- 1Biocatalysis and Organic Synthesis Group, Chemistry Institute, Federal University of Rio de Janeiro, Rio de Janeiro, Brazil
- 2Laboratório de Microbiologia Molecular e Proteínas, Biochemistry Department, Chemistry Institute, Federal University of Rio de Janeiro, Rio de Janeiro, Brazil
- 3Department of Chemistry, Federal University of São Carlos, São Carlos, São Paulo, Brazil
- 4Department of Biotechnology, Delft University of Technology, Delft, Netherlands
A visible-light-driven photocatalytic decarboxylation of palmitic acid and related fatty acids is described in this study. Remarkable decarboxylation rates have been observed with full conversion in less than 20 min. In this study, we have demonstrated that sunlight irradiation, even on cloudy days, can deliver similar results to traditional LED lamps while using much less energy and minimizing environmental impact. The findings indicate that the process of enzymatic decarboxylation could be useful for the production of different biofuels in the future.
1 Introduction
Ten years have passed since the 100th anniversary of the article published by Giacomo Ciamician in which he challenges chemists, especially organic chemists, to imagine a chemical industry that could mimic plants by using a more sustainable and renewable process to synthesize organic molecules (Ciamician, 1912). In the past decades, chemists have made remarkable advances regarding the use of light to perform chemical reactions and the renascence of organic photochemistry is underway (Akita and Koike, 2015). The biocatalysis field has also been part of this development with an increasing number of publications on photobiocatalysis where enzymes or co-factors could be activated or regenerated by light (Peng et al., 2021). Some examples of natural photobiotransformations include chlorophyll biosynthesis (Heyes et al., 2021), the repair of light-damaged DNA (Weber, 2005) and the photodecarboxylation of long-chain acids to the corresponding alkane/alkene via fatty acid photodecarboxylase (FAP) (Huijbers et al., 2018; Sorigué et al., 2018; Xu et al., 2019; Lakavath et al., 2020). Advances in photochemistry, specifically in the development of LED technology, have allowed a significant improvement in the investigation of new light-dependent reactions, transforming photochemistry into a powerful tool for organic chemistry and catalysis (Xie et al., 2020). Interestingly, the majority of photochemical studies do not utilize real sunlight, the ultimate green source of energy, but rather focus on artificial illumination (Esser et al., 19194). From a Green Chemistry perspective, the Sun represents a virtually inexhaustible energy source (Sambiagio and Noël, 2020). Therefore, we became interested in exploring the possibility of applying the experience of our group (Benincá et al., 2022) to further develop the field of sunlight decarboxylation mediated by Chlorella variabilis FAP (CvFAP).
2 Materials and methods
2.1 Strain, vector, and materials
Escherichia coli DH5α was used for DNA manipulation. The plasmid containing the CvFAP gene (short length; comprising residues 62-654) has been reported previously (Huijbers et al., 2018). Competent E. coli BL21 (DE3) cells were transformed with the plasmid for recombinant enzyme production. Kanamycin was purchased from Fluka. Palmitic acid was purchased from Sigma-Aldrich.
2.2 Preparation of the enzymatic extract
Enzyme expression was performed as described by Huijbers et al. (2018). After enzyme expression, cells were harvested by centrifugation (11,000 g at 4°C for 10 min), washed twice with washing buffer (50 mM Tris-HCl buffer, pH 8, containing 100 mM NaCl), resuspended in the same buffer with the addition of 1 mM PMSF and 20% glycerol, and stored at −80°C. The cells were prepared for lysis by centrifugation and resuspension in lysis buffer (100 mM Tris-HCl buffer, pH 8.5, containing 1 mM PMSF and 5% glycerol). Cell lysis was performed by VIBRA-CELL VCX 500 Sonics (United States) instrument using the following settings: 30% amplitude; 15 min sonication; ON/OFF pulses of 10 s on and 30 s off. The obtained extract was frozen with liquid nitrogen and stored at −80°C to be used in the photocatalytic reactions. The total protein concentration of the extracts was determined and standardized by Smith (1985) assays (Smith et al., 1985).
2.3 Photocatalytic reactions in batch with solar light
The photoenzymatic decarboxylation reactions using solar light irradiation were carried out in an open glass jacketed reactor at 35°C. The reactions were performed in Tris-HCl buffer (pH 8.5, 100 mM) containing 30 vol% DMSO as cosolvent and 13 mM of palmitic acid as substrate. The enzymatic extracts were used in a 5.6 mg × ml−1total protein corresponding to a CvFAP concentration of approx. 6.2 μM final concentration or as indicated. The reactor was exposed to sunlight during the summer season under constant and gentle magnetic stirring starting at 11 a.m. (in Rio de Janeiro, GPS coordinates: −22.8601423 and −43.2297134) on 6 April 2022. Aliquots (20 μl) were withdrawn, and the substrates and products were extracted with 1 ml of ethyl acetate. The remaining organic phase was analyzed by gas chromatography.
2.4 Gas chromatography analysis
Samples were prepared by extracting 20 μl of reaction crude in 1 ml of ethyl acetate. Conversion percentages were analyzed by chromatogram areas using the Shimadzu GC2014 GC-FID–Cpsil 5 CB column (50 m × 0.53 mm × 1.0 µm). Injection temperature 260°C, injection split ratio 20.0, carrier gas was N2, pressure 89.0 kPa, column flow 4.86 ml min−1. The oven temperature setting was: 110°C, heated at 25°C min−1 to 190°C for 3 min, and remained heating at 25°C min−1 to 280°C. Conversion percentages were analyzed by chromatogram area based on a calibration curve.
3 Results
Following our initial results using high power blue LEDs (300 W) to reduce the reaction time for full conversion, we decided to investigate the batch decarboxylation reaction of palmitic acid catalyzed by CvFAP under sunlight irradiation. The first set of experiments was conducted using conditions previously developed by our research group (13 mM of palmitic acid in DMSO/5.6 mg × ml−1 of cell extract at 37°C in Tris-HCl buffer) (Benincá et al., 2022) and evaluated how our reaction set-up would perform in terms of product yield (Table 1).
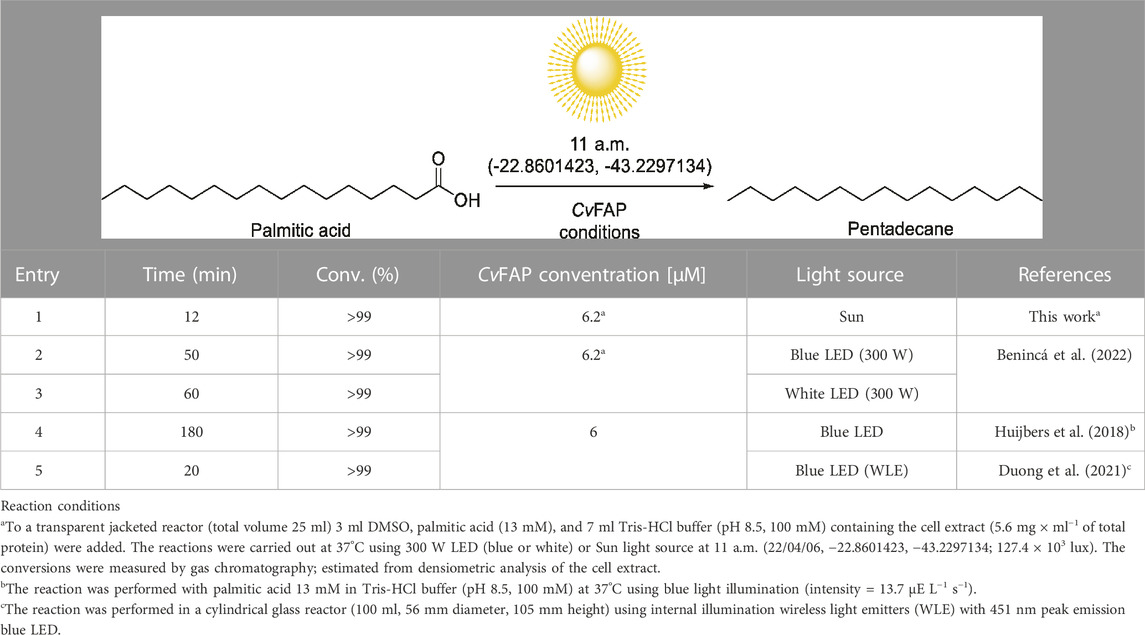
TABLE 1. Initial evaluation of solar light irradiation on photodecarboxylation reaction of palmitic acid mediated by CvFAP.
A remarkable response has been found for CvFAP when the Sun was used as the light source, with conversion rates that were comparable to those found with LED. The results revealed that reductions on reaction time are possible, allowing to achieve excellent conversions in just 12 min of reaction using sunlight (Entry 1, Table 1). Following these results, the total protein concentrations were reduced to 2.8 mg × ml−1 [(CvFAP) ≈ 3.1 μM], and higher palmitic acid concentrations (13–34.5 mM) were evaluated, limiting the reaction time to 1 h. The palmitic acid concentration is an important parameter in process optimizations while we aim to maximize the space-time-yield of the desired reaction system (Table 2).
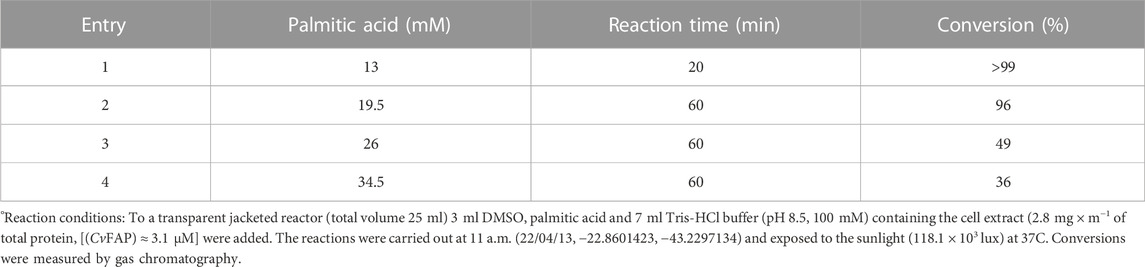
TABLE 2. Influence of higher palmitic acid concentration on the performance of the CvFAP-catalyzed photodecarboxylation under solar light irradiation.
As observed the reduction of total protein to 2.8 mg × ml−1 allowed us to obtain a full conversion of a 13 mM solution of palmitic acid in only 20 min (Entry 1, Table 2). The reaction carried out under higher concentrations (Entry 2, Table 3) proved to be effective in up to 19.6 mM, with a slight decrease in reaction conversion (96%). However, palmitic acid values higher than 26 mM (Entries 3 and 4, Table 3) demonstrate an abrupt decrease in reaction conversion. The turnover number of CvFAP in these experiments was always around 4,100 indicating that either the reaction time was too short to enable higher conversions or that the biocatalyst was photoinactivated.
Previous studies from the literature have shown that the increase in light intensity is efficient for enhancing the productivity of CvFAP (Sorigué et al., 2018; Duong et al., 2021; Benincá et al., 2022), although the present approach shows that the broader wavelength range provided by sunlight including more energetic wavelengths (<420 nm) with lower intensity light source (Loh et al., 2021) still can provide good conversions. In our experiments (Table 3), we observed a reduction in the efficiency of our process which can be attributed to the lower sunlight intensity. However, with these experiments, we were able to prove that even under low sunlight availability or considering different parts of the world where sunlight intensity is lower, the photodecarboxylation works, thus motivating us to investigate the reaction reproducibility under less intense luminosity conditions. The natural lower light condition used for the development of the present experiment are detailed described in the Supplementary Material (Supplementary Section S4.2.6) and their respective results are presented in Table 3.
As can be observed (Entries 3 and 4, Table 3) full conversions were achieved after 60 min, thus revealing that even under unfavorable natural light conditions, the reaction still leads to the desired product. At standard conditions (20 min) a slight decrease could be observed but maintaining conversion value close to the process described at intense lighting using high-power LEDs (Benincá et al., 2022).
4 Conclusion
The results have shown that photodecarboxylation reaction is possible to be performed under sunlight irradiation with very fast reaction times even in cloudy days. The contribution of <400 nm range, a wavelength range shorter than the blue light widely used to date, as a component of sunlight could justify the yields in the sunlight reactions using lower light intensity in comparison to blue LED lamps.
Data availability statement
The original contributions presented in the study are included in the article/Supplementary Material, further inquiries can be directed to the corresponding author.
Author contributions
AF, KD, GB, and RA performed the experimental work and analysed the data.
Funding
This study was financed in part by the Coordenação de Aperfeiçoamento de Pessoal de Nível Superior-Brasil (CAPES)-Finance Code 001. All experimental information is in the supporting information.
Acknowledgments
Authors acknowledge to the National Council for Scientific and Technological Development (Conselho Nacional de Desenvolvimento Cientifico e Tecnologico—CNPq) and to Carlos Chagas Filho Foundation for Support of Research of the State of Rio de Janeiro (Fundação Carlos Chagas Filho de Amparo a Pesquisa do Estado do Rio de Janeiro—FAPERJ) for financial support. Thanks are also due to FAPESP–São Paulo State Research Foundation (K.T.O. grants 2013/07276-1, 2019/27176-8, and 2020/06874-6).
Conflict of interest
The authors declare that the research was conducted in the absence of any commercial or financial relationships that could be construed as a potential conflict of interest.
Publisher’s note
All claims expressed in this article are solely those of the authors and do not necessarily represent those of their affiliated organizations, or those of the publisher, the editors and the reviewers. Any product that may be evaluated in this article, or claim that may be made by its manufacturer, is not guaranteed or endorsed by the publisher.
Supplementary material
The Supplementary Material for this article can be found online at: https://www.frontiersin.org/articles/10.3389/fctls.2023.1165079/full#supplementary-material
References
Akita, M., and Koike, T. (2015). “Sunlight-driven trifluoromethylation of olefinic substrates by photoredox catalysis: A green organic process,” in Comptes rendus chimie (Elsevier Masson SAS), 18, 742–751. doi:10.1016/j.crci.2015.01.013
Bai, X., Yuan, D., Li, Y., Song, H., Lu, Y., San, X., et al. (2021). Ambient sunlight-driven photothermal methanoldehydrogenation for syngas production with 32.9 % solar-to-hydrogen conversion efficiency. iScience 24 (2), 102056. doi:10.1016/j.isci.2021.102056
Benincá, L. A. D., França, A. S., Brêda, G. C., Leão, R. A. C., Almeida, R. v., Hollmann, F., et al. (2022). Continuous-flow CvFAP photodecarboxylation of palmitic acid under environmentally friendly conditions. Mol. Catal. 528, 112469. doi:10.1016/j.mcat.2022.112469
Ciamician, G. (1912). The photochemistry of the future. Science 36 (926), 385–394. doi:10.1126/science.36.926.385
Duong, H. T., Wu, Y., Sutor, A., Burek, B. O., Hollmann, F., and Bloh, J. Z. (2021). Intensification of photobiocatalytic decarboxylation of fatty acids for the production of biodiesel. ChemSusChem 14 (4), 1053–1056. doi:10.1002/cssc.202002957
Esser, P., Pohlmann, B., and Scharf, H.-D. (1994). The photochemical synthesis of fine chemicals with sunlight. Angew. Chem. Int. Ed. Engl. 33, 2009–2023. doi:10.1002/anie.199420091
Heyes, D. J., Zhang, S., Taylor, A., Johannissen, L. O., Hardman, S. J. O., Hay, S., et al. (2021). “Photocatalysis as the ‘master switch’ of photomorphogenesis in early plant development,” in Nature plants (Nature Research), 7, 268–276. doi:10.1038/s41477-021-00866-53
Huijbers, M. M. E., Zhang, W., Tonin, F., and Hollmann, F. (2018). Lichtgetriebene enzymatische decarboxylierung von Fettsäuren. Angew. Chem. 130 (41), 13836–13839. doi:10.1002/ange.201807119
Lakavath, B., Hedison, T. M., Heyes, D. J., Shanmugam, M., Sakuma, M., Hoeven, R., et al. (2020). Radical-based photoinactivation of fatty acid photodecarboxylases. Anal. Biochem. 600, 113749. doi:10.1016/j.ab.2020.113749
Loh, J. Y. Y., Mohan, A., Flood, A. G., Ozin, G. A., and Kherani, N. P. (2021). Waveguide photoreactor enhances solar fuels photon utilization towards maximal optoelectronic – photocatalytic synergy. Nat. Commun. 12 (1), 402. doi:10.1038/s41467-020-20613-2
Peng, Y., Chen, Z., Xu, J., and Wu, Q. (2021). “Recent advances in photobiocatalysis for selective organic synthesis,” in Organic process research and development (American Chemical Society). doi:10.1021/acs.oprd.1c00413
Sambiagio, C., and Noël, T. (2020). “Flow photochemistry: Shine some light on those tubes,” in Trends in chemistry (Cell Press), 2, 92–106. doi:10.1016/j.trechm.2019.09.003
Smith, P. K., Krohn, R. I., Hermanson, G. T., Mallia, A. K., Gartner, F. H., Frovenzano, M. D., et al. (1985). Measurement of protein using bicinchoninic acid’.
Sorigué, D., Légeret, B., Cuiné, S., Blangy, S., Moulin, S., Billon, E., et al. (2018). An algal photoenzyme converts fatty acids to hydrocarbons. Available at: http://science.sciencemag.org/.
Weber, S. (2005). Light-driven enzymatic catalysis of DNA repair: A review of recent biophysical studies on photolyase. Biochimica Biophysica Acta - Bioenergetics 1707, 1–23. doi:10.1016/j.bbabio.2004.02.010
Xie, C., Zhu, H., Chen, S., Wen, Y., Jin, L., Zhang, L., et al. (2020). Chronic retinal injury induced by white LED light with different correlated color temperatures as determined by microarray analyses of genome-wide expression patterns in mice. J. Photochem. Photobiol. B Biol. 210, 111977. doi:10.1016/j.jphotobiol.2020.111977
Keywords: photodecarboxylation, biocatalysis, green chemistry, sunlight, CvFAP
Citation: França AS, Breda GC, De Oliveira KT, Almeida RV, Hollmann F and De Souza ROMA (2023) Impact of sunlight irradiation on CvFAP photodecarboxylation. Front. Catal. 3:1165079. doi: 10.3389/fctls.2023.1165079
Received: 13 February 2023; Accepted: 23 March 2023;
Published: 13 April 2023.
Edited by:
Zheng Guo, Aarhus University, DenmarkReviewed by:
Jingbo Li, Ginkgo Bioworks, United StatesFlorian Rudroff, Vienna University of Technology, Austria
Copyright © 2023 França, Breda, De Oliveira, Almeida, Hollmann and De Souza. This is an open-access article distributed under the terms of the Creative Commons Attribution License (CC BY). The use, distribution or reproduction in other forums is permitted, provided the original author(s) and the copyright owner(s) are credited and that the original publication in this journal is cited, in accordance with accepted academic practice. No use, distribution or reproduction is permitted which does not comply with these terms.
*Correspondence: Rodrigo O. M. A. De Souza, c291emFyb2QyMUBnbWFpbC5jb20=
†These authors have contributed equally to this work