- 1Department of Chemical Engineering, University of Gujrat, Gujrat, Pakistan
- 2Department of Environmental and Biological Sciences, University of Eastern, Kuopio, Finland
- 3Department of Chemical Engineering, Dalian University of Technology, Dalian, China
In an agricultural country like Pakistan, producing affordable and clean energy can be a challenging task. However, Pakistan has the potential to utilize various biomass feedstocks to generate renewable energy and tackle climate change while promoting sustainable development. Wheat, rice, sugarcane, and corn are the four main crops that yield a significant amount of residue, totaling 112.1 million tons per year. These residues have the potential to produce 3,050 kWh/ton of energy, which can meet 14% of the energy demand in Pakistan, equivalent to 9.85TW, starting in 2022. Gasification technology is a versatile option that efficiently converts biomass into energy while reducing negative environmental impacts. The current research explores the feasibility of generating clean energy from crop residues with low emissions, addressing the country’s energy needs, and supporting policymakers in promoting the use of biomass for energy production. According to this study, rice husk, corn cobs, wheat straw, and sugar bagasse all produce hydrogen at rates of 6.9 wt.%, 6.4 wt.%, 5.69 wt.%, and 5.35 wt.%, respectively. Therefore, our study demonstrates that corn cobs have a significant potential for energy production.
1 Introduction
1.1 Biomass waste and energy production
According to Ren et al. (2019), energy is essential to any country’s ability to develop economically. Fossil fuels, including coal, crude oil, and natural gas, are the main fuels used to generate energy around the globe. However, their use pollutes the atmosphere, drives up energy prices, and depletes fossil fuel reserves. The excessive usage of fossil fuels leads to an increase in carbon dioxide, which is the most hazardous gas and contributes to a 30% rise in global warming’s effects by raising the surface temperature (Chai et al., 2021).
Biomass has emerged as a highly appealing replacement for fossil fuels, particularly for agricultural countries, and currently is the third most significant energy source for both heating and power (Saletnik et al., 2018). In recent years, the applications and purposes of biomass as a renewable energy source have expanded significantly (Dunn, 2020). It has been reported that carbon dioxide emissions from biomass during combustion are equal to the carbon sequestered during the plants’ growth. Additionally, the use of biomass-derived energy has socioeconomic and natural advantages that enhance the standard of living for those who work or belong to the agriculture sector. Thus, growing the role of biomass in the energy mix can boost job opportunities and elevate the living standards for people engaged in the production of biomass and the development of biomass conversion technologies. Additionally, it may extend the spectrum of resources and lifestyles available to people. Biomass currently makes up between 10% and 14% of the world’s energy supply, and it has traditionally been a vital source of energy for humans (Sonal, 2019).
Numerous processes have been devised for converting biomass into synthetic gas, biofuels, and chemicals. Among these technologies, the gasification process appears to be the most promising due to its numerous advantages. Gasification yields syngas, which can be utilized for electricity generation through a gas turbine, engines, and for producing different fluid fuels (Mojaver et al., 2019; Ramalingam et al., 2020; Ozbas et al., 2019; Zhang et al., 2019; Kumar et al., 2019; Salam et al., 2018; Kalinci et al., 2019; Ren et al., 2020). In practice, gasification relies on five essential processes: oxidation, reduction, pyrolysis, cracking, and drying. These processes convert biomass into syngas composed of hydrogen, carbon monoxide, carbon dioxide, methane, and other hydrocarbons. Gasification occurs with the addition of oxygen or air at high temperatures ranging from 700 to 1,000°C (M.A. Salam et al., 2018; Aydin et al., 2019). Several factors, including the type of reactor, feedstock flow rate, residence time, reactor pressure, and temperature, have an impact on the composition of syngas (Maisano et al., 2019).
The present study highlights the utilization of locally sourced biomass feedstock for power generation via the gasification process and specifically focuses on wheat straw, rice husk, sugarcane bagasse, and corn cob as potential sources because of their high heating value and abundance in Pakistan. However, Pakistan is generating electricity from other sources i.e., wind and solar, coal, oil products, nuclear, natural gas and hydro. The power generation from these routes are mentioned in Figure 1B.
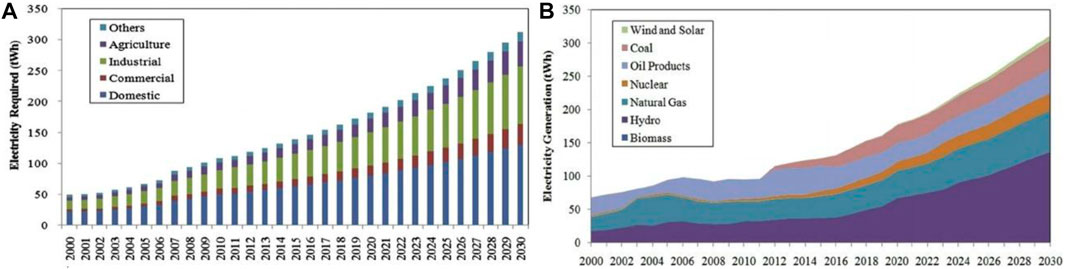
FIGURE 1. (A) Illustration of the electricity requirement and (B) electricity generation of Pakistan from 2000 to 2030 (Usama Perwez et al., 2015).
2 Agriculture crop residues scenario
Agriculture is the backbone of Pakistan’s economy. The majority of the population of Pakistan (65%–70%) depends upon agriculture for their livelihood. The most common crops in Pakistan are wheat, rice, corn, and sugarcane. The potential of these crops can be seen from Table 1. We obtained the rice straw, rice husk, wheat straw, corn cobs, and sugarcane bagasse from these agricultural crops (MOF, 2021). Pakistan has ranked 11th among the world’s top paddy rice producers, with a production of 10 million tons. The paddy-to-paddy rice ratio is important because a milling process is used. In the first stage, most of the husk is removed. In the second stage, the remaining husk and the broken pieces of rice are removed. This type of process is done in rice mills (Butt et al., 2019). In 2018, worldwide rice production was around 770 million tons of paddy rice; of these, 510 million tons is the husk (Akhter et al., 2021). Wheat, rice, corn/maize, and sugarcane are the major crops in Pakistan and have a prominent place in Pakistan’s economy. In 2021 and 2022, Pakistan produced 25.5 million tons of wheat, 10 million tons of rice, 70 million tons of sugarcane, and 6.6 million tons of corn/maize. Rice, wheat, and sugarcane are cultivated in the province of Punjab because of the good water system. Corn/maize is cultivated in the provinces of Punjab, Sindh, and Khyber Pakhtunkhwa (PARC, 2021; Punjab, 2021; USDA, 2022).

TABLE 1. Pakistan’s annual crop production and its potential to produce electricity (USDA, 2022; AEBD, n.d.).
Table 2 illustrates the potential energy of 2021 that could be produced from wheat straw, rice straw, sugarcane bagasse, and corn cobs (USDA, 2022; AEBD, n.d.).
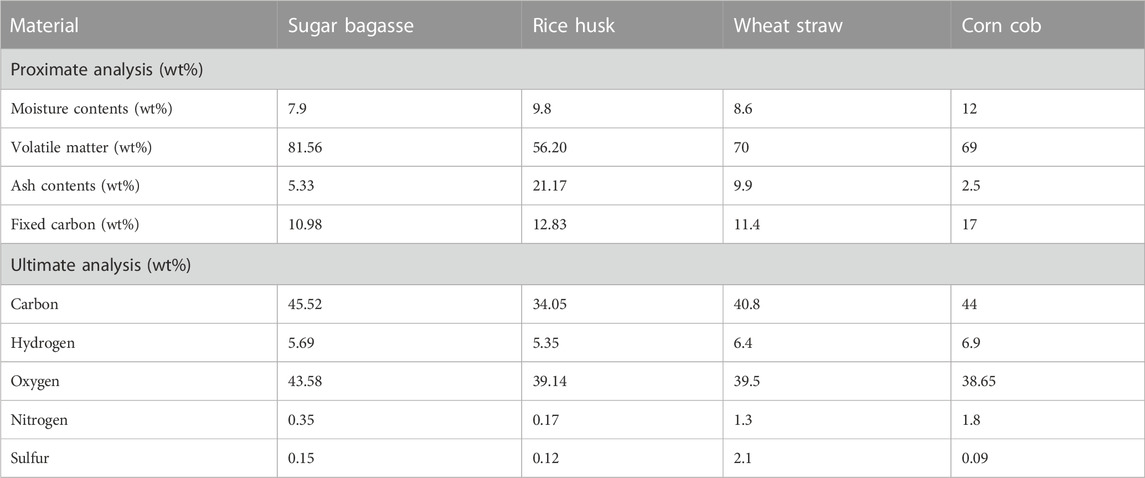
TABLE 2. Potential of energy from the analysis of the crop residues (Amin, 2018; Hafemann et al., 2020; Mishra et al., 2022; Pisutpaisal, 2022).
The potential energy production values listed in the table are approximate and can vary depending on factors such as the moisture content of biomass, the efficiency of the conversion process, and the type of technology used. The values are based on estimates and should be used as a general guide only. Note that the actual energy production can vary depending on the specific conditions and circumstances (USDA, 2022; AEBD). According to the Pakistan Electric Power Company (PEPCO), the annual energy demand in Pakistan has been steadily increasing in recent years. In 2020, the estimated demand for electricity was around 27,000 MW. The supply of electricity in Pakistan has not been able to keep up with the increasing demand. In 2020, the installed generation capacity was 33,346 MW and available was 38,719 MW, resulting in a shortfall of around 3,654 MW. As shown in Figure 1A, which depicts the electricity requirement and demand of Pakistan. This supply–demand gap has resulted in frequent power outages and load shedding in the country, particularly during the peak summer months. To address this issue, the government has been working to increase the country’s electricity generation capacity through various measures, such as building new power plants and encouraging investment in the renewable energy sector (Company, 2020).
Biomass gasifiers are divided into two main categories: fixed bed and fluidized bed. Some gasifier designs are described as follows. Fixed bed gasifier (updraft and downdraft): the gasifier’s classification is based on how the gasifying agent (air or steam) flows through the fixed bed, which is made of carbonaceous material. Updraft gasifier: a simple, inexpensive design in which biomass enters from the top and air enters from the bottom. A different name for this type is counterflow gasification. Downdraft gasifier: the biomass feed enters the gasifier from the upper section at the same time as the air is moving. Fluidized bed gasifier (bubbling bed, circulating fluidized bed): a warmed granular (sand) bed is loaded with feedstock particles from the reactor side, and air or steam is fed through the bottom to fluidize the biomass particles in the gasifier. Compared to updraft or downdraft gasifiers, the gasifier’s upward drag force thoroughly mixes the air inside with the suspended solid particles, increasing the amount of solid–fluid interactions (Golpour et al., 2017).
Sugarcane bagasse was used to perform the syngas production experiments using a downdraft gasifier. The downdraft gasifier working setup is shown in Figure 2, in which air is given from the top and takes the product gas from the bottom of the gasifier. The biomass feeds elemental analysis was performed with an elemental analyzer (CE 400). A bomb calorimeter (EE Bomb 1,341) was used to measure biomass heating values. The biomass sample was heated in an oven at a temperature of 104°C for 24 h to measure the moisture content of the feed mass sample. The biomass feed used in gasifiers is relatively uniform in size and shape. The high heating value and low heating value of biomass fuel were calculated by the equations given in Amin (2018), Hafemann et al. (2020), Mishra et al. (2022), and Pisutpaisal (2022). The feedstock is composed of C, H, S, O, and N.
Pakistan has not fully utilized its potential for producing electricity from biomass resources due to several reasons, including the lack of infrastructure, high capital costs, limited technology availability, uncertainty around feedstock availability, and a lack of government support. These factors have contributed to the slow development of the biomass-based electricity generation sector (Saletnik et al., 2018; Kumar et al., 2019; Mojaver et al., 2019; Ozbas et al., 2019; Ren et al., 2019; Sonal et al., 2019; Zhang et al., 2019; Dunn, 2020; Ramalingam et al., 2020; Chai et al., 2021) in Pakistan (Shabbir, 2017).
According to a report by the International Renewable Energy Agency (IRENA), Pakistan has significant potential for producing electricity from biomass resources. The report notes that the country has an estimated 25 million tons of crop residues, 22 million tons of animal manure, and 2.2 million hectares of forest land that could be used for biomass-based electricity generation (IRENA, 2015). However, despite this potential, the use of biomass for electricity generation remains relatively low in Pakistan. According to the Pakistan Renewable Energy and Energy Efficiency Status Report 2020, biomass-based electricity generation accounted for only 0.6% of the country’s total electricity generation in 2019 (AEBD, 2020).
The report highlights several barriers to developing the biomass-based electricity generation sector in Pakistan, including the lack of a supportive policy and regulatory framework, inadequate infrastructure, and limited access to financing. These barriers have made it difficult for investors to justify the high capital costs associated with setting up biomass-based power plants (AEBD; AEBD, 2020). Additionally, a study published in the Journal of Renewable and Sustainable Energy in 2017 found that the low cost of fossil fuel-based electricity generation in Pakistan has also been a significant obstacle to the development of the biomass-based electricity generation sector (Shabbir, 2017). In summary, the slow development of the biomass-based electricity generation sector in Pakistan is due to a combination of factors, including inadequate infrastructure, high capital costs, limited technology availability, uncertainty around feedstock availability, and a lack of government support (Butt et al., 2019; IRENA, 2015).
Pakistan’s energy crisis has been a significant challenge for the country, and while biomass resources have the potential to contribute to the energy mix, they are unlikely to be a comprehensive solution on their own (Shabbir, 2017; Butt et al., 2019; Khokhar, 2018). According to the Pakistan Renewable Energy and Energy Efficiency Status Report 2020, biomass-based electricity generation accounted for only 0.6% of the country’s total electricity generation in 2019. This suggests that the use of biomass resources has not been significant enough to overcome the country’s energy crisis (AEBD, 2020). However, the report notes that there is still potential for biomass-based electricity generation to increase in Pakistan, particularly through the use of agricultural waste and forestry residue. The report estimates that the country has an estimated 25 million tons of crop residues and 22 million tons of animal manure that could be used for biomass-based electricity generation (MOF, 2021; PARC, 2021).
Several steps must be taken to fully realize the potential of biomass-based electricity generation in Pakistan. These include improving infrastructure for biomass collection and storage, supporting research and development to improve biomass processing technologies, and providing incentives for private sector investment. Additionally, the government could consider policies to encourage the use of biomass in industrial processes, such as in the production of biofuels or for heating (Khokhar, 2018; AEBD, 2020). While biomass resources can play a role in Pakistan’s energy mix, it is unlikely that they will be a comprehensive solution to the country’s energy crisis. Other renewable sources of energy, such as wind power and solar, will also need to be developed to supply the country’s growing energy demand (AEBD, n.d.; Khokhar, 2018).
In Pakistan, renewable resources of biomass have been explored as potential feedstocks for the gasification process to produce energy, with a focus on using crop residues as a source of biomass. Several studies and initiatives have been undertaken to evaluate the feasibility of using crop residues as a source of renewable energy (Amin, 2018; Javaid and Kothari, 2018; Shahzad, 2019). One study evaluated the potential of using wheat straw and corn cobs as feedstock for gasification in Pakistan. The study found that agricultural waste, including crop residues, could be a promising source of biomass for gasification and could help meet the energy needs of rural communities (Amin, 2018; Shahzad, 2019). Another study focused on the performance evaluation of a gasification system using rice husk as a feedstock. The study found that the gasification system produced high-quality syngas that could be used for power generation (Javaid and Kothari, 2018).
One study focused on the performance evaluation of a gasification system using cotton stalks as a feedstock and found that the gasification system produced high-quality syngas that could be used for electricity generation (Shahzad, 2019). In addition, the government of Pakistan has implemented several initiatives to promote the use of renewable sources of energy, including biomass waste. For instance, the Alternative Energy Development Board (AEDB) has launched various projects related to biomass energy, such as the installation of biomass power plants in different parts of the country (AEBD, 2020; AEBD, n.d.). Furthermore, the Punjab Bio Energy Company (PBECL) has been established to promote the use of renewable energy in the province of Punjab. PBECL is responsible for implementing various renewable energy projects, including biomass gasification, to address the energy crisis in the region (Company, 2021). Traditional fuels, including firewood, dung, and crop leftovers, currently comprise a significant portion of how people in rural and low-income urban communities satisfy their daily energy needs. Much of the population (67%) resides in rural settings. As a result, the use of traditional fuels is much more prevalent in rural areas where the use of firewood for heating and cooking is more prevalent. Crop waste and animal manure are used when firewood is in short supply.
According to the 1991–1993 Household Energy Strategy Study (HESS), by 1992, biomass fuels were predicted to be contributing an energy equivalent of 19,256 TOE, or almost 27% of Pakistan’s overall energy supply. An estimated 60% of this supply came from firewood, with the remaining 21% coming from crop waste, 18% from manure, and 1% from charcoal.
Through a network of community biogas plants, Pakistan’s rural areas have high prospects for employing biogas energy. The amount of dung waste is sufficient to produce 21 million tonnes of bio-fertilizer annually and 12 million cubic meters of biogas per day, which is enough to cover the needs of 28 million rural residents. In 1974, the Ministry of Petroleum and Natural Resources’ Directorate General of New and Renewable Resources (DGNRER) launched a comprehensive biogas program that resulted in the commissioning of 4,137 biogas units around the nation by 1987. The units were built to produce 3,000 and 5,000 cubic feet of biogas daily that could be used in cooking and lighting (Mirza, Ahmad, and Majeed, 2008).
The government of Pakistan has taken several initiatives to promote the use of renewable energy sources, including biomass. The Alternative Energy Development Board (AEDB) has launched various projects related to biomass energy, such as the installation of biomass power plants in different parts of the country (AEBD; AEBD, 2020). Overall, these studies and initiatives demonstrate that crop residues can be a promising source of renewable energy for Pakistan, and gasification can be an effective way to convert crop residues into energy (AEBD, 2020; Amin, 2018; Javaid and Kothari, 2018; Shahzad, 2019).
Biomass gasification is the process of converting solid biomass waste, such as wood, agricultural waste, and municipal waste, into a gas mixture that can be used for energy production. This technology has several important benefits and applications, including reducing greenhouse gas emissions, producing renewable energy, and providing a source of locally sourced fuel (Sipilä, 2018).
3 Discussion
Gasification of biomass is a thermochemical process used to produce clean energy that can substitute for fossil fuels. The present study reveals that Pakistan has the potential to generate power by utilizing indigenous agricultural crops, as the annual production of wheat straw, rice straw, sugarcane bagasse and corncob is 25.5, 10, 70, and 6.6 million tons, respectively. These crops have the potential to produce 9.85 TW, which can fulfill 14% of Pakistan’s total energy demand. However, due to technological challenges, it is difficult for a developing country like Pakistan to shift to a new energy source that requires more study and practical knowledge for commercialization. Furthermore, researchers need to focus more on detailed characterization investigation to address the shortfall.
Some of the specific benefits and advantages of biomass gasification include renewable energy, reduction of greenhouse gas, reduction of waste, local fuel source, and flexibility (Bridgwater, 2019; Alipour Moghadam, 2019; Biomass et al., 2022). Renewable energy: biomass gasification produces a renewable source of energy that can be used to produce heat, electricity, and fuel. Unlike fossil fuels, which are finite and non-renewable, biomass is a sustainable resource that can be continuously replenished. Reduction of greenhouse gas emissions: biomass gasification can help reduce greenhouse gas emissions, which are a major contributor to climate change. When biomass is used as a fuel, it releases carbon dioxide into the atmosphere, but this is offset by the carbon dioxide that is absorbed by plants during their growth. Reduced waste: biomass gasification can be used to convert waste materials, such as agricultural residues and municipal solid waste, into energy. This can help reduce the amount of waste that is sent to landfills and also provide a source of energy that would otherwise go unused. Local fuel source: biomass gasification can provide a source of locally sourced fuel, which can help reduce dependence on imported fuels. This can also provide economic benefits by creating jobs and supporting local businesses. Flexibility: biomass gasification can be used with a wide range of feedstocks, including wood chips, sawdust, agricultural waste, and municipal solid waste. This provides flexibility in terms of the types of materials that can be used as fuel.
An updraft gasifier produces less pressure drop and good thermal efficiency but is highly sensitive to tar and fuel moisture content, and the internal combustion zone startup requires a long time. A downdraft gasifier is less sensitive to charcoal dust and fuel tar content and has an easy-to-control system and reduced maintenance cost due to a minimal wear rate. However, it is less feasible for small-particle-size fuel and difficult to handle when feedstock contains a high content of moisture and ash. A crossdraft gasifier has a compact design and high gas production. However, it is sensitive to slag formation, high pressure drops, and limited ash material. A fluidized bed has high heat transfer characteristics and good gas-to-solid contact, feasible temperature control, and a high degree of mixing, but it also has a high rate of tar production and a high capital cost. An entrained gasifier has high throughput and rapid feed conversion as a consequence of high temperature and pressure, but its thermal efficiency is reduced if water is introduced in the gasifier due to the slurry feedstock. A plasma bed does not require pulverized feedstock, but it has high operational costs and is highly corrosive due to the nature of the plasma flame.
4 Conclusion
Experiments were conducted for sugar bagasse, rice husk, wheat straw, and corn cobs in the downdraft biomass gasifier. The moisture content of sugar bagasse, rice husk, wheat straw, and corn cobs was 7.9 wt.%, 9.8 wt.%, 8.6 wt.%, and 12 wt%, respectively. The uses and functions of biomass as a renewable energy source have greatly increased in recent years. Energy is a crucial aspect of a country’s economic development, and up to 65%–70% of Pakistan’s economy depends on agriculture. The gasification of rice husk, cotton stalks, and sugar cane bagasse demonstrates that high-quality syngas is produced, which is then used to generate electricity. One resource that has the potential to meet the demands of rural communities is wheat straw and corn cobs. Tonnes of various garbage are produced each day, and we are attempting to use various feedstocks in order to provide safe and clean energy.
Author contributions
AA, MT and ZN contributed most of the part, though KA, HN and MT help in reviewing, proof reading etc.
Conflict of interest
The authors declare that the research was conducted in the absence of any commercial or financial relationships that could be construed as a potential conflict of interest.
Publisher’s note
All claims expressed in this article are solely those of the authors and do not necessarily represent those of their affiliated organizations, or those of the publisher, the editors, and the reviewers. Any product that may be evaluated in this article, or claim that may be made by its manufacturer, is not guaranteed or endorsed by the publisher.
References
AEBD Alternative energy development board. [Online] Available at: http://www.aebd.org.
AEBD, 2020. Pakistan renewable energy and energy efficiency status report 2020. [Online] Available at: https://www.aedb.org/RE%20Status%20Report%202020.pdf.
Akhter, F., Ahmed Soomro, S., Rauf Jamali, A., Ahmed Chandio, Z., and Muhammad, S. (2021). Rice husk ash as green and sustainable biomass waste for construction and renewable energy applications: A review. Germany: Biomass Convers. Biorefinery, 897–912.
Alipour Moghadam, E. (2019). An overview of biomass gasification and its potential applications. Renew. Sustain. Energy Rev. 104, 348–362.
Amin, M. I. M. A. N. (2018). Agricultural waste as a potential feedstock for gasification: A case study of Pakistan. Energy Sources, Part A Recovery, Util. Environ. Eff. 40 (3), 277–284.
Aydin, E. S., Yucel, O., and Sadikoglu, H. (2019). Experimental study on hydrogen-rich syngas production via gasification of pine cone particles and wood pellets in a fixed bed downdraft gasifier. Hydrogen Energy. 44, 17389–17396. doi:10.1016/j.ijhydene.2019.02.175
Biomass, A., Krylova, A. Y., Zhagfarov, F. G., and Krysanova, K. O. (2022). Animal biomass as a raw material for basic organic synthesis products. Chem. Technol. Fuels Oils 58, 46–50.
Bridgwater, A. V. (2019). Review of fast pyrolysis of biomass and product upgrading. Biomass Bioenergy 38, 68–94. doi:10.1016/j.biombioe.2011.01.048
Chai, W. S., Bao, Y., Jin, P., Tang, G., and Zhou, L. (2021). A review on ammonia, ammonia-hydrogen and ammonia-methane fuels, Renew. Sustain. Energy Rev. 147, 111–254. doi:10.1016/j.rser.2021.111254
Company, P. B. E., 2021. Punjab bio energy Company. [Online] Available at: http://www.eproc.punjab.gov.pk.
Company, P. E. P., 2020. Pakistan electric power Company. Annual Report 2020. [Online] Available at: http://www.pepco.gov.pk/annual-reports/PEPCO%20Annual%20Report%202020.pdf.
Golpour, H., Boravelli, T., Smith, J. D., and Safarpour, H. R. (2017). Production of syngas from biomass using a downdraft gasifier. Int. J. Eng. Res. Appl. 7 (6), 61–71. doi:10.9790/9622-0706026171
Hafemann, E., Bresolin, D., Marangoni, C., and Machado, R. A. F. (2020). Enhancing chlorine-free purification routes of rice husk biomass waste to obtain cellulose nanocrystals. Waste Biomass Valorization 11, 6595–6611. doi:10.1007/s12649-020-00937-2
IRENA (2015). International renewable energy agency. [Online], Available at: httpp//:www.irena.org/-/media/Files/IRENA/Agency/Publication/2015/IRENA REmap Pakistan report 2015.pdf.
Javaid, S. S. A. M. H. A., and Kothari, K. D. (2018). Performance evaluation of a gasification system using cotton stalks as feedstock for power generation. Energy Sources, Part A Recovery, Util. Environ. Eff. 40 (15), 1797–1806.
Kalinci, Y., Hepbasli, A., and Dincer, I. (2019). Biomass-based hydrogen production: A review and analysis, int. J. Hydrogen Energy. 34, 8799–8817.
Khokhar, I. (2018). Pakistan's energy crisis: Causes, consequences, and possible remedies. J. Energy Res. Rev. 2 (4), 1–10.
Kumar, M., Olajire Oyedun, A., and Kumar, A. (2019). A comparative analysis of hydrogen production from the thermochemical conversion of algal biomass. Hydrogen Energy 44, 10384–10397. doi:10.1016/j.ijhydene.2019.02.220
Mishra, K., Samarjeet Singh S., , , Adesh Kumar, S., and Vijay Kumar, T., 2022. Recent update on gasification and pyrolysis processes of lignocellulosic and algal biomass for hydrogen production. Fuel, Volume vol, 332, p. no. 2. doi:10.1016/j.fuel.2022.126169
Maisano, S., Cipitì, F., Freni, F., and Chiodo, V. (2019). Syngas production by BFB gasification: Experimental comparison of different biomasses. Hydrogen Energy 44, 4414–4422. doi:10.1016/j.ijhydene.2018.11.148
Mojaver, P., Khalilarya, S., and Chitsaz, A. (2019). Study of synthesis gas composition, exergy assessment, and multi-criteria decision-making analysis of fluidized bed gasifier. Hydrogen Energy 44, 27726–27740. doi:10.1016/j.ijhydene.2019.08.240
Ozbas, E. E., Ongen, A., Aydin, M. A., and Ozcan, H. K. (2019). Hydrogen production via biomass gasification, and modeling by supervised machine learning algorithms. Hydrogen Energy. 44, 17260–17268. doi:10.1016/j.ijhydene.2019.02.108
PARC, 2021. Pakistan agriculture research council. [Online] Available at: http://www.parc.gov.pk.
Pisutpaisal, V. S. a. N. (2022). Rice husk ash characterization and utilization as a source of silica material. Chem. Eng. 93, 79–84. doi:10.3303/CET2293014
Punjab, A., 2021. Agri Punjab. [Online] Available at: http://www.agripunjab.gov.pk.
Ramalingam, S., Rajendiran, B., and Subramiyan, S. (2020). Recent advances in the performance of Co-current gasification technology: A review. Hydrogen Energy 45, 230–262. doi:10.1016/j.ijhydene.2019.10.185
Ren, J., Jing-Pei, C., Xiao-Yan, Z., Fei-Long, Y., and Xian-Yong, W. (2019). Recent advances in syngas production from biomass catalytic gasification: A critical review on reactors, catalysts, catalytic mechanisms and mathematical models, renew. Sustain. Energy Rev. 116, 1094. doi:10.1016/j.rser.2019.109426
Ren, J., Zhao, X. Y., and Cao, J. P. (2020). Methanation of syngas from biomass gasification: An overview. Hydrogen Energy. 45, 4223–4243. doi:10.1016/j.ijhydene.2019.12.023
Salam, M. A., Akter, N., Hossain, T., and Abdullah, B. (2018). A review of hydrogen production via biomass gasification and its prospect in Bangladesh. Hydrogen Energy 43, 14944–14973. doi:10.1016/j.ijhydene.2018.06.043
Saletnik, B., Czernicka, M., and Puchalski, C. (2018). Biochar and biomass ash as a soil ameliorant: The effect on selected soil properties and yield of giant miscanthus (miscanthus x giganteus). (Miscanthus x giganteus), Energies Vol. 11, 2535. doi:10.3390/en11102535
Butt, S., Hartmann, I., and Lenz, V. 2019. Bioenergy potential and consumption in Pakistan. Biomass Bioenergy, Volume vol. 58, pp. p. 379–389. doi:10.1016/j.biombioe.2013.08.009
Shabbir, M. S. A. (2017). Biomass energy status and future trends in Pakistan. J. Renew. Sustain. Energy 9 (4), 403–405.
Shahzad, K. M. U. K. A. M. (2019). Performance evaluation of rice husk gasification for rural electrification in Pakistan. Int. J. Energy Res. 43 (12), 6274–6286.
Sipilä, K. (2018). Biomass gasification: A review of its technology, sustainability, and application. J. Renew. Resour. Sustain. 10 (9), 3194.
Sonal, E. A. S. U. K. P., Ahmad, E., Upadhyayula, S., and Pant, K. K. (2019). Biomass-derived CO2 rich syngas conversion to higher hydrocarbon via Fischer-Tropsch process over Fe–Co bimetallic catalyst. Hydrogen Energy. 44, 27741–27748. doi:10.1016/j.ijhydene.2019.09.015
Tariq Dilbar, M., Farhan, M., Ali Nawaz, M., Waqar Durrani, A., and Ali Memon, K. (2020). Present Status and potential of biomass energy in Pakistan based on existing and future renewable resources. Isalamabad: Renewable energy.
Usama Perwez, A., Sohail, A., Hassan, S. F., and Zia, U. (2015). The long-term forecast of Pakistan's electricity supply and demand:An application of long range energy alternatives planning. Energy 93, 2423–2435. doi:10.1016/j.energy.2015.10.103
Keywords: biomass, synthetic gas (syngas), gasification (biomass), energy, fossil fuels, electricity, Pakistan, energy demand and energy supply
Citation: Ali A, Ayub KS, Tahseen Sadiq M, Tanveer M, Naseer H, Nadeem Z and Aamir HM (2023) Potential and prospects of biomass as a source of renewable energy in Pakistan. Front. Chem. Eng. 5:1193806. doi: 10.3389/fceng.2023.1193806
Received: 25 March 2023; Accepted: 29 May 2023;
Published: 11 July 2023.
Edited by:
Muhammad Sajid, Yibin University, ChinaReviewed by:
Waqas Qamar Zaman, National University of Sciences and Technology (NUST), PakistanNisar Ali, Huaiyin Institute of Technology, China
Copyright © 2023 Ali, Ayub, Tahseen Sadiq, Tanveer, Naseer, Nadeem and Aamir. This is an open-access article distributed under the terms of the Creative Commons Attribution License (CC BY). The use, distribution or reproduction in other forums is permitted, provided the original author(s) and the copyright owner(s) are credited and that the original publication in this journal is cited, in accordance with accepted academic practice. No use, distribution or reproduction is permitted which does not comply with these terms.
*Correspondence: Asad Ali, YXNhZC5hbGlAdW9nLmVkdS5waw==
†These authors have contributed equally to this work