- 1Department of Urology, Peking University First Hospital, Beijing, China
- 2Institute of Urology, Peking University, Beijing, China
- 3National Urological Cancer Center, Beijing, China
- 4Department of Urology, Fudan University Shanghai Cancer Center, Shanghai, China
- 5Department of Oncology, Shanghai Medical College, Fudan University, Shanghai, China
- 6Department of Urology, The Second Affiliated Hospital of Nanchang University, Nanchang, China
- 7School of Statistics, Renmin University, Beijing, China
- 8Department of Urology, The First Affiliated Hospital of Zhengzhou University, Zhengzhou, China
- 9Department of Urology, Tianjin Institute of Urology, The Second Hospital of Tianjin Medical University, Tianjin, China
- 10Department of Urology, Guangdong General Hospital, Guangdong Academy of Medical Sciences, Guangzhou, China
- 11Department of Urology, Beijing Hospital, Beijing, China
von Hippel-Lindau (VHL) disease is a hereditary cancer syndrome with poor survival. The current recommendations have proposed uniform surveillance strategies for all patients, neglecting the obvious phenotypic varieties. In this study, we aim to confirm the phenotypic heterogeneity in VHL disease and the underlying mechanism. A total of 151 parent-child pairs were enrolled for genetic anticipation analysis, and 77 sibling pairs for birth order effect analysis. Four statistical methods were used to compare the onset age of patients among different generations and different birth orders. The results showed that the average onset age was 18.9 years earlier in children than in their parents, which was statistically significant in all of the four statistical methods. Furthermore, the first-born siblings were affected 8.3 years later than the other ones among the maternal patients. Telomere shortening was confirmed to be associated with genetic anticipation in VHL families, while it failed to explain the birth order effect. Moreover, no significant difference was observed for overall survival between parents and children (p = 0.834) and between first-born patients and the other siblings (p = 0.390). This study provides definitive evidence and possible mechanisms of intra-familial phenotypic heterogeneity in VHL families, which is helpful to the update of surveillance guidelines.
Introduction
von Hippel-Lindau (VHL) disease (MIM 193300) is a rare autosomal dominant cancer syndrome caused by germline mutations in the VHL tumor suppressor gene (Latif et al., 1993; Lonser et al., 2003). Generally, the first VHL-related manifestation occurred in the third decade of patient’s life, and the penetrance is more than 90% by 70 years old (Ong et al., 2007; Nordstrom-O’brien et al., 2010). Patients may develop various tumor types from early childhood through adulthood, including central nervous system hemangioblastomas (CHB), retinal hemangioblastomas (RA), clear cell renal cell carcinoma (RCC), pancreatic cyst and neuroendocrine tumors (PCT), pheochromocytomas (PHEO), endolymphatic sac tumors (ELST), epididymal, and broad ligament cystadenomas (Walther and Linehan, 1996; Lonser et al., 2003, 2004; Butman et al., 2008).
The VHL gene is located on chromosome 3p25–26 and codes for VHL protein (pVHL), which is one of the most important tumor suppressor proteins (Gossage et al., 2015). pVHL forms an E3 ligase complex with elongation factor C and B (elongin C/B), cullin 2 (CUL2), and RING finger protein (RBX1), and is critical for the ubiquitination and proteasomal degradation of HIF-α and other proteins (Gossage et al., 2015). The accumulation of HIF-α caused by mutations of VHL gene and dysregulation of several downstream proangiogenic factors have been identified to be the main cause for tumorigenesis in VHL disease. In recent studies, pVHL has also been found to act as a component of the ataxia telangiectasia mutated (ATM) dependent DNA-damage response (DDR) network, inactivation of which contributes to the genomic instability associated with sporadic RCC (Metcalf et al., 2014). Furthermore, ATM was reported to play a dual role in the sophisticated surveillance mechanisms at DNA double-strand breaks (DSBs) and in telomere regulation (Di Domenico et al., 2014). Thus, some HIF independent and DDR related pathways may play an important role in the pathogenesis of VHL related tumors.
Patients with VHL, a hereditary cancer syndrome, often ended with poor survival due to the complex phenotype and unclear pathogenesis (Wilding et al., 2012). Clinically, the mean life expectancy of VHL patients was reported to be 49 years old in a large cohort 30 years ago. Although there is no cure for the hereditary cancer syndrome, early diagnosis has made the median survival age improve to over 60 years old based on the establishment of active surveillance plans (Wang et al., 2018). However, the same plan was carried out for all the VHL patients according to the current surveillance recommendations, regardless of the fact that VHL disease demonstrates obvious phenotypic heterogeneity, which resulted in diagnostic and therapeutic delay of patients with early onset age. To provide personalized surveillance strategy for different individuals, it is a challenging issue to explore the phenotypic diversity in VHL disease. For patients in different families, genotype-phenotype correlations have been well constructed for years: patients with missense mutations are more likely to be affected by pheochromocytoma, while patients with truncating mutations confer a higher risk for RCC and CHB (Gallou et al., 2004; Ong et al., 2007). Nevertheless, the existing genotype-phenotype correlations do not work in VHL patients within the same family. Patients with consanguinity may develop tumors at different age even in the same family with the same genotype, implying that intra-familial phenotypic heterogeneity may be an important part in the complexity of VHL disease.
Genetic anticipation (GA) is one of the most common intra-familial phenotypic varieties for hereditary diseases, in which case the next generations are affected at an earlier age or manifest more serious presentations than their parents. Recently, GA has been reported in hereditary cancer syndromes including Lynch syndrome, Li-Fraumeni syndrome, dyskeratosis congenital and hereditary breast cancer, and was associated with genomic instability caused by shortening of telomere length in the next generations (Vulliamy et al., 2004; Tabori et al., 2007; Martinez-Delgado et al., 2011; Von Salome et al., 2017). However, studies on GA for VHL disease were rare. In our previous study, we for the first time found that telomere shortening was associated with GA in Chinese patients with 18 VHL families (Ning et al., 2014). Nevertheless, Laura reported that telomere abnormalities might just be primarily somatic in origin rather than a cause of GA in 20 families, making the conclusion ambiguous (Aronoff et al., 2018). Considering the small number of families enrolled in the two studies, only the anticipation of the first tumor was reported rather than separate analysis about the same tumor between different generations. Thus, further study on intra-familial phenotypic heterogeneity and the underlying mechanism is needed for better understanding of the disease.
In our present study, we recruited 80 unrelated VHL families and analyzed the phenotype between successive generations and among siblings in the same generation. The results showed that the average onset age was 18.9 years earlier in children than that in their parents, and the first-born siblings were affected by VHL-associated tumors 5.6 years later than the other ones, implying the existence of genetic anticipation and birth order effect within VHL families. Furthermore, we found that telomere shortening in the next generation was associated with genetic anticipation in VHL families, giving a clue that genomic instability might play an important role in the pathogenesis of VHL-associated tumors. The results are helpful for genetic counseling and future updates from the present uniform recommendations to individualized surveillance plan.
Materials and Methods
Editorial Policies and Ethical Considerations
This study was approved by the Medical Ethics Committee of Peking University First Hospital (Beijing, China) and written informed consent was obtained from all subjects.
Patients and Samples
From 2009 to 2016, a total of 348 patients from 133 families were diagnosed with VHL disease at the Institute of Urology, Peking University according to the clinical criteria and VHL gene detection as previously described (Wang et al., 2017; Liu et al., 2018). All the patients diagnosed by clinical symptoms had at least one affected relative identified by VHL mutation test, so that their genotype could be predicted. Forty-eight patients were excluded because of uncertain clinical information, and another 24 patients were excluded because they did not have affected parents, children or siblings. Therefore, a total of 276 patients from 80 families were enrolled in this study. Patients in the different generations formed 151 parent-child pairs for genetic anticipation analysis, and patients with the identical parent formed 77 sibling pairs for birth order effect analysis. Onset age was defined as the age when the first symptom or sign occurred. The basic clinical data was shown in Supplementary Table S1. Among all the individuals enrolled, 141 patients with available peripheral blood leukocyte DNA sample were brought into analysis for the relationship between relative telomere length and phenotypic diversity.
Relative Telomere Length Measurement
Genomic DNA was extracted from peripheral blood leukocyte using a blood DNA extraction kit (Tiangen Biotech). Quantitative real-time PCR was used to measure telomere length as described by Cawthon (2002). The PCR reaction was run in 7500 instrument (Applied Biosystems), containing 5 μL 2X SYBR master mix (Takara), 30 ng genomic DNA, 300 nmol/L telomere primer Tel1 and 900 nmol/L Tel2, or 200 nmol/L single copy gene primer 36B4u and 500 nmol/L 36B4d. The primer sequences and PCR reaction procedure were described in our previous study (Wang et al., 2017). Whenever possible, samples from different groups were run on the same plate. A standard curve was constructed to assess the amplification efficiency (E) using a control DNA sample (male, 45 years old) diluted by 1/4 serial from 50 to 0.19 ng, and the same sample was detected in every batch of PCRs as the inter-run calibration. The telomere repeats (T) was described by (ETel,sample)-Ct(Tel,sample)/(ETel,calibrator)-Ct(Tel,calibrator), and the copy number of 36B4 (S) was (E36B4,sample)-Ct(36B4,sample)/(E36B4,calibrator)-Ct(36B4,calibrator). Relative telomere length (RTL) was calculated by T/S. Age-adjusted RTL (aRTL) was obtained based on the telomere-age curve constructed in our study before (Ning et al., 2014).
Statistical Analysis
Patients’ entire lifetimes from birth until death or the end of follow-up in December 2016 were included for analysis, and the median observation time was 39.3 years/person (range 1–75 years) with a total 10860 person-years. The survival of patients and age-related penetrance of the five VHL-associated tumors were analyzed using Kaplan-Meier plots and log-rank test. For the analysis of genetic anticipation and birth order effect, we first used paired t-test to examine the difference of onset age between affected patient pairs. Considering the truncating bias caused by clinical data in paired t-test, we confirmed the results using the non-parametric method of Rabinowitz and Yang 1 (RY1) and non-parametric method of Rabinowitz and Yang 2 (RY2) which were special statistical methods for genetic anticipation analysis (Boonstra et al., 2010). Furthermore, the Cox proportional hazards model (CPH) was used to evaluate the effect of generation on phenotype when we included both affected and unaffected patients (Boonstra et al., 2010).
Statistical analyses were performed using SPSS13.0 and R software, and a two-sided p-value of less than 0.05 was considered to be statistically significant.
Results
Genetic Anticipation in Different Generations
Genetic anticipation was analyzed in 151 parent-child pairs from 80 VHL families. As we reported before, the age-related penetrance in children was obviously higher than that in their parents (p < 0.001) (Figure 1), and the result was not affected by mutation origin and mutation type (Figure 2). In this study, we further analyzed the five common VHL-related tumors CHB, RA, RCC, PCT, and PHEO, respectively. The results showed that children had higher age-related penetrance in all five tumors than their parents (Figure 1). To further support our results, we assessed the anticipation in 20 grandparents-parents-children groups from 16 families. The data revealed that patients showed higher age-related penetrance in successive generations (p < 0.001) (Figure 1).
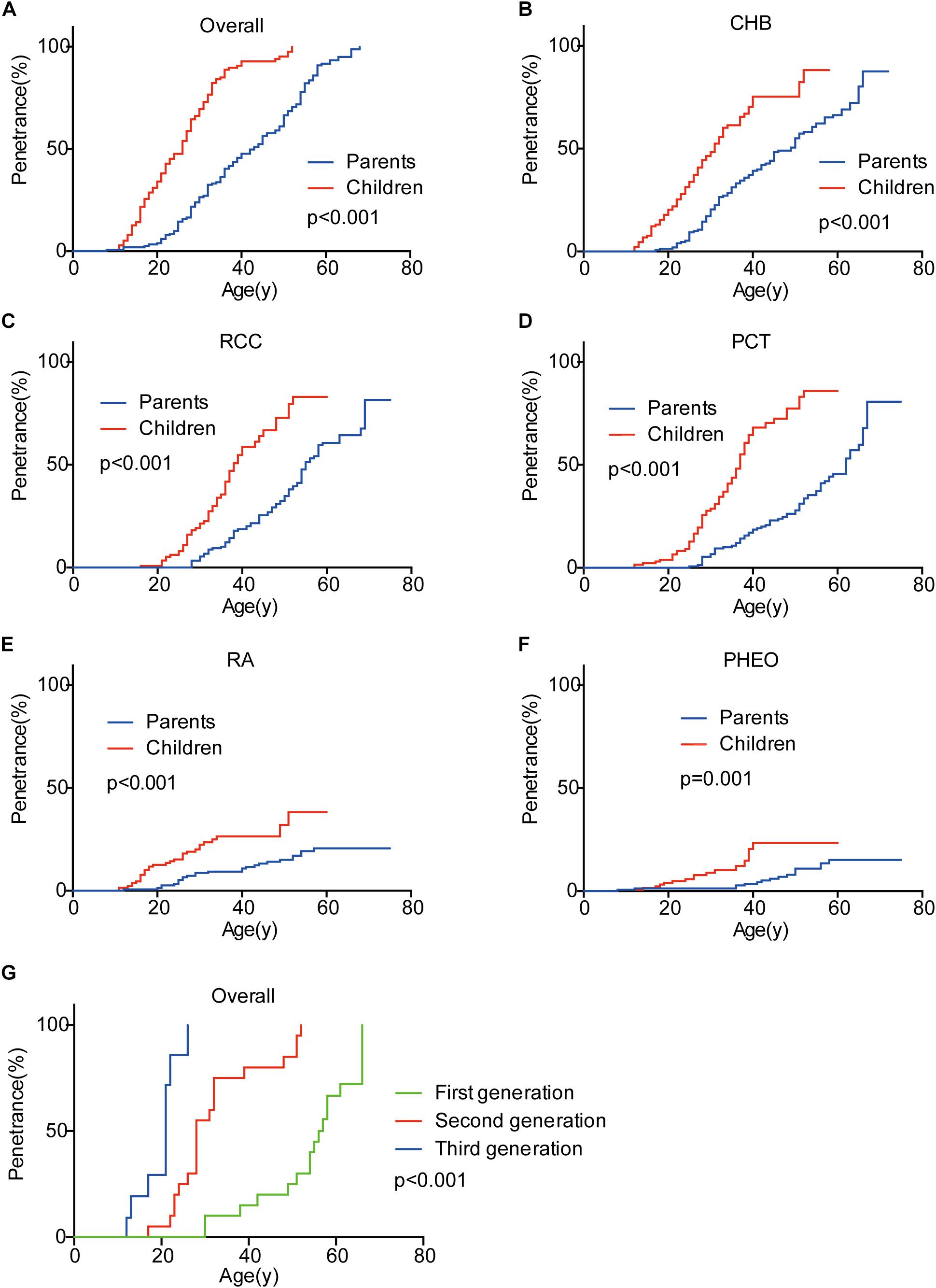
Figure 1. Genetic anticipation between parents and children in different generations. Children showed higher age-related risk than their parents for overall tumors (A) and CHB (B), RCC (C), PCT (D), RA (E), PHEO (F), respectively. Age-related penetrance was also assessed in 16 families with three generations and showed the same tendency (G).
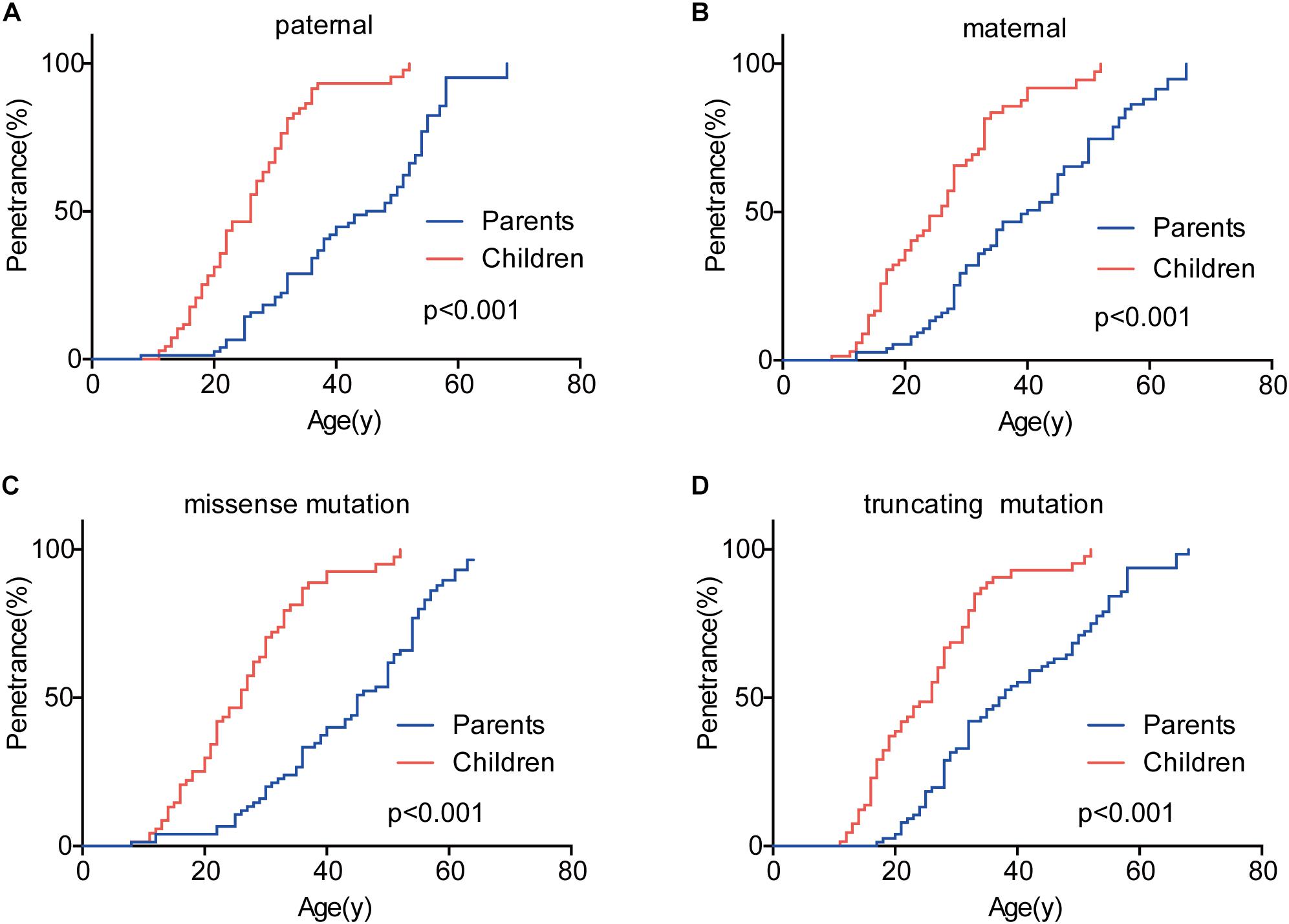
Figure 2. The effect of mutation origin and mutation type on genetic anticipation. Children had higher age-related penetrance than their parents among paternal (A) and maternal (B) patients. The mutation type had no effect on the genetic anticipation (C,D).
To evaluate the exact difference of onset age between parents and children, we analyzed the 114 tumor-affected parent-child pairs and found that the average onset age was 18.9 years earlier in children (Table 1) (p < 0.001), which was 2.1 years longer than we previously reported in 34 parent-child pairs (Ning et al., 2014). Considering the possible bias caused by truncating data, the result was reanalyzed using RY1 and RY2 with the affected parents-children pairs and at last by CPH model with affected and non-affected VHL patients. As expected, the difference of onset age between parents and children was statistically significant in all the three methods (Tables 1, 2). For the onset age of the five common VHL-related tumors, respectively, a similar tendency was observed (Supplementary Table S2). The mean of onset age difference (MOAD) ranged from 17.2 years (CHB) to 34.6 years (PHEO) in different tumors.
Birth Order Effect in the Same Generation
Given that the next generation showed a much earlier onset age than the first generation, we further assessed the phenotypic heterogeneity in the same generation, which was called the birth order effect. A total of 77 sibling pairs from the identical parents were analyzed, and the distribution of onset age similarly displayed a “shift to left” phenomenon in younger siblings (p = 0.036) (Figure 3). On average, the first-born siblings were affected by VHL-associated tumors 5.6 years later than the other ones, which was statistically significant in paired t-test (p < 0.001), RY1 (p < 0.05), and RY2 (p < 0.05) (Table 3). Interestingly, when we analyzed the data only in the paternal sibling pairs, the birth order effect disappeared (Figure 3 and Table 3). In contrast, in the maternal sibling pairs, the MOAD increased to 8.3 years later between the first-born siblings and others (Figure 3 and Table 3).
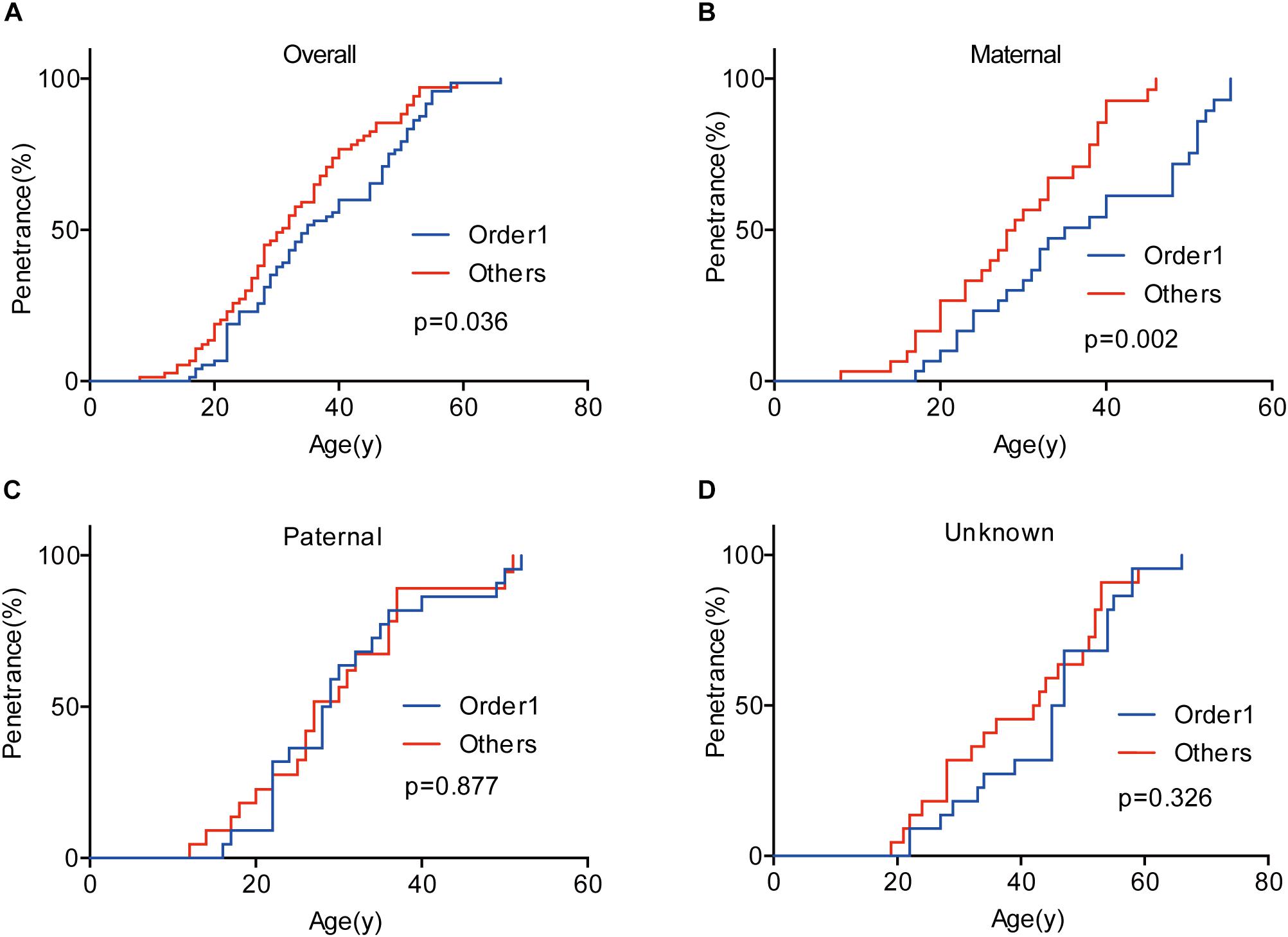
Figure 3. Birth order effect between the first-born siblings and the others in the same generation. The first-born siblings displayed lower age-related penetrance than the others for overall tumors (A). Among siblings with an identical affected mother, the birth order effect became more evident (B). The birth order effect disappeared among paternal patients (C). When the mutated VHL allele was from fathers or the origin was not clear, the effect disappeared (D).
Telomere Length and Intra-Family Phenotypic Diversity in VHL Disease
The relationship between telomere length and intra-family phenotypic diversity for VHL disease was next investigated. A total of 55 parent-child pairs with data of telomere length were enrolled for analysis, and the result showed that in 80% (44/55) pairs, children had significantly shorter aRTL than their parents (p < 0.001) (Figure 4). Furthermore, in the 33 parent-child pairs with clear onset ages, 28 children had younger onset age with shorter aRTL, while their parents had older onset age with longer aRTL (Table 4). Among the 16 families with three generations, only three families were available for DNA samples of three generations (Family 42, 48, 66), and all of them displayed a tendency of shorter telomere length along with generations (Figure 5). With regard to the sibling pairs, 16 out of 25 pairs showed relatively shorter aRTL in the younger siblings, but the result was not statistically significant (p = 0.585) (Figure 4). In the 19 tumor-affected sibling pairs, only 8 younger patients had shorter aRTL with younger onset age (Supplementary Table S3). The results indicated that telomere shortening was associated with genetic anticipation in VHL families, while the association between telomere length and birth order effect might be uncertain.
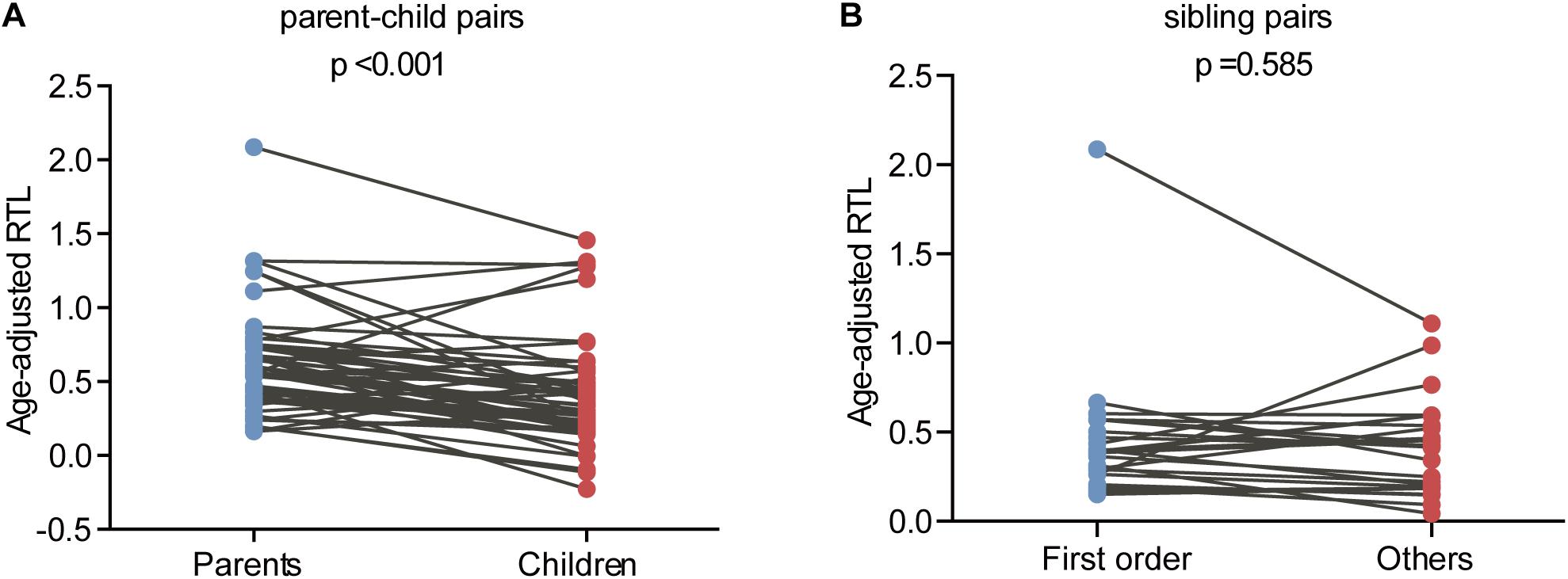
Figure 4. Age-adjusted relative telomere length in parents and children and in patients with different birth order. (A) Children showed a significant shorter telomere length than their parents. (B) No significant difference of telomere length was observed between the first-born sibling and the others.
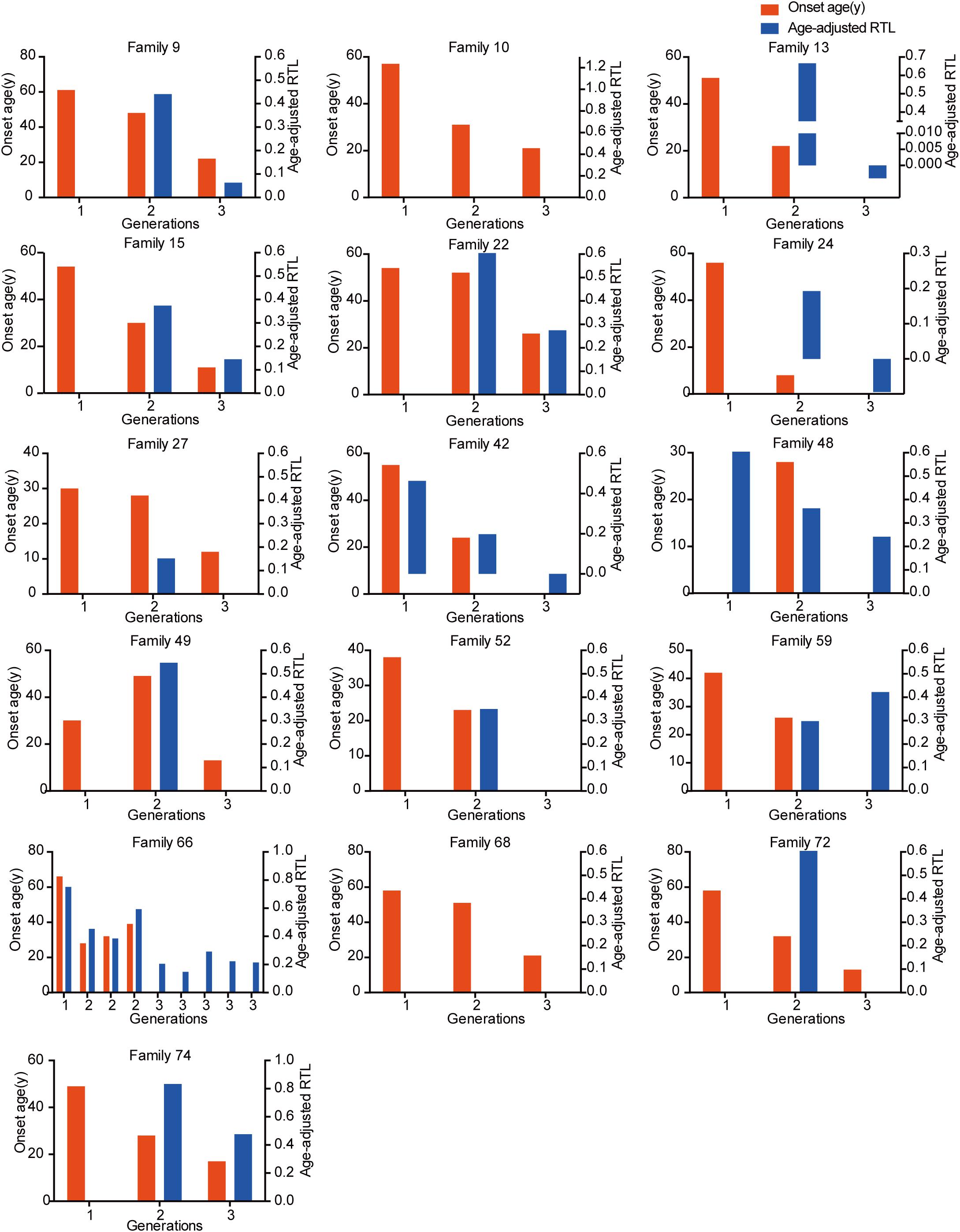
Figure 5. The relationship between genetic anticipation and telomere shortening in 16 VHL families with three generations.
Survival of VHL Patients in Different Generations and Birth Order
In the 151 parent-child pairs, 58 parents, and 10 children died. The median survival for parents was 62 years old, while the survival for children was undefined (Figure 6). No significant difference was observed for overall survival between parents and children (p = 0.834) (Figure 6). Similarly, there was no difference between the overall survival for the first-born patients and the other siblings (p = 0.390) (Figure 6). Considering the diagnostic and therapeutic improvement in the next generation and later-born siblings, the similar overall survival in some sense confirmed the genetic anticipation and birth order effect.
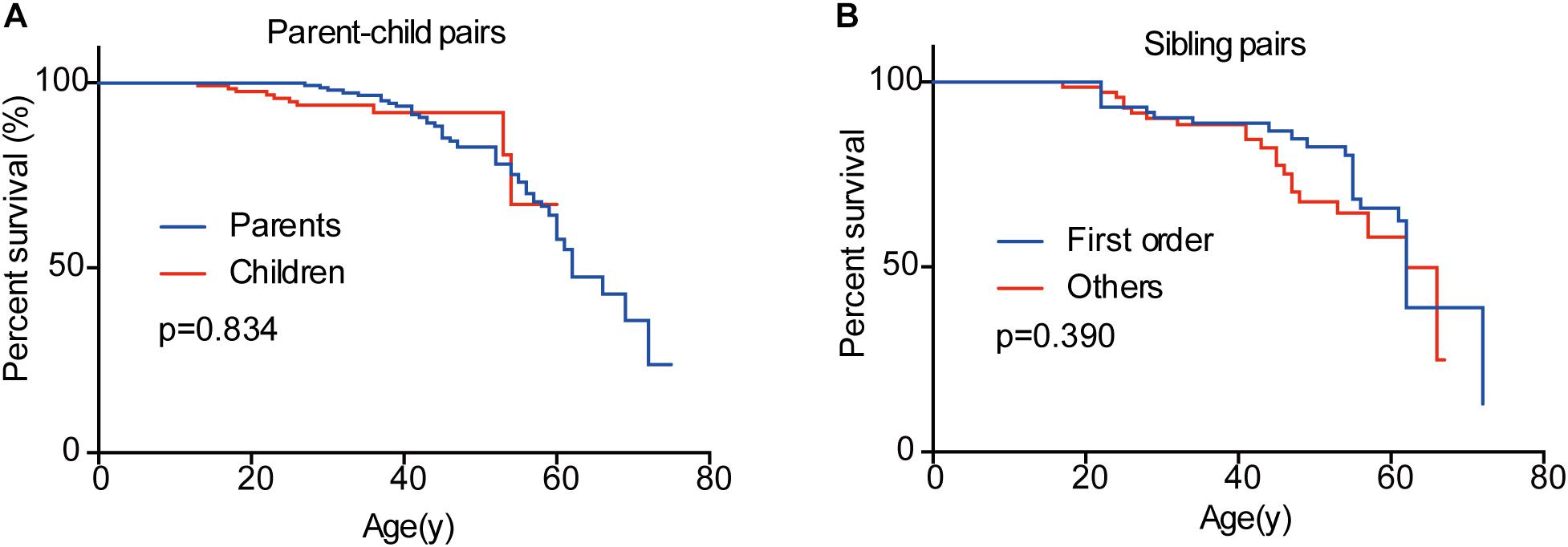
Figure 6. Overall survivals of patients in different generations and in different birth order. No significant difference was observed between parents and children (A) and between the first-born sibling and the others (B).
Discussion
Studies on the average onset ages of VHL-related tumors have helped the VHL Alliance to propose the VHL tumor surveillance regimen for the last decades, according to which routine imaging screening should start at 1 year old for RA, 8 for PHEO, 16 for CHB, and 8 for RCC and PCT (VHL Alliance, 2015). Clinically, there exist several strategies of surveillance for VHL disease in the world, and the initial screening age differ from each other because of the different phenotypic features of patients they refer to (Hes et al., 2001; Binderup et al., 2013; Kruizinga et al., 2014; Rednam et al., 2017). Recently, our previous study and another Canadian study demonstrated that children in VHL families presented manifestations more than 10 years earlier than their parents, suggesting that surveillance plan for children should not only consider the regular VHL tumor surveillance regimen, but also the onset age of their parents (Ning et al., 2014; Aronoff et al., 2018). However, the limited number of patients involved in the studies weakened the reliability, and both of the two studies did not analyze the onset age of the five common VHL-related tumors CHB, RA, RCC, PCT, and PHEO in different generations, respectively. In our present study, we confirmed the genetic anticipation phenomenon in VHL families, and interestingly, we also observed the birth order effect among VHL siblings from the same affected mother. This suggests that patients with affected parents or born in the later order within the family should start screening much earlier than others. Specifically, children in VHL families should receive MRI examination of brain and spinal cord before 12 years old rather than 14–16 years old recommended by the current strategies, since six children are affected by CHB before 14 (three for 12 years and three for 13) in our study. As to PHEO, we agree with the VHL Alliance and the regular abdominal imaging for children should start at 8 years old instead of 10 years recommended by Hes et al. (2001).
Genetic anticipation has been a controversial issue since it was first introduced because of the bias caused by data evaluation and statistical method the authors choose (Gruber and Mukherjee, 2009). Paired t-test is the most common method used for anticipation analysis, but it may introduce a truncation bias, which will increase the type I error (Heiman et al., 1996). To lower the truncation bias caused by paired t-test, we further used two special statistical methods for genetic anticipation analysis (RY1 and RY2) in this study, reaching the same results. However, all the three methods are carried out with affected parent-child pairs, missing the data of unaffected patients. Thus, the CPH model was finally used to confirm GA with all the 151 parent-child pairs. As expected, the difference of onset age between parents and children is significant in all the four statistical methods.
Genetic anticipation and birth order effect have also been thought to be the myth caused by the improvement of diagnosis and surveillance in the younger patients, which is a source of ascertainment bias. People tend to take more active surveillance plan when they have relatives diagnosed with the hereditary disease, and this may lead to early detection of tumors before symptom. To reduce this kind of bias, we only analyzed the data of patient pairs presenting symptoms. It turned out that the differences of onset age between different generations and different birth orders were highly significant (Tables 1, 3) (p < 0.001). Moreover, children are affected by VHL related tumors before their parents present symptoms in 24% parent-child pairs, and similarly, about 38% siblings born in later order suffer tumors before the first-born patients become symptomatic. Thus, the ascertainment bias will not influence the final conclusion in our study.
To explore the molecular mechanism for genetic anticipation, we measured the age-adjusted relative telomere length of blood leukocytes in 10 parent-child pairs in our previous study and found that the age-adjusted telomere length was significantly shorter in a child than in his or her parent in all of the 10 parent-child pairs (Ning et al., 2014). Furthermore, VHL patients showed significantly shorter telomere length than healthy family controls, and a positive correlation was found between telomere length and onset age of the five major VHL related tumors, respectively (Wang et al., 2017). However, in another study from Canada on genetic anticipation with 15 VHL patients, only granulocyte telomeres from VHL patients were significantly shorter than those from healthy controls, and no significant difference was observed between different generations in the six parent-child pairs, implying that genetic anticipation in VHL is not caused by telomere abnormalities (Aronoff et al., 2018). The different results in the two studies above may be due to bias caused by the small sample size and different methods in measuring the telomere length. In this study, we evaluate the relationship between telomere shortening and genetic anticipation in a large VHL cohort and confirm that the age-adjusted relative telomere length was shorter in children than in their parents in 44 out of 55 parent-child pairs. As we all know, the telomeres in most cells shorten throughout human life, meaning that the telomere length would be influenced by age in which the blood sample was collected. Therefore, we use the age-adjusted relative telomere length (calculated as the difference between predicted normal relative telomere length at the DNA-obtained age and the relative telomere length actually measured) instead of relative telomere length in our analysis. The results confirm that telomere shortening is the molecular mechanism for genetic anticipation in VHL families.
Nevertheless, telomere shortening seems not to play a role in the mechanism of birth order effect in VHL disease. Although parous women have been reported to have shorter telomere length than nulliparous women (Pollack et al., 2018), we did not observe significant difference for telomere length between siblings in the first order and others. Currently, there exist three explanations for birth order effect, namely more infectious exposures in later-born children, a higher level of hormonal exposures in first pregnancies and a higher level of microchimerism in the later-born individuals(Von Behren et al., 2011). In this study, we find that birth order effect only exists in maternal sibling pairs, giving us a clue that the differences of hormonal exposures during pregnancies may contribute to the phenotypic diversity of VHL patients in different birth order. However, we don’t have enough data to confirm the hypothesis. The specific mechanisms for birth order effect in VHL families need further exploration.
In conclusion, this study provides definitive evidence of intra-familiar phenotypic variety in VHL families including genetic anticipation and birth order effect. Clinicians should take the position in the family tree into consideration when they are making surveillance plan for VHL patients. Although we confirm that telomere shortening plays a role in the molecular mechanism of phenotypic heterogeneity within VHL families, the detailed effect of inactivated VHL protein on genomic instability remains unclear, and the explanation for birth order effect needs further exploration.
Ethics Statement
This study was carried out in accordance with the recommendations of “the Medical Ethics Committee of Peking University First Hospital (Beijing, China)” with written informed consent from all subjects. All subjects gave written informed consent in accordance with the Declaration of Helsinki. The protocol was approved by the “Medical Ethics Committee of Peking University First Hospital (Beijing, China).”
Author Contributions
KG developed the hypothesis and secured the funding. JW wrote the first draft of the manuscript. CC, XN, and XP carried out the statistical analyses. SP, BH, and TL dealt with figures and tables. SL, JZ, and KM performed the data collection. LC revised the manuscript. All authors critically commented on and approved the final version of the manuscript.
Funding
This work was supported by the National Natural Science Foundation of China (Grant Number 81572506), the Fundamental Research Funds for the Central Universities (Grant Number BMU2018JI002), Beijing Municipal Science and Technology Commission (Grant Number Z151100003915126), and Beijing Municipal Commission of Health and Family Planning (Grant Number 2016-2-4074).
Conflict of Interest Statement
The authors declare that the research was conducted in the absence of any commercial or financial relationships that could be construed as a potential conflict of interest.
Acknowledgments
We thank Tingting Yan, Division of Gastroenterology and Hepatology, Renji Hospital, Shanghai, China, for her revision of the manuscript.
Supplementary Material
The Supplementary Material for this article can be found online at: https://www.frontiersin.org/articles/10.3389/fgene.2019.00358/full#supplementary-material
References
Aronoff, L., Malkin, D., Van Engelen, K., Gallinger, B., Wasserman, J., Kim, R. H., et al. (2018). Evidence for genetic anticipation in von Hippel-Lindau syndrome. J. Med. Genet. 55, 395–402. doi: 10.1136/jmedgenet-2017-104882
Binderup, M. L., Bisgaard, M. L., Harbud, V., Moller, H. U., Gimsing, S., Friis-Hansen, L., et al. (2013). Von Hippel-Lindau disease (vHL). National clinical guideline for diagnosis and surveillance in Denmark. 3rd edition. Dan. Med. J. 60:B4763.
Boonstra, P. S., Gruber, S. B., Raymond, V. M., Huang, S. C., Timshel, S., Nilbert, M., et al. (2010). A review of statistical methods for testing genetic anticipation: looking for an answer in Lynch syndrome. Genet. Epidemiol. 34, 756–768. doi: 10.1002/gepi.20534
Butman, J. A., Linehan, W. M., and Lonser, R. R. (2008). Neurologic manifestations of von Hippel-Lindau disease. JAMA 300, 1334–1342. doi: 10.1001/jama.300.11.1334
Cawthon, R. M. (2002). Telomere measurement by quantitative PCR. Nucleic Acids Res. 30:e47. doi: 10.1093/nar/30.10.e47
Di Domenico, E. G., Romano, E., Del Porto, P., and Ascenzioni, F. (2014). Multifunctional role of ATM/Tel1 kinase in genome stability: from the DNA damage response to telomere maintenance. Biomed. Res. Int. 2014:787404. doi: 10.1155/2014/787404
Gallou, C., Chauveau, D., Richard, S., Joly, D., Giraud, S., Olschwang, S., et al. (2004). Genotype-phenotype correlation in von Hippel-Lindau families with renal lesions. Hum. Mutat. 24, 215–224. doi: 10.1002/humu.20082
Gossage, L., Eisen, T., and Maher, E. R. (2015). VHL, the story of a tumour suppressor gene. Nat. Rev. Cancer 15, 55–64. doi: 10.1038/nrc3844
Gruber, S. B., and Mukherjee, B. (2009). Anticipation in lynch syndrome: still waiting for the answer. J. Clin. Oncol. 27, 326–327. doi: 10.1200/JCO.2008.19.1445
Heiman, G. A., Hodge, S. E., Wickramaratne, P., and Hsu, H. (1996). Age-at-interview bias in anticipation studies: computer simulations and an example with panic disorder. Psychiatr. Genet. 6, 61–66. doi: 10.1097/00041444-199622000-00005
Hes, F. J., Van Der Luijt, R. B., and Lips, C. J. (2001). Clinical management of Von Hippel-Lindau (VHL) disease. Neth. J. Med. 59, 225–234. doi: 10.1016/S0300-2977(01)00165-6
Kruizinga, R. C., Sluiter, W. J., De Vries, E. G., Zonnenberg, B. A., Lips, C. J., Van Der Horst-Schrivers, A. N., et al. (2014). Calculating optimal surveillance for detection of von Hippel-Lindau-related manifestations. Endocr. Relat. Cancer 21, 63–71. doi: 10.1530/ERC-13-0308
Latif, F., Tory, K., Gnarra, J., Yao, M., Duh, F. M., Orcutt, M. L., et al. (1993). Identification of the von Hippel-Lindau disease tumor suppressor gene. Science 260, 1317–1320. doi: 10.1126/science.8493574
Liu, S. J., Wang, J. Y., Peng, S. H., Li, T., Ning, X. H., Hong, B. A., et al. (2018). Genotype and phenotype correlation in von Hippel-Lindau disease based on alteration of the HIF-alpha binding site in VHL protein. Genet. Med. 20, 1266–1273. doi: 10.1038/gim.2017.261
Lonser, R. R., Glenn, G. M., Walther, M., Chew, E. Y., Libutti, S. K., Linehan, W. M., et al. (2003). von Hippel-Lindau disease. Lancet 361, 2059–2067. doi: 10.1016/S0140-6736(03)13643-4
Lonser, R. R., Kim, H. J., Butman, J. A., Vortmeyer, A. O., Choo, D. I., and Oldfield, E. H. (2004). Tumors of the endolymphatic sac in von Hippel-Lindau disease. N. Engl. J. Med. 350, 2481–2486. doi: 10.1056/NEJMoa040666
Martinez-Delgado, B., Yanowsky, K., Inglada-Perez, L., Domingo, S., Urioste, M., Osorio, A., et al. (2011). Genetic anticipation is associated with telomere shortening in hereditary breast cancer. PLoS Genet. 7:e1002182. doi: 10.1371/journal.pgen.1002182
Metcalf, J. L., Bradshaw, P. S., Komosa, M., Greer, S. N., Stephen Meyn, M., and Ohh, M. (2014). K63-ubiquitylation of VHL by SOCS1 mediates DNA double-strand break repair. Oncogene 33, 1055–1065. doi: 10.1038/onc.2013.22
Ning, X. H., Zhang, N., Li, T., Wu, P. J., Wang, X., Li, X. Y., et al. (2014). Telomere shortening is associated with genetic anticipation in Chinese Von Hippel-Lindau disease families. Cancer Res. 74, 3802–3809. doi: 10.1158/0008-5472.CAN-14-0024
Nordstrom-O’brien, M., Van Der Luijt, R. B., Van Rooijen, E., Van Den Ouweland, A. M., Majoor-Krakauer, D. F., Lolkema, M. P., et al. (2010). Genetic analysis of von Hippel-Lindau disease. Hum. Mutat. 31, 521–537. doi: 10.1002/humu.21219
Ong, K. R., Woodward, E. R., Killick, P., Lim, C., Macdonald, F., and Maher, E. R. (2007). Genotype-phenotype correlations in von Hippel-Lindau disease. Hum. Mutat. 28, 143–149. doi: 10.1002/humu.20385
Pollack, A. Z., Rivers, K., and Ahrens, K. A. (2018). Parity associated with telomere length among US reproductive age women. Hum. Reprod. 33, 736–744. doi: 10.1093/humrep/dey024
Rednam, S. P., Erez, A., Druker, H., Janeway, K. A., Kamihara, J., Kohlmann, W. K., et al. (2017). Von Hippel-Lindau and hereditary pheochromocytoma/paraganglioma syndromes: clinical features, genetics, and surveillance recommendations in childhood. Clin. Cancer Res. 23, e68–e75. doi: 10.1158/1078-0432.CCR-17-0547
Tabori, U., Nanda, S., Druker, H., Lees, J., and Malkin, D. (2007). Younger age of cancer initiation is associated with shorter telomere length in Li-Fraumeni syndrome. Cancer Res. 67, 1415–1418. doi: 10.1158/0008-5472.CAN-06-3682
Von Behren, J., Spector, L. G., Mueller, B. A., Carozza, S. E., Chow, E. J., Fox, E. E., et al. (2011). Birth order and risk of childhood cancer: a pooled analysis from five US States. Int. J. Cancer 128, 2709–2716. doi: 10.1002/ijc.25593
Von Salome, J., Boonstra, P. S., Karimi, M., Silander, G., Stenmark-Askmalm, M., Gebre-Medhin, S., et al. (2017). Genetic anticipation in Swedish Lynch syndrome families. PLoS Genet. 13:e1007012. doi: 10.1371/journal.pgen.1007012
Vulliamy, T., Marrone, A., Szydlo, R., Walne, A., Mason, P. J., and Dokal, I. (2004). Disease anticipation is associated with progressive telomere shortening in families with dyskeratosis congenita due to mutations in TERC. Nat. Genet. 36, 447–449. doi: 10.1038/ng1346
Walther, M. M., and Linehan, W. M. (1996). Von Hippel-Lindau disease and pheochromocytoma. JAMA 275, 839–840. doi: 10.1001/jama.1996.03530350021025
Wang, J. Y., Peng, S. H., Li, T., Ning, X. H., Liu, S. J., Hong, B. A., et al. (2018). Risk factors for survival in patients with von Hippel-Lindau disease. J. Med. Genet. 55, 322–328. doi: 10.1136/jmedgenet-2017-104995
Wang, J. Y., Peng, S. H., Ning, X. H., Li, T., Liu, S. J., Liu, J. Y., et al. (2017). Shorter telomere length increases age-related tumor risks in von Hippel-Lindau disease patients. Cancer Med. 6, 2131–2141. doi: 10.1002/cam4.1134
Keywords: von Hippel-Lindau disease, phenotypic heterogeneity, genetic anticipation, birth order effect, telomere length
Citation: Wang J, Peng X, Chen C, Ning X, Peng S, Li T, Liu S, Hong B, Zhou J, Ma K, Cai L and Gong K (2019) Intra-Familial Phenotypic Heterogeneity and Telomere Abnormality in von Hippel- Lindau Disease: Implications for Personalized Surveillance Plan and Pathogenesis of VHL-Associated Tumors. Front. Genet. 10:358. doi: 10.3389/fgene.2019.00358
Received: 30 January 2019; Accepted: 03 April 2019;
Published: 24 April 2019.
Edited by:
Jordi Pérez-Tur, Instituto de Biomedicina de Valencia (IBV), SpainReviewed by:
Xiang Xue, The University of New Mexico, United StatesGiovanni Minervini, University of Padua, Italy
Copyright © 2019 Wang, Peng, Chen, Ning, Peng, Li, Liu, Hong, Zhou, Ma, Cai and Gong. This is an open-access article distributed under the terms of the Creative Commons Attribution License (CC BY). The use, distribution or reproduction in other forums is permitted, provided the original author(s) and the copyright owner(s) are credited and that the original publication in this journal is cited, in accordance with accepted academic practice. No use, distribution or reproduction is permitted which does not comply with these terms.
*Correspondence: Kan Gong, Z29uZ2thbl9wa3VAMTI2LmNvbQ==
†These authors have contributed equally to this work