, and physiology in an urban exploiter : A multi-component approach. PloS One 10, 1–19. doi: 10.1371/journal.pone.0135685
Monaghan P. (2008). Early growth conditions, phenotypic development and environmental change. R. Soc. B 363, 1635–1645. doi: 10.1098/rstb.2007.0011
Monros J. S., Belda E. J., Barba E. (2003). Post-fledging survival of individual great tits: the effect of hatching date and fledging mass. Oikos 99, 481–488. doi: 10.1034/j.1600-0706.2002.11909.x
Murphy M. E., Pearcy S. D. (1993). Dietary amino acid complementation as a foraging strategy for wild birds. Physiol. Behav. 53, 689–698. doi: 10.1016/0031-9384(93)90175-F
Nowicki S., Searcy W. A., Peters S. (2002). Brain development, song learning and mate choice in birds: A review and experimental test of the “nutritional stress hypothesis. J. Comp. Physiol. A: Neuroethology Sensory Neural Behav. Physiol. 188, 1003–1014. doi: 10.1007/s00359-002-0361-3
Partecke J., Schwabl I., Gwinner E. (2006). Stress and the city: urbanization and its effects on the stress physiology in European blackbirds. Ecology 87, 1945–1952. doi: 10.1890/0012-9658(2006)87[1945:SATCUA]2.0.CO;2
Payne R. B. (1977). The ecology of brood parasitism in birds. Annu. Rev. Ecology Evolution Systematics. 8, 1–28. doi: 10.1146/annurev.es.08.110177.000245
Payne R. (1998). Brood parasitism in birds: strangers in the nest. BioScience 48, 377–386. doi: 10.2307/1313376
Peters S., Searcy W. A., Nowicki S. (2014). “Developmental stress, song-learning, and cognition,” in Integrative and Comparative Biology, vol. 54. (Oxford University Press), 555–567.
Plavnik I., Hurwitz S. (1989). Effect of dietary protein, energy, and feed pelleting on the response of chicks to early feed restriction. Poultry Sci. 68, 1118–1125. doi: 10.3382/ps.0681118
Ramsay S. L., Houston D. C. (2003). Amino acid composition of some woodland arthropods and its implications for breeding tits and other passerines. Ibis 145, 227–232. doi: 10.1046/j.1474-919X.2003.00133.x
Razeng E., Watson D. M. (2015). Nutritional composition of the preferred prey of insectivorous birds: Popularity reflects quality. J. Avian Biol. 46, 89–96. doi: 10.1111/jav.2015.v46.i1
R Core Team (2023). R: A Language and Environment for Statistical Computing. Vienna, Austria: R foundation for Statistical Computing.
Reid R., Capilla-Lasheras P., Haddou Y., Boonekamp J., Dominoni D. M. (2024). The impact of urbanization on health depends on the health metric, life stage and level of urbanization: A global meta-analysis on avian species. Proc. R. Soc. B: Biol. Sci. 291. doi: 10.1098/rspb.2024.0617
Ricklefs R. E. (1979). Adaptation, constraint, and compromise in avian postnatal development. Biol. Rev. Cambridge Philos. Soc. 54, 269–290. doi: 10.1111/j.1469-185x.1979.tb01013.x
Rodewald A. (2009). Urban-associated habitat alteration promotes brood parasitism of Acadian Flycatchers. J. Field Ornithology 80, 234–241. doi: 10.1111/j.1557-9263.2009.00226.x
Rodewald A. D., Kearns L. J., Shustack D. P. (2013). Consequences of urbanizing landscapes to reproductive performance of birds in remnant forests. Biol. Conserv. 160, 32–39. doi: 10.1016/j.biocon.2012.12.034
Schmidt K. L., MacDougall-Shackleton E. A., MacDougall-Shackleton S. A. (2012). Developmental stress has sex-specific effects on nestling growth and adult metabolic rates but no effect on adult body size or body composition in song sparrows. J. Exp. Biol. 215, 3207–3217. doi: 10.1242/jeb.068965
Searcy W. A., Peters S., Nowicki S. (2004). Effects of early nutrition on growth rate and adult size in song sparrows Melospiza melodia. J. Avian Biol. 35, 269–279. doi: 10.1111/j.0908-8857.2004.03247.x
Seress G., Bókony V., Pipoly I., Szép T., Nagy K., Liker A. (2012). Urbanization, nestling growth and reproductive success in a moderately declining house sparrow population. J. Avian Biol. 43, 403–414. doi: 10.1111/j.1600-048X.2012.05527.x
Seress G., Hammer T., Bókony V., Vincze E., Preiszner B., Pipoly I., et al. (2018). Impact of urbanization on abundance and phenology of caterpillars and consequences for breeding in an insectivorous bird. Ecol. Appl. 28, 1143–1156. doi: 10.1002/eap.2018.28.issue-5
Seress G., Lipovits Á., Bókony V., Czúni L. (2014). Quantifying the urban gradient: A practical method for broad measurements. Landscape Urban Plann. 131, 42–50. doi: 10.1016/j.landurbplan.2014.07.010
Seto K. C., Güneralp B., Hutyra L. R. (2012). Global forecasts of urban expansion to 2030 and direct impacts on biodiversity and carbon pools. Proc. Natl. Acad. Sci. United States America 109, 16083–16088. doi: 10.1073/pnas.1211658109
Shochat E., Warren P. S., Faeth S. H., McIntyre N. E., Hope D. (2006). From patterns to emerging processes in mechanistic urban ecology. Trends Ecol. Evol. 21, 186–191. doi: 10.1016/j.tree.2005.11.019
Spencer K. A., MacDougall-Shackleton S. A. (2011). Indicators of development as sexually selected traits: The developmental stress hypothesis in context. Behav. Ecol. 22, 1–9. doi: 10.1093/beheco/arq068
Sumasgutner P., Cunningham S. J., Hegemann A., Amar A., Watson H., Nilsson J. F., et al. (2023). Interactive effects of rising temperatures and urbanisation on birds across different climate zones: A mechanistic perspective. Global Change Biol. 29, 2399–2420. doi: 10.1111/gcb.16645
Tesseraud S., Temim S., le Bihan-Duval E., Chagneau A. M. (2001). Increased responsiveness to dietary lysine deficiency of pectoralis major muscle protein turnover in broilers selected on breast development. J. Anim. Sci. 79, 927–933. doi: 10.2527/2001.794927x
Tewksbury J. J., Garner L., Garner S., Lloyd J. D., Saab V., Martin T. E. (2006). Tests of landscape influence: nest predation and brood parasitism in fragmented ecosystems. Ecology 87, 759–768. doi: 10.1890/04-1790
VanDiest I. J., Jones K. R., Fossett T. E., Lane S. J., Sewall K. B. (2024). Differences in urban arthropod communities may not limit the nestling diet of a generalist songbird. Sci. Total Environ. 954, 176518. doi: 10.1016/j.scitotenv.2024.176518
Wingfield J. C., Pérez J. H., Krause J. S., Word K. R., González-Gómez P. L., Lisovski S., et al. (2017). How birds cope physiologically and behaviourally with extreme climatic events. Philos. Trans. R. Soc. B: Biol. Sci. 372. doi: 10.1098/rstb.2016.0140
Keywords: urbanization, nutrition, songbirds, brood parasitism, amino acids, nestling growth
Citation: VanDiest IJ, Lane SJ, Fossett TE and Sewall KB (2025) Urbanization and brood parasitism affect growth, but not free amino acid concentrations in nestling song sparrows (Melospiza melodia). Front. Bird Sci. 4:1566509. doi: 10.3389/fbirs.2025.1566509
Received: 24 January 2025; Accepted: 02 April 2025;
Published: 25 April 2025.
Edited by:
Melissa Lin Grunst, Indiana State University, United StatesReviewed by:
Pierre J. Deviche, Arizona State University, United StatesMiroslav Šálek, Czech University of Life Sciences Prague, Czechia
Copyright © 2025 VanDiest, Lane, Fossett and Sewall. This is an open-access article distributed under the terms of the Creative Commons Attribution License (CC BY). The use, distribution or reproduction in other forums is permitted, provided the original author(s) and the copyright owner(s) are credited and that the original publication in this journal is cited, in accordance with accepted academic practice. No use, distribution or reproduction is permitted which does not comply with these terms.
*Correspondence: Isaac J. VanDiest, aXZhbmRpZXN0QHZ0LmVkdQ==
†Present address: Samuel J. Lane, Department of Biological Sciences, North Dakota State University, Fargo, ND, United States