- 1Chemical Engineering Department, Covenant University, Ota, Nigeria
- 2Department of Mechanical Engineering Science, University of Johannesburg, Johannesburg, South Africa
Pollution generated by solid waste has become a massive source of concern worldwide as the amount of waste being generated has become overwhelming. Waste paper contributes significantly to the overall solid municipal waste being generated daily and with control methods that are equally bad for the environment or just plain ineffective; better, effective, and environmentally friendly control solutions are required. This study reviews the use of various microorganisms as they aid in the control of waste papers in an environmentally conscious way. In addition to being an environmentally friendly solution to the issue of solid waste paper pollution, it is also a prominent source of renewable energy in the conversion of paper into fermentable sugars for the production of bio-ethanol. This review examines the vital revolution in the enzymatic hydrolysis of paper to sugar. Salient challenges that involve bioconversion were highlighted and a few solutions were suggested.
1 Introduction
The literature revealed that some classes of waste can be converted into useful products, and these include kitchen and organic waste, metal, glass, plastic, and paper (Ojewumi et al., 2019a; Ojewumi et al., 2019b; Ojewumi et al., 2019c; Ojewumi et al., 2020). They can also be categorized based on how dangerous they are to the general human population (Wanjohi, 2018; Subedi, 2019; Ursua, 2019), and this includes but is not limited to, radioactive waste material, inflammable material, and also materials that have fatal consequences when exposed to human beings.
The challenge of solid waste and its management is prominent in developing countries because of their population, the inability of the authorities of these countries to cope with the elevated costs of waste management, and the lack of qualified personnel with a good understanding of the different determinants that lead to various levels of waste control (Guerrero et al., 2012; Guerrero et al., 2013). Wanjohi (2018) proposed that a bulk amount of wastes (80%) is channeled into landfills, 10% is burned, and only the other 10% is reused. This statistic shows the rate of increase in untreated environmental waste, that is, if no viable changes are made. The landfill is part of the partial causes of climate change in the world today as one ton of biodegradable waste has been known to generate more than 400–500 cubic meters of landfill gas. Research shows that about a quarter of the family unit’s waste material comprises paper, and half of this amount is attributed to journals and magazines. The majority of this amount is either dumped or burnt and this significantly increases the rate of environmental pollution (Ojewumi et al., 2018). Even in cases of recycling, a huge amount of paper materials are produced from virgin fibers which make for good quality paper but recycling of such paper leads to lower quality of the paper, and with increasing uses of paper, there arises a huge demand of the environment to deal with the increasing amounts of waste paper (van Wyk et al., 1999; van Wyk and Mohulatsi, 2003). In a bid to control this, several alternatives have been looked into and invented for the possible conversion of the cellulosic content in paper and other waste materials into fermentable sugars such as glucose (Ojewumi et al., 2018; Ojewumi et al., 2019d). This process could provide an alternative way to conserve fuel by converting fermentable sugar into bio-ethanol (Varotkar et al., 2016). Limiting environmental pollution by recycling wastes into usable items is not the only good thing recycling brings to the table but it also helps to protect and conserve our natural resources. An environmentally friendly way of achieving this is by the enzymatic catalysis of naturally derived waste such as paper materials into fermentable sugars, for example, glucose (van Wyk et al., 1999).
The process in which cellulose is used in various forms of lignocellulosic materials to hydrolyze them is known as enzymatic hydrolysis. The rate at which enzymatic hydrolysis occurs is significantly affected by enzyme origins and the extent to which various enzyme components differ (Mansfield et al., 1999; Yang et al., 2011). Waste paper over the years has become an increasing source of concern for various developing countries and the whole world at large; with the ever-increasing demand for paper, an average individual consumes several pounds of paper a year, and adding different institutions and businesses to that statistic significantly increases the total amount of paper consumed (Mansfield et al., 1999). Present methods used to manage and control the inevitable waste paper products generated have proven to be ineffective, inefficient, or harmful to the environment. The use of bioconversion to convert these waste products into fermentable sugars should be considered as a more effective and environmentally friendly way to control waste paper products. In this study, bioconversion via enzymatic hydrolysis, its history, and its development over the years has been discussed in detail, including its merits and demerits and suggestions on how it can be improved (Cordell and White, 2011; Marshall and Farahbakhsh, 2013).
The scope of this work covers the use of lignocellulosic biomass in waste paper to produce fermentable sugars through the process of enzymatic hydrolysis. The review also covers the use of several pre-treatment techniques and how they affect the susceptibility of the lignocellulosic content of the waste paper to enzymatic hydrolysis. The future scope of this work lies in the enzymatic hydrolysis of waste paper since it may be an alternative method for the disposal of solid waste materials and the resulting glucose. This study aims to examine the bioconversion of waste papers via the process of enzymatic hydrolysis into fermentable sugars. Papers are made from biomass of different types; hence, their conversion to waste may be difficult without considering the initial processes for paper production.
2 Paper Recycling Challenges: Inherent Composition
One of the renewable natural resources available to us on Earth is lignocellulosic biomass (Qian, 2014). Cellulose, lignin, and hemicellulose are various parts of plants used for paper production (Ojewumi et al., 2018). The process involves all of the aforementioned components being held firmly and tightly by covalent bonds, several intermolecular bridges, and van der Waals forces. All these combined together make it extremely difficult for enzymatic hydrolysis to take place as it is very strong and, therefore, not susceptible to it (Adeeyo et al., 2015). It essentially comprises a plant cell wall which contains all of the aforementioned and also heterogeneous phenolic polymers as basic parts. Fundamental sections of biomasses comprising lignocellulose containing hemicellulose (15%–35%), cellulose (25%–50%), and lignin (10%–20%) can be divided into those three in the corresponding percentages. Nearly 70% of the total biomass is cellulose and hemicellulose, which are closely linked to lignin by covalent and hydrogen connections which makes the structure extremely strong and, therefore, resilient to treatment (Fatma et al., 2018).
2.1 Cellulose
The microfibers in the cellulose possess high stability which makes the degradation of cellulose very difficult. It can also be prescribed to the polysaccharide coating that surrounds the cellulose (Kucharska et al., 2018). The sub-atomic hydrogen bonds are shaped mainly because of the β-1, 4-glycosidic connections which cause the local cellulose to be extremely intense and crystalline which makes it invulnerable to catalyst activity. Amorphous locales (which can be defined in some cases as unpredictable) serve as separators between the plant cell wall which possesses extraordinary crystallinity (Fatma et al., 2018).
2.2 Hemicellulose
Hemicellulose can be defined as a bifurcated heteropolymer, which comprises hexoses, pentoses, D-glucuronic acid, and acetylated sugars. The hexoses can be better defined as D-galactose, L-galactose, D-mannose, and L-fructose while the pentoses are L-rhamnose, arabinose, and xylose. The chemical hydrolysis of hemicellulose when likened to that of cellulose has been discovered to occur in a simpler fashion. The subjective content of hemicellulose relies upon the material from which it originated. One prime example is straw or grass (from which hemicellulose can be obtained), which is made essentially of xylene which is basically coniferous trees and mainly comprises glucomannan (Kalpana et al., 2009; Kucharska et al., 2018).
2.3 Lignin
In addition to cellulosic materials, lignin has been described as the second major constituent of biomass. Although lignin is referred to as a non-carbohydrate polymer (Fatma et al., 2018), the complete removal of lignin increases the ability of the biomass for digestibility. The existence of lignin interferes with the ability of the biomass to hydrolyze because of the toxicity and non-specific hydrolyte adsorption in the lignocellulose structures. The extraction of lignin is known as delignification. The increment of the biomass is a change in the structural composition of lignin, which causes the zone of fibers made of lignocellulose (Table 1) presented to the cellulolytic compounds to expand (Kucharska et al., 2018). Lignin can also be described as high-atomic weight, insoluble plant polymers, and these polymers are said to possess very different and very complicated structural compositions. The magnitude of these differs between angiosperms and gymnosperms and between different plants (Yan et al., 2015; Mashadi et al., 20162016; Ponnusamy et al., 2019).

TABLE 1. Examples of lignocellulosic raw materials and their chemical compositions (% dry weight) (Fatma et al., 2018).
The way phenylpropanoid alcohols appear in the structure shows how ether linkages and carbon–carbon bonding are predominant, and also noticeable is the presence of a few hydroxyls that can play a strong part in the cross-links to other polymers (polysaccharides and proteins). The benzene rings are also noted to be predominant (Kucharska et al., 2018), (Vanholme et al., 2010; Renault et al., 2019; Ojewumi et al., 2021).
2.4 Municipal Solid Waste: Raw Material
Municipal solid waste (MSW) can be defined as a combination of different kinds of solid waste materials that are produced in towns and cities from different household activities. This may include waste products under the following categories: biodegradable refuse, composite waste, scraps from electrical and electronics, hazardous waste materials, and medical refuse; other forms of waste products include recyclable materials, inert waste, and toxic waste (Brethauer and Studer, 2015).
The rate of urbanization, however, coupled with the steady and exponential rise in the population has led to an increase in generated waste (Expósito and Velasco, 2018). Earth’s capacity to carry this generated waste has been over-run by 30% and, as predicted, this has led to the accumulation of waste in different areas (Jouhara et al., 2017). MSW has shown significant ability for recovering energy and materials, commonly nicknamed “urban ore,” and its potential for resource production is under study and a great amount of resources has been put into the search on how to tap into the resources (Jain et al., 2012).
2.5 Bioconversion of Waste Paper
Figure 1 shows the flow diagram for the process by which several forms of lignocellulosic waste materials are converted into reducing or soluble sugars. Waste paper is a type of lignocellulosic material that is available in large quantities in many countries irrespective of their climate conditions, which makes them an affordable and readily available feedstock for the production of ethanol in a sustainable way that is friendly to the environment.
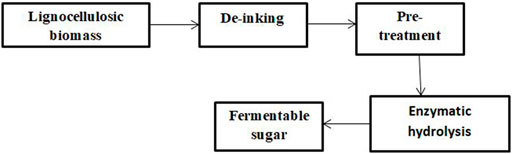
FIGURE 1. Process involved in the production of reducing sugars via block diagram (Ojewumi et al., 2018).
2.6 Enzymatic Hydrolysis
Hemicellulose and cellulose content in plants comprises the major compositions of lignocellulosic materials which are turned into reducing sugars via the enzymatic hydrolysis process. Enzymatic hydrolysis can be attained either by using microbes that secrete degrading enzymes that disintegrate lignocellulosic biomass during its media development or by using enzymes that are readily available for purchase (Fatma et al., 2018). Cellulase, which is present in some fungal and bacterial sources, exhibits moderately high activity on cellulose. When hydrolysis occurs, sugars are produced, some of which include glucose and cellobiose (van Wyk et al., 1999).
Hydrolysis is the most important step in the bioconversion process and can alternatively be carried out using chemical methods. This chemical process involves hydrolyzing the cellulolytic material by using an inorganic acid such as sulfuric or hydrochloric acid. This process is not cost-effective and also not good for the environment because it produces an acidic solution which is difficult to recycle and cannot be reused again on other cellulolytic materials (Ojewumi et al., 2018; Ojewumi et al., 2019d). The bioconversion process relies on lignocellulosic biomass as a source of fermentable sugars released by enzyme hydrolyses and converts to the necessary final products by different microorganisms (Brethauer and Studer, 2015).
3 Pretreatment Processes as Vital Routes to Waste to Wealth
The efficiency of hydrolysis during bioconversion is hampered by the hemicellulose and lignin content of the biomass as they hinder the in situ action of the enzymes. To curb this challenge, various pretreatment methods are used on the cellulosic material to enhance its enzymatic digestibility (Yáñez et al., 2003). Pretreatment is commonly performed to alter the layout of the cell wall and enhance the access to sugar polymers through hydrolytic enzymes. Usually, hydrolysis of a large portion of hemicellulose is carried out by acidic and water pretreatment methods, whereas the fraction of monomeric sugar is increased by reducing pH pretreatment. Lignin–carbohydrate ester combinations are targeted in alkaline pretreatment and results in the partial extraction of lignin. In addition, deacetylated hemicellulose has proven effective in pursuing enzyme hydrolysis (Brethauer and Studer, 2015).
The major components of cellulose include cell walls and these walls are responsible for the rigid nature of cellulose; the hemicellulose component also plays a part as it is the structural and backup substance of the plant. From the literature, different techniques such as physical, biological, or chemical techniques are used for pretreatment (Ruiz et al., 2008; El-Zawawy et al., 2011; Łukajtis et al., 2018a). Ohgren et al. (2007 suggested the following requirements to be considered for a pretreatment method:
1) The process must cause an increase in the ability of the material used to generate sugars after hydrolysis;
2) The process must evade any form of deterioration or depletion of carbohydrates;
3) The process must not generate any product or side effects that ultimately hinder the processes to be carried out ahead;
4) Most importantly, it must be cost-effective and efficient.
Several studies have focused on pretreatment as it can help make the entire process more cost-effective (Ohgren et al., 2007; Hernández et al., 2015; Łukajtis et al., 2018b)
3.1 Chemical Pretreatment
Chemical pretreatment, as the name implies, refers to the type of pretreatment techniques that make use of chemical reactions to change the difficult lignocellulosic structure of the biomass (Brodeur et al., 2011; Ravindran and Jaiswal, 2016; Taylor et al., 2019). The types of pretreatments are discussed as follows.
3.1.1 Pretreatment With Acids
Acidic pretreatment is described as one of the most successful techniques of hemicellulose solubilization which makes cellulose more available (Hendriks and Zeeman, 2009; Zhao et al., 2012). As the acid treatment is carried out, the main reactions involved are the hydrolysis of hemicellulose, lignin precipitation, and subsequent condensation. Now, at slight temperature levels, the use of strong acids for pretreatment produces high yields of sugar as the hydrolysis of the cellulosic content continues (Asgher et al., 2014). The dangers presented by the toxic nature of the reaction medium demand the need for reactors resistant to those attacks and are robust in nature. This affects the costs of processing the biomass. In considering all these, the need to make the process cost-effective is not lost and can be achieved by recovering, after hydrolysis, the acid concentration (Balat, 2011; Modenbach and Nokes, 2012).
Studies by Foyle et al. (2007) showed how effective sulfuric acid is over other acids. Idrees et al. (2013); Shimizu et al. (2018); and Ferreira da Silva et al. (2020) indicated that glucose production in banana pseudostem enzyme hydrolysis is influenced by the concentration of sulfuric acid on the chemical structure of the products. Dissolved glycosidic connections randomly caused by sulfuric acid and efficient hemicellulose riddance increase the cellulose and lignin content of non-treated products.
In dealing with the issue of high costs, the use of diluted acids has been shown to significantly help without compromising the process efficiency. Dilute-acid hydrolysis has also been used for the effective treatment of the materials before they are subjected to hydrolysis. Pretreatment using dilute H2SO4 is very capable of attaining impressive rates of the reaction and also significantly improving cellulose hydrolysis (Monte et al., 2011). Alongside the impressive reaction rates, the use of dilute acid in a pretreatment method helps limit the generation of inhibitors which can hamper the processes moving forward (Kim, 2018; Arora et al., 2020).
3.1.2 Alkaline Pretreatment
The mechanism involved in alkaline hydrolysis is the use of the saponification technique to process the intermolecular ester bonds that intertwine xylan and some other elements (Mamo et al., 2013; Ballesteros et al., 2018).
In the alkaline pretreatment of lignocellulosic materials, bases such as sodium hydroxide, potassium hydroxide, and calcium hydroxide are regularly used and, even in some cases, ammonia (NH3). The reaction during alkaline biomass pretreatment increases the area of the surface and minimizes the crystallinity of cellulose and also the extent of its polymerization (Zhao et al., 2012). Alkaline pretreatment is much more effective for materials with low lignin content, and the main advantages include cost savings because of the use of inexpensive chemicals, non-hard reaction conditions, and efficient erasure of the lignin, xylan, and biomass fractionation probability. Disadvantages of the pretreatment procedure include the long cycle duration and the strenuousness of neutralizing the post-treatment mixture.
3.1.3 Delignification With the Use of Oxidizing Agents
This treatment method of lignocellulosic materials is based on the pretreatment technique that uses an agent of oxidization such as ozone, hydrogen peroxide, oxygen, or air, in some cases. The procedure mainly includes extracting from the biomass structure, lignin, and enhancing cellulose’ accessibility (Rochefort et al., 2002; Zheng et al., 2014; Ravindran and Jaiswal, 2016). This pretreatment method was carried out on cane bagasse using hydrogen peroxide, and this strongly increased its vulnerability to enzymatic hydrolysis (Azzam, 1989). Bjerre et al. (1996) and Schmidt et al. (1996) also performed wet oxidation and alkaline hydrolysis on wheat straw (20 g straw/l, 170°C, 5–10 min), which gave an 85% glucose generation rate.
3.1.4 Acid Hydrolysis
In this technique, dried biomass is processed, pre-soaked in H2O, and lowered into an acidic medium, and a controlled temperature was used for an extended period. The pre-treated solution is also used to isolate the alcohol from the unhydrolyzed substrate. Then, washing is carried out to potentially balance previous saccharification, divide sugars, and eliminate acids. Normally, the particle focus is connected in a simple manner with a steady hydrolysis response; hence, the more negative the pKa estimation is, the more powerful the hydrolysis cycle will be (Bobleter, 1994). Sulfuric (H2SO4) and phosphoric (H3PO4) acids are generally used because of their modest nature and productivity rate in the hydrolyzing lignocellulose, despite the fact that the latter produces a milder impact and is more eco-friendly. Hydrochloric acid is more unstable, and furthermore, much easier to recuperate and produces better results than H2SO4. Nitric acid (HNO3) also produces an appreciable quality of sugar from cellulose. In any case, the two acids are costlier than sulfuric acid. In an acidic medium, the formless hemicelluloses in LB undergo hydrolysis faster to dissolvable sugars (Samuel et al., 2010) and some oligomers, particularly in a gentle state because of the disturbance propagated by the xylosidic bonds and cutting down of acetyl ester bunches, and the hold of lignin is debased through replacement responses and broken connections joined by build-up responses that forestall disintegration. Cellulose undergoes a special corruption of indistinct locales prompting augmented cellulose totals alongside an expansion in the crystallinity file of the material that was pretreated. This cycle is commonly influenced by the molecule size, response time, acidic fixation, fluid-to-strong proportion, and temperature.
3.1.5 Dilute Acid Pretreatment
Under a weak acid, temperatures along the range of 120°C–210°C and weights are used to accomplish response periods immediately or within a very short timeframe; hence, they are appropriate for nonstop procedures (Tao et al., 2011). The low acidic use is a significant bit of leeway regarding the cost and seriousness of the measure (Galbe and Zacchi, 2002). Many cycles require two pretreatment stages: in the first stage, most hemicelluloses are solubilized with a weaker acidic substrate; in the second, a stronger acid fixation for the hydrolysis of cellulose and the remaining hemicellulose is used (Anex et al., 2010). In most cases, expanding pretreatment can lead to a higher degree of glucose increase, but the conditions causing elevated increase in glucose do not essentially result in high concentrations of xylose. It was discovered that the glucose yield depended more on acidic fixation and protein stacking than the response temperature.
3.1.6 Concentrated Acid Pretreatment
This pretreatment variety uses concentrated sulfuric (65–86% w/v), hydrochloric (41%), or phosphoric (85% w/w) acids to retreat dried (5–10% dampness), pounded biomass at low temperatures (30–60°C) and pressures. The pretreated substance is weakened with deionized water for saccharification to occur at moderate temperatures (70–121°C), isolated into strong and fluid portions, after which washing and balancing of the strong substrates is carried out (Foyle et al., 2007). Another variety involves the expansion of natural solvents, for example, (CH3)2CO is added to the pretreated substance followed by the fomentation of the blend to stop the response and isolate the solids from the supernatant (containing lignin) which is further washed before enzymatic hydrolysis (Asgher et al., 2014). The proficiency of the cycle is influenced by acidic focus, acidic/biomass proportion, measure temperature, and time. Two licensed cycle arrangements are always involved—the Arkenol and the Biosulfurol measures—which depend on the concentration of H2SO4. The Arkenol cycle includes pretreatment (decrystallization of the biomass) at temperatures lower than 50°C at an acidic (70–77%)/biomass (10% dampness, <1 mm molecule size) proportion of 1.25 (Van Groenestijn et al., 2006). In the Biosulfurol cycle, the biomass is streamed in the acidic medium (70%) within the sight of dry CO2 from the fermenter followed by the weakening of the pretreated slurry with water. The acidic medium is mostly isolated from the biomass slurry by the use of layers before aging and somewhat in an anaerobic digester after aging. The general benefits of the Biosulfurol cycle (Van Groenestijn et al., 2006), incorporate the lack of requirement of catalysts, low-temperature treatment, low creation of some products, and the ability to fractionate different biomasses with high yields of ethanol.
3.2 Physical Pretreatment
Pretreatment using this method typically shows improvements in regards to the area of the surface, sizes of the particle, crystallinity indicator, or biomass polymerization standard. Physical pretreatment prevents unnecessary chemical use which could cause more harm than good, thus eliminating waste by-products and resulting reaction inhibitors.
3.2.1 Mechanical Process
The reduction of lignocellulosic materials into particles may be applied to minimize cellulose crystallinity by a joint concurrence of processes which include chipping, milling, and grinding. The material dimension post-chipping is typically 10–30 mm with a range of 0.2–2 mm after grinding/milling. The use of a vibratory ball in the reduction of cellulose was attributed to be more efficacious than the use of a normal ball, and it also aided its digestibility (Guerrero et al., 2012). Figure 2 shows various mechanical pretreatment processes while the pretreatment processes are shown in Table 2. The mechanical process has been researched by considering the following advantages and disadvantages:
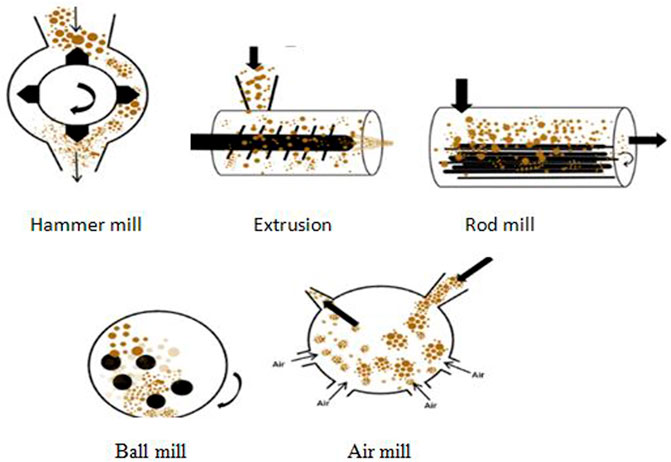
FIGURE 2. Various mechanical pretreatment techniques (Iswanto et al., 2010; Yang et al., 2011; Onumaegbu et al., 2018).
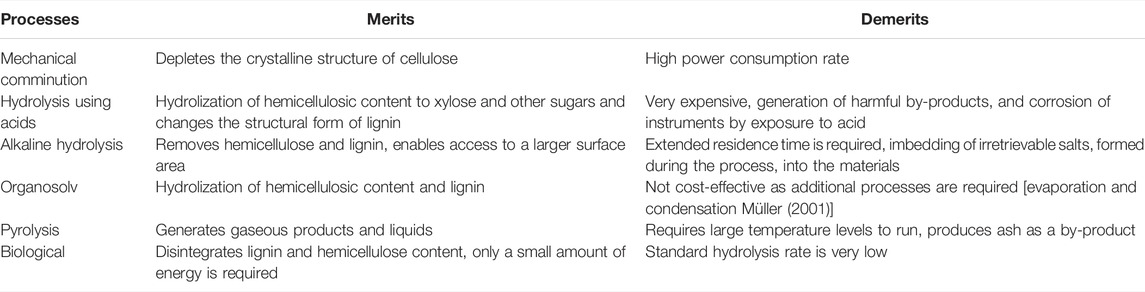
TABLE 2. Pretreatment processes (Kumar and Wyman, 2009; Karmakar, 1999; Müller, 2001; Müller et al., 2004).
3.2.1.1 Disadvantages
The increased rate in energy consumption is one of the only disadvantages of mechanical pretreatment as it leads to high costs in the processing of lignocellulosic biomass.
3.2.1.2 Advantages
1) Depletion of particle dimensions.
2) Bulk density expansion and increase in the specific surface area.
3) Gross cutback in the size of chemical wastes generated (Ballesteros et al., 2018).
3.3 Pretreatment Using Biological Methods
Biological treatment is a safe and environmentally friendly procedure that uses different types of rot fungi. This method of extracting lignin from lignocellulosic biomass is increasingly being used because it does not require high energy, notwithstanding the substantial degradation of lignin. In biological pretreatment processes, microorganisms such as brown, white, and soft-rot fungi are used to degrade lignin and hemicellulose in waste materials. White and soft rots focus mainly on both lignin and cellulose whereas brown rots primarily focus on only cellulose. Lignin degradation by white-rot fungi is caused by various enzymes which cause the degradation of lignin such as peroxidase and laccase (Kumar and Wyman, 2009). Biological pretreatment has the benefits of low consumption of energy with slight restrictions, although the hydrolysis rate is very small in their pretreatment processes (Tian et al., 2012; Kondusamy and Kalamdhad, 2014; Pramanik et al., 2019).
4 Bioconversion From Paper to Sugar: Progress and Limitations
Based on the preceding topics on initial processes for paper production, this section explains the common difficulty in the bioconversion of paper to fermentable sugar. Common problems were reviewed more intently and suggestions offered were considered.
Foyle et al. (2007)and Tian et al. (2018) carried out the bioconversion of wheat straw and waste paper. The waste paper involved included newspaper and office paper; they were the only two biomasses used to conduct the research. As stated in the work, the varying constitution of the different lignocellulosic materials used required the use of acid-based techniques. An adjusted version of the Bio-Rad Aminex HPX-87C HPLC column was used for the derivation of the sugar concentration obtained, with familiar alteration being applied to Grohmann’s method in which both the hydrolysis time and the residence time were extended. This alteration proved to be successful as it led to an increase in the production of glucose. However, the results of the trifluoroacetic acid method were poor; this was linked to the small weight of the lignocellulosic biomass used at the start. From this research, it can be seen that the use of sulfuric acid resulted in an efficient hydrolysis rate and more research should go into its use.
van Wyk et al. (2003) worked on the biodegradation of wastepaper by cellulase from Trichoderma viride. The various paper materials used for the biodegradation included office paper, filter paper, used foolscap paper, cardboard, and newspaper. After they were collected, they were all prepared and cut into pieces of 0.5 cm × 0.5 cm. For this study, T. viride-derived cellulase was dissolved in 1.0 ml of a 50 mM sodium acetate/NaOH buffer, the buffer had a concentration of 0.2 mg/ml with pH 4.5, and for a timeframe of 2 min-–8 h, and it was incubated with the various amounts of paper samples.
One of the major observations from this work was the sharp retrace of the effectiveness of bioconversion after an initial surge; it was noted that this was likely caused by a low wastepaper to enzyme ratio as the decline was noticed as the disintegration of all the paper materials progressed. The highest bioconversion rate was noticed in foolscap and office paper at 10.0 mg/incubation, in filter paper and cardboard it was at 20.0 mg, and newspaper had the lowest rate at 7.5 mg. Last, cardboard was shown to have the highest efficiency with office paper as a close second followed by foolscap. The only reservation in this work is the strict restrictions in the sense that the use of only one cellulase curbs the ability to consider a wider range of possibilities. Future works should make room for more questions to be asked by using different cellulose from varying sources so their effects can be studied and research can progress (Ojewumi et al., 2018).
Mtui and Nakamura (2005) worked on the bioconversion of lignocellulosic waste from dump sites. The study being used here is unique as the work also included the fermentation process of conversion into ethanol; in this work, lignocellulosic waste was also grouped as a whole, unlike previous works where they were differentiated. Here, they are simply called lignocellulosic biomass with the only distinction of the biomasses being the dump site they were collected from as each one was collected from a different location. The locations in question include University of Dar es Salaam (UDSM), Ubungo, Mwenge, Sinza, and Manzense; these locations lie in the municipal area of Kinondoni, Dar es Salaam region, Tanzania. The waste materials classified under lignocellulosic waste in this study include waste paper, vegetable fruit waste, hay, bagasse, and sawdust. They were then pulverized into a powdery form by using a grinding miller. This was done to increase the vulnerability of the waste to chemical pretreatment. A total of 20 ml of sulfuric acid (0.5 M) was added to 1 g of each of the samples from every site after they were liquefied in 980 ml of distilled water. Then, 50 ml of the mixture was autoclaved at 120°C for 15 min at 1 atm; this was done by pipetting it into a 100-ml conical flask. The cellulase enzyme obtained from Trichoderma reesei was then used to incubate the mixture for 8 h at 55°C. This was done at 2 h breaks as the concentration of fermentable sugars was discovered by using the dinitrosalicylic technique (DNSA). The resulting mixture was then fermented by using Saccharomyces cerevisiae with a known incubation temperature of 35°C. The concentration of ethanol was then determined by using a 120/80 6.6% carbowax column.
During the entire process, there was an initial increase as the saccharification duration also increased, but they reached a maximum at the 6 h incubation time. Finally, the concentration of glucose was determined, and the dumping sites at Ubungo had the least numbers (0.05 g/L) with Manzese having 0.07 g/L and Mwenge having 0.06 g/L. Sinza came had 0.1 g/L as its peak glucose level whereas the University of Dar es Salaam had the largest concentration of 0.13 g/L. This result, as stated in the report, can be a sort of validation showing that the type of lignocellulosic biomass also matters in the process of bioconversion and with the region with the highest concentration being a university (whose main lignocellulosic waste material would be paper), the use of paper as the premium feedstock for this procedure is supported.
Ikeda et al. (2006) worked on the bioconversion of office paper waste. The synthesis of gluconic acid in various cultures of Aspergillus niger (A. niger) was investigated using enzymatic hydrolysate from office paper waste (plain paper copies). The research included the use of repeated batch cultures using flasks, one involving the medium of saccharified solution and the other medium being that of glucose. Gluconic acid production yields based on the consumption of glucose were about 91% in the solution medium and 80% in the glucose medium. It was found that xylose and cellobiose present in the solution medium played a significant role in the development of gluconic acid and that the enzymatic hydrolysate of waste paper (paper copies) may be a strong carbon replacement for the development of gluconic acid in the culture of A. niger. Before the third replication of the batch culture, there was little diversity between the various mediums used in the processing of acid, and the gluconic concentration was observed to be 110 g/L in both mediums even after 72 h. The results obtained assert that enzymatic hydrolysate has no adverse effect on the production of gluconic acid in a culture of A. niger and can be used as an alternative source of carbon.
The addition of pure oxygen has quadrupled the acid production rate as opposed to air. The morphology of A. niger and the dry cell weight of the solution and the glucose content were found. Different morphologies resulted in a reduction in the oxygen transfer in the medium and in a reduction in the output of the gluconic acid, especially in the solution medium (which, in an electronic scan microscope, was larger and fluffier). This is a significant violation and, therefore, because of improved oxygen transfer, the glucose media should be used to produce gluconic acid. The production of gluconic acid by A. niger depends strongly on the rate of the transition of oxygen since glucose oxidation is literally the mechanism of production. There must also be a continuous supply of oxygen to increase production rates. Other than the paper copies used in this work, enzymatic hydrolysate can be used on several other waste paper samples. Several studies have been carried out with this perspective; some involving four separate waste papers were examined to observe saccharification under the same temperature conditions.
In addition to the known chemical methods, biological pretreatment has been advanced considering the tremendous results from enzymatic hydrolysis. Van et al., 2003 worked on the role of enzyme concentration in bioconversion processes. In this study, Penicillium funiculosum (P. funiculosum) was used to produce the cellulase used for the study with samples of waste foolscap, office paper, filter paper, cardboard, and newspaper as the substrate. The cellulase used in this study (Sigma; E. C. 3.2.1.4.) was obtained from P. funiculosum as stated earlier, and it was formulated with a concentration of 20.0 mg/ml inside a buffer of sodium acetate (50 mM) with a pH value of 4.5 which was obtained by adjusting it with HCL. The paper samples were all cut up into pieces of 0.5 cm by 0.5 cm, then of different sizes (2.5, 5.0, 7.5, 10.0, 20.0, 40.0, and 60.0 mg), and they were incubated with 1.0 ml of the cellulase solution at 50°C for a duration of 2 h. A constant paper size of 40 mg was also used, but in this case, the concentration of the cellulase was increased, varying from 0.2 to 0.8 mg/ml.
The concentration of the enzyme was obtained by using the Lowry method while using BSA as the standard; the sugar quantity generated was derived using the DNS method with glucose being used as the standard, with the concentration being made constant (and the paper size being increased). Foolscap, office paper, and cardboard all exhibited an initial increase in the generation of sugar until they all peaked at 10.0 mg; newspaper, on the other hand, reached its bioconversion peak at 7.5 mg, and filter paper did not reach any peak but instead exhibited an increasing move upward. However, with the concentration of enzymes being increased at 0.8 mg/ml which was the largest concentration of the enzyme, newspaper at 6,050 µg sugar/ml incubation volume showed the largest yield of bioconversion, after which came filter paper at 5,700 µg sugar/ml. The sugar content generated from cardboard and office paper was 50% smaller than that of filter paper and newspaper with foolscap showing the greatest opposition to bioconversion even with the large enzyme concentration. The resistance of foolscap could be attributed to the lack of pretreatment of waste papers before hydrolysis as treatment helps increase the susceptibility of the lignocellulosic materials.
Although the types of waste paper used varied, the use of only one cellulase enzyme provided the same challenges as before, with only one enzyme being used, we cannot compare with others under the same conditions to determine the enzyme in which the lignocellulosic content will be most susceptible to. This is a problem as different waste papers might require different cellulase compositions to be broken down effectively.
Varotkar et al. (2016) examined the enzymatic bioconversion of waste paper into bio-ethanol. In this study, wild strains of Aspergillus sp., Penicillium sp., and Trichoderma sp. were used for the saccharification of waste paper, namely, filter paper (Whatman No. 1), newspaper, and office paper. The reasons for the use of these fungi materials as stated in the study were that Trichoderma sp. is one of the most effective generators of enzymes (extracellular), which can be attributed to the fact that many different species of the aforementioned fungi are able to degrade cellulose since they contain large amounts of the cellulose enzyme which is strongly cellulolytic. Aspergillus sp. is one of the most frequently used fungi mold on an industrial scale. It breaks down polymers into simpler molecules by using acids and enzymes that are spread throughout the atmosphere. Aspergillus extracts nutrients by the degradation mechanism. Penicillium sp. can be located in various types of rotting plants such as dead wood. They (Penicillium sp.) offer a wide variety of extracellular enzymes which are known to play a vital part in the degradation of cellulosic substrates. The fungi samples were isolated following the general procedure, they were collected in sterile containers from different locations such as wood yards with sawdust, paw dust, and rotting wood flakes. They were then cultured in Czapek Dox agar (CDA), Sabouraud dextrose agar (SDA), and potato dextrose agar (PDA) media. The samples collected were then reduced to particles and sprinkled on the surface of the media under a shaded location and at ambient temperature till visible colonies began to form (after a period of 4 days). Grinding was conducted using a mixer grinder to cut the components into small parts. The grounded paper was then used as the substrates for saccharification. After hydrolysis, the researcher documented the following findings: across the board of fungi used (Aspergillus sp, Trichoderma sp., and Penicillium sp), office paper showed the most susceptibility with Penicillium sp. at 2.6 mg/ml, Trichoderma sp. at 3.26 mg/ml, and the greatest was Aspergillus sp. at 3.76 mg/ml.
A similar work performed by Rathnan and Balasaravanan (2014) using T. viride, Aspergillus oryzae, and Penicillium citrinum which gave similar results as office paper showed strong susceptibility to the hydrolysis process, although a high susceptibility rate was shown by P. citrinum instead of Aspergillus as mentioned earlier, and this was followed by newspaper and, finally, filter paper. A. oryzae also produced high reducing sugars with the highest coming from newspaper followed by office paper and then, finally, filter paper. This discrepancy between both works as it refers to office paper and different strains of Aspergillus could be due to operating conditions, temperature, pH, and also the incubation time which were all different across the board. It can be concluded in this work that the different strains of Aspergillus, Penicillium, and Trichoderma exhibited strongly for the saccharification of waste paper, with office paper being the most susceptible to hydrolysis and also releasing the highest amount of reducing sugar.
Rocha-Martin et al. (2017) worked on the enzymatic role in bioconversion. In their work, the effects of several additives on the yield of enzymatic hydrolysis were studied intently; corn stover (PCS), sugar cane straw (PSCS), and microcrystalline cellulose were all used in the hydrolysis process. The research aimed to reduce production costs and increase hydrolysis yields, which can be achieved by increasing the efficiency of the enzymatic cocktails. To achieve this and improve the enzymatic hydrolysis yields, the use of additives such as non-ionic surfactants and non-catalytic proteins has been a viable option.
Unlike this particular study, most of the works were obtained at low solid loadings, with laboratory substrates or with specific enzymes under non-industrially appropriate factors; this work, on the other hand, under industrial conditions (50°C, initial pH 5.5), studied the effect of various additives on the commercial cocktail developed in Myceliophthora thermophila using well-known feed stocks, such as pre-processed maize stover and sugarcane straw (PCS and PSCS).
To determine their capacity in enhancing enzymatic hydrolysis of PSCS and PCS, Tween 20, Tween 80, Brij 35, and Triton X-100, also known as surfactants, one polymer (PEG4000) also known as poly-ethylene glycol, and bovine serum albumin (BSA) were evaluated by the researcher. Additives such as surfactants and polymers are assumed to shape the lignin surface as a hydrated layer that impedes the non-production of cellulolytic enzymes.
PEG4000 tends to have a small improvement in enzyme hydrolysis in all materials than most additives (glucose level increased by 7.5% and PCS and PSCS hydrolyses by 10.5%, respectively). In comparison, even at low concentrations, BSA adversely influenced hydrolysis. During hydrolysis of PCS, the decrease was approximately 15%. PEG4000 allowed glucose levels to be increased in three materials by 10, 7.5, and 32%, respectively.
Ojewumi et al. (2018) re-examined the work by Rocha-Martin et al. (2017) on the enzymatic role in bioconversion. This present study showed a detailed demonstration of how waste paper has been converted to fermentable (reducing) sugar using cellulose at various temperatures; both A. niger and Pseudomonas aeruginosa were used for the enzymatic hydrolysis process. The work also classified the type of waste paper that exhibited the maximum fermentable sugar production. A. niger and P. aeruginosa were sourced from vegetative regions. Sabouraud dextrose agar (SDA) slant has been deposited in both isolates. The isolate was grown under SDA and incubated for 3–5 days at 27°C. SDA was sterile. Each isolate was modified and used for remedial purposes to 0.5 McFarland. These researchers used a metal wire (loop) which they sterilized quickly by heating in flames before each use; these were introduced to the cultivating medium of the inoculum (A. niger and P. aeruginosa). The enzymatic solution (100 ml) was then mixed with the pretreated papers (e.g., newspapers, bureau papers, and foolscap papers) which were then incubated at 25°C and 37°C for substrates with A. niger enzyme solution and at 37°C and 42°C for substrates with P. aeruginosa enzyme solution. The samples were removed weekly and examined using the dinitrosalicyclic acid process to test for sugar reduction. After the research was completed, it was discovered that the highest concentration of fermentable sugars was generated from foolscap paper at 486.66 mg/L using P. aeruginosa at 37°C for 2 weeks. Temperature effects, which are overlooked by most researchers, were also considered in this work, and office paper, at an ideal temperature of 25°C, produced the highest concentration of fermentable sugars (365 mg/L) when treated with A. niger. Several work has been done on the conversion of waste papers to fermentable sugar using different micrograms. Table 3 gives the summary of previously work done on the conversion with their percentage sugar yield respectively.

TABLE 3. Summary of the previous work carried out on waste paper to reducing sugars Van Wyk and Mohulatsi (2003).
5 Conclusion
Reported data obtained from different papers referenced previously have shown that waste paper materials are a reliable source for fermentable sugar production, although significantly affected by two factors, that is, the resistant nature of the biomass, and initial processes of paper production. It was observed that better knowledge of the various elements that control interactions between lignocellulosic biomass and enzymes would be fundamental to identify better pretreatments and enzyme systems to lower the cost of biomass conversion and meet the industrial needs. The enzymatic hydrolysis of waste paper is of considerable importance, since it may be an alternative method for the disposal of solid waste materials and the resulting glucose and, moreover, the processing of bioethanol. It is recommended that because of the health implication of some enzymes, it is essential that health authorities should synergize with the industry to avoid hazards. Also, a hybrid pretreatment method is recommended before hydrolysis to completely breakdown the resistant structure of the biomass.
Author Contributions
MEO was the major contributor in the writing of the manuscript. MEO edited the manuscript, and OO, DB, and EA collated previous works. All authors read and approved the final manuscript.
Conflict of Interest
The authors declare that the research was conducted in the absence of any commercial or financial relationships that could be construed as a potential conflict of interest.
Publisher’s Note
All claims expressed in this article are solely those of the authors and do not necessarily represent those of their affiliated organizations, or those of the publisher, the editors, and the reviewers. Any product that may be evaluated in this article, or claim that may be made by its manufacturer, is not guaranteed or endorsed by the publisher.
Funding
The authors acknowledge part-funding of this research by the Covenant University Centre for Research, Innovation and Discovery (CUCRID), Ota, Ogun State, Nigeria.
References
Adeeyo, O., Oresegun, O. M., and Oladimeji, T. E. (2015). Compositional Analysis of Lignocellulosic Materials: Evaluation of an Economically Viable Method Suitable for Woody and Non-woody Biomass. Ame J. Eng. Res. (AJER) (4), 14.
Anex, R. P., Aden, A., Kazi, F. K., Fortman, J., Swanson, R. M., Wright, M. M., et al. (2010). Techno-economic Comparison of Biomass-To-Transportation Fuels via Pyrolysis, Gasification, and Biochemical Pathways. Fuel 89, S29–S35. doi:10.1016/j.fuel.2010.07.015
Arora, A., Nandal, P., Singh, J., and Verma, M. L. (2020). Nanobiotechnological Advancements in Lignocellulosic Biomass Pretreatment. Mater. Sci. Energy Technol. 3, 308–318. doi:10.1016/j.mset.2019.12.003
Asgher, M., Bashir, F., and Iqbal, H. M. N. (2014). A Comprehensive Ligninolytic Pre-treatment Approach from Lignocellulose Green Biotechnology to Produce Bio-Ethanol. Chem. Eng. Res. Des. 92 (8), 1571–1578. doi:10.1016/j.cherd.2013.09.003
Azzam, A. M. (1989). Pretreatment of Cane Bagasse with Alkaline Hydrogen Peroxide for Enzymatic Hydrolysis of Cellulose and Ethanol Fermentation. J. Env. Sc. & Hlth., Part B 24 (4), 421–433. doi:10.1080/03601238909372658
Balat, M. (2011). Production of Bioethanol from Lignocellulosic Materials via the Biochemical Pathway: a Review. Energy Convers. Manag. 52 (2), 858–875. doi:10.1016/j.enconman.2010.08.013
Ballesteros, L. F., Michelin, M., Vicente, A. A., Teixeira, J. A., and Cerqueira, M. A. (2018). Lignocellulosic Materials and Their Use in Bio-Based Packaging. SpringerSpringerLink.
Bjerre, A. B., Olesen, A. B., Fernqvist, T., Plöger, A., and Schmidt, A. S. (1996). Pretreatment of Wheat Straw Using Combined Wet Oxidation and Alkaline Hydrolysis Resulting in Convertible Cellulose and Hemicellulose. Biotechnol. Bioeng. 49 (5), 568–577. doi:10.1002/(SICI)1097-0290(19960305)49:5<568::AID-BIT10>3.0.CO;2-6
Bobleter, O. (1994). Hydrothermal Degradation of Polymers Derived from Plants. Prog. Polym. Sci. 19 (5), 797–841. doi:10.1016/0079-6700(94)90033-7
Brethauer, S., and Studer, M. H. (2015). Biochemical Conversion Processes of Lignocellulosic Biomass to Fuels and Chemicals - A Review. Chimia 69, 572–582. doi:10.2533/chimia.2015.572
Brodeur, G., Yau, E., Badal, K., Collier, J., Ramachandran, K. B., and Ramakrishnan, S. (2011). Chemical and Physicochemical Pretreatment of Lignocellulosic Biomass: A Review. Enzyme Res. 2011, 1–17. doi:10.4061/2011/787532
Cordell, D., and White, S. (2011). Peak Phosphorus: Clarifying the Key Issues of a Vigorous Debate about Long-Term Phosphorus Security. Sustainability 3 (10), 2027–2049. doi:10.3390/su3102027
El-Zawawy, W. K., Ibrahim, M. M., Abdel-Fattah, Y. R., Soliman, N. A., and Mahmoud, M. M. (2011). Acid and Enzyme Hydrolysis to Convert Pretreated Lignocellulosic Materials into Glucose for Ethanol Production. Carbohydr. Polym. 84 (3), 865–871. doi:10.1016/j.carbpol.2010.12.022
Expósito, A., and Velasco, F. (2018). Municipal Solid-Waste Recycling Market and the European 2020 Horizon Strategy: A Regional Efficiency Analysis in Spain. J. Clean. Prod. 172, 938–948. doi:10.1016/j.jclepro.2017.10.221
Fatma, S., Hammed, A., Noman, M., Ahmed, T., Sohail, I., Shahid, M., et al. (2018). Lignocellulosic Biomass: A Sustainable Bioenergy Source for the Future. Ppl 25 (2), 148–163. doi:10.2174/0929866525666180122144504
Ferreira da Silva, I., Reis Fontinelle Souto, L., Collins, S. R. A., Elliston, A., de Queiroz, J. H., and Waldron, K. W. (2020). Impact of Hot Water and Alkaline Pre-treatments in Cellulosic Ethanol Production from Banana Pseudostem. Bioenerg. Res. 13 (4), 1159–1170. doi:10.1007/s12155-020-10123-w
Foyle, T., Jennings, L., and Mulcahy, P. (2007). Compositional Analysis of Lignocellulosic Materials: Evaluation of Methods Used for Sugar Analysis of Waste Paper and Straw. Bioresour. Technol. 98 (16), 3026–3036. doi:10.1016/j.biortech.2006.10.013
Galbe, M., and Zacchi, G. (2002). A Review of the Production of Ethanol from Softwood. Appl. Microbiol. Biotechnol. 59, 618–628. doi:10.1007/s00253-002-1058-9
Guerrero, L. A., Maas, G., and Hogland, W. (2012). Factors Influencing the Performance of Waste Management Systems.
Guerrero, L. A., Maas, G., and Hogland, W. (2013). Solid Waste Management Challenges for Cities Indeveloping Countries. Waste manag. 33 (1), 220.
Hendriks, A. T. W. M., and Zeeman, G. (2009). Pretreatments to Enhance the Digestibility of Lignocellulosic Biomass. Bioresour. Technol. 100 (1), 10–18. doi:10.1016/j.biortech.2008.05.027
Hernández, D., Riaño, B., Coca, M., and García-González, M. C. (2015). Saccharification of Carbohydrates in Microalgal Biomass by Physical, Chemical and Enzymatic Pre-treatments as a Previous Step for Bioethanol Production. Chem. Eng. J. 262, 939–945. doi:10.1016/j.cej.2014.10.049
Idrees, M., Adnan, A., Malik, F., and Qureshi, F. A. (2013). Enzymatic Saccharification and Lactic Acid Production from Banana Pseudo-stem through Optimized Pretreatment at Lowest Catalyst Concentration. EXCLI J. 12, 269.
Ikeda, Y., Park, E., and Okuda, N. (2006). Bioconversion of Waste Office Paper to Gluconic Acid in a Turbine Blade Reactor by the Filamentous Fungus Aspergillus niger. Bioresour. Technol. 97 (8), 1030–1035. doi:10.1016/j.biortech.2005.04.040
Iswanto, A. H., Febrianto, F., Wahyudi, I., Hwang, W.-J., Lee, H.-S., Kwon, J.-H., et al. (2010). Effect of Pre-treatment Techniques on Physical, Mechanical and Durability Properties of Oriented Strand Board Made from Sentang Wood (Melia Excelsa Jack). J. Fac. Agric Kyushu Univ. 55 (2), 371–377. doi:10.5109/18854
Jain, R., Urban, L., Balbach, H., and Webb, M. D. (2012). Handbook of Environmental Engineering Assessment: Strategy, Planning, and Management. Butterworth-Heinemann.
Jouhara, H., Czajczyńska, D., Ghazal, H., Krzyżyńska, R., Anguilano, L., Reynolds, A. J., et al. (2017). Municipal Waste Management Systems for Domestic Use. Energy 139, 485–506. doi:10.1016/j.energy.2017.07.162
Kalpana, D., Cho, S. H., Lee, S. B., Lee, Y. S., Misra, R., and Renganathan, N. G. (2009). Recycled Waste Paper-A New Source of Raw Material for Electric Double-Layer Capacitors. J. Power Sources 190 (2), 587–591. doi:10.1016/j.jpowsour.2009.01.058
Kim, D. (2018). Physico-chemical Conversion of Lignocellulose: Inhibitor Effects and Detoxification Strategies: a Mini Review. Molecules 23 (2), 309. doi:10.3390/molecules23020309
Kondusamy, D., and Kalamdhad, A. S. (2014). Pre-treatment and Anaerobic Digestion of Food Waste for High Rate Methane Production - A Review. J. Environ. Chem. Eng. 2 (3), 1821–1830. doi:10.1016/j.jece.2014.07.024
Kucharska, K., Rybarczyk, P., Hołowacz, I., Łukajtis, R., Glinka, M., and Kamiński, M. (2018). Pretreatment of Lignocellulosic Materials as Substrates for Fermentation Processes. Molecules 23 (11), 2937. doi:10.3390/molecules23112937
Kumar, R., and Wyman, C. E. (2009). Does Change in Accessibility with Conversion Depend on Both the Substrate and Pretreatment Technology? Bioresour. Technol. 100 (18), 4193–4202. doi:10.1016/j.biortech.2008.11.058
Łukajtis, R., Kucharska, K., Hołowacz, I., Rybarczyk, P., Wychodnik, K., Słupek, E., et al. (2018). Comparison and Optimization of Saccharification Conditions of Alkaline Pre-treated Triticale Straw for Acid and Enzymatic Hydrolysis Followed by Ethanol Fermentation. Energies 11 (3), 639. doi:10.3390/en11030639
Łukajtis, R., Rybarczyk, P., Kucharska, K., Konopacka-Łyskawa, D., Słupek, E., Wychodnik, K., et al. (2018). Optimization of Saccharification Conditions of Lignocellulosic Biomass under Alkaline Pre-treatment and Enzymatic Hydrolysis. Energies 11 (4), 886. doi:10.3390/en11040886
Mamo, G., Faryar, R., and Karlsson, E. N. (2013). “Microbial Glycoside Hydrolases for Biomass Utilization in Biofuels Applications,” in Biofuel Technologies (Heidelberg: Springer Berlin), 171–188. doi:10.1007/978-3-642-34519-7_7
Mansfield, S. D., Mooney, C., and Saddler, J. N. (1999). Substrate and Enzyme Characteristics that Limit Cellulose Hydrolysis. Biotechnol. Prog. 15 (5), 804–816. doi:10.1021/bp9900864
Marshall, R. E., and Farahbakhsh, K. (2013). Systems Approaches to Integrated Solid Waste Management in Developing Countries. Waste Manag. 33 (4), 988–1003. doi:10.1016/j.wasman.2012.12.023
Mashadi, K., Mokatse, P., Pieter, J., and Van Wyk, H. (2016). Cellulase Catalyzed Bioconversion of Different Waste Paper Materials into Fermentable Sugars. Int. J. Biosci. 8 (2), 66–76. doi:10.12692/ijb/8.2.66-76
Modenbach, A. A., and Nokes, S. E. (2012). The Use of High-Solids Loadings in Biomass Pretreatment-A Review. Biotechnol. Bioeng. 109 (6), 1430–1442. doi:10.1002/bit.24464
Monte, J. R., Brienzo, M., and Milagres, A. M. F. (2011). Utilization of Pineapple Stem Juice to Enhance Enzyme-Hydrolytic Efficiency for Sugarcane Bagasse after an Optimized Pre-treatment with Alkaline Peroxide. Appl. Energy 88 (1), 403–408. doi:10.1016/j.apenergy.2010.08.009
Mtui, G., and Nakamura, Y. (2005). Bioconversion of Lignocellulosic Waste from Selected Dumping Sites in Dar Es Salaam, Tanzania. Biodegradation 16 (6), 493–499. doi:10.1007/s10532-004-5826-3
Müller, J. A., Winter, A., and Struenkmann, G. (2004). Investigation and Assessment of Sludge Pre-treatment Processes. Water Sci. Technol. 49 (10), 97–104.
Müller, J. A. (2001). Prospects and Problems of Sludge Pre-treatment Processes. Water Sci. Technol. 44 (10), 121–128. doi:10.2166/wst.2001.0598
Ohgren, K., Bura, R., Saddler, J., and Zacchi, G. (2007). Effect of Hemicellulose and Lignin Removal on Enzymatic Hydrolysis of Steam Pretreated Corn Stover. Bioresour. Technol. 98 (13), 2503–2510. doi:10.1016/j.biortech.2006.09.003
Ojewumi, M. E., Emetere, M. E., Amefule, C. V., Adeniyi, D. O., and Durodola, B. M. (2019a). Bioconversion ofOrange Peel Waste by Escherichia Coli and Saccharomyces cerevisiae to Ethanol. Inter J. Pharm. Sci. Res. 10 (3), 1246–1252. doi:10.13040/IJPSR.0975-8232.10(3).1246-52
Ojewumi, M. E., Obanla, O. R., Ekanem, G. P., Ogele, P. C., and Ojewumi, E. O. (2020). Anaerobic Decomposition of Cattle Manure Blended with Food Waste for Biogas Production. Inter J. Recent Tech. Eng. 9 (2), 357–365. ISSN: 2277-3878.
Ojewumi, M. E., Ogele, P. C., Oyekunle, D. T., Omoleye, J. A., Taiwo, S. O., and Obafemi, Y. D. (2019b). Co-digestion of Cow Dung with Organic Kitchen Waste to Produce Biogasusing Pseudomonas aeruginosa. 3rd International Conference on Science and Sustainable Development (ICSSD 2019). IOP Conf Ser. J. Phys. 1299, 012011. doi:10.1088/1742-6596/1299/1/012011
Ojewumi, M. E., Ehinmowo, A. B., Obanla, O. R., Durodola, B. M., and Ezeocha, R. C. (2021). Comparative Analysis on the Bleaching of Crude Palm Oil Using Activated Groundnut Hull, Snail Shell and Rice Husk. Heliyon 7, e07747. doi:10.1016/j.heliyon.2021.e07747
Ojewumi, M. E., Oyekunle, D. T., Amaefule, C. V., Omoleye, J. A., and Ogunbiyi, A. T. (2019c). Investigation into Alternative Energy Sources from Waste Citrus Peel (Orange): Approach to Environmental Protection. J. Phys. Conf. Ser. 1378, 022066. doi:10.1088/1742-6596/1378/2/022066
Ojewumi, M., Kolawole, O., Oyekunle, D., Taiwo, S., and Adeyemi, A. (2019d). Bioconversion of Waste Foolscap and Newspaper to Fermentable Sugar. J. Ecol. Eng. 20 (4), 35–41. doi:10.12911/22998993/102614
Ojewumi, M., Obielue, B., Emetere, M., Awolu, O., and Ojewumi, E. (2018). Alkaline Pre-treatment and Enzymatic Hydrolysis of Waste Papers to Fermentable Sugar. J. Ecol. Eng. 19 (1), 211–217. doi:10.12911/22998993/79404
Onumaegbu, C., Mooney, J., Alaswad, A., and Olabi, A. G. (2018). Pre-treatment Methods for Production of Biofuel from Microalgae Biomass. Renew. Sustain. Energy Rev. 93, 16–26. doi:10.1016/j.rser.2018.04.015
Ponnusamy, V. K., Nguyen, D. D., Dharmaraja, J., Shobana, S., Banu, J. R., Saratale, R. G., et al. (2019). A Review on Lignin Structure, Pretreatments, Fermentation Reactions and Biorefinery Potential. Bioresour. Technol. 271, 462–472. doi:10.1016/j.biortech.2018.09.070
Pramanik, S. K., Suja, F. B., Zain, S. M., and Pramanik, B. K. (2019). The Anaerobic Digestion Process of Biogas Production from Food Waste: Prospects and Constraints. Bioresour. Technol. Rep. 8, 100310. doi:10.1016/j.biteb.2019.100310
Qian, E. W. (2014). Pretreatment and Saccharificationof Lignocellulosic Biomass. Research Approaches to Sustainable Biomass Systems. Cambridge: Academic Press, 184.
Rathnan, R. K., and Balasaravanan, T. (2014). Comparative Study of Saccharification of Fruit Waste by Mono and Mixed Cultures of Cellulolytic Fungi. Int. J. Curr. Microbiol. App Sci. 3 (6), 958.
Ravindran, R., and Jaiswal, A. K. (2016). A Comprehensive Review on Pre-treatment Strategy for Lignocellulosic Food Industry Waste: Challenges and Opportunities. Bioresour. Technol. 199, 92–102. doi:10.1016/j.biortech.2015.07.106
Renault, H., Werck-Reichhart, D., and Weng, J.-K. (2019). Harnessing Lignin Evolution for Biotechnological Applications. Curr. Opin. Biotechnol. 56, 105–111. doi:10.1016/j.copbio.2018.10.011
Rocha-Martín, J., Martinez-Bernal, C., Pérez-Cobas, Y., Reyes-Sosa, F. M., and García, B. D. (2017). Additives Enhancing Enzymatic Hydrolysis of Lignocellulosic Biomass. Biores Technol. 244, 48
Rochefort, D., Bourbonnais, R., Leech, D., and Paice, M. G. (2002). Oxidation of Lignin Model Compounds by Organic and Transition Metal-Based Electron Transfer Mediators. Chem. Commun. (11), 1182–1183. doi:10.1039/b202621j
Ruiz, E., Cara, C., Manzanares, P., Ballesteros, M., and Castro, E. (2008). Evaluation of Steam Explosion Pre-treatment for Enzymatic Hydrolysis of Sunflower Stalks. Enzyme Microb. Technol. 42 (2), 160–166. doi:10.1016/j.enzmictec.2007.09.002
Samuel, R., Pu, Y., Foston, M., and Ragauskas, A. J. (2010). Solid-state NMR Characterization of Switchgrass Cellulose after Dilute Acid Pretreatment. Biofuels 1, 85–90. doi:10.4155/BFS.09.1710.4155/bfs.09.17
Schmidt, A. S., Puls, J., and Bjerre, A. B. (1996). “Comparison of Wet Oxidation and Steaming for Solubilization of the Hemicellulose Fraction in Wheat Straw and Birch Wood,” in Biomass for Energy and the Environment (PergamonElsevier), 1510–1515. doi:10.1016/b978-0-08-042849-9.50011-2
Shimizu, F. L., Monteiro, P. Q., Ghiraldi, P. H. C., Melati, R. B., Pagnocca, F. C., Souza, W. d., et al. (2018). Acid, Alkali and Peroxide Pretreatments Increase the Cellulose Accessibility and Glucose Yield of Banana Pseudostem. Industrial Crops Prod. 115, 62–68. doi:10.1016/j.indcrop.2018.02.024
Subedi, S. (2019). Waste Management System Using the Internet of Things: Case Study of Kathmandu Valley. Bachelor's Theis, Department of Energy and Environmental Engineering,Tampere University of Applied Sciences.
Tao, L., Aden, A., Elander, R. T., Pallapolu, V. R., Lee, Y. Y., Garlock, R. J., et al. (2011). Process and Technoeconomic Analysis of Leading Pretreatment Technologies for Lignocellulosic Ethanol Production Using Switchgrass. Bioresour. Technol. 102 (24), 11105–11114. doi:10.1016/j.biortech.2011.07.051
Taylor, M., Alabdrabalameer, H., and Skoulou, V. (2019). Choosing Physical, Physicochemical and Chemical Methods of Pre-treating Lignocellulosic Wastes to Repurpose into Solid Fuels. Sustainability 11 (13), 3604. doi:10.3390/su11133604
Tian, S.-Q., Zhao, R.-Y., and Chen, Z.-C. (2018). Review of the Pretreatment and Bioconversion of Lignocellulosic Biomass from Wheat Straw Materials. Renew. Sustain. Energy Rev. 91, 483–489. doi:10.1016/j.rser.2018.03.113
Tian, X.-f., Fang, Z., and Guo, F. (2012). Impact and Prospective of Fungal Pre-treatment of Lignocellulosic Biomass for Enzymatic Hydrolysis. Biofuels, Bioprod. Bioref. 6 (3), 335–350. doi:10.1002/bbb.346
Ursua, J. R. S. (2019). Plastic Wastes, Glass Bottles, and Paper: Eco-Building Materials for Making Sand Bricks. J. Nat. Alli Sci. 3 (1), 46.
Van Groenestijn, J., Hazewinkel, O., and Bakker, R. (2006). Pretreatment of Lignocellulose with Biological Acid Recycling (Biosulfurol Process). Sugar Ind. 131, 639
van Wyk, J., and Mohulatsi, M. (2003). Biodegradation of Wastepaper by Cellulase from Trichoderma Viride. Biores Tech. 86 (1), 21–23. doi:10.1016/s0960-8524(02)00130-x
van Wyk, J. P. H., Mogale, M. A., and Moroka, K. S. (1999). Bioconversion of Waste Paper Materials to Sugars: An Application Illustrating the Environmental Benefit of Enzymes. Biochem. Educ. 27 (4), 227–228. doi:10.1016/s0307-4412(99)00053-9
Vanholme, R., Demedts, B., Morreel, K., Ralph, J., and Boerjan, W. (2010). Lignin Biosynthesis and Structure. Plant Physiol. 153 (3), 895–905. doi:10.1104/pp.110.155119
Varotkar, P., Tumane, P. M., and Wasnik, D. D. (2016). Bioconversion of Waste Paper into Bio-Ethanol by Co-culture of Fungi Isolated from Lignocellulosic Waste. Int. J. Pure App. Biosci. 4 (4), 264–274. doi:10.18782/2320-7051.2329
Wanjohi, B. K. (2018). Solid Waste Management and Effectiveness in the Context of Devolved System of Governance in Kenya: the Case Study of Kiambu County Municipal Areas.” in A Research Project Submitted in Partial Fulfillment of the Requirements for the Award of the Degree of Master of Arts in Sociology (Disaster Mangement). University of Nairobi.
Yan, P., Xu, Z., Zhang, C., Liu, X., Xu, W., and Zhang, Z. C. (2015). Fractionation of Lignin from eucalyptus Bark Using Amine-Sulfonate Functionalized Ionic Liquids. Green Chem. 17 (11), 4913–4920. doi:10.1039/c5gc01035g
Yáñez, R., Moldes, A. B., Alonso, J. L., and Parajó, J. C. (2003). Production of D (−)-lactic Acid from Cellulose by Simultaneous Saccharification and Fermentation Using Lactobacillus Coryniformis Subsp. Torquens. Biotechnol. Lett. 25 (14), 1161.
Yang, B., Dai, Z., Ding, S.-Y., and Wyman, C. E. (2011). Enzymatic Hydrolysis of Cellulosic Biomass. Biofuels 2 (4), 421–449. doi:10.4155/bfs.11.116
Zhao, X., Zhang, L., and Liu, D. (2012). Biomass Recalcitrance. Part II: Fundamentals of Different Pre-treatments to Increase the Enzymatic Digestibility of Lignocellulose. Biofuels, Bioprod. Bioref. 6 (5), 561–579. doi:10.1002/bbb.1350
Keywords: bioconversion, fermentation, municipal waste, hydrolysis, pollution
Citation: Ojewumi ME, Emetere ME, Obanla OR, Babatunde DE and Adimekwe EG (2022) Bio-Conversion of Waste Paper Into Fermentable Sugars—A Review. Front. Chem. Eng. 4:926400. doi: 10.3389/fceng.2022.926400
Received: 22 April 2022; Accepted: 13 June 2022;
Published: 17 August 2022.
Edited by:
Papita Das, Jadavpur University, IndiaReviewed by:
Dolanchapa Sikdar, Guru Nanak Institute of Technology, IndiaSagnik Chakraborty, Jiangsu University, China
Copyright © 2022 Ojewumi, Emetere, Obanla, Babatunde and Adimekwe. This is an open-access article distributed under the terms of the Creative Commons Attribution License (CC BY). The use, distribution or reproduction in other forums is permitted, provided the original author(s) and the copyright owner(s) are credited and that the original publication in this journal is cited, in accordance with accepted academic practice. No use, distribution or reproduction is permitted which does not comply with these terms.
*Correspondence: Modupe E. Ojewumi, bW9kdXBlLm9qZXd1bWlAY292ZW5hbnR1bml2ZXJzaXR5LmVkdS5uZw==, b3JjaWQub3JnLzAwMDAtMDAwMi05MjU0LTI0NTA=