- 1Department of Biochemistry and Molecular Biology, Federal University of Paraná, Curitiba, Brazil
- 2Department of Soils and Agricultural Engineer, Federal University of Paraná, Curitiba, Brazil
- 3Department of Pathology, Federal University of Paraná, Curitiba, Brazil
Introduction
Prebiotics are typically fibrous compounds that pass undigested through the upper part of the gastrointestinal tract and stimulate the growth or activity of advantageous bacteria that colonize the bowel and contribute to the well-being of their host (Gibson and Roberfroid, 1995). Some of the most widely used prebiotics in the poultry industry are fructo-oligosaccharides, mannan-oligosaccharides, galacto-oligosaccharides and beta-glucans (Huyghebaert et al., 2011).
Selective fermentation of some prebiotics has been shown to induce changes in the composition and/or activity of the gastrointestinal microbiota, improving the health of the host (Gibson et al., 2004). Zhenping et al. (2013) showed increased growth performance, enhanced endocrine metabolism, and improved immune function in broiler chickens after in-feed supplementation with xylo-oligosaccharides prebiotics. Moreover, changes in enteric bacteria in the cecum (Spring et al., 2000) and improved intestinal morphology have been observed in broilers fed with dietary mannan-oligosaccharide (Baurhoo et al., 2009).
Because the microbiota can be modified (Khoruts et al., 2010; Borody and Khoruts, 2012) it constitutes an attractive target for therapeutic manipulation. However, successful outcome of such manipulations require a better understanding of the interactions between the host and its microbiota (Hamilton et al., 2013; Van Nood et al., 2013).
The composition and diversity of chicken intestinal microbiota were previously investigated using cultivation-based methodologies (Fernandez et al., 2002; Cross et al., 2007). However, the use of DNA-based molecular biology techniques, such as metagenomics and new generation DNA sequencing, allowed new opportunities to characterize uncultivable members of intestinal microbiota (Gong et al., 2002) shedding light on the composition and temporal spatial location of the microbial population in broiler's intestine. Nevertheless, the knowledge of the structure, interactions and functions of the intestinal microbiota is still limited and fragmented (Oakley and Kogut, 2016).
In this work the effect of dietary supplement with prebiotics derived from yeast wall (mannan-oligosaccharide and nucleotide) on the cecal microbiota of broilers was evaluated by the massive parallel sequencing of the 16S rRNA gene.
Materials and Methods
Experimental Design and Sampling
Thirty female newborn chicks (Hubbart®) were placed on wood shavings litter for 35 days. Water and feed were given ad libitum. At arrival, chicks were randomly divided in groups for three different types of treatment (10 birds per treatment): treatment without additives in the feed (Neg), treatment with the prebiotics mannan-oligosaccharide (Mos), or nucleotides (Nuc). 200 g/ton of each prebiotic were incorporated in the ration from the first day until the 35th day. Briefly, the prebiotics used in this work were extracted from biomass derived from the sugar and beer industry. These by-products contain abundant in Saccharomyces cerevisiae yeast. The raw-matter was treated by industrial autolysis, obtaining two fractions. The first, composed mainly for yeast wall, was rich in mannan-oligosaccharides. The second fraction contained soluble yeast extract and was abundant in nucleotides (Chaud and Sgarbieri, 2006). All animal procedures were approved by the Animal Experimentation Ethics Committee of the Federal University of Paraná (authorization CEUA-Bio UFPR 898/2015). At 14 and 35 days of age, the chicks' body weights were recorded and samples were collected for DNA extraction, purification and sequencing. Immediately after euthanasia, the abdominal cavity was exposed and the cecum was dissected from the other intestinal sections. The cecum from each bird was cut open, and the contents were collected in a sterile 2-ml tube, stored on ice and later frozen and stored at −80°C until use. In total, 24 samples for cecal contents were collected (four chickens per treatment at 14 and 35 days of age).
DNA Extraction, 16S rRNA Gene Amplification and Sequencing
Genomic DNA from each sample was isolated from 200 mg of cecal luminal content using PowerFecal® DNA Isolation Kit (MO BIO laboratories, Inc.). The variable V4 region of 16S rRNA gene was amplified using the universal primers 515F and 806R (Caporaso et al., 2011) and KlenTaq Master Mix (Sigma). The PCR conditions used were 94°C for 3 min; 18 cycles of 94°C for 45 s, 50°C for 30 s and 68°C for 60 s; followed by 72°C for 10 min. The amplicons were quantified with Qubit using HS dsDNA kit (Invitrogen), diluted to 500pM and pooled. Then, 16pM of pooled DNA were sequenced using MiSeq reagent 500V2. Sequencing was performed using an Illumina MiSeq® sequencer (Illumina) obtaining paired-end reads of 250 bp as described (Caporaso et al., 2011). The dataset were submitted in NCBI site under the BioSample accession code SAMN07211773 (http://www.ncbi.nlm.nih.gov/biosample/7211773).
Diversity Analysis
Sequencing data were analyzed with the QIIME pipeline (Caporaso et al., 2010). Since the Illumina output ranged from approximately 40,000 to 172,499 reads, the read number was re-sampled to 41,800 reads per sample, allowing for the diversity comparisons. Sequences were quality filtered and identified at the phyla and genus levels using the open-reference OTU method implemented in QIIME and the SILVA database (123 release) (Yilmaz et al., 2013; Rideout et al., 2014). Basic diversity analysis (OTU number, Unifrac-distances and cluster by Neighbor-Joining method) was conducted using QIIME, and the OTU table exported to R for further analyses.
Statistical Analysis
Statistical analyses were performed using the Stats package included in RStudio software (RStudio-Team, 2015). First, data was explored using multivariate tools. Weighted unifrac-distances previously obtained from QIIME were used to sample ordination using PCoA and cluster analyzes. Since the samples showed a non-parametric distribution by the Shapiro Wilk test, the genera previously identified were compared according to the prebiotic treatments using the Kruskal Walli's test. Only statically significant results were reported (P < 0.05).
Results
The use of the prebiotics Mos and Nuc resulted in no changes in the body weight of the birds in 14 or 35 days (not shown). However, they did cause several changes in the gut microbiota. Using multivariate analysis, small changes in the microbiota were observed at 14 days, but differences were clearer after 35 days (Table 1). At 14 days, Firmicutes was the most predominant phylum in all treatments and, on average, accounted for 84.5% of identified sequences, followed by Proteobacteria (7.9%) and Tenericutes (4.3%).
After 35 days, Firmicutes remained the most predominant phylum in cecum in all treatments and, on average, accounted for 61.4% of all the bacterial sequences, followed by Bacteroidetes (29%), Proteobacteria (4.5%), and Tenericutes (4.2%). Interestingly, the phylum Bacteroidetes was only detected at 35 days (Supplementary Material 1). The prevalence of Firmicutes at 14 days might be explained by the higher need of butyrate by the young chicken growing intestine (Van der Wielen et al., 2000). Since in adult chickens maximal butyrate production is no longer required, digestion of complex polysaccharides by representatives of Bacteroidetes results in the production of both propionate and butyrate, which might be a more advantageous balance of nutrients (Polansky et al., 2016). In agreement with this assumption, Polansky et al. (2016) observed a gradual increase of Bacteroidetes counts in the cecum from the third week of life of chickens.
By further dissecting the 16S ribosomal sequences, a total of 665 OTUs corresponding to bacterial genera were detected, and were identified using the SILVA database (release 123) (Supplementary Material 2). Among all identified bacterial genera in the cecal microbiota, the most abundant in all treatments were Faecalibacterium, Bacteroides, Lactobacillus, Streptococcus, Lachnoclostridium, Anaerotruncus, Ruminiclostridium 5, Subdoligranulum, Parabacteroides, Escherichia-Shigella, and Alistipes (Supplementary Material 2). At 14 days, the most prevalent genera in all groups were Faecalibacterium accounting for, on average, 20.3% of all cecal bacterial sequences. The second most prevalent genera was Lactobacillus with 6.65% of bacterial sequences, followed by Subdoligranulum (5.42%) (Table 1). At 35 days, Bacteroides was the most predominant genera in all treatments, accounting for, on average, 21.5% of the cecal sequences. The second most prevalent was Anaerotruncus (5.59%), followed by Streptococcus (4.93%). Besides, at 35 days, the frequencies of 130 genera significantly differed between treatments. Over time an increase in the count of sequences was observed in Bacteroides, Anaerotruncus, and Alistipes genera. Interestingly, the Parabacteroides genus increased at 35 days only in the Mos treated group, while the genera Faecalibacterium, Lachnoclostridium, Ruminiclostridium 5, and Subdoligranulum decreased at 35 days (Supplementary Material 3).
Moreover, Principal Component Analysis of OTUs representation clearly showed the age explained most of the differences (57.8%), followed by treatment (17.4%) (Figure 1). This result was also observed in cluster analysis of microbial community by the Neighbor-Joining method (Supplementary Material 4).
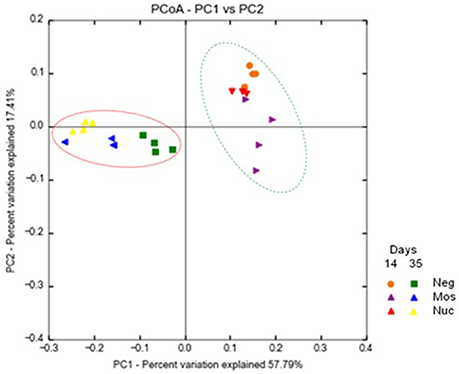
Figure 1. Principal Component Analysis (PCoA) of broiler's cecal bacterial community fed with yeast-derived prebiotics. Control group (Neg) mannan-oligosaccharide (Mos) and nucleotide (Nuc) are shown. Samples were compared according to the out composition at the genus level as identified by the SILVA database (SILVA 123 release) using the Unifrac distance weighted method.
The use of Nuc as prebiotics significantly increased the count of sequences of Lactobacillus and significantly decreased the count of sequences of Escherichia-Shigella at 35 day. On the other hand, the use of prebiotic Mos significantly increased the count of sequences of Butyricimonas and Roseburia genera at 35 day (Supplementary Material 6). The supplementation with both prebiotics significantly decreased the Chao1 index at 35 days, when compared to the negative group. This index is a species richness estimator, which estimates the total number of species present in a community (Supplementary Material 5).
Conclusion
In conclusion the composition of cecal microbiota is not constant and develops over time in chickens. Moreover, prebiotic supplementation significantly affected the microbial community structure in the cecum in broiler chickens with most significant shifts at 35 days. Although the prebiotics Mos and Nuc caused a decrease the richness and the diversity in the cecum, the prevalence of beneficial bacteria increased in both treatments. Nuc increased the count of sequences of Lactobacillus and decreased the count of sequences of Escherichia-Shigella, while Mos significantly increased the count of sequences of Butyricimonas and Roseburia. The Lactobacillus, Butyricimonas, and Roseburia genera are known for producing short-chain fatty acids (SCFAs) and have shown beneficial effects on the host's development and health (Pryde et al., 2002; Farnworth, 2005; Jhangi et al., 2014; Loman and Tappenden, 2016).
Author Contributions
MN provided the prebiotics. DM and DL analyzed the data and performed the numerical and statistical analyses. DM and ES designed the work and wrote the manuscript. DL and LMC processed the sequence raw data. CS and LFC designed the protocol. DM performed DA extraction from samples. EB performed amplicons library preparation and sequencing. FP and ES supervised the work.
Conflict of Interest Statement
The authors declare that the research was conducted in the absence of any commercial or financial relationships that could be construed as a potential conflict of interest.
Acknowledgments
To CAPES/PROEX by the doctoral scholarship.
Supplementary Material
The Supplementary Material for this article can be found online at: https://www.frontiersin.org/articles/10.3389/fgene.2017.00153/full#supplementary-material
References
Baurhoo, B., Ferket, P. R., and Zhao, X. (2009). Effects of diets containing different concentrations of mannanoligosaccharide or antibiotics on growth performance, intestinal development, cecal and litter microbial populations, and carcass parameters of broilers. Poul. Sci. 88, 2262–2272. doi: 10.3382/ps.2008-00562
Borody, T. J., and Khoruts, A. (2012). Fecal microbiota transplantation and emerging applications. Nat. Rev. Gastroenterol. Hepatol. 9, 88–96. doi: 10.1038/nrgastro.2011.244
Caporaso, J. G., Kuczynski, J., Stombaugh, J., Bittinger, K., Bushman, F. D., Costello, E. K., et al. (2010). QIIME allows analysis of high-throughput community sequencing data. Nat. Methods 7, 335–336. doi: 10.1038/nmeth.f.303
Caporaso, J. G., Lauber, C. L., Walters, W. A., Berg-Lyons, D., Lozupone, C. A., Turnbaugh, P. J., et al. (2011). Global patterns of 16S rRNA diversity at a depth of millions of sequences per sample. Proc. Natl. Acad. Sci. U.S.A. 108(Suppl. 1), 4516–4522. doi: 10.1073/pnas.1000080107
Chaud, S. G., and Sgarbieri, V. C. (2006). Propriedades funcionais (tecnológicas) da parede celular de leveduras da fermentação alcoólica e das frações glicana, manana e glicoproteína. Food Sci. Technol. 26, 369–379. doi: 10.1590/S0101-20612006000200020
Cross, D. E., McDevitt, R. M., Hillman, K., and Acamovic, T. (2007). The effect of herbs and their associated essential oils on performance, dietary digestibility and gut microflora in chickens from 7 to 28 days of age. Br. Poul. Sci. 48, 496–506. doi: 10.1080/00071660701463221
Farnworth, E. R. (2005). The Beneficial health effects of fermented foods-potential probiotics around the world. J. Nutraceut. Funct. Med. Foods 4, 93–117. doi: 10.1300/J133v04n03_07
Fernandez, F., Hinton, M., and Gils, B. V. (2002). Dietary mannan-oligosaccharides and their effect on chicken caecal microflora in relation to Salmonella Enteritidis colonization. Avian Pathol. 31, 49–58. doi: 10.1080/03079450120106000
Gibson, G. R., Probert, H. M., Loo, J. V., Rastall, R. A., and Roberfroid, M. B. (2004). Dietary modulation of the human colonic microbiota: updating the concept of prebiotics. Nut. Res. Rev. 17, 259–275. doi: 10.1079/NRR200479
Gibson, G. R., and Roberfroid, M. B. (1995). Dietary modulation of the human colonic microbiota: introducing the concept of prebiotics. J. Nut. 125, 1401–1412.
Gong, J., Forster, R. J., Yu, H., Chambers, J. R., Wheatcroft, R., Sabour, P. M., et al. (2002). Molecular analysis of bacterial populations in the ileum of broiler chickens and comparison with bacteria in the cecum. FEMS Microbiol. Ecol. 41, 171–179. doi: 10.1111/j.1574-6941.2002.tb00978.x
Hamilton, M. J., Weingarden, A. R., Unno, T., Khoruts, A., and Sadowsky, M. J. (2013). High-throughput DNA sequence analysis reveals stable engraftment of gut microbiota following transplantation of previously frozen fecal bacteria. Gut Microbes 4, 125–135. doi: 10.4161/gmic.23571
Huyghebaert, G., Ducatelle, R., and Immerseel, F. V. (2011). An update on alternatives to antimicrobial growth promoters for broilers. Vet. J. 187, 182–188. doi: 10.1016/j.tvjl.2010.03.003
Jhangi, S., Gandhi, R., Glanz, B., Cook, S., Nejad, P., Ward, D., et al. (2014). Increased archaea species and changes with therapy in gut microbiome of multiple sclerosis subjects (S24.001). Neurology 82(10 Suppl.). Available online at: http://www.neurology.org/content/82/10_Supplement/S24.001.short
Khoruts, A., Dicksved, J., Jansson, J. K., and Sadowsky, M. J. (2010). Changes in the composition of the human fecal microbiome after bacteriotherapy for recurrent clostridium difficile-associated diarrhea. J. Clin. Gastroenterol. 44, 354–360. doi: 10.1097/MCG.0b013e3181c87e02
Loman, B. R., and Tappenden, K. A. (2016). Prebiotic short-chain fructooligosaccharides (scFOS) increases abundance of the butyrate producing microbial community differentially when administered with or without probiotic lactobacillus rhamnosus GG (LGG) in piglets with short-bowel syndrome (SBS). FASEB J. 30(1 Suppl.), 683–685. Available online at: http://www.fasebj.org/content/30/1_Supplement/683.5.short
Oakley, B. B., and Kogut, M. H. (2016). Spatial and temporal changes in the broiler chicken cecal and fecal microbiomes and correlations of bacterial taxa with cytokine gene expression. Front. Vet. Sci. 3:11. doi: 10.3389/fvets.2016.00011
Polansky, O., Sekelova, Z., Faldynova, M., Sebkova, A., Sisak, F., and Rychlik, I. (2016). Important metabolic pathways and biological processes expressed by chicken cecal microbiota. Appl. Environ. Microbiol. 82, 1569–1576. doi: 10.1128/AEM.03473-15
Pryde, S. E., Duncan, S. H., Hold, G. L., Stewart, C. S., and Flint, H. J. (2002). The microbiology of butyrate formation in the human colon. FEMS Microbiol. Lett. 217, 133–139. doi: 10.1111/j.1574-6968.2002.tb11467.x
RStudio-Team (2015). RStudio: Integrated Development for R. Boston, MA: RStudio Team. Available online at: www.rstudio.com
Rideout, J. R., He, Y., Navas-Molina, J. A., Walters, W. A., Ursell, L. K., Gibbons, S. M., et al. (2014). Subsampled open-reference clustering creates consistent, comprehensive OTU definitions and scales to billions of sequences. PeerJ 2:e545. doi: 10.7717/peerj.545
Spring, P., Wenk, C., Dawson, K. A., and Newman, K. E. (2000). The effects of dietary mannaoligosaccharides on cecal parameters and the concentrations of enteric bacteria in the ceca of salmonella-challenged broiler chicks. Poul. Sci. 79, 205–211. doi: 10.1093/ps/79.2.205
Van der Wielen, P. W. J. J., Biesterveld, S., Notermans, S., Hofstra, H., Urlings, B. A. P., and van Knapen, F. (2000). Role of volatile fatty acids in development of the cecal microflora in broiler chickens during growth. Appl. Environ. Microbiol. 66, 2536–2540. doi: 10.1128/AEM.66.6.2536-2540.2000
Van Nood, E., Vrieze, A., Nieuwdorp, M., Fuentes, S., Zoetendal, E. G., de Vos, W. M., et al. (2013). Duodenal infusion of donor feces for recurrent clostridium difficile. N. Eng. J. Med. 368, 407–415. doi: 10.1056/NEJMoa1205037
Yilmaz, P., Parfrey, L. W., Yarza, P., Gerken, J., Pruesse, E., Quast, C., et al. (2013). The SILVA and “All-species Living Tree Project (LTP)” taxonomic frameworks. Nucleic Acids Res. 42, D643–D648. doi: 10.1093/nar/gkt1209
Keywords: mannan-oligosaccharides, chickens, gut microbiota, 16S rRNA, lactobacillus
Citation: Mesa D, Lammel DR, Balsanelli E, Sena C, Noseda MD, Caron LF, Cruz LM, Pedrosa FO and Souza EM (2017) Cecal Microbiota in Broilers Fed with Prebiotics. Front. Genet. 8:153. doi: 10.3389/fgene.2017.00153
Received: 12 June 2017; Accepted: 29 September 2017;
Published: 17 October 2017.
Edited by:
Peter Dovc, University of Ljubljana, SloveniaReviewed by:
Kieran G. Meade, Teagasc, The Irish Agriculture and Food Development Authority, IrelandShaojun Liu, Hunan Normal University, China
Copyright © 2017 Mesa, Lammel, Balsanelli, Sena, Noseda, Caron, Cruz, Pedrosa and Souza. This is an open-access article distributed under the terms of the Creative Commons Attribution License (CC BY). The use, distribution or reproduction in other forums is permitted, provided the original author(s) or licensor are credited and that the original publication in this journal is cited, in accordance with accepted academic practice. No use, distribution or reproduction is permitted which does not comply with these terms.
*Correspondence: Dany Mesa, ZG1lc2FmQHVuYWwuZWR1LmNv
Emanuel M. Souza, c291emFlbUB1ZnByLmJy