- 1Centre for Integrative Ecology, School of Life and Environmental Sciences, Deakin University, Geelong, VIC, Australia
- 2Deakin Genomics Centre, Deakin University, Geelong, VIC, Australia
- 3Centre for Sustainable Tropical Fisheries and Aquaculture, James Cook University, Townsville, QLD, Australia
- 4Southern Ocean Mariculture, Port Fairy, VIC, Australia
- 5Jade Tiger Abalone, Indented Head, VIC, Australia
Introduction
Marine molluscs of the family Haliotidae, commonly referred to as abalone, are a group of benthic reef species targeted by commercial fisheries in 11 countries, forming an important global industry worth approximately US $180 million (Gordon and Cook, 2013). Many abalone fisheries have collapsed in recent decades due to overexploitation, environmental change, and disease, with a number of target species now listed as endangered or considered “species of concern” (Hauck and Sweijd, 1999; Leiva and Castilla, 2002; Gruenthal and Burton, 2005; Kashiwada and Taniguchi, 2007). The world’s largest thriving abalone fisheries persist in southern Australia which is currently home to a thriving and rapidly growing aquaculture industry making up approximately 10% of Australia’s export market. In this region, the abalone species Haliotis rubra (Figure 1A) was targeted in five states extending from Western Australia to southern New South Wales and Tasmania with a net value of US $79 million (Mundy et al., 2014).
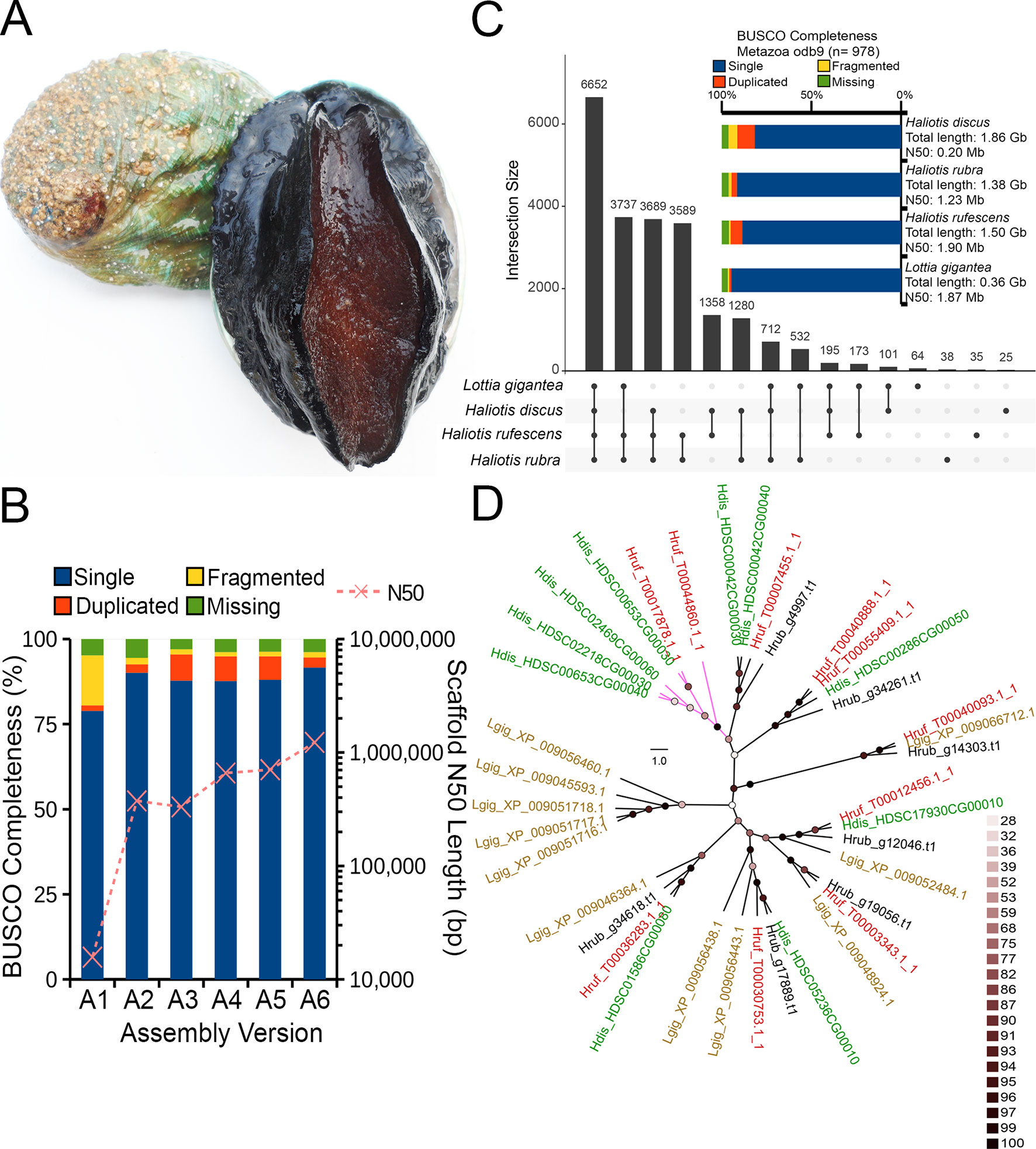
Figure 1 (A) The blacklip abalone (Haliotis rubra). Photo by Mark Gervis. (B) Scaffold N50 length and BUSCO genome assessment after each assembly iteration (see Table 1 for additional details). (C) UpSet plot showing unique and shared protein ortholog clusters across the four marine gastropod genomes. Connected dots represent the intersections of overlapping orthologs with the vertical black bars above showing the number of orthogroups in each intersection. (D) IQTree Maximum likelihood tree depicting the evolutionary relationships among HSP70 proteins. The tree was mid-point rooted, and nodes were colored according to the ultrafast bootstrap support values. Scale bar represents number of substitutions per site. Tip labels were colored according to species name, and clade without H. rubra was colored purple. Four-letter code preceding each protein accession indicates species name. Hdis, H. discus hannai; Hrub, H. rubra; Hruf, H. rufescens; Lgig, L. gigantea.
To keep up with global demand and to counter disease and environmental stress, effective management of wild stock and farmed abalone is vital to ensure maximized productivity, sustainability, and biosecurity of the industry. To date, population and quantitative genetic research have contributed genomic resources to assist H. rubra fisheries management and conservation (Baranski et al., 2008; Miller et al., 2016; Kijas et al., 2019); however, a fully assembled and annotated genome sequence for this species is still unavailable. Such a resource is needed to assist future selective breeding programs, environmental stress and adaptation research, and fundamental genomic and evolutionary studies geared toward bolstering H. rubra fisheries and aquaculture.
Recent years have seen an increased supplementation of genome assemblies with PacBio and/or Nanopore long read sequences which often led to a substantial improvement in the genome contiguity and scaffold/contig reduction (Austin et al., 2017; Daccord et al., 2017; Zimin et al., 2017; Tan et al., 2018). For example, hybrid assembly of the clownfish genome with 11× Nanopore and 54× Illumina reads generated 94% fewer scaffolds and 18-fold longer N50 length compared to the Illumina-only assembly (Tan et al., 2018). To date, two abalone genomes are publicly available and were both assembled using PacBio and Illumina reads (Nam et al., 2017; Masonbrink et al., 2019). The first abalone genome belonging to the species Haliotis discus hannai was reported 2 years ago with a scaffold N50 of 211 kb (Nam et al., 2017). Two years later, the second abalone genome (H. rufescens) was published with a substantially improved contiguity (pre- and post-HiRise2 scaffold N50 of 588 kb and 1.895 Mb, respectively) presumably due the availability of higher PacBio data coverage and the use of a dedicated MaSuRCA hybrid assembler (Zimin et al., 2017; Masonbrink et al., 2019).
It was recently demonstrated that contiguous and accurate de novo assembly of metazoan genomes can be obtained with modest long read coverage (<30× coverage) by merging long-read only assembly with hybrid assembly (Chakraborty et al., 2016). However, this meta-assembly approach has not been extensively tested on heterozygous genome assemblies where a large proportion of the assemblies may fail to be merged due to the presence of independently assembled allelic contigs (haplotig) exhibiting significant structural and sequence variation (Roach et al., 2018; Formenti et al., 2019).
In this study, we generated the first Nanopore long read data (∼ 30× coverage) for the blacklip abalone (Figure 1A) and applied the hybrid meta-assembly approach to assemble its genome. Although the inclusion of long read generally improved the abalone genome contiguity and completeness, several haplotigs remain in the meta-assembly, leading to a larger assembly size and a higher representation of duplicated single-copy genes. We overcome this by performing a haplotig removal step, generating the first high-quality genome assembly for an Australian abalone. To demonstrate the utility of this resource, we annotated the genome and explored the genome for heat-shock proteins (HSPs), a highly conserved protein family whose synthesis has been previously linked to thermal stress in Haliotis species (Farcy et al., 2007; Li et al., 2012; Shiel et al., 2015).
Materials and Methods
Sample Collection and Nucleic Acid Extraction
Sample DU_JTF1, an offspring from brother/sister mating, was collected in 2017 from Jade Tiger Abalone Farm (Indented Head, Victoria, Australia) and stored frozen at −20°. Sample DU_PF1 is a captive bred specimen and was freshly sourced from Southern Ocean Mariculture (Port Fairy, Victoria, Australia) in 2018. Immediately after euthanization, its liver/gonad tissue was dissected and homogenized in DNA/RNA shield (Zymo Research, Irvine, CA).
For DU_JTF1, genomic DNA was extracted from multiple muscle biopsies (50 mg wet weight each) using a modified high salt SDS-lysis approach (Sokolov, 2000). For DU_PF1, the extraction of total RNA and DNA from the DNA/RNA shield preserved tissue used Zymo Quick RNA Miniprep kit and Zymo Quick DNA Miniprep kit (Zymo Research, Irvine, CA), respectively, according to the manufacturer’s instructions.
Illumina Whole-Genome Sequencing
One hundred nanograms of gDNA from DU_JTF1 was sheared to 350 bp with a Q800 sonicator (Qsonica, Newtown, CT) and processed with NEB Ultra Library Preparation Kit (NEB, Ipswich, MA) according to the manufacturer’s instructions. The library was quantified on an Agilent 4200 TapeStation (Agilent, Santa Clara, CA) and sequenced on the Novaseq6000 (Illumina, San Diego, CA) located at the Deakin Genomics Centre using the run configuration of 2×150 bp.
Illumina Transcriptome Sequencing
The integrity of total RNA extracted from DU_PF1 was assessed on an Agilent 4200 TapeStation (Agilent, Santa Clara, CA), after which, approximately 1 µg of the total RNA was processed using the Nugen Universal Plus mRNA-seq library preparation kit (NuGEN, Redwood City, CA). The 200-bp insert size library was sequenced on the same Novaseq6000 instrument as described above.
Nanopore Sequencing
A total of 14 LSK-108 and five LSK-208 (obsolete) Nanopore libraries were prepared for sequencing (See Supplemental Table 1 for details). Sequencing was performed on multiple MinION devices attached to a desktop running Ubuntu 16.04. Subsequent base-calling of the raw fast5 data to fastq files used Albacore v.2.3.3. The DU_JTF1 Nanopore libraries were prepared using 2 µg of Bluepippin size-selected template DNA (> 5kb). On the other hand, the DU_PF1 Nanopore libraries were prepared using 1 µg of column-purified DU_PF1 gDNA without size selection and sequenced on 3 MinION R9.4 flowcells.
Pre-processing of Sequencing Reads and Genome Profiling
Illumina sequencing reads were processed with fastp v0.12.1 to remove low-quality bases (<Q20), as well as adapter and poly-G sequences at the 3′ end (Chen et al., 2018). Adapter-trimming and length filtering (>1,000 bp) of base called Nanopore reads used Porechop v0.2.4 (https://github.com/rrwick/Porechop), respectively. Prior to assembly, the processed Illumina reads were used to calculate kmer frequencies in Jellyfish2 v. 2.2.6 (Marçais and Kingsford, 2011). The kmer histogram for three different kmer length (k21, k25, and k31) were submitted to the Genomescope webserver for estimation of genome size, repeat content, and heterozygosity, based on a kmer-based statistical approach (Vurture et al., 2017).
Genome Assembly
A total of six assemblies were generated from the data set as detailed in Table 1. Assembly A1 represents an Illumina-only assembly performed using Platanus v.1.2.4 (default setting), suited to highly heterozygous genomes (Kajitani et al., 2014). Assembly A2 was constructed from Nanopore long read using wtdbg v1.2.8 (https://github.com/fantasticair/wtdbg-1.2.8) (Ruan and Li, 2019) followed by two rounds of Racon v1.2.0 polishing (Minimap2-aligned Nanopore reads) and one round of Pilon v1.21 polishing (BWA-aligned Illumina reads) (Li, 2013; Walker et al., 2014; Vaser et al., 2017). Assembly A3 represents a DBG2OLC hybrid assembly combining Illumina (Platanus-assembled contigs) and Nanopore data (Ye et al., 2016). The correction of DBG2OLC assembly was performed as similarly described for the assembly A2. Subsequent merging (meta-assembly) of assembly A2 (long-read only assembly) and A3 (hybrid assembly) used QuickMerge v0.2 (Chakraborty et al., 2016) with the setting “-hco 5.0 -c 1.5 -l 330,000 -ml 5,000,” generating assembly A4. RNA-sequencing data generated from DU_PF1 was aligned to assembly A4 with HiSat2 v2.1.0 (Kim et al., 2015) followed by transcriptome-guided genome scaffolding with L_RNA_P (Zhu et al., 2018) to produce assembly A5 (Table 1).
Removal of Redundant Contigs
Illumina reads were aligned to assembly A5 using bwa-mem v0.7.17 (Li, 2013). A read depth histogram (Supplemental Figure 1) was generated from this alignment in PurgeHaplotig v.1.0.2 to obtain the read depth cut off values required to identify haploid (redundant) contigs for subsequent reassignment or purging (Roach et al., 2018). To improve the handling of repetitive regions during haplotig purging, we also included a BED-format file containing the location of repetitive regions that was generated using Red, a machine learning-based de novo repeat detection tool (Girgis, 2015). The PurgeHaplotig-curated primary contigs representing the deduplicated primary haploid assembly was designated as assembly A6 and submitted to NCBI.
Genome Annotation
Transcriptome reads were mapped with STAR v2.7.1 to assembly A6. The transcriptome alignment in BAM format and the soft-masked assembly A6 fasta file were used as the input for Braker v.2.1.2 (Hoff et al., 2015) that fully automate the training of gene prediction tools, GeneMark-ET (Lomsadze et al., 2014) and AUGUSTUS (Stanke et al., 2006). The predicted proteins sequences from Braker2 were functionally annotated using InterProScan v5 (Jones et al., 2014). The number and length of transcripts, exons, and introns were inferred from the genome annotation gff3 file that was similarly generated by Braker2.
Comparative Genomics
The genome sequences and predicted proteins of red abalone (Haliotis rufescens), Pacific abalone (H. discus hannai), and owl limpet (Lottia gigantea) were obtained from their respective data repository for comparison with the newly sequenced blacklip abalone. Each genome assembly was assessed for completeness with BUSCOv3 based on the metazoa odb9 lineage dataset (Waterhouse et al., 2017). Then, orthologous groups of proteins were identified between H. rufescens, H. discus hannai, H. rubra, and L. gigantea using an all-vs-all DIAMOND blastp search and Markov Cluster (MCL) clustering approach as implemented in OrthoFinder v2.2.7 (default setting). The computation and visualization of ortholog intersections across the four marine gastropods used UpSetR (Conway et al., 2017).
Identification of Heat Shock Protein 70 (HSP70)
The InterProScan annotation of H. rubra proteins was filtered for proteins containing the InterPro domain IPR013126 (Heat shock protein 70 family). The putative H. rubra heat shock proteins and their respective orthologs from H. rufescens, H. discus hannai, and L. gigantea identified by OrthoFinder2 were submitted to the GUIDANCE2 web server (Sela et al., 2015) for the detection and removal of unreliable sequences and alignment regions. IQTree v.1.6.5 (Nguyen et al., 2014) was used to construct a maximum likelihood tree based on the GUIDANCE2 alignment output followed by visualization in FigTree v 1.4.3 (https://github.com/rambaut/figtree/).
Preliminary Analysis
Using the k-mer approach based on 291.7 Gb of Illumina short read data, the H. rubra haploid genome size was predicted to be between 1.24 to 1.31 Gb with moderate-high heterozygosity of 1.27 to 1.44% (Supplemental Table 2). Additional processing of the Illumina reads followed by another GenomeScope analysis with the max kmer coverage filter disabled resulted in a predicted haploid genome size of 1.56 Gb, a value closer to the 1.65 Gb haploid size estimated for a different haliotid species based on k-mer distributions (Nam et al., 2017; Masonbrink et al., 2019). Nanopore sequencing generated a total of 28 Gb (0.5-3.1 Gb per flowcell, more than 10 kb median read length) and 25 Gb data (6.8-11 Gb per flowcell, 1-2 kb median read length) for the DU_JTF1 and DU_PF1 libraries, respectively (Supplemental Table 1).
As expected, de novo assembly using only short reads resulted in a highly fragmented assembly contained in 419,307 scaffolds with an N50 of 15,704 bp. On the contrary, Nanopore long-read only assembly followed by polishing with Illumina data generated a significantly more contiguous assembly with an N50 of 374,753 bp with reduced fragmented BUSCO genes (assembly A2 in Figure 1B). A similar N50 length was observed for the DBG2OLC hybrid assembly albeit with a marked increase in the assembled genome length (Table 1) and the number of duplicated single-copy BUSCO genes (assembly A3 in Figure 1B). After the merging of assemblies A2 and A3 using QuickMerge, we observed a 200-kb increase in the N50 length and a slight decrease in assembled genome size (Table 1 and assembly A4 in Figure 1B). Another slight gain of 45.8 kb in N50 length was observed with the transcriptome-guided scaffolding (assembly A5 in Figure 1B).
Consistent with the high genome heterozygosity as estimated by GenomeScope (Supplemental Table 1), a substantial portion of assembly A5 was still represented by redundant scaffolds reflecting distinct haplotypes from the heterozygous genomic region as evidenced by the presence of a secondary peak representing allelic contigs in the read coverage frequency plot (Supplemental Figure 1). After processing with Purge Haplotigs, the final H. rubra haploid assembly was contained in 2,854 scaffolds with an N50 of 1.23 Mb. Compared with assembly A5, this represents a 75.36% decrease and 73.95% increase in the number of scaffolds and N50 length, respectively. The removal of redundant contigs also resulted in a genome assembly with lower duplicated gene content (Figure 1B).
A total of 44,137 protein-coding genes were predicted from the current genome assembly and training model, of which 24,743 were functionally annotated by InterProScan. A total of 22,180 orthologous groups were identified across the four marine gastropod genomes, of which 6,652 were core ortholog clusters, and 3,689 were exclusively shared by the currently sequenced Haliotis species (Figure 1C). Although L. gigantea is more divergent to H. rufescens and H. rubra compared with H. discus hannai, it shared 3,737 private ortholog clusters with H. rubra and H. rufescens. It is plausible that the training model used by Nam et al. (2017) may have under-predicted the protein-coding genes in the H. discus hannai genome since its BUSCO genome completeness is comparable to that of H. rufescens and H. rubra (Figure 1C).
Given the current threats of extreme temperature events, and rising sea surface temperatures in south-eastern Australia (Oliver et al., 2017), the identification of candidate genes associated with thermal stress, and variants influencing their expression, is important for fisheries management and conservation purposes. We identified seven putative HSP70 proteins from H. rubra (Figure 1D) that formed a strongly supported (ultrafast bootstrap support values > 95%) monophyletic clustering with their respective Haliotis spp. orthologs. Sister grouping of H. discus hannai and H. rufescens HSP70 orthologs with H. rubra occupying the basal position was observed in a majority of the haliotid HSP70 clades (Figure 1D).
Future Avenues
The draft genome sequence presented in this study is the first for an Australian abalone species only the third Haliotis genome sequence to be made available in the public data repository. With less than 3,000 scaffolds and more than 90% of the genome contained in scaffolds larger than or equal to 200 kb (Table 1), this draft genome will serve as a suitable base reference for future Hi-C scaffolding and chromosomal-level genome assemblies. The substantial improvement in various assembly metrics after a quick and resource-efficient haplotig-purging step indicates that this can be an important step for generating highly contiguous hybrid assembly from heterozygous marine organisms. The annotated genome is expected to assist in future genomic breeding program by enabling the precise identification of candidate gene variants affecting ecologically and commercially important traits.
Data Availability
Raw Illumina reads are available under the Bioproject PRJNA489521. Base-called Nanopore reads have been deposited in the Zenodo repository (https://doi.org/10.5281/zenodo.2602223). The Whole Genome Shotgun project has been deposited at DDBJ/EMBL/GenBank under accession number QKJH01. Genome annotation, BUSCO calculation, genome assemblies, and Orthofinder2 output have also been deposited in the Zenodo repository (http://doi.org/10.5281/zenodo.3320876).
Author Contributions
HG, AM, and CA conceived the project. HG designed the experiments. AM, CS, LM, and MG collected the specimens. YW performed RNA extraction and transcriptome sequencing. HG performed DNA extraction and whole-genome sequencing. HG, MT, and JS conducted genome analysis and assembly. HG, AM, and CA drafted the manuscript. All authors edited and contributed to the article.
Funding
Funding for this study was provided by the Deakin internal research grant scheme (SEBE-RGS-2018- 0509.31325.31.01) awarded to HG, AM, CS, and CA.
Conflict of Interest Statement
MG was employed by Southern Ocean Mariculture and LM was employed by Jade Tigers Abalone. The remaining authors declare that the research was conducted in the absence of any commercial or financial relationships that could be construed as a potential conflict of interest.
Acknowledgments
This project was funded by the Deakin Research Grant Scheme 2018 awarded to HG and AM. We thank the Deakin Genomics Centre staff for support in sequencing and data processing. We also thank Julian Vreugdenburg for the provision of computational resources for data analysis.
Supplementary Material
The Supplementary Material for this article can be found online at: https://www.frontiersin.org/articles/10.3389/fgene.2019.00889/full#supplementary-material.
Supplemental Figure 1 | Illumina read depth histogram of Assemblies A5 and A6
Supplemental Table 1 | Nanopore sequencing details and data output
Supplemental Table 2 | Alignment-free genome profiling with Illumina reads
References
Austin, C. M., Tan, M. H., Harrisson, K. A., Lee, Y. P., Croft, L. J., Sunnucks, P., et al. (2017). De novo genome assembly and annotation of Australia’s largest freshwater fish, the Murray cod (Maccullochella peelii), from Illumina and Nanopore sequencing read. Gigascience 6, gix063. doi: 10.1093/gigascience/gix063
Baranski, M., Rourke, M., Loughnan, S., Hayes, B., Austin, C., Robinson, N. (2008). Detection of QTL for growth rate in the blacklip abalone (Haliotis rubra Leach) using selective DNA pooling. Animal Genetics. 39 (6), 606-614. doi: 10.1111/j.1365-2052.2008.01773.x
Chakraborty, M., Baldwin-Brown, J. G., Long, A. D., Emerson, J. (2016). Contiguous and accurate de novo assembly of metazoan genomes with modest long read coverage. Nucleic Acids Res. 44, e147–e147. doi: 10.1093/nar/gkw654
Chen, S., Zhou, Y., Chen, Y., Gu, J. (2018). fastp: an ultra-fast all-in-one FASTQ preprocessor. Bioinformatics 34, i884–i890. doi: 10.1093/bioinformatics/bty560
Conway, J. R., Lex, A., Gehlenborg, N. (2017). UpSetR: an R package for the visualization of intersecting sets and their properties. Bioinformatics 33, 2938–2940. doi: 10.1093/bioinformatics/btx364
Daccord, N., Celton, J.-M., Linsmith, G., Becker, C., Choisne, N., Schijlen, E., et al. (2017). High-quality de novo assembly of the apple genome and methylome dynamics of early fruit development. Nat. Genet. 49, 1099. doi: 10.1038/ng.3886
Farcy, E., Serpentini, A., Fievet, B., Lebel, J. M. (2007). Identification of cDNAs encoding HSP70 and HSP90 in the abalone Haliotis tuberculata: transcriptional induction in response to thermal stress in hemocyte primary culture. Comp. Biochem. Physiol. B, Biochem. Mol. Biol. 146, 540–550. doi: 10.1016/j.cbpb.2006.12.006
Formenti, G., Chiara, M., Poveda, L., Francoijs, K. J., Bonisoli-Alquati, A., Canova, L., et al. (2019). SMRT long reads and Direct Label and Stain optical maps allow the generation of a high-quality genome assembly for the European barn swallow (Hirundo rustica rustica). Gigascience 8 (1), 1–8. doi: 10.1093/gigascience/giy142
Girgis, H. Z. (2015). Red: an intelligent, rapid, accurate tool for detecting repeats de-novo on the genomic scale. BMC Bioinformatics 16, 227. doi: 10.1186/s12859-015-0654-5
Gordon, H. R., Cook, P. A. (2013). World Abalone Supply, Markets, and Pricing: 2011 Update. SPIE. J. Shellfish Res. 32 (1), 5–7. doi: 10.2983/35.032.0102
Gruenthal, K., Burton, R. (2005). Genetic diversity and species identification in the endangered white abalone (Haliotis sorenseni). Conserv. Genet. 6, 929–939. doi: 10.1007/s10592-005-9079-4
Hauck, M., Sweijd, N. A. (1999). A case study of abalone poaching in South Africa and its impact on fisheries management. ICES J. Mar. Sci. 56, 1024–1032. doi: 10.1006/jmsc.1999.0534
Hoff, K. J., Lange, S., Lomsadze, A., Borodovsky, M., Stanke, M. (2015). BRAKER1: unsupervised RNA-Seq-based genome annotation with GeneMark-ET and AUGUSTUS. Bioinformatics 32, 767–769. doi: 10.1093/bioinformatics/btv661
Jones, P., Binns, D., Chang, H.-Y., Fraser, M., Li, W., Mcanulla, C., et al. (2014). InterProScan 5: genome-scale protein function classification. Bioinformatics 30, 1236–1240. doi: 10.1093/bioinformatics/btu031
Kajitani, R., Toshimoto, K., Noguchi, H., Toyoda, A., Ogura, Y., Okuno, M., et al. (2014). Efficient de novo assembly of highly heterozygous genomes from whole-genome shotgun short reads. Genome Res. 24 (8), 1384–1395. doi: 10.1101/gr.170720.113
Kashiwada, J. V., Taniguchi, I. K. (2007). Application of recent red abalone Haliotis rufescens surveys to management decisions outlined in the California Abalone Recovery and Management Plan. J. Shellfish Res. 26, 713–717. doi: 10.2983/0730-8000(2007)26[713:AORRAH]2.0.CO;2
Kijas, J., Hamilton, M., Botwright, N., King, H., McPherson, L., Krsinich, A., et al. (2019). Genome sequencing of blacklip and greenlip abalone for development and validation of a SNP based genotyping tool. Front. Genet. 9, 687. doi: 10.3389/fgene.2018.00687
Kim, D., Langmead, B., Salzberg, S. L. (2015). HISAT: a fast spliced aligner with low memory requirements. Nat. Methods 12, 357. doi: 10.1038/nmeth.3317
Leiva, G. E., Castilla, J. C. (2002). A review of the world marine gastropod fishery: evolution of catches, management and the Chilean experience. Rev. Fish Biol. Fish. 11, 283–300. doi: 10.1023/A:1021368216294
Li, H. (2013). Aligning sequence reads, clone sequences and assembly contigs with BWA-MEM. arXiv preprint arXiv:1303.3997, 1–3.
Li, J., He, Q., Sun, H., Liu, X. (2012). Acclimation-dependent expression of heat shock protein 70 in Pacific abalone (Haliotis discus hannai Ino) and its acute response to thermal exposure. Chin. J. Oceano. Limnol. 30, 146–151. doi: 10.1007/s00343-012-1026-x
Lomsadze, A., Burns, P. D., Borodovsky, M. (2014). Integration of mapped RNA-Seq reads into automatic training of eukaryotic gene finding algorithm. Nucleic Acids Res. 42, e119. doi: 10.1093/nar/gku557
Marçais, G., Kingsford, C. (2011). A fast, lock-free approach for efficient parallel counting of occurrences of k-mers. Bioinformatics 27, 764–770. doi: 10.1093/bioinformatics/btr011
Masonbrink, R., Purcell, C., Boles, S., Whitehead, A., Hyde, J., Seetharam, A., et al. (2019). An annotated genome for Haliotis rufescens (Red Abalone) and resequenced green, pink, pinto, black and white abalone species. Genome Biol. Evol. 11, 431–438. doi: 10.1093/gbe/evz006
Miller, A. D., van Rooyen, A., Rašić, G., Ierodiaconou, D. A., Gorfine, H. K., Day, R., et al. (2016).Contrasting patterns of population connectivity between regions in a commercially important mollusc Haliotis rubra: integrating population genetics, genomics and marine LiDAR data. Molecular Ecology. 25 (16), 3845–3864. doi: 10.1111/mec.13734
Mundy, C., Mayfield, S., Liggins, G. H. (2014). “Status of key Australian fish stocks reports 2014,” in Blacklip abalone Haliotis rubra. Eds. Flood, M., Stobutzki, I., Andrews, J. (Canberra: Fishesries Research and Development Corporation).
Nam, B.-H., Kwak, W., Kim, Y.-O., Kim, D.-G., Kong, H. J., Kim, W.-J., et al. (2017). Genome sequence of pacific abalone (Haliotis discus hannai): the first draft genome in family Haliotidae. Gigascience 6, 1–8. doi: 10.1093/gigascience/gix014
Nguyen, L.-T., Schmidt, H. A., Von Haeseler, A., Minh, B. Q. (2014). IQ-TREE: a fast and effective stochastic algorithm for estimating maximum-likelihood phylogenies. Mol. Biol. Evol. 32, 268–274. doi: 10.1093/molbev/msu300
Oliver, E. C. J., Benthuysen, J. A., Bindoff, N. L., Hobday, A. J., Holbrook, N. J., Mundy, C. N., et al. (2017). The unprecedented 2015/16 Tasman Sea marine heatwave. Nat. Commun. 8, 16101. doi: 10.1038/ncomms16101
Roach, M. J., Schmidt, S. A., Borneman, A. R. (2018). Purge Haplotigs: allelic contig reassignment for third-gen diploid genome assemblies. BMC Bioinformatics 19, 460. doi: 10.1186/s12859-018-2485-7
Ruan, J., Li, H. (2019). Fast and accurate long-read assembly with wtdbg2. bioRxiv 530972. doi: 10.1101/530972
Sela, I., Ashkenazy, H., Katoh, K., Pupko, T. (2015). GUIDANCE2: accurate detection of unreliable alignment regions accounting for the uncertainty of multiple parameters. Nucleic Acids Res. 43, W7–W14. doi: 10.1093/nar/gkv318
Shiel, B. P., Hall, N. E., Cooke, I. R., Robinson, N. A., Strugnell, J. M. (2015). De novo characterisation of the greenlip abalone transcriptome (Haliotis laevigata) with a focus on the heat shock protein 70 (HSP70) family. Mar. Biotechnol. (N. Y.) 17, 23–32. doi: 10.1007/s10126-014-9591-y
Sokolov, E. P. (2000). An improved method for DNA isolation from mucopolysaccharide-rich molluscan tissues. J. Molluscan Stud. 66, 573–575. doi: 10.1093/mollus/66.4.573
Stanke, M., Keller, O., Gunduz, I., Hayes, A., Waack, S., Morgenstern, B. (2006). AUGUSTUS: ab initio prediction of alternative transcripts. Nucleic Acids Res. 34, W435–W439. doi: 10.1093/nar/gkl200
Tan, M. H., Austin, C. M., Hammer, M. P., Lee, Y. P., Croft, L. J., Gan, H. M. (2018). Finding Nemo: hybrid assembly with Oxford Nanopore and Illumina reads greatly improves the clownfish (Amphiprion ocellaris) genome assembly. Gigascience 7, gix137. doi: 10.1093/gigascience/gix137
Vaser, R., Sovic, I., Nagarajan, N., Sikic, M. (2017). Fast and accurate de novo genome assembly from long uncorrected reads. Genome Res. 27, 737–746. doi: 10.1101/gr.214270.116
Vurture, G. W., Sedlazeck, F. J., Nattestad, M., Underwood, C. J., Fang, H., Gurtowski, J., et al. (2017). GenomeScope: fast reference-free genome profiling from short reads. Bioinformatics 33, 2202–2204. doi: 10.1093/bioinformatics/btx153
Walker, B. J., Abeel, T., Shea, T., Priest, M., Abouelliel, A., Sakthikumar, S., et al. (2014). Pilon: an integrated tool for comprehensive microbial variant detection and genome assembly improvement. PLoS One 9, e112963. doi: 10.1371/journal.pone.0112963
Waterhouse, R. M., Seppey, M., Simão, F. A., Manni, M., Ioannidis, P., Klioutchnikov, G., et al. (2017). BUSCO Applications from Quality Assessments to Gene Prediction and Phylogenomics. Mol. Biol. Evol. 35, 543–548. doi: 10.1093/molbev/msx319
Ye, C., Hill, C. M., Wu, S., Ruan, J., Ma, Z. S. (2016). DBG2OLC: efficient assembly of large genomes using long erroneous reads of the third generation sequencing technologies. Sci. Rep. 6, 31900. doi: 10.1038/srep31900
Zhu, B.-H., Xiao, J., Xue, W., Xu, G.-C., Sun, M.-Y., Li, J.-T. (2018). P_RNA_scaffolder: a fast and accurate genome scaffolder using paired-end RNA-sequencing reads. BMC Genomics 19, 175. doi: 10.1186/s12864-018-4567-3
Keywords: abalone, Oxford Nanopore, hybrid assembly, heterozygosity, Haliotis, heat shock protein 70
Citation: Gan HM, Tan MH, Austin CM, Sherman CDH, Wong YT, Strugnell J, Gervis M, McPherson L and Miller AD (2019) Best Foot Forward: Nanopore Long Reads, Hybrid Meta-Assembly, and Haplotig Purging Optimizes the First Genome Assembly for the Southern Hemisphere Blacklip Abalone (Haliotis rubra). Front. Genet. 10:889. doi: 10.3389/fgene.2019.00889
Received: 04 April 2019; Accepted: 23 August 2019;
Published: 25 September 2019.
Edited by:
Ross Houston, University of Edinburgh, United KingdomReviewed by:
Filippo Biscarini, Italian National Research Council (CNR), ItalyShikai Liu, Ocean University of China, China
Copyright © 2019 Gan, Tan, Austin, Sherman, Wong, Strugnell, Gervis, McPherson and Miller. This is an open-access article distributed under the terms of the Creative Commons Attribution License (CC BY). The use, distribution or reproduction in other forums is permitted, provided the original author(s) and the copyright owner(s) are credited and that the original publication in this journal is cited, in accordance with accepted academic practice. No use, distribution or reproduction is permitted which does not comply with these terms.
*Correspondence: Han Ming Gan, aGFuLmdhbkBkZWFraW4uZWR1LmF1; Adam D. Miller, YS5taWxsZXJAZGVha2luLmVkdS5hdQ==