- 1Laboratory of Human and Medical Genetics, Institute of Biological Sciences, Graduate Program of Genetics and Molecular Biology, Federal University of Pará, Belém, Brazil
- 2Research Center on Oncology, Graduate Program of Oncology and Medical Science, Federal University of Pará, Belém, Brazil
Background: MicroRNAs (miRNAs) play an important role in gastric carcinogenesis and have been associated with gastric field cancerization; however, their role is not fully understood in this process. We performed the miRNome sequencing of non-cancerous, adjacent to tumor and gastric cancer samples to understand the involvement of these small RNAs in gastric field cancerization.
Methods: We analyzed samples of patients without cancer as control (non-cancerous gastric samples) and adjacent to cancer and gastric cancer paired samples, and considered miRNAs with |log2(fold change)| > 2 and Padj < 0.05 to be statistically significant. The identification of target genes, functional analysis and enrichment in KEGG pathways were realized in the TargetCompare, miRTargetLink, and DAVID tools. We also performed receiver operating characteristic (ROC) curves and miRNAs that had an AUC > 0.85 were considered to be potential biomarkers.
Results: We found 14 miRNAs exclusively deregulated in gastric cancer, of which six have potential diagnostic value for advanced disease. Nine miRNAs with known tumor suppressor activities (TS-miRs) were deregulated exclusively in adjacent tissue. Of these, five have potential diagnostic value for the early stages of gastric cancer. Functional analysis of these TS-miRs revealed that they regulate important cellular signaling pathways (PI3K-Akt, HIF-1, Ras, Rap1, ErbB, and MAPK signaling pathways), that are involved in gastric carcinogenesis. Seven miRNAs were differentially expressed in both gastric cancer and adjacent regarding to non-cancerous tissues; among them, hsa-miR-200a-3p and hsa-miR-873-5p have potential diagnostic value for early and advanced stages of the disease. Only hsa-miR-196a-5p was differentially expressed between adjacent to cancer and gastric cancer tissues. In addition, the other miRNAs identified in this study were not differentially expressed between adjacent to cancer and gastric cancer, suggesting that these tissues are very similar and that share these molecular changes.
Conclusion: Our results show that gastric cancer and adjacent tissues have a similar miRNA expression profile, indicating that studied miRNAs are intimately associated with field cancerization in gastric cancer. The overexpression of TS-miRs in adjacent tissues may be a barrier against tumorigenesis within these pre-cancerous conditions prior to the eventual formation or relapse of a tumor. Additionally, these miRNAs have a great accuracy in discriminating non-cancerous from adjacent to tumor and cancer tissues and can be potentially useful as biomarkers for gastric cancer.
Introduction
Gastric cancer (GC) is an aggressive disease that is considered the third leading cause of cancer death worldwide (Ferlay et al., 2015). GC is usually diagnosed in late stages, due in part to the limited efficiency of existing biomarkers, and the 5-year survival rates of these patients do not exceed 30% (Correa, 2013; Yakirevich and Resnick, 2013). Studies have shown that microRNAs (miRNAs) have excellent sensitivity/specificity and a high discriminatory capacity, which make them potentially useful as molecular biomarkers for this type of cancer (Wu et al., 2014; Shin et al., 2015; Vidal et al., 2016).
MicroRNAs are small non-coding RNAs (∼18–30 nt) that regulate gene expression post-transcriptionally, disrupting the expression of target mRNAs (Bartel, 2004; Wu et al., 2014). These molecules play an important role in multiple pathways and the processes responsible for the maintenance of healthy tissue homeostasis (Schneider, 2012; Runtsch et al., 2014). In humans, studies suggest that miRNAs are part of the complex regulatory network of the healthy stomach (Ribeiro-dos-Santos et al., 2010; Moreira et al., 2014a). Therefore, when miRNAs are deregulated in the human stomach, they compromise important pathways that regulate the normal functions of this organ (Zhang et al., 2014), contributing to the onset and progression of gastric carcinogenesis (Wu et al., 2014).
In recent years, our research group joined efforts and used different technologies to understand the relationship between the deregulation of these small RNAs and gastric carcinogenesis (Assumpção et al., 2015; Darnet et al., 2015; Vidal et al., 2016; Magalhães et al., 2018; Pereira et al., 2019). More recently, we have demonstrated the deregulation of miRNAs during the evolution of Correa’s cascade (Vidal et al., 2016) and in field cancerization in GC (Assumpção et al., 2015; Pereira et al., 2019).
Field cancerization assumes that the tissues adjacent to the tumor have molecular changes (e.g., genetic and/or epigenetic) that make them susceptible to the onset of tumors or recurrences (Slaughter et al., 1953; Chai and Brown, 2009; Curtius et al., 2018). The preexisting molecular changes in this tissue (which may be caused by an insult to the healthy epithelium) are precursors of carcinogenesis and serve as background for the establishment and progression of the tumor (Hattori and Ushijima, 2016; Padmanabhan et al., 2017; Curtius et al., 2018). In fact, studies have shown that adjacent to the tumor tissues have similar molecular changes to those found in the tumor as well as unique and exclusive changes, which distinguish them from non-cancerous tissues (Assumpção et al., 2015; Takeshima et al., 2015; Aran et al., 2017; Vidal et al., 2017; Yoshida et al., 2017; Pereira et al., 2019). Recently, miRNAs were related to field cancerization in GC (Assumpção et al., 2015; Pereira et al., 2019), however, little is known about the true role played by these small non-coding RNAs in this process. The use of robust large-scale sequencing technologies is an excellent strategy in both the discovery of new biomarkers and in providing an overview of the complex relationship between miRNAs and field cancerization.
In this study, we used deep sequencing to evaluate the overall expression profile of miRNAs in non-cancerous gastric tissues, adjacent to cancer and with cancer tissues in order to identify miRNAs involved in the field cancerization. In addition, we evaluated the discriminatory performance of miRNAs as biomarkers of gastric carcinogenesis. Our results show new deregulated miRNAs, which are potentially useful as biomarkers for this cancer and suggest a new molecular mechanism involved in the biology of field cancerization.
Materials and Methods
Biological Material
A total of 45 fresh samples of stomach antrum tissues were included in the present study. The non-cancerous control (NC) samples were collected from 15 patients without cancer (chronic gastritis; ± H. pylori; mean age = 59.2) during an upper digestive endoscopy (tissue fragments of approximately 4 millimeters). In addition, 15 tumor-adjacent tissues (histopathologically without cancer) and 15 gastric adenocarcinoma samples were collected from patients with gastric cancer. The tumor-adjacent (ADJ) and gastric adenocarcinoma (GC) samples were all paired (± H. pylori; mean age = 59.9).
The tissues were obtained from patients treated at the Hospital Universitário João de Barros Barreto (HUJBB), Belém, Pará, Brazil. Samples were collected prior to antibiotic, chemotherapeutic, and/or radiotherapeutic treatment. Immediately after collection, all samples were frozen and stored in liquid nitrogen until analysis.
Histopathological characterization of the samples, such as tumor subtype, degree of differentiation, depth of invasion, involvement of lymph nodes, and/or distant metastases were extracted from pathological reports performed by the HUJBB Department of Pathology. Histopathological analysis of the tumor fragments was performed according to Lauren’s classification (Lauren, 1965).
Ethics Statement
This study was reviewed and approved by the Ethics Committee of the Center of Oncology Research of the Federal University of Pará (Protocol No. 1.081.340). All study participants or their legal guardian provided informed written consent in accordance with the Helsinki Declaration of 1964, the Nuremberg Code, in compliance with the National Health Council’s Research Guidelines Involving Human Beings (Res. CNS 466/12).
RNA Extraction, Small RNA Library Construction, and Sequencing
Total RNA was extracted using TRIzol® reagent (Thermo Fisher Scientific). After isolation, total RNA was stored at -80°C until further analysis. The total RNA amount was determined using a Qubit®2.0 (Life Technologies, Foster City, CA, United States), and an Agilent RNA ScreenTape assay and 2200 TapeStation Instrument (Agilent Technologies, United States) were used to detect RNA integrity. Samples with an RNA integrity number (RIN) ≥ 5 were sequenced.
For small RNA-seq, 1 μg of total RNA per sample was used for library preparation utilizing TruSeq Small RNA Sample Prep Kits (Illumina, San Diego, CA, United States). A DNA ScreenTape assay in a 2200 TapeStation Instrument (Agilent Technologies, United States) and real-time PCR with a KAPA Library Quantification Kit (KAPABIOSYSTEM, United States) were used to validate and quantify each library. A 4-nM library pool comprising all samples was sequenced using a MiSeq reagent kit v3 150 cycle on a MiSeq System (Illumina, San Diego, CA, United States).
The raw sequencing reads of all libraries have been deposited at EBI-ENA (PRJEB27213).
Bioinformatics Analysis
The resulting reads were pre-processed and quality filtered (qv > 25). We used STAR (Dobin et al., 2013) aligner to map the reads to the human genome reference (GRCH37). We quantified mature miRNA sequencing using miRBase human annotation (v20). Counting expression data was performed with HTSeq (Anders et al., 2015).
Differential expression analysis of all processed data was performed using the bioconductor-DESeq2 package (Love et al., 2014) in R software, with a detection threshold of 10 counts per miRNA (present at least 10 read counts in at least of the libraries). Comparison between (i) gastric cancer (GC) vs. non-cancerous (NC) samples; (ii) adjacent to gastric cancer (ADJ) vs. NC samples; and (iii) GC vs. ADJ samples were made separately. Adjusted P-values ≤ 0.05 and |log2(fold change)| > 2 were considered statistically significant.
For graphical analysis of miRNAs, expression data was normalized to RPKM. Heatmaps were used for hierarchical clustering of differentially expressed miRNAs. The area under the curve (AUC > 0.85) from the receiver operating characteristic (ROC) curves was used to identify biomarkers with the best sensitivity/specificity relation, and a discriminant analysis of principal components (DAPC) was constructed to infer the number of clusters of epigenetically related samples. All graphical analyses were performed using the R statistical platform.
Identification of Differentially Expressed miRNA Target Genes
We used two online tools to identify the differentially expressed (D. E.) miRNA target genes: (i) TargetCompare (Moreira et al., 2014b) and (ii) miRTargetLink Human (Hamberg et al., 2016). TargetCompare database allows the user to filter miRNAs and its targets genes that are associated with determined diseases, such as GC. miRTargetLink Human is a tool that allows the search for interactions between target genes and miRNAs that have been experimentally validated by molecular biology techniques.
The identified target genes were submitted to functional annotation and enrichment in KEGG pathways using DAVID Bioinformatics Resources v.6.8 online tool (Huang et al., 2009a, 2009b).
Results
After quality control (Supplementary Figure S1), alignment and transcript quantitation, several small non-coding RNAs (sncRNAs) and other transcript fragments were identified. From them, ∼30% (∼9.5 million reads) were recognized as microRNAs reads, identifying 1,144 mature miRNAs. Approximately 90% of the miRNA reads (∼8.5 million reads) were concentrated on the 35 most expressed miRNAs. The number and representativity of D. E. expressed miRNAs identified in this study is similar to other studies that performed miRNome analysis (Hou et al., 2011; Maltseva et al., 2014; Liu et al., 2015; Castro-Magdonel et al., 2017; Liang et al., 2017).
Differentially Expressed miRNAs in GC vs. NC Analysis
We found 21 differentially expressed (D. E.) miRNAs when we compared gastric cancer samples (GC) with non-cancerous gastric samples (NC) (Figure 1 and Supplementary Table S1), of which eight were down-regulated and 13 were up-regulated in GC (Supplementary Table S1). The heatmap obtained from the normalized expression of the D. E. miRNAs perfectly clustered the GC and NC samples together (Figure 2A).
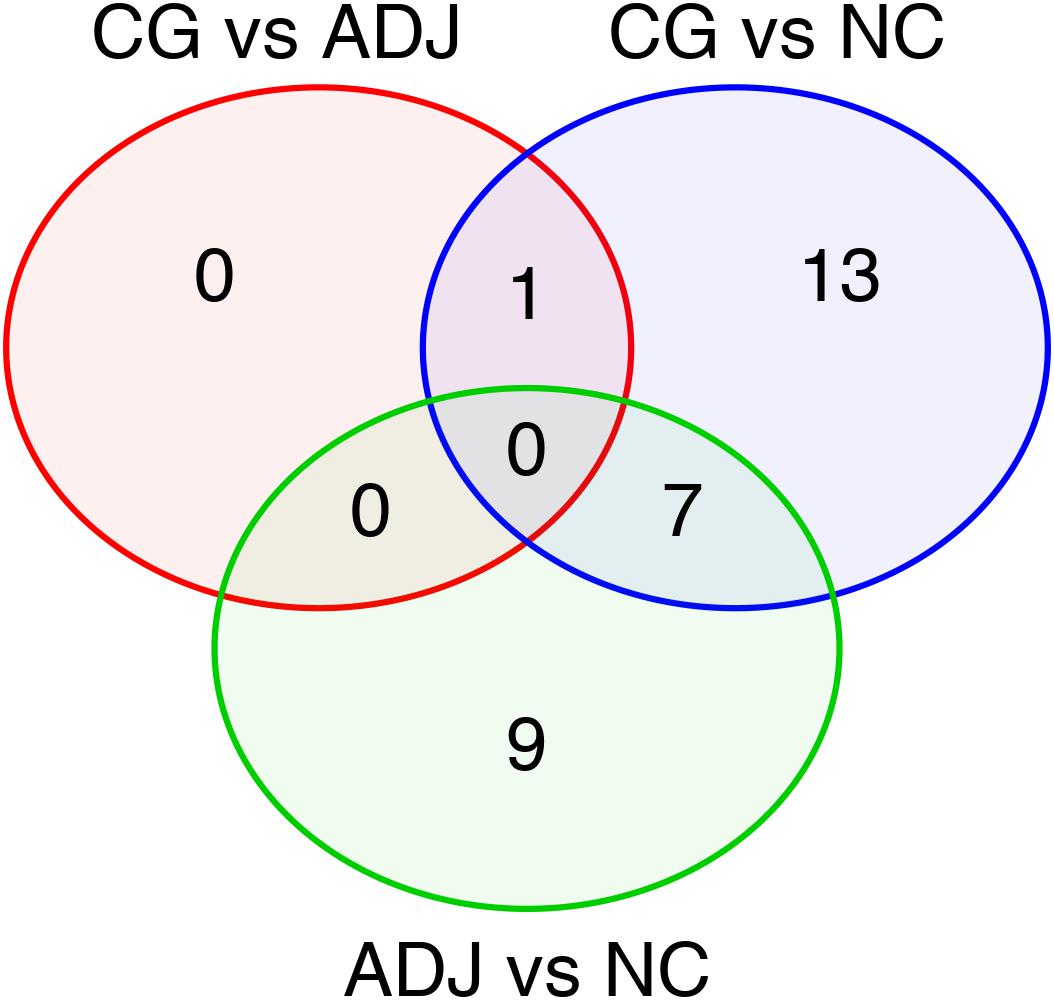
Figure 1. Differentially expressed miRNAs in all comparisons made among the studied groups. Venn’s Diagram of the differentially expressed miRNAs shared between GC vs. ADJ, GC vs. NC, and ADJ vs. NC analysis.
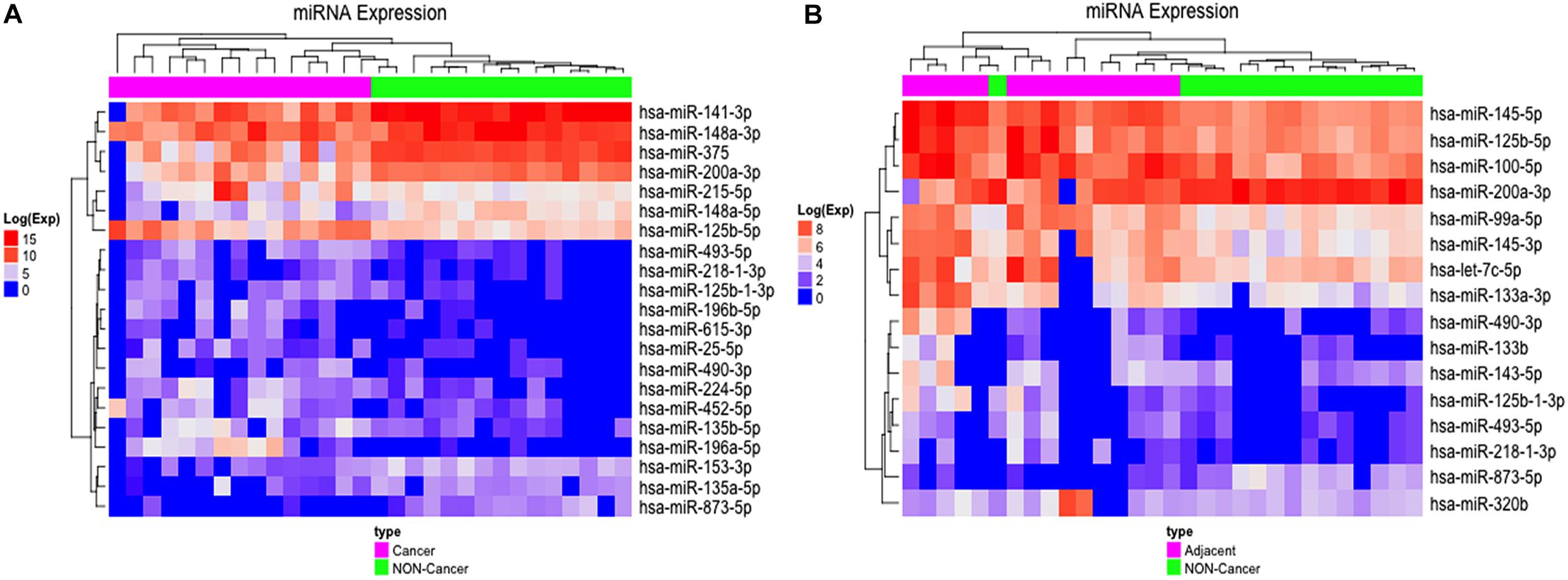
Figure 2. Differentially expressed miRNAs in GC vs. NC and ADJ vs. NC analysis. (A) Heatmap of the 21 most highly expressed miRNAs in each of the two distinct groups: GC (in purple) and NC (in green). (B) Heatmap of the 16 most highly expressed miRNAs in each of the two groups: ADJ (in purple) and NC (in green) samples.
Fourteen miRNAs were exclusively D. E. in the GC vs. NC analysis: nine were up-regulated (six oncomiRs, one TS-miR and two with unknown functions in GC) and five were down-regulated (all TS-miRs) in GC (Figure 1 and Table 1). Six miRNAs, of which five were down-regulated (hsa-miR-141-3p, -miR-148a-3p, -miR-148a-5p, -miR-153-3p, and -miR-375) and one were up-regulated (hsa-miR-196a-5p), presented the best sensitivity/specificity relation (AUC > 0.85) and were considered as potential biomarkers to identify GC (Figure 3).
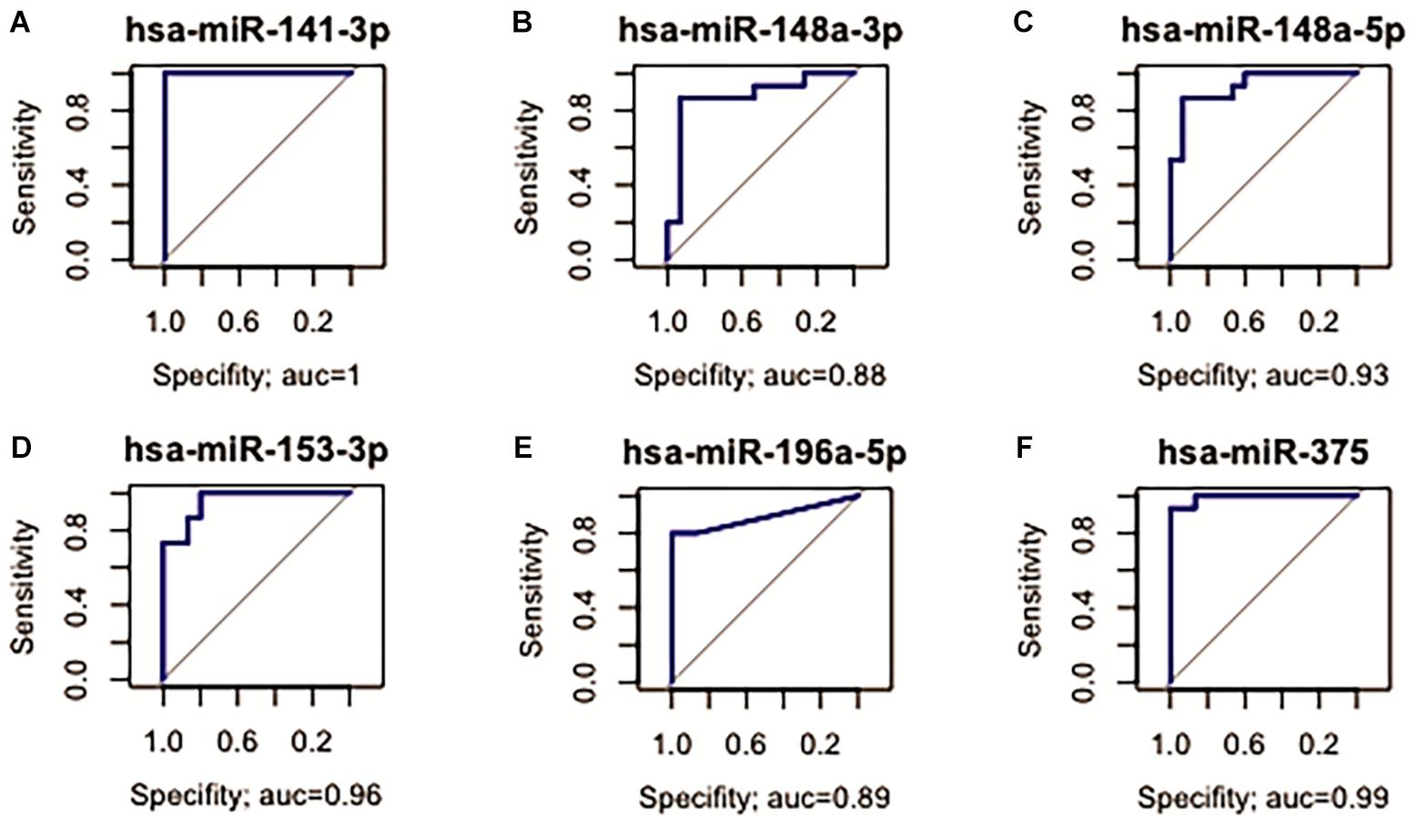
Figure 3. Receiver operating characteristic (ROC) curve analysis identified miRNAs with potential to identify GC. (A) hsa-miR-141-3p (AUC = 1; 95% CI: 1.000–1.000*); (B) hsa-miR-148a-3p (AUC = 0.88; 95% CI: 0.733–1.000); (C) hsa-miR-148a-5p (AUC = 0.93; 95% CI: 0.840–1.000); (D) hsa-miR-153-3p (AUC = 0.96; 95% CI: 0.893–1.000); (E) hsa-miR-196a-5p (AUC = 0.89; 95% CI: 0.767–1.000); (F) hsa-miR-375 (AUC = 0.99; 95% CI: 0.970–1.000).
Differentially Expressed miRNAs in ADJ vs. NC Analysis
Comparing adjacent to gastric cancer samples (ADJ) with NC revealed 16 D. E. miRNAs (Figure 1 and Supplementary Table S2), of which two were down-regulated and 14 were up-regulated (Supplementary Table S2). The heatmap obtained from the normalized expression of the D. E. miRNAs clustered ADJ and NC samples together (Figure 2B). Furthermore, nine miRNAs (eight TS-miRs and one with unknown function in GC) were exclusively D. E. in the ADJ vs. NC analysis: they were all up-regulated in ADJ (Figure 1 and Table 2).
Five D. E. miRNAs (hsa-miR-99a, -miR-100-5p, -miR-125b-5p, -miR-145-3p, and -miR-145b-5p, all up-regulated in ADJ) presented the best sensitivity/specificity relation (AUC > 0.85) and were considered as potential early biomarkers for GC (Figure 4).
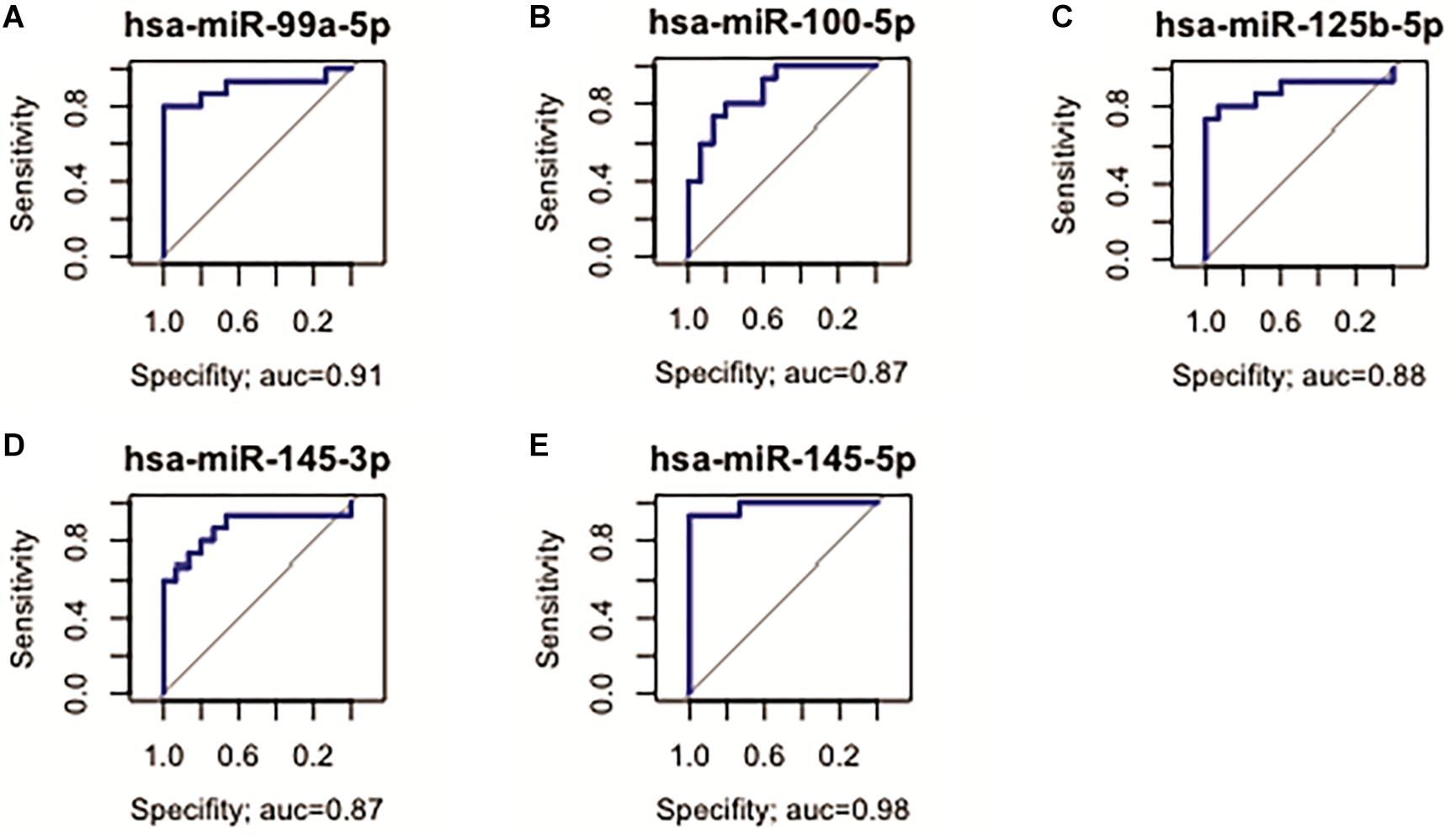
Figure 4. Receiver operating characteristic curve analysis of the five selected miRNAs with potential to predict alterations in ADJ tissue. (A) hsa-miR-99a-5p (AUC = 0.91; 95% CI: 0.783–1.000); (B) hsa-miR-100-5p (AUC = 0.87, 95% CI: 0.747–0.995); (C) hsa-miR-125b-5p (AUC = 0.88; 95% CI: 0.742–1.000); (D) hsa-miR-145-3p (AUC = 0.87; 95% CI: 0.721–1.000); (E) hsa-miR-145-5p (AUC = 0.98; 95% CI: 0.944–1.000).
Functional Analysis of the Up-Regulated TS-miRs in ADJ Tissue
To evaluate the biological and functional role of the eight up-regulated TS-miRs and hsa-miR-99a-5p in ADJ tissue (Table 2), we performed enrichment and functional annotation of their experimentally validated common target genes. We used nine miRNAs as input in the online tools TargetCompare (Moreira et al., 2014b) and miRTargetLink Human (Hamberg et al., 2016), which output five miRNAs (hsa-miR-let7-c-5p, -miR-99a-5p, -miR-100-5p, -miR-133a-3p, and -miR-145-5p) that regulate 18 common target genes (Figure 5A). The enrichment and functional annotation analysis of the 18 target genes performed in DAVID v.6.8 (Huang et al., 2009a, 2009b) revealed that 13 genes participate in 23 different biological pathways (Supplementary Table S3), and 11 genes are involved in nine biological pathways that are important for the development and progression of GC (Figure 5B).
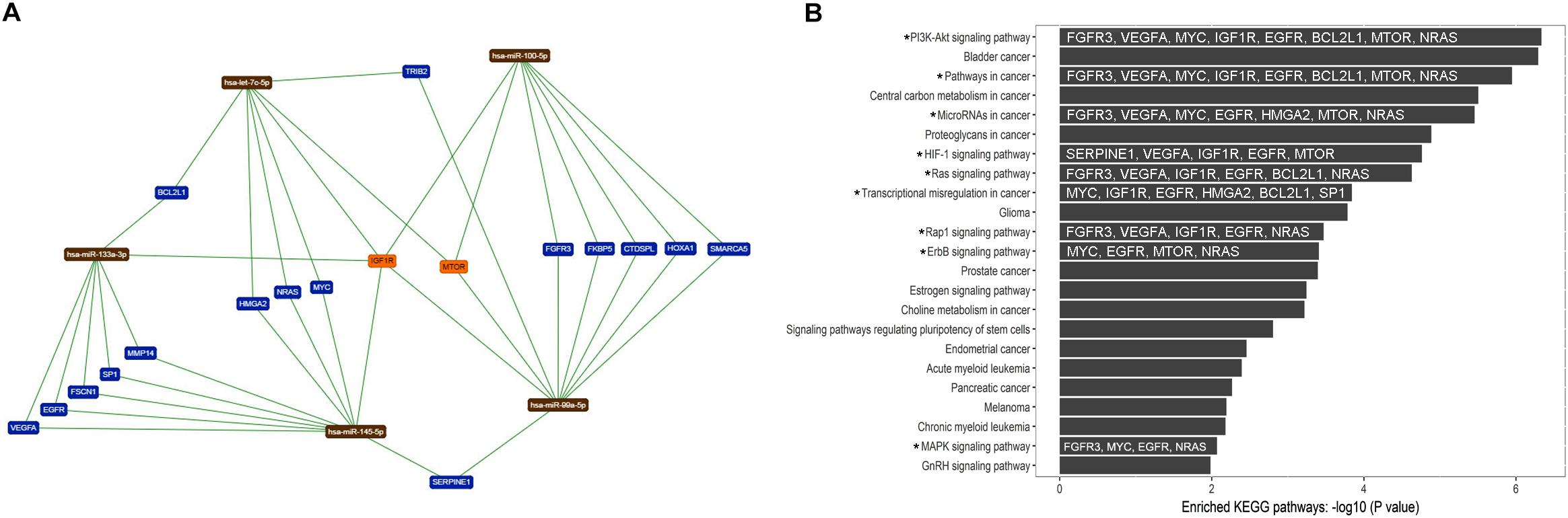
Figure 5. Target genes common to the five up-regulated TS-miRs in adjacent tissue and their relationship to important biological pathways. (A) Network of interaction among miRNAs and target genes generated by miRTargetLink Human (Hamberg et al., 2016). (B) Enhanced KEGG biological pathways in which the target genes of the studied miRNAs participate (by DAVID v.6.8). (*) Important pathway in GC. The target genes involved in important pathways in GC are in white inside the dark bars.
Differentially Expressed miRNAs Common to GC vs. ADJ vs. NC Analysis
hsa-miR-125b-5p, miR-125b-1-3p, -miR-200a-3p, miR-218-1-3p, -miR-490-3p, -miR-493-5p and -miR-873-5p were D. E. in both the GC vs. NC (Table 3 and Supplementary Table S1) and ADJ vs. NC (Table 3 and Supplementary Table S2) analyses. These miRNAs were not D. E. in the GC vs. ADJ analysis (Table 3), suggesting that these two tissues are similar regarding the expression of these miRNAs. In addition, the DAPC plot (Figure 6) generated by all D. E. miRNAs indicates that GC and ADJ, despite generating distinct clusters, have much more similarity in its expression profiles when compared with NC tissue, which clustered apart. Thus, we assembled the GC and ADJ samples in a single group to compare to the NC samples during the ROC curve analysis. Two down-regulated miRNAs (hsa-miR-200a-3p and hsa-miR-873-5p) had the best sensitivity/specificity relation and were considered as potential biomarkers to identify gastric carcinogenesis (Figures 7A,B).
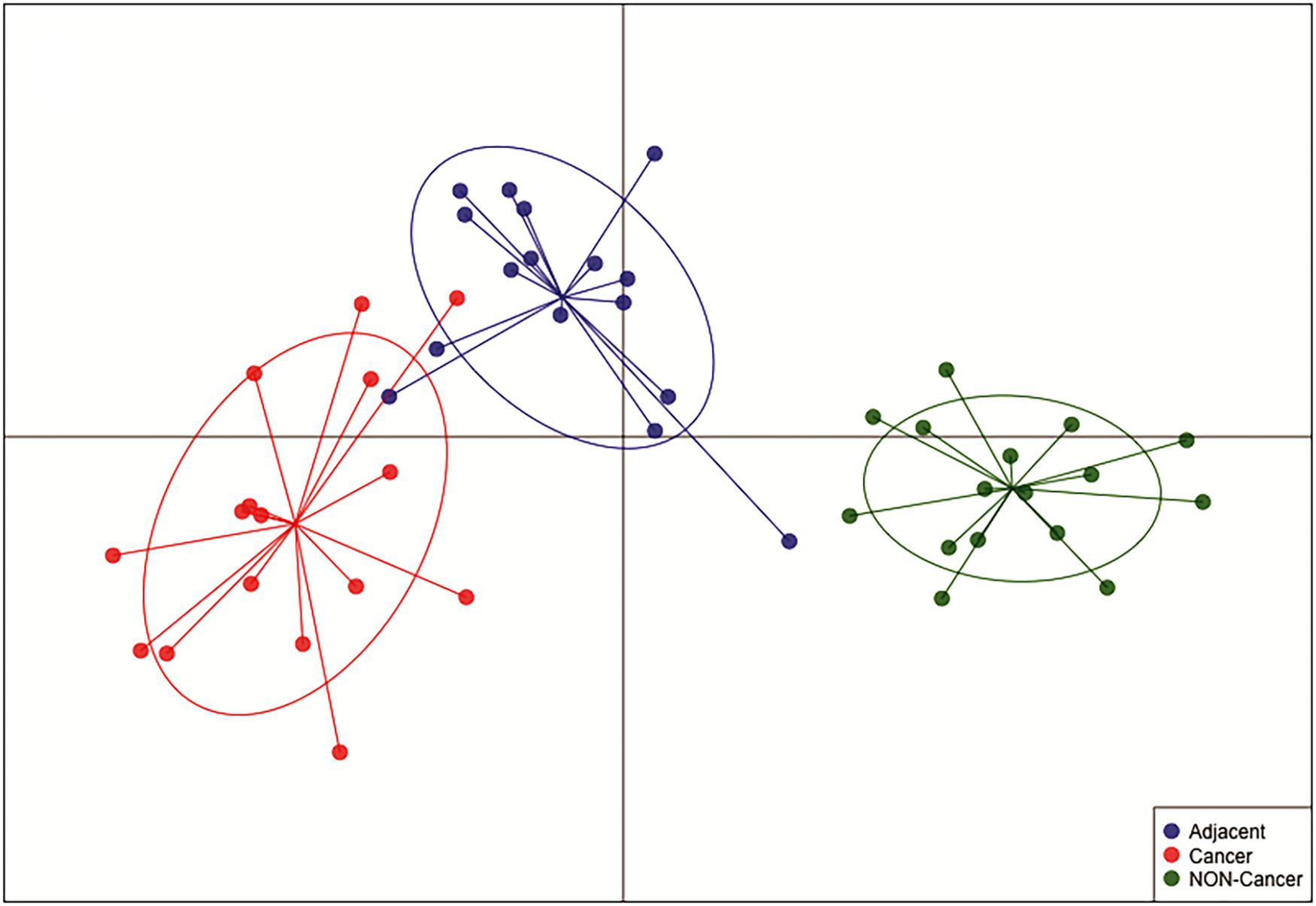
Figure 6. Discriminant analysis of principal component (DAPC) plot shows that NC, ADJ, and GC samples form distinct clusters. The DAPC analysis showed great similarity between GC and ADJ tissues.
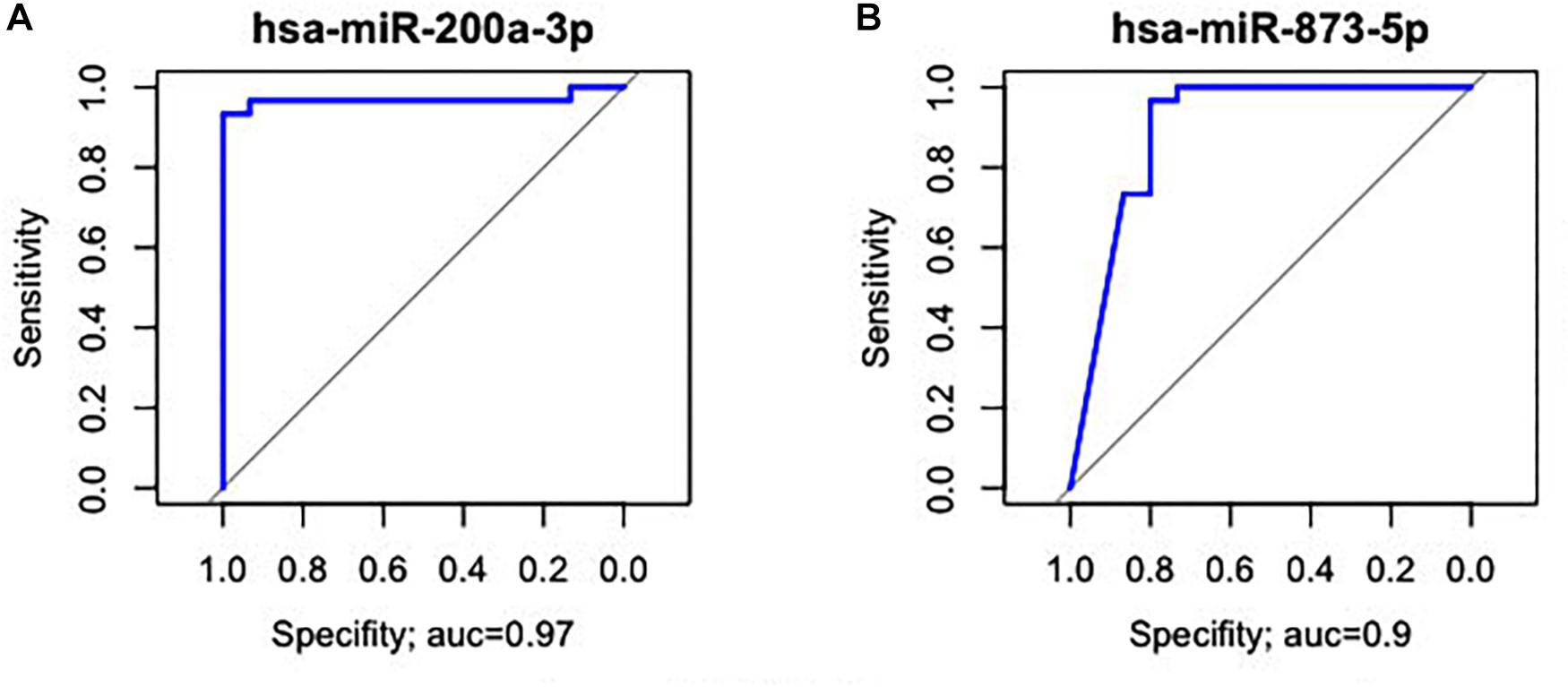
Figure 7. Receiver operating characteristic curve analysis of the two miRNAs with the potential to predict changes in both ADJ and GC tissues. (A) hsa-miR-200a-3p (AUC = 0.97; 95% CI: 0.983–1.000). (B) hsa-miR-873-5p (AUC = 0.90; 95% CI: 0.807–1.000).
Comparing GC with ADJ, only one miRNA (hsa-miR-196a-5p) was differentially expressed in GC (Figure 1 and Table 1). hsa-miR-196a-5p was significantly up-regulated in GC (P = 0.029) but had a low sensitivity/specificity relation in the ROC curve analysis (AUC < 0.85). hsa-miR-196a-5p was also up-regulated in the GC vs. NC comparison (Table 1 and Supplementary Table S1). The difference in the number of miRNAs D. E. in GC vs. ADJ compared with the previous analysis corroborates to premise of that these two tissues have similar miRNA expression profiles.
Discussion
miRNA deregulation is closely related to gastric cancer development (Wu et al., 2014; Zhang et al., 2014) and its relationship to field cancerization becomes evident (Assumpção et al., 2015; Pereira et al., 2019). In the search for new biomarkers and a better understanding of epigenetic field cancerization in GC, we evaluated the global expression profile of miRNAs in NC, ADJ, and GC gastric samples. Our data showed that all three types of tissue share many differentially expressed miRNAs and present miRNAs that occur exclusively in either ADJ or GC tissues, behaving as molecular signatures for those conditions (Tables 1, 2).
Among the deregulated miRNAs only in GC (Table 1), seven up-regulated (hsa-miR-135b-5p, -miR-196a-5p, -miR-196b-5p, -miR-215-5p, -miR-224-5p, -miR-615-3p, and -miR-25-5p) and five down-regulated (hsa-miR-135a-5p, -miR-148a-3p, -miR-148a-5p, -miR-153-3p, and -miR-375) miRNAs are likely oncomiRs and TS-miRs, respectively. In fact, studies demonstrate that miR-135b (Wang et al., 2012; Lu et al., 2018; Shao et al., 2019), miR-196a (Pan et al., 2017), miR-196b (Liao et al., 2012), miR-215 (Deng et al., 2014), miR-224 (He et al., 2017), miR-615-3p (Wang et al., 2018), and miR-25 (Li B.S. et al., 2015; LArki et al., 2018) were reported as oncomiRs, while miR-135a (Zhang et al., 2016; Xie et al., 2019), miR-148a (Zheng et al., 2011), miR-153 (Wang and Liu, 2016; Ouyang et al., 2018) and miR-375 (Ding et al., 2010; Chen et al., 2017; Hwang et al., 2018) were reported as TS-miRs in GC. These results suggest that the joint deregulation of both oncomiRs and TS-miRs is required for the support and progression of GC.
hsa-miR-196a-5p was up-regulated in GC when comparing to ADJ and NC tissues; at the same time, it was not differentially expressed between the ADJ and NC tissues. Deregulation of this miRNA is important for GC progression because it promotes cell proliferation by down-regulating the expression of CDKN1B (p27kip1) tumor suppressor (Sun et al., 2012) and invasion and epithelial to mesenchymal transition of cancer stem cells by down-regulating the expression of SMAD4 in GC (Pan et al., 2017). In addition, hsa-miR-196a has been associated with metastasis in lymph nodes and the clinical stage of GC (Li H.L. et al., 2015).
Nine miRNAs were found to be up-regulated only in ADJ tissue (hsa-miR-let7-c-5p, -miR-99a-5p, -miR-100-5p, -miR-133a-3p, -miR-133b-3p, -miR-143-5p, -miR-145-3p, -miR-145-5p, and -miR-320b) and may act as TS-miRs in this tissue. Studies have shown that miR-let7-c (Tsai et al., 2015), miR-133a (Qiu et al., 2014; Zhang et al., 2018), miR-133b (Qiu et al., 2014; Yang et al., 2017), miR-143-5p (Wu et al., 2013; Lei et al., 2017), miR-145 (Qiu et al., 2014; Lei et al., 2017), miR-100-5p (Shi et al., 2015), and miR-320b (Zhao et al., 2017) are down-regulated in GC and play the role of TS-miRs because they inhibit proliferation, migration, invasion, and cell cycle progression.
Gene enrichment analysis in KEGG pathways revealed that these miRNAs regulate genes involved in important pathways that contribute to gastric carcinogenesis, such as the PI3K-Akt, HIF-1, Ras, Rap1, ErbB, and MAPK signaling pathways (Figures 5A,B). These pathways control important cell functions such as proliferation, migration, invasion, and progression of the cell cycle. The overexpression of TS-miRs in ADJ tissue may be a mechanism to compensate for preexisting molecular alterations in an attempt to contain the tumorigenesis process. However, we believe that during the progression of carcinogenesis in ADJ tissue, the up-regulated TS-miRs identified herein are inversely deregulated (become down-regulated), contributing to the eventual onset, establishment and progression of GC. In a previous study, we reported the overexpression of the TS-miR hsa-miR-29c in adjacent to the gastric cancer tissues and its down-regulation in GC and NC tissues (Pereira et al., 2019).
The intense inflammatory process to which the adjacent tissue is subjected may be one of the causes of over representation of these TS-miRs, since inflammation can alter the local microenvironment by stimulating the expression of some miRNAs. Studies have shown that IL-6 and IL-17 pro-inflammatory interleukins activity may stimulate the expression of miRNAs with known oncogenic activity (miR-21 and miR-135b) in tissues submitted to the intense inflammatory process (Löffler et al., 2007; Iliopoulos et al., 2010; Matsuyama et al., 2011; Rozovski et al., 2013; Singh et al., 2015). Another possibility would be the existence of pre-cancerous lesions in adjacent tissues (e.g., atrophic and non-atrophic gastritis and intestinal metaplasia) and/or Helicobacter pylori infection, since the expression levels of many miRNAs (e.g., miR-21, miR-29c, miR-135b, miR-155, miR-204, and miR-223) may be change under these conditions (Link et al., 2015; Vidal et al., 2016; Pereira et al., 2019). Therefore, the local microenvironment and the histopathological characteristics of these tissues can directly influence the expression of many miRNAs, making them susceptible to eventual molecular alterations and carcinogenesis.
Our data (overexpression of nine TS-miRs only in adjacent to the gastric cancer tissues) corroborate with the findings of Aran et al. (2017), who used the transcriptomic profile of normal tissue adjacent to the tumor (NAT) to demonstrate that this tissue has an intermediate and unique expression profile when compared to truly normal and cancerous tissues. Therefore, despite the similarity between adjacent and tumor tissues, the former is not a malignant tissue, but it is not a molecularly normal tissue either (De Assumpção et al., 2016; Aran et al., 2017).
Many genetic and epigenetic changes identified in GC are shared by ADJ tissue (Assumpção et al., 2015; Takeshima et al., 2015; Aran et al., 2017; Vidal et al., 2017; Yoshida et al., 2017; Pereira et al., 2019). Probably some of these shared alterations were already present in the adjacent tissue before the onset of the tumor in situ and contributed to its establishment, making this tissue still susceptible to carcinogenesis even after surgical removal of the tumor.
We found that both ADJ and GC tissues share the up-regulation of hsa-miR-125b-5p and hsa-miR-125b-1-3p oncomiRs and the down-regulation of hsa-miR-200a-3p and hsa-miR-873-5p TS-miRs, suggesting that the joint deregulation of both oncomiRs and TS-miRs is required for the progression of gastric carcinogenesis. In addition, seven miRNAs (hsa-miR-125b-5p, -miR-125b-1-3p, -miR-200a-3p, -miR-218-1-3p, -miR-490-3p, -miR-873-5p, and -miR-493-5p) are deregulated in both ADJ and GC tissues when compared to NC tissue; however, these miRNAs are not D. E. between ADJ and GC tissues. Our results suggest that these two tissues share molecular alterations and that ADJ is an epigenetically altered tissue. Five miRNAs (hsa-miR-125b-5p, -miR-125b-1-3p, -miR-493-5p, -miR-200a-3p, and -miR-873-5p) can promote tumor onset and progression, as studies have shown that the deregulation of miR-125b (Wu et al., 2015; Zhang et al., 2017b), miR-493-5p (Zhou et al., 2015), miR-200a (Cong et al., 2013), and miR-873 (Cao et al., 2016; Chen et al., 2016) contributes to cell proliferation, migration, invasion, and cell growth in GC.
Although we have not performed further experimental assays to confirm the deregulation and the functional role of the identified miRNAs in our study, the literature consistently supports and corroborates our findings and hypothesis through studies that have applied safe, sensitive and reliable techniques and methods (e.g., RT-qPCR, Western Blot, Cell and/or Reporter Assays) that provides strong evidences of these miRNAs’ deregulation in GC (Ding et al., 2010; Zheng et al., 2011; Liao et al., 2012; Sun et al., 2012; Wang et al., 2012, 2017, 2018; Cong et al., 2013; Wu et al., 2013, 2015; Deng et al., 2014, 2017; Qiu et al., 2014; Li B.S. et al., 2015; Shen et al., 2015; Shi et al., 2015; Tsai et al., 2015; Zhou et al., 2015, 2019; Zuo et al., 2015; Cao et al., 2016; Chen et al., 2016, 2017; Gao et al., 2016; Tang et al., 2016; Vidal et al., 2016; Wang and Liu, 2016; Zhang et al., 2016, 2017a, 2017b, 2018; He et al., 2017; Lei et al., 2017; Pan et al., 2017; Qu et al., 2017; Yang et al., 2017; Zhao et al., 2017; Hwang et al., 2018; LArki et al., 2018; Lu et al., 2018; Magalhães et al., 2018; Ouyang et al., 2018; Pereira et al., 2019; Shao et al., 2019; Xie et al., 2019; Yu et al., 2019).
For the evaluation of the studied miRNAs as potential biomarkers, we selected three different groups of markers with AUC > 85%. Among the D. E. miRNAs only in GC, six miRNAs (hsa-miR-141-3p, -miR-148a-3p, -miR-148b-5p, -miR-153-3p, -miR-196a-5p, and -miR-375) are potentially useful in identifying patients with advanced disease (Figure 3). Among the deregulated miRNAs only in ADJ tissue, five (hsa-miR-99a-5p, -miR-100-5p, -miR-125b-5p, -miR-145-3p, and -miR-145-5p) are potentially useful in identifying patients susceptible to tumor development or the early stages of gastric cancer (Figure 4). Two miRNAs (hsa-miR-200a-3p and hsa-miR-873-5p) are potentially useful in identifying both patients with established disease and patients susceptible to developing it (Figure 7). Thus, these miRNAs may be a potential diagnostic alternative for this type of cancer because the currently available biomarkers do not have a good sensitivity/specificity relationship, which makes it difficult to diagnose the disease early and to start curative treatment.
Studies that analyzed the data from miRNA sequencing in gastric cancer demonstrated their deregulation in this type of tumor (Assumpção et al., 2015; Darnet et al., 2015; Liu et al., 2015; Liang et al., 2017). Among the miRNAs found in these studies, hsa-miR-99a, -miR-100, -miR-133a/b-3p, -miR-135b, -miR-141, -miR-143, -miR-145, -miR-148a, -miR-196a/b, -miR-200a, -miR-218-1, -miR-215, and -miR-490 were also observed in the present study. In addition to those miRNAs, we also found 16 novel differentially expressed miRNAs in the gastric field cancerization.
Liu et al. (2015) analyzed miRNome in gastric cancer downloaded from the TCGA; however, this database basically has samples from European and Asian populations. These authors identified 54 D. E. miRNAs (using the Padj < 0.05 and |log2 (fold change)| > 3) and two miRNAs (hsa-miR-133a/b) were the most D. E. Liang et al. (2017) also analyzed miRNomes in GC using samples from the same database (TCGA) and identified 43 D. E. miRNAs (using the FDR < 0.001 and | log2 (fold change)| > 1.5); of these, 5 miRNAs (hsa-miR-30a, -miR-135b, -miR-133b, -miR-143, and -miR-145) were associated with patient survival time. We emphasize that the “normal” or “healthy” tissues used in these two studies are of patients with the disease (adjacent to the gastric cancer tissues), since the TCGA does not have expression data of gastric tissues of patients without the history of GC. By using samples from the Brazilian population (that has genetic admixture), we identified 30 miRNAs differentially expressed when comparing patients with GC and individuals without the history of GC (we considered Padj < 0.05 and | log2(fold change)| > 2). In addition, we found that the hsa-miR-196a-5p was D. E. between the GC and ADJ tissues. Many D. E. miRNAs identified by Liu et al. (2015) and Liang et al. (2017) were also identified in this study; however, there are differences in the number of D. E. miRNAs found among these studies (mainly in GC and ADJ analysis), which may be a consequence of: (i) the statistical criteria used, (ii) the number of samples used, and/or (iii) the ethnical and genetic characteristics of the studied populations. Our data are important because we analyzed the miRNA sequencing of a larger number of samples from the Brazilian population. This population has a genetic contribution from different parental populations, such as the European, African, Asian, and Amerindian ones (Santos et al., 2010; Andrade et al., 2018). The strong genetic substructure and admixture (Santos et al., 2010) of our population may interfere in the expression profile of some genes (Pinto et al., 2015; Dluzen et al., 2016). Therefore, this study provides important and relevant information about the expression profile of miRNAs associated to gastric field cancerization in populations with genetic substructure and admixture, such as the Brazilian one.
Overall, our study was able to demonstrate that the tissue adjacent to gastric cancer shares some epigenetic changes (miRNAs deregulation) present in the tumor and also has unique and exclusive alterations; therefore, it should not be used as a healthy and/or normal tissue as a benchmark for gastric cancer. Thus, we recommend the use of gastric samples from patients with no history of GC as a control to exclude any biases that the adjacent tissue may provide in the miRNAs’ expression profile in GC.
In this study, we also observed that the tissues adjacent to GC have an over representation of microRNAs with known tumor suppressor activities, suggesting that these microRNAs may represent a barrier against tumorigenesis within these pre-cancerous tissues prior to the eventual formation of a tumor. The excellent performance of the studied miRNAs in identifying with good sensitivity and specificity, both early and advanced stages of the disease, make them potentially useful as biomarkers and therapeutic targets for GC.
Ethics Statement
This study was carried out in accordance with the recommendations of the National Health Council’s Research Guidelines Involving Human Beings (Res. CNS 466/12), Research Ethics Committees and National Research Ethics Commission. The protocol was approved by the Ethics Committee of the Center of Oncology Research of the Federal University of Pará (Protocol No. 1.081.340). All subjects gave written informed consent in accordance with the Declaration of Helsinki.
Author Contributions
AP and FM study design, formal analyses, interpretation of data, and drafting of manuscript. ÂRS, PA, and SS study concept and design and obtaining funding. PA, MA, and AC provided clinical materials. SD histopathological evaluation. TV-S, AV, PP, LM, and AMRS prepared nucleic acids, sequencing libraries, and performed the experiments. All authors reviewed the manuscript.
Funding
This study was supported by Rede de Pesquisa em Genômica Populacional Humana (CAPES/Biologia Computacional: No. 3381/2013/CAPES) and PROPESP/UFPA. AP was supported by CAPES Ph.D. fellowship (PROEX – 0487) in Genetics and Molecular Biology (UFPA) and is supported actually by CAPES/BRASIL Post-Doctoral fellowship (Edital No. 51/2013/Biologia Computacional; Proc. No. 88887.313819/2019-00) in Oncology and Medical Sciences (UFPA). ÂRS was supported by CNPq/Productivity: 304413/2015-1 and SS was supported by CNPq/Productivity: 305 256/2013-3.
Conflict of Interest Statement
The authors declare that the research was conducted in the absence of any commercial or financial relationships that could be construed as a potential conflict of interest.
Acknowledgments
We thank to Coordenação de Aperfeiçoamento de Pessoal de Nível Superior (CAPES), Conselho Nacional de Desenvolvimento Científico e Tecnológico (CNPq), and PROPESP/UFPA for the financial support and fellowships.
Supplementary Material
The Supplementary Material for this article can be found online at: https://www.frontiersin.org/articles/10.3389/fgene.2019.00592/full#supplementary-material
References
Anders, S., Pyl, P. T., and Huber, W. (2015). HTSeq—a Python framework to work with high-throughput sequencing data. Bioinformatics 31, 166–169. doi: 10.1093/bioinformatics/btu638
Andrade, R. B., Amador, M. A. T., Cavalcante, G. C., Leitão, L. P. C., Fernandes, M. R., Modesto, A. A. C., et al. (2018). Estimating Asian contribution to the Brazilian population: a new application of a validated set of 61 ancestry informative markers. G3 8, 3577–3582. doi: 10.1534/g3.118.200650
Aran, D., Camarda, R., Odegaard, J., Paik, H., Oskotsky, B., Krings, G., et al. (2017). Comprehensive analysis of normal adjacent to tumor transcriptomes. Nat. Commun. 8:1077. doi: 10.1038/s41467-017-01027-z
Assumpção, M. B., Moreira, F. C., Hamoy, I. G., Magalhães, L., Vidal, A., Pereira, A., et al. (2015). High-throughput miRNA sequencing reveals a field effect in gastric cancer and suggests an epigenetic network mechanism. Bioinform. Biol. Insights 9, 111–117. doi: 10.4137/BBI.S24066
Cao, D., Yu, T., and Ou, X. (2016). MiR-873-5P controls gastric cancer progression by targeting hedgehog-GLI signaling. Pharmazie 71, 603–606. doi: 10.1691/ph.2016.6618
Castro-Magdonel, B. E., Orjuela, M., Camacho, J., García-Chéquer, A. J., Cabrera-Muñoz, L., Sadowinski-Pine, S., et al. (2017). miRNome landscape analysis reveals a 30 miRNA core in retinoblastoma. BMC Cancer 17:458. doi: 10.1186/s12885-017-3421-3
Chai, H., and Brown, R. E. (2009). Field effect in cancer-an update. Ann. Clin. Lab. Sci. 39, 331–337.
Chen, B., Guo, S., Yu, Z., Feng, Y., and Hui, L. (2017). Downregulation of microRNA-375, combined with upregulation of its target gene Janus kinase 2, predicts unfavorable prognosis in patients with gastric cancer. Int. J. Clin. Exp. Pathol. 10, 11106–11113.
Chen, X., Chen, R., Wu, W., and Huang, Z. (2016). MicroRNA-873 inhibits proliferation and induces apoptosis by targeting CXCL1 in gastric cancer. Int. J. Clin. Exp. Pathol. 9, 10011–10019.
Cong, N., Du, P., Zhang, A., Shen, F., Su, J., Pu, P., et al. (2013). Downregulated microRNA-200a promotes EMT and tumor growth through the wnt/β-catenin pathway by targeting the E-cadherin repressors ZEB1/ZEB2 in gastric adenocarcinoma. Oncol. Rep. 29, 1579–1587. doi: 10.3892/or.2013.2267
Correa, P. (2013). Gastric cancer: overview. Gastroenterol. Clin. N. Am. 42, 211–217. doi: 10.1016/j.gtc.2013.01.002
Curtius, K., Wright, N. A., and Graham, T. A. (2018). An evolutionary perspective on field cancerization. Nat. Rev. Cancer 18, 19–32. doi: 10.1038/nrc.2017.102
Darnet, S., Moreira, F. C., Hamoy, I. G., Burbano, R., Khayat, A., Cruz, A., et al. (2015). High-throughput sequencing of miRNAs reveals a tissue signature in gastric cancer and suggests novel potential biomarkers. Bioinform. Biol. Insights 9, (Suppl. 1), 1–8. doi: 10.4137/BBI.S23773
De Assumpção, P. P., Dos Santos, S. E., Dos Santos, ÂK., Demachki, S., Khayat, A. S., Ishak, G., et al. (2016). The adjacent to tumor sample trap. Gastric Cancer 19, 1024–1025. doi: 10.1007/s10120-015-0539-3
Deng, M., Zeng, C., Lu, X., He, X., Zhang, R., Qiu, Q., et al. (2017). miR-218 suppresses gastric cancer cell cycle progression through the CDK6/Cyclin D1/E2F1 axis in a feedback loop. Cancer Lett. 403, 175–185. doi: 10.1016/j.canlet.2017.06.006
Deng, Y., Huang, Z., Xu, Y., Jin, J., Zhuo, W., Zhang, C., et al. (2014). MiR-215 modulates gastric cancer cell proliferation by targeting RB1. Cancer Lett. 342, 27–35. doi: 10.1016/j.canlet.2013.08.033
Ding, L., Xu, Y., Zhang, W., Deng, Y., Si, M., Du, Y., et al. (2010). MiR-375 frequently downregulated in gastric cancer inhibits cell proliferation by targeting JAK2. Cell Res. 20, 784–793. doi: 10.1038/cr.2010.79
Dluzen, D. F., Noren, H. N., Zhang, Y., Kim, Y., Glover, F. E., Tajuddin, S. M., et al. (2016). Racial differences in microRNA and gene expression in hypertensive women. Sci. Rep. 6:35815. doi: 10.1038/srep35815
Dobin, A., Davis, C. A., Schlesinger, F., Drenkow, J., Zaleski, C., Jha, S., et al. (2013). STAR: ultrafast universal RNA-seq aligner. Bioinformatics 29, 15–21. doi: 10.1093/bioinformatics/bts635
Ferlay, J., Soerjomataram, I., Dikshit, R., Eser, S., Mathers, C., Rebelo, M., et al. (2015). Cancer incidence and mortality worldwide: sources, methods and major patterns in GLOBOCAN 2012. Int. J. Cancer 136, E359–E386. doi: 10.1002/ijc.29210
Gao, H., Liu, P., Yang, Y., and Gao, F. (2016). Decreased miR-452 expression and its tumor suppressive function in human gastric cancer. Int. J. Clin. Exp. Med. 9, 16078–16085.
Hamberg, M., Backes, C., Fehlmann, T., Hart, M., Meder, B., Meese, E., et al. (2016). MiRTargetLink—miRNAs, Genes and Interaction Networks. Int. J. Mol. Sci. 17:564. doi: 10.3390/ijms17040564
Hattori, N., and Ushijima, T. (2016). Epigenetic impact of infection on carcinogenesis: mechanisms and applications. Genome Med. 8:10. doi: 10.1186/s13073-016-0267-2
He, C., Wang, L., Zhang, J., and Xu, H. (2017). Hypoxia-inducible microRNA-224 promotes the cell growth, migration and invasion by directly targeting RASSF8 in gastric cancer. Mol. Cancer 16:35. doi: 10.1186/s12943-017-0603-1
Hou, J., Lin, L., Zhou, W., Wang, Z., Ding, G., Dong, Q., et al. (2011). Identification of miRNomes in human liver and hepatocellular carcinoma reveals miR-199a/b-3p as therapeutic target for hepatocellular carcinoma. Cancer Cell 19, 232–243. doi: 10.1016/j.ccr.2011.01.001
Huang, W. D., Sherman, B. T., and Lempicki, R. A. (2009a). Bioinformatics enrichment tools: paths toward the comprehensive functional analysis of large gene lists. Nucleic Acids. Res. 37, 1–13. doi: 10.1093/nar/gkn923
Huang, W. D., Sherman, B. T., and Lempicki, R. A. (2009b). Systematic and integrative analysis of large gene lists using DAVID bioinformatics resources. Nat. Protoc. 4, 44–57. doi: 10.1038/nprot.2008.211
Hwang, J., Min, B.-H., Jang, J., Kang, S. Y., Bae, H., Jang, S. S., et al. (2018). MicroRNA expression profiles in gastric carcinogenesis. Sci. Rep. 8:14393.
Iliopoulos, D., Jaeger, S. A., Hirsch, H. A., Bulyk, M. L., and Struhl, K. (2010). STAT3 activation of miR-21 and miR-181b-1 via PTEN and CYLD are part of the epigenetic switch linking inflammation to cancer. Mol. Cell 39, 493–506. doi: 10.1016/j.molcel.2010.07.023
LArki, P., Ahadi, A., Zare, A., Tarighi, S., Zaheri, M., Souri, M., et al. (2018). Up-Regulation of miR-21, miR-25, miR-93, and miR-106b in Gastric Cancer. Iran Biomed. J. 22, 367–373.
Lauren, P. (1965). The two histological main types of gastric carcinoma: diffuse and so-called intestinal-type carcinoma. An attempt at a histo-clinical classification. Acta Pathol. Microbiol. Scand. 64, 31–49. doi: 10.1111/apm.1965.64.1.31
Lei, C., Du, F., Sun, L., Li, T., Li, T., Min, Y., et al. (2017). miR-143 and miR-145 inhibit gastric cancer cell migration and metastasis by suppressing MYO6. Cell Death Dis. 8:e3101. doi: 10.1038/cddis.2017.493
Li, B. S., Zuo, Q. F., Zhao, Y. L., Xiao, B., Zhuang, Y., Mao, X. H., et al. (2015). MicroRNA-25 promotes gastric cancer migration, invasion and proliferation by directly targeting transducer of ERBB2, 1 and correlates with poor survival. Oncogene 34, 2556–2565. doi: 10.1038/onc.2014.214
Li, H. L., Xie, S. P., Yang, Y. L., Cheng, Y. X., Zhang, Y., Wang, J., et al. (2015). Clinical significance of upregulation of mir-196a-5p in gastric cancer and enriched KEGG pathway analysis of target genes. Asian Pac. J. Cancer Prev. 16, 1781–1787. doi: 10.7314/apjcp.2015.16.5.1781
Liang, L., Zhang, L., Cui, D., and Yang, D. (2017). Identification of the key miRNAs associated with survival time in stomach adenocarcinoma. Oncol. Lett. 14, 4563–4572. doi: 10.3892/ol.2017.6792
Liao, Y. L., Hu, L. Y., Tsai, K. W., Wu, C. W., and Chan, W. C. (2012). Transcriptional regulation of miR-196b by ETS2 in gastric cancer cells. Carcinogenesis 33, 760–769. doi: 10.1093/carcin/bgs023
Link, A., Schirrmeister, W., Langner, C., Varbanova, M., Bornschein, J., Wex, T., et al. (2015). Differential expression of microRNAs in preneoplastic gastric mucosa. Sci. Rep. 5:8270. doi: 10.1038/srep08270
Liu, Y., Zhang, X., Zhang, Y., Hu, Z., Yang, D., Wang, C., et al. (2015). Identification of miRNomes in human stomach and gastric carcinoma reveals miR-133b/a-3p as therapeutic target for gastric cancer. Cancer Lett. 369, 58–66. doi: 10.1016/j.canlet.2015.06.028
Löffler, D., Brocke-Heidrich, K., Pfeifer, G., Stocsits, C., Hackermüller, J., Kretzschmar, A. K., et al. (2007). Interleukin-6 dependent survival of multiple myeloma cells involves the Stat3-mediated induction of microRNA-21 through a highly conserved enhancer. Blood 110, 1330–1333. doi: 10.1182/blood-2007-03-081133
Love, M. I., Huber, W., and Anders, S. (2014). Moderated estimation of fold change and dispersion for RNA-seq data with DESeq2. Genome Biol. 15:550. doi: 10.1186/s13059-014-0550-8
Lu, M., Huang, Y., Sun, W., Li, P., Li, L., and Li, L. (2018). miR-135b-5p promotes gastric cancer progression by targeting CMTM3. Int. J. Oncol. 52, 589–598. doi: 10.3892/ijo.2017.4222
Magalhães, L., Quintana, L. G., Lopes, D. C. F., Vidal, A. F., Pereira, A. L., D’Araujo, P. L. C., et al. (2018). APC gene is modulated by hsa-miR-135b-5p in both diffuse and intestinal gastric cancer subtypes. BMC Cancer 18:1055. doi: 10.1186/s12885-018-4980-7
Maltseva, D. V., Galatenko, V. V., Samatov, T. R., Zhikrivetskaya, S. O., Khaustova, N. A., Nechaev, I. N., et al. (2014). miRNome of inflammatory breast cancer. BMC Res. Notes 7:871. doi: 10.1186/1756-0500-7-871
Matsuyama, H., Suzuki, H. I., Nishimori, H., Noguchi, M., Yao, T., Komatsu, N., et al. (2011). miR-135b mediates NPM-ALK-driven oncogenicity and renders IL-17-producing immunophenotype to anaplastic large cell lymphoma. Blood 118, 6881–6892. doi: 10.1182/blood-2011-05-354654
Moreira, F. C., Assumpção, M., Hamoy, I. G., Darnet, S., Burbano, R., Khayat, A., et al. (2014a). MiRNA expression profile for the human gastric antrum region using ultra-deep sequencing. PLoS One 9:e92300. doi: 10.1371/journal.pone.0092300
Moreira, F. C., Dustan, B., Hamoy, I. G., Ribeiro-dos-Santos, A. M., and Ribeiro-dos-Santos, Â (2014b). TargetCompare: a web interface to compare simultaneous miRNAs targets. Bioinformation 10, 602–605. doi: 10.6026/97320630010602
Ouyang, Y., Yuan, W., and Qiu, S. (2018). MicroRNA-153 functions as a tumor suppressor in gastric cancer via targeting Kruppel-like factor 5. Exp. Ther. Med. 16, 473–482. doi: 10.3892/etm.2018.6226
Padmanabhan, N., Ushijima, T., and Tan, P. (2017). How to stomach an epigenetic insult: the gastric cancer epigenome. Nat. Rev. Gastroenterol. Hepatol. 14, 467–478. doi: 10.1038/nrgastro.2017.53
Pan, Y., Shu, X., Sun, L., Yu, L., Sun, L., Yang, Z., et al. (2017). miR-196a-5p modulates gastric cancer stem cell characteristics by targeting Smad4. Int. J. Oncol. 50, 1965–1976. doi: 10.3892/ijo.2017.3965
Pereira, A. L., Magalhães, L., Moreira, F. C., Reis-das-Mercês, L., Vidal, A. F., Ribeiro-dos-Santos, A. M., et al. (2019). Epigenetic field cancerization in gastric cancer: microRNAs as promising biomarkers. J. Cancer 10, 1560–1569. doi: 10.7150/jca.27457
Pinto, P. S. C., Santos, N. P., Santos, S., and Ribeiro-dos-Santos, Â (2015). Influence of genetic ancestry on INDEL markers of NFKβ1, CASP8, PAR1, IL4 and CYP19A1 genes in leprosy patients. PLoS Negl. Trop. Dis. 9:e0004050. doi: 10.1371/journal.pntd.0004050
Qiu, T., Zhou, X., Wang, J., Du, Y., Xu, J., Huang, Z., et al. (2014). MiR-145, miR-133a and miR-133b inhibit proliferation, migration, invasion and cell cycle progression via targeting transcription factor Sp1 in gastric cancer. FEBS Lett. 588, 1168–1177. doi: 10.1016/j.febslet.2014.02.054
Qu, M., Li, L., and Zheng, W. C. (2017). Reduced miR-490-3p expression is associated with poor prognosis of Helicobacter pylori induced gastric cancer. Eur. Rev. Med. Pharmacol. Sci. 21, 3384–3388.
Ribeiro-dos-Santos, Â, Khayat, A. S., Silva, A., Alencar, D. O., Lobato, J., Luz, L., et al. (2010). Ultra-deep sequencing reveals the microRNA expression pattern of the human stomach. PLoS One 5:e13205. doi: 10.1371/journal.pone.0013205
Rozovski, U., Calin, G. A., Setoyama, T., D’Abundo, L., Harris, D. M., Li, P., et al. (2013). Signal transducer and activator of transcription (STAT)-3 regulates microRNA gene expression in chronic lymphocytic leukemia cells. Mol. Cancer 12:50. doi: 10.1186/1476-4598-12-50
Runtsch, M. C., Round, J. L., and O’Connell, R. M. (2014). MicroRNAs and the regulation of intestinal homeostasis. Front. Genet. 5:347. doi: 10.3389/fgene.2014.00347
Santos, N. P., Ribeiro-Rodrigues, E. M., Ribeiro-Dos-Santos, A. K., Pereira, R., Gusmão, L., Amorim, A., et al. (2010). Assessing individual interethnic admixture and population substructure using a 48-insertion-deletion (INSEL) ancestry-informative marker (AIM) panel. Hum. Mutat. 31, 184–190. doi: 10.1002/humu.21159
Schneider, M. R. (2012). MicroRNAs as novel players in skin development, homeostasis and disease. Br. J. Dermatol. 166, 22–28. doi: 10.1111/j.1365-2133.2011.10568.x
Shao, L., Chen, Z., Soutto, M., Zhu, S., Lu, H., Romero-Gallo, J., et al. (2019). Helicobacter pylori-induced miR-135b-5p promotes cisplatin resistance in gastric cancer. FASEB J. 33, 264–274. doi: 10.1096/fj.201701456RR
Shen, J., Xiao, Z., Wu, W. K., Wang, M. H., To, K. F., Chen, Y., et al. (2015). Epigenetic silencing of miR-490-3p reactivates the chromatin remodeler SMARCD1 to promote Helicobacter pylori-induced gastric carcinogenesis. Cancer Res. 75, 754–765. doi: 10.1158/0008-5472.CAN-14-1301
Shi, D. B., Wang, Y. W., Xing, A. Y., Gao, J. W., Zhang, H., Guo, X. Y., et al. (2015). C/EBPα-induced miR-100 expression suppresses tumor metastasis and growth by targeting ZBTB7A in gastric cancer. Cancer Lett. 369, 376–385. doi: 10.1016/j.canlet.2015.08.029
Shin, V. Y., Ng, E. K., Chan, V. W., Kwong, A., and Chu, K. M. (2015). A three-miRNA signature as promising non-invasive diagnostic marker for gastric cancer. Mol. Cancer 14:202. doi: 10.1186/s12943-015-0473-3
Singh, M., Garg, N., Venugopal, C., Hallett, R., Tokar, T., McFarlane, N., et al. (2015). STAT3 pathway regulates lung-derived brain metastasis initiating cell capacity through miR-21 activation. Oncotarget 6, 27461–27477. doi: 10.18632/oncotarget.4742
Slaughter, D. P., Southwick, H. W., and Smejkal, W. (1953). Field cancerization in oral stratified squamous epithelium; clinical implications of multicentric origin. Cancer 6, 963–968. doi: 10.1002/1097-0142(195309)6:5<963::aid-cncr2820060515>3.0.co;2-q
Sun, M., Liu, X. H., Li, J. H., Yang, J. S., Zhang, E. B., Yin, D. D., et al. (2012). MiR-196a is upregulated in gastric cancer and promotes cell proliferation by downregulating p27(kip1). Mol. Cancer Ther. 11, 842–852. doi: 10.1158/1535-7163.MCT-11-1015
Takeshima, H., Niwa, T., Takahashi, T., Wakabayashi, M., Yamashita, S., Ando, T., et al. (2015). Frequent involvement of chromatin remodeler alterations in gastric field cancerization. Cancer Lett. 357, 328–338. doi: 10.1016/j.canlet.2014.11.038
Tang, S., Wang, D., Zhang, Q., and Li, L. (2016). miR-218 suppresses gastric cancer cell proliferation and invasion via regulation of angiopoietin-2. Exp. Ther. Med. 12, 3837–3842. doi: 10.3892/etm.2016.3893
Tsai, K. W., Hu, L. Y., Chen, T. W., Li, S. C., Ho, M. R., Yu, S. Y., et al. (2015). Emerging role of microRNAs in modulating endothelin-1 expression in gastric cancer. Oncol. Rep. 33, 485–493. doi: 10.3892/or.2014.3598
Vidal, A. F., Cruz, A. M. P., Magalhães, L., Pereira, A. L., Anaissi, A. K. M., Alves, N. C. F., et al. (2016). hsa-miR-29c and hsa-miR-135b differential expression as potential biomarker of gastric carcinogenesis. World J. Gastroenterol. 22, 2060–2070. doi: 10.3748/wjg.v22.i6.2060
Vidal, A. F., Ribeiro-Dos-Santos, A. M., Vinasco-Sandoval, T., Magalhães, L., Pinto, P. L., Anaissi, A. K. M., et al. (2017). The comprehensive expression analysis of circular RNAs in gastric cancer and its association with field cancerization. Sci Rep. 7:14551. doi: 10.1038/s41598-017-15061-w
Wang, G., Fu, Y., Liu, G., Ye, Y., and Zhang, X. (2017). miR-218 inhibits proliferation, migration, and EMT of gastric cancer cells by targeting WASF3. Oncol. Res. 25, 355–364. doi: 10.3727/096504016X14738114257367
Wang, J., Liu, L., Sun, Y., Xue, Y., Qu, J., Pan, S., et al. (2018). miR-615-3p promotes proliferation and migration and inhibits apoptosis through its potential target CELF2 in gastric cancer. Biomed. Pharmacother. 101, 406–413. doi: 10.1016/j.biopha.2018.02.104
Wang, L. P., Ma, X. Q., and Cai, J. C. (2012). Clinicopathological significance and function of miR-135b in the occurrence and development of gastric cancer. Zhonghua Yi Xue Za Zhi 92, 3269–3273.
Wang, Z., and Liu, C. (2016). MiR-153 regulates metastases of gastric cancer through Snail. Tumor Biol. 37, 15509–15515. doi: 10.1007/s13277-015-3846-8
Wu, H. H., Lin, W. C., and Tsai, K. W. (2014). Advances in molecular biomarkers for gastric cancer: miRNAs as emerging novel cancer markers. Expert. Rev. Mol. Med. 16, e1. doi: 10.1017/erm.2013.16
Wu, J. G., Wang, J. J., Jiang, X., Lan, J. P., He, X. J., Wang, H. J., et al. (2015). MiR-125b promotes cell migration and invasion by targeting PPP1CA-Rb signal pathways in gastric cancer, resulting in a poor prognosis. Gastric Cancer 18, 729–739. doi: 10.1007/s10120-014-0421-8
Wu, X. L., Cheng, B., Li, P. Y., Huang, H. J., Zhao, Q., Dan, Z. L., et al. (2013). MicroRNA-143 suppresses gastric cancer cell growth and induces apoptosis by targeting COX-2. World J. Gastroenterol. 19, 7758–7765. doi: 10.3748/wjg.v19.i43.7758
Xie, Y., Li, F., Li, Z., and Shi, Z. (2019). miR-135a suppresses migration of gastric cancer cells by targeting TRAF5-mediated NF-κB activation. Onco Targets Ther. 12, 975–984. doi: 10.2147/OTT.S189976
Yakirevich, E., and Resnick, M. B. (2013). Pathology of gastric cancer and its precursor lesions. Gastroenterol. Clin. N. Am. 42, 261–284. doi: 10.1016/j.gtc.2013.01.004
Yang, D., Zhao, D., and Chen, X. (2017). MiR-133b inhibits proliferation and invasion of gastric cancer cells by up-regulating FBN1 expression. Cancer Biomark. 19, 425–436. doi: 10.3233/CBM-160421
Yoshida, S., Yamashita, S., Niwa, T., Mori, A., Ito, S., Ichinose, M., et al. (2017). Epigenetic inactivation of FAT4 contributes to gastric field cancerization. Gastric Cancer 20, 136–145. doi: 10.1007/s10120-016-0593-5
Yu, H., Sun, J., Jiang, S., and Xu, Y. (2019). MicroRNA-490-3p regulates cell proliferation and apoptosis in gastric cancer via direct targeting of AKT1. Exp. Ther. Med. 17, 1330–1336. doi: 10.3892/etm.2018.7042
Zhang, C., Chen, X., Chen, X., Wang, X., Ji, A., Jiang, L., et al. (2016). miR-135a acts as a tumor suppressor in gastric cancer in part by targeting KIFC1. Onco Targets Ther. 9, 3555–3563. doi: 10.2147/OTT.S105736
Zhang, X., Li, Z., Xuan, Z., Xu, P., Wang, W., Chen, Z., et al. (2018). Novel role of miR-133a-3p in repressing gastric cancer growth and metastasis via blocking autophagy-mediated glutaminolysis. J. Exp. Clin. Cancer Res. 37:320. doi: 10.1186/s13046-018-0993-y
Zhang, X., Dong, J., He, Y., Zhao, M., Liu, Z., Wang, N., et al. (2017a). miR-218 inhibited tumor angiogenesis by targeting ROBO1 in gastric cancer. Gene 615, 42–49. doi: 10.1016/j.gene.2017.03.022
Zhang, X., Yao, J., Guo, K., Huang, H., Huai, S., Ye, R., et al. (2017b). The functional mechanism of miR-125b in gastric cancer and its effect on the chemosensitivity of cisplatin. Oncotargetics 9, 2105–2119. doi: 10.18632/oncotarget.23249
Zhang, Z., Li, Z., Li, Y., and Zang, A. (2014). MicroRNA and signaling pathways in gastric cancer. Cancer Gene Ther. 21, 305–316. doi: 10.1038/cgt.2014.37
Zhao, Y., Dong, Q., and Wang, E. (2017). MicroRNA-320 inhibits invasion and induces apoptosis by targeting CRKL and inhibiting ERK and AKT signaling in gastric cancer cells. Onco Targets Ther. 10, 1049–1058. doi: 10.2147/OTT.S123324
Zheng, B., Liang, L., Wang, C., Huang, S., Cao, X., Zha, R., et al. (2011). MicroRNA-148a suppresses tumor cell invasion and metastasis by downregulating ROCK1 in gastric cancer. Clin. Cancer Res. 17, 7574–7583. doi: 10.1158/1078-0432.CCR-11-1714
Zhou, W., Zhang, C., Jiang, H., Zhang, Z., Xie, L., and He, X. (2015). MiR-493 suppresses the proliferation and invasion of gastric cancer cells by targeting RhoC. Iran J. Basic Med. Sci. 18, 1027–1033.
Zhou, Y., Zhong, J. H., Gong, F. S., and Xiao, J. (2019). MiR-141-3p suppresses gastric cancer induced transition of normal fibroblast and BMSC to cancer-associated fibroblasts via targeting STAT4. Exp. Mol. Pathol. 107, 85–94. doi: 10.1016/j.yexmp.2018.11.014
Keywords: miRNome, miRNAs, gastric cancer, field cancerization, biomarkers
Citation: Pereira A, Moreira F, Vinasco-Sandoval T, Cunha A, Vidal A, Ribeiro-dos-Santos AM, Pinto P, Magalhães L, Assumpção M, Demachki S, Santos S, Assumpção P and Ribeiro-dos-Santos  (2019) miRNome Reveals New Insights Into the Molecular Biology of Field Cancerization in Gastric Cancer. Front. Genet. 10:592. doi: 10.3389/fgene.2019.00592
Received: 05 June 2018; Accepted: 04 June 2019;
Published: 19 June 2019.
Edited by:
Ge Shan, University of Science and Technology of China, ChinaReviewed by:
Dominic C. Voon, Kanazawa University, JapanMahshid Malakootian, Iran University of Medical Sciences, Iran
Copyright © 2019 Pereira, Moreira, Vinasco-Sandoval, Cunha, Vidal, Ribeiro-dos-Santos, Pinto, Magalhães, Assumpção, Demachki, Santos, Assumpção and Ribeiro-dos-Santos. This is an open-access article distributed under the terms of the Creative Commons Attribution License (CC BY). The use, distribution or reproduction in other forums is permitted, provided the original author(s) and the copyright owner(s) are credited and that the original publication in this journal is cited, in accordance with accepted academic practice. No use, distribution or reproduction is permitted which does not comply with these terms.
*Correspondence: Ândrea Ribeiro-dos-Santos, YWtlbHl1ZnBhQGdtYWlsLmNvbQ==
†These authors have contributed equally to this work