Fusarium verticillioides of maize plant: Potentials of propitious phytomicrobiome as biocontrol agents
- Food Security and Safety Focus Area, Faculty of Natural and Agricultural Science, North-West University, Mmabatho, South Africa
Disease outbreaks have been recorded due to exposure to Fusarium verticillioides and fumonisin, a mycotoxin produced by this fungus. F. verticillioides is a fungal pathogen of maize that causes infections, such as wilting and rotting, while contact with its fumonisin derivative manifests in the form of mild to severe illnesses in humans and animals. Maize infection by F. verticillioides causes loss or reduction in expected crop yield, thereby influencing households and nations’ economies. While several efforts have been made to control the pathogenic fungus and its occurrence in the environment, it remains a challenge in agriculture, particularly in maize production. Several microorganisms which are plant-associated, especially those associated with the rhizosphere niche have been noted to possess antagonistic effects against F. verticillioides. They can inhibit the pathogen and tackle its debilitating effects on plants. Hence this study reviews the use of rhizosphere-associated biocontrol agents, such as Bacillus spp., Pseudomonas, Enterobacter, and Microbacterium oleivorans which forms part of the phytomicrobiome in other to prevent and control this toxicogenic fungus. These microorganisms were found to not only be effective in controlling its occurrence on maize plants but are environmentally safe and promote crop yield.
1 Introduction
Fusarium verticillioides remains a major threatening pathogen in agriculture, particularly in maize production. This toxicogenic fungus possesses the ability to survive under extreme conditions, such as high temperatures (Czembor et al., 2015), and produces airborne spores, which explains its wide occurrence in the environment. It is a soil-borne pathogen, a part of the microbial community in the maize rhizosphere, which can contaminate the growing seed and roots of the maize plant, after which it moves up the plant itself (1).
It infects maize plants through contaminated seeds and contact of its contagious spores with the maize silk, while the invasion of maize kernels happens through cracks in the pericarp and the pedicel (Headrick and Pataky, 1991; Munkvold and Carlton, 1997; Munkvold et al., 1997; Oren et al., 2003; Ncube et al., 2017). The fungus can migrate to the soil through infected stalks or seeds (Oren et al., 2003; Ncube et al., 2017), with infection of maize by this pathogen being enhanced by late-season rainfall and the physiological state of the silk after pollination (Ncube et al., 2017).
F. verticillioides, which belongs to the fungi kingdom and Nectriaceae family, evolved approximately 7.3 million years ago following the divergence of the African and Asian clades of the Fusarium fujikuroi species complex (1). The fungus has been classified as part of the African clade, which produces a wide range of secondary metabolites (1). F. verticillioides is mainly associated with maize (1), where it colonizes the root, stem, and ears, resulting in diseases such as Fusarium ear rot, stalk rot, or even loss of the plant. According to Leslie et al. (1990), the fungus exists in crop residues in the soil, thus accounting for its occurrence in planted maize. It is a major pathogen and can be present in this crop without the plant showing any symptoms, its occurrence poses a severe risk due to its production of fumonisin (a kind of mycotoxin), which occurs frequently, and sometimes in high concentration. It also poses a major threat to the health of consumers (humans and animals), as it has been associated with illnesses such as esophageal cancer in humans (Shephard, 2011), and leukoencephalomalacia in equines and rabbits (Marasas, 2001; Giannitti et al., 2011).
The international agency for cancer research classified fumonisin as a possible human carcinogen (Humans IWGotEoCRt Cancer IAfRo, 2002), making the prevention of its occurrence in food advisable, this being more achievable (Omotayo et al., 2019) than its elimination. Several strategies (mechanical, physical, chemical) have been used to control the occurrence of various fungi and their derivatives in plants and foods, including the use of chemical disinfectants, such as sodium hypochlorite and chlorine dioxide. According to Chepkirui (2013), preventing fungi contamination in the field is regarded as a more effective method than post-harvest eradication. Several biological agents have been studied and proposed, being regarded as more promising and safer for plants, soil microorganisms, and the environment (Babalola and Glick, 2012).
The occurrence of this fungus in the maize rhizosphere ecosystem and the ubiquity of its fumonisin derivative, as well as their impact on the environment and plants, is hereby reviewed. This review also emphasizes the intensification of biological control agents for addressing F. verticilloides’ occurrence before maize cultivation and during growth instead of chemical pesticides. The introduction of these in the field can serve as a control strategy, which can result in maximum yield and production of quality maize with little or no toxins (1). It will also reduce the airborne spores from the environment and hence minimize the risk of contamination (Colmenarez et al., 2018).
2 Method of literature search
A literature search on Fusarium verticillioides and fumonisin, their effects, as well as biological agents capable of inhibiting these were conducted on materials published from 1968 to 2022 through scholarly search engines such as Scopus, PubMed, Web of Science, Google Scholar, and Science Direct. The keywords of this article (Biocontrol agents, Environmental pollution, Fusarium verticillioides, Maize pathogen, Maize rhizosphere, and Fumonisin) were majorly used for the search, with 325 articles being downloaded for review. A purposive selection of the articles was carried out yielding 125 articles (38% of the total downloaded articles) that were included in this review (Figure 1).
3 The impacts of F. verticillioides on plant, animal, and human health
F. verticillioides is a mycotoxigenic pathogen of maize that belongs to the fungi kingdom and the largest phylum of fungi, known as Ascomycota. The spores produced by this fungus contaminates the environment and poses a serious threat to human and animals. It was identified to be responsible for the Equine leukoencephalomalacia (ELEM) outbreak in South Africa in 1970 (Marasas et al., 1976; Omotayo et al., 2022). The pathogen was later found to be a causing agent of liver toxicity in rats and pulmonary edema in pigs (Kriek et al., 1981). In the United States of America (2), F. verticilloides was recorded to cause an outbreak of ELEM in 1989 (Marasas, 2001). The occurrence of this fungus in consumed maize has also been linked to the induction of proliferative hepatocyte lesions and tumors of the bile ducts in horses (Thiel et al., 1991; Alamu et al., 2016). In Argentina, an ELEM outbreak was recorded in 1945, followed by China in 1957, Egypt in 1968, South Africa in 1970, and Australia in 1999 after the consumption of contaminated maize. Table 1 shows other effects and consequences of F. verticillioides and fumonisin on plants and animals.
4 Infection routes for F. verticillioides and suitable environmental conditions for fumonisin production
In the field, F. verticillioides infects the maize plant, causing the production of fumonisin in the maize crop (Fandohan et al., 2006; Matić et al., 2013; Burger et al., 2013), which is often more concentrated in the embryo and pericarp of the maize kernel (Kimanya et al., 2008; Burger et al., 2013; Czembor et al., 2015). The growth of this fungus and fumonisin are favored by various factors such as high temperature (30-35°C) and the composition of kernel endosperm (Chulze, 2010; Picot et al., 2011; Alberts et al., 2016). These factors result in the fungi occurring in temperate and Mediterranean climate regions as the weather conditions are favorable to toxin accumulation in maize plants during their growth and development, particularly at the flowering stage (Kamle et al., 2019). Apart from higher temperatures, growing hybrids outside their adaptation areas, drought stress, and insect herbivory can also lead to infection by this pathogen (Betz et al., 2000; Alberts et al., 2016; Li et al., 2017). When comparing the fumonisin content in maize from different zones in Nigeria, Fandohan, et al. (Fandohan et al., 2005) observed that maize from regions with higher humidity levels has more fumonisin concentration than others. This study agrees with the findings of Mupunga (2013), on the correlation between fumonisin and high humidity.
The fungi gain access to the maize kernels commonly through wounds caused by insects such as the European corn borer, earthworms, and birds, and can also penetrate the maize through the silk by growing through the silk channel to the ear tip (Scarpino et al., 2015; Oldenburg et al., 2017). After harvest, some factors have been shown to contribute to fumonisin production in foods, these include high humidity, poor storage facilities, improper sorting, and unhygienic environments (Kamala et al., 2016; Moss and Frank, 1985). Furthermore, poor storage facilities prone to insect infestation and high moisture could lead to the production and contamination of foods by fumonisin. When sorting (which involves the removal of damaged, discolored, and molded grains) is not carefully done before storage, contamination with this pathogen might occur. It has been reported that insects like the European corn borer make a wound on the maize kernels which could create an avenue for infection by these fungi (Scarpino et al., 2015; Oldenburg et al., 2017) thereby causing fumonisin contamination.
4.1 Infection mechanisms of F. Verticillioides
Insects such as the European corn borer and western corn rootworm play a major role in F. verticillioides’ infection and cycle as they feed on maize ears and stalk respectively (Munkvold and Carlton, 1997; Oldenburg et al., 2017). Wounds created on the stalk by the western corn borer become infection routes/sites for F. verticillioides stalk rot under dry conditions (Figure 2). Likewise, the egg deposited by the European corn borer moth on the leaves surface develops into a larva and thus feeds on leaf tissues and stalks thereby providing infection courts for the development of F. verticillioides stalk and ear rots (Blacutt et al., 2018). These larvae proliferate and deposits another set of eggs across the plant tissues (Sobek and Munkvold, 1999) which develops into Larva and creates more route for F. verticillioides through wounds created on stalks.
Also, seeds planted in soils infected with F. verticillioides can become contaminated with the pathogen thus causing infection of the whole plant (Sobek and Munkvold, 1999) (Figures 2, 3). According to Blacutt et al. (2018), and Oren et al., (1), under favorable conditions for the seedlings, the fungus grows in a hyphal manner and progressed along the root surface, sparsely penetrating the lateral roots and mesocotyl about 72 hours after planting into infested soils. Thereafter, it progresses into the mesocotyl tissue within seven days, and in about fourteen days, it grows into aerial tissues although in an asymptomatic manner and undetectable by fluorescence microscopy. According to Blacutt et al. (2018), at this stage, the mesocotyl cells accumulate undefined organelles and are filled with conidia (which is essential for the systemic movement of F. verticillioides within the plant). After 30 days, according to the study of Oren et al. (1), the fungal biomass increase greatly resulting into necrosis of the mesocotyl and main root.
From the study of Duncan and Howard (2010), microscopic and histologic characterization at the silking stage showed that once the fungus has gained access to the maize plant, it populates young developing kernels through the stylar canal resulting in starburst pattern which is commonly seen/observed in crops like maize. Other insects important in the infection of F. verticillioides are the adult thrip and beetle which causes wound on maize ears thus promoting F. verticillioides infection. Through existing openings such as cracks in roots, lateral roots, and root hairs F. verticillioides can penetrate (1). Leaf stomata or trichrome can also serve as a route of infection of maize by F. verticillioides hyphae (Nguyen et al., 2016).
4.2 Pathogenicity of F. verticillioides
F. verticillioides can be a facultative endophyte that can exist by depending on the maize plant for survival without harming the host (biotrophically), as well as by relying on the nutrients from decayed maize rubble or soil (saprotrophically) (Samsudin, 2015). As an endophyte, it can be vertically transmitted through maize seeds, thus initiating asymptomatic and systemic infection of the plant. During systemic infection, the fungal conidia are carried inside the seeds or the surface of the seeds and later develop inside the growing maize plant. The developed F. verticillioides thereafter moves from the roots to the stalk, cobs, and kernels. The veins of the maize root can also be infested by some conidia and also grow along the root veins after germination, while some will infiltrate the plant cells, attach to them and form hyphae in other to penetrate the neighboring cells (Samsudin, 2015).
As a pathogen, in maize, F. verticillioides causes severe symptomatic infections such as root rot, stalk rot, seedling blight, and ear rot (Bacon et al., 2008). According to Samsudin (2015), this fungus moves from the contaminated soil upwards to the maize stalks, thus resulting in stalk rot which is presented by the rotting of the lower stems, maize internodes, and roots. Moreover, it can survive through the winter as viable spores and becomes airborne or splashed by rainfall, thus causing infection of the silks during flowering. Diseases caused by F. verticillioides like ear rot in maize (which is the most damaging and common fungal-borne disease in maize worldwide) decrease quality, and yield and make the maize kernel vulnerable to fumonisin contamination (Khan et al., 2020). Consumption of maize polluted by this mycotoxin causes severe diseases in humans and animal (Blacutt et al., 2018).
5 Overview of F. verticillioides and fumonisin
The invasion and occurrence of F. verticillioides in maize have continued to create persistent setbacks in its production, with several approaches having been used to address its presence, one being chemicals. According to the literature, some chemicals including captan + thiabendazole and fludioxonil + metalaxyl - M have been used to control the pathogen, but the possible shortcomings and deleterious effects on humans, animals, and other beneficial microbes in the soil is a challenge to their use (Nicolopoulou-Stamati et al., 2016). For example, it has been reported that despite the ability of fungicides to suppress fungal activity and increase the growth and production of maize, it does not have a significant effect on mycotoxins reduction, including fumonisin, which is a derivative of these fungi but rather increases their levels (Bordini et al., 2015). Likewise, Tridemorph was found to increase the production of T-2 toxin, although it inhibited the growth of Fusarium sporotrichioides (1). Moreover, the use of Prochloraz and tebuconazole was observed to promote the biosynthesis of trichothecenes (Doohan et al., 1999), with Trans-2-hexenal being found to be effective in controlling F. verticillioides, but does not halt the production of fumonisin (Menniti et al., 2010). Fungicides are also limited in eradicating F. verticillioides, as they are not effective in the long term to have made a significant impact (Bordini et al., 2015).
The consequences of fumonisin (produced by these fungi) on agricultural production globally and human health cannot be overemphasized. The presence of this mycotoxin in plants results in damage and loss in crop and livestock production while its infiltration into the food system may cause significant havoc to the lives of humans and animals (Omotayo et al., 2019). The effect of fumonisin on plants is not only limited to damage and loss but also affects the yield and quality of the crop (Omotayo et al., 2019). This mycotoxin is capable of attacking crops at any developmental stage and causing various plant diseases before and after harvest (Iqbal et al., 2021). Upon invasion of the human body through the food system, fumonisin suppresses the immune system and reduces the human ability to resist environmental and microbial stress thus increasing its susceptibility to diverse diseases (Mita et al., 2022). While it is classified into three forms: FB1, FB2, and FB3, FB1 is the most abundant, prevalent, and toxic, and poses the greatest threat to plant, animal, and human health. It stresses the endoplasmic reticulum and causes cellular autophagy, cytotoxicity, oxidative stress, apoptosis, DNA damage, and consequently cell carcinogenesis. Following the alteration of several components of the human cells, tissues, and organs, it exerts hepatotoxic, hematopoietic, and other toxic effects on the human body and also induces pathological changes (Blacutt et al., 2018) (Figure 2).
The occurrence of F. verticillioides in maize is fast becoming a challenge that cannot be easily resolved, leading to problems in maize production. In the quest for lasting preventive measures and a remedy to the consequences of these fungi on maize production, the use of biological agents has been examined. It was found that some organisms are capable of limiting the activity of this pathogen (Khan et al., 2017), thereby limiting/reducing its negative effect (Bressan and Figueiredo, 2005). It was also noted that biological agents are friendly to the atmosphere (Thavaselvam and Vijayaraghavan, 2010) and are also target-specific, as they only attack the pathogen (Usta, 2013). The rhizosphere has been noted to host some of these organisms, which can be enhanced to control F. verticillioides, some of these include members of the genera Bacillus, Enterobacter, and Pseudomonas.
6 Mode of action of F. verticillioides and fumonisin
The effects of biotic and abiotic factors such as plant genetics, high temperature, and low humidity results in the production of fumonisin from F. verticillioides. Fumonisins (FB1 and FB2) are structural analogs of sphingoid bases and they inhibit ceramide synthases (which acylate sphingoid bases) and sphingosine-sphinganine-transferases leading to the interference of the sphingolipid metabolism and accumulation of sphinganine and sphingosine in cells and tissues. Inhibition of ceramide synthases results in the production of reactive oxygen species which increases oxidative stress and causes the induction of lipid peroxidation and cell damage. Sphinganine and sphingosine-induced apoptosis, exert growth inhibitory effects and the accumulation of these two (sphingoid bases) is the primary cause of fumonisin toxicity (especially FB1). Figure 4 summarizes the mode of action of fumonisin.
7 Role of microbial inoculants in the management and control of fumonisin
While the complete eradication of mycotoxins including fumonisin remains a challenge, biological measures can be taken to prevent their invasion, control their spread and manage their effects. This involves the introduction of microbes such as Trichoderma harzianum and Ruminococcus flavefaciens (1). Trichoderma harzianum is a fungus present in the soil and commonly used in foliar preparation for animals, thus leading to the presence of these microorganisms in the gut of ruminant animals. Studies have shown that this fungus not only resists F. verticillioides but also can be used in controlling fumonisin. The capability of ruminant animals such as cows to resist the effects of various mycotoxins consumed in forages, and their ability to modify and degrade toxic substances is evidence of the potential of this cellulolytic microorganism in mitigating the effect of fumonisin (Rodrigues, 2014). Hence, the application of this fungus as microbial inoculants to plants would help to modify and degrade fumonisin thus preventing its invasion of crops. From the study of Bakri (2021) which evaluated the effect of T. harzianum and other cellulolytic enzymes on divers mycotoxin, it was found that this fungus together with Lactobacillus bulgaricus greatly inhibited the production of fumonisin and other mycotoxins.
Ruminococcus flavefaciens is another gut microbe that can be isolated and optimized for the control of fumonisin. As shown in previous studies, this microorganism is capable of modifying mycotoxin’s toxicity by causing changes to its toxicokinetics (Aisha, 2012; Guerre, 2020). That is, this microbe can cause the chemical transformation of fumonisin through the enzymes present in its cell or through the enzymes excreted by the microorganism into the gut. This process leads to the hydrolysis of fumonisin and the formation of less toxic metabolites. The cell wall of this microbe also can bind fumonisin and alter/reduce its absorption (Guerre, 2020).
Bacillus subtilis CE1 is not only capable of inhibiting F. verticillioides but reduces fumonisin accumulation (Mita et al., 2022),. This bacteria which is commonly found in the maize rhizosphere is one of the numerous microbes which hinder the production and buildup of the mycotoxin derivative-fumonisin. Collection of fumonisin in plants is hazardous not only to the plant but the environment, however, B. Subtilis would help in preventing fumonisin contamination in plants, thus making them free from this hazardous mycotoxin.
8 General effects of F. verticillioides contamination
Apart from the effects of these disease-causing pathogens on the maize plant, it also impacts food production, the environment, and the economy negatively, thereby causing compromise of maize quality, food, and environmental pollution, which further results in disease outbreaks and health complications.
8.1 Food and environmental pollution
Cereals such as wheat, barley, millet, rye, oat, and most frequently maize are the most commonly infected food groups by F. verticilloides, although garlic, asparagus, and dried figs can be contaminated by its fumonisin derivative (Heperkan et al., 2012; Kamle et al., 2019). In the study conducted to identify the most prevalent Fusarium species in an environment, Czembor, (Czembor et al., 2015) found that F. verticillioides and Fusarium temperatum were the most prevalent in several maize samples. Likewise, Borah (Borah et al., 2016), identified F. verticillioides to be the most prevalent fungi causing an infection on maize cultivated in a region in Assam.
Due to the production and spread of airborne spores by F. verticillioides, exposed food products may become contaminated by contact, the inhalation of which can result in various illnesses in animals and humans. According to the study of Abiala and Odebode (2015), in which the presence of fungal spores in the atmosphere was investigated, spores of F. verticilliodes were among those collected from the air at various locations in Nigeria. The spores travel easily in the air when dispersed, thus polluting the atmosphere and posing various threats to plants and animals. An increase of these mycotoxin-producing fungal spores was observed during summer, which is in line with the study of Omotayo (Omotayo et al., 2019a),. These fungal spores are capable of contaminating food, as noted by Badar (Badar et al., 2012), who observed them on some vegetables and fruits in India.
8.2 Compromise of maize quality
Maize is a crop with high economic value and impacts globally, it helps to minimize food shortages and is capable of limiting hunger and starvation, and boosting the food security of developing nations (Adiaha, 2018). Maize production raises the standard of living and contributes to the increasing income of farmers and foreign exchange earnings (Omotayo et al., 2016; Adiaha, 2018; Omotayo et al., 2022). Millions of tons of maize are produced each year in South Africa, with approximately 12.5 million tons being produced in the 2018/2019 season, and 16.1 million tons in 2019/2020 (1).
In South Africa, maize has yielded over 15% of the gross value of all agricultural products and covered approximately 4% of the cultivated area (Stats, 2017). This widespread production of maize contributes to the income of farmers globally. However, F. verticillioides in maize reduces the quality of this crop (Ncube et al., 2011). This implies that inadequate control of this fungus can compromise the quality of maize unknowingly due to its asymptomatic outward appearance (Rai, 2011; Ribes et al., 2018). This does not only influence the income of farmers but households and maize-producing regions.
8.3 Disease outbreak and health complications
The presence of fumonisin in crops can infiltrate food and feeds during production (Kamle et al., 2019), causing various reactions, from allergy to several illnesses. In southern Africa, maize and its derivatives are a major source of food (Du Plessis, 2003), constituting a significant part of infants’ diets. The consumption of contaminated maize and its end products, therefore, affects the health of not only adults but also infants, leading to various complications (Omotayo et al., 2016; Gai et al., 2018; Omotayo et al., 2019b) and in severe cases, death.
9 The role of the phytomicrobiome in biocontrol
The microorganisms associated with plants (also known as the phytomicrobiome) interact with the host plant in various ways and perform crucial roles in agriculture (Leveau, 2015; Omotayo, 2022). Bacillus amyloliquefaciens and Microbacterium oleovorans are part of the microorganisms that dominate the phytomicrobiome and produce various kinds of antibiotics which serve the host plant in protection against the pathogen. From previous studies, it has been found that these plant-associated microorganisms which are majorly bacteria, fungi, and archaea can be manipulated and engaged as biocontrol agents against the notorious F. verticillioides (1).
10 Biocontrol agents
The introduction of materials with biological origin to regulate or eliminate target organisms has been considered effective and environmentally secure (English, 1970; Harper, 2001; Fendrihan and Pop, 2021). It involves the use of suitable and effective pathogens, predators, and competitors, which can be bacteria, fungi, viruses, and protozoans (English, 1970; Aremu et al., 2017). Examples of these are Trichoderma spp., that is used against Macrophomina phaseola and Rhizoctonia solani to control charcoal rot and banded blight in maize, with Bacillus species being used against a wide array of pathogens, including Xanthomonas oryzae to control bacteria leaf blight in rice (Singh, 2014). Streptomyces isolates (designated as DAUFPE 11470 and DAUFPE 14632), that are isolated from the maize rhizosphere soil, have been proven to be effective in controlling Stenocarpella maydis in maize (Bressan and Figueiredo, 2005).
The above isolates suppress the development of Penicillium spp.,Curvularia lunata, Drechsleramaydis, Aspergillus spp., and other pathogenic fungi (Bressan and Figueiredo, 2005). However, the high specificity of these agents makes them environmentally benign, and their cost also has limited their use (Nations U, 1972). In the control of F. verticillioides, the following biological agents have been confirmed effective: Bacillus amyloliquefaciens, Microbacterium oleovorans, Enterobacter spp., and Pseudomonas spp.
10.1 Bacillus amyloliquefaciens and Microbacterium oleovorans
Bacillus species can produce resistant spores as well as antibiotics that are capable of inhibiting a wide range of plant pathogens by antibiosis (Cavaglieri et al., 2005; Adeniji et al., 2020). According to Khan (Khan et al., 2017), they resist numerous pathogens, including fungi, by producing certain metabolites that exert a toxic effect or create a systemic resistance in plants. The propensity of these microbes to withstand harsh environmental conditions, such as high temperatures, insufficient nutrients, inadequate water supply, and adverse pH (Shafi et al., 2017), enables them to thrive in several habitats, including soil and seawater (Hoch and Sonenshein, 1993). Bacillus amyloliquefaciens has been reported to be present in the maize rhizosphere due to its ability to survive under low oxygen concentrations (Nakano and Hulett, 1997), thus making it capable and effective as a biopesticide (Rosas-García, 2009) as well as a biocontrol agent against F. verticilloides.
Among these groups of organisms, Bacillus amyloliquefaciens impedes F. verticilliodes and other soil pathogens through the competitive exclusion principle when introduced into the soil before maize cultivation (Palma and Arteiro, 2007). It competes with this pathogen for nutrients while producing toxic compounds that are capable of inhibiting them (Palma and Arteiro, 2007). Pereira (Pereira et al., 2007), evaluated the ability of Bacillus amyloliquefaciens and Microbacterium oleovorans to reduce the occurrence of F. verticillioides in maize and showed that their application is effective. It was also found that Bacillus amyloliquefaciens can reduce the level of occurrence of this fungus in the maize rhizosphere (Pereira et al., 2007). Introducing it to the maize rhizosphere would bring about a substantial reduction in the spread of F. verticilliodes from the soil to the plant, hence reducing the fumonisin content in the maize.
Apart from the introduction of these biocontrol agents into the maize rhizosphere, seed coating with Bacillus amyloliquefaciens is also a technique that can be optimized to combat this pathogen (Chandra Nayaka et al., 2009). This will not only antagonize F. verticilloides in the rhizosphere but also repress it in the spermosphere, thus preventing its infiltration into the growing plant (Pereira et al., 2007). The observations of Siahmoshteh (Siahmoshteh et al., 2018), about the ability of Bacillus subtilis and amyloliquefaciens to produce antifungal substances that could subdue a wide range of pathogenic fungi associated with food, including maize, are also in line with the above findings.
Spraying maize ears at the flowering stage with Microbacterium oleovorans was effective in reducing the F. verticillioides count and its fumonisin B1 content in maize (Pereira et al., 2010). In the study by Pereira (Pereira et al., 2007), maize seeds were treated with Microbacterium oleivorans and then planted, after which it was found that treatment with the bacterium produced a significant reduction in the fungus counts at the root inner tissues of growing seedlings, and also reduced the quantity of Fumonisin (FB1 and FB2) significantly. According to Chulze (Chulze et al., 2015), Microbacterium oleivorans is effective in controlling this fungus and the accumulation of fumonisin in maize at the pre-harvest stage, these findings agree with that of Sartori (Sartori et al., 2012), who stated that its treatment with Microbacterium oleivorans significantly decreased its growth rate.
10.2 Enterobacter
Another species of bacteria that has been noted to be effective as a biocontrol agent against F. verticillioides is Enterobacter hormaechei, which belongs to the Enterobacteriaceae family, known previously as the Enteric group 75 (O'hara et al., 1989). The efficiency of rhizospheric Enterobacter in controlling F. verticilliodes especially when used with other bacteria such as B. amyloliquefaciens has been confirmed (Pereira et al., 2010). According to Pereira (Pereira et al., 2010), seed coating with B. amyloliquefaciens and E. hormaechei reduced significantly the level of fumonisin B1 in harvested maize. Moreover, Abiala and Odebode (2015), showed that rhizospheric Enterobacter species (OSR7 and IGGR11) are effective in suppressing F. verticilliodes. In their study, the potentials of rhizosphere Enterobacter species (OSR7 and IGGR11) on plant growth was explored, with the finding indicating that these bacterial species not only improved maize growth but also impeded the pathogenic activity of F. verticillioides, thereby leaving the crop with no symptom of the disease.
Enterobacter cloacae is another species noted to be effective in inhibiting F. verticillioides (Cavaglieri et al., 2005), Enterobacter cloacae together with Microbacterium oleovorans, were combined for treating maize seeds before cultivation. It was observed that the mixture inhibited the growth of F. verticillioides. According to Hinton and Bacon (1995), Enterobacter cloacae has been reported to be an excellent biocontrol agent against root colonization of this Fusarium species in maize.
10.3 Pseudomonas
These groups of microorganisms are known for their metabolic diversity and ability to occupy several habitats, being found in soil, water, and plants (Brock et al., 1994; Adeniji et al., 2020). Pseudomonas is a genus of Gram-negative Gammaproteobacteria, which belongs to the Pseudomonadaceae family (Euzéby, 2009). Nayaka et al. (Chandra Nayaka et al., 2009) observed that after spraying maize seeds with a pure culture and powder formulation of P. fluorescens, this biocontrol agent successfully inhibited the growth of F. verticillioides and suppressed the synthesis of fumonisin. Furthermore, antibiosis was observed by Cavaglieri et al. (Cavaglieri et al., 2005; Chandra Nayaka et al., 2009) when Pseudomonas solanacearum and Bacillus subtilis were used for maize seed treatment before planting, being able to antagonize F. verticillioides and diminish the production of fumonisin.
10.4 Burkholderia cepacia
Formerly known as Pseudomonas cepacia (Laraya-Cuasay et al., 1977), this resides in the rhizosphere of many plants and is noted to produce active metabolites, which resist and inhibit many disease-causing fungi. Bevivino (Bevivino et al., 2005), confirmed the ability of this bacteria to inhibit the growth of F. verticillioides. According to Zeidan (Zeidan et al., 2019), the inhibitory activity of the thermostable metabolites of these bacteria makes them suitable biocontrol agents against toxigenic fungi. It was also discovered that the bacteria possess antifungal activity against mycotoxigenic and phytopathogenic fungi, their ability to inhibit Aspergillus carbonarius and Penicillium verrucosum being recorded. Table 2 provides recommended strategies from the literature that can be adopted to effectively control F. verticillioides and its fumonisin derivative, and indicates how various organisms can be adopted in their control, such as colonizing the maize root with biological control agents, inoculation, seed priming/treatment as well as an in-planta assay.
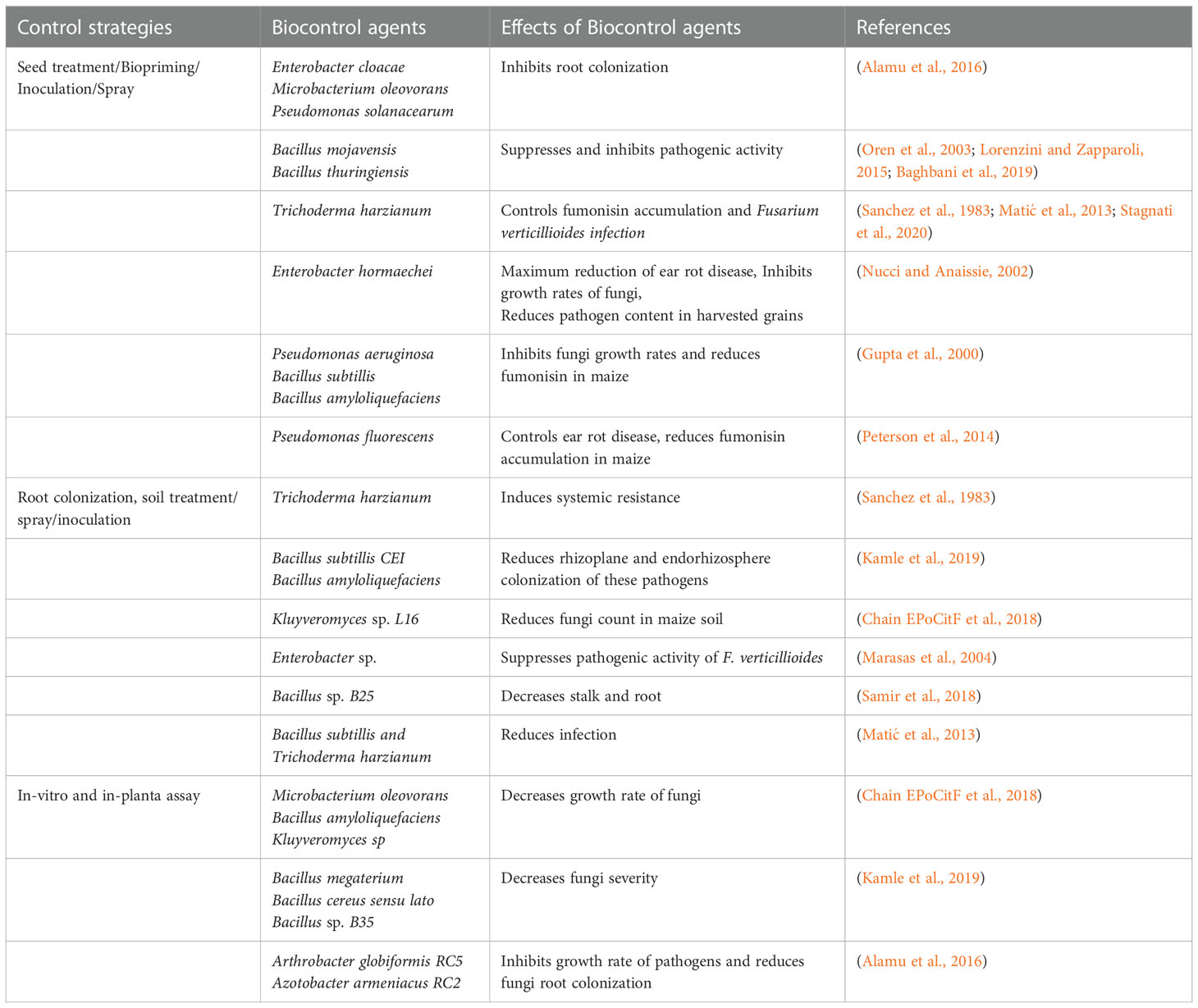
Table 2 Other plant associated microorganisms with potentials for Fusarium verticillioides and fumonisin biocontrol.
11 Conclusion
The common maize rhizosphere inhabitant and pathogen, F. verticillioides, has contributed to various agricultural challenges, including soil contamination. It has led to several recorded disease outbreaks that have killed livestock and contributed to the loss of produced grains (maize). Several techniques have been adopted to eliminate this fungus from plants and fields but to no avail. However, the use of biological agents has been considered an excellent option, which is not only effective but environmentally friendly and safe. Bacillus spp, Enterobacter, Pseudomonas, and Microbacterium oleovorans are proven and efficient microbes that can be utilized to control and inhibit this pathogen. The outbreaks and occurrence of illnesses caused by F. verticilliodes and its fumonisin derivative, and the deadly threat they pose, call for a corporate intervention on how this pathogen can be resisted and impeded through the use of biological control agents due to their advantages over synthetic pesticides and fungicides.
Author contributions
OPO conceptualize the article which was critique by OOB. Both authors agreed on the final version of the manuscript before submission. All authors contributed to the article and approved the submitted version.
Acknowledgments
OPO appreciate the North-West University, South Africa, for the enabled environment provided for the research.
Conflict of interest
The authors declare that the research was conducted in the absence of any commercial or financial relationships that could be construed as a potential conflict of interest.
Publisher’s note
All claims expressed in this article are solely those of the authors and do not necessarily represent those of their affiliated organizations, or those of the publisher, the editors and the reviewers. Any product that may be evaluated in this article, or claim that may be made by its manufacturer, is not guaranteed or endorsed by the publisher.
References
Abiala M. A., Odebode A. C. (2015). Rhizospheric enterobacter enhanced maize seedling health and growth. Biocontrol Sci. technol 25 (4), 359–372. doi: 10.1080/09583157.2014.981248
Adeniji A. A., Aremu O. S., Babalola O. O. (2020). Pseudomonas fulva HARBPS9. 1: candidate anti-fusarium agent in south Africa. Eur. J. Plant Pathol 157 (4), 767–781. doi: 10.1007/s10658-020-02035-4
Adiaha M. S. (2018). Economic value of maize (Zea mays l.) in Nigeria and its impacts on the global food production. Int. J. Sci. World 9 (257), 280–284. doi: 10.14419/ijsw.v6i1.8771
Aisha K. (2012). Ruminal microflora, mycotoxin inactivation by ruminal microflora and conditions favouring mycotoxicosis in ruminants: a review. Int. J. veterin science 1 (1), 37–44.
Alamu O. P., Adeeyo O. A., Babalola O. O. (2016). Effect of aqueous extracts of mangifera indica linn. on the testes of adult male wistar rats. J. Hum. Ecol 56 (1-2), 135–138. doi: 10.1080/09709274.2016.11907047
Alberts J. F., Van Zyl W. H., Gelderblom W. C. (2016). Biologically based methods for control of fumonisin-producing fusarium species and reduction of the fumonisins. Front. Microbiol. 7, 548–556. doi: 10.3389/fmicb.2016.00548
Aremu B. R., Alori E. T., Kutu R. F., Babalola O. O. (2017). Potentials of microbial inoculants in soil productivity: an outlook on African legumes. Microorg Green Revol 4, 53–75. doi: 10.1007/978-981-10-6241-4_3
Babalola O. O., Glick B. R. (2012). The use of microbial inoculants in African agriculture: current practice and future prospects. J. Food Agric. Environ. 10 (3&4), 540–549.
Bacon C., Glenn A., Yates I. (2008). Fusarium verticillioides: managing the endophytic association with maize for reduced fumonisins accumulation. Toxin Rev. 27 (3-4), 411–446. doi: 10.1080/15569540802497889
Badar K., Kakde R. B., Pawar S., Chavan A. M. (2012). Bioactivity of plants gums against pathogenic fungi. Int. Multidiscip. Res. J. 2 (3), 9–12.
Baghbani F., Lotfi R., Moharramnejad S., Bandehagh A., Roostaei M., Rastogi A., et al. (2019). Impact of fusarium verticillioides on chlorophyll fluorescence parameters of two maize lines. Eur. J. Plant Pathol 154 (2), 337–346. doi: 10.1007/s10658-018-01659-x
Bakri M. M. (2021). Evaluating the effects of cellulolytic enzymes and lactobacillus bulgaricus on mycotoxins production and the quality of maize silage. BioResources. 16 (4), 8366–8378. doi: 10.15376/biores.16.4.8366-8378
Betz F. S., Hammond B. G., Fuchs R. L. (2000). Safety and advantages of bacillus thuringiensis-protected plants to control insect pests. Regul. Toxicol. Pharmacol. 32 (2), 156–173. doi: 10.1006/rtph.2000.1426
Bevivino A., Peggion V., Chiarini L., Tabacchioni S., Cantale C., Dalmastri C. (2005). Effect of fusarium verticillioides on maize-root-associated burkholderia cenocepacia populations. Res. Microbiol. 156 (10), 974–983. doi: 10.1016/j.resmic.2005.05.007
Blacutt A. A., Gold S. E., Voss K. A., Gao M., Glenn A. E. (2018). Fusarium verticillioides: Advancements in understanding the toxicity, virulence, and niche adaptations of a model mycotoxigenic pathogen of maize. Phytopathology. 108 (3), 312–326. doi: 10.1094/PHYTO-06-17-0203-RVW
Borah S., Deka S., Sarma H. (2016). First report of fusarium verticillioides causing stalk rot of maize in Assam, India. Plant Dis. 100 (1501), 10.1094. doi: 10.1094/PDIS-01-16-0074-PDN
Bordini J., Saito G., Andrade C., Ono M., Hirooka E., Vizoni É, et al. (2015). Effect of fungicide on fusarium verticillioides mycelial morphology and fumonisin B1 production. Braz. J. Microbiol. 46 (1), 293–299. doi: 10.1590/S1517-838246120120383
Bressan W., Figueiredo J. (2005). Biological control of stenocarpella maydis in maize seed with antagonistic streptomyces sp. isolates. J. phytopathol 153 (10), 623–626. doi: 10.1111/j.1439-0434.2005.01014.x
Brock T., Madigan M., Martinko J., Parker J. (1994). Biology of microorganisms 7th ed Vol. 648 (New Jersey: Prentice Hall Upper Saddle River), 650.
Burger H., Shephard G., Louw W., Rheeder J., Gelderblom W. (2013). The mycotoxin distribution in maize milling fractions under experimental conditions. Int. J. Food Microbiol. 165 (1), 57–64. doi: 10.1016/j.ijfoodmicro.2013.03.028
Caldeira A. T., Feio Savluchinske S., Arteiro S. J.M., Roseiro J. C. (2007). Bacillus amyloliquefaciens CCMI 1051 in vitro activity against wood contaminant fungi. Ann Microbiol 57 (1), 29–34.
Cavaglieri L., Orlando J., Etcheverry M. (2005). In vitro influence of bacterial mixtures on fusarium verticillioides growth and fumonisin B1 production: effect of seeds treatment on maize root colonization. Lett. Appl. Microbiol. 41 (5), 390–396. doi: 10.1111/j.1472-765X.2005.01785.x
Chain EPoCitF, Knutsen H. K., Alexander J., Barregård L., Bignami M., Brüschweiler B., et al. (2018). Risks for animal health related to the presence of fumonisins, their modified forms and hidden forms in feed. EFSA J. 16 (5), e05242.
Chandra Nayaka S., Udaya Shankar A. C., Reddy M. S., Niranjana S. R., Prakash H. S., Shetty H. S., et al. (2009). Control of fusarium verticillioides, cause of ear rot of maize, by pseudomonas fluorescens. Pest Manage. Science: former Pesticide Science 65 (7), 769–775. doi: 10.1002/ps.1751
Chepkirui C. (2013). Antifungal secondary metabolites from monanthotaxis littoralis with activity against mycotoxigenic fungi from maize. Egerton Univ.
Chulze S. (2010). Strategies to reduce mycotoxin levels in maize during storage: a review. Food Additives Contamin 27 (5), 651–657. doi: 10.1080/19440040903573032
Chulze S. N., Palazzini J. M., Torres A. M., Barros G., Ponsone M. L., Geisen R., et al. (2015). Biological control as a strategy to reduce the impact of mycotoxins in peanuts, grapes and cereals in Argentina. Food Additives Contamin: Part A. 32 (4), 471–479. doi: 10.1080/19440049.2014.984245
Colmenarez Y. C., Corniani N., Jahnke S. M., Sampaio M. V., Vásquez C. (2018). “Use of parasitoids as a biocontrol agent in the Neotropical region: Challenges and potential,” in Horticulture (IntechOpen). Available at: https://www.intechopen.com/chapters/64004.
Czembor E., Stępień Ł, Waśkiewicz A. (2015). Effect of environmental factors on fusarium species and associated mycotoxins in maize grain grown in Poland. PloS One 10 (7), e0133644. doi: 10.1371/journal.pone.0133644
Demir C., Simsek O., Arici M. (2010). Incidence of fusarium verticillioides and levels of fumonisin b 1 and b 2 in corn in Turkey. Food Sci. Biotechnol. 19 (4), 1103–1106. doi: 10.1007/s10068-010-0156-1
Doohan F., Weston G., Rezanoor H., Parry D., Nicholson P. (1999). Development and use of a reverse transcription-PCR assay to study expression of Tri5 byFusarium species In vitro and in planta. Appl. Environ. Microbiol. 65 (9), 3850–3854. doi: 10.1128/AEM.65.9.3850-3854.1999
Duncan K. E., Howard R. J. (2010). Biology of maize kernel infection by fusarium verticillioides. Mol. Plant-Microbe interact 23 (1), 6–16. doi: 10.1094/MPMI-23-1-0006
English O. (1970). Public law Vol. 91 (United States Department Of Labor), 596. doi: 10.1002/9780470015902.a0000344.pub3
Esterhuizen D. (2020) South Africa: Produces 2nd highest corn crop. Available at: https://wwwallaboutfeednet/animal-feed/raw-materials/south-africa-produces-2nd-highest-corn-crop/.
Euzéby J. (2009). List of prokaryotic names with standing in nomenclature. http://www bacterio cict fr. Available at: https://lpsn.dsmz.de/phylum.
Fandohan P., Ahouansou R., Houssou P., Hell K., Marasas W., Wingfield M. (2006). Impact of mechanical shelling and dehulling on fusarium infection and fumonisin contamination in maize. Food additives contamin 23 (4), 415–421. doi: 10.1080/02652030500442516
Fandohan P., Gnonlonfin B., Hell K., Marasas W., Wingfield M. (2005). Natural occurrence of fusarium and subsequent fumonisin contamination in preharvest and stored maize in Benin, West Africa. Int. J. Food Microbiol. 99 (2), 173–183. doi: 10.1016/j.ijfoodmicro.2004.08.012
Fazekas B., Bajmocy E., Glavits R., Fenyvesi A., Tanyi J. (1998). Fumonisin B1 contamination of maize and experimental acute fumonisin toxicosis in pigs. J. Veterin Medicine Ser. B. 45 (1-10), 171–181. doi: 10.1111/j.1439-0450.1998.tb00780.x
Fendrihan S., Pop C. (2021). Biotechnological potential of plant associated microorganisms. Rom Biotechnol. Lett. 26, 22700–22706. doi: 10.25083/rbl/26.3/2700-2706
Gai X., Dong H., Wang S., Liu B., Zhang Z., Li X., et al. (2018). Infection cycle of maize stalk rot and ear rot caused by fusarium verticillioides. PloS One 13 (7). doi: 10.1371/journal.pone.0201588
Giannitti F., Diab S. S., Pacin A. M., Barrandeguy M., Larrere C., Ortega J., et al. (2011). Equine leukoencephalomalacia (ELEM) due to fumonisins B1 and B2 in Argentina. Pesquisa Veterinária Brasileira 31 (5), 407–412. doi: 10.1590/S0100-736X2011000500007
Guerre P. (2020). Mycotoxin and gut microbiota interactions. Toxins. 12 (12), 769. doi: 10.3390/toxins12120769
Gupta A. K., Baran R., Summerbell R. C. (2000). Fusarium infections of the skin. Curr. Opin. Infect. diseases 13 (2), 121–128. doi: 10.1097/00001432-200004000-00005
Harper D. R. (2001). Biological control by microorganisms (eLS). Available at: https://link.springer.com/chapter/10.1007/978-3-319-08575-3_4.
Headrick J., Pataky J. (1991). Maternal influence on the resistance of sweet corn lines to kernel infection by. Fusariummoniliforme 81, 268–274.
Heperkan D., Güler F. K., Oktay H. (2012). Mycoflora and natural occurrence of aflatoxin, cyclopiazonic acid, fumonisin and ochratoxin a in dried figs. Food Additives Contamin: Part A. 29 (2), 277–286. doi: 10.1080/19440049.2011.597037
Hinton D. M., Bacon C. W. (1995). Enterobacter cloacae is an endophytic symbiont of corn. Mycopathologia. 129 (2), 117–125. doi: 10.1007/BF01103471
Hirata T., Kimishima E., Aoki T., Nirenberg H. I., O’Donnell K. (2001). Morphological and molecular characterization of fusarium verticillioides from rotten banana imported into Japan. Mycoscience 42 (2), 155–166. doi: 10.1007/BF02464132
Hoch J. A., Sonenshein A. L. (1993). Bacillus subtilis and other gram-positive bacteria: biochemistry, physiology and molecular genetics.
Humans IWGotEoCRt Cancer IAfRo (2002). Some traditional herbal medicines, some mycotoxins, naphthalene and styrene. Available at: https://www.ncbi.nlm.nih.gov/books/NBK326619/.
Iqbal N., Czékus Z., Poór P., Ördög A. (2021). Plant defence mechanisms against mycotoxin fumonisin B1. Chemico-Biolog Interact 343, 109494. doi: 10.1016/j.cbi.2021.109494
Kamala A., Kimanya M., Haesaert G., Tiisekwa B., Madege R., Degraeve S., et al. (2016). Local post-harvest practices associated with aflatoxin and fumonisin contamination of maize in three agro ecological zones of Tanzania. Food Additives Contamin: Part A. 33 (3), 551–559. doi: 10.1080/19440049.2016.1138546
Kamle M., Mahato D. K., Devi S., Lee K. E., Kang S. G., Kumar P. (2019). Fumonisins: impact on agriculture, food, and human health and their management strategies. Toxins. 11 (6), 328. doi: 10.3390/toxins11060328
Khan N., Maymon M., Hirsch A. M. (2017). Combating fusarium infection using bacillus-based antimicrobials. Microorganisms. 5 (4), 75. doi: 10.3390/microorganisms5040075
Khan M., Pandey A., Athar T., Choudhary S., Deval R., Gezgin S., et al. (2020). Fusarium head blight in wheat: contemporary status and molecular approaches. 3 Biotech 10 (4), 1–17. doi: 10.1007/s13205-020-2158-x
Kimanya M. E., De Meulenaer B., Tiisekwa B., Ndomondo-Sigonda M., Devlieghere F., Van Camp J., et al. (2008). Co-Occurrence of fumonisins with aflatoxins in home-stored maize for human consumption in rural villages of Tanzania. Food additives contamin 25 (11), 1353–1364. doi: 10.1080/02652030802112601
Kriek N., Kellerman T. S., Marasas W. F. O. (1981). A comparative study of the toxicity of fusarium verticillioides (= f. moniliforme) to horses, primates, pigs, sheep and rats. Onderstepoort J Vet Res 48 (2), 129–31.
Laraya-Cuasay L. R., Lipstein M., Huang N. N. (1977). Pseudomonas cepacia in the respiratory flora of patients with cystic fibrosis (CF). Pediatr. Res. 11 (4), 502. doi: 10.1203/00006450-197704000-00792
Ledoux D. R., Brown T. P., Weibking T. S., Rottinghaus G. E. (1992). Fumonisin toxicity in broiler chicks. J. Veterin Diagn. Invest. 4 (3), 330–333. doi: 10.1177/104063879200400317
Leslie J. F., Pearson C., Nelson P. E., Toussoun T. (1990). Fusarium spp. from corn, sorghum, and soybean fields in the central and eastern united states. Ecol. Stud. 44 (63), 66.
Leveau J. H. (2015). “Life of microbes on aerial plant parts,” in Principles of plant-microbe interactions (Springer), 17–24.
Li T., Gong L., Wang Y., Chen F., Gupta V. K., Jian Q., et al. (2017). Proteomics analysis of fusarium proliferatum under various initial pH during fumonisin production. J. proteomics 164, 59–72. doi: 10.1016/j.jprot.2017.05.008
Lorenzini M., Zapparoli G. (2015). Occurrence and infection of cladosporium, fusarium, epicoccum and aureobasidium in withered rotten grapes during post-harvest dehydration. Antonie Van Leeuwenhoek 108 (5), 1171–1180. doi: 10.1007/s10482-015-0570-8
Marasas W. (2001). Discovery and occurrence of the fumonisins: a historical perspective. Environ. Health Perspect. 109 (suppl 2), 239–243.
Marasas W. F. O., Naude T., Pienaar J., Kellerman T. S. (1976). Leukoencephalomacia: a mycotoxicosis of equidae caused by fusarium moniliforme Sheldon. Onderstepoort J Vet Res 43 (3), 113–22.
Marasas W. F., Riley R. T., Hendricks K. A., Stevens V. L., Sadler T. W., Gelineau-van Waes J., et al. (2004). Fumonisins disrupt sphingolipid metabolism, folate transport, and neural tube development in embryo culture and in vivo: a potential risk factor for human neural tube defects among populations consuming fumonisin-contaminated maize. J. Nutr. 134 (4), 711–716. doi: 10.1093/jn/134.4.711
Marnewick J. L., van der Westhuizen F. H., Joubert E., Swanevelder S., Swart P., Gelderblom W. C. (2009). Chemoprotective properties of rooibos (Aspalathus linearis), honeybush (Cyclopia intermedia) herbal and green and black (Camellia sinensis) teas against cancer promotion induced by fumonisin B1 in rat liver. Food Chem. Toxicol 47 (1), 220–229. doi: 10.1016/j.fct.2008.11.004
Matić S., Spadaro D., Prelle A., Gullino M. L., Garibaldi A. (2013). Light affects fumonisin production in strains of fusarium fujikuroi, fusarium proliferatum, and fusarium verticillioides isolated from rice. Int. J. Food Microbiol. 166 (3), 515–523. doi: 10.1016/j.ijfoodmicro.2013.07.026
Menniti A., Gregori R., Neri F. (2010). Activity of natural compounds on fusarium verticillioides and fumonisin production in stored maize kernels. Int. J. Food Microbiol. 136 (3), 304–309. doi: 10.1016/j.ijfoodmicro.2009.10.008
Mita M. M., Jannat M., Bashar S., Protic I. A., Saha P., Masud M. M., et al. (2022). Potential native bacilli reduce fumonisin contamination in maize. Agronomy. 12 (11), 2608. doi: 10.3390/agronomy12112608
Moss M., Frank J. (1985). Influence of the fungicide tridemorph on T-2 toxin production by fusarium sporotrichioides. Trans. Br. Mycolog Society 84 (4), 585–590. doi: 10.1016/S0007-1536(85)80111-X
Munkvold G., Carlton W. (1997). Influence of inoculation method on systemic fusarium moniliforme infection of maize plants grown from infected seeds. Plant Dis. 81 (2), 211–216. doi: 10.1094/PDIS.1997.81.2.211
Munkvold G. P., Hellmich R. L., Showers W. (1997). Reduced fusarium ear rot and symptomless infection in kernels of maize genetically engineered for European corn borer resistance. Phytopathology 87 (10), 1071–1077. doi: 10.1094/PHYTO.1997.87.10.1071
Mupunga I. (2013). A comparative study of natural contamination with aflatoxins and fumonisins in selected food commodities from Botswana and Zimbabwe (University of South Africa). Available at: https://uir.unisa.ac.za/handle/10500/13339.
Myburg R. B., Dutton M. F., Chuturgoon A. A. (2002). Cytotoxicity of fumonisin B1, diethylnitrosamine, and catechol on the SNO esophageal cancer cell line. Environ. Health perspect 110 (8), 813–815. doi: 10.1289/ehp.02110813
Nakano M. M., Hulett F. M. (1997). Adaptation of bacillus subtilis to oxygen limitation. FEMS Microbiol. letters 157 (1), 1–7. doi: 10.1111/j.1574-6968.1997.tb12744.x
Nations U (1972). Convention on the prohibition of the development, production and stockpiling of bacteriological (Biological) and toxin weapons and on their destruction. Available at: https://www.un.org/disarmament/biological-weapons/.
Ncube E., Flett B. C., Van den Berg J., Erasmus A., Viljoen A. (2017). The effect of busseola fusca infestation, fungal inoculation and mechanical wounding on fusarium ear rot development and fumonisin production in maize. Crop Prot. 99, 177–183. doi: 10.1016/j.cropro.2017.05.024
Ncube E., Flett B. C., Waalwijk C., Viljoen A. (2011). Fusarium spp. and levels of fumonisins in maize produced by subsistence farmers in south Africa. South Afr. J. Science 107 (1-2), 1–7.
Nguyen T. T. X., Dehne H.-W., Steiner U. (2016). Histopathological assessment of the infection of maize leaves by fusarium graminearum, f. proliferatum and f. verticillioides. Fungal Biol. 120 (9), 1094–1104.
Nicolopoulou-Stamati P., Maipas S., Kotampasi C., Stamatis P., Hens L. (2016). Chemical pesticides and human health: the urgent need for a new concept in agriculture. Front. Public Health 4, 148. doi: 10.3389/fpubh.2016.00148
Nucci M., Anaissie E. (2002). Cutaneous infection by fusarium species in healthy and immunocompromised hosts: implications for diagnosis and management. Clin. Infect. Diseases 35 (8), 909–920. doi: 10.1086/342328
O'hara C., Steigerwalt A., Hill B., Farmer J., Fanning G., Brenner D. (1989). Enterobacter hormaechei, a new species of the family enterobacteriaceae formerly known as enteric group 75. J. Clin. Microbiol. 27 (9), 2046–2049. doi: 10.1128/jcm.27.9.2046-2049.1989
O’Donnell K., Rooney A. P., Proctor R. H., Brown D. W., McCormick S. P., Ward T. J., et al. (2013). Phylogenetic analyses of RPB1 and RPB2 support a middle Cretaceous origin for a clade comprising all agriculturally and medically important fusaria. Fungal Genet. Biol. 52, 20–31. doi: 10.1016/j.fgb.2012.12.004
Oldenburg E., Höppner F., Ellner F., Weinert J. (2017). Fusarium diseases of maize associated with mycotoxin contamination of agricultural products intended to be used for food and feed. Mycotoxin Res. 33 (3), 167–182. doi: 10.1007/s12550-017-0277-y
Omotayo O. P. (2022). The role of rhizosphere microbiome in agricultural sustainability. PONTE Int. J. Sci. Res. 78 (10). doi: 10.21506/j.ponte.2022.10.7
Omotayo A. O., Aremu B. R., Alamu O. P. (2016). Food utilization, nutrition, health and farming households’ income: A critical review of literature. J. Hum. Ecol. 56 (1-2), 171–182. doi: 10.1080/09709274.2016.11907053
Omotayo O. P., Igiehon O. N., Babalola O. O. (2022). Microbial genes of agricultural importance in maize rhizosphere unveiled through shotgun metagenomics. Spanish J. Soil Science 6. doi: 10.3389/sjss.2022.10427
Omotayo O. P., Omotayo A. O., Babalola O. O., Mwanza M. (2019a). Comparative study of aflatoxin contamination of winter and summer ginger from the north West province of south Africa. Toxicol. Rep. 6, 489–495. doi: 10.1016/j.toxrep.2019.05.011
Omotayo O. P., Omotayo A. O., Babalola O. O., Mwanza M. (2019b). Dataset on the toxic effects of aflatoxin and ochratoxin a on the human gastric smooth muscle cells. Data brief. 25, 104089. doi: 10.1016/j.dib.2019.104089
Omotayo O. P., Omotayo A. O., Mwanza M., Babalola O. O. (2019). Prevalence of mycotoxins and their consequences on human health. Toxicolog Res. 35 (1), 1–7. doi: 10.5487/TR.2019.35.1.001
Omotayo A. O., Omotoso A. B., Daud S. A., Omotayo O. P., Adeniyi B. A. (2022). Rising food prices and farming households food insecurity during the COVID-19 pandemic: Policy implications from SouthWest Nigeria. Agriculture. 12 (3), 363. doi: 10.3390/agriculture12030363
Oren L., Ezrati S., Cohen D., Sharon A. (2003). Early events in the fusarium verticillioides-maize interaction characterized by using a green fluorescent protein-expressing transgenic isolate. Appl. Environ. Microbiol. 69 (3), 1695–1701. doi: 10.1128/AEM.69.3.1695-1701.2003
Pereira P., Nesci A., Castillo C., Etcheverry M. (2010). Impact of bacterial biological control agents on fumonisin B1 content and fusarium verticillioides infection of field-grown maize. Biol. Control 53 (3), 258–266. doi: 10.1016/j.biocontrol.2010.02.001
Pereira P., Nesci A., Etcheverry M. (2007). Effects of biocontrol agents on fusarium verticillioides count and fumonisin content in the maize agroecosystem: Impact on rhizospheric bacterial and fungal groups. Biol. control 42 (3), 281–287. doi: 10.1016/j.biocontrol.2007.05.015
Peterson A., Pham M. H., Lee B., Commins D., Cadden J., Giannotta S. L., et al. (2014). Intracranial fusarium fungal abscess in an immunocompetent patient: case report and review of the literature. J. neurolog Surg. Rep. 75 (02), e241–e2e5.
Picot A., Barreau C., Caron D., Lannou C., Richard-Forget F. (2011). The dent stage of maize kernels is the most conducive for fumonisin biosynthesis under field conditions. Appl. Environ. Microbiol. 77 (23), 8382–8390. doi: 10.1128/AEM.05216-11
Rai M. (2011). Natural antimicrobials in food safety and quality. Cabi 30, 368. doi: 10.1079/9781845937690.0000
Ribes S., Fuentes A., Talens P., Barat J. M. (2018). Prevention of fungal spoilage in food products using natural compounds: a review. Crit. Rev. Food Sci. Nutr. 58 (12), 2002–2016. doi: 10.1080/10408398.2017.1295017
Rodrigues I. (2014). A review on the effects of mycotoxins in dairy ruminants. Anim. Product Science 54 (9), 1155–1165. doi: 10.1071/AN13492
Rosas-García N. M. (2009). Biopesticide production from bacillus thuringiensis: an environmentally friendly alternative. Recent Patents Biotechnol. 3 (1), 28–36. doi: 10.2174/187220809787172632
Kouzi SA, Wright NJ, Dirks-Naylor A, Uddin MN. (2018). Fumonisins: Effects on human and animal health and mechanisms of toxicity. EC Pharmacol. Toxicol 6, 187–208.
Samsudin N. I. P. B. (2015). Potential biocontrol of fumonisin B1 production by fusarium verticillioides under different ecophysiological conditions in maize. .p
Sanchez R., Hall A., Trapani N., De Hunau R. C. (1983). Effects of water stress on the chlorophyll content, nitrogen level and photosynthesis of leaves of two maize genotypes. Photosynthes Res. 4 (1), 35–47. doi: 10.1007/BF00041799
Sartori M., Nesci A., Magan N., Etcheverry M. (2012). Accumulation of the betaine and ectoine in osmotic stress adaptation of biocontrol agents against fusarium verticillioides in maize. J Agric Sci. 3 (1), 83–89. doi: 10.4236/as.2012.31011
Scarpino V., Reyneri A., Vanara F., Scopel C., Causin R., Blandino M. (2015). Relationship between European corn borer injury, fusarium proliferatum and f. subglutinans infection and moniliformin contamination in maize. Field Crops Res. 183, 69–78. doi: 10.1016/j.fcr.2015.07.014
Schoeman A., Flett B., van Rensburg B. J., Ncube E., Viljoen A. (2018). Pathogenicity and toxigenicity of fusarium verticillioides isolates collected from maize roots, stems and ears in south Africa. Eur. J. Plant Pathol. 152 (3), 677–689. doi: 10.1007/s10658-018-1510-z
Shafi J., Tian H., Ji M. (2017). Bacillus species as versatile weapons for plant pathogens: a review. Biotechnol. Biotechnol. Equip 31 (3), 446–459. doi: 10.1080/13102818.2017.1286950
Shephard G. (2011). Fusarium mycotoxins and human health. Plant Breed. Seed Sci. 64, 113. doi: 10.2478/v10129-011-0034-x
Siahmoshteh F., Hamidi-Esfahani Z., Spadaro D., Shams-Ghahfarokhi M., Razzaghi-Abyaneh M. (2018). Unraveling the mode of antifungal action of bacillus subtilis and bacillus amyloliquefaciens as potential biocontrol agents against aflatoxigenic aspergillus parasiticus. Food Control 89, 300–307. doi: 10.1016/j.foodcont.2017.11.010
Singh H. (2014). Management of plant pathogens with microorganisms. Proc. Indian Natl. Sci. Acad. 2, 443–454. doi: 10.16943/ptinsa/2014/v80i2/55120
Sobek E., Munkvold G. (1999). European Corn borer (Lepidoptera: Pyralidae) larvae as vectors of fusarium moniliforme, causing kernel rot and symptomless infection of maize kernels. J. economic entomol 92 (3), 503–509. doi: 10.1093/jee/92.3.503
Stagnati L., Rahjoo V., Samayoa L. F., Holland J. B., Borrelli V. M., Busconi M., et al. (2020). A genome-wide association study to understand the effect of fusarium verticillioides infection on seedlings of a maize diversity panel. G3: Genes Genomes Genet. 10 (5), 1685–1696. doi: 10.1534/g3.119.400987
Stats (2017) Census of commercial agriculture. Available at: http://wwwstatssagovza/publications/Report-11-02-01/CoCA%202017%20Fact%20Sheetspdf.
Taranu I., Marin D. E., Bouhet S., Pascale F., Bailly J.-D., Miller J. D., et al. (2005). Mycotoxin fumonisin B1 alters the cytokine profile and decreases the vaccinal antibody titer in pigs. Toxicolog Sci. 84 (2), 301–307. doi: 10.1093/toxsci/kfi086
Terciolo C., Bracarense A. P., Souto P. C., Cossalter A.-M., Dopavogui L., Loiseau N., et al. (2019). Fumonisins at doses below EU regulatory limits induce histological alterations in piglets. Toxins. 11 (9), 548. doi: 10.3390/toxins11090548
Thavaselvam D., Vijayaraghavan R. (2010). Biological warfare agents. J. Pharm. Bioallied Sci. 2 (3), 179. doi: 10.4103/0975-7406.68499
Thiel P. G., Shephard G. S., Sydenham E. W., Marasas W. F., Nelson P. E., Wilson T. M. (1991). Levels of fumonisins B1 and B2 in feeds associated with confirmed cases of equine leukoencephalomalacia. J. Agric. Food Chem. 39 (1), 109–111. doi: 10.1021/jf00001a021
Usta C. (2013). Microorganisms in biological pest control–a review (bacterial toxin application and effect of environmental factors). Curr. Prog. Biol. Res. 10, 287–317. doi: 10.5772/55786
van Lenteren J. C., Bolckmans K., Köhl J., Ravensberg W. J., Urbaneja A. (2018). Biological control using invertebrates and microorganisms: plenty of new opportunities. BioControl. 63 (1), 39–59. doi: 10.1007/s10526-017-9801-4
Voss K. A., Riley R. T., Norred W., Bacon C. W., Meredith F. I., Howard P. C., et al. (2001). An overview of rodent toxicities: liver and kidney effects of fumonisins and fusarium moniliforme. Environ. Health Perspect. 109 (suppl 2), 259–266. doi: 10.1289/ehp.01109s2259
Zeidan R., Ul-Hassan Z., Al-Thani R., Migheli Q., Jaoua S. (2019). In-vitro application of a Qatari burkholderia cepacia strain (QBC03) in the biocontrol of mycotoxigenic fungi and in the reduction of ochratoxin a biosynthesis by aspergillus carbonarius. Toxins. 11 (12), 700. doi: 10.3390/toxins11120700
Keywords: biocontrol agents, Fusarium verticillioides, maize pathogen, maize rhizosphere, phytomicrobiome, fumonisin, mycotoxin
Citation: Omotayo OP and Babalola OO (2023) Fusarium verticillioides of maize plant: Potentials of propitious phytomicrobiome as biocontrol agents. Front. Fungal Biol. 4:1095765. doi: 10.3389/ffunb.2023.1095765
Received: 11 November 2022; Accepted: 26 January 2023;
Published: 07 February 2023.
Edited by:
M. Y. Sreenivasa, University of Mysore, IndiaReviewed by:
Akansha Jain, Banaras Hindu University, IndiaUdai B. Singh, National Bureau of Agriculturally Important Microorganisms (ICAR), India
Copyright © 2023 Omotayo and Babalola. This is an open-access article distributed under the terms of the Creative Commons Attribution License (CC BY). The use, distribution or reproduction in other forums is permitted, provided the original author(s) and the copyright owner(s) are credited and that the original publication in this journal is cited, in accordance with accepted academic practice. No use, distribution or reproduction is permitted which does not comply with these terms.
*Correspondence: Olubukola Oluranti Babalola, olubukola.babalola@nwu.ac.za