- 1EcotoQ, Institut National de la Recherche Scientifique, Centre Eau Terre Environnement, Québec, QC, Canada
- 2Univ. Bordeaux, CNRS, Bordeaux INP, EPOC, UMR 5805, F-33600 Pessac, France
Natural dissolved organic matter (DOM) is a heterogeneous mixture of a variety of organic compounds, with a great importance for the environmental fate of metals and their ecotoxicity. However, its complex nature and variable composition make the understanding of its role a challenge. Lanthanum (La) has a strong affinity for DOM and is one of the rare earth elements that is widely used in many electronic and green technologies, and for which the demand may potentially increase for the foreseeable future. The present study examines the links between the optical and chemical properties of organic matter and its influence on the bioavailability and toxicity of La toward the green microalga Chlorella fusca. A total of four DOMs, two natural organic matter samples from Ontario (Luther Marsh and Bannister Lake) and two Suwannee River fulvic and humic acids, were characterized by absorbance and fluorescence spectroscopy as well as by asymmetrical flow field-flow fractionation. These suggest that Luther Marsh and the Suwannee River humic acid have higher molecular weight and are more aromatic, compared to the other two DOMs. The concentrations of free metal ion La3+ were measured by an ion exchange technique and a partial ultrafiltration method, and related to biological response. Toxicity tests over 96 h at pH = 5.0 were separately conducted with and without 3 mg C·L−1 of each DOM. All DOM samples reduced La bioavailability but as opposed to what can be expected based on the Biotic Ligand Model, the presence of DOM exacerbated the toxicity of La and its accumulation in algal cells when only the free La3+ ion concentration was considered. These results indicate that the role of natural organic matter in modulating metal bioavailability remains to be deciphered.
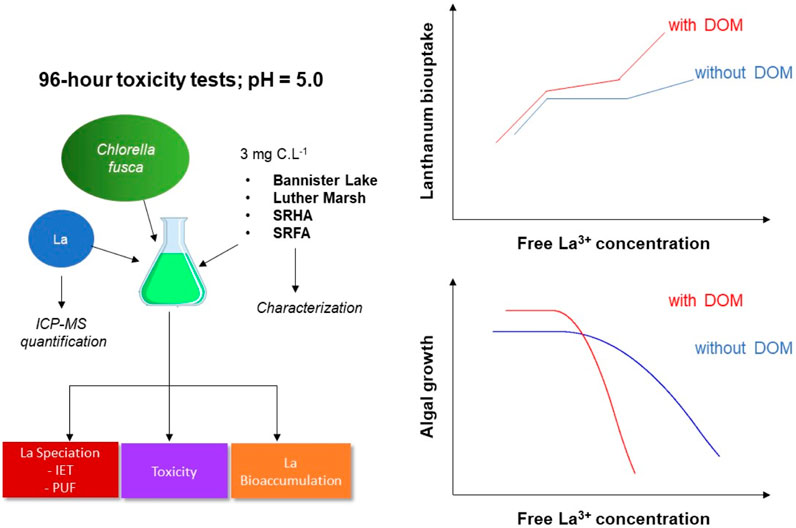
GRAPHICAL ABSTRACT | Experimental design for the exposure of a green alga to lanthanum in the presence or absence of natural organic matter. Results show that accumulation and effects are greater than those expected based on the free ion concentration.
1 Introduction
Dissolved organic matter (DOM) is ubiquitous in aquatic systems, with concentrations generally varying from 1 to 15 mg C·L−1 (Sobek et al., 2007). It is a complex and heterogeneous mixture of compounds that naturally comes from living beings (by secretion/excretion) or decomposition within the watershed, as well as from anthropogenic sources. DOM plays a crucial role in several physical, chemical and biological processes in freshwater aquatic systems, being the main pool of carbon. The most studied operational fractions of DOM are a combination of humic (HA) and fulvic (FA) acids, also called humic substances. In general, DOM comprises a large number of molecules with various structures and properties such as those found in carbohydrates, proteins or amino acids. The chemical structure of DOM provides metal-binding properties with moieties such as carboxyl, phenol and thiol functional groups (Perdue and Ritchie, 2003).
The presence of DOM can therefore alter metal speciation and bioavailability toward aquatic organisms. According to the currently accepted paradigm, the biotic ligand model (BLM), the free metal ion is the key species to be considered when studying the bioavailability and toxicity of metals in solution. When metals are bound by DOM, their biouptake and toxicity generally decrease, and so do the biological responses (Campbell and Fortin, 2013; Mebane et al., 2020; Fortin, 2024). This model has been tested in systems containing DOM and there is a growing body of evidence that for most metals bioavailability can be predicted by the free metal ion concentration (Vigneault and Campbell, 2005; Crémazy et al., 2022; Lachaux et al., 2022). DOM can protect against metal toxicity because of its complexation properties, but it can also directly affect microalgal membranes. Indeed, DOM can adsorb to algal surfaces as the result of a hydrogen bonding mechanism involving electronegative functional groups present in the DOM and on the cell surface, and as a result of hydrophobic interactions (Ojwang and Cook, 2013). This latter trend has been confirmed by DOM loss from the solution, when phytoplankton cells were present at pHs ranging from 4 to 7 (Campbell et al., 1997), and by electrophoretic mobility measurements at pH 5 (Knauer and Buffle, 2001). This surface adsorption of DOM can possibly increase metal uptake by algae. Lamelas et al. (2009) have shown that Pb uptake flux and binding to internalization and adsorption sites of Chlorella kesslerii were higher in the presence of Suwannee River humic acid (SRHA; 10 mg C·L−1). The authors suggested this resulted from an increase in Pb binding to the internalization sites, forming ternary complexes (Pb-HA-alga). Shi et al. (2022) separated SRHA into high (>1 kDa) and low (<1 kDa) molecular weight fractions and exposed Chlorella vulgaris to increasing concentrations of Pb. They found an increased inhibitory effect with the low molecular weight (LMW) fraction while the high molecular weight (HMW) fraction provided a protection against Pb toxicity. They found no significant Pb binding by the LMW fraction while strong Pb complexation was observed for the HMW fraction. The authors hypothesized that LMW DOM may have modified the membrane permeability. Several studies have shown a modification of algal membrane permeability by DOM (Parent et al., 1996; Vigneault et al., 2000; Steinberg et al., 2008) and an influence of DOM hydrophobicity on its affinity for cell membranes (Al-Reasi et al., 2012). Several other studies have investigated the influence of DOM quality and physicochemical properties on metal toxicity (Al-Reasi et al., 2011; Al-Reasi et al., 2012; Al-Reasi et al., 2013), but questions remain unanswered because of the extreme heterogeneity and complexity of DOM.
Lanthanides have a strong affinity for DOM and in circumneutral and alkaline aquatic systems is expected to be mostly complexed with natural organic ligands (Lead et al., 1998; Pourret et al., 2007; Leguay et al., 2016). Naturally present in the environment, lanthanum (La) is a metal of interest because it is one of the rare earth elements widely used in electronic and modern technologies, and needed for renewable energy resources, for which the demand is predicted to increase for the next several years (Zhou et al., 2017). Its extraction and increasing use therefore highlight the need to study its toxicity, in light of its speciation in the presence of natural DOM. It was in this context that we investigated the toxicity of La toward the unicellular freshwater alga Chlorella fusca, in the presence of DOM from different sources. At the base of the trophic chain, microalgae are of great interest for ecotoxicological studies, given their ecological importance and their high surface/volume ratios. Algae such as C. fusca are widely used for chronic toxicity tests due to their ease of harvest and their short generation time.
The objective of this work was to determine the influence of different DOMs and their composition on the speciation of La, on the uptake of La by C. fusca and its subsequent toxicity. We hypothesized that La binding by DOM would result in a decrease in metal bioavailability as predicted by the BLM. The binding of La by DOM was characterized by partial ultrafiltration (PUF), an ion-exchange technique (IET) and asymmetrical flow field-flow fractionation (AF4). In parallel, the uptake and toxicity of La to C. fusca were determined through 96 h exposures at pH 5.0 with 3 mg C·L−1 of DOM, by measuring intracellular metal content and growth inhibition, respectively. Finally, the optical properties of each DOM were characterized by UV-visible and fluorescence spectroscopy, while their size (with and without La) were investigated using AF4.
2 Experimental methods
2.1 Material and reagents
Before their use, all materials related to algal culture were rinsed three times with deionized water, then soaked during 24 h in 10 % (v/v) nitric acid, rinsed again three times with deionized water and three times with ultrapure water (18 MΩ·cm), and finally dried under a laminar flow hood, and autoclaved at 121°C for 15 min.
Lanthanum was added from a standard solution (PlasmaCAL) of 1002 ± 6 mg La·L−1 obtained from the dissolution of La2O3 in 4 % HNO3. For the preparation of the exposure media, intermediate acidic solutions were prepared for different La concentrations, the dilution being carried out with ultrapure water. The Suwannee River humic (SRHA) and fulvic (SRFA) acids were obtained from the International Humic Substances Society (IHSS; HA catalog number: 3S101H and FA catalog number: 1S101F). Solutions of 250 mg·L−1 were prepared by dissolving HA and FA powders in 10 mM NaOH. The solutions were then stirred for 24 h in the dark, filtered through a 0.45 μm polyethersulfone (PES) filter, and stored at 4°C, protected from light. Natural DOM samples were collected from a lake (Bannister Lake) and a marsh (Luther Marsh) in Ontario, Canada as described in Chen et al. (2018) and Al-Reasi et al. (2012). Removal of metal cations from the natural DOM samples was done using a cation exchange resin at acidic pH. Solutions were then filtered through a 0.45 μm PES filter and stored in a dark and cool place (4°C). An analysis of the dissolved organic carbon (DOC; Shimadzu Vcph) present in all stock DOM solutions was carried out in order to verify their concentration. A calibration curve was made for each analysis (with a homemade stock solution of 1000 mg·L−1 of total organic carbon (TOC)) and a standard solution of 100 mg C·L−1 of TOC (batch number: S170309025; SCP Science) was used as a control. Targeted DOC concentrations were fixed at 3 mg C·L−1 for all DOM used. This concentration is well within the typical values observed in natural systems and it results in significant complexation of La (Leguay et al., 2016).
2.2 Dissolved organic matter characterization
2.2.1 Absorbance spectroscopy
The fraction of DOM that absorbs in both ultraviolet and visible light is called colored or chromophoric dissolved organic matter (CDOM). UV-visible absorption spectroscopy was used to determine general properties of DOM, using the Aqualog (Horiba Jobin-Yvon) spectrophotometer. Several parameters can be calculated from absorbance spectra (recorded between 200 and 800 nm), in order to characterize the aromatic character of DOM and to approximate its size. First, the Specific UV Absorbance (SUVA) parameter, expressed in L·mg C−1·m−1, is the UV absorbance at 254 nm, measured in units of absorbance per meter of path length, normalized for DOC concentration (Weishaar et al., 2003). It is correlated with the degree of aromaticity of DOM. Also, the ratio E2/E3 represents the absorbance at 254 nm divided by the absorbance at 365 nm and is often used to characterize aromaticity and molecular size: the ratio increases with decreasing aromaticity and size (Peuravuori and Pihlaja, 1997; Thomsen et al., 2002). Finally, the ratio of spectral slopes (SR) is calculated as the ratio of the slope of the absorbance spectrum for short wavelengths (S275–295 nm) to the spectral slope of longer wavelengths (S350–400 nm). The SR ratio is negatively correlated with the size of the DOM: when SR increases, DOM molecular weight decreases (Helms et al., 2008).
2.2.2 Excitation-emission matrix fluorescence spectroscopy
A part of chromophoric DOM components possess fluorescence properties which provide further information about its composition and source and alteration processes in aquatic environments. Fluorescence is a very sensitive technique that allows a small volume of DOM sample to be quickly characterized even at low sample concentrations. Excitation-emission matrix (EEM) fluorescence spectra were recorded using an Aqualog spectrofluorometer (Horiba, Jobin-Yvon), equipped with a double excitation monochromator, a 150-Watt Xenon lamp, with a 5 nm bandpass, and 2 s integration time at wavelengths of 240–800 nm at 5 nm intervals. The samples were placed in a 1 cm path length quartz cuvette thermostatically controlled at 20°C. Emission spectra were collected with high charge-coupled device detector gain at approximately 0.58 nm (1 pixel) intervals at wavelengths between 245 and 830 nm. The sample EEM spectra were obtained by subtracting the EEM blank spectrum of ultrapure water (Milli-Q, Millipore) daily recorded under the same conditions as those for the DOM samples. The intensity and position of the fluorescence maxima were considered to characterize the observed peaks. Fluorescence intensities were normalized to the area under the Raman peak of an ultrapure water blank at an excitation wavelength of 350 nm and are expressed in Raman units. EEM data treatment was performed with TreatEEM software. The trilinear decomposition algorithm PARAFAC (Parallel Factor Analysis) was used with MATLAB software (R14-6.5) and the DOMFluor toolbox (ver. 1.7). It is a multidimensional procedure which decomposes a whole EEM spectra dataset into different independent groups of fluorescent components. This data analysis consists of building a linear model by estimating the excitation and emission spectra of fluorophores and the coefficient applied to each of these matrices. The multi-way PARAFAC model was run for three to six components with non-negativity constraints as described by Stedmon and Bro (2008).
Fluorescence indices, such as the biological activity index (BIX) and the fluorescence index (FI), can provide information on the origin and source of DOM. The BIX index is calculated from the emission spectrum at 310 nm excitation wavelength by dividing the fluorescence intensity emitted at 380 nm by that emitted at 430 nm (Huguet et al., 2009). Increasing BIX is linked to a predominant autochthonous origin of DOM and the presence of freshly produced organic matter in the environment. The FI corresponds to the ratio of fluorescence emission intensities at 450 and 500 nm for an excitation wavelength of 370 nm. Values > 1.9 typically reflect an aquatic or microbial contribution and < 1.3 a terrestrial contribution (McKnight et al., 2001).
2.2.3 Asymmetrical flow field-flow fractionation (AF4)
Asymmetric Flow Field-Flow Fractionation (AF4) is a non-destructive chromatographic-like separation method suitable for a wide range of molecules and particle sizes (between 1 and 1,000 nm). It allows molecules to be separated according to their diffusion coefficient, the separating force being a flow applied perpendicularly to the direction of the mobile phase flow. As a result, and due to the parabolic form of the mobile phase flow, the smaller size molecules are eluted earlier than the largest ones.
Each DOM size fraction was characterized in the absence and presence of La (5 µM) using AF4 (Eclipse 3 from Wyatt Technology Europe) coupled with a UV/Vis detector (HP1200 series, Agilent). The AF4 was equipped with a separation channel with a spacer of 490 µm and a 1 kDa PES membrane (NADIR NP010). A mobile phase containing 5 mM NaNO3 at pH 6.8 was used. The detector flow rate was set at 1 mL·min−1. The volume of injection was 1 mL with an inject flow rate of 0.3 mL·min−1. The separation conditions were optimized as follows: 5 min of sample injection and 6 min of relaxation with a focus flow rate of 1 mL·min−1, followed by elution with a constant cross-flow rate of 4 mL·min−1 for 5 min, then a linear gradient cross-flow from 4 mL·min−1 to 0 mL·min−1 for 10 min, and finally no cross-flow for 5 min. Between each sample injection, an ultrapure water blank was run and the sample injection system was purged.
2.3 Algae culture conditions
The unicellular green alga Chlorella fusca var. vacuolata Shihira and Krauss was obtained from the Canadian Phycological Culture Centre (University of Waterloo, Canada; CPCC #89). This species has been frequently used for metal uptake/toxicity studies and is known to grow normally at acidic pHs (Parent and Campbell, 1994). Algae cells were cultured in polycarbonate Erlenmeyer flasks in sterile modified high-salt medium (MHSM-2, composition detailed in Supplementary Table S1) at pH 5.0 (buffered with 10 mM MES; 2-ethanesulfonic acid). This pH was selected to avoid the formation of colloidal forms that have been observed at circumneutral pHs for cerium (El-Akl et al., 2015). Organic phosphorus (β-glycerol phosphate) was used at a concentration of 10 µM instead of inorganic phosphate to avoid the problem of La precipitation (Joonas et al., 2017; Aharchaou et al., 2020). All stock solutions (nutrients and trace metals) for the preparation of MHSM-2 growth media were prepared from ultrapure water and high-purity chemicals. These solutions were all filtered through a 0.2 μm Millipore polycarbonate membrane, except for the 0.10 M NaOH solution which was filtered through a 0.2 μm PES membrane. This latter membrane was pre-immersed in ultrapure water 24 h before use. Stock solutions were sterilized by autoclaving at 121°C for 15 min and stored at 4°C protected from light. MHSM-2 medium was also autoclaved under the same conditions before use. The cultures were placed on a stirring plate (∼60 rpm) in an environmental growth chamber (Conviron CMP4030) at 20.0°C ± 0.1°C and under a continuous light intensity of 100 ± 10 μE·m−2·s−1. Algae cultures were maintained by transferring (under sterile conditions) 2 mL of the culture into 100 mL of fresh sterile MHSM-2 growth medium, every 2 weeks. Absence of bacterial contamination was checked monthly by plating onto agar gel.
2.4 Exposure conditions
Metal exposures were performed in sterile MHSM-2 medium. Uptake and toxicity of La were determined in the absence and presence of DOM. Each exposure medium was prepared and left to equilibrate for 72 h before algal inoculation. Algae were kept in the exponential growth phase during the 4 days preceding inoculation. For this latter step, the algae were filtered and collected on a 2.0 μm polycarbonate filter. They were then rinsed three times with 10 mL of EDTA-free rinse medium (without trace elements and MES buffer, MHSM-R composition detailed in Supplementary Table S1), then resuspended in fresh MHSM-2 medium. Cell density was checked in the resuspended algae medium using a particle counter (Coulter Counter Multisizer III) and the algae were inoculated in order to obtain an initial cell density between 15,000 and 20,000 cells·mL−1. The inoculated Erlenmeyer flasks were then placed in the growth chamber, under the same conditions as mentioned above for the algal culture.
Cells were exposed to La (0–5 µM) and DOM (0 or 3 mg C·L−1) during 96 h at pH 5.0. An aliquot of each Erlenmeyer was taken every 24 h in order to measure the cell density and average surface area of the cells with the particle counter. To do so, a 1-mL sample was taken and placed in a cuvette, for a 10 mL final volume using Isoton II solution as diluent. A blank containing only Isoton II solution was analyzed at the start and the end of each use. The pH was measured daily in one Erlenmeyer flask per concentration and remained constant throughout the experiment (4.98 ± 0.02). For the determination of total lanthanum in solution, aliquots were taken every 24 h and centrifuged at 5,000 rpm for 10 min, then the supernatant was recovered and acidified with HNO3 for analysis by inductively coupled plasma mass spectrometry (ICP-MS; XSeries 2, Thermo Scientific). A control exposure experiment without algae was carried out under the same exposure conditions as in the presence of algae (MHSM-2; pH = 5.0, n = 3) for 96 h, and La concentrations did not significantly vary (p > 0.05) during the exposure (less than 7% variation), suggesting that metal adsorption on the container walls was negligible. For exposures in the presence of DOM, TOC was indirectly determined by measuring fluorescence using a spectrofluorometer, at the same frequency as the total La concentration and pH measurements (i.e., every 24 h during the 96 h toxicity tests). The excitation wavelength was set at 350 nm for an emission wavelength of 463 nm. A range of standards with measured concentrations of TOC (0–6 mg C·L−1) were used to obtain a calibration curve. TOC concentrations determined in this manner tended to increase with time (p > 0.05), up to 11 % (mean value for all experiments and all La concentrations). The excretion of organic compounds by algae cells was likely responsible for the observed increase. On the other hand, the measured La concentrations decreased 12 % with time (mean value for all experiments and La concentrations), due to adsorption and assimilation by the algae. This change was however not significant (p > 0.05), so that La remained stable over time for all experiments. Other analytical measurements (PUF and IET; see next section) were carried out at the beginning (0 h) and the end (96 h) of the exposure.
After 96 h, all Erlenmeyer flasks were filtered through two superposed 2.0 μm polycarbonate filters (Millipore). The upper filter was used to quantify lanthanum accumulated by algae while the lower filter was used to control for potential La adsorption onto the filter membrane. The filtrate was recovered to determine the total concentration of La, La speciation and TOC concentration in solution. The algae on the filters were rinsed twice with 10 mL of a MHSM-R rinse solution containing 1 mM EDTA, to remove La bound to the surface of algal cells (Hassler et al., 2004). La was quantified in the upper (intracellular La) and lower (background adsorption control) filters after being dried at 70°C for 48 h, and digested in Teflon tubes with 5 mL of concentrated HNO3 (70 %) and 1 mL of H2O2 at 95°C for 4 h. The final volume was completed to 50 mL with ultrapure water. Digestion quality controls were carried out, at the same time as the algae digestions, with a certified reference material from lichen (IAEA-336; certified value of 0.66 mg La·kg−1 [0.56–0.76; 95 % confidence interval]). Average La recovery of this digested reference material was 94% ± 3 % (n = 5). There were no significant differences between the obtained values and the reference La value of the standard. Metal accumulation per cell was finally calculated by normalizing the results by the mean cell population at 96 h.
2.5 Lanthanum speciation
The speciation of La in the exposure media without organic matter was calculated using MINEQL+ software (Version 5.0). In the media with DOM, the Windermere Humic Acid Model software (WHAM, model version 7.0.5) was used to calculate the proportion of free La3+. The model requires as input the concentrations of humic and fulvic acids. Three scenarios were tested for predicting the La-binding by DOM (Supplementary Table S2): 1) the fulvic and humic acid concentrations are taken into account based on literature; 2) only the fulvic acid fraction, determined by PARAFAC, is taken into account; 3) DOM is composed only of fulvic acid concentration, which represents 65 % of the measured (Bryan et al., 2002).
2.5.1 Ion-exchange technique (IET)
The IET was used to determine free La3+ concentrations in each exposure medium as described by Leguay et al. (2016). This speciation technique is based on the establishment of an equilibrium between the free metal ion contained in solution and the binding sites on the resin in the presence of sufficient competing cations. The resin was equilibrated by a batch equilibration approach. Briefly, the following steps were followed: first 9 mg of the resin (Dowex 50W-X8, 50–100 mesh, Sigma Aldrich #60H0614) were weighed and successively put in contact with 1) 10 mL of 1.5 M HNO3 for 4 min, 2) 10 mL of ultrapure water for 30 s, 3) 20 mL of 1.0 M NaOH for 4 min, 4) 10 mL of ultrapure water for 30 s, 5) 10 mL of NaNO3 1.0 M for 2 min, repeated five times, and 6) 50 mL of sample until equilibrium is reached (24 h according to Leguay et al., 2016). Between each of these steps, a centrifugation at 5,000 rpm for 10 min was performed, to remove the supernatant. Total La concentrations in the supernatant were measured by ICP-MS after the equilibrium had been reached. The concentration of La3+ can be obtained using the quantity of La eluted from the resin. Elution of the La bound to the resin was accomplished with the following steps: 1) resin rinsed two times with 10 mL of ultrapure water, 2) 10 mL of 1.5 M HNO3 was added and left for 5 h and repeated three times. These three elutions provided a La recovery > 95 %. Finally, La3+ concentrations were calculated following Eq. 1.
where [La3+] is the free ion concentration of La (mol·L−1), V(el) is the elution volume (mL), [La]el is the concentration of La in the eluate (mol·L−1), mr is the mass of the resin (g), and λ is the distribution coefficient (387 ± 51 L·g−1; n = 5; pH = 5.0; in MHSM-2 growth medium supplemented with NaNO3 to reach an ionic strength of 0.1 M). This coefficient was first determined with solutions of known [La3+], having the same cationic composition, pH and equilibration time as the samples. Due to IET requirements, La3+ concentrations were determined using an ionic strength of 0.1 M, by adding NaNO3 to the medium. Since the exposure media had a lower ionic strength (0.02 M), PUF was also performed in these two conditions, for comparison.
2.5.2 Partial ultrafiltration (PUF)
The PUF technique was used as described by Hourtané et al. (2022). Briefly, polycarbonate tubes (Vivaspin; Sartorius) with 3 kDa PES membranes were washed to remove glycerine from the membranes, which is an important potential source of organic carbon that could interfere with our DOC measurements, according to the following steps: the membranes were first soaked in ultrapure water for 96 h, then 20 mL of fresh ultrapure water was added and centrifuged during 35 min at 5,000 rpm (4,300 g), three times. The membranes were then soaked again in ultrapure water for 48 h, and three centrifugations under the same conditions as before were carried out. This last step was repeated one more time. For each experiment, 20 mL of exposure medium was centrifuged for 8 min at 5,000 rpm, so that only a small volume (2–4 mL) can pass through the membrane (hence the “partial” UF). This was done to minimize changes in metal speciation in the retentate over time in which the total volume decreases during centrifugation while the total quantity of organic carbon remains constant, resulting in an increase in DOC concentration. The retentates and filtrates were collected for ICP-MS dosage of La and fluorescence measurements of TOC concentration. La3+ was calculated from the La ultrafiltrable fraction concentration (in which HA should not be present), using MINEQL+ to account for complexation by EDTA and inorganic ligands [see Leguay et al. (2016) for the thermodynamic data used]. A mass balance calculation was performed to verify organic matter recovery. The percentages of organic carbon measured in the ultrafiltrates were always less than 10 % of the sample TOC. Lanthanum mass balances were also calculated for all experiments and the recovery was higher than 87 %. As mentioned above, PUF was both determined in media with an ionic strength of 0.02 M and 0.1 M.
2.5.3 Lanthanum quantification
The La concentration in the different samples were measured by ICP-MS (Thermo Scientific model X series II). The instrument was calibrated before each analysis with a 1 μg·L−1 reference multi-element solution (Li, Be, Co, Ba, Ce, In and U). The detection limit was between 2 and 4 ng·L−1. The isotopes 115In and 205Tl were used as internal standards. The calibration curve was prepared with solutions of different concentrations of La (between 0 and 20 μg·L−1). This curve was then validated with a PlasmaCal certified standard (C00-061-406) at a concentration of 10 μg·L−1 and diluted to reach 0.5 μg·L−1, resulting in recoveries of 97.2 ± 3.7 (n = 102) and 97.5% ± 3.4 % (n = 104), respectively.
2.6 Data treatment
2.6.1 Statistical analyses
The R software with R studio was used for the statistical analyses of this work. The error was set at α = 0.05. One-way analysis of variance (ANOVA) followed by the Tukey’s post hoc test was used for differences in effective concentrations (EC50 values) in experiments with various DOMs, for the methodology verifications of PUF and IET and for the digestion method. To do so, normality was checked with the Shapiro-Wilks test and homogeneity of variance with the Levene test. Differences between mean values of La and TOC concentration and pH over time were performed using the non-parametric equivalent of ANOVA, the Kruskal-Wallis test. The relationship between each DOM quality parameters and La3+ EC50 values was performed by linear regression. To do so, four conditions must be verified: centering of the residuals (graphical method), independence of the residuals (Durbin-Watson test), homoscedasticity or homogeneity (Goldfeld-Quandt test) and normality (Shapiro-Wilks test).
2.6.2 Dose responses
The Toxicity Relationship Analysis Program (TRAP; USEPA) was used to fit the relative cell density versus the La concentrations measured over the experiment, from the toxicity test data. The data from the dose-response curves obtained were used to produce graphs with SigmaPlot software. The TRAP software also provides the EC50 (with confidence intervals), which indicate the concentration of La needed for a 50 % inhibition of algal cell yield after 96 h of exposure.
3 Results and discussion
3.1 Dissolved organic matter characterization
3.1.1 Absorbance indices
The calculated values of the E2/E3 index (Table 1) suggest that LM and the SRHA have higher molecular weight and more aromatic DOM, compared to BL and the SRFA. The study by Al-Reasi et al. (2012) also showed that LM had a lower value for this index than BL. The SR ratio results (Table 1) confirm this molecular weight classification, more precisely in the following order: LM > SRHA > SRFA > BL. According to the values of the SUVA index (Table 1), the most terrigenous DOMs (SRHA, SRFA, LM) are more aromatic than BL. These results show that the most autochthonous DOM (BL) has lower molecular weight and aromaticity.
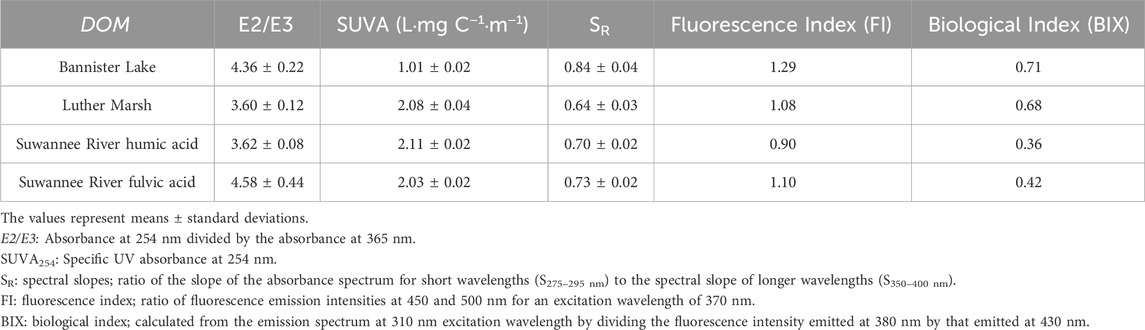
TABLE 1. Dissolved organic matter (DOM) optical parameters calculated from absorbance (E2/E3, SUVA254, SR; n = 3) and fluorescence (FI, BIX; n = 1) spectra.
3.1.2 Fluorescence indices
The fluorescence index (FI) can be used to determine the origin of DOM. Autochthonous DOM, which is microbially derived in the water column, is generally characterized by a FI value > 1.9, whereas terrigenous DOM, which is derived from higher plant degradation, has a value around 1.3 (McKnight et al., 2001). The values obtained for the FI (from 0.90 to 1.29 for the tested DOM samples) were outside the range of McKnight et al. (2001). Nonetheless, the results (Table 1) lead to the conclusion that there is a dominant terrestrial contribution for all organic matter tested. However, according to the results of Al-Reasi et al. (2012), the value obtained for BL was 1.46, which points to a mixed origin of DOM. The FI value obtained in our study for BL, although lower than that measured by Al-Reasi et al. (2012), remains nevertheless the highest value of the four studied DOMs, suggesting a higher microbial contribution for this sample. Finally, the FI values obtained for the SRHA and SRFA were of the same order of magnitude as those reported in the literature (0.84–1.06 for the SRHA and < 1.2 for the SRFA) (Korak et al., 2014; Ateia et al., 2017; Awfa et al., 2020). In addition, the BIX index can be used to characterize the autochthonous production of DOM due to biological activity. Values > 0.8 suggest the presence of freshly produced organic matter in the environment, while values around 0.5 represent terrigenous and/or mature material. According to our results (Table 1), LM and especially BL, are characterized by a mixture of sources with the presence of recent autochthonous material (significant biological activity in the environment), while the extracted humic and fulvic acids present a terrestrial/mature signature.
3.1.3 Excitation-emission matrix fluorescence
The EEM spectra of DOM were processed by PARAFAC (67 samples). A 4-component model was thus determined and validated, explaining 94.4 % of the variability of the entire dataset (Murphy et al., 2014). The spectra of the four components determined by PARAFAC (Figure 1) show a relatively high background noise in the signal, due to a high dilution of the samples required because of their high absorbance values and strong scattering. All components identified in the study were compared with OpenFluor’s database fluorescence spectra (Murphy et al., 2014). Components C1, C2, C3 and C4 were respectively matched with a total of 70, 1, 62 and 11 studies. The first two components (C1 and C2), which contribute to the fluorescence of the α′ and α bands (Parlanti et al., 2000), correspond to terrigenous material commonly found in fresh waters and generally associated with HMW and aromatic material of terrestrial origin (Osburn et al., 2016; Peleato et al., 2017; Du et al., 2021). Component C3 is associated with a protein-like material (Guéguen et al., 2014; Kida et al., 2019; Catalán et al., 2021), since its emission maximum is below 400 nm, similar to the γ fluorophore (Fellman et al., 2008). Component C4 corresponds to the β fluorophore and to freshly produced material (Huguet et al., 2009; Gao and Guéguen, 2017) or to a microbially derived component (Derrien et al., 2019). The fluorescence intensity in Raman units of each DOM component is shown in Figure 2. The fluorescence intensity distribution of the four components determined by PARAFAC shows a predominance of C3 and C4 for the natural DOM of Bannister Lake and Luther Marsh, reflecting a mixture of sources with a strong autochthonous biological contribution. In contrast, the distribution is logically dominated by C1 and C2 for the IHSS humic substances SRFA and SRHA.
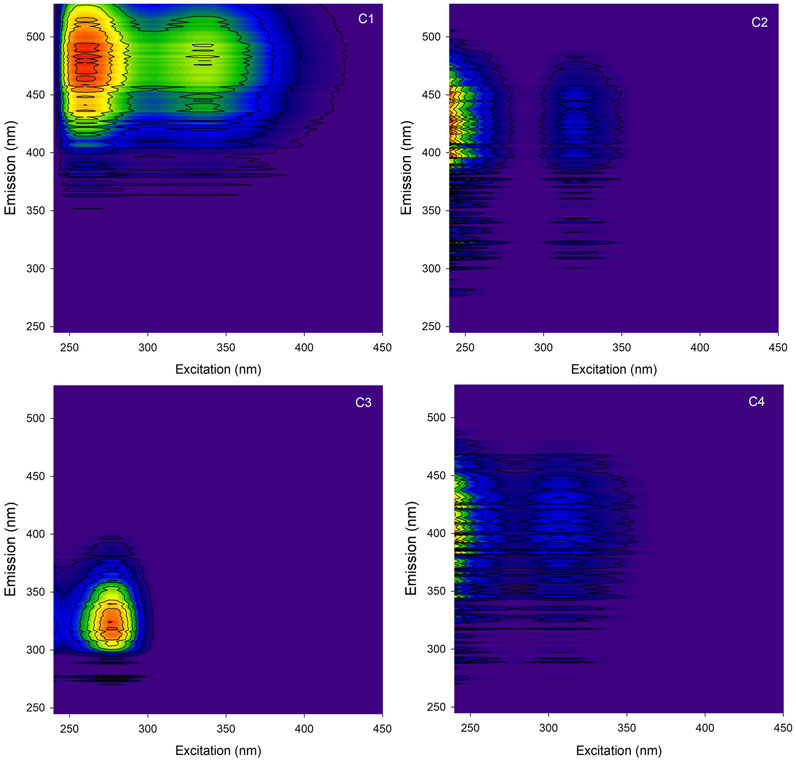
FIGURE 1. Excitation-emission matrices of the four components determined by parallel factor analysis.
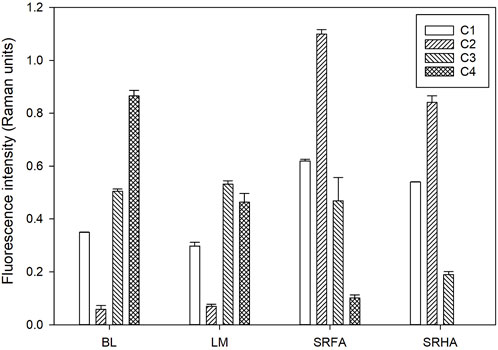
FIGURE 2. Fluorescence intensity in Raman units of each dissolved organic matter component identified by parallel factor analysis.
The complexation of metals with DOM generally leads to changes in different regions of its EEM fluorescence spectrum, with quenching (or enhancement) of emission intensities (Antunes et al., 2007; Ohno et al., 2008). In particular, fluorescence quenching has been widely used to study DOM-metal interactions (Manciulea et al., 2009; Wu et al., 2011; Wei et al., 2015). However, our results show that the presence of La does not significantly modify the fluorescence of DOM (i.e., the maximal intensities of the fluorophores and the FI and BIX indices; p > 0.05 or the component composition as indicated by PARAFAC analysis; p > 0.05). The absence of quenching is probably due to the relatively low metal concentrations and low pH used in our study as observed by others for Sm (Nduwayezu et al., 2016).
3.2 Speciation
In order to achieve good sample fractionation, several parameters affecting the separation efficiency must be optimized. The AF4 method was optimized in order to obtain adequate fractograms (i.e., the recovery rate, the shape of the peaks and the repeatability of the method) for all DOMs. According to the results obtained (Figure 3), it can be seen that Luther Marsh has three size classes of molecules, Bannister Lake has two, while SRHA and SRFA have one.
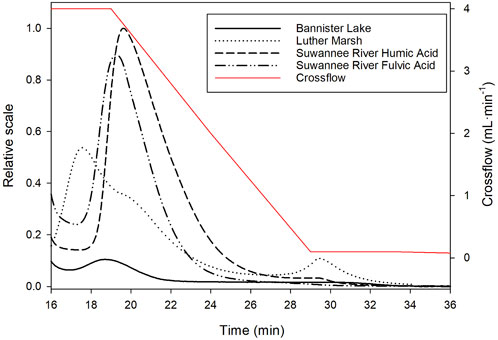
FIGURE 3. Fractograms of the different DOM obtained by UV detection at 254 nm as a function of retention time (1 kDa PES membrane, elution program with crossflow 4 mL·min−1 decreasing linearly to 0 mL·min−1 for 20 min). The crossflow is represented by the red line.
According to the fractograms obtained by AF4, the presence of La (5 µM) modified the size distribution of molecules for BL and LM (fewer classes), as well as their size (increased) by changing the retention time as shown for LM in Figure 4. The different size classes of the LM DOM sample with La were also collected to quantify La by ICP-MS. Assuming that no changes in speciation occurs during the analysis, the results indicate that La was predominantly associated with the smallest molecular size class of LM (Supplementary Figure S1). Such an observation can raise questions about the usefulness of size-based speciation method such as ultrafiltration if LMW ligands can preferentially bind metals and effectively pass through the ultrafiltration membrane. Two different methods (IET and PUF) were thus used to determine La speciation in the presence of DOM. The comparison of the two methods allowed us to verify the applicability of PUF to speciation determination in the presence of DOM.
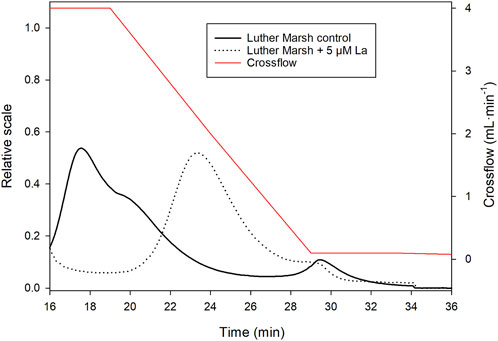
FIGURE 4. Fractograms of Luther Marsh with and without La (5 µM) obtained by UV detection at 254 nm as a function of retention time (1 kDa PES membrane, elution program with a crossflow of 4 mL·min−1 decreasing linearly to 0 mL·min−1 for 20 min). The crossflow is represented by the red line.
In the medium at pH 5.0 without organic matter, La3+ was the dominant species in solution based on thermodynamic calculations using MINEQL+, followed by LaEDTA− (ranging from 48% to 3% as [La] is increased) and LaSO4+ (0.7%–1.2%), while all other species were below 1 % of the mean measured total La concentrations. Comparison between PUF at 0.02 M and 0.1 M showed no significant differences for all tested DOM, except for SRHA for which a small but significant decrease in La binding at high ionic strength was observed. At 0.1 M ionic strength, the fraction of La bound to DOM showed no significant differences between IET and PUF. These results suggest that both methods are suitable to determine La speciation in the presence of DOM and that no significant La-DOM complexes ends up in the ultrafiltrate.
Finally, no significant differences were obtained between the % of La complexation with DOM between the start (0 h) and the end (96 h) of the exposure, for both the IET and PUF methods, indicating no significant speciation changes occurring during the algal growth. Both methods provided similar results, but lower standard deviations were observed for PUF compared to IET (Supplementary Figure S2). Values obtained with PUF were thus used for all subsequent data analyses.
In the presence of 3 mg C·L−1 of DOM, free La3+ concentrations decreased due to the strong complexation of La by DOM. For all La concentrations, there were no significant differences (p > 0.05) in La complexation among DOM types. Furthermore, the metal binding curves, represented by [La3+] versus [La]tot, compared among DOMs (Supplementary Figure S2) were similar. The change in slope at high La concentrations could be due to the formation of colloidal forms or precipitation. In a speciation study by Chen et al. (2018), the comparison of several metals (Pb, Zn and Cd) in the presence of different DOM samples (which included BL, LM and SRHA) showed that Pb was the metal for which the DOM source had the highest impact while for Cd and Zn few differences were observed. This could be explained by the metal affinity for complexation (Pb > Zn ≥ Cd). Lanthanum would be expected to have a binding affinity for DOM that is closer to that of Pb than those of Cd and Zn, given the hard character of La and its +3 electronic charge. The absence of any apparent differences between DOM sources in our case could be due to the relatively low pH used [5.0 as opposed to 8.0 in Chen et al. (2018)] that would shield most high pKa functional groups such as phenolic and amine groups.
The concentrations of La3+ measured experimentally and calculated using WHAM were compared, and a good relationship with [La3+] measured by PUF was obtained, which is presented in the Supplementary Figure S3. At low La concentrations, slightly greater complexation of La by DOM was predicted with WHAM compared to what was observed using PUF while the opposite was observed at high La total concentrations. To investigate the impact of how organic carbon is entered into WHAM input data, three different scenarios were tested. All resulted in good agreement between calculated and measured La3+ (Supplementary Table S3). More specifically, natural DOM (BL and LM) had a stronger relationship when 65 % of the DOM was considered as fulvic acid [the default settings recommended by WHAM developers (Bryan et al., 2002)], and for the two Suwannee River DOMs when the estimated fulvic concentration was used. In their work, Mueller et al. (2012) measured spectroscopically the proportion of FA by absorbance and fluorescence measurements in tested DOM, with the aim of incorporating this measure into WHAM. The relationships obtained were in good agreement for Cd, Cu, Zn and Ni, and demonstrated that DOM input parameters could be adjusted based on spectroscopic characteristics of the DOM to improve predictions by chemical speciation models. The authors pointed out that the affinity constants used in WHAM should also be adjusted, especially for Ni (Mueller et al., 2012). In other work, the performance of WHAM in predicting free metal ion concentrations varied with the metal considered and the analytical technique used to measure it (Batley et al., 2004; Lofts et al., 2008; Fortin et al., 2010; Tipping et al., 2016; Chen et al., 2018). If the biotic ligand model of acute metal toxicity is to be implemented using the WHAM model, it is clearly necessary to predict free metal ion concentrations in solution as accurately as possible. Our comparison indicates that WHAM can be successfully used for this purpose and that the default scenario to determine the model input data for DOM is adequate.
3.3 Toxicity and bioaccumulation
3.3.1 Toxicity
No differences were observed between control groups (without La) whether they were grown in the presence or absence of DOM. The dose-response curves (Figure 5; Supplementary Figure S4) and the resulting EC50 (Table 2) show higher toxicity based on measured free [La3+] in the presence of organic matter, compared to the condition without. Although the addition of DOM reduced the EC50 determined on the basis of the total La concentration in the medium, DOM did not protect as much as anticipated based on the measured free La3+ concentration. In the absence of DOM, the final yield of the culture (cell density after 96 h) remained close to that of the control up to ∼100 μg·L−1 free La3+ whereas in the presence of DOM, cultures were highly inhibited at that concentration. These results are in contradiction with the assumptions of the BLM, since the EC50 obtained, expressed as the free ion concentrations, are not similar between the experiments in the presence and in the absence of organic matter. The EC50 values expressed as the free ion concentration were very similar (i.e., the confidence intervals overlapped) for LM, BL and SRHA, whereas in the medium with SRFA the EC50 value was slightly higher than for the other DOM samples. When the relative yields of the cultures were plotted as a function of the internalized lanthanum (Figure 6), very similar trends were obtained with almost identical EC50 values based on internalized La (Table 2). The EC50 value for the cells exposed to BL DOM was however statistically different, about twice those of the other exposure conditions. This suggests that cells exposed to BL were slightly more tolerant to internal La than the other cells. In all the other exposures, a given internal dose resulted in the same effect level, indicating that the intracellular fate of La is likely identical.
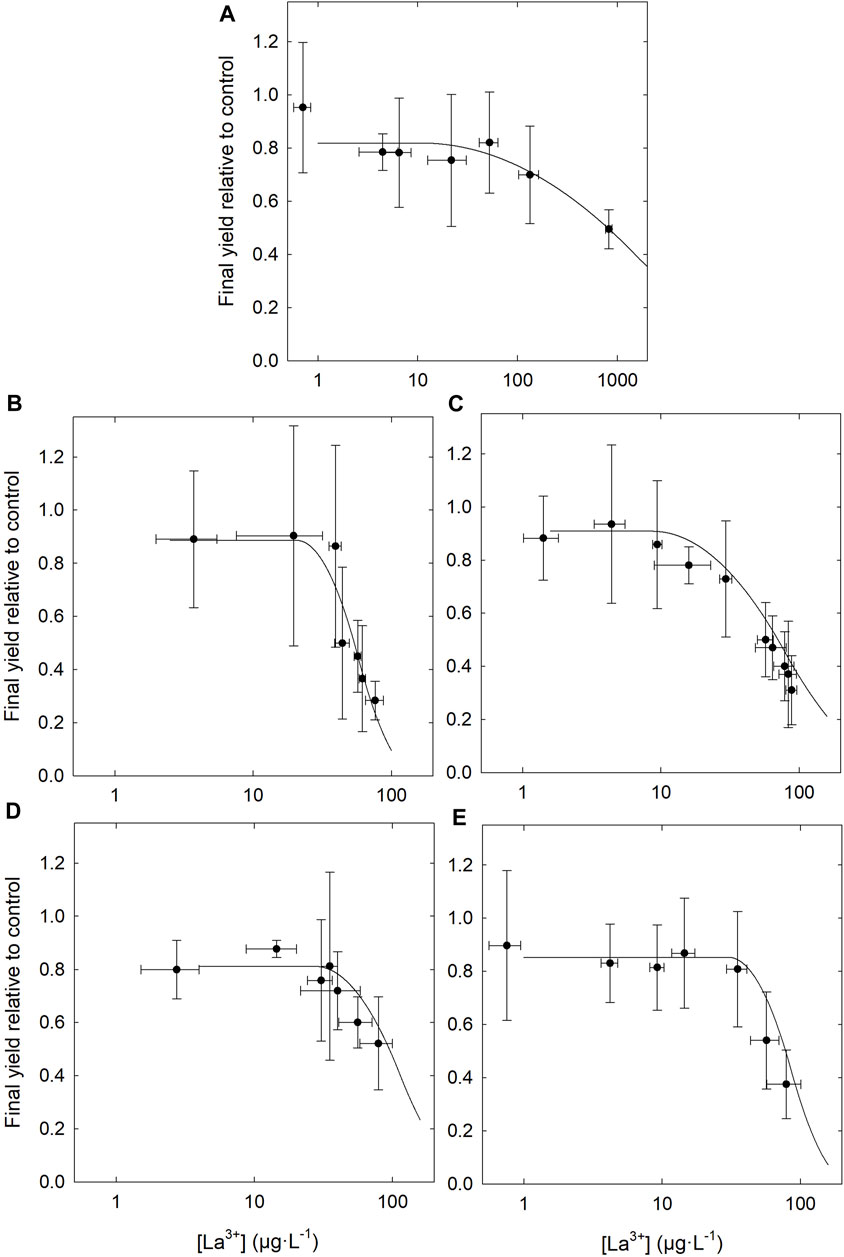
FIGURE 5. Dose-response curves for Chlorella fusca as a function of free La3+ concentrations measured by partial ultrafiltration. (A) Without DOM; and in the presence of 3 mg C·L−1 of DOM, (B) Bannister Lake, (C) Luther Marsh, (D) Suwannee River fulvic acid and (E) Suwannee River humic acid; in MHSM-2 growth medium for 96 h; pH = 5.0; (n = 3).
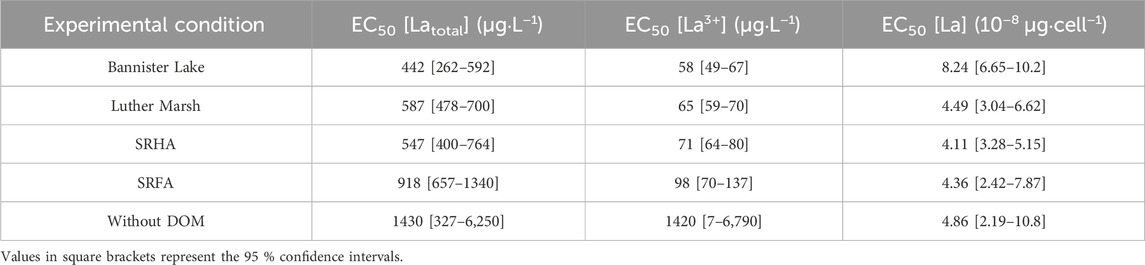
TABLE 2. Calculated EC50 of La for Chlorella fusca after 96 h of exposure in the absence and presence (3 mg C·L−1) of DOM; MHSM-2; pH = 5.0; (n = 3).
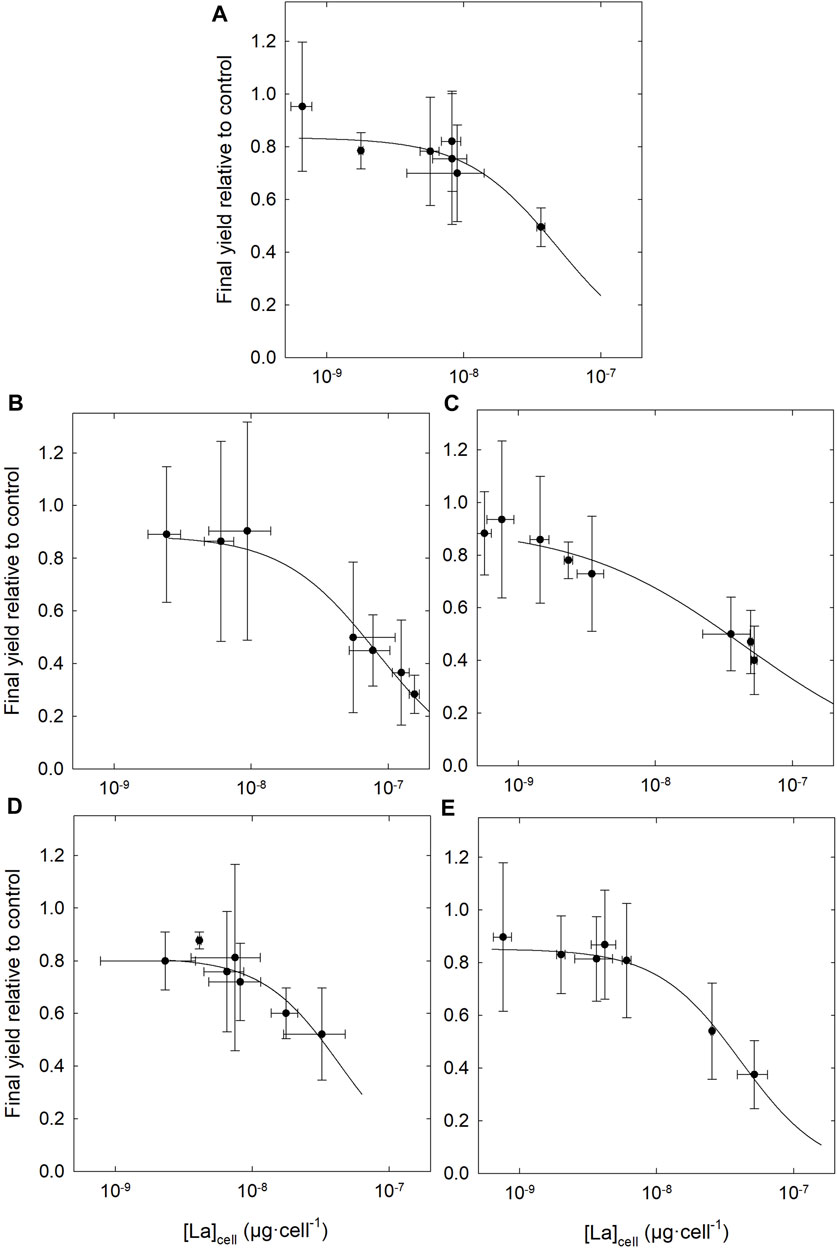
FIGURE 6. Dose-response curves for Chlorella fusca as a function of intracellular La content measured. (A) Without DOM; and in the presence of 3 mg C·L−1 of DOM (B) Bannister Lake, (C) Luther Marsh, (D) Suwannee River fulvic acid and (E) Suwannee River humic acid; in MHSM-2 growth medium for 96 h; pH = 5.0; (n = 3).
The EC50 values expressed as total dissolved La (Table 2) are much higher than the typical environmental concentrations (MacMillan et al., 2017). Therefore, La should not pose a direct risk at least for this species in natural aquatic systems unless a significant anthropogenic input occurs. The EC50 values obtained in the absence of organic ligands are in the range of values reported for algae in recent literature reviews on lanthanum aquatic toxicity (Herrmann et al., 2016; Liu et al., 2022). In a recent study by Aharchaou et al. (2020), the toxicity of La on the same biological model as this work, Chlorella fusca, was studied in relation to metal speciation. They compared exposure media containing simple monomeric organic ligands such as malic acid or NTA and media without ligands. Their EC50 values expressed as free La3+ were comparable between the different tested conditions (with and without an organic ligand), which suggest that the BLM can be used to predict La accumulation in algae in their conditions. Our DOM containing media results were in good agreements with their conditions with malic acid or NTA, but not for the condition without organic ligands, expressed as the free La3+. The experimental conditions in our study and that of Aharchaou et al. (2020) were slightly different [i.e., pH = 5.5 and absence of organic phosphorus for Aharchaou et al. (2020); pH = 5.0 for the present study with the presence of organic phosphorus], which may partly explain the difference in EC50 observed in the absence of DOM. Nevertheless, our data set clearly shows that the addition of DOM results in higher-than-expected toxicity based on the measured free La3+ ion concentrations. In this work, a slightly acidic pH was used. At circumneutral pHs, it can be expected that the extent of La binding by DOM will be much more important (Leguay et al., 2016). It remains to be determined whether an increase in complexation would result in an increase in La toxicity.
3.3.2 Bioaccumulation
Different trends were observed for the La cell content after 96 h between the exposure with and without DOM when plotted as a function of free La3+ exposure concentrations (Figure 7). In the medium without organic matter, net internalization reached a plateau between 10 and 100 μg·L−1 before increasing again at the highest concentration tested at which point the cell division was strongly inhibited. In the presence of DOM, a linear increase is observed with a change in slope at the onset of toxic effects (>60 μg·L−1). In this upper range of La3+ concentrations, C. fusca internalizes much more La in media with DOM than without DOM, with an order of magnitude difference on average. At lower [La3+] (subtoxic concentration range; 20–40 μg·L−1), however, accumulation seemed to remain in the same range for all exposure media except in the presence of LM DOM for which accumulation was lower. Finally, at the lowest La3+ concentrations (∼1 μg·L−1), less La was accumulated in the presence of DOM than in its absence, although results need to be interpreted with caution at these very low levels due to the scatter in the data. Among the exposure conditions with DOM, C. fusca appears to accumulate more La in the presence of DOM from BL than in the experiments with the other DOM samples. Overall, these results do not comply with the predictions of the BLM. Indeed, it seems that the presence of DOM promotes the entry of lanthanum into algal cells at high La concentrations, inducing greater toxicity than in the absence of DOM. These results suggest that natural DOM does not merely act as a metal binding ligand but also changes metal accumulation and toxicity.
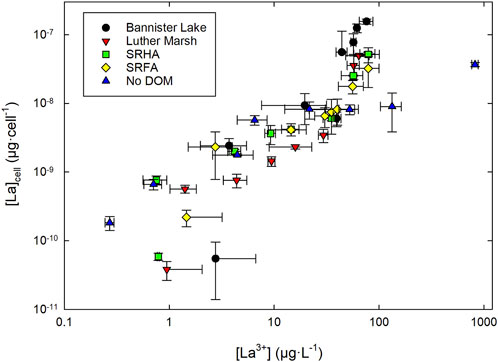
FIGURE 7. Internalized La by Chlorella fusca after 96 h of exposure, as a function of free measured [La3+] with (3 mg C·L−1) and without organic matter (log-log scale), in MHSM-2 growth medium; pH = 5.0; n = 3. The values represent means ± standard deviations.
The results obtained by Vigneault et al. (2000) showed that the adsorption of organic matter to the algal membrane surface can alter the membrane permeability of cells. Another study by Slaveykova et al. (2003) with Pb describes changes in algal surface charge due to adsorption of SRFA. This postulate could partly explain their observed increase of Pb uptake in the presence of SRFA, compared to the same concentration of Pb2+ in the presence of synthetic ligands. We hypothesize that DOM is altering the permeability of algal membranes to some metals such as Pb and La, but clearly the exact mechanism remains to be elucidated. The present results corroborate previous observations that metal uptake and toxicity in the presence of DOM cannot be fully explained by complexation (Parent et al., 1996; Lamelas et al., 2009; Worms et al., 2015; Hourtané et al., 2022; Barber-Lluch et al., 2023; Price et al., 2023).
3.4 Lanthanum toxicity as a function of optical parameters of DOM
Relationships were explored between the optical parameters of DOM and the EC50 values expressed as free La3+ concentrations. Relationships between E2/E3, SR and SUVA indices of DOM and La3+ CE50 values were not significant. The relationship between FI and La toxicity to C. fusca was also not statistically significant, although EC50 values do tend to decrease with increasing FI. In this case, the autochthonous-mixed BL organic matter promoted the most toxic conditions, based on La3+ EC50 and bioaccumulation values, in comparison with the other mostly allochthonous DOM. The relationship between BIX and the C2 component and La3+ toxicity was not significant either. However, the linear regression revealed that most variation in La3+ EC50 could be explained by the presence of the C1 component in DOMs (r2 = 0.87, p = 0.046). These statistical results were obtained by removing two values (out of 20) of C1 for LM, which were aberrant (outside the scatter plot). In other words, approximately 87 % of the variability in La toxicity is possibly explained by the aromatic character of DOM. Indeed, C1 is related to high molecular weights and aromatic material of terrestrial origin. Accordingly, SRHA and to a lesser extent SRFA exacerbated La toxicity less than DOM such as that from BL that contained less aromatic material. Note, however, that since EC50 values did not statistically differ among DOM sources, the relationship between aromaticity and the resulting metal toxicity remains speculative for now. This could be further tested in short term experiments using metal accumulation as an endpoint instead of toxicity. Such measurements can typically be determined with much more precision than long term biological responses such as growth inhibition.
In general, optically darker allochthonous DOM contributes to decrease metal toxicity more than does low-color autochthonous DOM (Richards et al., 2001; Luider et al., 2004; Schwartz et al., 2004; Al-Reasi et al., 2013). Al-Reasi et al. (2013) showed that autochthonous DOM, in their case the Bannister Lake DOM, was less protective against Cu toxicity to D. magna in terms of LC50 values than terrigenous DOM. In a study conducted by Schwartz et al. (2004), the time to reach 50 % mortality (LT50) for Cu and Pb to rainbow trout were well correlated to the source of some Canadian DOM samples. Allochthonous-like DOM decreased Cu and Pb toxicity more than did the autochthonous-like DOM. Another study by Richards et al. (2001) provided similar results for six metals (Pb, Hg, Cd, Cu, Ag, Co) with juvenile of rainbow trout, in the presence of 4, 6 and 10 mg C·L−1 of DOM.
The absorbance ratio of DOM in octanol versus water is obtained by measuring absorption at 254 nm of the aqueous and octanol fractions of the sample. This ratio indicates the overall lipophilicity of DOM, and therefore the potential capacity of DOM molecules to be associated with other organic molecules, such as biological membranes (Al-Reasi et al., 2012). Although this absorbance ratio was not measured in this study, Al-Reasi et al. (2012) found a significant correlation between this index and Cu toxicity (LC50), at 6 mg C·L−1 DOM concentration. Due to their size, DOM molecules are unlikely to cross the plasma membrane, but a lipophilic moiety could interact with the lipidic bilayer and alter its functions. This relationship was not significant at a DOM concentration of 3 mg C·L−1 for Cu, but it could be a possible explanation for the increased uptake of La by C. fusca in the presence of DOM from with Bannister Lake. Indeed, the octanol/water absorbance coefficients at 254 nm for SRHA, Bannister Lake and Luther Marsh are respectively 0.030, 0.024 and 0.016 (Al-Reasi et al., 2011).
4 Conclusion
In this work, we determined the accumulation and toxicity of La in the absence and presence of DOM of different origins using a freshwater green alga. In order to relate La speciation to its accumulation and effects, two methods were tested (IET and PUF). Both approaches gave comparable results, indicating that all four DOMs tested had similar binding affinities for La. Although AF4 results suggested that La preferentially binds to smaller size DOM molecules, PUF can be used with confidence to determine metal binding by natural DOM. PUF offers several advantages over IET: no calibration is needed, little specialized training is necessary and it does not require a fixed ionic strength.
As exposure La concentrations were increased, two patterns emerged in algal response: 1) at low ambient [La3+] (up to ∼20–40 μg·L−1), where little or no toxicity is observed, accumulation was similar under all tested conditions and was overall related to free La3+ concentration; 2) at high ambient [La3+], DOM-containing media exhibited much greater toxicity than in the absence of DOM. However, the internal dose of La required to induce growth inhibition remained similar among treatments. In other words, at a given toxic free La3+ ion concentration, more La is taken up by the cells in the presence of DOM. Further studies are required to understand how DOM can enhance La uptake in algal cells.
Scope statement
There are concerns about the increasing presence of lanthanides in aquatic ecosystems and knowledge gaps about their toxicity. In this study, we examined the role of organic matter on the uptake and toxicity of lanthanum to a freshwater alga. Although the presence of organic matter from four different origins decreased metal bioavailability, lanthanum accumulation and toxicity were greater than expected when expressed as a function of free lanthanide ion concentration.
Data availability statement
The datasets presented in this study can be found in online repositories. The names of the repository/repositories and accession number(s) can be found in the article/Supplementary Material.
Author contributions
LZ: Data curation, Formal Analysis, Investigation, Methodology, Validation, Visualization, Writing–original draft. EP: Writing–review and editing. CF: Conceptualization, Formal Analysis, Funding acquisition, Methodology, Project administration, Resources, Supervision, Visualization, Writing–review and editing.
Funding
The authors declare financial support was received for the research, authorship, and/or publication of this article. Funding provided by Environment and Climate Change Canada (Contribution Agreement for Metals in the Environment: Mitigating Environmental Risk and Increasing Sustainability, grant number GCXE17S011) and the Natural Sciences and Engineering Research Council of Canada (grant number STPGP 521467-18) is acknowledged. CF was supported by the Canada Research Chair Program (grant number 950-231107).
Acknowledgments
The authors are grateful to Scott Smith and James McGeer for providing the natural organic matter samples from Ontario. Also, we would like to thank Kim Racine from INRS, Antoine Lerat and Alexandra Coynel from Université de Bordeaux, EPOC, for the ICP-MS analyses of lanthanum as well as Mahaut Sourzac for her help in fluorescence analyses and AF4 fractionation. Louise Zilber was supported by the INRS - Université de Bordeaux joint master programme (MOBBIDIQ). Comments provided by Huy Dang and Peter Campbell on a previous version of the manuscript are gratefully acknowledged.
Conflict of interest
The authors declare that the research was conducted in the absence of any commercial or financial relationships that could be construed as a potential conflict of interest.
The authors declared that they were an editorial board member of Frontiers, at the time of submission. This had no impact on the peer review process and the final decision.
Publisher’s note
All claims expressed in this article are solely those of the authors and do not necessarily represent those of their affiliated organizations, or those of the publisher, the editors and the reviewers. Any product that may be evaluated in this article, or claim that may be made by its manufacturer, is not guaranteed or endorsed by the publisher.
Supplementary material
The Supplementary Material for this article can be found online at: https://www.frontiersin.org/articles/10.3389/fenvc.2024.1342500/full#supplementary-material
References
Aharchaou, I., Beaubien, C., Campbell, P. G. C., and Fortin, C. (2020). Lanthanum and cerium toxicity to the freshwater green alga Chlorella fusca: applicability of the Biotic Ligand Model. Environ. Toxicol. Chem. 39 (5), 996–1005. doi:10.1002/etc.4707
Al-Reasi, H. A., Smith, D. S., and Wood, C. M. (2012). Evaluating the ameliorative effect of natural dissolved organic matter (DOM) quality on copper toxicity to Daphnia magna: improving the BLM. Ecotoxicology 21 (2), 524–537. doi:10.1007/s10646-011-0813-z
Al-Reasi, H. A., Wood, C. M., and Smith, D. S. (2011). Physicochemical and spectroscopic properties of natural organic matter (NOM) from various sources and implications for ameliorative effects on metal toxicity to aquatic biota. Aquat. Toxicol. 103 (3-4), 179–190. doi:10.1016/j.aquatox.2011.02.015
Al-Reasi, H. A., Wood, C. M., and Smith, D. S. (2013). Characterization of freshwater natural dissolved organic matter (DOM): mechanistic explanations for protective effects against metal toxicity and direct effects on organisms. Environ. Int. 59, 201–207. doi:10.1016/j.envint.2013.06.005
Antunes, M. C. G., Pereira, C. C. C., and Esteves da Silva, J. C. G. (2007). MCR of the quenching of the EEM of fluorescence of dissolved organic matter by metal ions. Anal. Chim. Acta 595 (1), 9–18. doi:10.1016/j.aca.2006.12.017
Ateia, M., Apul, O. G., Shimizu, Y., Muflihah, A., Yoshimura, C., and Karanfil, T. (2017). Elucidating adsorptive fractions of natural organic matter on carbon nanotubes. Environ. Sci. Technol. 51 (12), 7101–7110. doi:10.1021/acs.est.7b01279
Awfa, D., Ateia, M., Fujii, M., and Yoshimura, C. (2020). Photocatalytic degradation of organic micropollutants: inhibition mechanisms by different fractions of natural organic matter. Water Res. 174, 115643. doi:10.1016/j.watres.2020.115643
Barber-Lluch, E., Nieto-Cid, M., Santos-Echeandía, J., and Sánchez-Marín, P. (2023). Effect of dissolved organic matter on copper bioavailability to a coastal dinoflagellate at environmentally relevant concentrations. Sci. Total Environ. 901, 165989. doi:10.1016/j.scitotenv.2023.165989
Batley, G. E., Apte, S. C., and Stauber, J. L. (2004). Speciation and bioavailability of trace metals in water: progress since 1982. Aust. J. Chem. 57 (10), 903–919. doi:10.1071/CH04095
Bryan, S. E., Tipping, E., and Hamilton-Taylor, J. (2002). Comparison of measured and modelled copper binding by natural organic matter in freshwaters. Comp. Biochem. Physiol. C 133 (1-2), 37–49. doi:10.1016/S1532-0456(02)00083-2
Campbell, P. G. C., and Fortin, C. (2013). “Biotic ligand model,” in Encyclopedia of aquatic ecotoxicology. Editors J.-F. Férard, and C. Blaise (Dordrecht: Springer Netherlands), 237–246. doi:10.1007/978-94-007-5704-2
Campbell, P. G. C., Twiss, M. R., and Wilkinson, K. J. (1997). Accumulation of natural organic matter on the surfaces of living cells: implications for the interaction of toxic solutes with aquatic biota. Can. J. Fish. Aquat. Sci. 54 (11), 2543–2554. doi:10.1139/f97-161
Catalán, N., Pastor, A., Borrego, C. M., Casas-Ruiz, J. P., Hawkes, J. A., Gutiérrez, C., et al. (2021). The relevance of environment vs composition on dissolved organic matter degradation in freshwaters. Limnol. Oceanogr. 66 (2), 306–320. doi:10.1002/lno.11606
Chen, W., Guéguen, C., Smith, D. S., Galceran, J., Puy, J., and Companys, E. (2018). Metal (Pb, Cd, and Zn) binding to diverse organic matter samples and implications for speciation modeling. Environ. Sci. Technol. 52 (7), 4163–4172. doi:10.1021/acs.est.7b05302
Crémazy, A., Braz-Mota, S., Brix, K. V., Duarte, R. M., Val, A. L., and Wood, C. M. (2022). Investigating the mechanisms of dissolved organic matter protection against copper toxicity in fish of Amazon's black waters. Sci. Total Environ. 843, 157032. doi:10.1016/j.scitotenv.2022.157032
Derrien, M., Shin, K.-H., and Hur, J. (2019). Biodegradation-induced signatures in sediment pore water dissolved organic matter: implications from artificial sediments composed of two contrasting sources. Sci. Total Environ. 694, 133714. doi:10.1016/j.scitotenv.2019.133714
Du, Y., Lu, Y., Roebuck, J. A., Liu, D., Chen, F., Zeng, Q., et al. (2021). Direct versus indirect effects of human activities on dissolved organic matter in highly impacted lakes. Sci. Total Environ. 752, 141839. doi:10.1016/j.scitotenv.2020.141839
El-Akl, P., Smith, S., and Wilkinson, K. J. (2015). Linking the chemical speciation of cerium to its bioavailability in water for a freshwater alga. Environ. Toxicol. Chem. 34 (8), 1711–1719. doi:10.1002/etc.2991
Fellman, J. B., D’Amore, D. V., Hood, E., and Boone, R. D. (2008). Fluorescence characteristics and biodegradability of dissolved organic matter in forest and wetland soils from coastal temperate watersheds in southeast Alaska. Biogeochemistry 88 (2), 169–184. doi:10.1007/s10533-008-9203-x
Fortin, C. (2024). Environmental toxicology of trace elements in aquatic systems-Beyond complexation and competition. Front. Environ. Chem - Inorg. Pollu. Sec. 1, 1345484. doi:10.3389/fentx.2023.1345484
Fortin, C., Couillard, Y., Vigneault, B., and Campbell, P. G. C. (2010). Determination of free Cd, Cu and Zn concentrations in lake waters by in situ diffusion followed by column equilibration ion-exchange. Aquat. Geochem 16 (1), 151–172. doi:10.1007/s10498-009-9074-3
Gao, Z., and Guéguen, C. (2017). Size distribution of absorbing and fluorescing DOM in beaufort sea, Canada basin. Deep Sea Res. Part I 121, 30–37. doi:10.1016/j.dsr.2016.12.014
Guéguen, C., Cuss, C. W., Cassels, C. J., and Carmack, E. C. (2014). Absorption and fluorescence of dissolved organic matter in the waters of the Canadian arctic archipelago, baffin bay, and the labrador sea. J. Geophys Res-Oceans 119 (3), 2034–2047. doi:10.1002/2013JC009173
Hassler, C. S., Slaveykova, V. I., and Wilkinson, K. J. (2004). Discriminating between intra- and extracellular metals using chemical extractions. Limnol. Oceanogr. Methods 2, 237–247. doi:10.4319/lom.2004.2.237
Helms, J. R., Stubbins, A., Ritchie, J. D., Minor, E. C., Kieber, D. J., and Mopper, K. (2008). Absorption spectral slopes and slope ratios as indicators of molecular weight, source, and photobleaching of chromophoric dissolved organic matter. Limnol. Oceanogr. 53 (3), 955–969. doi:10.4319/lo.2008.53.3.0955
Herrmann, H., Nolde, J., Berger, S., and Heise, S. (2016). Aquatic ecotoxicity of lanthanum – a review and an attempt to derive water and sediment quality criteria. Ecotoxicol. Environ. Saf. 124, 213–238. doi:10.1016/j.ecoenv.2015.09.033
Hourtané, O., Rioux, G., Campbell, P. G. C., and Fortin, C. (2022). Algal bioaccumulation and toxicity of platinum are increased in the presence of humic acids. Environ. Chem. 19 (4), 144–155. doi:10.1071/EN22037
Huguet, A., Vacher, L., Relexans, S., Saubusse, S., Froidefond, J. M., and Parlanti, E. (2009). Properties of fluorescent dissolved organic matter in the Gironde Estuary. Org. Geochem 40 (6), 706–719. doi:10.1016/j.orggeochem.2009.03.002
Joonas, E., Aruoja, V., Olli, K., Syvertsen-Wiig, G., Vija, H., and Kahru, A. (2017). Potency of (doped) rare earth oxide particles and their constituent metals to inhibit algal growth and induce direct toxic effects. Sci. Total Environ. 593, 478–486. doi:10.1016/j.scitotenv.2017.03.184
Kida, M., Kojima, T., Tanabe, Y., Hayashi, K., Kudoh, S., Maie, N., et al. (2019). Origin, distributions, and environmental significance of ubiquitous humic-like fluorophores in Antarctic lakes and streams. Water Res. 163, 114901. doi:10.1016/j.watres.2019.114901
Knauer, K., and Buffle, J. (2001). Adsorption of fulvic acid on algal surfaces and its effect on carbon uptake. J. Phycol. 37 (1), 47–51. doi:10.1046/j.1529-8817.2001.037001047.x
Korak, J. A., Dotson, A. D., Summers, R. S., and Rosario-Ortiz, F. L. (2014). Critical analysis of commonly used fluorescence metrics to characterize dissolved organic matter. Water Res. 49, 327–338. doi:10.1016/j.watres.2013.11.025
Lachaux, N., Catrouillet, C., Marsac, R., Poirier, L., Pain-Devin, S., Gross, E. M., et al. (2022). Implications of speciation on rare earth element toxicity: a focus on organic matter influence in Daphnia magna standard test. Environ. Pollut. 307, 119554. doi:10.1016/j.envpol.2022.119554
Lamelas, C., Pinheiro, J. P., and Slaveykova, V. I. (2009). Effect of humic acid on Cd(II), Cu(II), and Pb(II) uptake by freshwater algae: kinetic and cell wall speciation considerations. Environ. Sci. Technol. 43 (3), 730–735. doi:10.1021/Es802557r
Lead, J. R., Hamilton-Taylor, J., Peters, A., Reiner, S., and Tipping, E. (1998). Europium binding by fulvic acids. Anal. Chim. Acta 369 (1), 171–180. doi:10.1016/S0003-2670(98)00230-X
Leguay, S., Campbell, P. G. C., and Fortin, C. (2016). Determination of the free-ion concentration of rare earth elements by an ion-exchange technique: implementation, evaluation and limits. Environ. Chem. 13 (3), 478–488. doi:10.1071/en15136
Liu, S., Wang, Y., Zhang, R., Guo, G., Zhang, K., Fan, Y., et al. (2022). Water quality criteria for lanthanum for freshwater aquatic organisms derived via species sensitivity distributions and interspecies correlation estimation models. Ecotoxicology 31, 897–908. doi:10.1007/s10646-022-02557-z
Lofts, S., Tipping, E., and Hamilton-Taylor, J. (2008). The chemical speciation of Fe(III) in freshwaters. Aquat. Geochem 14 (4), 337–358. doi:10.1007/s10498-008-9040-5
Luider, C. D., Crusius, J., Playle, R. C., and Curtis, P. J. (2004). Influence of natural organic matter source on copper speciation as demonstrated by Cu binding to fish gills, by ion selective electrode, and by DGT gel sampler. Environ. Sci. Technol. 38 (10), 2865–2872. doi:10.1021/es030566y
MacMillan, G. A., Chételat, J., Heath, J. P., Mickpegak, R., and Amyot, M. (2017). Rare earth elements in freshwater, marine, and terrestrial ecosystems in the eastern Canadian Arctic. Environ. Sci. Process. Impacts 19 (10), 1336–1345. doi:10.1039/c7em00082k
Manciulea, A., Baker, A., and Lead, J. R. (2009). A fluorescence quenching study of the interaction of Suwannee River fulvic acid with iron oxide nanoparticles. Chemosphere 76 (8), 1023–1027. doi:10.1016/j.chemosphere.2009.04.067
McKnight, D. M., Boyer, E. W., Westerhoff, P. K., Doran, P. T., Kulbe, T., and Andersen, D. T. (2001). Spectrofluorometric characterization of dissolved organic matter for indication of precursor organic material and aromaticity. Limnol. Oceanogr. 46 (1), 38–48. doi:10.4319/lo.2001.46.1.0038
Mebane, C. A., Chowdhury, M. J., De Schamphelaere, K. A. C., Lofts, S., Paquin, P. R., Santore, R. C., et al. (2020). Metal bioavailability models: current status, lessons learned, considerations for regulatory use, and the path forward. Environ. Toxicol. Chem. 39 (1), 60–84. doi:10.1002/etc.4560
Mueller, K. K., Lofts, S., Fortin, C., and Campbell, P. G. C. (2012). Trace metal speciation predictions in natural aquatic systems: incorporation of dissolved organic matter (DOM) spectroscopic quality. Environ. Chem. 9 (4), 356–368. doi:10.1071/en11156
Murphy, K. R., Stedmon, C. A., Wenig, P., and Bro, R. (2014). OpenFluor– an online spectral library of auto-fluorescence by organic compounds in the environment. Anal. Methods 6 (3), 658–661. doi:10.1039/C3AY41935E
Nduwayezu, I., Mostafavirad, F., Hadioui, M., and Wilkinson, K. J. (2016). Speciation of a lanthanide (Sm) using an ion exchange resin. Anal. Methods 8 (37), 6774–6781. doi:10.1039/c6ay02018f
Ohno, T., Amirbahman, A., and Bro, R. (2008). Parallel factor analysis of excitation–emission matrix fluorescence spectra of water soluble soil organic matter as basis for the determination of conditional metal binding parameters. Environ. Sci. Technol. 42 (1), 186–192. doi:10.1021/es071855f
Ojwang, L. M., and Cook, R. L. (2013). Environmental conditions that influence the ability of humic acids to induce permeability in model biomembranes. Environ. Sci. Technol. 47 (15), 8280–8287. doi:10.1021/es4004922
Omanović, D., Marcinek, S., and Santinelli, C. (2023). TreatEEM—A software tool for the interpretation of fluorescence excitation-emission matrices (EEMs) of dissolved organic matter in natural waters. Water, 15, 2214. doi:10.3390/w15122214
Osburn, C. L., Boyd, T. J., Montgomery, M. T., Bianchi, T. S., Coffin, R. B., and Paerl, H. W. (2016). Optical proxies for terrestrial dissolved organic matter in estuaries and coastal waters. Front. Mar. Sci. 2. doi:10.3389/fmars.2015.00127
Parent, L., and Campbell, P. G. C. (1994). Aluminum bioavailability to the green alga Chlorella pyrenoidosa in acidified synthetic soft water. Environ. Toxicol. Chem. 13 (4), 587–598. doi:10.1002/etc.5620130407
Parent, L., Twiss, M. R., and Campbell, P. G. C. (1996). Influences of natural dissolved organic matter on the interaction of aluminum with the microalga Chlorella: a test of the free-ion model of trace metal toxicity. Environ. Sci. Technol. 30 (5), 1713–1720. doi:10.1021/ES950718S
Parlanti, E., Wörz, K., Geoffroy, L., and Lamotte, M. (2000). Dissolved organic matter fluorescence spectroscopy as a tool to estimate biological activity in a coastal zone submitted to anthropogenic inputs. Org. Geochem 31 (12), 1765–1781. doi:10.1016/S0146-6380(00)00124-8
Peleato, N. M., Sidhu, B. S., Legge, R. L., and Andrews, R. C. (2017). Investigation of ozone and peroxone impacts on natural organic matter character and biofiltration performance using fluorescence spectroscopy. Chemosphere 172, 225–233. doi:10.1016/j.chemosphere.2016.12.118
Perdue, E. M., and Ritchie, J. D. (2003). “Dissolved organic matter in freshwaters,” in Surface and ground water, weathering, and soils'. Editor J. I. Drever (Amsterdam: Elsevier), 273–318. doi:10.1016/B978-0-08-095975-7.00509-X
Peuravuori, J., and Pihlaja, K. (1997). Molecular size distribution and spectroscopic properties of aquatic humic substances. Anal. Chim. Acta 337 (2), 133–149. doi:10.1016/S0003-2670(96)00412-6
Pourret, O., Davranche, M., Gruau, G., and Dia, A. (2007). Organic complexation of rare earth elements in natural waters: evaluating model calculations from ultrafiltration data. Geochim. Cosmochim. Acta 71 (11), 2718–2735. doi:10.1016/j.gca.2007.04.001
Price, G. A. V., Stauber, J. L., Jolley, D. F., Koppel, D. J., Van Genderen, E. J., Ryan, A. C., et al. (2023). Natural organic matter source, concentration, and pH influences the toxicity of zinc to a freshwater microalga. Environ. Pollut. 318, 120797. doi:10.1016/j.envpol.2022.120797
Richards, J. G., Curtis, P. J., Burnison, B. K., and Playle, R. C. (2001). Effects of natural organic matter source on reducing metal toxicity to rainbow trout (Oncorhynchus mykiss) and on metal binding to their gills. Environ. Toxicol. Chem. 20 (6), 1159–1166. doi:10.1002/etc.5620200604
Schwartz, M. L., Curtis, P. J., and Playle, R. C. (2004). Influence of natural organic matter source on acute copper, lead, and cadmium toxicity to rainbow trout (Oncorhynchus mykiss). Environ. Toxicol. Chem. 23 (12), 2889–2899. doi:10.1897/03-561.1
Shi, Z., Du, H., Wang, C., and Xu, H. (2022). Quantifying the bioaccumulation of Pb to Chlorella vulgaris in the presence of dissolved organic matters with different molecular weights. Environ Sci Pollut Res 29, 70921–70932. doi:10.1007/s11356-022-19699-6
Slaveykova, V. I., Wilkinson, K. J., Ceresa, A., and Pretsch, E. (2003). Role of fulvic acid on lead bioaccumulation by Chlorella kesslerii. Environ. Sci. Technol. 37 (6), 1114–1121. doi:10.1021/es025993a
Sobek, S., Tranvik, L. J., Prairie, Y. T., Kortelainen, P., and Cole, J. J. (2007). Patterns and regulation of dissolved organic carbon: an analysis of 7,500 widely distributed lakes. Limnol. Oceanogr. 52 (3), 1208–1219. doi:10.4319/lo.2007.52.3.1208
Stedmon, C. A., and Bro, R. (2008). Characterizing dissolved organic matter fluorescence with parallel factor analysis: a tutorial. Limnol. Oceanogr. Methods 6, 572–579. doi:10.4319/lom.2008.6.572
Steinberg, C. E. W., Meinelt, T., Timofeyev, M. A., Bittner, M., and Menzel, R. (2008). Humic substances. Part 2: interactions with organisms. Environ Sci Pollut Res 15 (2), 128–135. doi:10.1065/espr2007.07.434
Thomsen, M., Dobel, S., Lassen, P., Carlsen, L., Bügel Mogensen, B., and Erik Hansen, P. (2002). Reverse quantitative structure–activity relationship for modelling the sorption of esfenvalerate to dissolved organic matter: a multivariate approach. Chemosphere 49 (10), 1317–1325. doi:10.1016/S0045-6535(02)00510-6
Tipping, E., Lofts, S., and Stockdale, A. (2016). Metal speciation from stream to open ocean: modelling v measurement. Environ. Chem. 13 (3), 464–477. doi:10.1071/EN15111
Vigneault, B., and Campbell, P. G. C. (2005). Uptake of cadmium by freshwater green algae: effects of pH and aquatic humic substances. J. Phycol. 41 (1), 55–61. doi:10.1111/j.1529-8817.2005.04068.x
Vigneault, B., Percot, A., Lafleur, M., and Campbell, P. G. C. (2000). Permeability changes in model and phytoplankton membranes in the presence of aquatic humic substances. Environ. Sci. Technol. 34 (18), 3907–3913. doi:10.1021/es001087r
Wei, J., Han, L., Song, J., and Chen, M. (2015). Evaluation of the interactions between water extractable soil organic matter and metal cations (Cu(II), Eu(III)) using excitation-emission matrix combined with parallel factor analysis. Int. J. Mol. Sci. 16 (7), 14464–14476. doi:10.3390/ijms160714464
Weishaar, J. L., Aiken, G. R., Bergamaschi, B. A., Fram, M. S., Fujii, R., and Mopper, K. (2003). Evaluation of specific ultraviolet absorbance as an indicator of the chemical composition and reactivity of dissolved organic carbon. Environ. Sci. Technol. 37 (20), 4702–4708. doi:10.1021/es030360x
Worms, I. A. M., Slaveykova, V. I., and Wilkinson, K. J. (2015). Lead bioavailability to freshwater microalgae in the presence of dissolved organic matter: contrasting effect of model humic substances and marsh water fractions obtained by ultrafiltration. Aquat. Geochem 21 (2-4), 217–230. doi:10.1007/s10498-015-9256-0
Wu, J., Zhang, H., He, P.-J., and Shao, L.-M. (2011). Insight into the heavy metal binding potential of dissolved organic matter in MSW leachate using EEM quenching combined with PARAFAC analysis. Water Res. 45 (4), 1711–1719. doi:10.1016/j.watres.2010.11.022
Keywords: Chlorella fusca, metal speciation, biotic ligand model, absorbance, fluorescence, DOM quality, rare earth element
Citation: Zilber L, Parlanti E and Fortin C (2024) Impact of organic matter of different origins on lanthanum speciation, bioavailability and toxicity toward a green alga. Front. Environ. Chem. 5:1342500. doi: 10.3389/fenvc.2024.1342500
Received: 21 November 2023; Accepted: 02 February 2024;
Published: 16 February 2024.
Edited by:
Ying Ge, Nanjing Agricultural University, ChinaReviewed by:
Ming Lei, Hunan Agricultural University, ChinaYu Yan, Huaqiao University, China
Fengjie Liu, Imperial College London, United Kingdom
Copyright © 2024 Zilber, Parlanti and Fortin. This is an open-access article distributed under the terms of the Creative Commons Attribution License (CC BY). The use, distribution or reproduction in other forums is permitted, provided the original author(s) and the copyright owner(s) are credited and that the original publication in this journal is cited, in accordance with accepted academic practice. No use, distribution or reproduction is permitted which does not comply with these terms.
*Correspondence: Claude Fortin, Y2xhdWRlLmZvcnRpbkBldGUuaW5ycy5jYQ==