- 1Department of Entomology, Michigan State University, East Lansing, MI, United States
- 2USDA-ARS, Corn Insects and Crop Genetics Research Unit, Ames, IA, United States
Ten constitutively differentially expressed miRNAs were previously described between DDT-resistant 91-R and -susceptible control Drosophila melanogaster strains, and among their predicted target genes were those associated with metabolic DDT resistance mechanisms. The present study evaluated the inducibility of miRNA expression and putative downstream regulation of cytochrome P450s in response to DDT exposure in a time-dependent manner in 91-R and the susceptible Canton-S strain. Specifically, RT-qPCR analysis showed that DDT exposures led to the significant down-regulation (repression) of miR-310-3p, miR-311-3p, miR-312-3p, miR-313-3p, and miR-92a-3p levels in Canton-S. This is contrasted with the lack of significant changes in 91-R at most time-points following DDT exposure. The levels of expression among miRNAs exhibited opposite expression patterns compared to their corresponding putative target cytochrome P450s at the same time points after DDT exposure. Collectively, results from this study suggest that miR-310-3p, miR-311-3p, miR-312-3p, miR-313-3p, and miR-92a-3p might have a potential role in the control of DDT detoxification through the post-transcriptional regulation of target cytochrome P450s in Canton-S. Conversely, the lack of significant changes of these same miRNAs in 91-R following DDT-exposure suggests a possible adaptive mutation that removes repressive control mechanisms. These data are important for the understanding impact of adaptive changes in miRNA expression on post-transcriptional regulatory mechanism involved in the evolution of DDT resistance in 91-R.
Introduction
For well over half a century, second generation insecticides have been used across the globe as one of the most cost-effective ways to control pest insects, directly impacting human health through methods of crop production and the management of vector-borne diseases. However, the extensive and frequent application of insecticide has contributed to the evolution of insect populations with resistance to one or more classes of insecticides (Mallet, 1989). The organochlorine insecticide dichlorodiphenyltrichloroethane (DDT) was widely used in the decades post-WWII for the control of agricultural and disease vectoring pest insects (Edman, 2004; Hemingway, 2009), however, it was ultimately banned in most countries due to deleterious environmental side effects as well as its impacts on non-target mammalian and avian species (Krupke et al., 2007). Although DDT is no longer used for agricultural pest control, DDT resistance in the fruit fly, Drosophila melanogaster (hereafter referred to as Drosophila), provides a model system for studying the evolution of insecticide resistance (Wilson, 1988). In some Drosophila strains, historically, moderate and high levels of DDT resistance have been considered to be polygenic and associated with contribution of multiple resistance genes to the phenotype (Kim et al., 2018), including metabolic-based resistance influenced by the alteration of enzyme activities that degrade or sequester insecticides (Li et al., 2007). Metabolic resistance to DDT in Drosophila has been associated with constitutively up-regulated genes and increased quantities of cytochrome P450s (P450s), glutathione-S-transferases (GSTs), and esterases (Feyereisen, 1999; Vontas et al., 2002; Tu and Akgul, 2005). DDT resistance in Drosophila also is dependent upon expression of a Phase III detoxification gene, the ABC transporter, which likely may enhance excretion of xenobiotic metabolites through the up-regulation of mdr50 and mdr65 (Strycharz et al., 2013) and the structural change of mdr49 in DDT resistant phenotypes (Seong et al., 2016). Furthermore, DDT resistance in the Drosophila strain 91-R has been associated with genetic and signal transduction pathways that converge to modify stress response, cell survival, and neurological functions (Seong et al., 2017).
A large number of studies have focused on the role of P450s in the detoxification of xenobiotic compounds in insect species. Much of the DDT resistance observed in Drosophila has been attributed to constitutive increases in expression of the P450s Cyp6g1, Cyp6a2, and Cyp12d1 (Festucci-Buselli et al., 2005; Kuruganti et al., 2007; Jones et al., 2010), and amino acid changes in CYP6W1 involved in the metabolism of DDT resistance phenotype (Schmidt et al., 2010). Seong et al. (2018a) recently described the evolutionary adaptive response of functional and structural variation within the P450 gene family as a result of chronic high levels of DDT exposure in the DDT-resistant strain 91-R when compared to its control strain, 91-C. Additionally, the transcriptional regulation of P450s expression has become of the focus of recent studies to better understand the underlying mechanisms involved in constitutive over-transcription of P450 genes in pesticide resistant insects. For example, the Nrf2/Maf transcription factor-mediated regulatory pathway governing Cyp6a2 gene up-regulation is associated with DDT resistance in Drosophila (Wan et al., 2014). More recent studies have demonstrated the functional roles of microRNAs (miRNAs) in regulating the expression of P450s that are in turn involved in insecticide resistance in insect species (Hong et al., 2014; Etebari et al., 2018).
The miRNAs are endogenous small non-coding RNA species that are generally 19–25 nucleotides in length, and function in the post-transcriptional regulation of gene expression by directly targeting to the 3′ untranslated region (3′ UTR) of mRNA targets (Bartel, 2004). miRNAs can play important regulatory roles in biological processes including development, immunity, and metamorphosis (Ambros, 2004; Wei et al., 2018). Several studies have characterized the role of miRNAs in responses to environmental chemical and pathogen exposures. For instance, a number of miRNAs are differentially expressed in a dose-dependent response to copper concentrations in zebrafish and these up/down-regulated miRNAs were predicted to post-transcriptionally control of transcripts involved in olfactory signal transduction pathways and neurological processes (Wang et al., 2013). miRNAs also have complex interactions with Drosophila innate immune systems in response to bacterial infections (Wei et al., 2018), which can lead to dynamic changes in miRNA expression and downstream regulation of multiple mRNAs targets involved in Drosophila immune response. Strikingly, miRNAs have attracted considerable attention for their involvement in mechanisms of resistance to insecticides such as DDT (Seong et al., 2018b), permethrin (pyrethroid) (Ma et al., 2017), fenpropathrin (Zhang et al., 2016), and imidacloprid (Morin et al., 2017). A number of studies have shown that several miRNAs are significantly differentially-expressed in insects following exposure to different insecticides, and a subset of their putative target genes are involved metabolic detoxification processes. For example, quantitative comparison of miRNA libraries from chlorantraniliprole-resistant and -susceptible strains of Plutella xylostella revealed that a number of miRNAs were differentially expressed and their predicted target genes were associated with chlorantraniliprole resistance mechanisms (Zhu et al., 2017). Furthermore, miR-2b-3p was predicted to regulate the expression of a putative P450 target gene, Cyp9f2, in P. xylostella (Etebari et al., 2018). In a recent study, levels of Cyp9j5 and Cyp325bg3 transcripts in Culex pipiens were significantly decreased upon miR-2∼13∼71 cluster mimic injection, which demonstrated the regulation of these target genes by a regulatory miRNA (Guo et al., 2017). Moreover, miR-1-3p may be involved in cyflumetofen resistance by targeting an mRNA encoding a GST in Tetranychus cinnabarinus (Zhang et al., 2018).
A number of studies suggest that the induction of detoxification genes, such as P450s, play a critical role in the metabolism of insecticide in insects and may also be adaptive (Liu et al., 2011; Gong et al., 2013). However, the role of insecticide induced miRNA expression in the post-transcriptional regulation of genes associated with insecticide resistance in Drosophila remains poorly understood. Our previous studies comparing constitutive miRNA expression levels between DDT-resistant and -susceptible Drosophila strains identified multiple up- and down-regulated miRNAs predicted to potentially contribute for the development of DDT resistance via targeting transcripts of multiple detoxification genes. Conversely, there has been no corresponding studies demonstrating any inducible miRNA response to pesticide exposure in pesticide resistant and susceptible strains. In the present study, the induction or repression of miRNAs in response to DDT exposure is demonstrated in the DDT-resistant 91-R and -susceptible Canton-S Drosophila strains and are putatively linked to the regulation of transcript levels of their target P450 genes. The study also reports that, when compared to their susceptible counterpart, the DDT resistance phenotype in 91-R is associated with constitutively lower levels of the same miRNAs. These data are important for understanding the potential roles of differential miRNA expression on the evolution of DDT resistance in the Drosophila strains, and may provide insight into processes that lead to the evolution of phenotypes adapted to increased survivorship when exposed to xenobiotics.
Materials and Methods
Drosophila Strains
For the purposes of this study, two Drosophila strains, 91-R and Canton-S, were reared and used as previously described (Seong et al., 2017). The 91-R strain has remained under continual selection by maintaining the flies in bottles with filter paper disks impregnated with 150 mg/ml of DDT, while Canton-S has been maintained without any exposure to insecticides. The 91-R strain was previously shown to be 1,526-fold more resistant to DDT compared to the Canton-S susceptible strain using contact vial bioassays (Strycharz et al., 2013).
DDT Treatment
To determine Drosophila miRNA responses to DDT, the response of miRNA expression was determined by using DDT contact exposure bioassays as described previously (Seong et al., 2016). In brief, 15 3-day-old female adult flies from each the 91-R and Canton-S strains were exposed to vials coated with DDT at their respective LC10 (5 mg/vial for 91-R; 1.5 μg/vial for Canton-S), and samples taken at 12, 24, 48, and 72 h after the DDT exposure for each strain. Control flies that had not received the DDT treatment (treated with acetone alone) were collected at the same time points as DDT treatment counterparts. Each treatment by time point experiment was performed in triplicate.
RNA Extraction and cDNA Preparation
Total RNA was extracted per replicate from a pool of 15 flies surviving DDT treatment and acetone treatment using the Qiagen miRNeasy Mini Kit according to the manufacturer’s instructions (Qiagen, Valencia, CA, United States), which was followed by treatment of each sample with DNase I (Qiagen) before cDNA synthesis to remove contaminating genomic DNA. First-strand cDNA was synthesized using the miScript II RT kit (Qiagen) with the provided miScript HiFlex Buffer according to the manufacturer’s instructions.
Gene Expression by Reverse Transcriptase-Quantitative PCR (RT-qPCR)
RT-qPCR validation was performed on eight miRNAs (miR-310-3p, miR-311-3p, miR-312-3p, miR-313-3p, miR-92a-3p, miR-286-3p, miR-4919-3p, and miR-986-5p), which were estimated to be constitutively differentially expressed between DDT-resistant 91-R and -susceptible 91-C strains by Seong et al. (2018b). RT-qPCR reactions were performed using the miScript SYBR® Green PCR Kit (Qiagen) according to the manufacturer’s instructions with miRNA-specific forward primers and target mRNA-specific primers (Supplementary Table S1). All RT-qPCR reactions were carried out on a StepOnePlus Real-Time PCR system (Applied Biosystems, Inc., Foster City, CA, United States), with three technical replicates across all biological replicates. Normalized miRNA and target transcript expression levels were calculated using the 2-ΔΔC(t) method (Schmittgen and Livak, 2008) with the references U6 snRNA and 5S rRNA for miRNA normalization and rp49 for target mRNA normalization. The amplification efficiency of target miRNAs, mRNAs and reference genes were estimated using the formula: E = 10(-1/slope) where slope was derived from plotting the cycle threshold (Ct) value versus five serially 10n-fold diluted cDNA template concentrations. RT-qPCR was performed across three biological replicates. The statistical analysis of gene expression was performed using a two-way analysis of variance (ANOVA) to determine the changes in miRNA expression level through DDT exposure is dependent on the genetic background with Tukey’s multiple comparison test (XLSTAT 2008, Addinsoft, United States); a P-value < 0.05 was considered statistically significant. Pearson correlation coefficients between a particular miRNA and its predicted target P450 mRNAs were computed to determine whether the expression levels of each miRNA and of its mRNA targets were negatively correlated. Correlation were considered significant at P < 0.05.
Potential Target P450s Prediction of Eight Differentially Expressed miRNAs
The putative P450 target transcripts of miRNAs investigated in this study were previously predicted using three different types of software packages (RNAhybrid, DIANA, and ComiR) as described by Seong et al. (2018b) and another software, miRanda, to predict potential target P450s (Betel et al., 2010). In brief, for each prediction method, high efficacy targets were selected by the following criteria: (1) RNAhybrid: the target site prediction was restricted to the 3′-UTR region obtained from the 3′-UTR database of D. melanogaster (dmel-all-three_prime_UTR-r6.19.fasta at http://www.flybase.org) with MFE ≤-30 kcal mol-1; (2) DIANA: miTG score ≥ 0.8; (3) ComiR: high threshold ≥ 0.8; (4) miRanda: total score ≥ 120, total energy ≤-25 kcal/mol.
Results
Time-Dependent Expression of Multiple miRNAs in Response to DDT Exposure in DDT-Resistant 91-R and -Susceptible Canton-S Strains
Among 10 miRNAs previously shown to be differentially expressed between 91-R and 91-C strains (Seong et al., 2018b), eight miRNAs (miR-310-3p, miR-311-3p, miR-312-3p, miR-313-3p, miR-92a-3p, miR-286-3p, miR-4919-3p, and miR-986-5p) showed significant levels of differential expression and were herein investigated with respect to induction or repression in DDT resistant 91-R and DDT susceptible Canton-S strains following DDT exposure. No significant changes were detected in miRNA expression (induction or repression) for any of the eight miRNAs within acetone-treated control flies from either strain across any sampling time points (P-values ≥ 0.05; Supplementary Table S2). In contrast, five miRNAs (miR-310-3p, miR-311-3p, miR-312-3p, miR-313-3p, miR-92a-3p) were significantly down-regulated following DDT exposure in Canton-S strain as compared to acetone treated controls. Repression of these miRNAs occurred universally across 12 to 72 h after DDT exposure (P-values < 0.05).
Specifically, the initial repression of miR-311-3p in Canton-S occurred at 12 h (0.78-fold), followed by a 0.39- and 0.35-fold decreases in expression respectively at 24 and 48 h after DDT treatment compared to the acetone-treated control flies (Figure 1B). Similarly, the initial down-regulation of miR-310-3p, miR-312-3p, miR-313-3p, and miR-92a-3p occurred at 24 h after exposure to DDT with respective down-regulation of 0.48-, 0.35-, 0.29-, and 0.5-fold as compared to the control flies (Figures 1A,C–E). In the Canton-S strain, the expression of miR-310-3p, miR-311-3p, miR-312-3p, and miR-92a-3p were most highly down-regulated at 48 h after exposure to DDT with reduced levels respectively of 0.41-, 0.35-, 0.32-, and 0.39-fold (Figures 1A–C,E). Comparatively, miR-313-3p was most highly down-regulated in Canton-S at 24 h after DDT treatment with a reduced level of 0.29-fold (Figure 1D). At 72 h after DDT exposure, the expression of miR-310-3p, miR-311-3p, and miR-313-3p showed no significant difference compared with acetone-treated flies (Figures 1A,B,D), whereas the miR-312-3p and miR-92a-3p remained down-regulated after 72 h post-DDT treatment in Canton-S with approximate 0.6- and 0.42-fold reductions (Figures 1C,E). In contrast, the estimated expression of miR-986-5p increased by approximately 2.6-fold at 24 h after DDT treatment in Canton-S strain (Figure 2). Both miR-286-3p and miR-4919-3p showed no significant difference at any time points after DDT treatment as compared to the acetone-treated control (P-values ≥ 0.05) in both 91-R and Canton-S strains (Supplementary Figures S1, S2).
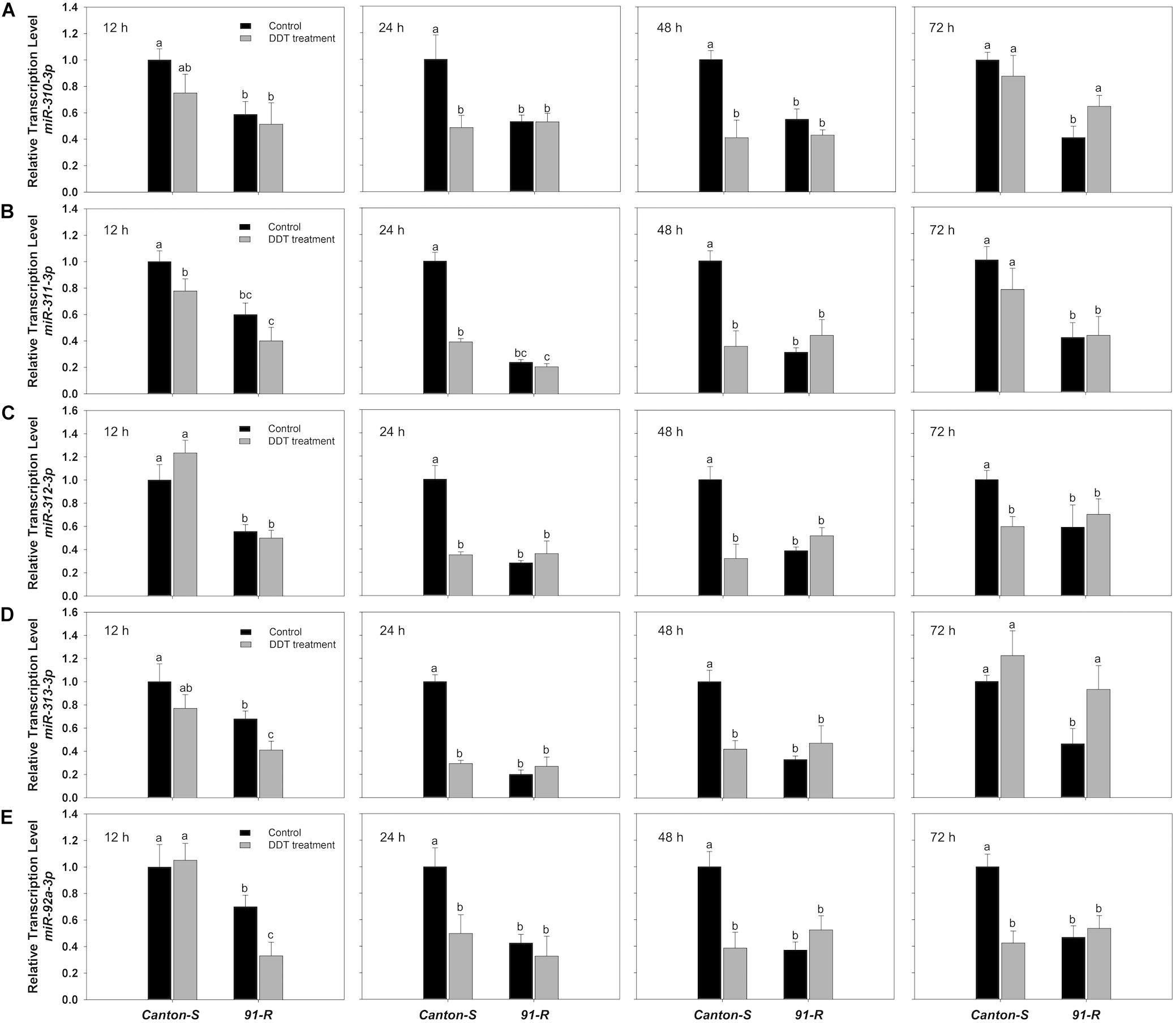
Figure 1. The expression of miR-310 family, (A) miR-310, (B) miR-311, (C) miR-312, (D) miR-313, and (E) miR-92a, in DDT-resistant 91-R and the DDT-susceptible Canton-S strains following DDT exposure. The induction or repression level of expression were analyzed 12, 24, 48, and 72 h after DDT exposure. The relative levels of gene expression shown along the Y axis represent the ratio of the gene expression in each treatment compared to the acetone-treated Canton-S strain. The experiments were repeated three times. The vertical bars indicate standard error of the mean (SEM). Different letters on the bars indicate that the means are significantly different throughout the strains where the P-value < 0.05.
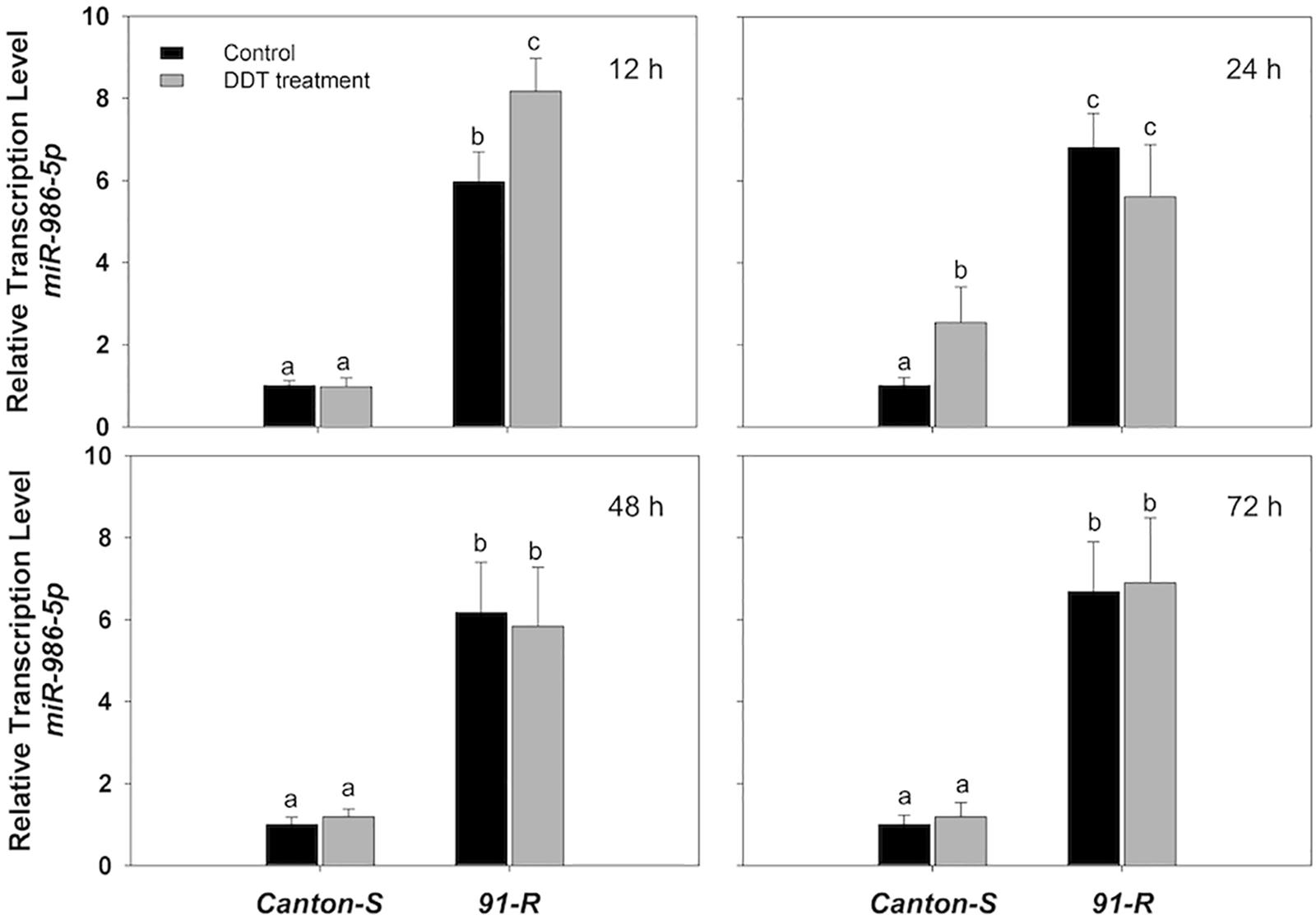
Figure 2. The expression of miR-986-5p in DDT-resistant 91-R and the DDT-susceptible Canton-S strains following DDT exposure. The induction or repression level of expression were analyzed 12, 24, 48, and 72 h after DDT exposure. The relative levels of gene expression shown along the Y axis represent the ratio of the gene expression in each treatment compared to the acetone-treated Canton-S strain. The experiments were repeated three times. The vertical bars indicate SEM. Different letters on the bars indicate that the means are significantly different throughout the strains where the P-value < 0.05.
The miRNAs in 91-R were constitutively expressed and for the most part showed non-significant degrees of change in response to DDT exposure. Specifically, analysis of RT-qPCR results indicated that all eight miRNAs showed significant levels of differential expression between 91-R and Canton-S across time points post-DDT exposure, whereby seven miRNAs were significantly down-regulated, and only one (miR-986-5p) was significantly up-regulated in 91-R as compared to Canton-S (P-values < 0.05). DDT exposure induced the up-regulation of two miRNAs (miR-310-3p and miR-313-3p) with varying levels and in a time-dependent manner in 91-R strain. The expression of miR-310-3p in 91-R strain reached maximum level of induction (1.59-fold) at 72 h after exposure to DDT (Figure 1A). Similarly for miR-313-3p, the significant induction level was also observed at 72 h with maximum expression levels of 2.0-fold (Figure 1D). Similar to Canton-S strain, down-regulation of miRNAs by DDT exposure was shown for miR-313-3p, and miR-92a-3p in 91-R strain only at 12 h after treatment of DDT with expression level reduced 0.6- and 0.42-fold, respectively, as compared to the acetone-treated control (Figures 1D,E). For both miR-286-3p and miR-4919-3p, no inducibility of expression was detected in 91-R tested at any time points after treatment with DDT at LC10 (Supplementary Figures S1, S2). In the 91-R strain, the initial induction of up-regulation resulting in a 1.37-fold change in of miR-986-5p occurred at 12 h after exposure to DDT, but no significant induction was found after 24 h following DDT treatment (Figure 2). Overall, the expression level of miRNAs in 91-R showed no significant difference at most time points after DDT treatment compared to the acetone-treated control (P-values ≥ 0.05). Notably, the two-way treatment × genotype statistical interaction showed that the differentiation of most miRNA expression induced by DDT exposure at each time point is dependent on the genetic background of fly strains (P-values < 0.05; Supplementary Table S3).
Co-expression Patterns Between miRNAs and Their Potential Target P450 mRNAs
Using the P450 sequences from the Drosophila, we predicted the target P450 gene of miRNAs using miRNA seed regions binding to the 3′ UTR of mRNAs. We found the 3′ UTR sequence of Cyp6g1, Cyp6g2, Cyp6a8, and Cyp4g1 contained a target site for miR-310-3p, 311-3p, 312-3p, 313-3p, and 92a-3p (Supplementary Figure S3). The relative expression of corresponding putative P450 targets of the eight miRNAs (Table 1) were estimated using RT-qPCR and correlations made with the expression levels estimated for corresponding miRNAs. Specifically, estimates of RT-qPCR showed that Cyp6g1 was induced significantly in Canton-S, with up-regulation of 6.4-fold at 24 h, 3.2-fold at 48 h, and 4-fold at 72 h after DDT treatment (Figure 3A). The corresponding relative expression level of Cyp6g2 was also significantly increased 1.8-, 2.11-, and 1.78-fold in Canton-S strain respectively at 24, 48, and 72 h after DDT exposure compared to control flies (Figure 3B). The relative expression level of Cyp6a8 in Canton-S was significantly up-regulated 8.0-, 6.1-, and 7.7-fold compared to control flies at 24, 48, and 72 h after DDT exposure (Figure 3C). Additionally, the expression of Cyp4g1 was significantly up-regulated 2.0- 1.5-, and 1.6-fold in Canton-S respectively at 24, 48, and 72 h after DDT exposure (Figure 3D). In general, expression of P450s generally was increased in response to DDT compared to control flies and remained significantly lower in Canton-S compared to 91-R strains across all time points following DDT-exposure (P-values < 0.05).
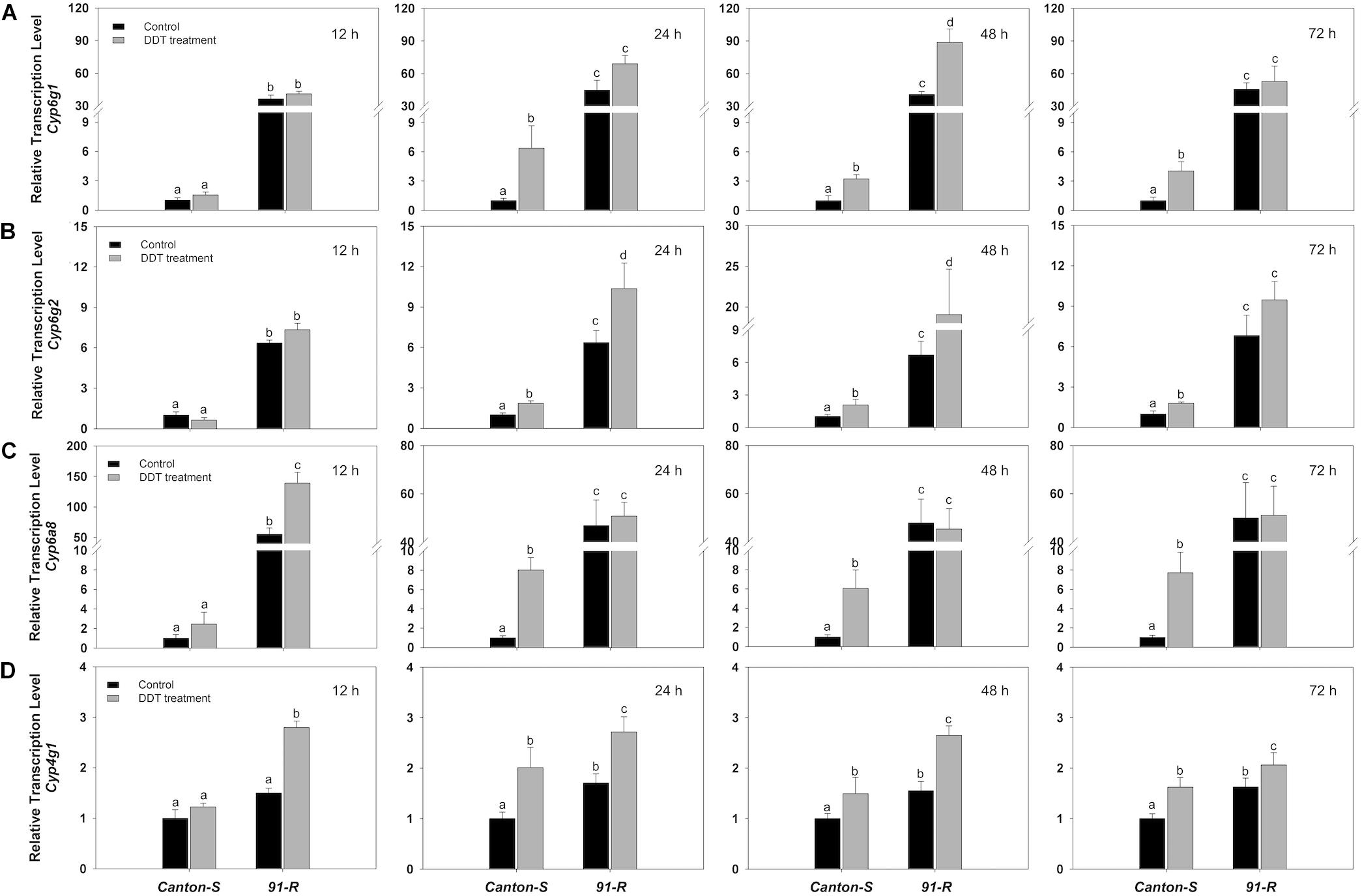
Figure 3. The expression of target P450s (A) Cyp6g1, (B) Cyp6g2, (C) Cyp6a8, and (D) Cyp4g1 in DDT-resistant 91-R and the DDT-susceptible Canton-S strains following DDT exposure. The induction or repression level of expression were analyzed 12, 24, 48, and 72 h after DDT exposure. The relative levels of gene expression shown along the Y axis represent the ratio of the gene expression in each treatment compared to the acetone-treated Canton-S strain. The experiments were repeated three times. The vertical bars indicate SEM. Different letters on the bars indicate that the means are significantly different throughout the strains where the P-value < 0.05.
The levels of expression among these putative target P450s for Canton-S exhibited opposite expression patterns with their corresponding miRNAs at the same time points after DDT exposure. For example, the relative expression level of Cyp6a8 in Canton-S was significantly up-regulated compared to control flies at 24, 48, and 72 h after DDT exposure. At the same time points, its corresponding miRNA, miR-312-3p, showed significant down-regulation in response to DDT exposure. Similarly, Cyp4g1 was predicted as a potential target for miR-312-3p and miR-92a-3p. The expressions of miR-312-3p and miR-92a-3p were significantly down-regulated at 24, 48, and 72 h in response to DDT exposure in Canton-S, while the expression of their corresponding target, Cyp4g1, was significantly up-regulated at the same time points after DDT exposure. Cyp6g2 was predicted as a putative target for miR-310-3p, miR-311-3p, miR-313-3p, and miR-92a-3p and the expression of Cyp6g2 was significantly up-regulated at 24, 48, and 72 h after DDT exposure, while the expressions of corresponding miRNAs were significantly down-regulated at 24 and 48 h for miR-310-3p and miR-313-3p, at 12, 24, and 48 h for miR-311-3p, and at 24, 48, and 72 h for miR-92a-3p in response to DDT exposure in Canton-S. Relative expression level of Cyp6g1 in Canton-S as well was significantly up-regulated compared to control flies at 24, 48, and 72 h after DDT exposure, while, at the same time points, the putative corresponding regulating miRNA, the miR-310-313 cluster, showed significant changes that were inversely related. Overall, although the correlation between miRNAs and their target mRNA expressions was not statistically significant (except a pair of miR-92a and Cyp6g2 : P-value = 0.014; Supplementary Table S4), mRNA expression of target P450s showed a negative relationship with the expression of their corresponding miRNAs at the same time points after DDT exposure.
Discussion
High-throughput small RNA (sRNA) sequencing from the DDT-resistant Drosophila strain, 91-R, previously revealed that 10 miRNAs were constitutively differentially expressed when compared with the susceptible control strain, 91-C, and predicted that these miRNAs putatively target a number of P450 gene transcripts (Seong et al., 2018b). The present study adds to this knowledge by showing that among these 10 differentially expressed miRNAs, miR-310-3p, miR-311-3p, miR-312-3p, miR-313-3p, and miR-92a-3p have significantly decreased levels of expression following DDT exposure at different time points in the wild-type DDT susceptible strain Canton-S. Furthermore, we showed that the expression of the putative target P450 targets, Cyp6a8, Cyp6g1, Cyp6g2, and Cyp4g1, have corresponding significantly increased levels of expression in Canton-S following DDT exposure, which is an inverse pattern of response compared to their predicted targeting miRNAs at some time points. Interestingly, our results demonstrate that significant differences in expression among the six miRNAs (miR-310-3p, miR-311-3p, miR-312-3p, miR-313-3p, miR-92a-3p, and miR-986-5p) between susceptible Canton-S and highly resistant 91-R strains are inducible following DDT exposures, as opposed to being constitutive. Specifically, this study showed that the exposure to DDT has no significant impact on miRNA expression levels in 91-R, whereas the same treatments led to a significant induction of down-regulation in miRNA expression in Canton-S at different time points (P-values < 0.05).
It was previously shown that miRNA levels are induced or repressed following animal exposures to chemical insecticides. For example, miR-21, miR-221, and miR-222 expression levels were estimated to be 2–3 times lower in DDT-treated rat liver compared to untreated controls (Gulyaeva et al., 2016). Recently, analogous levels of differential miRNA expression patterns were associated with insecticide resistance or were shown to be induced following exposure to insecticides. Specifically, the expression of miR-216b and miR-499 were down-regulated in Danio rerio following exposure to the phenylpyrazole insecticide, fipronil (Zhou et al., 2016). Comparative analyses have estimated significant levels of differential expression in 33 miRNAs when comparing imidacloprid-exposed and non-exposed control groups of the Colorado potato beetle, Leptinotarsa decemlineata (Morin et al., 2017). Additionally, following different concentrations and durations of exposure of P. xylostella to the ryanoid insecticide, chlorantraniliprole, several miRNAs were significantly changed in abundance (Etebari et al., 2018). These prior, and our current, results indicate that the inducible expression level changes of miRNAs in response to insecticides may play a potential role in arthropod responses to chemical insecticides exposures and that these miRNAs might be involved in the regulating the expression of multiple target genes facilitating survival in insect species.
In this study, five miRNAs were down-regulated in response to DDT exposure in Canton-S (miR-310-3p, miR-311-3p, miR-312-3p, miR-313-3p, and miR-92a-3p) belonging to the miR-310 family, which are clustered within the Drosophila genome. Although miR-313-3p is derived from the same precursor as -5p, the latter was not differentially expressed. This is consistent with recent evidence that despite these two miRNAs being derived from as a single precursor, miR-313-3p and -5p can show independent regulation (Zhao et al., 2018). Among these five miRNAs, the miR-310-313 cluster is required for regulating neurotransmitter release in motor neurons during synaptic growth in Drosophila larval development (Tsurudome et al., 2010). Additionally, the miR-310 family can down-regulate the escargot (esg) gene that is involved in nicotine sensitivity in Drosophila (Sanchez-Diaz et al., 2015). Sanchez-Diaz et al. (2015) observed that the over-expression of miR-310-313 led to a significant decrease in the esg transcription levels, thereby disrupting the function of sensory organs associated with chemical perception as well as cuticle development. The current research validated that the significant down-regulation of miR-310-313 was induced in wild-type DDT susceptible strain Canton-S following DDT exposure, suggesting the possibility that the miR-310-313 cluster may be regulated to mediate functional targets involved in neurotransmitter release in response to neurotoxicity of DDT. Interestingly, Seong et al. (2017) also demonstrated that differences in expression of transcripts involved in neurologic function are fixed between 91-R and its susceptible control strain, 91-C. This suggestively hints that a connection may lay between the differential expression of miR-310 family and potential differences in response of the nervous system to DDT exposures, but such a hypothesis still requires experimental testing.
Several P450 genes, including Cyp6a8, Cyp6g1, Cyp6g2, and Cyp4g1 were previously associated with metabolic resistance against DDT in Drosophila (Seong et al., 2018a). For example, Cyp6a8, Cyp6g1, and Cyp6g2 are constitutively up-regulated in DDT-resistant 91-R strain compared to susceptible counterparts (Pedra et al., 2004; Seong et al., 2017). Furthermore, it was previously shown that Cyp4g1 is constitutively up-regulated in the DDT-resistant 91-R strain compared to the insecticide-susceptible Canton-S in untreated conditions (Gellatly et al., 2015) and Cyp4g1 is located within a genome region previously defined as having undergone a selective sweep that reduced nucleotide diversities in 91-R as compared to the susceptible counterpart 91-C, and thus was putatively associated with the evolution of DDT resistance (Steele et al., 2015). Since Cyp4g1 is involved in endogenous metabolism that is responsible for the decarbonylation of long chain aldehydes to form cuticular hydrocarbons (Qiu et al., 2012), it could be possible that up-regulation functions to reduce DDT penetration through the cuticle. Findings from this current study further demonstrate that the expression of Cyp6a8, Cyp6g1, Cyp6g2, and Cyp4g1 are significantly induced after DDT exposure in susceptible Canton-S, which compares to their constitutive and higher relative expression in 91-R. Interestingly, the increased expression of the targeted P450s in Canton-S is accompanied by the significant down-regulation of their putative targeting miR-310-3p, miR-311-3p, miR-312-3p, miR-313-3p, and miR-92a-3p.
Prior research has implicated miRNAs in the post-transcriptional regulation of P450 expression levels through the binding of the 3′-UTRs of transcripts for the latter. For example, the expression of P450s Cyp9j35, Cyp325bg3, and Cyp6n23 is controlled by miR-2∼13∼71 cluster and miR-285, and this regulatory network is directly involved in pyrethroid insecticide resistance in Culex pipiens (Tian et al., 2016; Guo et al., 2017). Evidence from the current study demonstrate that the expression level of P450 genes and corresponding putative targeting miRNAs, miR-310-3p, miR-311-3p, miR-312-3p, miR-313-3p and miR-92a-3p, showed an inverse relationship at 24, 48, and 72 h after DDT exposure in the susceptible strain Canton-S. These associative data may suggest that the down-regulation of miRNAs could be involved in the regulation of P450 expression levels in Canton-S. However, these interactions need to be validated by functional experiments in order to confirm predicted interactions between miRNAs and putative target genes in vitro or in vivo. Therefore, the potential that these miRNAs are involved in post-transcriptional regulation of P450s, or modulate associated biochemical pathways which influence DDT resistance phenotypes remains unknown. Regardless, the inducible expression of miRNAs and their corresponding putatively targeted P450s in response to DDT exposure enticingly suggests the potential role of Drosophila miRNAs in coordinating detoxification responses during the DDT exposure or (alternatively) could point to their involvement in a general response to cellular stress pathway.
Temporal differences in the responses of miRNA expression following DDT exposure were predicted between 91-R and Canton-S strains. Specifically, some changes of miRNAs expression were transient in Canton-S in response to a low dose of DDT, possibly suggesting that miR-310-3p, miR-311-3p, miR-312-3p, miR-313-3p, and miR-92a-3p may form a response network that is sensitive to DDT exposure or general cellular stress. The involvement of these changes in the response of susceptible Drosophila to DDT detoxification remains undetermined, but might involve mechanisms for short-term cellular clearance of xenobiotics or responses to cellular stress conditions prior to the reestablishment of homeostasis. As stated above, the temporal expression level of these same miRNAs remains relatively unchanged at low levels in 91-R following the same DDT exposures. These results could suggest that reduced miRNA expression, and corresponding putative reduced levels of post-transcriptional repression of target P450s, may represent adaptive changes in response to multigenerational neurotoxic DDT selection in 91-R. Specifically, the selection for mutations in miRNA regulation that impact the degree to which miRNAs are induced following exposure to DDT may have become fixed in 91-R and resulted in the observed fixed differences in expression. Therefore, the constitutive down-regulation of multiple miRNAs and the general lack of induction following DDT in 91-R could be indicative of adaptive changes that modify cellular responses via underlying regulatory mechanisms of miRNA expression. Previous work analogously reported that inducible expression of detoxification genes, including P450s, GSTs or esterase genes, are not inducible in response to insecticide exposures, even though these genes have roles in insecticide resistance and metabolism in Drosophila (Willoughby et al., 2006). The specific mutations that give rise to these regulatory changes in miRNA expression in 91-R remain unknown, but undoubtedly will be the focus of future research.
Conclusion
Insects have metabolic detoxification systems that allow transient changes in response to variations in environmental conditions, including responses to chemical insecticide exposure. Furthermore, selection for mutations that enhance cellular detoxification of xenobiotics are known within insecticide-resistant phenotypes of various arthropod species. A number of studies indicate that both constitutively increased expression and induction of detoxification genes such as P450s, GSTs, and esterases in insects are linked or associated with insecticide resistance traits and the ability of arthropods to adapt to a changing environment (Russell et al., 2011; Ffrench-Constant, 2013). As such, miRNAs are now known as mediators of detoxification gene expression levels via post-transcriptional control, which has led to their candidacy as potential regulators of detoxification pathway (Wang and Cui, 2012; Yu and Cho, 2015). By integrating the expression of both differentially expressed miRNAs in conjunction with their predicted target P450s, this study identified an inverse pattern between the two in the DDT susceptible strain, Canton-S. In contrast, the relative level of expression among miRNAs remained lower and the P450 genes constitutively higher in 91-R compared to Canton-S.
As such, we demonstrated that inducible changes in miRNA in response to low-level DDT exposures may impact temporal expression levels of P450s in Canton-S, thus highlighting the importance of transient changes in expression levels in the response to cellular stress. Furthermore, this study showed the likely influence of adaptive changes in miRNA expression in response to DDT selection and the evolution of the resistant phenotype in 91-R. This study provides important information regarding the changes in miRNA expression levels and the regulation of detoxification genes in short term responses to low-level DDT exposures, as well as within adaptive phenotypes that are resistant to high levels of DDT phenotypes, and increases scientific understanding of potential involvement of miRNAs in the adaption of stress-response pathways in DDT resistant strains of Drosophila.
Author Contributions
KS performed most of the experiments with the help of BC and BP. KS analyzed the results and wrote the manuscript assisted by BC and BP. All authors reviewed and approved the manuscript.
Funding
This work was primarily supported by MSU Foundation and AgBioResearch funds provided to BP. Part of the research resulted from a joint contribution from the United States Department of Agriculture (USDA), Agricultural Research Service (ARS) (CRIS Project No. 5030-22000-018-00D), and the Iowa Agriculture and Home Economics Experiment Station, Ames, IA, United States (Project No. 3543). This article reports the review of current research only and any mention of products or services does not constitute an endorsement by USDA-ARS. USDA-ARS is an equal opportunity employer and provider.
Conflict of Interest Statement
The authors declare that the research was conducted in the absence of any commercial or financial relationships that could be construed as a potential conflict of interest.
Supplementary Material
The Supplementary Material for this article can be found online at: https://www.frontiersin.org/articles/10.3389/fgene.2019.00045/full#supplementary-material
References
Bartel, D. P. (2004). MicroRNAs: genomics, biogenesis, mechanism, and function. Cell 116, 281–297. doi: 10.1016/S0092-8674(04)00045-5
Betel, D., Koppal, A., Agius, P., Sander, C., and Leslie, C. (2010). Comprehensive modeling of microRNA targets predicts functional non-conserved and non-canonical sites. Genome Biol. 11:R90. doi: 10.1186/gb-2010-11-8-r90
Coronnello, C., and Benos, P. V. (2013). ComiR: combinatorial microRNA target prediction tool. Nucleic Acids Res. 41, W159–W164. doi: 10.1093/nar/gkt379
Daborn, P., Boundy, S., Yen, J., Pittendrigh, B., and ffrench-Constant, R. (2001). DDT resistance in Drosophila correlates with Cyp6g1 over-expression and confers cross-resistance to the neonicotinoid imidacloprid. Mol. Genet. Genomics 266, 556–563. doi: 10.1007/s004380100531
Edman, J. D., and Okhawa, H. (eds) (2004). “Emerging vectorborne diseases and their control,” in New Discoveries in Agrochemicals. ACS Symposium Series 892, ed. J. M. Clark (Washington D.C: ACS Books), 314–325. doi: 10.1021/bk-2005-0892.ch030
Enright, A. J., John, B., Gaul, U., Tuschl, T., Sander, C., and Marks, D. S. (2003). MicroRNA targets in Drosophila. Genome Biol. 5:R1. doi: 10.1186/gb-2003-5-1-r1
Etebari, K., Afrad, M. H., Tang, B., Silva, R., Furlong, M. J., and Asgari, S. (2018). Involvement of microRNA miR-2b-3p in regulation of metabolic resistance to insecticides in Plutella xylostella. Insect Mol. Biol. 27, 478–491. doi: 10.1111/imb.12387
Festucci-Buselli, R. A., Carvalho-Dias, A. S., de Oliveira-Andrade, M., Caixeta-Nunes, C., Li, H. M., Stuart, J. J., et al. (2005). Expression of Cyp6g1 and Cyp12d1 in DDT resistant and susceptible strains of Drosophila melanogaster. Insect Mol. Biol. 14, 69–77. doi: 10.1111/j.1365-2583.2005.00532
Feyereisen, R. (1999). Insect P450 enzymes. Annu. Rev. Entomol. 44, 507–533. doi: 10.1146/annurev.ento.44.1.507
Ffrench-Constant, R. H. (2013). The molecular genetics of insecticide resistance. Genetics 194, 807–815. doi: 10.1534/genetics.112.141895
Gellatly, K. J., Yoon, K. S., Doherty, J. J., Sun, W., Pittendrigh, B. R., and Clark, J. M. (2015). RNAi validation of resistance genes and their interactions in the highly DDT-resistant 91-R strain of Drosophila melanogaster. Pestic. Biochem. Physiol. 121, 107–115. doi: 10.1016/j.pestbp.2015.01.001
Gong, Y., Li, T., Zhang, L., Gao, X., and Liu, N. (2013). Permethrin induction of multiple cytochrome P450 genes in insecticide resistant mosquitoes, Culex quinquefasciatus. Int. J. Biol. Sci. 9, 863–871. doi: 10.7150/ijbs.6744
Gulyaeva, L. F., Chanyshev, M. D., Kolmykov, S. K., Ushakov, D. S., and Nechkin, S. S. (2016). The effect of xenobiotics on microRNA expression in rat liver. Biomed. Khim. 62, 154–159. doi: 10.18097/PBMC20166202154
Guo, Q., Huang, Y., Zou, F., Liu, B., Tian, M., Ye, W., et al. (2017). The role of miR-2∼13∼71 cluster in resistance to deltamethrin in Culex pipiens pallens. Insect Biochem. Mol. Biol. 84, 15–22. doi: 10.1016/j.ibmb.2017.03.006
Hemingway, J. (2009). “Vector biology diagnostics and public health pesticide development through the product development partnership route,” in Advances in Vector Human Vector Control. ACS Symposium Series 1014, eds J. M. Clark, J. R. Bloomquist, and H. Kawada (Washington, DC: ACS Books), 1–12. doi: 10.1021/bk-2009-1014.ch001
Hong, S., Guo, Q., Wang, W., Hu, S., Fang, F., Lv, Y., et al. (2014). Identification of differentially expressed microRNAs in Culex pipiens and their potential roles in pyrethroid resistance. Insect Biochem. Mol. Biol. 55, 39–50. doi: 10.1016/j.ibmb.2014.10.007
Jones, R. T., Bakker, S. E., Stone, D., Shuttleworth, S. N., Boundy, S., McCart, C., et al. (2010). Homology modelling of Drosophila cytochrome P450 enzymes associated with insecticide resistance. Pest Manag. Sci. 66, 1106–1115. doi: 10.1002/ps.1986
Kim, J. H., Moreau, J. A., Zina, J. M., Mazgaeen, L., Yoon, K. S., Pittendrigh, B. R., et al. (2018). Identification and interaction of multiple genes resulting in DDT resistance in the 91-R strain of Drosophila melanogaster by RNAi approaches. Pestic. Biochem. Physiol. 151, 90–99. doi: 10.1016/j.pestbp.2018.03.003
Kruger, J., and Rehmsmeier, M. (2006). RNAhybrid: microRNA target prediction easy, fast and flexible. Nucleic Acids Res. 34, W451–W454. doi: 10.1093/nar/gkl243
Krupke, C. H., Prasad, R. P., and Anelli, C. M. (2007). Professional entomology and the 44 noisy years since Silent Spring Part 2: response to Silent Spring. Am. Entomol. 53, 16–26. doi: 10.1093/ae/53.1.16
Kuruganti, S., Lam, V., Zhou, X., Bennett, G., Pittendrigh, B. R., and Ganguly, R. (2007). High expression of Cyp6g1, a cytochrome P450 gene, does not necessarily confer DDT resistance in Drosophila melanogaster. Gene 388, 43–53. doi: 10.1016/j.gene.2006.09.019
Li, X., Schuler, M. A., and Berenbaum, M. R. (2007). Molecular mechanisms of metabolic resistance to synthetic and natural xenobiotics. Annu. Rev. Entomol. 52, 231–253. doi: 10.1146/annurev.ento.51.110104.151104
Liu, N., Li, T., Reid, W. R., Yang, T., and Zhang, L. (2011). Multiple cytochrome P450 genes: their constitutive overexpression and permethrin induction in insecticide resistant mosquitoes, Culex quinquefasciatus. PLoS One 6:e23403. doi: 10.1371/journal.pone.0023403
Ma, K., Li, X., Hu, H., Zhou, D., Sun, Y., Ma, L., et al. (2017). Pyrethroid-resistance is modulated by miR-92a by targeting CpCPR4 in Culex pipiens pallens. Comp. Biochem. Physiol. B Biochem. Mol. Biol. 203, 20–24. doi: 10.1016/j.cbpb.2016.09.002
Mallet, J. (1989). The evolution of insecticide resistance: have the insects won? Trends Ecol. Evol. 4, 336–340. doi: 10.1016/0169-5347(89)90088-8
Maragkakis, M., Reczko, M., Simossis, V. A., Alexiou, P., Papadopoulos, G. L., Dalamagas, T., et al. (2009). DIANA-microT web server: elucidating microRNA functions through target prediction. Nucleic Acids Res. 37, W273–W276. doi: 10.1093/nar/gkp292
Morin, M. D., Lyons, P. J., Crapoulet, N., Boquel, S., and Morin, P. J. (2017). Identification of differentially expressed miRNAs in colorado potato beetles (Leptinotarsa decemlineata (Say) exposed to imidacloprid. Int. J. Mol. Sci. 18:e2728. doi: 10.3390/ijms18122728
Pedra, J. H., McIntyre, L. M., Scharf, M. E., and Pittendrigh, B. R. (2004). Genome-wide transcription profile of field- and laboratory-selected dichlorodiphenyltrichloroethane (DDT)-resistant Drosophila. Proc. Natl. Acad. Sci. U.S.A. 101, 7034–7039. doi: 10.1073/pnas.0400580101
Qiu, Y., Tittiger, C., Wicker-Thomas, C., Le Goff, G., Young, S., Wajnberg, E., et al. (2012). An insect-specific P450 oxidative decarbonylase for cuticular hydrocarbon biosynthesis. Proc. Natl. Acad. Sci. U.S.A. 109, 14858–14863. doi: 10.1073/pnas.1208650109
Russell, R. J., Scott, C., Jackson, C. J., Pandey, R., Pandey, G., Taylor, M. C., et al. (2011). The evolution of new enzyme function: lessons from xenobiotic metabolizing bacteria versus insecticide-resistant insects. Evol. Appl. 4, 225–248. doi: 10.1111/j.1752-4571.2010.00175
Sanchez-Diaz, I., Rosales-Bravo, F., Reyes-Taboada, J. L., Covarrubias, A. A., Narvaez-Padilla, V., and Reynaud, E. (2015). The esg gene is involved in nicotine sensitivity in Drosophila melanogaster. PLoS One 10:e0133956. doi: 10.1371/journal.pone.0133956
Schmidt, J. M., Good, R. T., Appleton, B., Sherrard, J., Raymant, G. C., Bogwitz, M. R., et al. (2010). Copy number variation and transposable elements feature in recent, ongoing adaptation at the Cyp6g1 locus. PLoS Genet. 6:e1000998. doi: 10.1371/journal.pgen.1000998
Schmittgen, T. D., and Livak, K. J. (2008). Analyzing real-time PCR data by the comparative C(T) method. Nat. Protoc. 3, 1101–1108. doi: 10.1038/nprot.2008.73
Seong, K. M., Coates, B. S., Berenbaum, M. R., Clark, J. M., and Pittendrigh, B. R. (2018a). Comparative CYP-omic analysis between the DDT-susceptible and -resistant Drosophila melanogaster strains 91-C and 91-R. Pest Manag. Sci. 74, 2530–2543. doi: 10.1002/ps.4936
Seong, K. M., Coates, B. S., Kim, D. H., Hansen, A. K., and Pittendrigh, B. R. (2018b). Differentially expressed microRNAs associated with changes of transcript levels in detoxification pathways and DDT-resistance in the Drosophila melanogaster strain 91-R. PLoS One 13:e0196518. doi: 10.1371/journal.pone.0196518
Seong, K. M., Coates, B. S., Sun, W., Clark, J. M., and Pittendrigh, B. R. (2017). Changes in neuronal signaling and cell stress response pathways are associated with a multigenic response of Drosophila melanogaster to DDT Selection. Genome Biol. Evol. 9, 3356–3372. doi: 10.1093/gbe/evx252
Seong, K. M., Sun, W., Clark, J. M., and Pittendrigh, B. R. (2016). Splice form variant and amino acid changes in MDR49 confers DDT resistance in transgenic Drosophila. Sci. Rep. 6:23355. doi: 10.1038/srep23355
Steele, L. D., Coates, B., Valero, M. C., Sun, W., Seong, K. M., Muir, W. M., et al. (2015). Selective sweep analysis in the genomes of the 91-R and 91-C Drosophila melanogaster strains reveals few of the ‘usual suspects’ in dichlorodiphenyltrichloroethane (DDT) resistance. PLoS One 10:e0123066. doi: 10.1371/journal.pone.0123066
Strycharz, J. P., Lao, A., Li, H., Qiu, X., Lee, S. H., Sun, W., et al. (2013). Resistance in the highly DDT-resistant 91-R strain of Drosophila melanogaster involves decreased penetration, increased metabolism, and direct excretion. Pestic. Biochem. Physiol. 107, 207–217. doi: 10.1016/j.pestbp.2013.06.010
Tian, M., Liu, B., Hu, H., Li, X., Guo, Q., Zou, F., et al. (2016). MiR-285 targets P450 (CYP6N23) to regulate pyrethroid resistance in Culex pipiens pallens. Parasitol. Res. 115, 4511–4517. doi: 10.1007/s00436-016-5238-4
Tsurudome, K., Tsang, K., Liao, E. H., Ball, R., Penney, J., Yang, J. S., et al. (2010). The Drosophila miR-310 cluster negatively regulates synaptic strength at the neuromuscular junction. Neuron 68, 879–893. doi: 10.1016/j.neuron.2010.11.016
Tu, C. P., and Akgul, B. (2005). Drosophila glutathione S-transferases. Methods Enzymol. 401, 204–226. doi: 10.1016/S0076-6879(05)01013-X
Vontas, J. G., Small, G. J., Nikou, D. C., Ranson, H., and Hemingway, J. (2002). Purification, molecular cloning and heterologous expression of a glutathione S-transferase involved in insecticide resistance from the rice brown planthopper, Nilaparvata lugens. Biochem. J. 362, 329–337. doi: 10.1042/bj3620329
Wan, H., Liu, Y., Li, M., Zhu, S., Li, X., Pittendrigh, B. R., et al. (2014). Nrf2/Maf-binding-site-containing functional Cyp6a2 allele is associated with DDT resistance in Drosophila melanogaster. Pest Manag. Sci. 70, 1048–1058. doi: 10.1002/ps.3645
Wang, J., and Cui, Q. (2012). Specific roles of microRNAs in their interactions with environmental factors. J. Nucleic Acids 2012:978384. doi: 10.1155/2012/978384
Wang, L., Bammler, T. K., Beyer, R. P., and Gallagher, E. P. (2013). Copper-induced deregulation of microRNA expression in the zebrafish olfactory system. Environ. Sci. Technol. 47, 7466–7474. doi: 10.1021/es400615q
Wei, G., Sun, L., Li, R., Li, L., Xu, J., and Ma, F. (2018). Dynamic miRNA-mRNA regulations are essential for maintaining Drosophila immune homeostasis during Micrococcus luteus infection. Dev. Comp. Immunol. 81, 210–224. doi: 10.1016/j.dci.2017.11.019
Willoughby, L., Chung, H., Lumb, C., Robin, C., Batterham, P., and Daborn, P. J. (2006). A comparison of Drosophila melanogaster detoxification gene induction responses for six insecticides, caffeine and phenobarbital. Insect Biochem. Mol. Biol. 36, 934–942. doi: 10.1016/j.ibmb.2006.09.004
Wilson, T. G. (1988). Drosophila melanogaster (Diptera: Drosophilidae): a model insect for insecticide resistance studies. J. Econ. Entomol. 81, 22–27. doi: 10.1093/jee/81.1.22
Yu, H. W., and Cho, W. C. (2015). The role of microRNAs in toxicology. Arch. Toxicol. 89, 319–325. doi: 10.1007/s00204-014-1440-2
Zhang, Y., Feng, K., Hu, J., Shi, L., Wei, P., Xu, Z., et al. (2018). A microRNA-1 gene, tci-miR-1-3p, is involved in cyflumetofen resistance by targeting a glutathione S-transferase gene, TCGSTM4, in Tetranychus cinnabarinus. Insect Mol. Biol. 27, 352–364. doi: 10.1111/imb.12375
Zhang, Y., Xu, Z., Wu, Q., Peng, M., Liu, Y., Liu, X., et al. (2016). Identification of differentially expressed microRNAs between the fenpropathrin resistant and susceptible strains in Tetranychus cinnabarinus. PLoS One 11:e0152924. doi: 10.1371/journal.pone.0152924
Zhao, Y., Lin, P., Liufu, Z., Yang, H., Lyu, Y., Shen, X., et al. (2018). Regulation of large number of weak targets - New insights from twin-microRNAs. Genome Biol. Evol. 10, 1255–1264. doi: 10.1093/gbe/evy079
Zhou, Y., Huang, H., Zhang, K., Ding, X., Jia, L., Yu, L., et al. (2016). miRNA-216 and miRNA-499 target cyb561d2 in zebrafish in response to fipronil exposure. Environ. Toxicol. Pharmacol. 45, 98–107. doi: 10.1016/j.etap.2016.05.019
Keywords: Drosophila melanogaster, microRNA, DDT resistance, expression analysis, cytochrome P450
Citation: Seong KM, Coates BS and Pittendrigh BR (2019) Impacts of Sub-lethal DDT Exposures on microRNA and Putative Target Transcript Expression in DDT Resistant and Susceptible Drosophila melanogaster Strains. Front. Genet. 10:45. doi: 10.3389/fgene.2019.00045
Received: 20 September 2018; Accepted: 21 January 2019;
Published: 05 February 2019.
Edited by:
TingFung Chan, The Chinese University of Hong Kong, ChinaReviewed by:
René Massimiliano Marsano, Università degli Studi di Bari, ItalyYang Lyu, University of Michigan, United States
Copyright © 2019 Seong, Coates and Pittendrigh. This is an open-access article distributed under the terms of the Creative Commons Attribution License (CC BY). The use, distribution or reproduction in other forums is permitted, provided the original author(s) and the copyright owner(s) are credited and that the original publication in this journal is cited, in accordance with accepted academic practice. No use, distribution or reproduction is permitted which does not comply with these terms.
*Correspondence: Keon Mook Seong, seongkeo@msu.edu