- 1Centro de Investigación en Biodiversidad y Conservación, Universidad Autónoma del Estado de Morelos, Cuernavaca, Mexico
- 2Centro de Investigación en Alimentación y Desarrollo AC, Unidad Guaymas en Aseguramiento de Calidad y Aprovechamiento Sustentable de Recursos Naturales, Guaymas, Sonora, Mexico
- 3Laboratorio de Neuroecología Cognitiva, Facultad de Psicologia, Universidad Nacional Autónoma de México (UNAM), Ciudad Universitaria, Mexico City, Mexico
Heavy metals and metalloids (HM) such as lead, arsenic, cadmium, and mercury are well known to have toxic effects in numerous biological systems. Mining waste is a major source of HM pollution worldwide. Here, we address the effects of HM exposure from living near abandoned mining waste in the Huautla mining district of central Mexico on the exploratory behavior of the streak-backed oriole. Using a field-implemented novel environment test, we quantified individuals’ movements, visual scans, and self-directed behaviors; then, we quantified the concentrations of four toxic (arsenic, lead, mercury, cadmium) and two essential HM (copper, zinc) from their contour feathers. Using principal components analysis to summarize behavioral variables showed that there were three main axes of variation (77% of variance), describing overall activity (32.8%), visual scans versus self-directed behaviors (24.5%), and exploration latency (19.9%). None of these components was affected by any of the HM or interactions among them. This was somewhat surprising, given that we found exceedingly high levels of arsenic and moderate levels of lead, mercury, and copper (though no detectable cadmium or zinc). Further work will be needed to disentangle the relative importance of different HM, temporal mismatch between HM circulating in the body and those measured in feathers, and the possibility of detoxification, adaptation, or selective filters earlier in the life cycle of this population, which has been exposed to mining waste for many generations.
1 Introduction
Metal mining has caused environmental and social disturbance around the world, including in areas of high ecological importance (Durán et al., 2013; Murguía et al., 2016). One of its most far-reaching and lasting impacts is pollution from persistent toxic heavy metals (and metalloids), including lead (Pb), arsenic (As), cadmium (Cd), and mercury (Hg), as well as elevated levels of essential trace metals such as copper (Cu) and zinc (Zn) (Bridge, 2004; Durán et al., 2013). Centuries of mining combined with lax or nonexistent legislation has resulted in numerous sites with accumulations of finely ground waste known as “tailings”. In Mexico, the number of such sites is conservatively estimated in the thousands (Velasco Trejo et al., 2004; Téllez Ramírez and Sánchez-Sálazar, 2018). Furthermore, there is a nearly 1.5 million ha overlap between mining concessions and natural protected areas in the country, and 63% of the nation’s Biosphere Reserves contain mining concessions (Armendáriz-Villegas et al., 2015).
Given the frequency of these contaminated sites and their overlap with wildlands and wildlife, it is important to understand how heavy metals and metalloids (hereafter, HM) from mining waste affect wild birds. In general, it is well known that high doses of HM can be lethal, while even low concentrations can have a wide range of sublethal neurotoxic, genotoxic, and cytotoxic effects, many of which are related to oxidative stress (reviewed by Isaksson, 2010). Wild birds living near mining sites have been repeatedly shown to have elevated tissue HM levels (e.g., Custer et al., 2009; Monzalvo-Santos et al., 2016; Yang et al., 2018; Durkalec et al., 2022). While quantifying HM in tissues is an important first step toward determining exposure, it does not address how that exposure affects individuals’ health, behavior, and ultimately, fitness, especially since for most taxa and HM there are no well-established reference levels. Furthermore, real-world pollution scenarios are complex due to the interplay among multiple contaminants, exposure to other stressors, indirect effects, and even the potential for detoxification and adaptation to HM exposure (see, e.g., reviews by Isaksson, 2010; Sánchez-Virosta et al., 2015).
One important aspect of birds’ lives that has been shown to be affected by HM pollution is their movement behavior, which is related to cognition and energy budgets and can affect resource acquisition and survival. For instance, a telemetry study of wild golden eagles (Aquila chrysaetos) showed that individuals with elevated Pb levels had lower flight distance and height, and there was a positive association between high blood Pb and movement-related causes of death, including starvation and collisions (Ecke et al., 2017). At a smaller scale, great tits (Parus major) exposed to a mixture of HM near a smelting plant in Belgium have lower rates of hops and flights in a novel environment test (Grunst et al., 2018, 2019). The novel environment test is a brief behavioral assay in which an individual is allowed to explore a standardized arena. It is frequently used to characterize movement and exploratory behavior in birds and aspects of avian personality, and it has been shown to be repeatable, heritable, and correlate with ecologically relevant traits in some species (e.g., Dingemanse et al., 2002; Jablonszky et al., 2020). While it has a long history of use in captivity, it is also increasingly implemented directly in the field using portable arenas (e.g., Huang et al., 2016; Jablonszky et al., 2020).
Here, we investigate the potential associations between HM levels and exploratory behavior in a wild bird near abandoned tailings piles in the Huautla mining district of the central Mexican state of Morelos. This site is located in the Sierra de Huautla Biosphere Reserve (REBIOSH), which contains some of the largest expanses of seasonally dry tropical forest in the country and is an Important Bird Area of the Americas (Vidal et al., 2009). Our study species, the streak-backed oriole (Icterus pustulatus, hereafter STRO), offers several advantages as a study system. It is abundant even in disturbed areas and widely distributed from the southern USA through Central America. In central Mexico, it is resident year-round (Jaramillo and Burke, 1999). A previous study at our site found that both sexes defended a small territory (~200 m radius around the nest) during the breeding season, responded to playbacks during the post-breeding season, and were frequently re-sighted in the same area between seasons (Murphy et al., 2009), suggesting relatively low adult dispersal. Thus, HM in STRO tissues can be reasonably expected to be influenced by sources near the location where they are captured. The routes of exposure in this species have not been characterized but may include food (e.g., bioaccumulation in fruits, arthropods, nectar), water, incidental ingestion of contaminated sediment, and/or absorption through respiratory tissues (Mann et al., 2011). A previous study of this species using museum specimens found some associations of plumage color with proximity to mining concessions at a regional scale (Kiere et al., 2021), but there are no previous studies examining the association between feather HM levels and behavior in this species.
Given that this is the first study of STRO in a novel environment test, our first objective was to characterize the main axes of variation in exploratory behavior and evaluate the effects of variables related to individual and testing conditions (age/sex, body mass, time of day, time between capture and testing, and date). Second, we aimed to determine to what extent behavioral variation is explained by feather levels of toxic (Pb, Cd, As, Hg) and/or essential (Cu, Zn) HM. We expected that with increasing levels of toxic HM, individuals’ exploratory behaviors would reflect increasing lethargy and anxiety; thus, with increasing concentrations of Pb, Cd, As, and/or Hg, we expected decreasing movement behaviors (i.e., less time in flight, fewer total flights, and fewer total features visited) and increasing self-directed behaviors (preening/leg band manipulation). We also expected that individuals with higher levels of these HM might spend more time performing visual scans as a less energetically demanding alternative to physically exploring the space (sensu Huang et al., 2016). We considered interactions among HM in order to account for potential synergistic or antagonistic effects.
2 Method
2.1 Study site
Our study area centered on a defunct silver and Pb mine in the Huautla mining district of Morelos, Mexico. This area was mined for centuries, from artisanal mines in the XVI century through high-volume industrial processes in the XX century, until the mine’s abrupt abandonment in 1993 (Velasco Trejo et al., 2004). This has had profound social and environmental impacts, including the presence of ≥780,000 tons of tailings that are highly enriched with several HM (e.g., 7 and 11 times permissible As and Pb in residential soils, respectively; Velasco Trejo et al., 2004). There are at least five known open-air tailings piles, which show evidence of severe erosion (Márquez-Huitzil et al., 2025; Márquez-Huitzil et al., 2022). There are also elevated levels of As and other HM in local water supplies (Esteller et al., 2015) and in tissues of humans (Tóvar-Sanchez et al., 2016), rodents (Tovar-Sánchez et al., 2012), and anurans (Chávez-Ramírez et al., 2024) in the area. The mining district is now encompassed by the REBIOSH, which was decreed in 1999 and consists of a mosaic of well-preserved seasonally dry tropical forest, crop fields, induced grassland used for cattle grazing, small towns, and dirt and paved roads (CONANP, 2005). In order to increase the likelihood of sampling STRO over a wide range of HM levels, we captured birds at four localities: two adjacent to the two largest known tailings piles (“El Portón”: 18°26’24.57” N, 99°1’58.47” W and “Las Presas”: 18°26’6.32” N, 99°1’12.43” W; (Márquez-Huitzil et al., 2022), one ~3 km downstream from the tailings piles along the Huautla Stream (18°25’16.14” N, 99°0’43.05” W), and one ~8 km northeast (upstream) of the tailings sites near the community of Quilamula, which has no known history of mining or mining waste deposits (18°30’43.56” N, 99°0’55.47” W). We alternated among these localities daily to avoid confounding locality with date.
2.2 Field methods
STRO were captured by mist netting in May–June 2017 and February–March 2020 from sunrise to ~12:00 h, intermittently using passive netting, vocal playback and/or taxidermic mounts. Birds were sexed and aged (second-year versus after-second-year) based on tail coloration and wear (Pyle, 1997; Murphy et al., 2009). We banded the birds (one aluminum and three color bands) and measured their wing chord, tail length, tarsus length, and body mass. We took ventral, dorsal, and lateral photographs for a separate study on plumage coloration and a 75 μL blood sample by brachial venipuncture for a separate study on erythrocyte malformations and blood parasites. Finally, we plucked a sample of contour feathers from the crown, throat, breast, and rump. After processing, the individual was either placed directly in the habituation compartment of the novel environment setup (described below), or if the arena was occupied, in a cloth bag to wait on a line in the shade. We noted the time of capture and the time the test was initiated to include this delay as a covariable in our models (delay mean ± SD: 19 ± 17 min). We took several actions to minimize stress and monitor the birds’ condition throughout this time (see Ethics Statement).
The novel environment test arena was adapted from Huang et al. (2016) and is depicted in Figure 1. It consisted of an inner exploration zone of vinyl mosquito netting within a muslin structure supported by a polyvinyl chloride (PVC) frame, and had a wooden box (“habituation compartment”) with a door that could be opened to release the bird into the test arena. The ground was covered with muslin, and the arena contained four artificial perches (PVC with a wooden cross-piece for the perch). This resulted in a total of ten “features” inside the arena: the four walls, ceiling, and floor of the arena, and the four perches.
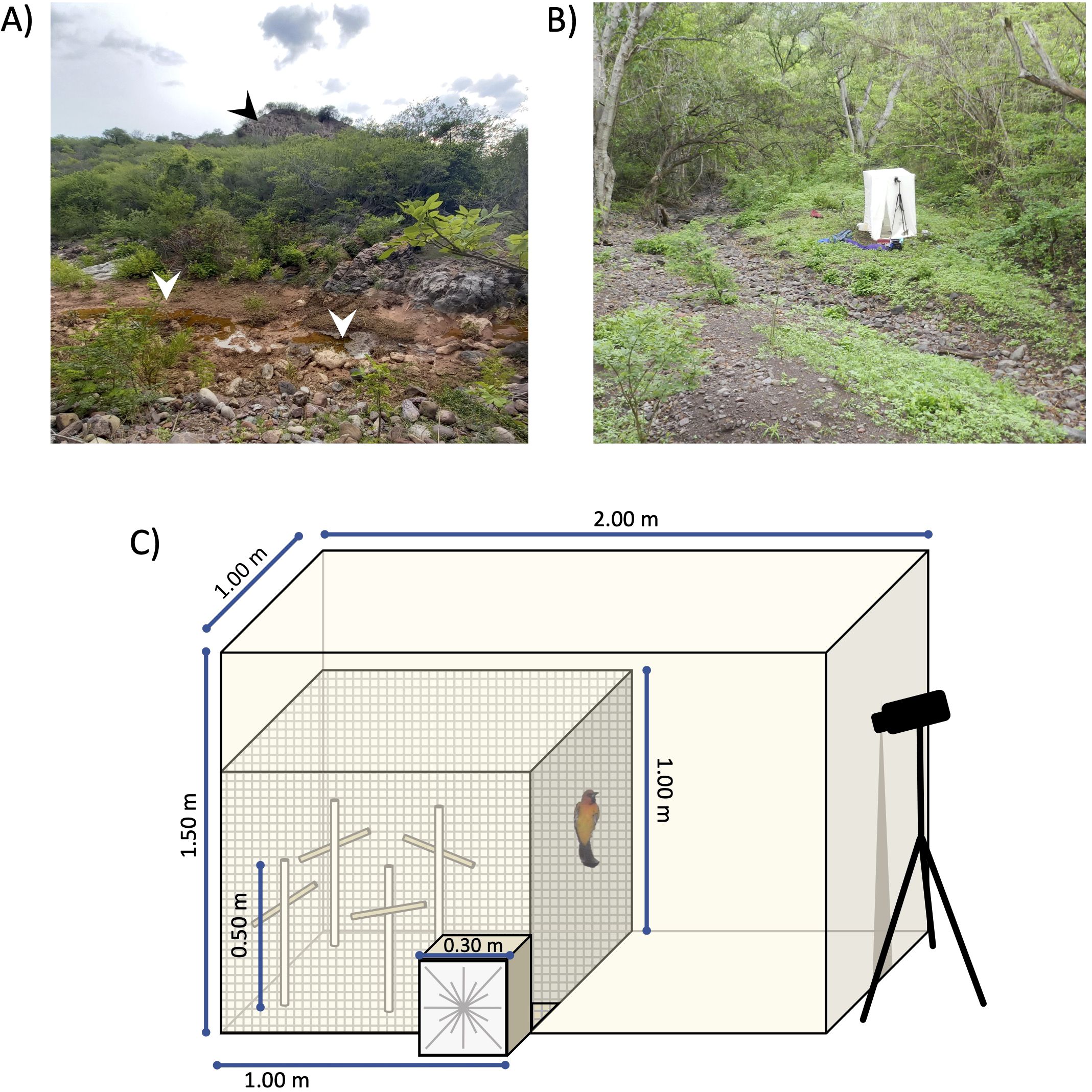
Figure 1. Illustrative images of the study site and novel environment test arena. (A) Photograph of one of two main tailings piles in the study area (“El Portón”: 18°26’24.57” N, 99°1’58.47” W) during the rainy season; the tailings pile is visible in the background (black arrowhead) as a large hill mostly denuded of vegetation on the slopes, and sediment runoff from the tailings is evident in the seasonal Huautla Stream (foreground; white arrowheads). (B) Photograph of the novel environment test arena assembled in the field. (C) Schematic representation of the novel environment test arena. To perform the test, the bird was introduced into the wooden habituation compartment (0.30×0.30×0.30 m) through a fabric panel with a drawstring closure (foreground). After a 5-min habituation period, a vertical trap door was released from the outside (falling into the arena), to release the bird into the exploration area (1×1×1 m) constructed from gray vinyl mosquito screen. The exploration area was covered with white muslin fabric, and the whole apparatus was supported by a collapsible PVC frame. The test was filmed using a video camera mounted on a tripod outside the arena, filming through a slit in the fabric which was closed at the bottom with clothespins so that the bird could not see out of the arena. After the 5-min exploration period, an observer entered the area between the camera and the exploration area and recaptured the bird using an entomological net to release it where it was originally captured. Note that for illustrative purposes the outer fabric is shown here as transparent, but in reality it was opaque white to prevent visual distraction and escape attempts (as is visible in B).
To begin the habituation period, the bird was transferred by hand into the habituation compartment from the outside through a fabric opening secured with a drawstring. After 5 min, the exploration period was initiated by opening the door into the arena and tapping the side of the box to encourage the bird to enter the arena. The individual was allowed to explore the arena for 5 min, which was recorded with a digital video camera placed on a tripod outside the structure. After the test, the individual was released where it had been captured. A total of 69 individuals were tested.
2.3 Determination of feather HM concentrations
We measured the concentrations of Pb, As, Cd, Hg, Cu, and Zn in the contour feathers plucked from each individual. The determinations were performed at the Laboratorio de Ciencias Ambientales, CIAD in Guaymas, Mexico, as in Ceyca-Contreras et al. (2020) with some modifications. Feathers were pooled by individual and washed in an ultrasonic bath to remove external contaminants (three 5-min washes with distilled water followed by one 5-min wash with acetone) then dried under a fume hood at room temperature. Once dry, 0.1000–0.2000 g of each sample was subjected to microwave-assisted acid digestion (EPA Method 3052; CEM Corp. Mod. MARS X microwave digestion system) with 50% nitric acid followed by 30% hydrogen peroxide. Finally, the concentration of each HM (mg/kg dry weight of feather) was determined by anodic stripping voltammetry (797 VA Computrace instrument; Metrohm AG, Ionestrasse 9100, Herisau, Switzerland) from a solution of 5 mL of digestate, 1 mL of ammonium acetate buffer, and 10 mL of deionized water. Pb, Cd, Zn, and Cu were determined simultaneously (protocol VA Application Note V-35), and As and Hg were determined in separate runs (Application Note V-037 and Application Bulletin 96/5 e, respectively). The sample volume was insufficient to determine As and Hg in two individuals. Quality control/quality assurance was performed daily using duplicate samples and Dolt-5 certified dogfish liver reference material (see Table 1). We could not quantify blood HM due to logistical constraints.
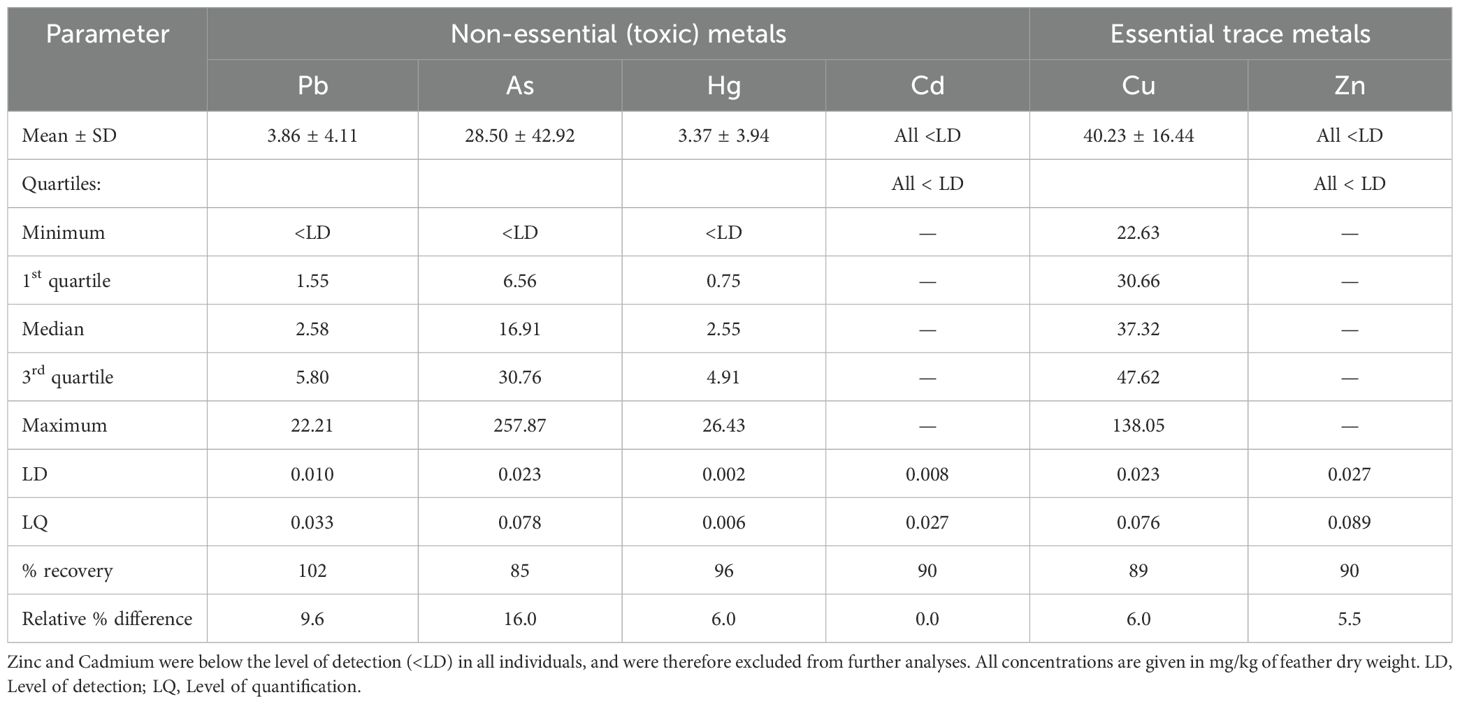
Table 1. Concentration in streak-backed oriole feathers and quality control/quality assurance parameters of the non-essential (toxic) metals lead (Pb), arsenic (As), mercury (Hg), and cadmium (Cd) and the essential trace metals copper (Cu) and zinc (Zn), as determined by anodic stripping voltammetry.
Our sampling captured a wide range of feather HM levels (Table 1), except for Cd and Zn, which were below the limit of detection for all individuals and therefore excluded from subsequent analyses. Correlations among HM were weak (Spearman’s rho ≤ 0.26; see Supplementary Figure S1).
2.4 Behavior variables, scoring, and reliability
We quantified exploratory behaviors from videos rather than in real time during the tests to ensure that the observer was blind to the individual’s capture location (expected to influence HM levels), facilitate scoring of detailed behaviors, and allow quantification of intra- and inter-observer reliability (see below).
We defined our behavioral variables based on previous novel environment tests in birds (Huang et al., 2016; Grunst et al., 2019), supplemented with a cursory viewing of the videos by JNMG and OJAF to identify behaviors with notable inter-individual variability (Maldonado Gómez, 2020; Amaro Flores, 2023). This resulted in seven focal variables: 1) number of flights, 2) total features visited, 3) time spent performing visual scans, 4) time spent in flight, 5) latency to emerge from the habituation compartment, 6) time spent on self-directed behaviors (i.e., preening or manipulation of leg bands), and 7) time elapsed between the first and last features touched. Each of these variables except for total features visited was divided by the test duration, subtracting any time during which the individual’s position prohibited determination of its behavior.
We used Adobe Photoshop to quantify the number of flights by tracing the path of each flight as a separate line and counting the number of lines. For the remaining variables, we used the program CowLog (Pastell, 2016). OAF quantified the time in flight and number of flights, and LMK scored the remaining variables. For all variables, the observer trained on a subset of 10 randomly selected videos until reaching a difference in total scored time <2 s or ≥95% agreement. To ensure that scoring criteria had not shifted for the two most challenging variables—time spent on visual scans and on self-directed behaviors—we re-scored ten videos after finishing scoring. Agreement remained high (average agreements of 95.5% and 92.9%, average differences of 7.35 s and 1.73 s respectively).
2.5 Principal components analysis
We determined that our behavioral data were suitable for principal components analysis (PCA) by confirming an overall Kaiser-Mayer-Olkin measure of sample adequacy>0.5 (check_factorstructure in the “performance” package; Lüdecke et al., 2021). This parameter quantifies the proportion of variance that is shared among variables and thus the extent to which PCA can combine the original variables into underlying factors; below 0.5, the relationships among variables are considered too weak for PCA to be useful (Hair et al., 2006). We then performed a PCA on the standardized data using the prcomp command in R, retaining PCs with eigenvalues >1 and considering loadings with absolute value ≥0.40 to indicate an important contribution of the variable to the PC (Stevens, 1992). The (unrotated) PC scores were used as response variables in subsequent models.
2.6 Linear models
First, we determined the potential effects of variables related to individual and testing conditions on exploratory behavior. We constructed a separate linear model for each PC, with the PC score as the response variable and age/sex category, body mass, date, time of day, and test delay as explanatory variables. From this model, we retained only the terms whose 95% confidence interval (CI) excluded 0, which we refer to as the “base model” (or null model, if no variables were retained; following Kiere et al., 2021).
We then evaluated the effects of feather HM levels on exploratory behavior by generating a set of linear models with the PC score as the response variable, any important covariables (above), and individual, additive, and/or interaction effects of feather HM concentrations. Given our limited sample size, we could not include all potentially relevant terms and interactions in a single global model. We therefore generated a set of hypothesis-based models (Table 2; including the base/null model), which we ranked based on Akaike’s Information Criterion, adjusted for small sample size (AICc). In cases where the model with the lowest AICc was within two points of a model with fewer terms, the simpler model was preferred (Arnold, 2010).
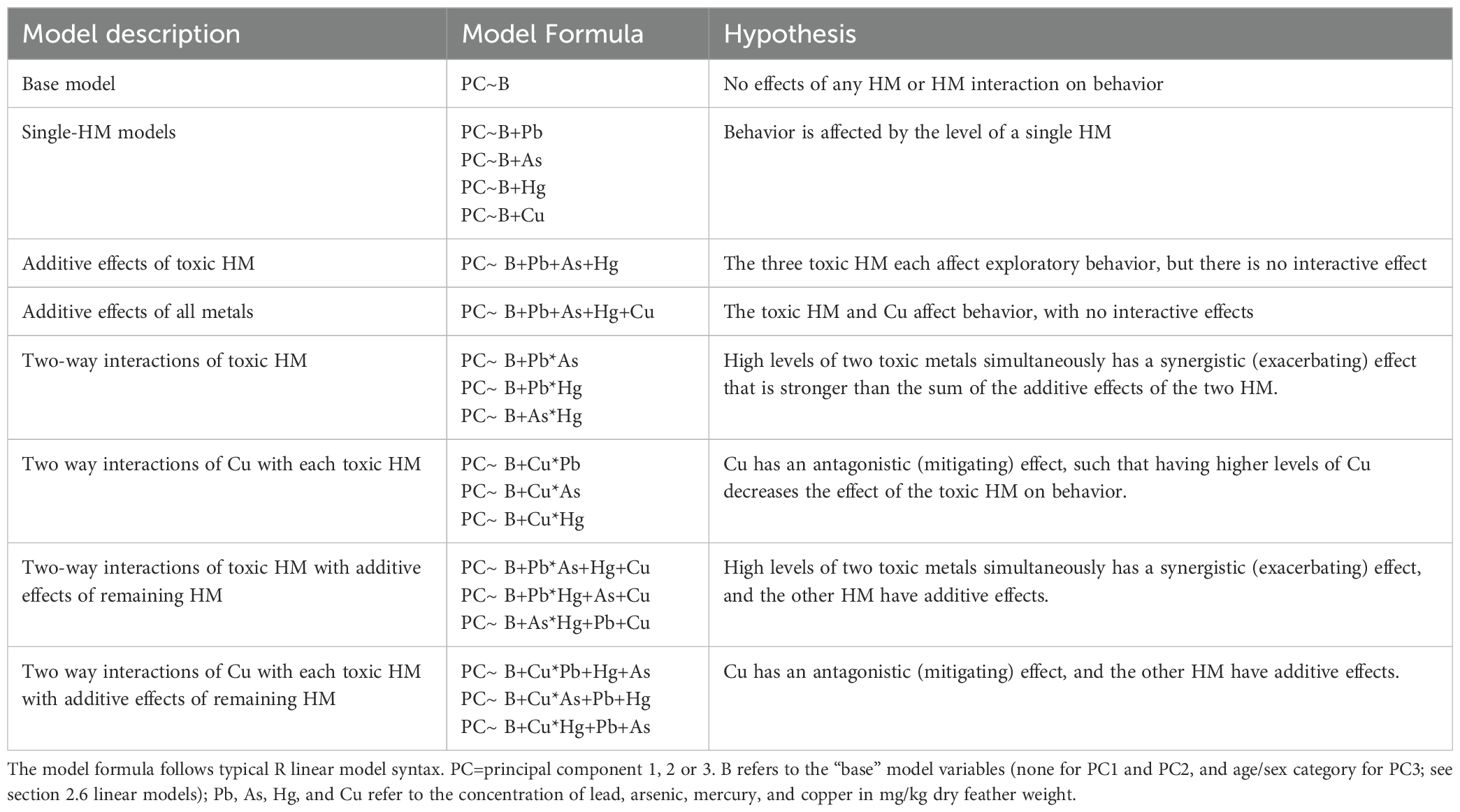
Table 2. Models and hypotheses tested with the 19 combinations of explanatory variables considered for AICc-based model selection to evaluate the effect of heavy metals/metalloids and their interactions on the three principal components expressing exploratory behavior in streak-backed orioles.
We performed all analyses in R v4.3.2 (R Core Team, 2025) using RStudio (Posit Team, 2023). In addition to the previously noted R packages, we used “factoextra” (Kassambara and Mundt, 2020) to visualize the PCA, and “performance” (Lüdecke et al., 2021) to evaluate linear model assumptions, which were fulfilled for all models. Since AICc-based model selection requires that all models be fit from the same dataset, the two individuals for which As and Hg could not be determined were excluded from all linear models.
3 Results
PCA showed three main axes of variation in STRO exploratory behavior that together explained 77.1% of the variance. PC1 accounted for 32.8% of the variance and described overall activity level, with positive loadings for time spent in flight (0.59), number of flights (0.57), and total features visited (0.44). PC2 accounted for 24.4% of the total variance and expressed a continuum of time spent on visual scans (-0.67) versus time spent on self-directed behaviors (0.52). PC3 explained 19.9% of the variance and described exploration latency, with positive loadings on latency to emerge from the habituation compartment (0.55) and the time elapsed between the first and last features visited (0.57); thus, individuals with high positive PC3 scores took longer to enter the arena and continued to reach novel parts of the arena later into the test. See Table 3 for full details of the PCA. PCA biplots are provided as supplementary material (Supplementary Figure S2).
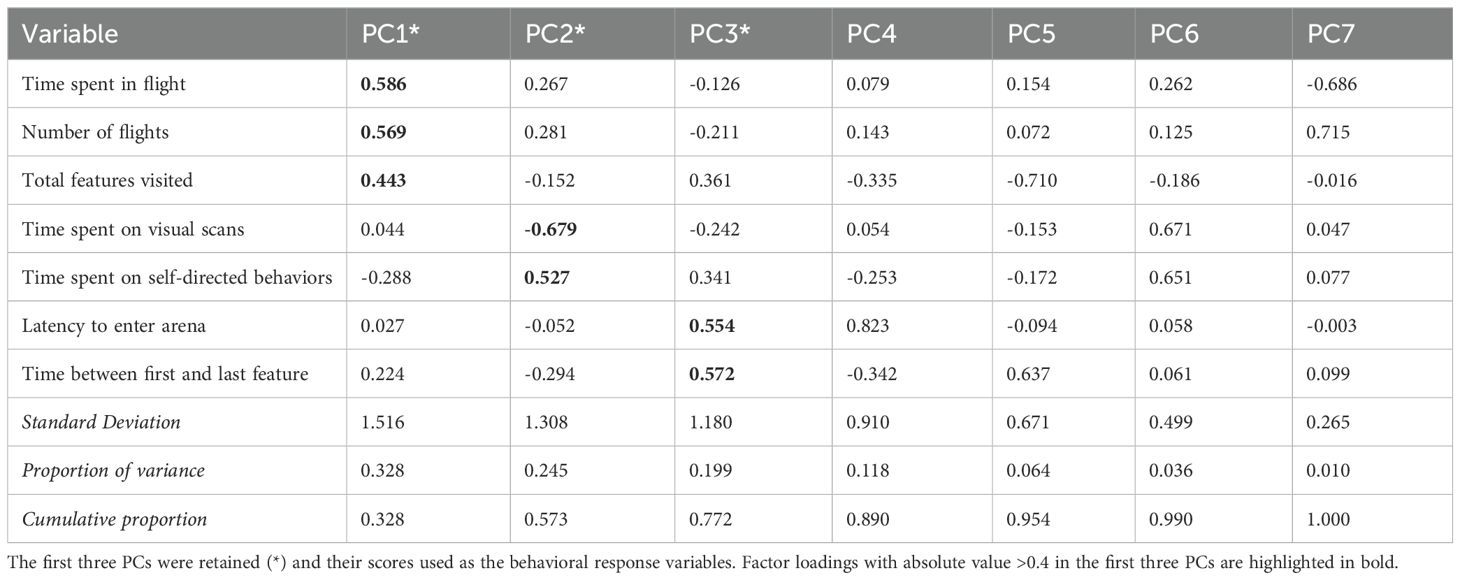
Table 3. Results of Principal Components (PC) analysis summarizing variation in exploratory behavior of streak-backed orioles during a novel environment test performed in the field.
Model comparison tables and summaries of the preferred models are provided as Supplementary Material. We found no important effects of age/sex category, body mass, date, time of day, or test delay on PC1 or PC2 (Supplementary Tables S1, S2, S4). For PC3, age/sex category was important: after-second-year females had lower PC3 scores than second-year males, indicating that they were quicker to emerge from the habituation box and reached the last novel feature sooner than second-year males (Supplementary Tables S3, S4). We therefore included age/sex category in subsequent analyses of PC3.
We found no important effects of feather HM levels or their interactions on exploratory behavior, as the null model was preferred for PC1 and PC2, and the base model containing only age/sex category was preferred for PC3 (Supplementary Table S4).
4 Discussion
We found no apparent effects of feather Pb, As, Hg, or Cu on STRO exploratory behavior in the novel environment test. This was somewhat surprising, given that our sample contained individuals with notably high feather HM levels, especially As. Previous studies of HM concentrations in feathers generally report <1 mg/kg in areas where no pollution is expected and <10 mg/kg in polluted sites (reviewed by Sánchez-Virosta et al., 2015). The highest reported feather As that we found in the literature was ~50 mg/kg, from Passer montanus near an abandoned arsenic sulfide mine in China (Yang et al., 2018), followed by Parus major with ~20–30 mg/kg from the well-studied Umicore smelter in Belgium (e.g., Geens et al., 2010; Grunst et al., 2019). In our sample, individuals frequently exceeded these levels: ~25% of our sample had >30 mg/kg, ~10% >50 mg/kg, and the highest As level we recorded was 257.87 mg/kg. Meanwhile, the feather Pb levels we found (maximum of 22 mg/kg) were lower than or comparable to those reported in other sites with known pollution (e.g., ~100 mg/kg at the Umicore smelter; Geens et al., 2010; Grunst et al., 2019, 20–30 mg/kg at an abandoned mine in northern Mexico; Monzalvo-Santos et al., 2016). Hg and Cu levels were also moderate compared to previous reports: about a quarter of our sample had Hg levels above the 5 mg/kg “toxicity threshold” proposed by Burger and Gochfeld (1997), and Cu levels were comparable to previous reports at the Umicore smelter (e.g., Grunst et al., 2019). It is also important to point out that none of the birds we captured were in appreciably poor condition; we saw no signs of acute HM poisoning, notable physical deformities, emaciated body condition, or other overt indicators of poor health in the birds included in this test or in a larger sample of >200 STRO captured over four seasons of fieldwork (LMK, pers. obs.).
In contrast to our findings, Grunst et al. (2019) did find an effect of feather HM levels on exploratory behavior in a novel environment test: individuals with higher combined feather Pb, Cd, Cu, and As (and higher blood Pb, specifically) performed fewer total hops and flights. There are several possible explanations for this difference in findings, both methodological and biological. On one hand, it is possible that HM levels do affect STRO behavior, but these effects were obscured by high behavioral variance due to other individual or environmental factors. We made a concerted effort to standardize the test protocol and statistically account for some suspected sources of variation (none of which had important effects). However, there were factors that we could not control that could affect birds’ behavior during the test, such as noise coming from outside the test arena or individuals’ energy budget at the time of capture that could have masked subtle HM effects.
On the other hand, the HM profile in our study was notably different from that found in Grunst et al. (2019); in their sample, it was Pb, not As, that was most severely elevated. This, in conjunction with a clear effect of blood Pb led the authors to propose that it was mainly Pb that was responsible for the behavioral effects. A recent experiment exposing captive house sparrows (Passer domesticus) to Pb similarly showed decreased activity during a novel environment test (Di Liberto et al., 2024). Comparing our results to these studies suggests that Pb has a stronger effect on exploratory behavior than As and that Pb levels in our sample were below the effect threshold. The fact that HM levels were not strongly correlated with each other in our sample allowed us to address the effects of each HM and their interactions independently, which is difficult in many real-world pollution scenarios.
It is also possible that feather HM did not reflect circulating HM at the time of the test. There has been some debate around the suitability of feathers as a biological matrix for HM measurements, depending on study objectives (Jaspers et al., 2019). HM are accumulated endogenously in feathers from blood flow during feather growth, and levels are fixed once the feather is mature (though there is potential for some external accumulation, which cannot be completely removed by washing; Jaspers et al., 2019). STRO undergo a single post-reproductive molt that is generally completed by October (Jaramillo and Burke, 1999), so the feathers we sampled reflected circulating HM levels in the latter part of the rainy season, approximately 6–10 months before the behavioral test. While this is advantageous in that it reflects exposure over a longer time window than blood or feces, it is possible that circulating HM levels had changed by the time of the test (see, e.g., Grunst et al., 2019). The strong precipitation seasonality at our study site could affect HM levels through several routes of exposure, including seasonal shifts in diet, concentration of HM in water sources due to evaporation (Barats et al., 2020), and dispersion of tailings sediments by wind and water erosion (Márquez-Huitzil et al., 2025). However, seasonal variations in HM are not detectable in feathers since STRO molt around the same time each year.
On the other hand, chemical affinity of HM—especially As, Cu, and Hg—for the disulphide bonds in keratin can lead to their chelation and thus over-accumulation in feathers (Dmowski, 1999). Interestingly, this could provide a mechanism for detoxification, as HM are removed from the body and deposited in feathers, where they are inert (Lewis and Furness, 1991; Burger and Gochfeld, 1992). Currently, the dynamics of As accumulation in feathers are poorly known, making it difficult to assess to what extent a potential mismatch between circulating and feather As concentrations could explain our results. If As in feathers is correlated with concentrations in blood or internal organs, it should be a relevant measure of relative exposure among individuals, even if the absolute concentration is higher than in other tissues (e.g., Yao et al., 2021). However, feather As concentration could become decoupled from levels in other tissues if there is individual variation and/or selection on the efficiency of HM deposition in feathers, or if As accumulation in feathers is non-linear with respect to blood levels, especially with high As exposure. Comparing feather HM to blood and fecal levels collected throughout the year would help address these important questions.
Finally, it is possible that the exploratory behavior of the STRO in our study area is truly unaffected by their exposure to HM, perhaps due to selection throughout a long history of exposure, or action of a selective filter at earlier stages, such as egg or nestling survival. Recent work has shown genomic evidence of adaptation to Pb exposure in Passer domesticus within ~100 years of arriving to contaminated regions of Australia (Andrew et al., 2019), substantially less time than the centuries-long history of mining in the Huautla district. Untangling possible methodological explanations for our negative results from biological ones will require further work. An important next step is to evaluate whether there are other signs of negative effects of HM on STRO, such as reduced reproductive success, altered parental care behavior, plumage coloration, fluctuating asymmetry, body size, genotoxicity, or survival at various life stages.
Data availability statement
The raw data supporting the conclusions of this article will be made available by the authors, without undue reservation.
Ethics statement
All birds were handled in accordance with national law. We were granted specific permission to work on the collectively owned and managed lands of the Huautla and Quilamula Ejidos by the local authorities. Permits for fieldwork were granted by the Mexican Secretaría del Medio Ambiente y Recursos Naturales and administration of the Sierra de Huautla Biosphere Reserve (permits SGPA/DGVS/03032/17 and SGPA/DGVS/1149/19). We followed guidelines for the ethical treatment of animals in general (Animal Behaviour Society, 2012) and wild birds in particular (Smith et al., 1999; Fair et al., 2010). We took several measures to minimize stress, especially heat stress, given the warm climate: mist nets were placed in the maximum shade possible and monitored constantly; birds were extracted from nets immediately; nets were closed early on hot days or whenever the rate of capture became too high for the available personnel; individuals showing signs of acute stress were not subjected to further processing until they had recovered; and all birds were gently offered water to drink from a clean plastic pipette before releasing them. The novel environment test arena was always mounted in the shade, and we checked the temperature inside the arena and habituation compartment frequently. At the end of the novel environment test, individuals were not released by allowing them to fly directly out of the test arena; rather, they were carefully recaptured from inside the arena using an entomological net and released by hand after offering them water to verify that they were released in adequate condition and in the same location where they had been captured. We sampled contour feathers rather than flight feathers to minimize the pain and energetic impact of feather plucking and took the minimum amount of feathers required for reliable HM determination. The total duration of captivity ranged from approximately 25 to 90 min, but was less than 1 hour for 95% of the individuals. No individual died or showed signs of serious harm. Many individuals were re-sighted or recaptured weeks after release with the plucked feathers partially regrown.
Author contributions
LK: Conceptualization, Data curation, Formal Analysis, Funding acquisition, Investigation, Methodology, Supervision, Visualization, Writing – original draft, Writing – review & editing. OA: Data curation, Investigation, Methodology, Writing – review & editing. JM: Data curation, Investigation, Methodology, Writing – review & editing. JG: Investigation, Methodology, Resources, Supervision, Writing – review & editing. IG: Funding acquisition, Project administration, Supervision, Writing – review & editing. MO: Conceptualization, Funding acquisition, Project administration, Resources, Supervision, Writing – review & editing.
Funding
The author(s) declare that financial support was received for the research and/or publication of this article. Funding for fieldwork and laboratory analyses was provided by a Ciencia de Frontera grant from the Mexican Consejo Nacional de Humanides, Ciencia, y Tecnología (CONAHCyT, group modality Grant #191975, managed by MOB and IGS). Additional funding for fieldwork in 2017 was provided by a “Developing Nations Award” from the Animal Behavior Society awarded to LK. LK was funded by two postdoctoral fellowship programs during the course of this work, from the Programa para el Desarrollo Profesional Docente through the Universidad Autónoma del Estado de Morelos (PRODEP-UAEM), and by the Dirección General de Asuntos del Personal Académico through the Universidad Nacional Autónoma de México (DGAPA-UNAM). OA received a Master’s Scholarship from CONAHCyT.
Acknowledgments
We appreciate the work of many volunteers in the field, especially Claudia Cristina Valenzuela Insunza and Cristina Organista Nava. Thanks to Felix Noel Estrada Piñero for assistance in determining heavy metal levels. Initial exploration of STRO behavior in the novel environment test was performed as part of the bachelor’s and master’s theses of JNMG and OJAF, respectively; those works are substantively different from this study, as they did not include individual heavy metal levels. We are grateful to the residents of Huautla, Rancho Viejo, and Quilamula, Morelos for their invaluable friendship, logistical help, and permission to work in their communities. We hope that our work contributes to their well-being.
Conflict of interest
The authors declare that the research was conducted in the absence of any commercial or financial relationships that could be construed as a potential conflict of interest.
Generative AI statement
The author(s) declare that no Generative AI was used in the creation of this manuscript.
Publisher’s note
All claims expressed in this article are solely those of the authors and do not necessarily represent those of their affiliated organizations, or those of the publisher, the editors and the reviewers. Any product that may be evaluated in this article, or claim that may be made by its manufacturer, is not guaranteed or endorsed by the publisher.
Supplementary material
The Supplementary Material for this article can be found online at: https://www.frontiersin.org/articles/10.3389/fbirs.2025.1568877/full#supplementary-material
References
Amaro Flores O. J. (2023). Conducta de exploración en la calandria dorso rayado (Icterus pustulatus) y su relación con la exposición a desechos de la minería metálica en la reserva de la biosfera sierra de Huautla, Morelos (Cuernavaca, Morelos, Mexico: Universidad Autónoma del Estado de Morelos). Available at: http://riaa.uaem.mx/handle/20.500.12055/3944 (Accessed January 30, 2025).
Andrew S. C., Taylor M. P., Lundregan S., Lien S., Jensen H., Griffith S. C. (2019). Signs of adaptation to trace metal contamination in a common urban bird. Sci. Total Environ. 650, 679–686. doi: 10.1016/j.scitotenv.2018.09.052
Animal Behaviour Society (2012). Guidelines for the treatment of animals in behavioral research and teaching. Anim. Behav. 83, 301–309. doi: 10.1016/j.anbehav.2011.10.031
Armendáriz-Villegas E. J., Covarrubias-García M., de los Á., Troyo-Diéguez E., Lagunes E., Arreola-Lizárraga A., et al. (2015). Metal mining and natural protected areas in Mexico: Geographic overlaps and environmental implications. Environ. Sci. Policy 48, 9–19. doi: 10.1016/j.envsci.2014.12.016
Arnold T. W. (2010). Uninformative parameters and model selection using akaike’s information criterion. J. Wildlife Manage. 74, 1175–1178. doi: 10.2193/2009-367
Barats A., Renac C., Orani A. M., Durrieu G., Saint Martin H., Esteller M. V., et al. (2020). Tracing source and mobility of arsenic and trace elements in a hydrosystem impacted by past mining activities (Morelos state, Mexico). Sci. Total Environ. 712, 135565. doi: 10.1016/j.scitotenv.2019.135565
Bridge G. (2004). Contested terrain: mining and the environment. Annu. Rev. Environ. Resour. 29, 205–259. doi: 10.1146/annurev.energy.28.011503.163434
Burger J., Gochfeld M. (1992). Trace element distribution in growing feathers: Additional excretion in feather sheaths. Arch. Environ. Contamination Toxicol. 23, 105–108. doi: 10.1007/BF00226002
Burger J., Gochfeld M. (1997). Risk, mercury levels, and birds: relating adverse laboratory effects to field biomonitoring. Environ. Res. 75, 160–172. doi: 10.1006/enrs.1997.3778
Ceyca-Contreras J. P., Cortés-Gutiérrez E., García-Salas J., Dávila-Rodríguez M., García-Hernández J. (2020). Evaluation of the genotoxic effect of heavy metals in pigeons from urban and rural habitat in Monterrey, Mexico using the chromatin dispersion assay. Biomarkers: Biochem. Indic. exposure response susceptibility to chemicals 25, 670–676. doi: 10.1080/1354750X.2020.1825811
Chávez-Ramírez E. A., Mussali-Galante P., Tovar-Sánchez E., Ochoa-Ochoa L. M., Mercado-Silva N. (2024). Metal bioaccumulation and assemblage attributes for anurans in the sierra de huautla biosphere reserve, Mexico. Trop. Conserv. Sci. 17, 1–19. doi: 10.1177/19400829241245353
CONANP (Comisión Nacional de Áreas Naturales Protegidas) (2005). Programa de conservación y manejo de la Reserva de la Biósfera Sierra de Huautla, México. Dorado O., Maldonado B., Arias D. M., Sorani V., Ramirez R., et al, editors.
Custer C. M., Yang C., Crock J. G., Shearn-bochsler V., Smith K. S., Hageman P. L. (2009). Exposure of insects and insectivorous birds to metals and other elements from abandoned mine tailings in three Summit County drainages, Colorado. Environ. Monitoring Assess. 153, 161–177. doi: 10.1007/s10661-008-0346-y
Di Liberto J. F., Griffith S. C., Hall C. J., Mendelsohn A. S., Swaddle J. P. (2024). Exposure to sublethal concentrations of lead (Pb) affects ecologically relevant behaviors in house sparrows (Passer domesticus). Arch. Environ. Contamination Toxicol. 86, 199–216. doi: 10.1007/s00244-024-01062-0
Dingemanse N., Both C., Drent P. J., Van Oers K., Van Noordwijk A. J. (2002). Repeatability and heritability of exploratory behaviour in great tits from the wild. Anim. Behav. 64, 929–938. doi: 10.1006/anbe.2002.2006
Dmowski K. (1999). “Birds as bioindicators of heavy metal pollution: review and examples concerning European species,” in Acta Ornithologica-Polska Akademia Nauk-Original Edition, vol. 34. (Warsaw, Poland: Museum and Institute of Zoology), 1–26.
Durán A. P., Rauch J., Gaston K. J. (2013). Global spatial coincidence between protected areas and metal mining activities. Biol. Conserv. 160, 272–278. doi: 10.1016/j.biocon.2013.02.003
Durkalec M., Martínez-Haro M., Nawrocka A., Pareja-Carrera J., Smits J. E.G., Mateo R. (2022). Factors influencing lead, mercury and other trace element exposure in birds from metal mining areas. Environ. Res. 212, 113575. doi: 10.1016/j.envres.2022.113575
Ecke F., Singh N. J., Arnemo J. M., Bignert A., Helander B., Berglund Å. M.M., et al. (2017). Sublethal lead exposure alters movement behavior in free-ranging golden eagles. Environ. Sci. Technology. Am. Chem. Soc. 51, 5729–5736. doi: 10.1021/acs.est.6b06024
Esteller M. V., Domínguez-Mariani E., Garrido S. E., Avilés M. (2015). Groundwater pollution by arsenic and other toxic elements in an abandoned silver mine, Mexico. Environ. Earth Sci. 74, 2893–2906. doi: 10.1007/s12665-015-4315-9
Fair J. M., Paul E., Jones J., Barrett Clark A., Davie C., Kaiser G. (2010). Guidelines to the use of wild birds in research, The Ornithological Council. Eds. Fair J. M., Paul E., Jones. J. (Washington D.C: Ornithological Council).
Geens A., Dauwe T., Bervoets L., Blust R., Eens M. (2010). Haematological status of wintering great tits (Parus major) along a metal pollution gradient. Sci. Total Environ. 408, 1174–1179. doi: 10.1016/j.scitotenv.2009.11.029
Grunst A. S., Grunst M. L., Thys B., Raap T., Daem N., Pinxten R., et al. (2018). Variation in personality traits across a metal pollution gradient in a free-living songbird. Sci. Total Environ. 630, 668–678. doi: 10.1016/j.scitotenv.2018.02.191
Grunst A. S., Grunst M. L., Daem N., Pinxten R., Bervoets L., Eens M. (2019). An important personality trait varies with blood and plumage metal concentrations in a free-living songbird. Environ. Sci. Technol. 53, 10487–10496. doi: 10.1021/acs.est.9b03548
Hair J. E., Anderson R. E., Tatham R. L., Black W. C. (2006). Multivariate DAta Analysis. 5th editio (Upper Saddle River: Prentice Hall).
Huang P., Kerman K., Sieving K. E., St. Mary C. M. (2016). Evaluating the novel-environment test for measurement of exploration by bird species. J. Ethology 34, 45–51. doi: 10.1007/s10164-015-0444-6
Isaksson C. (2010). Pollution and its impact on wild animals: A meta-analysis on oxidative stress. EcoHealth 7, 342–350. doi: 10.1007/s10393-010-0345-7
Jablonszky M., Krenhardt K., Markó G., Szász E., Hegyi G., Herényi M., et al. (2020). A behavioral trait displayed in an artificial novel environment correlates with dispersal in a wild bird. Ethology 126, 540–552. doi: 10.1111/eth.13005
Jaramillo A., Burke P. (1999). New World Blackbirds: The Icterids. (Princeton, NJ: Princeton University Press).
Jaspers V. L. B., Covaci A., Herzke D., Eulaers I., Eens M. (2019). Bird feathers as a biomonitor for environmental pollutants: Prospects and pitfalls. TrAC Trends Analytical Chem. 118, 223–226. doi: 10.1016/j.trac.2019.05.019
Kassambara A., Mundt F. (2020). factoextra: extract and visualize the results of multivariate data analyses. R package version 1.0.7. Available at: https://CRAN.R-project.org/package=factoextra.
Kiere L. M., Osorio-Beristain M., Sorani V., Prieto-Torres D. A., Navarro-Sigüenza A. G., Sánchez-González L. A. (2021). Do metal mines and their runoff affect plumage color ? Streak-backed Orioles in Mexico show unexpected patterns. Ornithological Applications 123, duab023. doi: 10.1093/ornithapp/duab023
Lewis S. A., Furness R. W. (1991). Mercury accumulation and excretion in laboratory reared black-headed gull Larus ridibundus chicks. Arch. Environ. Contamination Toxicol. 21, 316–320. doi: 10.1007/BF01055352
Lüdecke D., Ben-Shachar M. S., Patil I., Waggoner P., Makowski D. (2021). performance: an R package for assessment, comparison and testing of statistical models. J. Open Source Software 6, 3139. doi: 10.21105/joss.03139
Maldonado Gómez J. N. (2020). Conducta de exploración de Icterus pustulatus expuestos a un gradiente de concentración de contaminantes de los desechos mineros en Huautla, Morelos (Cuernavaca, Morelos, Mexico: Universidad Autónoma del Estado de Morelos). Available at: http://riaa.uaem.mx/handle/20.500.12055/3290 (Accessed January 30, 2025).
Mann R. M., Vijver M. G., Peijnenburg W. J. G. M. (2011). “Metals and metalloids in terrestrial systems: biomagnification and subsequent adverse effects,” in Ecological Impacts of Toxic Chemicals. Eds. Sánchez-Bayo F., van den Brink P. J., Mann R. M. (Bentham Science Publishers Ltd., USA), 43–62. doi: 10.2174/978160805121211101010043
Márquez-Huitzil R., Martínez-Garza C., Hinojosa-Espinoza S. I., Sorani V. (2022). Identification of mines and tailings in Huautla, Morelos, Mexico. Investigaciones Geográficas 109, e60633. doi: 10.14350/rig.60633
Márquez-Huitzil R., Vázquez-Selem L., Martínez-Garza C., Osorio-Beristain M., Ceccon E., Lindig-Cisneros R. (2025). Caracterización de la erosión y de la susceptibilidad al movimiento en masa de jales mineros en Huautla, Morelos. Investigaciones Geográficas 116, e60920. doi: 10.14350/rig.60920
Monzalvo-Santos K., Alfaro-De la Torre M. C., Chapa-Vargas L., Castro-Larragoitia J., Rodríguez-Estrella R. (2016). Arsenic and lead contamination in soil and in feathers of three resident passerine species in a semi-arid mining region of the Mexican plateau. J. Environ. Sci. Health Part A 51, 825–832. doi: 10.1080/10934529.2016.1181451
Murguía D. I., Bringezu S., Schaldach R. (2016). Global direct pressures on biodiversity by large-scale metal mining: Spatial distribution and implications for conservation. J. Environ. Manage. 180, 409–420. doi: 10.1016/j.jenvman.2016.05.040
Murphy T. G., Hernandez-Mucino D., Osorio-Beristain M., Montgomerie R., Omland K. E. (2009). Carotenoid-based status signaling by females in the tropical streak-backed oriole. Behav. Ecol. 20, 1000–1006. doi: 10.1093/beheco/arp089
Pastell M. (2016). CowLog – cross-platform application for coding behaviours from video. J. Open Res. Software 4, e15. doi: 10.5334/jors.113
Posit Team (2023). RStudio: Integrated Development Environment for R (Boston, MA: Posit Software, PBC). Available at: http://www.posit.co/ (Accessed January 30, 2025).
Pyle P. (1997). Identification Guide to North American Birds. (Bolinas, California: Slate Creek Press).
R Core Team (2025). ‘R: A Language and Environment for Statistical Computing’ (Vienna: R Foundation for Statistical Computing). Available at: https://www.r-project.org/ (Accessed January 30, 2025).
Sánchez-Virosta P., Espín S., García-Fernández A. J., Eeva T. (2015). A review on exposure and effects of arsenic in passerine birds. Sci. Total Environ. 512–513, 506–525. doi: 10.1016/j.scitotenv.2015.01.069
Smith H., Mccracken J., Shepherd D., Velez P. (1999). The Mist Netter’s Bird Safety Handbook: A Bird Bander’s Guide to Safe and Ethical Mist Netting and Banding Procedures. (Point Reyes, California: Institute for Bird Populations in Point Reyes).
Stevens J. P. (1992). Applied Multivariate Statistics For The Social Sciences. 2nd edn (Hillsdale, NJ: Erlbaum). doi: 10.14350/rig.59607
Téllez Ramírez I., Sánchez-Sálazar M. T. (2018). La expansión territorial de la minería mexicana durante el periodo 2000-2017. Una lectura desde el caso del estado de Morelos. Investigaciones Geográficas 96, 1–18.
Tovar-Sánchez E., Cervantes L. T., Martínez C., Rojas E., Valverde M., Ortiz-Hernández M. L., et al. (2012). Comparison of two wild rodent species as sentinels of environmental contamination by mine tailings. Environ. Sci. Pollution Res. 19, 1677–1686. doi: 10.1007/s11356-011-0680-4
Tóvar-Sanchez E., Mussali-Galante P., Martínez-Pacheco M., Ortiz-Hernández M. L., Sánchez-Salinas E., Olvera-Velona A. (2016). Relationship between genotoxic damage and arsenic blood concentrations in individuals residing in an arsenic contaminated area in Morelos, Mexico. Rev. Internacional Contaminación Ambiental 32, 101–117.
Velasco Trejo J. A., de la Rosa Pérez D. A., Solórzano Ochoa G., Volke Sepúlveda T. L. (2004). Primer informe del proyecto: Evaluación de tecnologías de remediación para suelos contaminados con metales (Mexico D.F: Secretaria de Medio Ambiente y Recursos Naturales of Mexico).
Vidal R. M., Berlanga H., Arizmendi M. D. C. (2009). “Mexico,” in Important Bird Areas Americas - Priority sites for biodiversity conservation. Eds. Devenish C., Díaz Fernández D.F., Clay R. P., I. D., I. Y. Z. (BirdLife International, BirdLife C. Quito, Ecuador), 269–280.
Yang F., Xie S., Liu J., Wei C., Zhang H., Chen T., et al. (2018). Arsenic concentrations and speciation in wild birds from an abandoned realgar mine in China. Chemosphere 193, 777–784. doi: 10.1016/j.chemosphere.2017.11.098
Keywords: mine tailings, metal poisoning, anthropogenic effects, exploration, Icteridae, open field test
Citation: Kiere LM, Amaro Flores OJ, Maldonado Gómez JN, García-Hernández J, G-Santoyo I and Osorio-Beristain M (2025) No apparent effect of feather heavy metal levels on exploratory behavior of streak-backed orioles (Icterus pustulatus) living near mining waste in central Mexico. Front. Bird Sci. 4:1568877. doi: 10.3389/fbirs.2025.1568877
Received: 30 January 2025; Accepted: 15 April 2025;
Published: 09 May 2025.
Edited by:
Andrea Grunst, Indiana State University, United StatesReviewed by:
Ana López Antia, Spanish National Research Council (CSIC), SpainDavide Taurozzi, Roma Tre University, Italy
Copyright © 2025 Kiere, Amaro Flores, Maldonado Gómez, García-Hernández, G-Santoyo and Osorio-Beristain. This is an open-access article distributed under the terms of the Creative Commons Attribution License (CC BY). The use, distribution or reproduction in other forums is permitted, provided the original author(s) and the copyright owner(s) are credited and that the original publication in this journal is cited, in accordance with accepted academic practice. No use, distribution or reproduction is permitted which does not comply with these terms.
*Correspondence: Lynna Marie Kiere, bHlubmFtYXJpZWtpZXJlQGdtYWlsLmNvbQ==