- 1Numaferm GmbH, Düsseldorf, Germany
- 2Institute of Biochemistry, Heinrich Heine University, Düsseldorf, Germany
- 3NanoTag Biotechnologies GmbH, Göttingen, Germany
The application of long-chained peptides (+30 aa) and relatively short proteins (<300 aa) has experienced an increasing interest in recent years. However, a reliable production platform is still missing since manufacturing is challenged by inherent problems such as mis-folding, aggregation, and low production yields. And neither chemical synthesis nor available recombinant approaches are effective and efficient. This in particular holds true for disulfide-rich targets where the correct isomer needs to be formed. With the technology Numaswitch, we have now developed a biochemical tool that circumvents existing limitations and serves as first production platform for pepteins, hard-to-be-produced peptides and proteins between 30 and 300 amino acids in length, including disulfide-rich candidates. Numaswitch is based on bifunctional Switchtag proteins that force the high-titer expression of pure inclusion bodies and simultaneously assist in the efficient refolding of pepteins into functional pepteins. Here, we demonstrate the successful application of the Numaswitch platform for disulfide-containing pepteins, such as an antimicrobial fusion peptide, a single-chain variable fragment (scFv), a camelid heavy chain antibody fragment (VHH) and the human epidermal growth factor.
1 Introduction
Bacteria are simple and cost-effective hosts for the recombinant production of peptides and proteins. Peptides are conventionally defined as molecules consisting of 2–50 amino acids (Friedberg et al., 1947) while polypeptides composing of more than 100 amino acids are classified as proteins (Kim et al., 2015). Here, the term “pepteins” refer to peptides and small proteins made of 30–300 amino acids, a class of polypeptide chains whose importance in many biological functions and cellular processes was not recognized until recently (Su et al., 2013; Storz et al., 2014). Today, Escherichia coli-based expression systems are used successfully in many biopharmaceuticals productions (Walsh, 2018). Among other post translational modifications, disulfide bond formation plays a crucial role for folding into the native state and hereby often influences stability, function, and activity (Bulleid, 2012). Production of disulfide bonds containing peptide and protein-derived bioactive compounds, such as antimicrobial peptides, growth factors, antibody fragments or nanobodies have been difficult using E. coli-based expression systems as the cytoplasm of E. coli has a reducing environment, preventing the formation of disulfide bonds (Bessette et al., 1999; Gaciarz et al., 2017). Several systems and procedures have been developed to optimize the formation of cysteine bridges, including modified E. coli host cells for enhanced oxidative folding in the cytoplasm or the translocation to the oxidative environment of the E. coli periplasm (de Marco, 2009; Salinas et al., 2011; Berkmen, 2012; Lobstein et al., 2012; Manta et al., 2019). In both cases, native disulfide bond formation relies on the correct de novo disulfide bond formation or the subsequential isomerization to the correct isomer. Despite successful examples, many of such targets tend to accumulate as insoluble aggregates and are forming incomplete or wrong disulfide bonds, resulting in low overall production yields (Teilum et al., 1999; Bhatwa et al., 2021). The formation of inclusion bodies (IBs, insoluble protein aggregates) in E. coli is currently considered as obstacle since the refolding of unfolded IBs to functional proteins is regarded as inefficient. Advantages, such as high-titer expression, easy separation from host cell proteins, resistance to proteolytic degradation, the possibility to express toxic targets and others, however, have made inclusion bodies already a versatile strategy for diverse industrial applications (Singhvi et al., 2020; Bhatwa et al., 2021). To this end, we developed the production platform Numaswitch (Nguyen et al., 2021) that grants access to the described advantages of IBs and simultaneously solves the problem of the inefficient refolding step. Numaswitch is based on bifunctional Switchtag proteins which are derived from a family of exoproteins being present in Gram-negative bacteria (Linhartova et al., 2010).
The bifunctionality of Switchtags relies on Ca2+ binding domains, so called GG repeats that are characterized by non-apeptides with the consensus sequence GGXGXDXUX (X: any amino acid, U: large, hydrophobic amino acid), that bind Ca2+ with µM affinities (Lecher et al., 2012). GG repeats are typically part of type 1 secretion substrates (T1SS) i.e., the protein Hemolysin A (Kanonenberg et al., 2018). Inside the cytoplasm of E. coli (low Ca2+ concentration of ∼100 nM), Switchtags and T1SS are rather unfolded proteins and prone to aggregation. After secretion to the surrounding by a dedicated type 1 secretion complex, where the Ca2+ concentration is ∼ mM, the binding of Ca2+ converts T1SS into folded, stable, and functional proteins. Here, the applicability of the Switchtags for the production of four disulfide bond-containing pepteins was studied demonstrating that the Numaswitch approach is a powerful tool for folding of these biomolecules efficiently into the correct and functional conformation.
2 Materials and methods
2.1 Cloning, expression and IB preparation
The DNA sequence encoding for Switchtags pepteins fusion including Dermaseptin-Thanatin (DS-THA), the camelid heavy chain antibody fragment (VHH), the single-chain variable fragment (scFv, anti-tubulin clone F2C) and the human epidermal growth factor (hEGF) (Supplementary Tables S1, S2) were cloned into a pBAD plasmid. Expression was carried out by high cell density fermentation in E. coli BL21 using arabinose induction in an extended fed batch approach. For IB preparation cells were disrupted by pressure homogenization (LM-20, Microfluidics). IBs were washed with buffer (10 mM Tris/HCl pH 7.3, 10 mM NaCl, 2 mM EDTA). Numacut TEV protease (https://numaferm.com/product/) was sourced in appropriate amounts (Numaferm GmbH, Germany).
2.2 Renaturation of IBs and Numacut TEV protease cleavage
Switchtag peptein fusion IBs were solubilized in guanidinium hydrochloride (GuHCl, 6 M) or Urea (8 M) (1:4-8, w/v). Protein concentrations were determined by UV/Vis spectroscopy at 280 nm using the calculated molecular weights and extinction coefficients (ProtParam, Expasy). Renaturations were performed in Tris/HCl or HEPES-based buffer (pH 8, 20 mM, 10 mM CaCl2, 0.5 mM EDTA) adjusting the protein concentration to 1.1–1.7 mg/ml. Numacut TEV protease was added in a molar ratio of 1:25-50 and cleavage reactions were incubated at RT for 3–24 h.
2.3 Purification from cleavage reactions and mass analysis
Purifications of hEGF, VHH and scFv by ion exchange (IEX) chromatography and/or immobilized metal affinity chromatography (IMAC) were carried out using the ÄKTA™ pure chromatography system. The cleavage reactions of VHH and scFv were cleared by centrifugation and filtration (0.45 µm). Negative mode IMAC (Cytiva, HisTrap™ FF) was performed in 50 mM Tris/HCl pH 8.0, 10 mM CaCl2 to separate the Switchtags and Numacut TEV protease (carrying both His6-tags) from VHH and scFv, respectively. Elutions of VHH and scFv in the flow through fractions were monitored by UV absorbance at 280 nm, pooled, concentrated and buffer exchanged to TBS (50 mM Tris-HCl, pH 8, 150 mM NaCl) by ultrafiltration (Millipore, Amicon Ultra-15, 3 kDa cut-off). hEGF cleavage reaction was cleared by filtration (0.45 µm) before loaded on IEX column (Cytiva, HiTrap Capto Q ImpRes) pre-equilibrated in 20 mM Tris/HCl pH 8.0. hEGF was eluted by using a gradient of NaCl (0–500 mM). Elution signals were monitored by UV absorbance at 280 nm. Remaining Switchtag impurities were removed from target by negative mode IMAC (Cytiva, HisTrap™ FF) in 20 mM Tris/HCl pH 8.0. hEGF containing flow through fractions were pooled, concentrated by ultrafiltration (Sartorius, Vivaspin 15R, 2 kDa cut-off) and buffer exchanged to PBS (137 mM NaCl, 2.7 mM KCl, 8 mM Na2HPO4, and 2 mM KH2PO4) by gel filtration (Cytiva, PD-10 Sephadex G-25). The cleavage sample of DS-THA was supplemented with 3 M urea, 5% acetonitrile (ACN) and acidified by addition of 0.1% trifluoro acetic acid (TFA). Separation was carried out by RP FLASH chromatography (BGB, Scorpius Cyano (CN), 100 Å, 30 µm) using the BUCHI Pure C-850 Flash/Prep chromatography system. DS-THA was purified using a linear water/acetonitrile gradient supplemented with 0.1% TFA. DS-THA containing fractions were pooled and lyophilized. RP-HPLC analyses were performed (Alliance QDa detector, Waters, ZORBAX 300SB-C18 column, 4.6 × 250 mm, 5 μm, Agilent) with a water/acetonitrile gradient supplemented with 0.1% TFA. Elutions signals of DS-THA were monitored by UV absorbance at 205 nm, respectively. Mass analyses of hEGF, VHH, scFv and DS-THA were performed by UPLC/MS (BioAccord, ACQUITY RDa, Waters) using BioResolve RP mAb Polyphenyl (450 Å, 2.7 μm, 2.1 × 50 mm) or ACQUITY UPLC CSH C18 column (130 Å, 1.7 µm, 2.1 mm × 100 mm) with a water/acetonitrile gradient supplemented with 0.1% formic acid.
2.3.1 Functional studies
2.3.1.1 Minimal inhibitory concentration (MIC) assay for DS-THA
A 0.5 mM solution of DS-THA was prepared in 0.01% acetic acid and serials of 2-fold dilutions were prepared in a 96-well plate. Cell cultures were diluted to the required number of cells with 1.6 × 106 CFU/ml for E. coli and B. subtilis in Mueller Hinton Broth medium. Lyophilized peptides were dissolved in MOPS (10 mM, 120 mM NaCl2, 10 mM CaCl2) buffer, adjusted to 2 mM after protein determination by UV/VIS spectroscopy at 280 nm, and 10 µl of the serial diluted peptide solutions were added to 90 µl of the respective culture in the 96-well plate giving final peptide concentrations of 0.1–50 µM. As a control, 90 µl of each of the cultures was mixed with 10 µl of 0.01% acetic acid. The 96-well plates were incubated for 24 h at 37°C and the optical density at 595 nm was measured. The MIC corresponds to the lowest measured concentration where the growth was inhibited by ≥ 95% compared to the control without antimicrobial peptide.
2.3.1.2 mCherry binding assays for VHH
Binding of VHH to monomeric fluorescent protein mCherry (provided by Nanotag Biotechnologies GmbH) was tested. VHH was mixed with a 2-fold molar excess of mCherry in TBS-buffer (50 mM Tris/HCl pH 7.4, 150 mM NaCl). After 1 h incubation on ice, mixture was cleared by centrifugation (20,000xg, 5 min, RT) and the supernatant analyzed via Size exclusion chromatography (SEC). SEC analysis was performed monitoring the absorption at 280 nm on an Agilent Bio SEC-5 column (5 μm, 300 Å, 4.6 × 300 mm) in TBS-buffer (0.2 ml/min flow rate).
2.3.1.3 Target engagement assays for hEGF
Target engagement assay (Catalog ref., 86-0006P-2742AG) for hEGF was carried out by Eurofins DiscoverX Corporation (Fremont, United States of America). Evaluations of potency and efficacy (Emax) of hEGF compounds for the human EGFR (ErbB1) receptor in stably transfected U2OS cells were determined in a PathHunter Receptor Tyrosine Kinase (RTK) cell-based assay. Pre-incubated U2OS cells were incubated in presence of different hEGF dilutions and response induction of ErbB1 receptor was measured by chemiluminescent signal detection. The efficacy and compound concentrations were fitted in a dose response curve for determination the half maximal effective concentration (EC50). Measurements were conducted in duplicates.
2.3.1.4 Detection of tubulin in COS-7 cells by Atto 647 conjugated scFv
250 µg recombinantly produced anti-tubulin scFv (F2C) was labeled with an Atto 647 NHS ester (ATTO-TEC, cat. No. AD 647-31) according to the manufacturer´s protocol. The degree of labelling (DOL, dye-to-protein ratio) was determined to be 1.5. Excess dye was removed by desalting the conjugate with a gravity flow column (Cytiva, Cat. No 17085101). For epifluorescence imaging, COS-7 cells were fixed in 4% paraformaldehyde (PFA) (w/v) for 30 min at room temperature (RT). Cells were blocked and permeabilized in PBS containing 10% normal goat serum (v/v) and 0.1% Triton-X 100 (v/v) for 15 min at RT. Fluorescently labeled scFv anti-tubulin Atto647 was diluted 1:500 in PBS containing 3% normal goat serum and 0.1% Triton-X 100 (v/v). The cells were incubated in this staining solution for 1 h at RT and subsequently washed 3 times for 5 min with PBS. To stain the nucleus, DAPI (0.4 μg/l) was included in one of the PBS washing steps. Cover slips were mounted on glass-slides using Mowiol solution, dried at 37 °C and imaged.
3 Results
3.1 Expression and renaturation of Switchtag peptein fusion IBs
Expression of the antimicrobial adhesive fusion peptide DS-THA, the camelid heavy chain antibody fragment (VHH), the anti-tubulin single-chain variable fragment (scFv) and human epidermal growth factor (hEGF) as Switchtag fusion proteins was carried out as E. coli BL21 high cell density fermentations in an extended fed-batch approach. Expression yields of 10–19 g per liter fermentation broth were reached (Table 1). All Switchtag peptein fusion proteins were expressed quantitatively as IBs (Figure 1) demonstrating the functionality of Switchtags as IB-tags. Remarkably, all Switchtag fusion proteins could be refolded in the presence of Ca2+ and the renaturation efficiencies were as high as 90% (Table 1). Subsequently, the Switchtags were cleaved-off quantitatively and traceless from the pepteins by Numacut TEV protease, yielding native pepteins without any non-desired amino acids left (Figure 1).
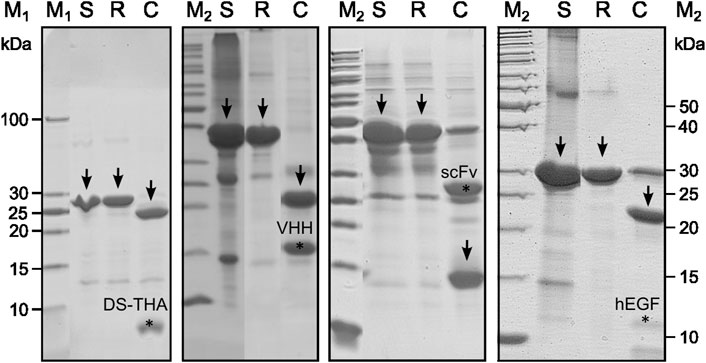
FIGURE 1. SDS-PAGE analysis (Coomassie-stained) of Switchtag peptein fusion proteins from solubilization to the cleavage reaction. Arrows indicate the Switchtag peptein fusion proteins after solubilization from IBs (S) and the refolding step (R) and the Switchtags after protease cleavage (C). Target signals were marked (∗) and found for all pepteins.
3.2 Purification of target pepteins
The pepteins VHH, scFv, and hEGF were purified by applying an ion exchange (IEX) chromatography and/or immobilized metal affinity chromatography (IMAC) (for details see Supplementary Figures S1–S4). DS-THA was purified via reversed phase (RP) FLASH chromatography from the crude cleavage reaction. Production yields of pepteins were calculated to be 1.1–4.5 g/l reaching ≥95% purity, respectively (Table 1).
3.3 Identification of oxidation state
The purified pepteins were analyzed by RP-HPLC/MS to identify their oxidation states (Table 2, Supplementary Figure S5). In case of VHH and DS-THA, the determined masses correspond to the oxidized stage proving the presence of the disulfide bridge. For scFv, a mass of −2 Da compared to the fully reduced protein was measured indicating the presence of an intermediate isomer. Treatment with the oxidizing reagent Cu-phenanthroline yielded the fully oxidized mass (Kobashi, 1968) (Supplementary Figure S6). In case of hEGF containing six cysteine residues, mass spectrometry demonstrated the presence of the fully oxidized peptein.
3.4 Functional characterization
Analysis of the antimicrobial activity of DS-THA was carried out by determining the minimal inhibitory concentration (MIC) for E. coli and B. subtilis. For both microorganisms MIC values of 1.6 µM were determined, being consistent with previous reported values (Chen et al., 2021; Dash and Bhattacharjya, 2021) and demonstrating the production of functional target (Supplementary Figure S7). After co-incubation of VHH, a single chain antibody fragment with binding affinity towards the fluorescent protein mCherry (Wang et al., 2021), the quantitative formation of VHH-mCherry heterodimers was observed by size exclusion chromatography (SEC) analysis (Supplementary Figure S8), proving the functional fold of VHH. Activity of the recombinantly produced anti-tubulin scFv was analyzed by epifluorescence imaging (Nizak et al., 2003). Tubulin was detected in COS-7 cells using the recombinantly produced scFv (Supplementary Figure S9) demonstrating the functionality of scFv. hEGF activity was evaluated in an EGFR human receptor tyrosine kinase (RTK) cell-based assay. hEGF showed a potency (EC50) and efficacy (Emax) which are in line with the reference standard of hEGF (Table 3, Supplementary Figure S10).
4 Discussion
Pepteins are hard-to-be-produced biomolecules, and neither chemical synthesis nor available expression strategies are serving as reliable and efficient manufacturing strategies, in particular for disulfide-containing targets (Miranda and Alewood, 1999; Huang et al., 2012). The application of E. coli is hindered by i.e. the reducing environment of the cytoplasm and, despite important developments in this context, like improved oxidative folding or translocation into the periplasm, aggregation and the formation of wrong isomers are causing low production titers (Singhvi et al., 2020; Bhatwa et al., 2021). In this study, we successfully applied bifunctional Switchtag proteins to evaluate their potential to produce disulfide-containing pepteins.
Switchtag proteins induce the aggregation of pepteins as inclusion bodies with all beneficial features, like high expression titers, high initial purity grades, accessibility of toxic pepteins and the synthesis accuracy of ribosomes (>99.99%). After being extracted and solubilized, Switchtags assist in the refolding step taking place in a biochemical reaction containing Ca2+ at mM concentration. Here, the binding of Ca2+ to the GG repeats induces the quantitative refolding of Switchtags and water-soluble, stable Switchtag peptein fusions are formed. Switchtags act as solubility tags acting chaperon-like and preventing intermolecular interactions of fused pepteins. Aggregation and/or precipitation of fused pepteins are prevented and pepteins are kept soluble. This is important, since it allows the time-dependent folding of pepteins along the thermodynamical energy landscape reaching finally the native state. Switchtags enable the efficient refolding of unfolded pepteins being produced as inclusion bodies and assist in the folding step as bifunctional tag. In particular, for disulfide-containing targets, keeping them soluble and counteracting intermolecular interactions allows auto-oxidation and/or the application of redox shuffle systems allowing the formation of the correct isomer (lowest thermodynamic energy level).
Since proteogenic impurities being present in the IB samples are not efficiently refolded, the refolding step represents a purification step yielding highly pure Switchtag peptein fusions (see Figure 1, Lanes S compared to R). In addition, prior to refolding when the pepteins are still unfolded, the IBs can be washed by i.e., detergents or organic solvents removing process related impurities like endotoxins quantitatively (data not shown).
The presence of disulfide bonds in antimicrobial peptides, like DS-THA is often involved in modulating the activity (Ramamoorthy et al., 2006; Castro et al., 2011; Dash and Bhattacharjya, 2021). Furthermore, expression of antimicrobial peptides, like DS-THA, in recombinant systems is often difficult, due to the toxicity towards the expression hosts. The Switchtag approached, grants access to these kinds of disulfide bond containing compounds, where cytoplasmic expression usually is no option. Nanobodies, such as VHH, can be produced in E. coli, for example in combination with a SUMO tag (Wang et al., 2021). The refolding of functional VHH demonstrates that the Numaswitch approach is applicable in case of proteins with distinct, maybe even, complex conformations. In case of hEGF, where incomplete formation of disulfide bonds or the formation of wrong isoforms is a challenge, (Chang et al., 1995; Chang et al., 2001), Switchtags enable the production of fully-oxidized, functional molecules. Importantly, the application of Switchtags is not limited to dedicated E. coli strains or expression hosts as it can be regarded as biochemical toolbox.
If Switchtag peptein fusion proteins are expressed as IBs, the Numaswitch approach is applicable and the IBs can be efficiently and functionally refolded in the presence of Ca2+. Cytoplasmic expression of scFv or hEGF usually reaches production yields of up to 30 mg or 250 mg per liter fermentation broth, respectively, and rely on the use of solubility tags, redox shuffle system or systems like CyDisCo for production and disulfide formation (Ma et al., 2016; Shams et al., 2019; Kim et al., 2021). The application of shuffle strains with an oxidative cytoplasmic environment is especially hindered by slow growth rates and low titers in combination with limited upscalabilitly (Lobstein et al., 2012). The obtained production yields, to the best of our current knowledge, are the highest achieved so far.
Current efforts focus on the production of larger proteins (>300 aa) with complex tertiary structures (i.e. enzymes, antibodies) in industrial-relevant scales. However, first data indicate that refolding efficiencies, hence the production yields of target proteins decrease with larger protein sizes attached to the Switchtag clearly drawing out limitations of the Numaswitch technology (unpublished data). To addess this challenge we develop Switchtag proteins which can be used for these kind of proteins and act Ca2+-independent or in the presence of alternative ions (unpublished data). For this, alternative proteins of the huge RTX familiy are analyzed which comprises more than 1,000 members (Linhartova et al., 2010). Such Switchtags would not only grant access to a broader class of proteins, but also be applicable for candidates that are instable or non-functional in the presence of Ca2+ ions.
In this study the Numaswitch technology was successfully used to produce peptein candidates ranging from 50 to 265 amino acids clearly demonstrating its potential of being an efficient and universal platform for peptide (2−50 aa) (Nguyen et al., 2021) and peptein (30−300 aa) production. For the first time, the advantages of IBs get accessible by solving the major bottleneck; Switchtags ensure the efficient and reliable refolding of IBs into functional pepteins. Since the expression of IBs and the biochemical refolding step is reliable and efficient, production processes can be established within weeks making Numaswitch a versatile and scalable production platform compared to chemical synthesis and alternative bioprocesses.
Data availability statement
The original contributions presented in the study are included in the article/Supplementary Material, further inquiries can be directed to the corresponding author.
Author contributions
B-NN, CS, FN designed the study. B-NN, FN, HG performed research. The first draft of the manuscript was written by B-NN and FT. CS, HG, LS and FT revised the manuscript. All authors commented on previous versions of the manuscript. All authors read and approved the final manuscript.
Conflict of interest
CS is chief executive officer of Numaferm GmbH. HG is chief executive officer of NanoTag Biotechnologies GmbH. B-NN, FN, FT are employees of Numaferm GmbH.
The remaining author declares that the research was conducted in the absence of any commercial or financial relationships that could be construed as a potential conflict of interest.
Publisher’s note
All claims expressed in this article are solely those of the authors and do not necessarily represent those of their affiliated organizations, or those of the publisher, the editors and the reviewers. Any product that may be evaluated in this article, or claim that may be made by its manufacturer, is not guaranteed or endorsed by the publisher.
Supplementary material
The Supplementary Material for this article can be found online at: https://www.frontiersin.org/articles/10.3389/fddsv.2023.1082058/full#supplementary-material.
References
Berkmen, M. (2012). Production of disulfide-bonded proteins in Escherichia coli. Protein Expr. Purif. 82 (1), 240–251. doi:10.1016/j.pep.2011.10.009
Bessette, P. H., Aslund, F., Beckwith, J., and Georgiou, G. (1999). Efficient folding of proteins with multiple disulfide bonds in the Escherichia coli cytoplasm. Proc. Natl. Acad. Sci. U. S. A. 96 (24), 13703–13708. doi:10.1073/pnas.96.24.13703
Bhatwa, A., Wang, W., Hassan, Y. I., Abraham, N., Li, X. Z., and Zhou, T. (2021). Challenges associated with the formation of recombinant protein inclusion bodies in Escherichia coli and strategies to address them for industrial applications. Front. Bioeng. Biotechnol. 9, 630551. doi:10.3389/fbioe.2021.630551
Bulleid, N. J. (2012). Disulfide bond formation in the mammalian endoplasmic reticulum. Cold Spring Harb. Perspect. Biol. 4 (11), a013219. doi:10.1101/cshperspect.a013219
Castro, J. R. M., Fuzo, C. A., and Degrève, L. (2011). Effect of disulfide bridges deletion on the conformation of the androctonin, polyphemusin-I, and thanatin antimicrobial peptides: Molecular dynamics simulation studies. J. Biophysical Chem. 02 (03), 244–257. doi:10.4236/jbpc.2011.23030
Chang, J. Y., Li, L., and Lai, P. H. (2001). A major kinetic trap for the oxidative folding of human epidermal growth factor. J. Biol. Chem. 276 (7), 4845–4852. doi:10.1074/jbc.M005160200
Chang, J. Y., Schindler, P., Ramseier, U., and Lai, P. H. (1995). The disulfide folding pathway of human epidermal growth factor. J. Biol. Chem. 270 (16), 9207–9216. doi:10.1074/jbc.270.16.9207
Chen, J., Hao, D., Mei, K., Li, X., Li, T., Ma, C., et al. (2021). In vitro and in vivo studies on the antibacterial activity and safety of a new antimicrobial peptide dermaseptin-AC. Microbiol. Spectr. 9 (3), e0131821. doi:10.1128/Spectrum.01318-21
Dash, R., and Bhattacharjya, S. (2021). Thanatin: An emerging host defense antimicrobial peptide with multiple modes of action. Int. J. Mol. Sci. 22 (4), 1522. doi:10.3390/ijms22041522
de Marco, A. (2009). Strategies for successful recombinant expression of disulfide bond-dependent proteins in Escherichia coli. Microb. Cell Fact. 8, 26. doi:10.1186/1475-2859-8-26
Friedberg, F., Winnick, T., and Greenberg, D. M. (1947). Peptide synthesis in vivo. J. Biol. Chem. 169 (3), 763–764. doi:10.1016/s0021-9258(17)30896-7
Gaciarz, A., Khatri, N. K., Velez-Suberbie, M. L., Saaranen, M. J., Uchida, Y., Keshavarz-Moore, E., et al. (2017). Efficient soluble expression of disulfide bonded proteins in the cytoplasm of Escherichia coli in fed-batch fermentations on chemically defined minimal media. Microb. Cell Fact. 16 (1), 108. doi:10.1186/s12934-017-0721-x
Huang, C. J., Lin, H., and Yang, X. (2012). Industrial production of recombinant therapeutics in Escherichia coli and its recent advancements. J. Ind. Microbiol. Biotechnol. 39 (3), 383–399. doi:10.1007/s10295-011-1082-9
Kanonenberg, K., Spitz, O., Erenburg, I. N., Beer, T., and Schmitt, L. (2018). Type I secretion system-it takes three and a substrate. FEMS Microbiol. Lett. 365 (11). doi:10.1093/femsle/fny094
Kim, E., Barrett, S. M. B., Scott, B., and Brooks, H. (2015). Ganong's review of medical physiology. 25th Edition. New York: McGraw Hill Professional.
Kim, Y. S., Lee, H. J., Han, M. H., Yoon, N. K., Kim, Y. C., and Ahn, J. (2021). Effective production of human growth factors in Escherichia coli by fusing with small protein 6HFh8. Microb. Cell Fact. 20 (1), 9. doi:10.1186/s12934-020-01502-1
Kobashi, K. (1968). Catalytic oxidation of sulfhydryl groups by o-phenanthroline copper complex. Biochimica Biophysica Acta (BBA) - General Subj. 158 (2), 239–245. doi:10.1016/0304-4165(68)90136-0
Lecher, J., Schwarz, C. K., Stoldt, M., Smits, S. H., Willbold, D., and Schmitt, L. (2012). An RTX transporter tethers its unfolded substrate during secretion via a unique N-terminal domain. Structure 20 (10), 1778–1787. doi:10.1016/j.str.2012.08.005
Linhartova, I., Bumba, L., Masin, J., Basler, M., Osicka, R., Kamanova, J., et al. (2010). RTX proteins: A highly diverse family secreted by a common mechanism. FEMS Microbiol. Rev. 34 (6), 1076–1112. doi:10.1111/j.1574-6976.2010.00231.x
Lobstein, J., Emrich, C. A., Jeans, C., Faulkner, M., Riggs, P., and Berkmen, M. (2012). SHuffle, a novel Escherichia coli protein expression strain capable of correctly folding disulfide bonded proteins in its cytoplasm. Microb. Cell Fact. 11, 56. doi:10.1186/1475-2859-11-56
Ma, Y., Yu, J., Lin, J., Wu, S., Li, S., and Wang, J. (2016). High efficient expression, purification, and functional characterization of native human epidermal growth factor in Escherichia coli. Biomed. Res. Int. 2016, 3758941. doi:10.1155/2016/3758941
Manta, B., Boyd, D., and Berkmen, M. (2019). Disulfide bond formation in the periplasm of Escherichia coli. EcoSal Plus 8 (2). doi:10.1128/ecosalplus.ESP-0012-2018
Miranda, L. P., and Alewood, P. F. (1999). Accelerated chemical synthesis of peptides and small proteins. Proc. Natl. Acad. Sci. 96 (4), 1181–1186. doi:10.1073/pnas.96.4.1181
Nguyen, B. N., Tieves, F., Rohr, T., Wobst, H., Schöpf, F. S., Solano, J. D. M., et al. (2021). Numaswitch: An efficient high-titer expression platform to produce peptides and small proteins. Amb. Express 11 (1), 48. doi:10.1186/s13568-021-01204-w
Nizak, C., Martin-Lluesma, S., Moutel, S., Roux, A., Kreis, T. E., Goud, B., et al. (2003). Recombinant antibodies against subcellular fractions used to track endogenous Golgi protein dynamics in vivo. Traffic 4 (11), 739–753. doi:10.1034/j.1600-0854.2003.00132.x
Ramamoorthy, A., Thennarasu, S., Tan, A., Gottipati, K., Sreekumar, S., Heyl, D. L., et al. (2006). Deletion of all cysteines in tachyplesin I abolishes hemolytic activity and retains antimicrobial activity and lipopolysaccharide selective binding. Biochemistry 45 (20), 6529–6540. doi:10.1021/bi052629q
Salinas, G., Pellizza, L., Margenat, M., Flo, M., and Fernandez, C. (2011). Tuned Escherichia coli as a host for the expression of disulfide-rich proteins. Biotechnol. J. 6 (6), 686–699. doi:10.1002/biot.201000335
Shams, D., Alizadeh, M., Azari, S., Hosseini, S., Yasami-Khiabani, S., Samani, S., et al. (2019). High expression level of human epidermal growth factor (hEGF) using a well-designed fusion protein-tagged construct in E-coli. Bratisl. Lek. Listy 120 (10), 757–763. doi:10.4149/BLL_2019_126
Singhvi, P., Saneja, A., Srichandan, S., and Panda, A. K. (2020). Bacterial inclusion bodies: A treasure trove of bioactive proteins. Trends Biotechnol. 38 (5), 474–486. doi:10.1016/j.tibtech.2019.12.011
Storz, G., Wolf, Y. I., and Ramamurthi, K. S. (2014). Small proteins can no longer be ignored. Annu. Rev. Biochem. 83, 753–777. doi:10.1146/annurev-biochem-070611-102400
Su, M., Ling, Y., Yu, J., Wu, J., and Xiao, J. (2013). Small proteins: Untapped area of potential biological importance. Front. Genet. 4, 286. doi:10.3389/fgene.2013.00286
Teilum, K., Ostergaard, L., and Welinder, K. G. (1999). Disulfide bond formation and folding of plant peroxidases expressed as inclusion body protein in Escherichia coli thioredoxin reductase negative strains. Protein Expr. Purif. 15 (1), 77–82. doi:10.1006/prep.1998.0985
Walsh, G. (2018). Biopharmaceutical benchmarks 2018. Nat. Biotechnol. 36 (12), 1136–1145. doi:10.1038/nbt.4305
Keywords: inclusion bodies, disulfide bonds, Numaswitch, Switchtag, hEGF, pepteins, recombinant expression
Citation: Nguyen B-N, Tieves F, Neusius FG, Götzke H, Schmitt L and Schwarz C (2023) Numaswitch, a biochemical platform for the efficient production of disulfide-rich pepteins. Front. Drug Discov. 3:1082058. doi: 10.3389/fddsv.2023.1082058
Received: 27 October 2022; Accepted: 02 February 2023;
Published: 21 February 2023.
Edited by:
John Mayer, University of Colorado Boulder, United StatesReviewed by:
Sayed K. Goda, University of Derby, United KingdomJosé Rafael De Almeida, Regional College Amazon Ikiam, Ecuador
Copyright © 2023 Nguyen, Tieves, Neusius, Götzke, Schmitt and Schwarz. This is an open-access article distributed under the terms of the Creative Commons Attribution License (CC BY). The use, distribution or reproduction in other forums is permitted, provided the original author(s) and the copyright owner(s) are credited and that the original publication in this journal is cited, in accordance with accepted academic practice. No use, distribution or reproduction is permitted which does not comply with these terms.
*Correspondence: Christian Schwarz, Y2hyaXN0aWFuLnNjaHdhcnpAbnVtYWZlcm0uY29t