- 1Special Mycology Laboratory, Escola Paulista de Medicina, Universidade Federal de São Paulo, São Paulo, Brazil
- 2Clinical Laboratory, Hospital Israelita Albert Einstein, São Paulo, Brazil
Antifungal resistance in humans, animals, and the environment is an emerging problem. Among the different fungal species that can develop resistance, Candida tropicalis is ubiquitous and causes infections in animals and humans. In Asia and some Latin American countries, C. tropicalis is among the most common species related to candidemia, and mortality rates are usually above 40%. Fluconazole resistance is especially reported in Asian countries and clonal spread in humans and the environment has been investigated in some studies. In Brazil, high rates of azole resistance have been found in animals and the environment. Multidrug resistance is still rare, but recent reports of clinical multidrug-resistant isolates are worrisome. The molecular apparatus of antifungal resistance has been majorly investigated in clinical C. tropicalis isolates, revealing that this species can develop resistance through the conjunction of different adaptative mechanisms. In this review article, we summarize the main findings regarding antifungal resistance and Candida tropicalis through an “One Health” approach.
Introduction
The yeast Candida tropicalis is a member of the kingdom Fungi, division Ascomycota, order Saccharomycetales. They are diploid dimorphic yeasts, showing ellipsoidal budding cells or as a pseudomycelium and can form true hyphae (Ann Chai et al., 2010). On Sabouraud dextrose agar C. tropicalis grows as cream-colored colonies with a slightly mycelial border, however, it is impossible to differentiate it from other Candida species. On CHROMagar™ Candida (CHROMagar Company Ltd), after 24-72 hours, colonies appear a metallic blue coloration, and that can be used as presumptive identification (Ann Chai et al., 2010; Silva et al., 2012).
Molecular data shows that C. tropicalis belong to the CUG-Ser 1 clade, meaning that the codon CUG is translated to serine instead of leucine (Krassowski et al., 2018). The diploid genome of C. tropicalis has about 14.5 Mb, and the species can mate via a parasexual cycle (Butler et al., 2009; Porman et al., 2011).
The pathogenicity of C. tropicalis is mediated by several virulence factors including extracellular secreted enzymes (phospholipase, hemolysin, coagulase, and proteinase), biofilm formation, and antifungal resistance. These extracellular enzymes contribute to C. tropicalis virulence, and, they have been reported to disrupt the host tissue, increasing the dissemination and evasion of macrophages after yeast cells phagocytosis (Togni et al., 1996; Zaugg et al., 2001; Deorukhkar et al., 2014; Staniszewska, 2020; Sasani et al., 2021). C. tropicalis is a greater biofilm producer in the Candida genus (Kumari et al., 2018), and mature biofilms are composed of a dense network of blastoconidia, containing an adhesive basal layer with cells that can form filaments with a large amount of extracellular matrix (Al-Fattani and Douglas, 2006; Soll, 2014; Araújo et al., 2017; Zheng et al., 2017; Dominguez et al., 2018). The extracellular matrix is composed of carbohydrates, proteins, phosphorus, uronic acid, and hexosamine, the major component in the matrix biofilm (Al-Fattani and Douglas, 2006).
Ubiquitous, C. tropicalis and has been recovered from different environmental sources. In Taiwan, the species has been retrieved from agricultural fields, forest soil, petroleum-contaminated, and sludge soil (Yang et al., 2012). In tropical China, C. tropicalis is the most frequent yeast found in the environment (Liu et al., 2022). One study that collected 968 environmental samples from soil, freshwater, and seawater from eight regions in tropical China showed 21% positivity for C. tropicalis (Liu et al., 2022). Brazilian researchers have shown that C. tropicalis can be found in the Amazon forest soil (Mok et al., 1984), agricultural fields (Sidrim et al., 2021), rivers and lakes (Medeiros et al., 2008), sand beaches (Zuza-Alves et al., 2019), sugar cane bagasse (Lara et al., 2014), coconut water (Maciel et al., 2013). It has also been recovered from soil samples collected in the USA and Ireland (O’Brien et al., 2021).
Microbiota analysis of terrestrial and marine mammals, as well as birds, crustaceans, and insects, have also revealed the presence of C. tropicalis (Takahashi et al., 2010; Hamad et al., 2014; Cordeiro et al., 2015). In humans, C. tropicalis can be found on the skin (Kam and Xu, 2002), nails (Kam and Xu, 2002), oral mucosa (Cui et al., 2015), gut (Hager and Ghannoum, 2017), and vagina (Barousse et al., 2004). Of note, patients with Crohn’s disease have increased levels of C. tropicalis in the gut compared to healthy individuals (Hager and Ghannoum, 2017).
Beyond colonization, C. tropicalis has been related to different infections in a wide range of animals, from gastrointestinal disease in sows (Zhai et al., 2021), bovine mastitis (Seker, 2010), oral mucositis in vultures exposed to antibiotics from livestock (Pitarch et al., 2020), urinary tract infection in dogs and cats (Ozawa et al., 2005), to genital tract infection in mares (Shokri et al., 2010). In humans, C. tropicalis has been related to superficial mycosis like onychomycosis (Arrua et al., 2015), otomycosis (Sabz et al., 2019), oral (Salehi et al., 2020) or skin candidiasis (Sanchez et al., 2021), keratitis (Rosa et al., 1994), and genital tract infections (Mushi et al., 2019). Regarding the invasive infections, C. tropicalis is an important agent of candidemia, ranking as the most common species in medical centers in India, Pakistan (Wang et al., 2016), Thailand (Ngamchokwathana et al., 2021), Algeria (Megri et al., 2020), and Tunisia (Sellami et al., 2011), and as the second most common Candida species in medical centers from, Brazil (Colombo et al., 2006; Agnelli et al., 2022), Colombia (Cortés et al., 2013), Venezuela (Dolande Franco et al., 2008), Mexico (Corzo-Leon et al., 2014), China (Liu et al., 2021), Singapore (Teo et al., 2017). Among countries in North hemisphere, cancer patients have a higher risk to be infected with C. tropicalis and fungemia by this species is associated to mortality rates that are usually above 40% (Kontoyiannis et al., 2001; Muñoz et al., 2011). In Asia and Latin America, C. tropicalis has been documented as a common agent of candidemia not only in cancer patients but also in adult and child critically ill patients (Nucci and Colombo, 2007; Agnelli et al., 2022).
The burden of antifungal-resistant Candida tropicalis in humans, animals, and the environment
Recent evidence points out that there is an increasing prevalence of fluconazole and multidrug resistance among C. tropicalis recovered from humans (Favarello et al., 2021; Wang et al., 2021). In China, some centers report a prevalence of 20-50% of fluconazole non-susceptibility among C. tropicalis clinical isolates (Fan et al., 2019; Wang et al., 2020; Wang et al., 2021), while in Algeria and Japan, reports show that more than 30% of C. tropicalis bloodstream isolates are fluconazole-resistant (Megri et al., 2020; Khalifa et al., 2022). In Turkey, a recent multicentric study showed that over 10% of the bloodstream isolates collected from 2017 to 2019 are resistant to fluconazole (Arastehfar et al., 2020b).
The burden of antifungal resistance in C. tropicalis has been well estimated in India (Mathews et al., 2001; Chakrabarti et al., 2015). Based on a multicentric study (Chakrabarti et al., 2015), a burden of 6.51 candidemia cases per 1000 ICU admissions were estimated, of which 41.6% (n=382) were C. tropicalis and resistance rates against fluconazole was 2.6% (n=10). In Brazil, the incidence of candidemia in Brazil is over 2 cases per 1000 admissions (Nucci et al., 2013). Taking into account a prevalence of 20% of the C. tropicalis in blood cultures (da Matta et al., 2017), and that approximately 4% of the C. tropicalis bloodstream isolates are fluconazole-resistant in the country (Favarello et al., 2021), one can estimate that for every 100000 admissions there will be at least one case of ARCT candidemia episode in Brazil.
The emergence of fluconazole resistance leads to echinocandin or amphotericin exposure and multidrug resistance is a future concern with C. tropicalis and human health. Despite its low prevalence (<1%), azole and echinocandin resistant C. tropicalis clinical isolates have been reported in the USA, in cancer patients (Garcia-Effron et al., 2008; Kofteridis et al., 2010; Sfeir et al., 2020), and Taiwan (Chen et al., 2019) and India (Chakrabarti et al., 2015). Amphotericin B resistance is also possible (Yang et al., 2004; Forastiero et al., 2013), although it is rarely reported in the world.
The burden of antifungal resistant C. tropicalis in animals is less estimated than it is in humans. However, the high prevalence of ARCT in animals has been pointed out in some studies (Khalifa et al., 2022). In Japan, 4% to 75% of C. tropicalis recovered from aquarium dolphins were fluconazole-resistant (Takahashi et al., 2010; Khalifa et al., 2022). In Brazil, over 50% of the C. tropicalis isolates from the microbiota of healthy animals (goat, sheep, rheas, psittacines, horses, sirenians, shrimp, tortoises, sea turtles) were also considered fluconazole-resistant (Brilhante et al., 2015; Cordeiro et al., 2015). In India, one study showed that C. tropicalis from health poultry microbiome had high fluconazole minimal inhibitory concentrations [MICs (4-16mg/L)] (Subramanya et al., 2017).
Rates of azole resistance in environmental isolates are still poorly investigated. In one Brazilian study, a high level of azole resistance in environmental C. tropicalis isolates was documented (Zuza-Alves et al., 2016a). Briefly, the study was carried out at the Northeastern coastal area from Brazil, and C. tropicalis isolates from sandy beaches showed 25% of azole resistance (Zuza-Alves et al., 2016a). A robust study that analyzed the prevalence of yeasts and antifungal resistance among environmental samples in tropical China revealed that over 21% of the C. tropicalis isolates were fluconazole-resistant (Liu et al., 2022).
Known antifungal tolerance and resistance mechanisms
C. tropicalis is known to have an effective response against osmotic stress being able to tolerate high salt concentrations. This species can grow in the presence of 10-15% sodium chloride and it was isolated in the Dead Sea and Amazonian forest in a hypersaline environment (Tokuoka, 1993; Ribeiro Bastos et al., 2000; Butinar et al., 2005). Its ability to tolerate an environment with high osmotic pressure is related to the activation of transporters that promote a rapid efflux of ions by efflux pumps (García et al., 1997; Zuza-Alves et al., 2017). A study has demonstrated the osmotolerance of C. tropicalis isolates obtained from the coastal environment in Brazil and its relation to virulence expression in vitro (Zuza-Alves et al., 2016b). The authors suggested that the presence of these yeasts in coastal environments may have led to the overexpression of efflux pumps, which may explain the high MICs values found on several drugs (azoles and amphotericin B). Another relevant biologic characteristic of C. tropicalis is its ability to tolerate and degrade phenol (Páca et al., 2005; Wang et al., 2011). Indeed, phenol is an aromatic compound applied in the production of pesticides, antiseptics, slimicides, and medicinal preparations (Mishra and Kumar, 2019), and is considered a marker of environmental pollution (Montaño et al., 2013). Recently, a study showed that C. tropicalis exposed to phenol compounds accumulated intracellular fatty acids and cell wall modifications that promote stress resistance. Moreover, the study also showed that phenol exposure leads to upregulation of efflux mechanisms correlated with azole resistance (Wang et al., 2011).
Antifungal tolerance precedes resistance and it has been investigated in clinical C. tropicalis strains. The calcineurin signaling pathway is activated under stress and turns C. tropicalis tolerant to azoles and echinocandins (Chen et al., 2014). The protein kinase A pathway and its catalytic subunits Tpk1 have also been associated with cell wall integrity and stress tolerance (Lin et al., 2018). The trailing phenomenon or residual growth above the minimal inhibitory concentration has been considered by some authors as a phenotypic expression of drug tolerance (Arastehfar et al., 2020b) or a less-susceptible phenotype (Choi et al., 2016a). Indeed, trailing-producing C. tropicalis strains have higher expression of efflux pumps compared to the azole wildtype ones (Astvad et al., 2018). Despite being a different population, tolerant or trailing-producing C. tropicalis strains (growth inhibition >50%) are not related to treatment failure. A study demonstrated that only microorganisms showing fluconazole growth inhibition that fulfill the definition of resistance (growth inhibition <50% starting from the MIC of 4mg/L) had treatment failure in an in vivo murine model of disseminated candidiasis (Astvad et al., 2018). Moreover, a multicentric study conducted in Spain showed that candidemia by trailing-producing Candida spp. was not associated with azole treatment failure (Rueda et al., 2017). Other authors (Colombo-Judith Berman) have found a correlation between fluconazole-tolerant Candida albicans and persistent candidemia in patients treated with fluconazole (Rosenberg et al., 2018).
Among the main factors in the development of fluconazole resistance, mutations in the ergosterol biosynthesis pathway genes are well characterized in C. tropicalis. The enzyme lanosterol 14 α-demethylase encoded by the ERG11 gene is the main target for azole antifungals (Cowen et al., 2014; Maubon et al., 2014; Sanglard, 2016; Robbins et al., 2017; Jordá and Puig, 2020). At least 31 mutations in ERG11 may be correlated to fluconazole resistance in C. tropicalis (Table 1), and the most common mutations are Y132F and S154F (Morio et al., 2010; Jiang et al., 2013; Cowen et al., 2014; Perlin et al., 2017; Robbins et al., 2017; Ksiezopolska and Gabaldón, 2018; Fan et al., 2019; Teo et al., 2019; Zhang et al., 2019; Castanheira et al., 2020).
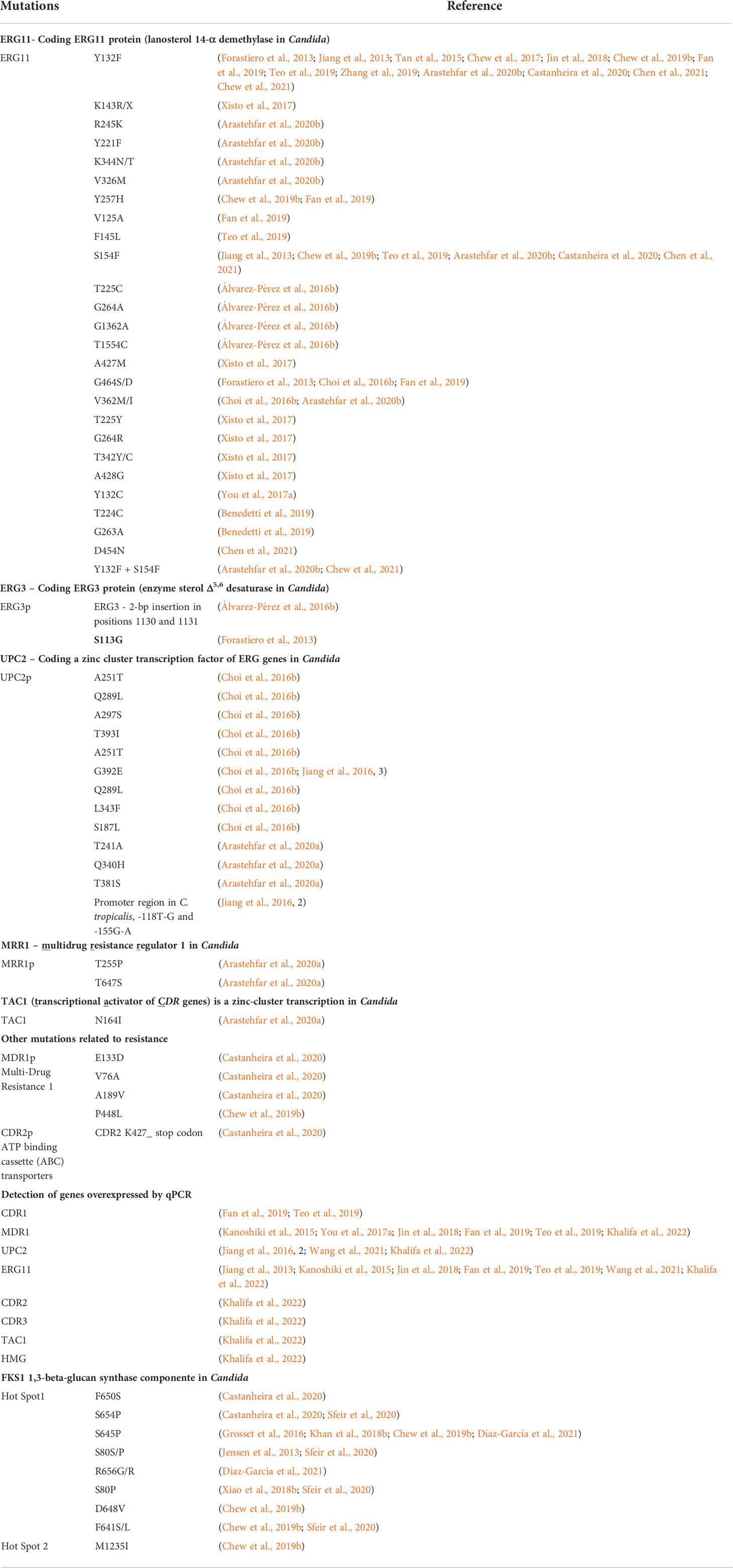
Table 1 Molecular mechanisms of resistance to fluconazole or echinocandins in C. tropicalis from invasive fungal infections.
The increased expression of genes related to the ergosterol biosynthesis process constitutes another mechanism of resistance to triazoles in C. tropicalis. These mechanisms are associated with the overexpression of the transcription factor UPC2, or with the formation of isochromosomes (Jiang et al., 2013; Cowen et al., 2014; Kanoshiki et al., 2015; Choi et al., 2016b; Perlin et al., 2017; Robbins et al., 2017; Jin et al., 2018; Fan et al., 2019; Teo et al., 2019).
Activation of efflux pumps is also described as an important mechanism of resistance to azole antifungal agents and in Candida spp. two different drug efflux systems have been associated with resistance to triazoles: the ATP-Binding Cassette (ABC) superfamily of proteins, and the Major Facilitator Superfamily (MFS) carriers (Sanglard, 2016; Perlin et al., 2017; Robbins et al., 2017; You et al., 2017a, 1). Proteins belonging to the ABC superfamily are primary transporters that hydrolyze ATP molecules and are essential to the transport of substrates. In C. tropicalis, the transporters named Candida Drug Resistance 1 and 2 (CDR1 and CDR2) are well characterized as responsible for the increase in resistance to triazoles (Table 2) (Cowen et al., 2014; Perlin et al., 2017; Robbins et al., 2017; Khalifa et al., 2022). The second efflux system associated with triazole resistance involves MFS transporters, which transport various substrates using a proton gradient generated in the plasma membrane. Among the described MFS transporters, only the Multi-Drug Resistance 1 (MDR1) gene has been associated with azole resistance in clinical isolates of C.tropicalis (Table 1) (Cowen et al., 2014; Perlin et al., 2017; Robbins et al., 2017; You et al., 2017a; Jin et al., 2018, 1). Moreover, gain-of-function mutations in transcription factors such as MMR1 and TAC1 genes were also described in C. tropicalis and can lead to higher expression of drug efflux pumps (Table 1).
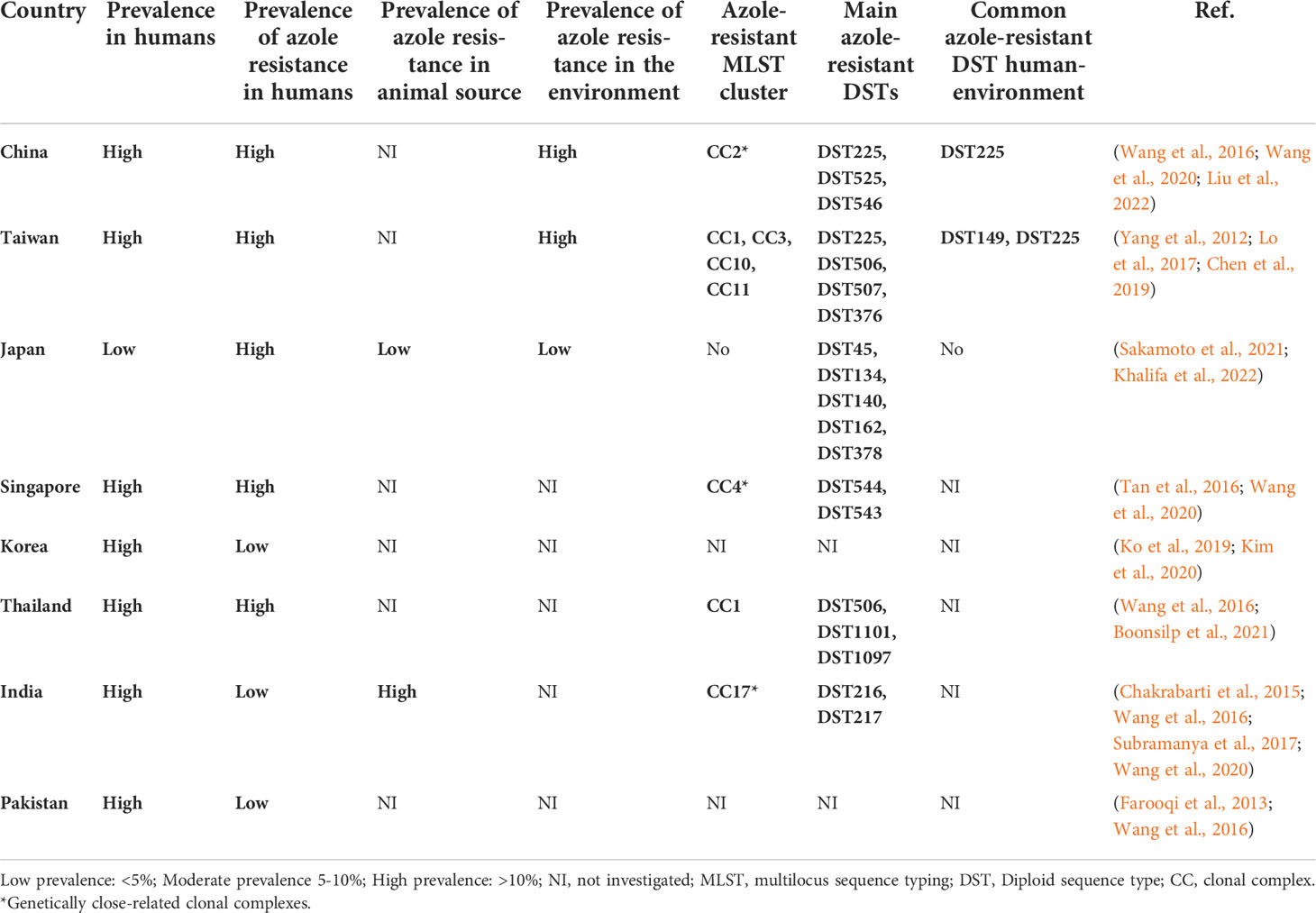
Table 2 Prevalence of Candida tropicalis from different Asian countries, niches, and multilocus sequence typing data.
Like in other Candida spp., echinocandin resistance is associated with mutations in the FKS1 gene (β-1, 3-D-glucan synthase, hotspot 1) in C. tropicalis (Table 1) (Cowen et al., 2014; Robbins et al., 2017; Khan et al., 2019; Pfaller et al., 2019; Castanheira et al., 2020).
Amphotericin B resistance is rare in C. tropicalis and only a few studies have tried to explore it. In Spain, a clinical isolate showing azole and amphotericin B cross-resistance had ERG11p (G464D) and ERG3p (S113G) mutations associated with the absence of ergosterol in the cell membrane (Forastiero et al., 2013). Moreover, it also had increased β-D-glucan in the cell wall, tolerance to reactive oxygen species, and stronger production of cytokines by peripheral blood mononuclear cells than amphotericin B- susceptible isolates (Mesa-Arango et al., 2014; Mesa-Arango et al., 2016).
Reservoirs of antifungal resistance
The human digestive tract is a relevant source of ARCT. Immunocompromised or critically ill patients exposed to antimicrobials and antifungals may have the selection of ARCT in the digestive tract and further develop an infection if the mucosa barrier is damaged(You et al., 2017b). ARCT has been isolated in patients with hematologic malignancies, mainly with acute leukemia (de Carvalho Parahym et al., 2011; Chong et al., 2012; You et al., 2017b). A study analyzed the azole resistance mechanisms behind blood isolates of ARCT recovered from a neutropenic patient with acute lymphoblastic leukemia under posaconazole prophylaxis. ERG11p Y132F and S154C mutations and MDR1 upregulation were related to azole resistance in these strains (You et al., 2017b). A study from Singapore also reported azole and echinocandin resistance in neutropenic patients with C. tropicalis fungemia (Chew et al., 2019a). Of note, the three isolates carried different FKS1p mutations, including F641L, D648V, and S645P (Chew et al., 2019a). Invasive devices are niches for biofilm production and resistance development. In China, a patient had a pan-echinocandin-resistant isolated from a thoracic drain after 18 days of exposure to micafungin. The isolate had a FKS1p mutation S80P(Xiao et al., 2018a). In Kuwait, a patient under mechanical ventilation had two echinocandin-resistant C. tropicalis recovered from endotracheal aspirates after short-term caspofungin exposure (Khan et al., 2018a).
C. tropicalis can be obtained from the microbiota of different wild and domesticated animal species, and livestock (Brito et al., 2009; Cordeiro et al., 2015; Zhai et al., 2021). In that context, fluconazole-resistant C. tropicalis has been recovered from mammals, birds, and crustaceans (Lord et al., 2010; Cordeiro et al., 2015; Álvarez-Pérez et al., 2016a; Ohno et al., 2019). A well-investigated case of C. tropicalis recurrent urinary tract in a dog showed a progressive increase in azole resistance after fluconazole exposure (Álvarez-Pérez et al., 2016a). No ERG11p mutations were detected despite high fluconazole MICs (>16mg/L), but isolates recovered after bladder irrigation with amphotericin B had an insertion at the ERG3 gene of two consecutive guanine nucleotides resulting in a change in the reading frame (Álvarez-Pérez et al., 2016a). Captive dolphins may develop pulmonary mycosis which is usually treated with itraconazole and voriconazole (Delaney et al., 2013). Antifungal exposed dolphins have azole-resistant C. tropicalis recovered from the respiratory in Japan (Takahashi et al., 2010; Ohno et al., 2019). Moreover, one study showed that the water from the dolphins’ aquarium had been contaminated with azole-resistant C. tropicalis (Takahashi et al., 2010).
Azoles have been used in agriculture, aquaculture, and wood preservation (Jørgensen and Heick, 2021). Almost 10% and 15% of azoles sold in the world are being used in rice and soybean cultures, respectively (Jørgensen and Heick, 2021). Moreover, fluconazole is the most consumed antifungal in medicine and now is among the most common drugs found in the wastewater from hospitals(Cai et al., 2021). In China, it has been estimated that over 17 tons of fluconazole is discarded every year in the wastewater (Liu et al., 2017). Water treatment methods are not able to eliminate fluconazole that has poor biodegradability (Cai et al., 2021), and has been found in some countries in drinkable water (Reis et al., 2019; Assress et al., 2020). Therefore, azole fungicides are now widely found in nature and may be exerting selective pressure in ubiquitous C. tropicalis. In this context, recent evidence from Asia is pointing out that environmental and human ARCT isolates have identical genetic profiles, suggesting that the environment may be the source of these opportunistic resistant pathogens (Table 2). In Taiwan, ARCT clinical isolates belong mainly to close-related diploid sequence types (DSTs) that form a cluster named clonal complex 3 (CC3)(Chen et al., 2019). The DST225 from the CC3 has been reported from fruits and hospitals in Taiwan(Lo et al., 2017). In Wuhan, continental China, one study showed similar findings and demonstrated that human ARCP strains belong to close-related DSTs (Table 2). Like the previously described data from Taiwan, the DST225 was also found in the environment in Wuhan (Wang et al., 2020). Moreover, in Thailand, clinical ARCP isolates belonging to the close-related DST225 and DST506 have also been described (Tulyaprawat et al., 2020; Boonsilp et al., 2021).
Conclusions
This study summarized the evidence showing that C. tropicalis is a relevant pathogenic species not only for humans but also for different domesticated and wild animals. Azole resistance in humans is particularly relevant in Asian countries but is emerging in other countries such as Turkey, Spain, and Algeria. Data from Brazil and India show that different animals may carry ARCT, but more information from other countries is necessary to better understand this phenomenon. Antifungal resistance mechanisms such as ERG11 mutations and efflux pumps hyperexpression have been described in ARCT clinical isolates, but veterinary and environmental molecular data are scarce. Azole fungicides are now widely found in nature and may be exerting selective pressure in ubiquitous C. tropicalis. As a consequence, environmental samplings have revealed a high prevalence of ARCT in the soil, freshwater, and seawater, especially in China and Brazil. Worrisome molecular data from China show clustering of ARCT from humans and the environment, highlighting the possible environmental source of antifungal resistance in the area.
Author contributions
JJ and RL designed the manuscript. RL, FR, and JJ did a literature review. RL, FR, AC, and JJ wrote the manuscript. JJ and AC revised the manuscript. All authors contributed to the article and approved the submitted version.
Funding
This study was supported by Fundação de Amparo à Pesquisa do Estado de São Paulo (FAPESP): 2017/02203-7. JNAJ has received a scholarship grant (FAPESP 2018/19347-4) and ALC a research Grant (FAPESP 2017/02203-7).
Conflict of interest
The authors declare that the research was conducted in the absence of any commercial or financial relationships that could be construed as a potential conflict of interest.
Publisher’s note
All claims expressed in this article are solely those of the authors and do not necessarily represent those of their affiliated organizations, or those of the publisher, the editors and the reviewers. Any product that may be evaluated in this article, or claim that may be made by its manufacturer, is not guaranteed or endorsed by the publisher.
References
Agnelli C., Valerio M., Bouza E., Guinea J., Sukiennik T., Guimarães T., et al. (2022). Prognostic factors of candida spp. bloodstream infection in adults: A nine-year retrospective cohort study across tertiary hospitals in Brazil and Spain. Lancet Regional Health - Americas 6, 100117. doi: 10.1016/j.lana.2021.100117
Al-Fattani M. A., Douglas L. J. (2006). Biofilm matrix of candida albicans and candida tropicalis: chemical composition and role in drug resistance. J. Med. Microbiol. 55, 999–1008. doi: 10.1099/jmm.0.46569-0
Álvarez-Pérez S., García M. E., Cutuli M. T., Fermín M. L., Daza M.Á., Peláez T., et al. (2016a). Acquired multi-azole resistance in candida tropicalis during persistent urinary tract infection in a dog. Med. Mycol Case Rep. 11, 9–12. doi: 10.1016/j.mmcr.2016.02.001
Álvarez-Pérez S., García M. E., Cutuli M. T., Fermín M. L., Daza M.Á., Peláez T., et al. (2016b). Acquired multi-azole resistance in candida tropicalis during persistent urinary tract infection in a dog. Med. Mycol Case Rep. 11, 9–12. doi: 10.1016/j.mmcr.2016.02.001
Ann Chai L. Y., Denning D. W., Warn P. (2010). Candida tropicalis in human disease. Crit. Rev. Microbiol. 36, 282–298. doi: 10.3109/1040841X.2010.489506
Arastehfar A., Daneshnia F., Hafez A., Khodavaisy S., Najafzadeh M.-J., Charsizadeh A., et al. (2020a). Antifungal susceptibility, genotyping, resistance mechanism, and clinical profile of candida tropicalis blood isolates. Med. Mycol. 58, 766–773. doi: 10.1093/mmy/myz124
Arastehfar A., Hilmioğlu-Polat S., Daneshnia F., Hafez A., Salehi M., Polat F., et al. (2020b). Recent increase in the prevalence of fluconazole-non-susceptible candida tropicalis blood isolates in Turkey: Clinical implication of azole-non-susceptible and fluconazole tolerant phenotypes and genotyping. Front. Microbiol. 11. doi: 10.3389/fmicb.2020.587278
Araújo D., Henriques M., Silva S. (2017). Portrait of candida species biofilm regulatory network genes. Trends Microbiol. 25, 62–75. doi: 10.1016/j.tim.2016.09.004
Arrua J. M. M., Rodrigues L. A. S., Pereira F. O., Lima E. O. (2015). Prevalence of candida tropicalis and candida krusei in onychomycosis in joão pessoa, paraiba, Brazil from 1999 to 2010. Acad. Bras. Cienc 87, 1819–1822. doi: 10.1590/0001-3765201520130418
Assress H. A., Nyoni H., Mamba B. B., Msagati T. A. M. (2020). Occurrence and risk assessment of azole antifungal drugs in water and wastewater. Ecotoxicol. Environ. Saf. 187, 109868. doi: 10.1016/j.ecoenv.2019.109868
Astvad K. M. T., Sanglard D., Delarze E., Hare R. K., Arendrup M. C. (2018). Implications of the EUCAST trailing phenomenon in candida tropicalis for the In vivo susceptibility in invertebrate and murine models. Antimicrob. Agents Chemother. 62, e01624–e01618. doi: 10.1128/AAC.01624-18
Barousse M. M., van der Pol B. J., Fortenberry D., Orr D., Fidel P. L. (2004). Vaginal yeast colonisation, prevalence of vaginitis, and associated local immunity in adolescents. Sex Transm. Infect. 80, 48–53. doi: 10.1136/sti.2002.003855
Benedetti V. P., Savi D. C., Aluizio R., Adamoski D., Kava V., Galli-Terasawa L. V., et al. (2019). ERG11 gene polymorphisms and susceptibility to fluconazole in candida isolates from diabetic and kidney transplant patients. Rev. Soc. Bras. Med. Trop. 52, e20180473. doi: 10.1590/0037-8682-0473-2018
Boonsilp S., Homkaew A., Phumisantiphong U., Nutalai D., Wongsuk T. (2021). Species distribution, antifungal susceptibility, and molecular epidemiology of candida species causing candidemia in a tertiary care hospital in Bangkok, Thailand. J. Fungi (Basel) 7, 577. doi: 10.3390/jof7070577
Brilhante R. S. N., Rodrigues P. H., de A., de Alencar L. P., Riello G. B., Ribeiro J. F., et al. (2015). Evidence of fluconazole-resistant candida species in tortoises and Sea turtles. Mycopathologia 180, 421–426. doi: 10.1007/s11046-015-9923-0
Brito E. H. S., Fontenelle R. O. S., Brilhante R. S. N., Cordeiro R. A., Monteiro A. J., Sidrim J. J. C., et al. (2009). The anatomical distribution and antimicrobial susceptibility of yeast species isolated from healthy dogs. Vet. J. 182, 320–326. doi: 10.1016/j.tvjl.2008.07.001
Butinar L., Santos S., Spencer-Martins I., Oren A., Gunde-Cimerman N. (2005). Yeast diversity in hypersaline habitats. FEMS Microbiol. Lett. 244, 229–234. doi: 10.1016/j.femsle.2005.01.043
Butler G., Rasmussen M. D., Lin M. F., Santos M. A. S., Sakthikumar S., Munro C. A., et al. (2009). Evolution of pathogenicity and sexual reproduction in eight candida genomes. Nature 459, 657–662. doi: 10.1038/nature08064
Cai W., Ye P., Yang B., Shi Z., Xiong Q., Gao F., et al. (2021). Biodegradation of typical azole fungicides in activated sludge under aerobic conditions. J. Environ. Sci. (China) 103, 288–297. doi: 10.1016/j.jes.2020.11.007
Castanheira M., Deshpande L. M., Messer S. A., Rhomberg P. R., Pfaller M. A. (2020). Analysis of global antifungal surveillance results reveals predominance of Erg11 Y132F alteration among azole-resistant candida parapsilosis and candida tropicalis and country-specific isolate dissemination. Int. J. Antimicrob. Agents 55, 105799. doi: 10.1016/j.ijantimicag.2019.09.003
Chakrabarti A., Sood P., Rudramurthy S. M., Chen S., Kaur H., Capoor M., et al. (2015). Incidence, characteristics and outcome of ICU-acquired candidemia in India. Intensive Care Med. 41, 285–295. doi: 10.1007/s00134-014-3603-2
Chen P.-Y., Chuang Y.-C., Wu U.-I., Sun H.-Y., Wang J.-T., Sheng W.-H., et al. (2019). Clonality of fluconazole-nonsusceptible candida tropicalis in bloodstream infections, Taiwan 2011-2017. Emerg. Infect. Dis. 25, 1660–1667. doi: 10.3201/eid2509.190520
Chen J., Hu N., Xu H., Liu Q., Yu X., Zhang Y., et al. (2021). Molecular epidemiology, antifungal susceptibility, and virulence evaluation of candida isolates causing invasive infection in a tertiary care teaching hospital. Front. Cell Infect. Microbiol. 11. doi: 10.3389/fcimb.2021.721439
Chen Y.-L., Yu S.-J., Huang H.-Y., Chang Y.-L., Lehman V. N., Silao F. G. S., et al. (2014). Calcineurin controls hyphal growth, virulence, and drug tolerance of candida tropicalis. Eukaryot. Cell 13, 844–854. doi: 10.1128/EC.00302-13
Chew K. L., Cheng J. W. S., Jureen R., Lin R. T. P., Teo J. W. P. (2017). ERG11 mutations are associated with high-level azole resistance in clinical candida tropicalis isolates, a Singapore study. Mycoscience 58, 111–115. doi: 10.1016/j.myc.2016.11.001
Chew K. L., Octavia S., Jureen R., Lin R. T. P., Teo J. W. P. (2021). Targeted amplification and MinION nanopore sequencing of key azole and echinocandin resistance determinants of clinically relevant candida spp. from blood culture bottles. Lett. Appl. Microbiol. 73 (3), 286–293. doi: 10.1111/lam.13516
Chew K. L., Octavia S., Lin R. T. P., Yan G. Z., Teo J. W. P. (2019a). Delay in effective therapy in anidulafungin-resistant candida tropicalis fungaemia: Potential for rapid prediction of antifungal resistance with whole-genome-sequencing. J. Global Antimicrob. Resist. 16, 105–107. doi: 10.1016/j.jgar.2018.12.010
Chew K. L., Octavia S., Lin R. T. P., Yan G. Z., Teo J. W. P. (2019b). Delay in effective therapy in anidulafungin-resistant candida tropicalis fungaemia: Potential for rapid prediction of antifungal resistance with whole-genome-sequencing. J. Glob. Antimicrob. Resist. 16, 105–107. doi: 10.1016/j.jgar.2018.12.010
Choi M. J., Won E. J., Shin J. H., Kim S. H., Lee W.-G., Kim M.-N., et al. (2016a). Resistance mechanisms and clinical features of fluconazole-nonsusceptible candida tropicalis isolates compared with fluconazole-Less-Susceptible isolates. Antimicrob. Agents Chemother. 60, 3653–3661. doi: 10.1128/AAC.02652-15
Choi M. J., Won E. J., Shin J. H., Kim S. H., Lee W.-G., Kim M.-N., et al. (2016b). Resistance mechanisms and clinical features of fluconazole-nonsusceptible candida tropicalis isolates compared with fluconazole-Less-Susceptible isolates. Antimicrob. Agents Chemother. 60, 3653–3661. doi: 10.1128/AAC.02652-15
Chong Y., Shimoda S., Yakushiji H., Ito Y., Miyamoto T., Shimono N., et al. (2012). Fatal candidemia caused by azole-resistant candida tropicalis in patients with hematological malignancies. J. Infect. Chemother. 18, 741–746. doi: 10.1007/s10156-012-0412-9
Colombo A. L., Nucci M., Park B. J., Nouér S. A., Arthington-Skaggs B., da Matta D. A., et al. (2006). Epidemiology of candidemia in Brazil: a nationwide sentinel surveillance of candidemia in eleven medical centers. J. Clin. Microbiol. 44, 2816–2823. doi: 10.1128/JCM.00773-06
Cordeiro R. A., de Oliveira J. S., de Castelo-Branco D. S. C. M., Teixeira C. E. C., Marques F. J., Bittencourt P. V., et al. (2015). Candida tropicalis isolates obtained from veterinary sources show resistance to azoles and produce virulence factors. Med. Mycol. 53, 145–152. doi: 10.1093/mmy/myu081
Cortés J. A., Jaimes J. A., Leal A. L. (2013). [Incidence and prevalence of candidemia in critically ill patients in Colombia]. Rev. Chil. Infectol. 30, 599–604. doi: 10.4067/S0716-10182013000600004
Corzo-Leon D. E., Alvarado-Matute T., Colombo A. L., Cornejo-Juarez P., Cortes J., Echevarria J. I., et al. (2014). Surveillance of candida spp bloodstream infections: epidemiological trends and risk factors of death in two Mexican tertiary care hospitals. PloS One 9, e97325. doi: 10.1371/journal.pone.0097325
Cowen L. E., Sanglard D., Howard S. J., Rogers P. D., Perlin D. S. (2014). Mechanisms of antifungal drug resistance. Cold Spring Harb. Perspect. Med. 5, a019752. doi: 10.1101/cshperspect.a019752
Cui L., Lucht L., Tipton L., Rogers M. B., Fitch A., Kessinger C., et al. (2015). Topographic diversity of the respiratory tract mycobiome and alteration in HIV and lung disease. Am. J. Respir. Crit. Care Med. 191, 932–942. doi: 10.1164/rccm.201409-1583OC
da Matta D. A., Souza A. C. R., Colombo A. L. (2017). Revisiting species distribution and antifungal susceptibility of candida bloodstream isolates from Latin American medical centers. J. Fungi (Basel) 3 (2), 24. doi: 10.3390/jof3020024
de Carvalho Parahym A. M. R., da Silva C. M., Leão M. P. C., Macario M. C., Filho G. A., da T. M. H., et al. (2011). Invasive infection in an acute myeloblastic leukemia patient due to triazole-resistant candida tropicalis. Diagn. Microbiol. Infect. Dis. 71, 291–293. doi: 10.1016/j.diagmicrobio.2011.08.004
Delaney M. A., Terio K. A., Colegrove K. M., Briggs M. B., Kinsel M. J. (2013). Occlusive fungal tracheitis in 4 captive bottlenose dolphins (Tursiops truncatus). Vet. Pathol. 50, 172–176. doi: 10.1177/0300985812446153
Deorukhkar S. C., Saini S., Mathew S. (2014). Virulence factors contributing to pathogenicity of Candida tropicalis and its antifungal susceptibility profile. Int. J. Microbiol. 2014, 1–6. doi: 10.1155/2014/456878
Díaz-García J., Mesquida A., Sánchez-Carrillo C., Reigadas E., Muñoz P., Escribano P., et al. (2021). Monitoring the epidemiology and antifungal resistance of yeasts causing fungemia in a tertiary care hospital in Madrid, Spain: Any relevant changes in the last 13 years? Antimicrob. Agents Chemother. 65, e01827–e01820. doi: 10.1128/AAC.01827-20
Dolande Franco M. E., Reviákina V., Panizo M. M., Macero C., Moreno X., Calvo A., et al. (2008). [Distribution and antifungal susceptibility of candida clinical isolations coming from six health care centers in the metropolitan area of Caracas, Venezuela (years 2003-2005)]. Rev. Iberoam Micol. 25, 17–21. doi: 10.1016/s1130-1406(08)70005-6
Dominguez E., Zarnowski R., Sanchez H., Covelli A. S., Westler W. M., Azadi P., et al. (2018). Conservation and divergence in the Candida species biofilm matrix mannan-glucan complex structure, function, and genetic control. mBio 9, e00451–e00418. doi: 10.1128/mBio.00451-18
Fan X., Xiao M., Zhang D., Huang J.-J., Wang H., Hou X., et al. (2019). Molecular mechanisms of azole resistance in candida tropicalis isolates causing invasive candidiasis in China. Clin. Microbiol. Infect. 25, 885–891. doi: 10.1016/j.cmi.2018.11.007
Farooqi J. Q., Jabeen K., Saeed N., Iqbal N., Malik B., Lockhart S. R., et al. (2013). Invasive candidiasis in Pakistan: clinical characteristics, species distribution and antifungal susceptibility. J. Med. Microbiol. 62, 259–268. doi: 10.1099/jmm.0.048785-0
Favarello L. M., Nucci M., Queiroz-Telles F., Guimarães T., Salles M. J., Sukiennik T. C. T., et al. (2021). Trends towards lower azole susceptibility among 200 candida tropicalis bloodstream isolates from Brazilian medical centres. J. Glob. Antimicrob. Resist. 25, 199–201. doi: 10.1016/j.jgar.2021.03.018
Forastiero A., Mesa-Arango A. C., Alastruey-Izquierdo A., Alcazar-Fuoli L., Bernal-Martinez L., Pelaez T., et al. (2013). Candida tropicalis antifungal cross-resistance is related to different azole target (Erg11p) modifications. Antimicrob. Agents Chemother. 57, 4769–4781. doi: 10.1128/AAC.00477-13
Garcia-Effron G., Kontoyiannis D. P., Lewis R. E., Perlin D. S. (2008). Caspofungin-resistant candida tropicalis strains causing breakthrough fungemia in patients at high risk for hematologic malignancies. Antimicrob. Agents Chemother. 52, 4181–4183. doi: 10.1128/AAC.00802-08
García M. J., Ríos G., Ali R., Bellés J. M., Serrano R. (1997). Comparative physiology of salt tolerance in candida tropicalis and saccharomyces cerevisiae. Microbiology 143, 1125–1131. doi: 10.1099/00221287-143-4-1125
Grosset M., Desnos-Ollivier M., Godet C., Kauffmann-Lacroix C., Cazenave-Roblot F. (2016). Recurrent episodes of candidemia due to candida glabrata, candida tropicalis and candida albicans with acquired echinocandin resistance. Med. Mycol. Case Rep. 14, 20–23. doi: 10.1016/j.mmcr.2016.12.004
Hager C. L., Ghannoum M. A. (2017). The mycobiome: Role in health and disease, and as a potential probiotic target in gastrointestinal disease. Dig. Liver Dis. 49, 1171–1176. doi: 10.1016/j.dld.2017.08.025
Hamad I., Keita M. B., Peeters M., Delaporte E., Raoult D., Bittar F. (2014). Pathogenic eukaryotes in gut microbiota of western lowland gorillas as revealed by molecular survey. Sci. Rep. 4, 6417. doi: 10.1038/srep06417
Jørgensen L. N., Heick T. M. (2021). Azole use in agriculture, horticulture, and wood preservation – is it indispensable? Front. Cell. Infect. Microbiol. 11. doi: 10.3389/fcimb.2021.730297
Jensen R. H., Johansen H. K., Arendrup M. C. (2013). Stepwise development of a homozygous S80P substitution in Fks1p, conferring echinocandin resistance in candida tropicalis. Antimicrob. Agents Chemother. 57, 614–617. doi: 10.1128/AAC.01193-12
Jiang C., Dong D., Yu B., Cai G., Wang X., Ji Y., et al. (2013). Mechanisms of azole resistance in 52 clinical isolates of candida tropicalis in China. J. Antimicrob. Chemother. 68, 778–785. doi: 10.1093/jac/dks481
Jiang C., Ni Q., Dong D., Zhang L., Li Z., Tian Y., et al. (2016). The role of UPC2 gene in azole-resistant candida tropicalis. Mycopathologia 181, 833–838. doi: 10.1007/s11046-016-0050-3
Jin L., Cao Z., Wang Q., Wang Y., Wang X., Chen H., et al. (2018). MDR1 overexpression combined with ERG11 mutations induce high-level fluconazole resistance in candida tropicalis clinical isolates. BMC Infect. Dis. 18, 162. doi: 10.1186/s12879-018-3082-0
Jordá T., Puig S. (2020). Regulation of ergosterol biosynthesis in saccharomyces cerevisiae. Genes (Basel) 11, E795. doi: 10.3390/genes11070795
Kam A. P., Xu J. (2002). Diversity of commensal yeasts within and among healthy hosts. Diagn. Microbiol. Infect. Dis. 43, 19–28. doi: 10.1016/S0732-8893(02)00364-4
Kanoshiki R. L., de Paula S. B., Santos J. P., Morey A. T., Souza N. B., Yamauchi L. M., et al. (2015). Effects of fluconazole treatment of mice infected with fluconazole-susceptible and -resistant candida tropicalis on fungal cell surface hydrophobicity, adhesion and biofilm formation. Indian J. Med. Microbiol. 33 Suppl, 97–101. doi: 10.4103/0255-0857.148834
Khalifa H. O., Watanabe A., Kamei K. (2022). Azole and echinocandin resistance mechanisms and genotyping of candida tropicalis in Japan: cross-boundary dissemination and animal-human transmission of c. tropicalis infection. Clin. Microbiol. Infect. 28, 302.e5–302.e8. doi: 10.1016/j.cmi.2021.10.004
Khan Z., Ahmad S., Al-Sweih N., Mokaddas E., Al-Banwan K., Alfouzan W., et al. (2019). Changing trends in epidemiology and antifungal susceptibility patterns of six bloodstream candida species isolates over a 12-year period in Kuwait. PloS One 14, e02176250. doi: 10.1371/journal.pone.0216250
Khan Z., Ahmad S., Mokaddas E., Meis J. F., Joseph L., Abdullah A., et al. (2018a). Development of echinocandin resistance in candida tropicalis following short-term exposure to caspofungin for empiric therapy. Antimicrob. Agents Chemother. 62, e01926–e01917. doi: 10.1128/AAC.01926-17
Khan Z., Ahmad S., Mokaddas E., Meis J. F., Joseph L., Abdullah A., et al. (2018b). Development of echinocandin resistance in candida tropicalis following short-term exposure to caspofungin for empiric therapy. Antimicrob. Agents Chemother. 62, e01926–e01917. doi: 10.1128/AAC.01926-17
Kim E. J., Lee E., Kwak Y. G., Yoo H. M., Choi J. Y., Kim S. R., et al. (2020). Trends in the epidemiology of candidemia in intensive care units from 2006 to 2017: Results from the Korean national healthcare-associated infections surveillance system. Front. Med. (Lausanne) 7. doi: 10.3389/fmed.2020.606976
Kofteridis D. P., Lewis R. E., Kontoyiannis D. P. (2010). Caspofungin-non-susceptible candida isolates in cancer patients. J. Antimicrob. Chemother. 65, 293–295. doi: 10.1093/jac/dkp444
Ko J.-H., Jung D. S., Lee J. Y., Kim H. A., Ryu S. Y., Jung S.-I., et al. (2019). Changing epidemiology of non-albicans candidemia in Korea. J. Infect. Chemother. 25, 388–391. doi: 10.1016/j.jiac.2018.09.016
Kontoyiannis D. P., Vaziri I., Hanna H. A., Boktour M., Thornby J., Hachem R., et al. (2001). Risk factors for candida tropicalis fungemia in patients with cancer. Clin. Infect. Dis. 33, 1676–1681. doi: 10.1086/323812
Krassowski T., Coughlan A. Y., Shen X.-X., Zhou X., Kominek J., Opulente D. A., et al. (2018). Evolutionary instability of CUG-leu in the genetic code of budding yeasts. Nat. Commun. 9, 1887. doi: 10.1038/s41467-018-04374-7
Ksiezopolska E., Gabaldón T. (2018). Evolutionary emergence of drug resistance in candida opportunistic pathogens. Genes (Basel) 9, E461. doi: 10.3390/genes9090461
Kumari A., Mankotia S., Chaubey B., Luthra M., Singh R. (2018). Role of biofilm morphology, matrix content and surface hydrophobicity in the biofilm-forming capacity of various candida species. J. Med. Microbiol. 67, 889–892. doi: 10.1099/jmm.0.000747
Lara C. A., Santos R. O., Cadete R. M., Ferreira C., Marques S., Gírio F., et al. (2014). Identification and characterisation of xylanolytic yeasts isolated from decaying wood and sugarcane bagasse in Brazil. Antonie Van Leeuwenhoek 105, 1107–1119. doi: 10.1007/s10482-014-0172-x
Lin C.-J., Wu C.-Y., Yu S.-J., Chen Y.-L. (2018). Protein kinase a governs growth and virulence in candida tropicalis. Virulence 9, 331–347. doi: 10.1080/21505594.2017.1414132
Liu Y., Chen Z., Li J., Zhu Z., Pang S., Xu J., et al. (2022). Extensive diversity and prevalent fluconazole resistance among environmental yeasts from tropical China. Genes (Basel) 13, 444. doi: 10.3390/genes13030444
Liu W.-R., Yang Y.-Y., Liu Y.-S., Zhang L.-J., Zhao J.-L., Zhang Q.-Q., et al. (2017). Biocides in wastewater treatment plants: Mass balance analysis and pollution load estimation. J. Hazardous Mater. 329, 310–320. doi: 10.1016/j.jhazmat.2017.01.057
Liu F., Zhong L., Zhou F., Zheng C., Zhang K., Cai J., et al. (2021). Clinical features, strain distribution, antifungal resistance and prognosis of patients with non-albicans candidemia: A retrospective observational study. Infect. Drug Resist. 14, 3233–3246. doi: 10.2147/IDR.S323583
Lord A. T. K., Mohandas K., Somanath S., Ambu S. (2010). Multidrug resistant yeasts in synanthropic wild birds. Ann. Clin. Microbiol. Antimicrob. 9, 11. doi: 10.1186/1476-0711-9-11
Lo H.-J., Tsai S.-H., Chu W.-L., Chen Y.-Z., Zhou Z.-L., Chen H.-F., et al. (2017). Fruits as the vehicle of drug resistant pathogenic yeasts. J. Infect. 75, 254–262. doi: 10.1016/j.jinf.2017.06.005
Maciel N. O. P., Piló F. B., Freitas L. F. D., Gomes F. C. O., Johann S., Nardi R. M. D., et al. (2013). The diversity and antifungal susceptibility of the yeasts isolated from coconut water and reconstituted fruit juices in Brazil. Int. J. Food Microbiol. 160, 201–205. doi: 10.1016/j.ijfoodmicro.2012.10.012
Mathews M. S., Samuel P. R., Suresh M. (2001). Emergence of candida tropicalis as the major cause of fungaemia in India. Mycoses 44, 278–280. doi: 10.1111/j.1439-0507.2001.00641.x
Maubon D., Garnaud C., Calandra T., Sanglard D., Cornet M. (2014). Resistance of candida spp. to antifungal drugs in the ICU: where are we now? Intensive Care Med. 40, 1241–1255. doi: 10.1007/s00134-014-3404-7
Medeiros A. O., Kohler L. M., Hamdan J. S., Missagia B. S., Barbosa F. A. R., Rosa C. A. (2008). Diversity and antifungal susceptibility of yeasts from tropical freshwater environments in southeastern Brazil. Water Res. 42, 3921–3929. doi: 10.1016/j.watres.2008.05.026
Megri Y., Arastehfar A., Boekhout T., Daneshnia F., Hörtnagl C., Sartori B., et al. (2020). Candida tropicalis is the most prevalent yeast species causing candidemia in Algeria: the urgent need for antifungal stewardship and infection control measures. Antimicrob. Resist. Infect. Control 9, 50. doi: 10.1186/s13756-020-00710-z
Mesa-Arango A. C., Rueda C., Román E., Quintin J., Terrón M. C., Luque D., et al. (2016). Cell wall changes in amphotericin b-resistant strains from candida tropicalis and relationship with the immune responses elicited by the host. Antimicrob. Agents Chemother. 60, 2326–2335. doi: 10.1128/AAC.02681-15
Mesa-Arango A. C., Trevijano-Contador N., Román E., Sánchez-Fresneda R., Casas C., Herrero E., et al. (2014). The production of reactive oxygen species is a universal action mechanism of amphotericin b against pathogenic yeasts and contributes to the fungicidal effect of this drug. Antimicrobial Agents Chemother. 58, 6627–6638. doi: 10.1128/AAC.03570-14
Mishra V. K., Kumar N. (2019). Microbial degradation of phenol: A review. J. Water pollut. Purif. Res. 4, 17–22. doi: 10.37591/jowppr.v4i1.465
Mok W. Y., Luizão R. C., do Socorro Barreto da Silva M., Teixeira M. F., Muniz E. G. (1984). Ecology of pathogenic yeasts in Amazonian soil. Appl. Environ. Microbiol. 47, 390–394. doi: 10.1128/aem.47.2.390-394.1984
Montaño M., Gutleb A. C., Murk A. J. (2013). Persistent toxic burdens of halogenated phenolic compounds in humans and wildlife. Environ. Sci. Technol. 47, 6071–6081. doi: 10.1021/es400478k
Morio F., Loge C., Besse B., Hennequin C., Le Pape P. (2010). Screening for amino acid substitutions in the candida albicans Erg11 protein of azole-susceptible and azole-resistant clinical isolates: new substitutions and a review of the literature. Diagn. Microbiol. Infect. Dis. 66, 373–384. doi: 10.1016/j.diagmicrobio.2009.11.006
Muñoz P., Giannella M., Fanciulli C., Guinea J., Valerio M., Rojas L., et al. (2011). Candida tropicalis fungaemia: incidence, risk factors and mortality in a general hospital. Clin. Microbiol. Infect. 17, 1538–1545. doi: 10.1111/j.1469-0691.2010.03338.x
Mushi M. F., Mmole A., Mshana S. E. (2019). Candida vaginitis among symptomatic pregnant women attending antenatal clinics in mwanza, Tanzania. BMC Res. Notes 12, 775. doi: 10.1186/s13104-019-4793-z
Ngamchokwathana C., Chongtrakool P., Waesamaae A., Chayakulkeeree M. (2021). Risk factors and outcomes of non-albicans candida bloodstream infection in patients with candidemia at siriraj hospital-thailand’s largest national tertiary referral hospital. J. Fungi (Basel) 7, 269. doi: 10.3390/jof7040269
Nucci M., Colombo A. L. (2007). Candidemia due to candida tropicalis: clinical, epidemiologic, and microbiologic characteristics of 188 episodes occurring in tertiary care hospitals. Diagn. Microbiol. Infect. Dis. 58, 77–82. doi: 10.1016/j.diagmicrobio.2006.11.009
Nucci M., Queiroz-Telles F., Alvarado-Matute T., Tiraboschi I. N., Cortes J., Zurita J., et al. (2013). Epidemiology of candidemia in Latin America: a laboratory-based survey. PloS One 8, e59373. doi: 10.1371/journal.pone.0059373
O’Brien C. E., Oliveira-Pacheco J., Ó Cinnéide E., Haase M. A. B., Hittinger C. T., Rogers T. R., et al. (2021). Population genomics of the pathogenic yeast candida tropicalis identifies hybrid isolates in environmental samples. PloS Pathog. 17, e1009138. doi: 10.1371/journal.ppat.1009138
Ohno Y., Akune Y., Inoshima Y., Kano R. (2019). First isolation of voriconazole-resistant candida albicans, c. tropicalis, and aspergillus niger from the blowholes of bottlenose dolphins (Tursiops truncatus). J. Vet. Med. Sci. 81, 1628–1631. doi: 10.1292/jvms.18-0749
Ozawa H., Okabayashi K., Kano R., Watari T., Watanabe S., Hasegawa A. (2005). Rapid identification of candida tropicalis from canine cystitis. Mycopathologia 160, 159–162. doi: 10.1007/s11046-005-1151-6
Páca J., Páca J., Kostecková A., Stiborová M., Sobotka M., Gerrard A. M., et al. (2005). Continuous aerobic phenol degradation by defined mixed immobilized culture in packed bed reactors. Folia Microbiol. (Praha) 50, 301–308. doi: 10.1007/BF02931410
Perlin D. S., Rautemaa-Richardson R., Alastruey-Izquierdo A. (2017). The global problem of antifungal resistance: prevalence, mechanisms, and management. Lancet Infect. Dis. 17, e383–e392. doi: 10.1016/S1473-3099(17)30316-X
Pfaller M. A., Diekema D. J., Turnidge J. D., Castanheira M., Jones R. N. (2019). Twenty years of the SENTRY antifungal surveillance program: Results for candida species from 1997-2016. Open Forum Infect. Dis. 6, S79–S94. doi: 10.1093/ofid/ofy358
Pitarch A., Gil C., Blanco G. (2020). Vultures from different trophic guilds show distinct oral pathogenic yeast signatures and co-occurrence networks. Sci. Total Environ. 723, 138166. doi: 10.1016/j.scitotenv.2020.138166
Porman A. M., Alby K., Hirakawa M. P., Bennett R. J. (2011). Discovery of a phenotypic switch regulating sexual mating in the opportunistic fungal pathogen candida tropicalis. Proc. Natl. Acad. Sci. U.S.A. 108, 21158–21163. doi: 10.1073/pnas.1112076109
Reis E. O., Foureaux A. F. S., Rodrigues J. S., Moreira V. R., Lebron Y. A. R., Santos L. V. S., et al. (2019). Occurrence, removal and seasonal variation of pharmaceuticals in brasilian drinking water treatment plants. Environ. pollut. 250, 773–781. doi: 10.1016/j.envpol.2019.04.102
Ribeiro Bastos A. E., Moon D. H., Rossi A., Trevors J. T., Tsai S. M. (2000). Salt-tolerant phenol-degrading microorganisms isolated from Amazonian soil samples. Arch. Microbiol. 174, 346–352. doi: 10.1007/s002030000216
Robbins N., Caplan T., Cowen L. E. (2017). Molecular evolution of antifungal drug resistance. Annu. Rev. Microbiol. 71, 753–775. doi: 10.1146/annurev-micro-030117-020345
Rosa R. H., Miller D., Alfonso E. C. (1994). The changing spectrum of fungal keratitis in south Florida. Ophthalmology 101, 1005–1013. doi: 10.1016/s0161-6420(94)31225-5
Rosenberg A., Ene I. V., Bibi M., Zakin S., Segal E. S., Ziv N., et al. (2018). Antifungal tolerance is a subpopulation effect distinct from resistance and is associated with persistent candidemia. Nat. Commun. 9, 2470. doi: 10.1038/s41467-018-04926-x
Rueda C., Puig-Asensio M., Guinea J., Almirante B., Cuenca-Estrella M., Zaragoza O., et al. (2017). Evaluation of the possible influence of trailing and paradoxical effects on the clinical outcome of patients with candidemia. Clin. Microbiol. Infect. 23, 49.e1–49.e8. doi: 10.1016/j.cmi.2016.09.016
Sabz G., Gharaghani M., Mirhendi H., Ahmadi B., Gatee M. A., Sisakht M. T., et al. (2019). Clinical and microbial epidemiology of otomycosis in the city of yasuj, southwest Iran, revealing aspergillus tubingensis as the dominant causative agent. J. Med. Microbiol. 68, 585–590. doi: 10.1099/jmm.0.000948
Sakamoto Y., Kawabe K., Suzuki T., Sano K., Ide K., Nishigaki T., et al. (2021). Species distribution of candidemia and their susceptibility in a single Japanese university hospital: Prior micafungin use affects the appearance of candida parapsilosis and elevation of micafungin MICs in non-parapsilosis candida species. J. Fungi (Basel) 7, 596. doi: 10.3390/jof7080596
Salehi M., Ahmadikia K., Mahmoudi S., Kalantari S., Jamalimoghadamsiahkali S., Izadi A., et al. (2020). Oropharyngeal candidiasis in hospitalised COVID-19 patients from Iran: Species identification and antifungal susceptibility pattern. Mycoses 63, 771–778. doi: 10.1111/myc.13137
Sanchez A., Lee B., Gandhi K., Garza J., Ventolini G. (2021). A rare case report: Cutaneous candida tropicalis infection disguised as a sacral decubitus rash in an immunocompetent individual. J. Infect. Public Health 14, 1018–1020. doi: 10.1016/j.jiph.2021.05.013
Sanglard D. (2016). Emerging threats in antifungal-resistant fungal pathogens. Front. Med. (Lausanne) 3. doi: 10.3389/fmed.2016.00011
Sasani E., Yadegari M. H., Khodavaisy S., Rezaie S., Salehi M., Getso M. I. (2021). Virulence factors and azole-resistant mechanism of candida tropicalis isolated from candidemia. Mycopathologia 186, 847–856. doi: 10.1007/s11046-021-00580-y
Seker E. (2010). Identification of candida species isolated from bovine mastitic milk and their in vitro hemolytic activity in Western Turkey. Mycopathologia 169, 303–308. doi: 10.1007/s11046-009-9255-z
Sellami A., Sellami H., Néji S., Makni F., Abbes S., Cheikhrouhou F., et al. (2011). Antifungal susceptibility of bloodstream candida isolates in sfax hospital: Tunisia. Mycopathologia 171, 417–422. doi: 10.1007/s11046-010-9388-0
Sfeir M. M., Jiménez-Ortigosa C., Gamaletsou M. N., Schuetz A. N., Soave R., Van Besien K., et al. (2020). Breakthrough bloodstream infections caused by echinocandin-resistant candida tropicalis: An emerging threat to immunocompromised patients with hematological malignancies. J. Fungi (Basel) 6, 20. doi: 10.3390/jof6010020
Shokri H., Khosravi A., Sharifzadeh A., Tootian Z. (2010). Isolation and identification of yeast flora from genital tract in healthy female camels (Camelus dromedarius). Vet. Microbiol. 144, 183–186. doi: 10.1016/j.vetmic.2009.12.012
Sidrim J. J. C., de Maria G. L., Paiva M., de A. N., Araújo G. D. S., da Graça-Filho R. V., et al. (2021). Azole-resilient biofilms and non-wild type c. albicans among candida species isolated from agricultural soils cultivated with azole fungicides: an environmental issue? Microb. Ecol. 82, 1080–1083. doi: 10.1007/s00248-021-01694-y
Silva S., Negri M., Henriques M., Oliveira R., Williams D. W., Azeredo J. (2012). Candida glabrata, candida parapsilosis and Candida tropicalis;: biology, epidemiology, pathogenicity and antifungal resistance. FEMS Microbiol. Rev. 36, 288–305. doi: 10.1111/j.1574-6976.2011.00278.x
Soll D. R. (2014). The evolution of alternative biofilms in an opportunistic fungal pathogen: An explanation for how new signal transduction pathways may evolve. Infect. Genet. Evol. 22, 235–243. doi: 10.1016/j.meegid.2013.07.013
Staniszewska M. (2020). Virulence factors in candida species. CPPS 21, 313–323. doi: 10.2174/1389203720666190722152415
Subramanya S. H., Sharan N. K., Baral B. P., Hamal D., Nayak N., Prakash P. Y., et al. (2017). Diversity, in-vitro virulence traits and antifungal susceptibility pattern of gastrointestinal yeast flora of healthy poultry, gallus gallus domesticus. BMC Microbiol. 17, 113. doi: 10.1186/s12866-017-1024-4
Takahashi H., Ueda K., Itano E. N., Yanagisawa M., Murata Y., Murata M., et al. (2010). Candida albicans and c. tropicalis isolates from the expired breathes of captive dolphins and their environments in an aquarium. Vet. Med. Int. 2010, 349364. doi: 10.4061/2010/349364
Tan T. Y., Hsu L. Y., Alejandria M. M., Chaiwarith R., Chinniah T., Chayakulkeeree M., et al. (2016). Antifungal susceptibility of invasive candida bloodstream isolates from the Asia-pacific region. Med. Mycol 54, 471–477. doi: 10.1093/mmy/myv114
Tan J., Zhang J., Chen W., Sun Y., Wan Z., Li R., et al. (2015). The A395T mutation in ERG11 gene confers fluconazole resistance in candida tropicalis causing candidemia. Mycopathologia 179, 213–218. doi: 10.1007/s11046-014-9831-8
Teo J. Q.-M., Candra S. R., Lee S. J.-Y., Chia S. Y.-H., Leck H., Tan A.-L., et al. (2017). Candidemia in a major regional tertiary referral hospital - epidemiology, practice patterns and outcomes. Antimicrob. Resist. Infect. Control 6, 27. doi: 10.1186/s13756-017-0184-1
Teo J. Q.-M., Lee S. J.-Y., Tan A.-L., Lim R. S.-M., Cai Y., Lim T.-P., et al. (2019). Molecular mechanisms of azole resistance in candida bloodstream isolates. BMC Infect. Dis. 19, 63. doi: 10.1186/s12879-019-3672-5
Togni G., Sanglard D., Quadroni M., Foundling S. I., Monod M. (1996). Acid proteinase secreted by candida tropicalis: Functional analysis of preproregion cleavages in c. tropicalis and saccharomyces cerevisiae. Microbiology 142, 493–503. doi: 10.1099/13500872-142-3-493
Tokuoka K. (1993). Sugar- and salt-tolerant yeasts. J. Appl. Bacteriol. 74, 101–110. doi: 10.1111/j.1365-2672.1993.tb03002.x
Tulyaprawat O., Pharkjaksu S., Chongtrakool P., Ngamskulrungroj P. (2020). An association of an eBURST group with triazole resistance of candida tropicalis blood isolates. Front. Microbiol. 11. doi: 10.3389/fmicb.2020.00934
Wang D., An N., Yang Y., Yang X., Fan Y., Feng J. (2021). Candida tropicalis distribution and drug resistance is correlated with ERG11 and UPC2 expression. Antimicrob. Resist. Infect. Control 10, 54. doi: 10.1186/s13756-021-00890-2
Wang L., Lin Y., Yang L., Yu P., Xie Z., Luo Y. (2011). Candida tropicalis: characterization of a strain capable of degrading high concentrations of phenol. Biotechnol. Lett. 33, 943–946. doi: 10.1007/s10529-011-0534-7
Wang Q., Tang D., Tang K., Guo J., Huang Y., Li C. (2020). Multilocus sequence typing reveals clonality of fluconazole-nonsusceptible candida tropicalis: A study from wuhan to the global. Front. Microbiol. 11. doi: 10.3389/fmicb.2020.554249
Wang H., Xu Y.-C., Hsueh P.-R. (2016). Epidemiology of candidemia and antifungal susceptibility in invasive candida species in the Asia-pacific region. Future Microbiol. 11, 1461–1477. doi: 10.2217/fmb-2016-0099
Xiao M., Fan X., Hou X., Chen S. C., Wang H., Kong F., et al. (2018a). Clinical characteristics of the first cases of invasive candidiasis in China due to pan-echinocandin-resistant candida tropicalis and candida glabrata isolates with delineation of their resistance mechanisms. Infect. Drug Resist. 11, 155–161. doi: 10.2147/IDR.S152785
Xiao M., Fan X., Hou X., Chen S. C., Wang H., Kong F., et al. (2018b). Clinical characteristics of the first cases of invasive candidiasis in China due to pan-echinocandin-resistant candida tropicalis and candida glabrata isolates with delineation of their resistance mechanisms. Infect. Drug Resist. 11, 155–161. doi: 10.2147/IDR.S152785
Xisto M. I. D. S., Caramalho R. D. F., Rocha D. A. S., Ferreira-Pereira A., Sartori B., Barreto-Bergter E., et al. (2017). Pan-azole-resistant candida tropicalis carrying homozygous erg11 mutations at position K143R: a new emerging superbug? J. Antimicrob. Chemother. 72, 988–992. doi: 10.1093/jac/dkw558
Yang Y.-L., Ho Y.-A., Cheng H.-H., Ho M., Lo H.-J. (2004). Susceptibilities of candida species to amphotericin b and fluconazole: the emergence of fluconazole resistance in candida tropicalis. Infect. Control Hosp. Epidemiol. 25, 60–64. doi: 10.1086/502294
Yang Y.-L., Lin C.-C., Chang T.-P., Lauderdale T.-L., Chen H.-T., Lee C.-F., et al. (2012). Comparison of human and soil candida tropicalis isolates with reduced susceptibility to fluconazole. PloS One 7, e34609. doi: 10.1371/journal.pone.0034609
You L., Qian W., Yang Q., Mao L., Zhu L., Huang X., et al. (2017a). ERG11 gene mutations and MDR1 upregulation confer pan-azole resistance in candida tropicalis causing disseminated candidiasis in an acute lymphoblastic leukemia patient on posaconazole prophylaxis. Antimicrob. Agents Chemother. 61, e02496–e02416. doi: 10.1128/AAC.02496-16
You L., Qian W., Yang Q., Mao L., Zhu L., Huang X., et al. (2017b). ERG11 gene mutations and MDR1 upregulation confer pan-azole resistance in candida tropicalis causing disseminated candidiasis in an acute lymphoblastic leukemia patient on posaconazole prophylaxis. Antimicrob. Agents Chemother. 61, e02496–e02416. doi: 10.1128/AAC.02496-16
Zaugg C., Borg-von Zepelin M., Reichard U., Sanglard D., Monod M. (2001). Secreted aspartic proteinase family of Candida tropicalis. Infect. Immun. 69, 405–412. doi: 10.1128/IAI.69.1.405-412.2001
Zhai L., Zhou Y., Wu Y., Jin Y., Zhu Q., Gao S., et al. (2021). Isolation and identification of candida tropicalis in sows with fatal infection: a case report. BMC Vet. Res. 17, 108. doi: 10.1186/s12917-021-02821-0
Zhang H., Tan J., Kontoyiannis D. P., Zhou Y., Liu W., Zhu P., et al. (2019). Screening the in vitro susceptibility of posaconazole in clinical isolates of candida spp. and aspergillus spp. and analyzing the sequence of ERG11 or CYP51A in non-wild-type isolates from China. Diagn. Microbiol. Infect. Dis. 95, 166–170. doi: 10.1016/j.diagmicrobio.2019.05.003
Zheng Q., Zhang Q., Bing J., Ding X., Huang G. (2017). Environmental and genetic regulation of white-opaque switching in Candida tropicalis: Regulation of phenotypic switching in c. tropicalis. Mol. Microbiol. 106, 999–1017. doi: 10.1111/mmi.13862
Zuza-Alves D. L., de Medeiros S. S. T. Q., de Souza L. B. F. C., Silva-Rocha W. P., Francisco E. C., de Araújo M. C. B., et al. (2016a). Evaluation of virulence factors In vitro, resistance to osmotic stress and antifungal susceptibility of candida tropicalis isolated from the coastal environment of northeast Brazil. Front. Microbiol. 7. doi: 10.3389/fmicb.2016.01783
Zuza-Alves D. L., de Medeiros S. S. T. Q., de Souza L. B. F. C., Silva-Rocha W. P., Francisco E. C., de Araújo M. C. B., et al. (2016b). Evaluation of virulence factors In vitro, resistance to osmotic stress and antifungal susceptibility of candida tropicalis isolated from the coastal environment of northeast Brazil. Front. Microbiol. 7. doi: 10.3389/fmicb.2016.01783
Zuza-Alves D. L., Silva-Rocha W. P., Chaves G. M. (2017). An update on candida tropicalis based on basic and clinical approaches. Front. Microbiol. 8. doi: 10.3389/fmicb.2017.01927
Zuza-Alves D. L., Silva-Rocha W. P., Francisco E. C., de Araújo M. C. B., de Azevedo Melo A. S., Chaves G. M. (2019). Candida tropicalis geographic population structure maintenance and dispersion in the coastal environment may be influenced by the climatic season and anthropogenic action. Microb. Pathog. 128, 63–68. doi: 10.1016/j.micpath.2018.12.018
Keywords: Candida tropicalis, animals, humans, environment, resistance
Citation: Lima R, Ribeiro FC, Colombo AL and de Almeida JN Jr (2022) The emerging threat antifungal-resistant Candida tropicalis in humans, animals, and environment. Front. Fungal Biol. 3:957021. doi: 10.3389/ffunb.2022.957021
Received: 30 May 2022; Accepted: 28 July 2022;
Published: 15 August 2022.
Edited by:
Miguel Cacho Teixeira, University of Lisbon, PortugalReviewed by:
Shreya Singh, Dr B R Ambedkar Institute of Medical Sciences, IndiaCopyright © 2022 Lima, Ribeiro, Colombo and de Almeida. This is an open-access article distributed under the terms of the Creative Commons Attribution License (CC BY). The use, distribution or reproduction in other forums is permitted, provided the original author(s) and the copyright owner(s) are credited and that the original publication in this journal is cited, in accordance with accepted academic practice. No use, distribution or reproduction is permitted which does not comply with these terms.
*Correspondence: Joăo N. de Almeida Jr, am9hby5ub2JyZWdhYUBlaW5zdGVpbi5icg==; am5hajk5QGdtYWlsLmNvbQ==